DOI:
10.1039/D2TB00614F
(Review Article)
J. Mater. Chem. B, 2022,
10, 7397-7417
Latest on biomaterial-based therapies for topical treatment of psoriasis
Received
21st March 2022
, Accepted 25th May 2022
First published on 9th June 2022
Abstract
Psoriasis is an autoimmune inflammatory disease which is fundamentally different from dermatitis. Its treatments include topical medications and systemic drugs depending on different stages of the disease. However, these commonly used therapies are falling far short of clinical needs due to various drawbacks. More precise therapeutic strategies with minimized side effects and improved compliance are highly demanded. Recently, the rapid development of biomaterial-based therapies has made it possible and promising to attain topical psoriasis treatment. In this review, we briefly describe the significance and challenges of the topical treatment of psoriasis and emphatically overview the latest progress in novel biomaterial-based topical therapies for psoriasis including microneedles, nanoparticles, nanofibers, and hydrogels. Current clinical trials related to each biomaterial are also summarized and discussed.
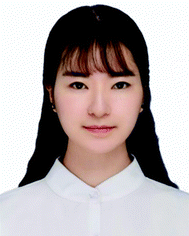
Anqi Chen
| Anqi Chen obtained her doctor's degree in Biological Therapy and Reproductive Medicine from Wenzhou Medical University in 2021. She is now working as a physician in the First Affiliated Hospital of Wenzhou Medical University. Her research interests focus on exploring mechanisms of inflammatory skin disorders. |
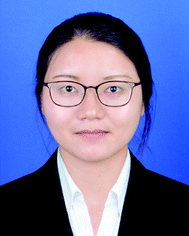
Yuting Luo
| Yuting Luo received her PhD degree from Nanjing University of Science & Technology in 2019. She is currently a lecturer at the School of Pharmaceutical Sciences, Wenzhou Medical University. Her research interests focus on the design, synthesis, and engineering of functional nanomaterials and their biomedical applications. |
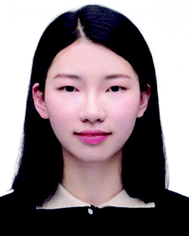
Jie Xu
| Jie Xu received her bachelor's degree in pharmacy from Wenzhou Medical University in 2020. She is now a master student in Wenzhou Medical University under the supervision of Professor Jiang Wu. Her research interests mainly focus the development of advanced biomaterials for wound healing. |
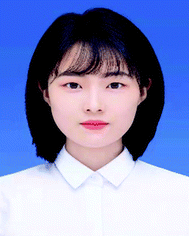
Xueran Guan
| Xueran Guan received her master's degree in pharmacy from Wenzhou Medical University in 2022. She is now pursuing her PhD degree in Wenzhou Medical University under the guidance of Professor Jiang Wu. Her research interests focus on fibroblast growth factor and psoriasis. |
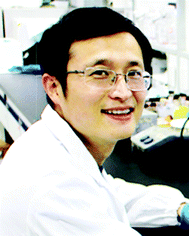
Huacheng He
| Huacheng He is now an associate professor in Wenzhou University. He received his bachelor degree from Zhejiang University in 2009 in China and PhD degree from University of South Carolina in USA. His research interests focus on the development of biomaterials for wound therapy and diagnosis. |
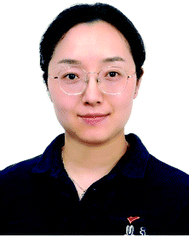
Xuan Xuan
| Xuan Xuan received her master's degree in Clinical Medicine from Wenzhou Medical University in 2010. She obtained her doctor's degree in Biological Therapy and Reproductive Medicine from Wenzhou Medical University in 2019. She is now a physician of dermatology in the First Affiliated Hospital of Wenzhou Medical University. Her research interests focus on the advanced therapies of skin-related diseases. |
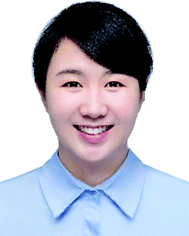
Jiang Wu
| Jiang Wu is a full Professor in the School of Pharmaceutical Sciences at Wenzhou Medical University. She received her bachelor and PhD degrees from Zhejiang University in 2009 and 2014, respectively. After graduation, she joined Wenzhou Medical University and worked in Professor Xiaokun Li's group from then on. Her research interests mainly focus on the design and development of advanced biomaterials for growth factor delivery, wound healing, and regenerative medicine. |
1. Introduction
As one of the frequently encountered chronic inflammatory skin diseases, psoriasis affects 2–3% of the global population.1–3 It adversely burdens one's quality of life with disfiguring lesions, itching, and even serious mental disorders such as helplessness, embarrassment and anger.4 However, there is still a lack of effective approaches for psoriasis therapy. Great efforts have been made by researchers in the way to understand the disease. It has been intensively demonstrated that psoriasis is an immune-driven inflammatory skin disease, which involves Th17 secreting IL-17A, IL-17F and IL-22 to trigger epidermal hyperproliferation. Upon stimulation, keratinocytes do not only expand rapidly with aberrant differentiation, but also secrete inflammatory cytokines (e.g., IL-1β, IL-6, and TNF-α) and chemokines (e.g., CXCL8, CXCL9, and CXCL10) to induce neutrophil infiltration and further Th17 cell recruitment into the epidermis, thus self-amplifying skin inflammation.5,6 This makes psoriasis fundamentally different from dermatitis. Dermatitis is also a frequently encountered chronic inflammatory disease whose initiation is ascribed to the activation of the Th2 immune response.7
According to the clinical features, psoriasis is mainly divided into four variants, namely, plaque psoriasis, erythrodermic psoriasis, guttate psoriasis, and pustular psoriasis.8 Among the variants, plaque psoriasis, also known as psoriasis vulgaris, is the most common psoriatic form (accounting for 90%).9 It develops limited and localized skin lesions, characterized by clearly demarcated plaques with erythema and scaling. These directly result from the aberrant hyperplasia of the epidermis.9,10 Epidermal acanthosis, hyperkeratosis, parakeratosis and elongated epidermal rete ridges are representative hallmarks of psoriatic histopathology.9 Additionally, in the dermis, obvious infiltration of immune cells could be observed, including neutrophils, macrophages, and T cells. Furthermore, blood vessels in the psoriatic dermis are abnormally dilated and contorted (Fig. 1(A)).
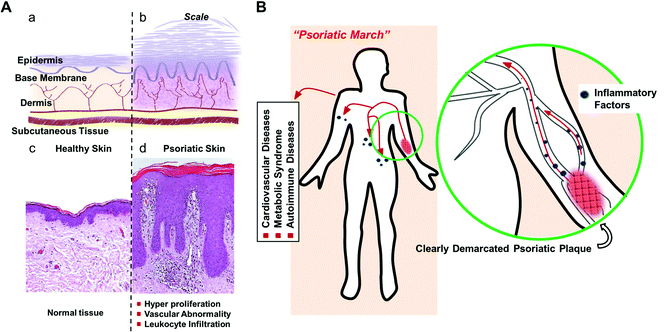 |
| Fig. 1 (A) The pathological histological structure of skin developed with psoriatic inflammation. Illustration of the skin structure of non-psoriatic (a) and psoriatic lesions (b). Histopathological images of healthy skin (c) and the typical psoriatic plaque (d). Reproduced with permission.9 Copyright (2015) Elsevier. (B) The schematic sketch of “psoriatic march” indicating the clinical need for early effective control over topical psoriasis progression. | |
The conventional and commonly used medications for psoriasis therapy include palliative agents, topical corticosteroids, vitamin D derivatives, calcineurin inhibitors, folic acid antagonists and targeting biologics.11–17 Phototherapy is also another widely accepted strategy for psoriasis treatment.18 According to the psoriasis status (mild, moderate, and severe), specific medications or treatment methods or combination therapies are selected. Despite the varying degrees of the therapeutic effect from those conventional methods, the rather intricate network and crosslink beneath psoriasis makes it far from meeting the demands of both improved symptoms and minimized side effects. There are several excellent literature studies that have already extensively discussed the current treatments for psoriasis in terms of therapeutic efficacy and limitations.8,19 The readers are encouraged to refer to these papers. With the deepening of the research on psoriasis, various therapies have also been developed. Particularly, the application of biomaterials to locally deliver conventional medications for psoriasis therapy has gained increasing attention as the biomaterial-based therapies can achieve an enhanced therapeutic effect along with limited adverse outcomes. Therefore, in this review, we mainly emphasize on the biomaterial-based therapies for the topical treatment of psoriasis. The significance and challenges of topical treatment of psoriasis are firstly introduced, and then the different biomaterials for psoriasis therapy are comprehensively reviewed. Finally, current clinical trials related to the biomaterials are discussed.
2. Methodology
This study was conducted using the Web of Science database (WOS). Specifically, to summarize the number of publications that related to psoriasis, the term of Topic (TS) was selected in WOS with TS = “psoriasis” OR “psoriatic” OR “psoriasiform”. The publication time ranged from January 1st, 2000 to December 31st, 2021. To summarize the number of publications of nanoparticles, microneedles, nanofibers or hydrogels that were used to treat psoriasis, the term of Topic (TS) was selected with TS = “psoriasis” AND “Nanoparticle”, “psoriasis” AND “Microneedle”, “psoriasis” AND “Nanofibers”, “psoriasis” AND “Hydrogel”, respectively. The publication time also ranged from January 1st, 2000 to December 31st, 2021. To search the clinical trials for biomaterial-based therapies, the database of ClinicalTrials.gov (URL: https://www.clinicaltrials.gov) was used. The study status was selected as “All studies” with “psoriasis” for the term of “Condition or disease” and “Nanoparticle”, “Microneedle”, “Nanofiber” or “Hydrogel” for the term of “Other terms”.
3. Local treatment of psoriasis: significance and challenges
Currently, the treatment of psoriasis is achieved either systematically or topically. In systemic administration, a more efficient interaction of the drug with the target is achieved due to the circulation of the medication. This ensures that pathogenic factors are more specifically targeted. Even so, the cumulative organ toxicity or various side effects go along with the improvement of the primary disease on a long-term basis. Systematic administrations will also increase the overall drug consumption with low local drug retention in the skin tissue. Moreover, in some special cases, psoriasis patients are too weak or have too complex conditions to receive systemic treatment with high risks of systemic side effects. In such cases, the topical treatment must be applied. Actually, the topical therapy for psoriasis is of more clinical significance, not only because of the abovementioned issues of systematic therapy but also because of the following reasons: (i) most psoriasis cases involve less than 5% of body surface area,20 making topical treatment practicable; (ii) most psoriatic cases are of mild or moderate severity, and thus the topical therapeutics are recommended to avoid systemic side effects;21 (iii) the local treatment of medicines, especially biologics, is proved to be more effective due to their direct action on the disease sites while the systemic administration may lead to the loss of activities of biologics during circulation before reaching the action sites; (iv) the complex causal relationship between psoriasis and its comorbidities (e.g., metabolic syndrome and cardiovascular diseases) remains controversial. It is speculated that topical lesions release proinflammatory cytokines into circulation thus negatively affecting other systems or even the entire body (Fig. 1(B)).22,23 It was reported that in the blood of psoriasis patients, several inflammation biomarkers are detected in relation to disease activity,24 which suggests the importance of an early and active control of local limited psoriatic inflammation.
