DOI:
10.1039/D3RA06045D
(Review Article)
RSC Adv., 2023,
13, 34646-34651
NH-1,2,3-triazoles as versatile building blocks in denitrogenative transformations
Received
5th September 2023
, Accepted 22nd November 2023
First published on 27th November 2023
Abstract
The utilization of NH-1,2,3-triazoles as easily accessible building blocks in denitrogenative ring cleavage transformations with electrophiles to provide multifunctionalized nitrogen heterocycles and N-alkenyl compounds is reviewed. Leveraging the ready availability of NH-1,2,3-triazoles, these processes provide a convenient route to a range of pharmaceutically relevant heterocyclic cores and N-alkenyl compounds. The synthetic usefulness of in situ acylated NH-1,2,3-triazoles as viable alternatives to widely explored N-sulfonyl-1,2,3-triazoles in ring cleavage processes is highlighted.
1,2,3-Triazoles are nitrogen heterocycles with versatile reactivity1 and great medicinal importance.2 Since the discovery of azide–alkyne click chemistry in 2002,3 triazole derivatives have gained enormous attention in organic, medicinal, biomolecular, and material sciences. Among them, 1,2,3-triazoles bearing an electron-withdrawing group at position N1 are of special importance because of their propensity to undergo N1–N2 bond cleavage in denitrogenative triazole ring opening transformations (Scheme 1a).1 N-sulfonyl-1,2,3-triazoles4 and N-fluoroalkyl-1,2,3-triazoles5 are the most explored building blocks, which undergo ring cleavage under metal catalysis or by the action of Lewis or Brønsted acids. Very recently, a new strategy based on the use of NH-1,2,3-triazoles involving the installation of an electron-withdrawing group with in situ ring cleavage was described and used with success (Scheme 1b). The present review features the use of free NH-1,2,3-triazoles 1 in denitrogenative transformations, proceeding via N-acyl-1,2,3-triazoles or their analogues as key intermediates.
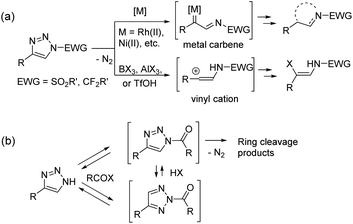 |
| Scheme 1 Schematic representation of the utilization of (a) N–sulfonyl- or N-fluoroalkyl-substituted 1,2,3-triazoles and (b) NH-1,2,3-triazoles in ring cleavage denitrogenative transformations. | |
N-unsubstituted NH-1,2,3-triazoles 1, considered in the present review, are the simplest and most readily available triazoles.6 They can be prepared by azide–alkyne cycloaddition6 or alternative methods such as cycloaddition/elimination with activated ketones7 or nitroalkenes.7 In the last five years, there has been a notable surge of innovative methods for the synthesis of NH-1,2,3-triazoles and several one-pot protocols from inexpensive and commercially available reagents have been developed.6 To underline the most efficient and practical routes, NH-1,2,3-triazoles were synthesized from TMSN3 and alkynes via CuI-catalysed cycloaddition (Scheme 2a),8 or sodium azide, aldehydes and nitroalkanes via a tandem Henry reaction/[3 + 2] cycloaddition (Scheme 2b),9a–c including recently developed green chemistry approaches.9d–h In 2022, NH-1,2,3-triazoles became available from NaN3/H2SO4 and alkynes, which is so far the simplest and the most straightforward route, although the generation of HN3 raises safety concerns (Scheme 2c).10 Finally, the approach utilizing azidyl radical–alkyne cycloaddition with the use of the NaN3/PhI(OAc)2 system in mild conditions is highly efficient for complex disubstituted triazoles such as 4,5-diaryltriazoles (Scheme 2d),11a and it was also possible to efficiently synthesize these compounds without an oxidant, albeit only under harsh conditions (MW heating at 200 °C).11b More examples of novel synthetic methods to access NH-1,2,3-triazoles appeared in recent reviews.6
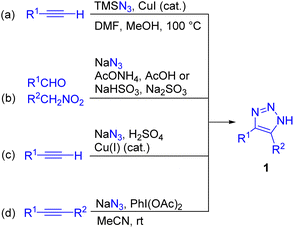 |
| Scheme 2 Overview of efficient routes for the synthesis of NH-1,2,3-triazoles (a–d). | |
Due to better atom economy, the utilization of readily available NH-triazoles is advantageous compared to the use of N-sulfonyl- or N-fluoroalkyl-triazoles. Additionally, access to NH-1,2,3-triazoles was possible using “alkyne-free” methods. Both, primary nitro compounds and aromatic aldehydes are easily accessible industrial scale products.12
One of the first denitrogenative transformations of 1,2,3-NH-triazoles 1 was reported in 2014. In this process, in situ sulfonylation with triflic anhydride and 2,6-di(tert-butyl)-4-methylpyridine (DTBMP) as a base was used to generate reactive N-triflyl triazoles 2.13 Their ring cleavage by a chiral Rh(II) catalyst in the presence of an excess of alkene 3 afforded 2,3-dihydropyrroles 4 with low to good enantiocontrol (Scheme 3).