Considering the high significance of topical treatment of psoriasis in clinics, novel topical medication with a more precise target, minimized side effects and improved compliance is extremely expected. However, there are some obstacles to the development of topical medication for psoriasis. Firstly, it is impossible for hydrophilic agents or macromolecules to penetrate the psoriatic epidermis with simple topical application, especially when the hyperplastic SC poses as a rate-limiting hurdle to transdermal drug diffusion. Secondly, considering the complex micro-environment of the skin, it is necessary to develop an effective long-term working system with minimized damage to the structure and bioactivity of those biological agents. Thirdly, under the chronic pathological conditions, the psoriatic skin shows altered biophysical and biomechanical properties compared with healthy non-lesional skin, making the topical therapy of psoriasis more challenging. It is shown that psoriatic lesions had significantly lower SC hydration and friction, and significantly higher transepidermal water loss (TEWL), pH and superficial stiffness.25 It is also demonstrated that psoriasis plaques have significant lower skin distensibility and elasticity.26 Also, the full epidermal layer and SC layer in the psoriatic skin are abnormally thicker, around 2.71 and 2.41 times greater than those of the control skin, respectively.27 The thickened stratum corneum makes it even harder to achieve effective transdermal delivery of anti-psoriatic drugs, especially for those drugs predominantly exerting effects on the dermal layer. Therefore, the significantly altered biophysical and biomechanical properties of the psoriatic skin can influence the effect of the treatment, which should be taken into consideration when developing therapies for localized psoriasis management.
4. Latest developments of biomaterials for psoriasis therapy
Over the past decades, an increasing number of studies on psoriasis have been reported (Fig. 2(A)) and a variety of biomaterials have been employed to improve the efficiency of topical anti-psoriatic drug delivery for better therapeutic effects on psoriatic plagues. The representative types of biomaterials include nanoparticles, microneedles, nanofibers, and hydrogels (Fig. 2(B)). Among them, nanoparticles are the predominant one with a rapid increase of publications in the past two decades. Additionally, microneedles and nanofibers are two emerging fields in psoriasis research. As for hydrogels, they have long been a type of base that carries anti-psoriatic agents. The representative biomaterials for the topical treatment of psoriasis covered in this review are listed in Table 1. In the following paragraphs, we will focus on the designs and applications of biomaterials in combination with anti-psoriatic drugs for psoriasis plaque treatment.
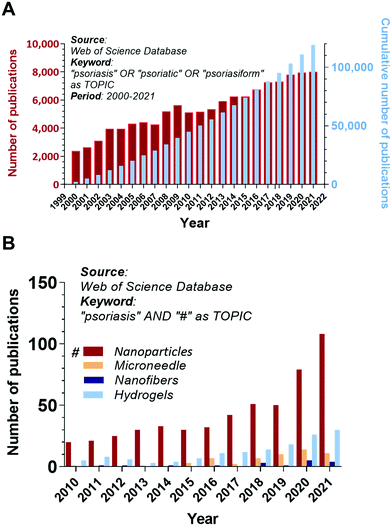 |
| Fig. 2 (A) The annual number (red, left) and the cumulative number (blue, right) of publications on psoriasis from 2000 to 2021. (B) The number of publications on four major categories of biomaterials (nanoparticles, microneedles, nanofibers, and hydrogels) for psoriasis management per year from 2010 to 2021. | |
Table 1 Representative biomaterials for the topical treatment of psoriasis
Formulation |
Properties |
Materials/drugs |
Study types |
Outcomes |
Ref. |
Microneedles |
Transdermal, sustained release, painlessness, convenient administration, self-use. |
HA |
In vitro
|
Water solubility, biocompatibility, mechanically strong, accurate drug release, inflammation inhibition. |
28
|
MTX |
Ex vivo
|
|
In vivo
|
CMC |
In vitro
|
Effective delivery, reduced psoriatic skin thickness and expression of the inflammatory factor IL-1β. |
29
|
Anti-TNF-α Ab |
In vivo
|
HA |
In vivo
|
Strong anti-inflammatory, improved psoriasis symptoms and had good safety. |
30
|
CTP |
CRISPR-Cas9 glucocorticoids |
Solid lipid nanoparticles (SLNs) |
Controlled release, drug stability, low toxicity, limited drug pack rate, storage of drug leaks and other issues. |
Lipids |
In vitro
|
The deposition of the drug in the skin was increased compared with conventional administration, and the antipsoriatic effect was increased. |
31 and 32 |
Cetyl palmitate |
Ex vivo
|
MTX |
In vivo
|
Etanercept cyclosporine calcipotriol |
|
Betamethasone dipropionate capsaicin |
|
Nanostructured lipid carriers (NLCs) |
Better drug carrying performance, large drug loading less drug leakage |
Lipid |
In vivo
|
Sustained release of the drug and better anti-psoriasis and anti-inflammatory effects were achieved. |
33
|
PTX |
Liposomes |
Targeted local, good permeability |
Lipid |
In vitro
|
Good permeability in psoriatic-like lesions, reduced the thickness and inflammation, low toxicity. |
34
|
OA |
In vivo
|
MTX |
|
Ethosomes |
Reduced vesicle volume, increased flexibility load rate of fat-soluble drugs. |
Lipid |
In vitro
|
Percutaneous absorption, increased drug retention time of the drugs in psoriasis-like lesions and improved the psoriasis-like symptoms. |
35
|
Propylene glycol HA |
In vivo
|
Curcumin |
|
Niosomes |
Stable, hydrophilic and lipophilic drugs can be loaded, extended release. |
Span 20 |
In vitro
|
Increased the skin penetration, alleviated skin damage, splenomegaly, inflammation, etc. |
17
|
Span 60 |
In vivo
|
Cholesterol Celastrol |
|
Metallic nanoparticles |
Controllability of volume and area. |
AuNP |
In vitro
|
Improved the immunomodulatory effect of MTX, alleviated the symptoms of psoriasis. |
36
|
MTX |
In vivo
|
Polymeric nanoparticles |
Biodegradable, anti-inflammatory |
Chitosan/alginate or PLGA or VES-g-ε-PLL, curcumin. |
|
Inhibit TNFα-induced HaCaT over-proliferation, good transdermal absorption and continuous release properties and improved the anti-psoriasis activity. |
37–39
|
Nanofibers |
Regulated cellular behavior, promoted skin regeneration, skin adhesion, drug carrying capacity. |
Cellulose |
In vitro
|
Increased the hydration of the skin, further promoted the absorption of the drug and improved the efficacy, and also relieved the symptoms of psoriasis. |
40
|
Curcumin |
In vivo
|
Hydrogels/hybrid |
Accelerated tissue repair, drug captured, controlled release, water locking, high patient compliance. |
Carbopol 934 |
In vivo
|
Improved the hydration of the skin and promoted the drug infiltration, sustained release, good anti-psoriasis effect. |
41
|
MTX |
4.1 Microneedles
Recently, microneedles (MNs) have been extensively used for topical transdermal drug delivery.42 They are usually composed of a baseplate and an array with tens, hundreds or even thousands of microsized needles (usually <1000 μm). The microsized needles are used to pierce the skin and create numerous microchannels in the skin,43–45 by which macromolecules (e.g., insulin,46–48 bovine serum albumin,49 and allergen ovalbumin50) are able to efficiently locate to the right places beneath the stratum corneum of the skin. According to the difference in drug transportation, MNs can be divided into solid microneedles (SMNs), coated microneedles (CMNs), dissolving microneedles (DMNs) and hollow microneedles (HMNs)51 (Fig. 3(A)). In the following sections, each microneedle is introduced and its applications for psoriasis treatment are also discussed.
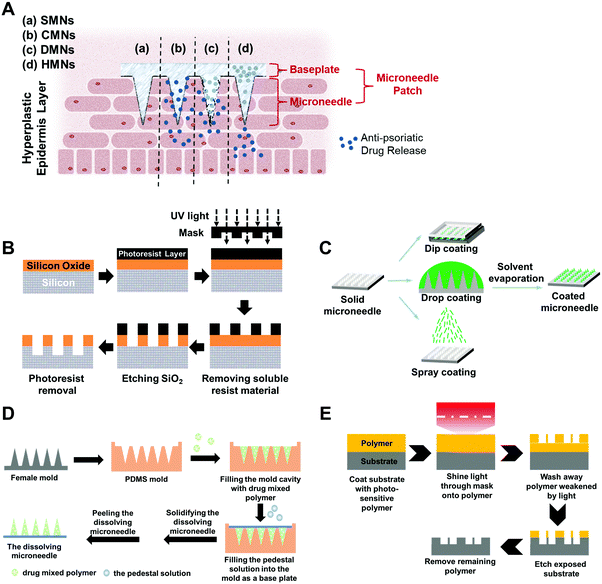 |
| Fig. 3 (A) Schematic diagram of four major types of microneedle (MN) patches. (a) Solid MNs (SMNs) to simply create micropores in the skin for enhanced permeability; (b) coating anti-psoriatic drugs on the MNs (CMNs); (c) encapsulating anti-psoriatic drugs in the dissolvable MNs (DMNs); (d) releasing anti-psoriatic drugs entrapped in the baseplate through hollow MNs (HMNs). (B) Schematic illustration of the fabrication of SMNs with MEMS. Reproduced with permission.52 Copyright (2016) Elsevier. (C) Illustration of the fabrication of CMNs with coating methods. Reproduced with permission.53 Copyright (2019) American Society for Pharmacology and Experimental Therapeutics. (D) Illustration of the micromolding strategy for DMN fabrication. Reproduced with permission.54 Copyright (2021) Elsevier. (E) Illustration of the fabrication of HMNs with MEMS. Reproduced with permission.55 Copyright (2021) Elsevier. | |
4.1.1 Solid microneedles (SMNs).
SMNs are the most common and simplest MNs. They only contain a solid microneedle array, in which no distribution or flow passages exist for drugs (Fig. 3(A)-a). Thus, SMNs are usually used for pretreatment. Typically, SMNs firstly disrupt skin barriers, and then the opened barriers will allow the efficient penetration of locally applied drugs to the skin.56 Since no drug encapsulation is needed, SMNs can be made with a wide range of materials (e.g., stainless steel,57 and silicon58) to achieve sufficient rigidity to penetrate the skin. The fabrication of SMNs mainly uses micro-electromechanical system (MEMS) technology (Fig. 3(B)).59 Although specific procedures of the MEMS may differ among different SMNs due to the various shapes and geometries, the MEMS includes three key techniques in preparing SMNs:52 (i) a patterned mask is introduced on top of a film by photolithographic imaging; (ii) the film is deposited to a substrate (e.g., silicon); and (iii) the film is selectively etched to the mask. Besides, many other methods have also been applied for SMN fabrication, such as electroplating, photochemical etching and 3D laser ablation.
The fabrication of SMNs is technically simple with no drug coating or encapsulation. In addition, due to the existence of solid microsized needles, SMNs demonstrate relatively strong mechanical strength, which makes them easy to create microchannels in the epidermis and dermis for drug transdermal delivery. However, the application of SMNs may be limited by the rapid retraction of pierced skin. Once the SMN patch is removed from the skin after piercing, the transient micro-channels that remain on the skin surface begin to shrink due to the natural resilience of the skin, making it hard for a long-term drug delivery. With the increase of the molecular weight of the drug, the skin permeation may decrease.60 Additionally, SMN application requires a two-step procedure, which brings inconvenience for practical use.
4.1.2 Coated microneedles (CMNs).
In coated microneedles, drug containing coatings are applied to the surface of the solid microneedles (Fig. 3(A)-b). When the CMNs are inserted into the skin, the drugs will be released out from the coatings after interaction with the tissue environment.52,56 Many coating methods have been developed for CMN manufacturing, which include dip coating, drop coating, spray coating, immersion coating, and layer-by-layer coating.53 In a typical coating process, the microsized needles are firstly exposed to the coating solutions, either through dipping, dropping, or spraying. After the evaporation of the solvent, a coating layer will be formed on the outer surface of the microneedles (Fig. 3(C)). Other strategies, such as inkjet printing and electro hydrodynamic atomization (EHDA), are also utilized for the CMN fabrication, which may require more complicated devices.53,59
Since CMNs are fabricated by coating the solid microneedles, the mechanical strength of CMNs will be maintained. The strong mechanical forces of the solid microneedles will assist the penetration of CMNs to the skin, where the coated drugs will be released for psoriasis therapy. Besides, the simple “coat and poke” procedure makes CMNs more convenient to use than the two-step procedure of SMNs. However, the preparation of CMNs is more sophisticated than that of SMNs because additional coating is required. Moreover, due to the dose limitation, CMNs are not suitable for drug delivery with a large amount.61 To increase the drug loading, a thicker coating has to be considered by repeating the coating process. But the thick coating can reduce the sharpness of the microneedles which may impair the penetration capacity of CMNs.62 Furthermore, the quick dissolving of the coating of CMNs will result in the rapid release of the drugs, which dramatically shorten the therapeutic window of the drugs for psoriasis. Because of the above issues, to date few studies have been investigated by using CMNs for psoriasis therapy.
4.1.3 Dissolving microneedles (DMNs).
DMNs are usually made of biocompatible, biodegradable, and water-soluble materials (e.g., carboxymethyl cellulose, hyaluronic acid,63,64 polyvinylpyrrolidone,65,66 and saccharides54). When DMNs are inserted in the skin, the microsized needles of DMNs will be dissolved due to the exposure to the watery environment of the tissue, following which the drug encapsulated in the microneedles will be spontaneously released (Fig. 3(A)-c). The most commonly used method to fabricate DMNs is micromolding (Fig. 3(D)).54,67 Generally, a polydimethylsiloxane (PDMS) mold is firstly made from a master mold. Next, the mixture solution of drugs and matrix materials is added to the PDMS mold. The materials in the mold are then solidified (e.g., heating and light irradiation) to form microneedles. Finally, the microneedles are obtained by peeling from the mold. Other methods for DMN fabrication include droplet-born air blowing, drawing lithography, ultrasonic welding, laser machining, and hot embossing.59
By selecting suitable materials, DMNs can achieve enough mechanical strength for skin penetration and effectively control drug release as the matrix material dissolves in the tissue. Besides, DMNs have good biodegradability and compatibility, which make them a promising choice for long-term psoriasis therapy with good patient compliance. Although there is great achievement of DMNs in transdermal drug delivery, several drawbacks are still associated with DMNs. For example, relatively low drug encapsulation sometimes occurs in DMNs, which will lead to low therapeutic efficacy. Due to the use of organic solvents, light and even elevated temperature in preparing the microneedles, biomacromolecular drugs (e.g., proteins and DNA) may lose activities.62 In addition, the residue solvents and the matrix materials in DMNs may induce skin irritation. Nowadays more efforts have been made to improve the therapeutic effect of DMNs as well as reduce the side effects.
4.1.4 Hollow microneedles (HMNs).
In hollow microneedles, the needles are hollow in the center with holes at the needle tips. As the HMNs are inserted into the skin, the drugs will pass through the cavities of the needles to reach the dermis under the assistance of pressure systems (e.g., micropumps) (Fig. 3(A)-d).59,68 Usually, HMNs are used for delivering high molecular weight compounds such as proteins, oligonucleotides, and vaccines.56 The fabrication of HMNs is often based on MEMSs, and mainly contains three steps:55 (i) depositing a photosensitive thin film on a substrate (e.g., silicon, metals, ceramics, and glass); (ii) making a pattern on the substrate by lithography techniques; and (iii) removing excessive materials in the substrate by etching (Fig. 3(E)).
In comparison with SMNs, CMNs, and DMNs, HMNs possess higher drug delivery capacity by which drugs can continuously pass through the cavities of HMNs to the skin. Also, the drug flow rate can be regulated by adjusting pressure systems to acquire the expected drug dosage for disease treatment.68 The possible limitation associated with HMNs is that the pores at the needle tips may be blocked by the skin tissue during the insertion of the needles to the skin. To avoid the occurrence of this issue, the microneedles can be withdrawn or manufacture the pores on the side walls of the needles.55 Another disadvantage of HMNs is the use of pressure devices making the application of these microneedles more sophisticated. Nevertheless, the advantageous properties of HMNs make them a novel and promising transdermal delivery system for psoriasis therapy.
4.1.5 Application of microneedles for psoriasis therapy.
In the past decades, studies have proved that microneedles are effective in the treatment of psoriasis. J. H. Lee et al. conducted a small-sized clinical trial on psoriatic patients resistant to the therapy of topical calcipotriol/betamethasone ointment.69 In this study, an HA-based SMN patch with 650 μm in height was placed over the lesions after topical calcipotriol/betamethasone ointment application simply for enhancing drug permeation. The one-week trial showed improved therapeutic effects of those topical agents with the assistance of MNs. In another study, HA-based DMN patches with MTX encapsulated in the needles were applied to an imiquimod (IMQ)-induced psoriasiform model in mice (Fig. 4).64 The needles had heights of 650 ± 19 μm and their mechanical properties made it possible for them to be successfully inserted into the full-thickness porcine cadaver skin (Fig. 4(B)) with rapid (not controlled) drug release. This MN-based topical medication ameliorates mouse psoriatic lesions with lower drug doses and alleviated systemic side effects compared with oral MTX administration (Fig. 4(C)). Recently, an HA-based DMN patch was developed to mitigate inflammatory skin diseases (ISDs) including psoriasis via genetically disrupting NLRP3 inflammasome, an inflammatory protein complex closely associated with inflammatory skin conditions and causes glucocorticoid resistance in inflammatory diseases.70 In this work, the DMNs were used for the transdermal delivery of NLRP3-targeted Cas9 nanocomplexes and dexamethasone (Dex)-loaded nanoparticles, after which the two nanoparticles were internalized into cells to specifically inhibit NLRP3 inflammasome for enhanced psoriasis therapy via CRISPR-Cas9 induced genome editing (Fig. 4(D)).
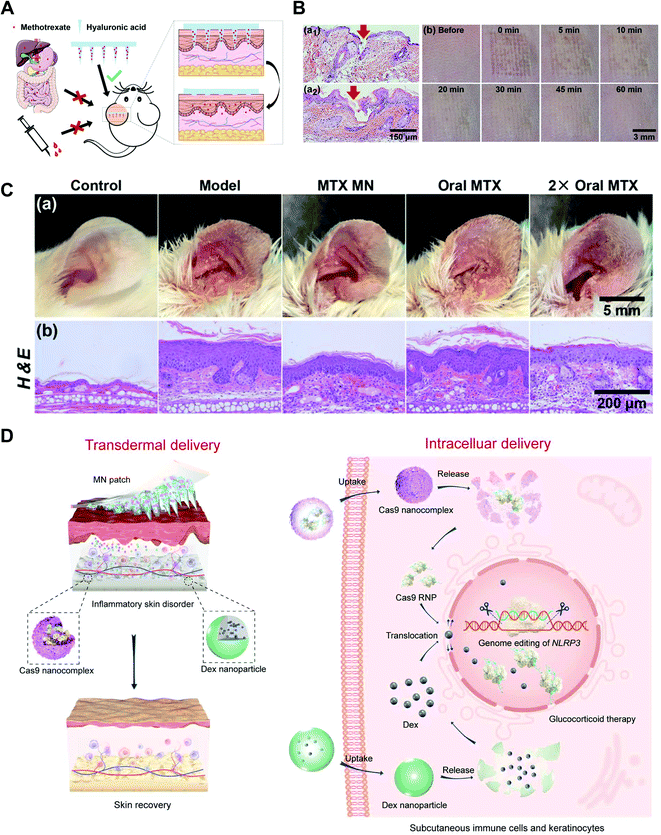 |
| Fig. 4 Representative drug loading MN patches for psoriasis management. (A) Topical delivery of MTX through MN patches reduced the systemic toxicity of MTX. (B) In vivo skin inserting performance of the HA MNs. Representative images of H&E staining of skin tissues from a healthy mouse and (a1) and IMQ-induced psoriatic mouse (a2) after 3 min insertion of MTX-encapsulated MNs. Red arrows indicated inserted sites. (b) Representative photographs of HA MN-inserted mouse skin at predetermined time points. (C) In vivo application of MTX-encapsulated MN patches and oral administration of MTX on an IMQ-induced psoriasiform mouse ear. (a) Representative photographs of ear lesions and (b) representative images of H&E staining of skin tissues from ear lesions after 7 days of administration. Reproduced with permission.64 Copyright (2019) American Chemical Society. (D) Schematic diagram of transdermal delivery (left) and intracellular delivery (right) of the Cas9 ribonucleoprotein nanocomplex and dexamethasone (Dex) nanoparticles for inflammatory skin disorder (ISD) management. Reprinted from. ref. 70 ©The Authors, some rights reserved; exclusive licensee AAAS. Distributed under a CC BY-NC 4.0 license https://creativecommons.org/licenses/by-nc/4.0/. | |
It is worthy to note that most of the MN patches are fabricated by top-down approaches with moldings, the manufacture of which is expensive and technically demanded. Moreover, the MN arrays fabricated by this approach are usually upright and tend to fall off the skin. In order to overcome these drawbacks, a novel serrated clamping MN was developed by Zhang and coworkers with the aim of making a feasible, inexpensive and wearable MN-based device (Fig. 5).71 This MN was inspired by the serrated microstructure of mantises’ forelegs (Fig. 5(A)) and showed firm adherence to the skin even when moving (Fig. 5(B)). The mouse psoriasis model verified that this angled MN patch was effective in treating psoriasis (Fig. 5(C)). This study was highly inspiring for its innovative modification of conventional MN design and fabrication, making MN-based devices more versatile for better clinical application.
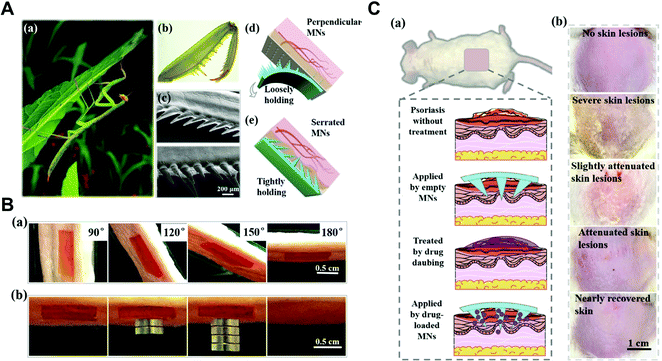 |
| Fig. 5 Serrated clamping MN patch mimicking mantises’ forelegs using flexible ferrofluid-configured moldings. (A) Schematic diagram of bio-mimetic serrated clamping MNs. (a) Photo of a leaf-climbing mantis. (b) Optical image of mantises’ forelegs. (c) Scanning electron microscopy (SEM) image of the microstructure of mantises’ forelegs. (d) Loosely holding perpendicular MNs. (e) Tightly holding serrated MNs. (B) Good adhesion of the bio-mimicking serrated clamping MN patch. (a) The MN patch tightly attached to the swinging arm. (b) Load bearing test of the MN patch. (C) In vivo administration on IMQ-induced psoriatic mice skin. (a) Schematic diagram of different treatments for IMQ-induced psoriatic skin. (b) Photos of the differently treated skin lesions after administration for 3 days. Reproduced with permission.71 Copyright (2019) Elsevier. | |
As a straightforward way to create microchannels in the skin, the MN patch stands out as a promising strategy for effective transdermal drug delivery for psoriasis treatment. When designing MN patches for psoriasis therapy, the following aspects should be considered: (i) the types of microneedles. For burst release, CMNs are more applicable while for sustained and controlled release, DMNs and HMNs are advised. (ii) The mechanical properties (e.g., hardness, toughness, and strength) and the length of MNs. As mentioned above, the psoriatic lesion showed higher superficial stiffness,25 which may require a higher hardness of MNs, and the hyperplastic SC layer with a thickened epidermal and SC layer27 may ask for a longer length of MNs. (iii) The differences of biomechanical properties between human psoriatic skin and animal skin (e.g., pigs, mice and rats). The animal results should be carefully evaluated before moving forward to the application of MN patches on human patients.