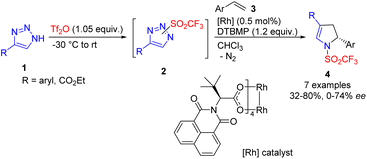 |
| Scheme 3 Synthesis of 2,3-dihydropyrroles by cleavage of NH-triazole in the presence of triflic anhydride. DTBMP – 2,6-di(tert-butyl)-4-methylpyridine. | |
However, besides triflation, there are no other examples of in situ sulfonylation of NH-1,2,3-triazoles followed by denitrogenative transformations. Therefore, this reaction is limited to the extremely electron-accepting triflyl group and analogous ring cleavage did not proceed with other N-sulfonyl triazoles.
In contrast to sulfonylation, acylation of NH-1,2,3-triazoles is more versatile and has developed into a highly active area of research in recent years.14 Tandem acylation followed by ring cleavage without isolation of N-acyltriazoles was performed using acyl halides or acid anhydrides. The mechanism of this transformation, recently confirmed by us,14 involved the formation of N1 (5) and N2-acylated (6) triazoles in equilibrium, followed by acid-mediated cleavage of the former. Denitrogenation and formation of a vinyl cation in an irreversible step was the driving force of N2–N1-acyltriazole interconversion, which ensured the complete transformation of triazoles into ring cleavage products 7–9 (Scheme 4).
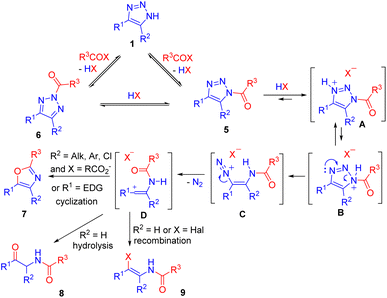 |
| Scheme 4 Mechanism of NH-triazole cleavage with electrophiles. | |
Cleavage of NH-1,2,3-triazoles 1 with an excess of acyl halides 10 (X = Cl, Br) under elevated temperature led to the formation of β-enamido halides 9 in moderate to good yields (Scheme 5).15 β-Enamido halides are difficult to access by other synthetic routes and are present in natural products, which underlines the synthetic value of the method.