4.2 Nanoparticles
Nanoparticles (NPs) are a major category and have been extensively explored as an efficient medium in nanoscale dimensions for drug delivery. NPs have a large surface area-to-volume ratio and generally penetrate through the hair follicle's orifices of the skin. Specifically, NPs penetrate deep through the skin via the intercellular lipid route, transcellular route or follicular route for the delivery of both hydrophilic and hydrophobic molecules. The effect of drug permeation through NP carriers is influenced by various physical and chemical properties of NPs such as the diffusion coefficient, lipid-water (o/w) partition coefficient, surface charge, and pH value. It was reported that NPs with small size, small molecular weight (MW), positive charge and moderate pH were more likely to penetrate through the skin.72The skin under psoriatic conditions exhibits varied properties compared with healthy skin. For example, psoriatic skin was reported to have a higher pH value than normal skin. Thus, adjusting the characteristics of NPs for psoriasis application is of great significance for better NP permeation. To date, a great deal of work has been trying to explore NPs in psoriasis treatment. There are mainly three categories of NPs that have been under intensive exploration for transdermal drug delivery and psoriasis management, which are lipid-based nanoparticles (Fig. 6(A)), metallic nanoparticles (Fig. 6(B)), and polymeric nanoparticles (Fig. 6(C)).
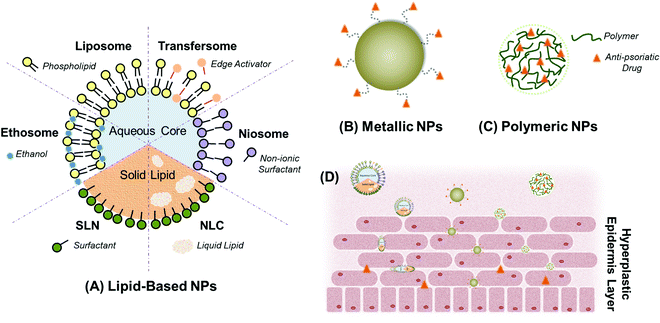 |
| Fig. 6 Schematic diagram of three major types of nanoparticles (NPs) in psoriatic skin permeation. (A) Lipid-based NPs: solid lipid nanoparticles (SLNs), nanostructured lipid carriers (NLCs), liposomes, transfersomes, ethosomes, and niosomes. (B) Metallic NPs. (C) Polymeric NPs. (D) Schematic illustration of the transdermal mechanism of different nanoparticles. | |
4.2.1 Lipid-based nanoparticles.
Lipid-based NPs are the most widely investigated nanoparticles for psoriasis therapy. They have a variety of types, including solid lipid nanoparticles (SLNs), nanostructured lipid carriers (NLCs), liposomes, transfersomes, ethosomes, and niosomes (Fig. 6(A)). In fact, lipid-based nanocarriers are more advantageous for transdermal drug delivery than other nanoparticles. This is because the lipid-based nanoparticles are highly flexible, which can be transformed to pass through the extracellular matrix (ECM) in the SC layer (Fig. 6(D)). Moreover, the ECM is composed of a mixture of lipids including cholesterol (CH), ceramides and free fatty acids (FFAs),73 which provides a lipophilic environment for better penetration of lipid-based nanoparticles.
SLNs are synthesized with solid lipids such as triglycerides, glycerides and fatty acids.74 In a study on MTX topical administration, Ferreira et al. formed cetyl palmitate based SLNs incorporated with MTX, with the addition of etanercept conjugation to achieve a co-delivery of two types of anti-psoriatic drugs.75 A significant accumulation of MTX within the human normal and psoriatic skin biopsies was observed. Besides, SLNs have been chosen for assisting the topical transdermal delivery of anti-psoriatic drugs such as cyclosporine, calcipotriol, betamethasone dipropionate and capsaicin.76–78 While SLNs are synthesized with solid lipids, the lipid polymorphism brings significant drawbacks including limited drug loading and drug leakage during storage.79 Thus, an optimized type of SLN, namely NLCs, was developed with superior drug loading properties. This was due to the addition of a liquid lipid to the system. The liquid lipid was blended with a solid lipid to obtain an amorphous crystalline structure with more space for drug loading and limited drug expulsion.80,81 Pinto et al. produced NLCs encapsulated with MTX with a high encapsulation efficiency (>60%) and a prolonged drug release profile (8 h).82 The in vitro drug permeation test verified the efficacy of NLCs as a transdermal drug carrier. Ghate et al. developed NLCs for pentoxifylline (PTX, a hydrophilic methylxanthine derivative for treating psoriasis) encapsulation and transdermal delivery in psoriasiform lesions in mice.83 In this study, NLCs were manufactured using microwave irradiation, providing a novel rapid synthetic method for NLCs with simplicity and feasibility in laboratories. With a controlled drug release for 7 h, NLCs presented better anti-inflammatory effects on lesions than single drug administration.
Liposomes are hollow spherical nanostructures with a lipid bilayer, conventionally produced from natural phosphatidylcholine (PC) and CH.84 Liposomes release cargo into the skin through either fusion with the lipids or their disintegration in the SC layer.85 Phospholipids are the major component with self-assembly capacity to form liposomes in aqueous media while CH works to enhance the stability of lipid bilayers of liposomes. However, it has been reported that conventional liposomes had unsatisfactory permeability through the intercellular pathway due to their limited deformability and its resulting rupture.86 Thus, optimized methods of liposome preparation for a better skin permeation are under development. For instance, a study conducted by Srisuka et al. reported a kind of deformable liposome entrapped with MTX prepared from PC with the addition of oleic acid (OA) other than conventional CH.87 After the comparison between the deformable liposomes (PC2.5:OA1) and conventional liposomes (PC2:CH1 and PC9:CH1), it was found that the former had enhanced MTX permeation through porcine skin, owing to the increased skin permeability by OA (a skin penetration enhancer). Another study further proved that deformable liposomes formulated with the addition of OA helped achieve topical administration of MTX in a mouse model with anti-psoriasis effects.34 Recently, Xi et al. developed a dendritic cell (DC) targeted liposome for psoriasis therapy. DCs played an essential role in psoriasis initiation and maintenance through presenting antigens to naive T cells as well as activating them for subsequent inflammatory cytokine production. The targeting of liposomes to DCs inhibited the maturation of dendritic cells (DCs), subsequently ameliorating psoriasis.88
In order to enhance the deformability and flexibility of conventional liposomes for better skin permeation, transfersomes are created as a modified form of liposomes with the addition of edge activators (e.g., sodium cholate, sodium deoxycholate, Span 60, Span 65, and Span 80) into the vesicular membrane, which can change the interfacial tension and endow lipid vesicles with ultra-deformability.32,72,89 Additionally, edge activators also disturb the ordered arrangement of lipid bilayers to lower the transition temperature (Tm) of lipid membranes.90 Several studies have investigated the employment of transfersomes for transdermal drug delivery, such as lipophilic drugs, dexamethasone89 and retinyl palmitate,91 in terms of drug permeation, distribution and therapeutic effects, which imply the potential of transfersomes for psoriasis treatment.
Ethosomes are a novel type of ethanol-containing deformable liposome, in which ethanol serves as a penetration enhancer for assisting topical transdermal drug delivery. The addition of ethanol is also reported to cause a reduction in vesicular size through conferring negative charges on vesicles.92 In addition to ethanol, other short-chain alcohols such as propylene glycol and glycerol can be used to prepare ethosomes, which increases both the flexibility of the nanocarriers and liposoluble drug loading.93 For instance, a novel targeted hyaluronic acid (HA)-modified ethosome (HA-ES) drug delivery system was constructed (Fig. 7(A)). Its aim was to topically deliver curcumin for effective psoriasis treatment by targeting the overexpressed CD44 protein in the psoriatic skin.35 Its enhanced transdermal drug delivery function was observed by fluorescence as shown in Fig. 7(B). HA-ES significantly mitigated the epidermal hyperplasia of the IMQ induced psoriatic ear in a mouse (Fig. 7(C)). In another research, Guo et al. adopted D-α-tocopherol acid polyethylene glycol succinate (TPGS) as a permeation enhancer and a stabilizer to modify curcumin loaded ethosomes.94 This kind of multifunctional ethosome was proved to have synergistic therapeutic effects on the animal model of psoriasis.
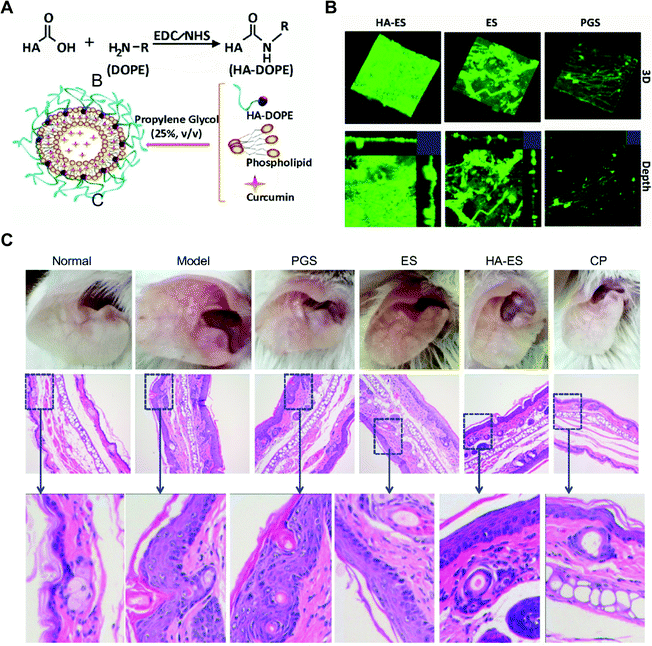 |
| Fig. 7 Hyaluronan (HA)-modified ethosome (HA-ES) loaded with curcumin for treating psoriasiform mouse skin through targeting CD44. (A) Schematic diagram of the fabrication of HA-ES. (B) Fluorescence images of psoriasiform mouse skin captured using a confocal laser scanning microscope. (C) Representative photographs of mice ears and H&E staining of skin tissues from different groups on day 10. PGS: curcumin 25% propylene glycol solution. CP: clobetasol propionate cream. Reproduced with permission.35 Copyright (2019) Ivyspring. | |
Niosomes are a novel type of vesicle composed mainly of non-ionic surfactants and CH (or its derivatives). Surfactants are amphiphilic molecules with a hydrophilic head and a lipophilic tail, which participate in forming a vesicle with an aqueous core and lipophilic domains of the bilayers. The addition of surfactant achieves a looser SC layer for better permeation. Compared with conventional liposomes, niosomes are more stable and capable of entrapping both hydrophilic and lipophilic substances for sustained and continuous drug delivery.95 Due to their excellent properties, recent years have witnessed the emerging investigation of niosomes for psoriasis management. In a study, MTX loaded niosomes were prepared with Span 60 and CH. In vivo drug deposition study and histopathological analysis proved that niosomes were effective in transdermal drug delivery with systematic safety, implying the high potential of the niosomes for psoriasis therapy.96 Similarly, in another study, diacerein loaded CH-rich niosomes were formulated with Span 60 and CH (weight ratio: 9
:
1), which exhibited high stability and promoted the penetration of diacerein in the normal rat skin, holding the potential for topical psoriasis treatment.97 Recently, niosomes composed of Span 20, Span 60, and CH (weight ratio: 3
:
1
:
1) were loaded with celastrol for in vivo psoriasis mouse application, showing effectively alleviated psoriatic inflammation.98