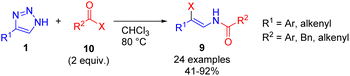 |
| Scheme 5 Cleavage of NH-1,2,3-triazoles with acyl halides. | |
This transformation in the presence of sodium sulfonates was employed in the synthesis of enamido triflates or sulfonates 11. Mainly compounds with the phenacyl group at the nitrogen were accessed by the mentioned route (Scheme 6).15
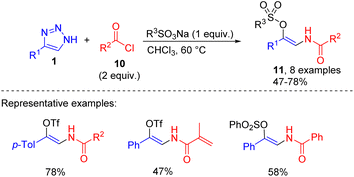 |
| Scheme 6 Synthesis of β-enamido triflates and sulfonates from NH-1,2,3-triazoles, acyl halides, and sodium sulfonates. | |
An alternative method, applicable to the synthesis of β-fluoroacylenamido triflates is based on the formation of N2-acyltriazoles 6 via the in situ acylation of NH-1,2,3-triazoles with fluorinated acid anhydrides followed by their treatment with triflic acid, which proceeds through N2–N1 acyltriazole interconversion and ring cleavage (Scheme 7).14
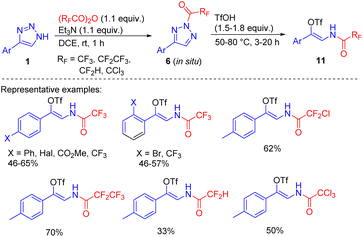 |
| Scheme 7 One-pot two step synthesis of β-fluoroacylenamido triflates. DCE = 1,2-dichloroethane. | |
The products are useful building blocks, that can get involved in Pd-catalysed cross-coupling substitution reactions of the triflate group to access multifunctionalized enamide derivatives – attractive drug candidates and synthetic intermediates.16 None of the methods mentioned was applicable with the less reactive alkyl-substituted acylating agents (Ac2O, AcCl), because the resulting acyltriazoles were resistant to ring cleavage even at elevated temperatures.14
The cleavage of NH-1,2,3-triazoles with an excess of fluoroalkylated acid anhydrides led to highly pharmaceutically relevant 2-fluoroalkyl oxazoles 7 (in the cases of 4,5-disubstituted triazoles) or 2-acylaminoketones (for 4-substituted triazoles, R = H) (Scheme 8).17 In the first case intramolecular cyclization took place, whereas in the second, unstable β-acyloxyenamide 8′ formed, which underwent ester hydrolysis to 2-acylaminoketone 8 upon treatment with an aqueous base. The difference in chemoselectivity was attributed to the increased vinyl cation stability of disubstituted examples, which made them more prone to intramolecular cyclization.
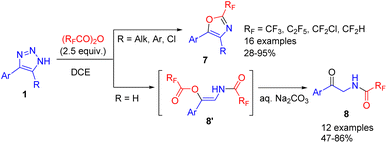 |
| Scheme 8 Synthesis of fluoroalkylated oxazoles and 2-acylaminoketones from NH-1,2,3-triazoles with fluoroalkylated acid anhydrides. | |
4,5-disubstituted NH-1,2,3-triazole reacted with trichloroacetic anhydride to give 2-unsubstituted oxazole 13, due to the low stability of the trichloromethyl-substituted product 12 during silica gel column chromatography. The whole transformation is a rare and unique case of a reaction involving trichloroacetic anhydride as a one-carbon building block (Scheme 9).17
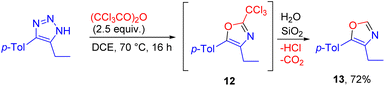 |
| Scheme 9 Formation of 2-unsubstituted oxazole from NH-1,2,3-triazole and trichloroacetic anhydride. | |
The limitation of oxazole synthesis to only disubstituted triazoles was overcome by the cyclization of in situ formed β-acyloxyenamide 8′ to oxazoles 7 using Et3N and proceeded quickly and nearly quantitatively under ambient conditions.18 This one-pot triazole cleavage procedure provided an efficient access to 2-fluoroalkylated oxazoles from monosubstituted triazoles in good to excellent yields (Scheme 10).
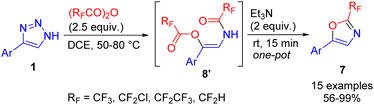 |
| Scheme 10 One-pot synthesis of fluoroalkylated oxazoles from NH-1,2,3-triazoles, acid anhydrides and Et3N. | |
The easy access to fluorinated 2-acylaminoketones 8 was utilized in a number of one-pot syntheses of fluoroalkylated heterocycles directly from NH-1,2,3-triazoles 1. First, 2-fluoroalkyl imidazoles 14 were prepared by cleavage with trifluoroacetic or perfluoropropanoic anhydrides, followed by the treatment of the ketamide intermediate with an aqueous solution of the primary amine (or ammonium acetate for R = H) under microwave conditions. The acid formed after hydrolysis of the enamide to yield 2-acylaminoketone promoted the Robinson–Gabriel cyclization of the latter. This procedure afforded imidazoles 14 in moderate to good yields in a one-pot manner starting from triazoles (Scheme 11).17
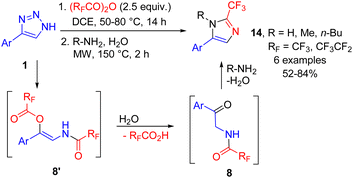 |
| Scheme 11 One-pot synthesis of 2-fluoroalkyl-imidazoles from NH-1,2,3-triazoles. | |
Alternatively, condensation of the formed acyloxyenamide 8′ with hydrazine hydrate after switching the solvent to acetic acid provided fluoroalkylated 1,2,4-triazines 15 (Scheme 12).17
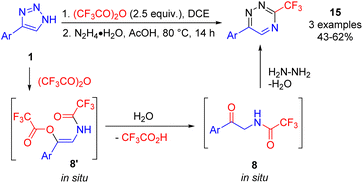 |
| Scheme 12 Synthesis of 3-fluoroalkyl-1,2,4-triazines from NH-1,2,3-triazoles. | |
The formation of the vinyl cation intermediate in N-acyltriazole cleavage was confirmed by changing the solvent from a chlorinated one to acetonitrile or propionitrile.18 In one special case (Scheme 13, R = p-Tol) adducts 16 of the Ritter reaction were formed and hydrolysed to bis(enamides) 17. However, this reaction was not general and in the cases of electron-richer triazoles, cyclization of the vinyl cation to oxazoles 7 took place (Scheme 13, R = H, EDG). This route is an alternative to one mentioned above (Scheme 10), and is applicable to electron-rich substrates. The straightforward formation of oxazoles 7 rather than enamides 8′ in polar MeCN was explained by the decreased stability of the vinyl cation–trifluoroacetate anion contact ion pair, which prevented recombination and favoured cyclization.18
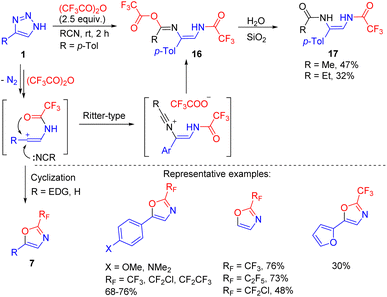 |
| Scheme 13 Formation of bis(enamides) 17 and oxazoles 7 by the cleavage of NH-1,2,3-triazoles with trifluoroacetic anhydride in nitrile solvent. | |
Several efficient NH-1,2,3-triazole ring cleavage protocols were also developed for NH-benzotriazole 18, which can be easily and regioselectively acylated on N1. The treatment of the formed N-acylbenzotriazole 20 with AlCl3 as a Lewis acid promotor facilitated ring cleavage leading to benzoxazoles under relatively harsh conditions (Scheme 14).19
 |
| Scheme 14 Synthesis of benzoxazoles from NH-benzotriazoles. | |
Rare examples of ortho-iodoacetanilide 22 formation in moderate yields from NH- and related N-acylbenzotriazole were reported in which the All3/Ac2O system or aluminium and iodine in acetonitrile were used.20 These are the only cases of N-acetylbenzotriazole 23 ring cleavage known. Importantly, the reaction of N-acetylbenzotriazole 23 with AlCl3 was not efficient and led only to deacylation, and not to the desired ring cleavage product (Scheme 15).19
 |
| Scheme 15 Formation of o-iodoacetanilide by AlI3-mediated cleavage of N1-acetylbenzotriazole. | |
Cleavage of electron-rich 4-aryl-NH-1,2,3-triazoles 1 was successfully achieved with thiophosgene leading to the formation of vinyl isothiocyanates 24 by HCl elimination from the vinyl chloride intermediate (Scheme 16).21 The vinyl isothiocyanate moiety is present in natural products with antifungal and antibacterial activity and is difficult to access by traditional methods. Switching from electron-rich aromatic NH-triazoles to unsubstituted NH-1,2,3-triazole afforded product 25 of HCl addition across the double bond in moderate yield.