4.2.2 Metallic nanoparticles.
Metallic NPs pose as rigid nanocarriers with highly tunable size and surface characteristics (Fig. 6(B)). NPs composed of gold, silver, metallic oxides of iron, titanium or zinc are of great research potential for drug delivery in psoriatic skin. For instance, Bessar H et al. used functionalized gold nanoparticles (AuNPs) to load and successfully deliver MTX in the C57BL/6 mouse skin with both epidermal and dermal drug absorption.99 Compared with single MTX treatment, these novel nanocarriers significantly improved the inhibitory effect of MTX on keratinocytes in vitro. This study provided a preliminary proof for AuNPs in percutaneous drug delivery. A recent study applied MTX-coupling AuNPs to an IMQ-induced mouse model and a human xenograft mouse model to investigate their therapeutic effects in vivo (Fig. 8). It was shown that AuNPs significantly enhanced the immunomodulatory effects of MTX, leading to alleviated psoriatic lesions and reduced CD45+ immune cell infiltration and IL-17 levels.100 With the assistance of AuNPs, researchers could further investigate the underlying mechanisms whereby the drug exerts the therapeutic effects. In another study, AuNPs functionalized with 3-mercapto-1-propanesulfonate loaded with MTX were also topically applied in vivo and exhibited therapeutic effects on the psoriasiform lesions.101 Crisan et al. demonstrated that AuNPs and silver nanoparticles (AgNPs) complexed with Cornus mas (anti-inflammatory polyphenol-rich extract) showed both in vitro anti-inflammatory effects on bone marrow-derived murine macrophages and in vivo anti-psoriatic function in patients.102
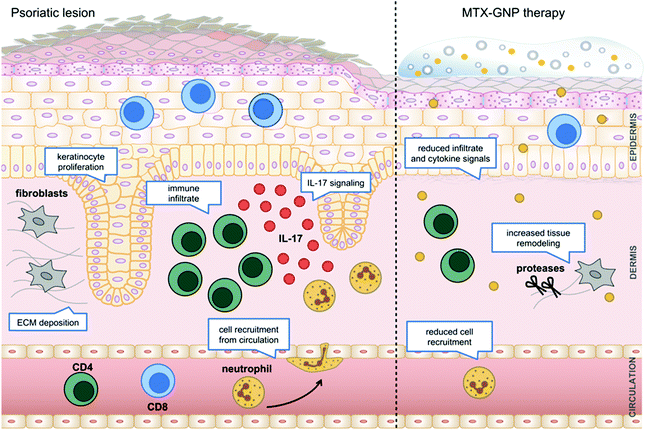 |
| Fig. 8 Schematic illustration of MTX-coupling AuNP mitigating psoriatic lesion and its underlying mechanisms including reducing inflammatory cell infiltration and inflammatory factors, as well as increasing tissue remodeling. Reproduced with permission.100 Copyright (2019) Elsevier. | |
4.2.3 Polymeric nanoparticles.
A wide range of polymeric NPs are being studied in transdermal drug delivery, constructed with natural or synthetic polymers (Fig. 6(C)). For natural polymeric NPs, chitosan is the most studied ingredient for the formulation. It is positively charged and possesses anti-inflammatory effects,103 making it rather appropriate for drug delivery especially in psoriatic inflamed skin. Gomez et al. synthesized chitosan/alginate-based nanoparticles for curcumin encapsulation, which was applied to an in vitro psoriasis-like cell model induced by tumor necrosis factor-α (TNF-α) on keratinocytes with the combination of photo-irradiation.37 In this study, curcumin worked as a photosensitizer and further investigation using animal models is required. Despite their natural origin and biocompatibility, several drawbacks of natural polymeric NPs hinder their applications, such as the varying purity between batches and the difficulty to achieve reproducible release profiles.72 Therefore, synthetic polymeric NPs with better defined characteristics were developed for drug delivery. Poly(lactide-co-glycolic acid) (PLGA) is a kind of FDA-approved aliphatic polyester being widely investigated in biomaterials, due to its biodegradability and potential for drug encapsulation. For psoriasis treatment, PLGA NPs loaded with curcumin of two different sizes were fabricated by Sun et al. (Fig. 9(A)) and the study showed improved in vitro drug permeation through psoriatic mouse skin (Fig. 9(B)) and in vivo anti-psoriasis effects (Fig. 9(C)).38 Recently, researchers fabricated cationic nanoparticles (cNPs) with PLGA and poly(2-(diethylamino)ethyl methacrylate) (PDMA) to inhibit cfDNA (cell free DNA)-LL37 immunocomplex-driven cell activation in psoriasis.104 The high DNA-binding affinity of this cationic polymer allows effective pulling of cfDNA out of the DNA-LL37 complex and thus improves the therapy of psoriasis (Fig. 9(D)).
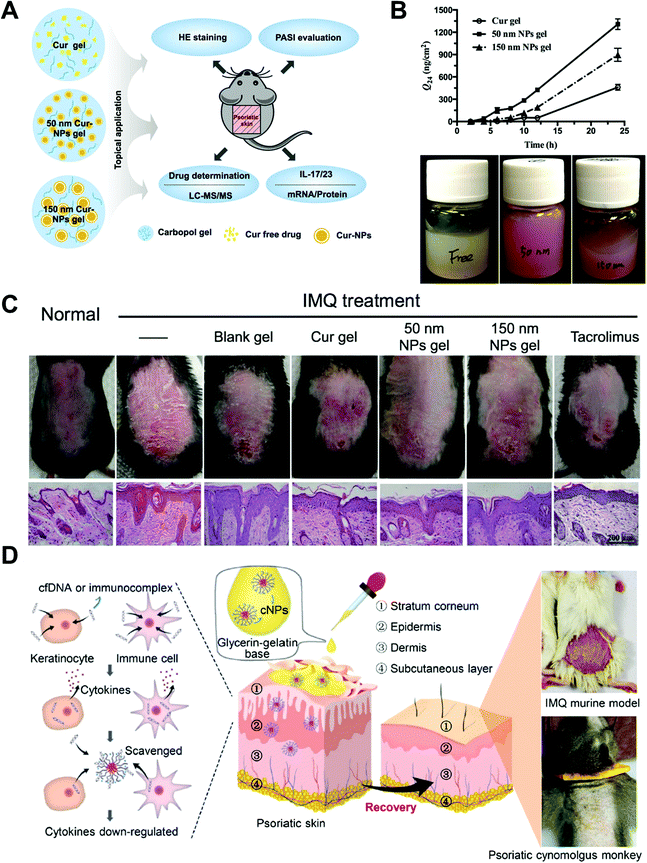 |
| Fig. 9 Representative studies investigating synthetic polymeric NPs for psoriasis treatment. (A) Schematic illustration of curcumin PLGA NPs of two different sizes, 50 nm and 100 nm, loaded with curcumin for mouse psoriasis treatment. (B) Permeability curves of the Cur gel, 50 nm Cur-NP gel and 150 nm Cur-NP gel (upper) and macroscopic photos of the Cur gel, 50 nm Cur-NP gel and 150 nm Cur-NP gel (below). (C) Representative photos of mouse psoriatic skin (upper) and H&E stained skin sections (below) after 7-day different management. Scale bar = 200 μm. Tacrolimus cream was used as a positive control. Cur: curcumin. NPs: nanoparticles. Reproduced with permission.38 Copyright (2017) Elsevier. (D) Schematic illustration of the underlying mechanisms of cationic nanoparticles (cNPs) in improving psoriatic lesions. It involves the scavenging of cell free DNA (cfDNA), the decrease of the DNA-LL37 complex and the downregulation of the inflammation level. Reprinted from.104 ©The Authors, some rights reserved; exclusive licensee AAAS. Distributed under a CC BY-NC 4.0 license https://creativecommons.org/licenses/by-nc/4.0/. | |
To sum up, NPs have great potentials for transdermal drug delivery in psoriatic skin. Despite the large number of studies, it is still challenging to achieve satisfactory therapeutic effects. There are several key factors that need to be taken into consideration. Firstly, the unique psoriatic skin tissue structure, especially the varied organization and composition of the SC layer in the lesion area, may cause changes in NP-penetrating behavior. Besides, the pH value and charge are also altered in psoriatic skin.25 Unlike the normal skin, whether psoriatic skin poses as a more fragile or more impregnable barrier for NP penetration needs further investigation. Secondly, specific penetration and drug-release mechanisms of different types of NPs lack updated and more detailed research. For example, do lipid-based NPs achieve drug delivery through ruptures in the superficial layers or through maintaining an intact structure during skin permeation? Thirdly, the targeted NP is advised for higher therapeutic efficiency and limited adverse effects. To dig out the suitable targeting ligands, differential proteomics or genomics of psoriatic skin may be conducted. Fourthly, the stability, permeating velocity and drug-release properties of NPs should balance well to realize an ideal drug delivery for optimized psoriasis therapy.
4.3 Nanofibers
Nanofibers are fibers with a nanosized diameter. They are now intensively investigated and employed in the fields of tissue engineering and drug delivery. To date, NFs have found application as dressings in dermal wound healing as extracellular matrix (ECM)-mimic scaffolds. The arrangement and diameter of NFs could be orchestrated for affecting cellular behaviors thus promoting skin tissue regeneration.105–107 The fabrication of NFs mainly depended on the electrospinning (Fig. 10(A)).108 In the general process of electrospinning, the polymer and drug solution are charged into a syringe. Then a high voltage is applied between the syringe nozzle and the collector, under which nanofibers will continuously form as the polymer solution gradually pumped out from the syringe. Solution blow spinning is also applied to prepare NFs.109 In this method, the compressed air replaces the high voltage of electrospinning, which propels the continuous injection of polymer solution to form NFs (Fig. 10(B)). Another strategy to manufacture NFs is centrifugal spinning, in which high-speed centrifugation is applied to generate a liquid jet to form NFs (Fig. 10(C)).110 Besides, other approaches, such as CO2 laser supersonic drawing, plasma-induced synthesis and electrohydrodynamic direct writing, have also been reported for NF fabrication.111
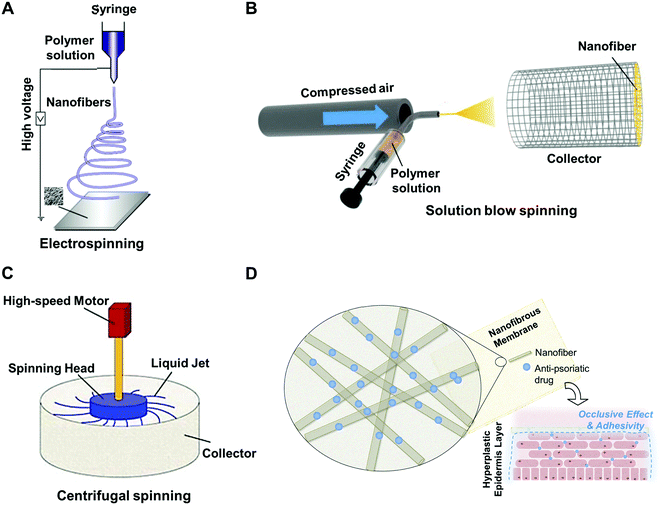 |
| Fig. 10 Schematic illustration of the setup of the electrospinning (A), solution blow spinning (B) and centrifugal spinning (C) for NF fabrication. (D) Schematic diagram of nanofibrous membranes fabricated with anti-psoriatic drugs as skin adhesive dressings with occlusive effects that enhance drug permeation. Figure A: reproduced with permission.117 Copyright (2020) MDPI. Figure B: reproduced with permission.118 Copyright (2021) MDPI. Figure C: reproduced with permission.119 Copyright (2014) Taylor & Francis. | |
For psoriasis management, NFs have been employed as vehicles for anti-psoriatic agent delivery in several studies in recent years (Fig. 10D).112,113 In one study, researchers developed electrospun PMVE/MA-ES NFs loaded with three therapeutic agents (salicylic acid, methyl salicylate, and capsaicin) as keratolytics and analgesic agents for alleviating the dermal symptoms of psoriasis.114 This novel biodegradable NF-based delivery system significantly prolonged the stability of encapsulated drugs to up to fifteen days and effectively activated the TRPV1 (transient receptor potential cation channel 1, a target of analgesic therapy) at a cellular level. Particularly, at the end of the study, the NFs were electrospun onto an adhesive patch to observe the effects on a human volunteer. After 8 h treatment, NF degradation and skin redness were observed which confirmed the successful agent release from the NFs and its subsequent effects on skin. Besides, several recent studies have reported anti-psoriatic drug loaded NF membranes as candidates for psoriasis management, although the in vivo effects of these membranes are lacking. For example, bacterial cellulose/carboxymethylcellulose (BC/CMC) based NF membranes were loaded with MTX for efficient release.115 A hydrocortisone-loaded polyacrylonitrile (PAN) electrospun nanobandage was fabricated and tested for drug release and bio-safety at the cell level, as a candidate for psoriasis management.116 The concept of the “anti-psoriatic nanobandage” put forward in this study was inspiring. Due to its characteristic membranous structure and drug loading capacity, the NF film was a highly potential candidate for the manufacture of anti-psoriasis skin adhesive dressings, which were easy to use and could closely and firmly fit psoriatic lesions for prolonged interaction time.