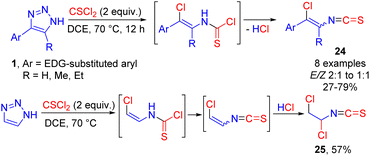 |
| Scheme 16 Cleavage of NH-1,2,3-triazoles with thiophosgene. | |
A similar transformation with triazoles 1 bearing an electron-rich aryl or alkenyl substituent in position 4 proceeded with triphosgene.21 The in situ formed carbamoyl chlorides 26 were treated with nucleophiles to gain access to multifunctional compounds 27, such as N-alkenyl carbamates, ureas and thiocarbamates (Scheme 17).
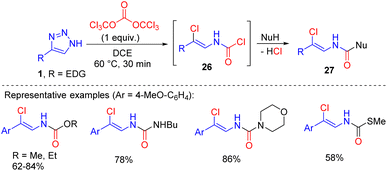 |
| Scheme 17 Synthesis of multifunctional N-alkenyl compounds by the cleavage of NH-1,2,3-triazoles with triphosgene. | |
The denitrogenative transformation of NH-1,2,3-triazoles was studied also on more complex substrates such as 4-(1-hydroxycyclobutyl)-1,2,3-triazoles 28. Their cleavage with acyl chlorides 10 catalysed by triflic acid provided efficient access to cyclic enaminones 29 (Scheme 18).22 The reaction proceeded via the cleavage of N-acyltriazole and semipinacol rearrangement cascade. The procedure was found to be easily scalable to give multifunctional substrates in good yields.
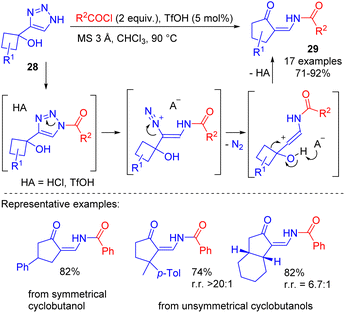 |
| Scheme 18 Synthesis of cyclic enaminones by TfOH-catalyzed cleavage of 4-(1-hydroxycyclobutyl)-1,2,3-triazoles with acyl chlorides. | |
Conclusions
In conclusion, NH-1,2,3-triazoles are commercially available or easily synthesized starting materials that exhibit a remarkable versatility in transformations to diverse nitrogen-containing heterocycles and functionalized N-alkenyl compounds via denitrogenative cleavage. In situ prepared N-acylated 1,2,3-triazoles are key intermediates in these transformations. Acid-mediated triazole ring opening of N-acylated 1,2,3-triazoles, followed by nitrogen elimination affords vinyl cation intermediates, which undergo a variety of reactions such as cyclization or heteroatom capture. Further development of denitrogenation of NH-1,2,3-triazoles accompanied by C–C bond forming reactions, C–H insertion or rearrangement of the vinyl cation can be expected, providing access to a structural diversity of products with potential applications in drug development. Moreover, due to easy availability of NH-1,2,3-triazoles they are excellent starting materials for the development of new industrial synthetic processes.
Conflicts of interest
There are no conflicts to declare.
Acknowledgements
The work was financially supported by the Czech Academy of Sciences (Research Plan RVO: 61388963).
Notes and references
-
(a) Chemistry of 1,2,3-triazoles, in Topics in Heterocyclic Chemistry, ed. W. Dehaen and V. A. Bakulev, Springer, 2015, vol. 40 Search PubMed;
(b) H. M. Davies and J. S. Alford, Reactions of metallocarbenes derived from N-sulfonyl-1,2,3-triazoles, Chem. Soc. Rev., 2014, 43, 5151 RSC;
(c) W. Li and J. Zhang, Synthesis of Heterocycles through Denitrogenative Cyclization of Triazoles and Benzotriazoles, Chem. – Eur. J., 2020, 26, 11931 CrossRef CAS PubMed.
- S. Kumar, B. Sharma, V. Mehra and V. Kumar, Recent accomplishments on the synthetic/biological facets of pharmacologically active 1H-1,2,3-triazoles, Eur. J. Med. Chem., 2021, 212, 113069 CrossRef CAS PubMed.
-
(a) C. W. Tornøe, C. Christensen and M. Meldal, Peptidotriazoles on Solid Phase: [1,2,3]-Triazoles by Regiospecific Copper(I)-Catalyzed 1,3-Dipolar Cycloadditions of Terminal Alkynes to Azides, J. Org. Chem., 2002, 67, 3057 CrossRef PubMed;
(b) V. V. Rostovtsev, L. G. Green, V. V. Fokin and K. B. Sharpless, A Stepwise Huisgen Cycloaddition Process: Copper(I)-Catalyzed Regioselective “Ligation” of Azides and Terminal Alkynes, Angew. Chem., Int. Ed., 2002, 41, 2596 CrossRef CAS.
-
(a) M. Akter, K. Rupa and P. Anbarasan, 1,2,3-Triazole and Its Analogues: New Surrogates for Diazo Compounds, Chem. Rev., 2022, 122, 13108 CrossRef CAS PubMed;
(b) B. Chattopadhyay and V. Gevorgyan, Transition-Metal-Catalyzed Denitrogenative Transannulation: Converting Triazoles into Other Heterocyclic Systems, Angew. Chem., Int. Ed., 2012, 51, 862 CrossRef CAS PubMed;
(c) P. Anbarasan, D. Yadagiri and S. Rajasekar, Recent Advances in Transition-Metal-Catalyzed Denitrogenative Transformations- of 1,2,3-Triazoles and Related Compounds, Synthesis, 2014, 46, 3004 CrossRef CAS;
(d) Z.-F. Xu, H. C. Dai, L. H. Shan and C.-Y. Li, Metal-Free Synthesis of (E)-Monofluoroenamine from 1-Sulfonyl-1,2,3-triazole and Et2O·BF3 via Stereospecific Fluorination of α-Diazoimine, Org. Lett., 2018, 20, 1054 CrossRef CAS PubMed;
(e) D. Yang, L. Shan, Z.-F. Xu and C.-Y. Li, Metal-free synthesis of imidazole by BF3·Et2O promoted denitrogenative transannulation of N-sulfonyl-1,2,3-triazole, Org. Biomol. Chem., 2018, 16, 1461 RSC.
-
(a) V. Motornov, A. Markos and P. Beier, A rhodium-catalyzed transannulation of N-(per)fluoroalkyl-1,2,3-triazoles under microwave conditions – a general route to N-(per)fluoroalkyl-substituted five-membered heterocycles, Chem. Commun., 2018, 54, 3258 RSC;
(b) V. Motornov and P. Beier, Chemoselective Aza-[4+3]-annulation of N-Perfluoroalkyl-1,2,3-triazoles with 1,3-Dienes: Access to N-Perfluoroalkyl-Substituted Azepines, J. Org. Chem., 2018, 83, 15195 CrossRef CAS PubMed;
(c) V. Motornov, V. Košťál, A. Markos, D. Taffner and P. Beier, General approach to 2-fluoroalkyl 1,3-azoles via the tandem ring opening and defluorinative annulation of N-fluoroalkyl-1,2,3-triazoles, Org. Chem. Front., 2019, 6, 3776 RSC;
(d) O. Bakhanovich, V. Khutorianskyi, V. Motornov and P. Beier, Synthesis of N-perfluoroalkyl-3,4-disubstituted pyrroles by rhodium-catalyzed transannulation of N-fluoroalkyl-1,2,3-triazoles with terminal alkynes, Beilstein J. Org. Chem., 2021, 17, 504 CrossRef CAS PubMed;
(e) A. Markos, S. Voltrová, V. Motornov, D. Tichý, B. Klepetářová and P. Beier, Stereoselective Synthesis of (Z)-β-Enamido Triflates and Fluorosulfonates from N-Fluoroalkylated Triazoles, Chem. – Eur. J., 2019, 25, 7640 CrossRef CAS PubMed;
(f) A. Markos, L. Janecký, T. Chvojka, T. Martinek, H. Martinez-Seara, B. Klepetářová and P. Beier, Haloalkenyl Imidoyl Halides as Multifacial Substrates in the Stereoselective Synthesis of N-Alkenyl Compounds, Adv. Synth. Catal., 2021, 363, 3258 CrossRef CAS.