4.4 Hydrogels
Hydrogels are the hydrophilic polymer networks with high water content. They are formed either from natural polymers or synthetic polymers through physical or chemical crosslinking (Fig. 11).120 They have been extensively used as scaffolds for accelerating tissue repair, or directly entrapped therapeutic agents for effectively controlled topical release. In psoriasis management, hydrogels usually work for sustained anti-psoriatic drug release.38,121–125 For instance, early in 1992, Ormerod et al. prepared a dithranol-impregnated hydrogel to treat psoriasis. The drug-loaded hydrogel was fabricated by directly adding dithranol solution to the preformed polyurethaneurea hydrogel film for drying. The clinical result proved that the slow release of dithranol from the hydrogel film could effectively improve psoriasis treatment. In a recent study, Rana et al. developed a betamethasone-loaded topical hydrogel (B-Gel) for psoriasis treatment.125 The hydrogel was prepared by heating and cooling the mixture solution of betamethasone and A6 gelator. The hydrogel could efficaciously overcome the drawbacks of the ineffective entrapment of steroids, burst release of the entrapped drugs, poor skin permeability, and high toxicity, which finally mitigate psoriasis associated symptoms. In another study, He et al. developed a hydrogel-based microemulsion drug delivery system for the transdermal delivery of indirubin.122 The result demonstrated that the hydrogel system could effectively keep the microenvironment moisture, and promote the drug penetration and retention inside the skin, thus achieving better therapeutic effects.
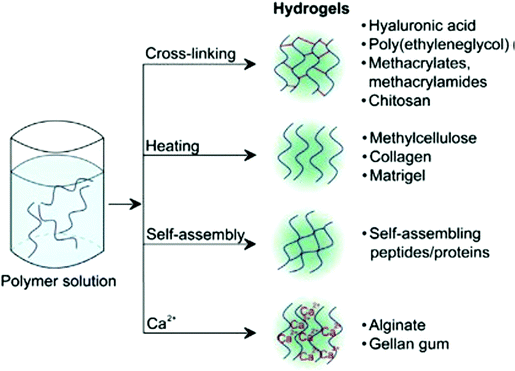 |
| Fig. 11 Different hydrogels and their formation mechanism. Reproduced with permission.120 Copyright (2018) Elsevier. | |
4.5 Hybrid systems
NFs hold the potential of being fabricated into adhesive anti-psoriasis dressings due to their membranous structure, while hydrogels could provide a much moister environment to the diseased skin. Transdermal drug delivery simply through these devices may have limited efficacy, thus research has combined NPs with NFs40 and hydrogels126–128 for more effective psoriasis therapy. The hybrid system possesses the advantages of various materials, exhibiting a promising prospect in the psoriasis management.41,129,130 In one study, cellulose nanofiber (CNF) films were prepared using a vacuum filtration method for topically delivering curcumin-loaded nanostructured lipid carriers (NLCs) to a mouse model of psoriasis.40 The CNF film in this system played an important role in not only delivering drugs, but also improving and maintaining skin hydration through occlusive effects when tightly adhered to the skin surface (Fig. 12(A)), thus helping enhance the efficacy of both NLCs and curcumin as well as minimizing psoriatic symptoms via enhanced skin moisture. SEM (Fig. 12(B)-b,c) and AFM (Fig. 12(B)-d) demonstrated that NLC-containing CNF films were much smoother than those without NLC.
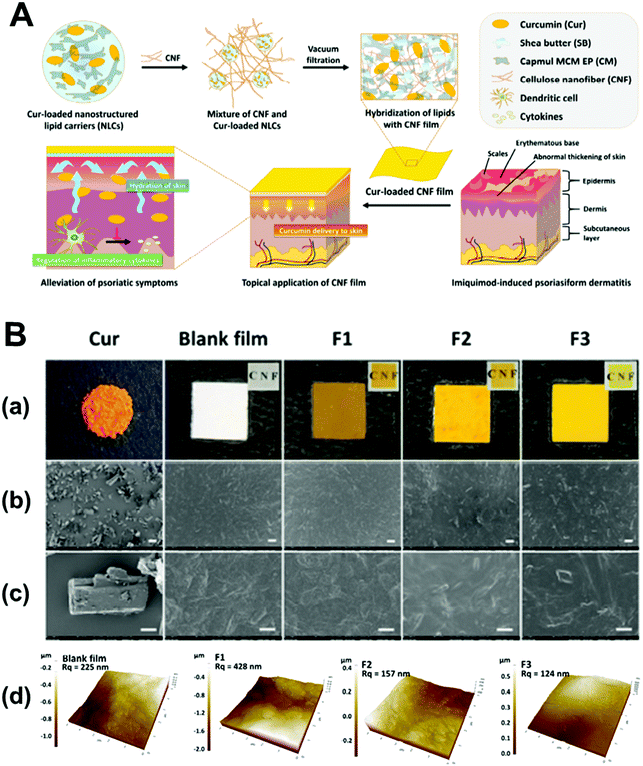 |
| Fig. 12 Cellulose nanofiber (CNF) films prepared for transdermally delivering curcumin loaded NLCs in a mouse model of psoriasis. (A) Schematic sketch of the fabrication and application of a curcumin-loaded lipid-hybridized CNF film. (B) Macroscopic images (a) and representative SEM images (b), (c) of the curcumin blank (Cur), CNF film (blank film) and curcumin loaded NLCs (F1, F2 and F3). Scale bar = 20 μm. (d) AFM results of the blank CNF film and curcumin loaded NLCs (F1, F2 and F3). Blank film: CNF without Cur; F1: CNF/Cur; F2: CNF/Cur/SB; F3: CNF/Cur/CM. Cur, Curcumin. SB: Shea butter. CM: Capmul MCM EP. Reproduced with permission.40 Copyright (2018) Elsevier. | |
Recent studies have incorporated nanoparticles into various kinds of semi-solid gelling agents for topical management, including the hydroxy propyl methyl cellulose (HPMC) gel,131 and carbopol gel.34,75,76,132 This improves viscosity in easy topical skin application to prolong the retention of therapeutic nanoparticles on the inflamed skin. Besides, colloid hydrogels are also under investigation for nanoparticle delivery such as the silk fibroin hydrogel133 for providing a moist environment to the psoriatic lesions and the resulting enhanced skin hydration help facilitate the deep penetration of drug-loaded nanoparticles into the skin (Fig. 13(A)). Compared to other agents for skin external use like ointment and emulsions, hydrogels are less greasy and sticky, which increases compliance in patients, and serve as a moisturizer to ameliorate psoriatic syndrome (Fig. 13(B)).
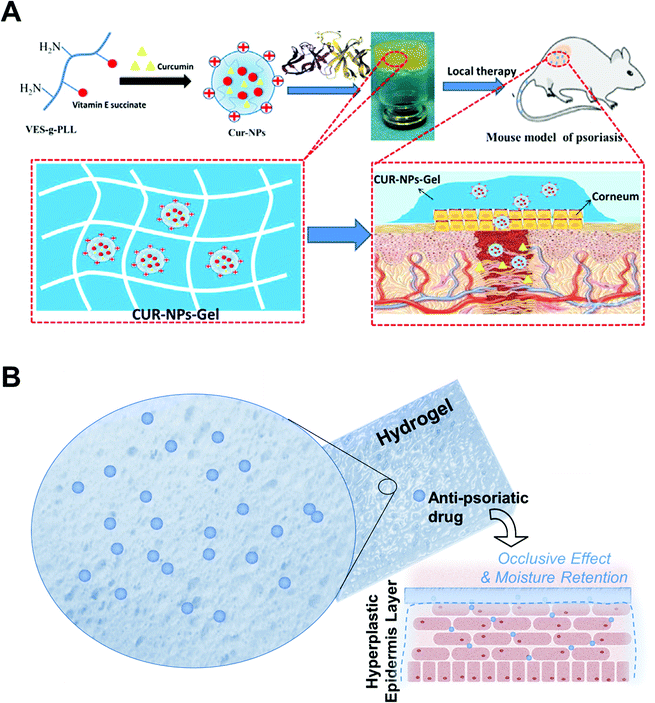 |
| Fig. 13 (A) Schematic illustration of a silk fibroin hydrogel raising the permeating efficiency of drug loaded NPs for improved psoriasis therapeutic effect. Reproduced with permission.133 Copyright (2017) Elsevier. (B) Schematic diagram of hydrogels encapsulated with anti-psoriatic drugs that mitigate psoriatic lesions through both drug efficacy and skin moisture retention. | |
5. Clinical trials
Currently, several clinical trials are completed or undergoing for biomaterial-based treatment for psoriasis. Two clinical trials on the use of microneedle patches for the treatment of psoriasis have been completed, both of which were related to the delivery of corticosteroids. This evaluated the effect of hyaluronic acid (HA) microneedle patches enhancing the transdermal administration of the topical drug (calcipotriene/betamethasone ointment) in the treatment of psoriasis-resistant plaques.134 Recently, another randomized controlled trial of autolytic microneedle patches coated with corticosteroids for nail psoriasis was reported.135 There are relatively many clinical applications of nanoparticles as drug carriers in the treatment of psoriasis. In 2017, Maha et al.136 prepared a jojoba-based microemulsion system coated with tazarotene and applied it to 10 patients with plaque psoriasis, which had advantages in reducing tazarotene irritation, enhancing skin deposition, and improving the therapeutic effect of patients with psoriasis. In a recent study, Riham et al.137 prepared oleuropein into a microemulsion formulation. It had also been demonstrated in 20 patients with plaque psoriasis that it was superior to the marketed clobetasol propionate cream, with the ability to significantly improve the clinical manifestations of psoriasis. In addition, there is a completed phase 4 clinical trial, which evaluates the efficacy of ethosome and liposome preparation of anthralin in psoriasis.
Clinical trials on hydrogels for the treatment of psoriasis began earlier. As early as 1992, a clinical trial of dithranol-impregnated hydrogels was reported.138 Since then, several trials have focused on the preparation and application of 0.25% MTX hydrogels or liposome hydrogels to clinical patients, all of which had shown some anti-psoriasis activity and were well tolerated by patients.139,140 Over the past two decades, several clinical trials have focused on evaluating the effectiveness, duration, and safety of occlusive hydrogels combined with topical drugs. Tejas et al.141 conducted a double-blind, single-center controlled trial involving 30 patients, and observed that occlusive hydrogels had an increased therapeutic effect on 0.005% calcipotriene −0.064% betamethasone malonate ointment without adverse effects, including skin irritation. In addition, there are several trials with no published results.
6. Conclusions
In this work, we have comprehensively reviewed all types of biomaterials for topical psoriasis therapy. The significance and challenges of local treatment of psoriasis are firstly discussed. Then biomaterials, including microneedles, nanoparticles, nanofibers and hydrogels, are extensively reviewed for their capacity in psoriasis treatments. The fabrication strategies, and the advantages and disadvantages of these biomaterials in treating psoriasis are particularly discussed. Finally, clinical trials of these biomaterials for psoriasis therapy are summarized. In fact, biomaterial-based strategies for psoriasis treatments are still in the very early stage. More investigations are highly demanded to expedite the clinical translation of biomaterials.
Abbreviation
Th17 | T helper cell 17 |
IL-17A | Interleukin-17A |
IL-17F | Interleukin-17F |
IL-22 | Interleukin-22 |
IL-1β | Interleukin-1β |
IL-6 | Interleukin-6 |
TNF-α | Tumor necrosis factor-α |
CXCL8 | CXC chemokine ligand 8 |
CXCL9 | CXC chemokine ligand 9 |
CXCL10 | CXC chemokine ligand 10 |
SC | Stratum corneum |
CTP | Collagen tripeptide |
MTX | Methotrexate |
PTX | Pentoxifylline |
IL-2 | Interleukin-2 |
FDA | The US food and drug administration |
IL-23 | Interleukin-23 |
TEWL | Transepidermal water loss |
MNs | Microneedles |
SMNs | Solid microneedles |
CMNs | Coating microneedles |
DMNs | Dissolvable microneedles |
HMNs | Hollow microneedles |
MEMS | Micro-electromechanical systems |
HA | Hyaluronic acid |
PVP | Polyvinylpyrrolidone |
IMQ | Imiquimod |
ISDs | Inflammatory skin diseases |
RNP | Ribonucleoprotein |
Dex | Dexamethasone |
H&E | Hematoxylin & eosin |
SEM | Scanning electron microscopy |
NPs | Nanoparticles |
ECM | Extracellular matrix |
CH | Cholesterol |
FFAs | Free fatty acids |
SLNs | Solid lipid nanoparticles |
NLCs | Nanostructured lipid carriers |
PC | Phosphatidylcholine |
OA | Oleic acid |
DCs | Dendritic cells |
Tm | Transition temperature |
AuNPs | Gold nanoparticles |
PLGA | Poly(lactide-co-glycolic acid) |
PDMA | Poly(2-(diethylamino)ethyl methacrylate) |
NFs | Nanofibers |
CNF | Cellulose nanofiber |
BC/CMC | Bacterial cellulose/carboxymethylcellulose |
PAN | Polyacrylonitrile |
HCD | Hydrocolloid occlusive dressing |
HPMC | Hydroxy propyl methyl cellulose |
Conflicts of interest
The authors declare no conflict of interest.
Acknowledgements
The authors would like to acknowledge the financial support from the National Natural Science Foundation of China (82172226), the Natural Science Foundation of Zhejiang Province (LY21H180005) and the Wenzhou Municipal Science & Technology Bureau of China (ZY2021020).
References
- Y. Liu, J. G. Krueger and A. M. Bowcock, Genes Immun., 2007, 8, 1–12 CrossRef CAS PubMed.
- H. Valdimarsson, R. H. Thorleifsdottir, S. L. Sigurdardottir, J. E. Gudjonsson and A. Johnston, Trends Immunol., 2009, 30, 494–501 CrossRef CAS PubMed.
- A. M. Bowcock, Annu. Rev. Genomics Hum. Genet., 2005, 6, 93–122 CrossRef CAS PubMed.
- K. G. Linden and G. D. Weinstein, Am. J. Med., 1999, 107, 595–605 CrossRef CAS.
- M. Furue and T. Kadono, Innate Immun., 2019, 25, 337–343 CrossRef CAS PubMed.
- Y. Liang, M. K. Sarkar, L. C. Tsoi and J. E. Gudjonsson, Curr. Opin. Immunol., 2017, 49, 1–8 CrossRef CAS.
- Z. Guo, Y. Yang, Y. Liao, Y. Shi and L.-j Zhang, JID Innov., 2022, 2, 100064 CrossRef PubMed.
- A. W. Armstrong and C. Read, JAMA, 2020, 323, 1945–1960 CrossRef CAS PubMed.
- W. H. Boehncke and M. P. Schon, Lancet, 2015, 386, 983–994 CrossRef CAS.
- H. Valdimarsson, B. S. Bake, I. Jonsdotdr and L. Fry, Immunol. Today, 1986, 7, 256–259 CrossRef CAS.
- H. M. Wang, C. Wu, Y. Y. Jiang, W. M. Wang and H. Z. Jin, Nutr. Metab., 2020, 17, 5 CrossRef CAS PubMed.
- M. Ohtsuki, Y. Okubo, M. Komine, S. Imafuku, R. M. Day, P. Chen, R. Petric, A. Maroli and O. Nemoto, J. Dermatol., 2017, 44, 873–884 CrossRef CAS PubMed.
- A. Rendon and K. Schäkel, Int. J. Mol. Sci., 2019, 20, 1475 CrossRef CAS PubMed.
- J. Hughes and M. Rustin, Clin. Dermatol., 1997, 15, 715–721 CrossRef CAS.
- D. D. Gladman, Lancet, 2017, 389, 482–483 CrossRef.
- L. Guenther, C. Lynde and Y. Poulin, J. Cutaneous Med. Surg., 2019, 23, 27S–34S CrossRef CAS PubMed.
- S. Meng, L. Sun, L. Wang, Z. Lin, Z. Liu, L. Xi, Z. Wang and Y. Zheng, Colloids Surf., B, 2019, 182, 110352 CrossRef CAS PubMed.
- T. Wong, L. Hsu and W. Liao, J. Cutaneous Med. Surg., 2013, 17, 6–12 CrossRef CAS PubMed.
- L.-j Zhang, Exp. Dermatol., 2021, 30, 756–764 CrossRef PubMed.
- P. Tristani-Firouzi and G. G. Krueger, Cutis, 1998, 61, 11–21 CAS.
- A. Menter, N. J. Korman, C. A. Elmets, S. R. Feldman, J. M. Gelfand, K. B. Gordon, A. Gottlieb, J. Y. Koo, M. Lebwohl, H. W. Lim, A. S. Van Voorhees, K. R. Beutner, R. Bhushan and American Academy of Dermatology, J. Am. Acad. Dermatol., 2009, 60, 643–659 CrossRef.
- W. H. Boehncke, S. Boehncke, A. M. Tobin and B. Kirby, Exp. Dermatol., 2011, 20, 303–307 CrossRef PubMed.
- S. Boehncke, D. Thaci, H. Beschmann, R. J. Ludwig, H. Ackermann, K. Badenhoop and W. H. Boehncke, Br. J. Dermatol., 2007, 157, 1249–1251 CrossRef CAS PubMed.
- A. W. Armstrong, S. V. Voyles, E. J. Armstrong, E. N. Fuller and J. C. Rutledge, Exp. Dermatol., 2011, 20, 544–549 CrossRef CAS PubMed.
- T. Yazdanparast, K. Yazdani, P. Humbert, A. Khatami, S. Ahmad Nasrollahi, H. Hassanzadeh, A. H. Ehsani, L. Izadi Firouzabadi and A. Firooz, Med. J. Islam. Repub. Iran, 2018, 32, 108 Search PubMed.
- H. Dobrev, Acta Derm.-Venereol., 2000, 80, 263–266 CrossRef CAS.
- M. Alper, A. Kavak, A. H. Parlak, R. Demirci, I. Belenli and N. Yesildal, Braz. J. Med. Biol. Res., 2004, 37, 111–117 CrossRef CAS PubMed.
- H. Du, P. Liu, J. Zhu, J. Lan, Y. Li, L. Zhang, J. Zhu and J. Tao, ACS Appl. Mater. Interfaces, 2019, 11, 43588–43598 CrossRef CAS PubMed.
- E. Korkmaz, E. E. Friedrich, M. H. Ramadan, G. Erdos, A. R. Mathers, O. Burak Ozdoganlar, N. R. Washburn and L. D. Falo, Jr., Acta Biomater., 2015, 24, 96–105 CrossRef CAS PubMed.
- T. Wan, Q. Pan and Y. Ping, Sci. Adv., 2021, 7, 33692106 Search PubMed.
- M. Ferreira, L. Barreiros, M. A. Segundo, T. Torres, M. Selores, S. A. Costa Lima and S. Reis, Colloids Surf., B, 2017, 159, 23–29 CrossRef CAS PubMed.
- R. Arora, S. S. Katiyar, V. Kushwah and S. Jain, Expert Opin. Drug Delivery, 2017, 14, 165–177 CrossRef CAS PubMed.
- V. M. Ghate, A. K. Kodoth, A. Shah, B. Vishalakshi and S. A. Lewis, Colloids Surf., B, 2019, 181, 389–399 CrossRef CAS PubMed.
- M. Bahramizadeh, M. Bahramizadeh, B. Kiafar, A. H. Jafarian, A. R. Nikpoor, M. Hatamipour, H. Esmaily, Z. Rezaeemehr, S. Golmohammadzadeh, S. A. Moosavian and M. R. Jafari, Int. J. Pharm., 2019, 569, 118623 CrossRef CAS PubMed.
- Y. Zhang, Q. Xia, Y. Li, Z. He, Z. Li, T. Guo, Z. Wu and N. Feng, Theranostics, 2019, 9, 48–64 CrossRef CAS PubMed.
- A. Özcan, D. Sahin, D. Impellizzieri, T. T. Nguyen, J. Hafner, N. Yawalkar, D. Kurzbach, G. Tan, C. A. Akdis, J. Nilsson, O. Boyman and A. G. A. Kolios, J. Invest. Dermatol., 2020, 140, 1003–1014.e1008 CrossRef PubMed.
- C. Gomez, C. Muangnoi, F. N. Sorasitthiyanukarn, J. Wongpiyabovorn, P. Rojsitthisak and P. Rojsitthisak, Molecules, 2019, 24, 1388 CrossRef CAS PubMed.
- L. Sun, Z. Liu, L. Wang, D. Cun, H. H. Y. Tong, R. Yan, X. Chen, R. Wang and Y. Zheng, J. Controlled Release, 2017, 254, 44–54 CrossRef CAS PubMed.
- K. L. Mao, Z. L. Fan, J. D. Yuan, P. P. Chen, J. J. Yang, J. Xu, D. L. ZhuGe, B. H. Jin, Q. Y. Zhu, B. X. Shen, Y. Sohawon, Y. Z. Zhao and H. L. Xu, Colloids Surf., B, 2017, 160, 704–714 CrossRef CAS PubMed.
- N. W. Kang, M. H. Kim, S. Y. Sohn, K. T. Kim, J. H. Park, S. Y. Lee, J. Y. Lee and D. D. Kim, Biomaterials, 2018, 182, 245–258 CrossRef CAS.
- J. Xu, H. Chen, Z. Chu, Z. Li, B. Chen, J. Sun, W. Lai, Y. Ma, Y. He, H. Qian, F. Wang and Y. Xu, J. Nanobiotechnol., 2022, 20, 155 CrossRef CAS.
- D. Yang, M. Chen, Y. Sun, Y. Jin, C. Lu, X. Pan, G. Quan and C. Wu, Acta Biomater., 2021, 121, 119–133 CrossRef CAS PubMed.
- A. H. Sabri, Y. Kim, M. Marlow, D. J. Scurr, J. Segal, A. K. Banga, L. Kagan and J. B. Lee, Adv. Drug Delivery Rev., 2020, 153, 195–215 CrossRef CAS.
- Y. Ye, J. Yu, D. Wen, A. R. Kahkoska and Z. Gu, Adv. Drug Delivery Rev., 2018, 127, 106–118 CrossRef CAS.
- Y. C. Kim, J. H. Park and M. R. Prausnitz, Adv. Drug Delivery Rev., 2012, 64, 1547–1568 CrossRef CAS PubMed.
- S. Kim, H. Yang, J. Eum, Y. Ma, S. Fakhraei Lahiji and H. Jung, Biomaterials, 2020, 232, 119733 CrossRef CAS PubMed.
- C. P. P. Pere, S. N. Economidou, G. Lall, C. Ziraud, J. S. Boateng, B. D. Alexander, D. A. Lamprou and D. Douroumis, Int. J. Pharm., 2018, 544, 425–432 CrossRef CAS PubMed.
- Y. Zhang, G. Jiang, W. Yu, D. Liu and B. Xu, Mater. Sci. Eng., C, 2018, 85, 18–26 CrossRef CAS PubMed.
- Y. K. Demir, Z. Akan and O. Kerimoglu, PLoS One, 2013, 8, e63819 CrossRef CAS.