-
(a) T. Opsomer and W. Dehaen, Metal-free syntheses of N-functionalized and NH-1,2,3-triazoles: an update on recent developments, Chem. Commun., 2021, 57, 1568 RSC;
(b) C. P. Parambil, S. P. Veethil and W. Dehaen, The Synthesis of Five-Membered N-Heterocycles by Cycloaddition of Nitroalkenes with (In)Organic Azides and Other 1,3-Dipoles, Synthesis, 2022, 54, 910 CrossRef.
- Use of activated ketones:
(a) R. Prakash, T. Opsomer and W. Dehaen, Triazolization of Enolizable Ketones with Primary Amines: A General Strategy toward Multifunctional 1,2,3-Triazoles, Chem. Rec., 2021, 21, 376 CrossRef CAS PubMed;
(b) J. John, J. Thomas and W. Dehaen, Organocatalytic routes toward substituted 1,2,3-triazoles, Chem. Commun., 2015, 51, 10797 RSC , Use of nitroalkenes.;
(c) V. A. Motornov, S. L. Ioffe and A. A. Tabolin, [3+ 2]-Annulation reactions with nitroalkenes in the synthesis of aromatic five-membered nitrogen heterocycles, Targets Heterocycl. Syst., 2019, 23, 237 CAS.
- T. Jin, S. Kamijo and Y. Yamamoto, Copper-Catalyzed Synthesis of N-Unsubstituted 1,2,3-Triazoles from Nonactivated Terminal Alkynes, Eur. J. Org Chem., 2004, 3789 CrossRef CAS.
-
(a) R. Hui, M. Zhao, M. Chen, Z. Ren and Z. Guan, One-Pot Synthesis of 4-Aryl-NH-1,2,3-Triazoles through Three-Component Reaction of Aldehydes, Nitroalkanes and NaN3, Chin. J. Chem., 2017, 35, 1808 CrossRef CAS;
(b) L. Wu, X. Wang, Y. Chen, Q. Huang, Q. Lin and M. Wu, 4-Aryl-NH-1,2,3-Triazoles via Multicomponent Reaction of Aldehydes, Nitroalkanes, and Sodium Azide, Synlett, 2016, 27, 437 CAS;
(c) Q. Hu, Y. Liu, X. Deng, Y. Li and Y. Chen, Aluminium(III) Chloride-Catalyzed Three-Component Condensation of Aromatic Aldehydes, Nitroalkanes and Sodium Azide for the Synthesis of 4-Aryl-NH-1,2,3-triazoles, Adv. Synth. Catal., 2016, 358, 1689 CrossRef CAS . For “green” protocols to NH-1,2,3-triazoles, see;
(d) A. Garg, R. Hazarika, N. Dutta, B. Dutta and D. Sarma, Bio-waste Derived Catalytic Approach Towards NH-1,2,3-Triazole Synthesis, ChemistrySelect, 2021, 6, 7266 CrossRef CAS;
(e) A. Garg, D. Sarma and A. A. Ali, Curr. Res. Green Sustainable Chem., 2020, 3, 100013 CrossRef;
(f) J. Sultana, A. Garg, A. Kulshrestha, S. S. Rohman, B. Dutta, K. Singh, A. Kumar, A. K. Guha and D. Sharma, Catal. Lett., 2023, 153, 3516 CrossRef CAS;
(g) P. Phukan, R. Chetia, R. Boruah, S. Konwer and D. Sharma, Adv. Mater., 2021, 2, 6996 RSC;
(h) S. Bagheri, M. J. Nejad, F. Pazoki, M. K. Miraki and A. Heydari, ChemistrySelect, 2019, 4, 11930 CrossRef CAS.
- D. Jankovic, M. Virant and M. Gazvoda, Copper-Catalyzed Azide–Alkyne Cycloaddition of Hydrazoic Acid Formed In Situ from Sodium Azide Affords 4-Monosubstituted-1,2,3-Triazoles, J. Org. Chem., 2022, 87, 4018 CrossRef CAS PubMed.
-
(a) L. Hu, C. Muck-Lichtenfield, T. Wang, G. He, M. Gao and J. Zhao, Reaction between Azidyl Radicals and Alkynes: A Straightforward Approach to NH-1,2,3-Triazoles, Chem. – Eur. J., 2016, 22, 911 CrossRef CAS PubMed;
(b) S. Roshandel, S. C. Suri, J. C. Marcischak, G. Rasul and G. K. S. Prakash, Catalyst and solvent free microwave-assisted synthesis of substituted 1,2,3-triazoles, Green Chem., 2018, 20, 3700 RSC.
- Benzaldehydes are by the order of magnitude less expensive than corresponding aryl acetylenes according to price lists of common chemical suppliers. Also, nitroalkanes are very inexpensive large scale industrial products. See: N. Ono, The Nitro Group in Organic Synthesis, John Wiley & Sons, New York, 2002 Search PubMed.
- S. W. Kwok, L. Zhang, N. P. Grimster and V. V. Fokin, Catalytic Asymmetric Transannulation of NH-1,2,3-Triazoles with Olefins, Angew. Chem., Int. Ed., 2014, 53, 3452 CrossRef CAS PubMed.
- V. Motornov, R. Pohl, B. Klepetářová and P. Beier, N-Acyl-1,2,3-triazoles – key intermediates in denitrogenative transformations, Chem. Commun., 2023, 59, 9364 RSC.
- T. Wang, Z. Tang, H. Luo, Y. Tian, M. Xu, Q. Lu and B. Li, Access to (Z)-β-Substituted Enamides from N1-H-1,2,3-Triazoles, Org. Lett., 2021, 23, 6293 CrossRef CAS PubMed.
-
(a) Q. Lu, T. Wang, Q. Wu, L. Cheng, H. Luo, L. Liu, G. Chu, L. Wang and B. Li, C–H heteroarylation of aromatics via catalyst free SN2′ coupling cycloaromatization, Green Chem., 2022, 24, 4399 RSC;
(b) T. Chvojka, A. Markos, S. Voltrová, R. Pohl and P. Beier, Ligand-dependent stereoselective Suzuki–Miyaura cross-coupling reactions of β-enamido triflates, Beilstein J. Org. Chem., 2021, 17, 2657 CrossRef CAS PubMed.
- V. Motornov and P. Beier, Access to Fluoroalkylated Azoles and 2-Acylaminoketones via Fluorinated Anhydride-Mediated Cleavage of NH-1,2,3-Triazoles, Org. Lett., 2022, 24, 1958 CrossRef CAS PubMed.
- V. Motornov and P. Beier, One-pot synthesis of 4-substituted 2-fluoroalkyloxazoles from NH-1,2,3-triazoles and fluoroalkylated acid anhydrides, New J. Chem., 2022, 46, 14318 RSC.
- A. S. Singh, N. Mishra, D. Kumar and V. K. Tiwari, Lewis-Acid-Mediated Benzotriazole Ring Cleavage (BtRC) Strategy for the Synthesis of 2-Aryl Benzoxazoles from N-Acylbenzotriazoles, ACS Omega, 2017, 2, 5044 CrossRef CAS PubMed.
- B. Dong, Y. Liu, P. Yang, D. Sang, J. Tian, L. Li and S. Long, Denitrogenative cleavage of benzotriazoles and benzotriazinones, and selective N-desulfonylation of benzotriazoles by aluminum halides, Tetrahedron Lett., 2022, 103, 153965 CrossRef CAS.
- V. Motornov and P. Beier, Synthesis of N-vinyl isothiocyanates and carbamates by the cleavage of NH-1,2,3-triazoles with one-carbon electrophiles, Org. Biomol. Chem., 2023, 21, 1147 RSC.
- M. Xu, L. Liu, T. Wang, H. Luo, M. Hou, L. Du, X. Xin, Q. Lu and B. Li, Acid-catalyzed ring-expansion of 4-(1-hydroxycyclobutyl)-1,2,3-triazoles, Org. Chem. Front., 2022, 9, 1065 RSC.
|
This journal is © The Royal Society of Chemistry 2023 |
Click here to see how this site uses Cookies. View our privacy policy here.