- H. Vallhov, W. Xia, H. Engqvist and A. Scheynius, J. Mater. Chem. B, 2018, 6, 6808–6816 RSC.
- M. R. Prausnitz and R. Langer, Nat. Biotechnol., 2008, 26, 1261–1268 CrossRef CAS PubMed.
- E. Larrañeta, R. E. M. Lutton, A. D. Woolfson and R. F. Donnelly, Mater. Sci. Eng., R, 2016, 104, 1–32 CrossRef.
- R. S. J. Ingrole and H. S. Gill, J. Pharmacol. Exp. Ther., 2019, 370, 555 CrossRef CAS PubMed.
- L. Zhang, R. Guo, S. Wang, X. Yang, G. Ling and P. Zhang, Int. J. Pharm., 2021, 604, 120749 CrossRef CAS PubMed.
- Á. Cárcamo-Martínez, B. Mallon, J. Domínguez-Robles, L. K. Vora, Q. K. Anjani and R. F. Donnelly, Int. J. Pharm., 2021, 599, 120455 CrossRef PubMed.
- Z. Zhao, Y. Chen and Y. Shi, RSC Adv., 2020, 10, 14040–14049 RSC.
- W. Martanto, S. P. Davis, N. R. Holiday, J. Wang, H. S. Gill and M. R. Prausnitz, Pharm. Res., 2004, 21, 947–952 CrossRef CAS PubMed.
- G. Yan, K. S. Warner, J. Zhang, S. Sharma and B. K. Gale, Int. J. Pharm., 2010, 391, 7–12 CrossRef CAS PubMed.
- S. H. Shravanth, R. A. M. Osmani, S. L. Jyothi, V. P. Anupama, M. Rahamathulla and H. V. Gangadharappa, J. Drug Delivery Sci. Technol., 2021, 64, 102668 CrossRef CAS.
- S. Zhang, Y. Qiu and Y. Gao, Acta Pharm. Sin. B, 2014, 4, 100–104 CrossRef PubMed.
- J. H. Jung and S. G. Jin, J. Pharm. Invest., 2021, 51, 503–517 CrossRef PubMed.
- K. van der Maaden, W. Jiskoot and J. Bouwstra, J. Controlled Release, 2012, 161, 645–655 CrossRef CAS PubMed.
- M. Jang, S. Baek, G. Kang, H. Yang, S. Kim and H. Jung, Int. J. Cosmet. Sci., 2020, 42, 302–309 CrossRef CAS.
- H. Du, P. Liu, J. Zhu, J. Lan, Y. Li, L. Zhang, J. Zhu and J. Tao, ACS Appl. Mater. Interfaces, 2019, 11, 43588–43598 CrossRef CAS PubMed.
- C. Dillon, H. Hughes, N. J. O'Reilly, C. J. Allender, D. A. Barrow and P. McLoughlin, Int. J. Pharm., 2019, 565, 9–19 CrossRef CAS PubMed.
- P. Ronnander, L. Simon, H. Spilgies, A. Koch and S. Scherr, Eur. J. Pharm. Sci., 2018, 114, 84–92 CrossRef CAS PubMed.
- M. Leone, J. Mönkäre, J. A. Bouwstra and G. Kersten, Pharm. Res., 2017, 34, 2223–2240 CrossRef CAS PubMed.
- T. Waghule, G. Singhvi, S. K. Dubey, M. M. Pandey, G. Gupta, M. Singh and K. Dua, Biomed. Pharmacother., 2019, 109, 1249–1258 CrossRef CAS PubMed.
- J. H. Lee, Y. S. Jung, G. M. Kim and J. M. Bae, Br. J. Dermatol., 2018, 178, e24–e25 CAS.
- T. Wan, Q. Pan and Y. Ping, Sci. Adv., 2021, 7, eabe2888 CrossRef CAS PubMed.
- X. Zhang, F. Wang, Y. Yu, G. Chen, L. Shang, L. Sun and Y. Zhao, Sci. Bull., 2019, 64, 1110–1117 CrossRef CAS.
- P. Carter, B. Narasimhan and Q. Wang, Int. J. Pharm., 2019, 555, 49–62 CrossRef CAS PubMed.
- M. A. Lampe, M. L. Williams and P. M. Elias, J. Lipid Res., 1983, 24, 131–140 CrossRef CAS.
- S. Mukherjee, S. Ray and R. S. Thakur, Indian J. Pharm. Sci., 2009, 71, 349–358 CrossRef CAS PubMed.
- M. Ferreira, L. Barreiros, M. A. Segundo, T. Torres, M. Selores, S. A. Costa Lima and S. Reis, Colloids Surf., B, 2017, 159, 23–29 CrossRef CAS PubMed.
- R. Arora, S. S. Katiyar, V. Kushwah and S. Jain, Expert Opin. Drug Delivery, 2017, 14, 165–177 CrossRef CAS PubMed.
- R. Sonawane, H. Harde, M. Katariya, S. Agrawal and S. Jain, Expert Opin. Drug Delivery, 2014, 11, 1833–1847 CrossRef CAS PubMed.
- U. Agrawal, M. Gupta and S. P. Vyas, Artif. Cells Nanomed. Biotechnol., 2015, 43, 33–39 CrossRef CAS PubMed.
- A. J. Almeida and E. Souto, Adv. Drug Delivery Rev., 2007, 59, 478–490 CrossRef CAS PubMed.
- F. Q. Hu, S. P. Jiang, Y. Z. Du, H. Yuan, Y. Q. Ye and S. Zeng, Colloids Surf., B, 2005, 45, 167–173 CrossRef CAS.
- C. L. Fang, S. A. Al-Suwayeh and J. Y. Fang, Recent Pat. Nanotechnol., 2013, 7, 41–55 CrossRef CAS.
- M. F. Pinto, C. C. Moura, C. Nunes, M. A. Segundo, S. A. Costa Lima and S. Reis, Int. J. Pharm., 2014, 477, 519–526 CrossRef CAS PubMed.
- V. M. Ghate, A. K. Kodoth, A. Shah, B. Vishalakshi and S. A. Lewis, Colloids Surf., B, 2019, 181, 389–399 CrossRef CAS PubMed.
- L. Coderch, J. Fonollosa, M. De Pera, J. Estelrich, A. De La Maza and J. L. Parra, J. Controlled Release, 2000, 68, 85–95 CrossRef CAS PubMed.
- S. Barua and S. Mitragotri, Nano Today, 2014, 9, 223–243 CrossRef CAS PubMed.
- M. Sala, R. Diab, A. Elaissari and H. Fessi, Int. J. Pharm., 2018, 535, 1–17 CrossRef CAS PubMed.
- P. Srisuk, P. Thongnopnua, U. Raktanonchai and S. Kanokpanont, Int. J. Pharm., 2012, 427, 426–434 CrossRef CAS PubMed.
- L. Xi, Z. Lin, F. Qiu, S. Chen, P. Li, X. Chen, Z. Wang and Y. Zheng, Acta Pharm. Sin. B, 2022, 12, 339–352 CrossRef CAS PubMed.
- S. Jain, P. Jain, R. B. Umamaheshwari and N. K. Jain, Drug Dev. Ind. Pharm., 2003, 29, 1013–1026 CrossRef CAS PubMed.
- C. Yang, X. Dai, S. Yang, L. Ma, L. Chen, R. Gao, X. Wu and X. Shi, Colloids Surf., B, 2019, 183, 110462 CrossRef CAS PubMed.
- E. Pena-Rodriguez, M. C. Moreno, B. Blanco-Fernandez, J. Gonzalez and F. Fernandez-Campos, Pharmaceutics, 2020, 12, 112 CrossRef CAS PubMed.
- N. Nainwal, S. Jawla, R. Singh and V. A. Saharan, J. Liposome Res., 2019, 29, 103–113 CrossRef CAS PubMed.
- E. Touitou, N. Dayan, L. Bergelson, B. Godin and M. Eliaz, J. Controlled Release, 2000, 65, 403–418 CrossRef CAS PubMed.
- T. Guo, J. Lu, Y. Fan, Y. Zhang, S. Yin, X. Sha and N. Feng, Int. J. Pharm., 2021, 604, 120762 CrossRef CAS PubMed.
- S. Moghassemi and A. Hadjizadeh, J. Controlled Release, 2014, 185, 22–36 CrossRef CAS PubMed.
- A. A. Abdelbary and M. H. AbouGhaly, Int. J. Pharm., 2015, 485, 235–243 CrossRef CAS PubMed.
- S. R. Moghddam, A. Ahad, M. Aqil, S. S. Imam and Y. Sultana, Mater. Sci. Eng., C, 2016, 69, 789–797 CrossRef CAS PubMed.
- S. Meng, L. Sun, L. Wang, Z. Lin, Z. Liu, L. Xi, Z. Wang and Y. Zheng, Colloids Surf., B, 2019, 182, 110352 CrossRef CAS PubMed.
- H. Bessar, I. Venditti, L. Benassi, C. Vaschieri, P. Azzoni, G. Pellacani, C. Magnoni, E. Botti, V. Casagrande, M. Federici, A. Costanzo, L. Fontana, G. Testa, F. F. Mostafa, S. A. Ibrahim, M. V. Russo and I. Fratoddi, Colloids Surf., B, 2016, 141, 141–147 CrossRef CAS PubMed.
- A. Ozcan, D. Sahin, D. Impellizzieri, T. T. Nguyen, J. Hafner, N. Yawalkar, D. Kurzbach, G. Tan, C. A. Akdis, J. Nilsson, O. Boyman and A. G. A. Kolios, J. Invest. Dermatol., 2019, 140, 1003–1014.e1008 CrossRef PubMed.
- I. Fratoddi, L. Benassi, E. Botti, C. Vaschieri, I. Venditti, H. Bessar, M. A. Samir, P. Azzoni, C. Magnoni, A. Costanzo, V. Casagrande, M. Federici, L. Bianchi and G. Pellacani, Nanomedicine, 2019, 17, 276–286 CrossRef CAS PubMed.
- D. Crisan, K. Scharffetter-Kochanek, M. Crisan, S. Schatz, A. Hainzl, L. Olenic, A. Filip, L. A. Schneider and A. Sindrilaru, Exp. Dermatol., 2018, 27, 1166–1169 CrossRef CAS PubMed.
- M. G. Arafa, G. N. S. Girgis and M. S. El-Dahan, Int. J. Nanomed., 2020, 15, 1335–1347 CrossRef CAS PubMed.
- H. Liang, Y. Yan, J. Wu, X. Ge, L. Wei, L. Liu and Y. Chen, Sci. Adv., 2020, 6, eabb5274 CrossRef CAS PubMed.
- Y. Li, Z. Xiao, Y. Zhou, S. Zheng, Y. An, W. Huang, H. He, Y. Yang, S. Li, Y. Chen, J. Xiao and J. Wu, ACS Appl. Mater. Interfaces, 2019, 11, 28377–28386 CrossRef CAS PubMed.
- C. O. Chantre, P. H. Campbell, H. M. Golecki, A. T. Buganza, A. K. Capulli, L. F. Deravi, S. Dauth, S. P. Sheehy, J. A. Paten, K. Gledhill, Y. S. Doucet, H. E. Abaci, S. Ahn, B. D. Pope, J. W. Ruberti, S. P. Hoerstrup, A. M. Christiano and K. K. Parker, Biomaterials, 2018, 166, 96–108 CrossRef CAS PubMed.
- J. Xie, M. R. Macewan, W. Z. Ray, W. Liu, D. Y. Siewe and Y. Xia, ACS Nano, 2010, 4, 5027–5036 CrossRef CAS PubMed.
- R. Sridhar, R. Lakshminarayanan, K. Madhaiyan, V. Amutha Barathi, K. H. Lim and S. Ramakrishna, Chem. Soc. Rev., 2015, 44, 790–814 RSC.
- A. M. Behrens, B. J. Casey, M. J. Sikorski, K. L. Wu, W. Tutak, A. D. Sandler and P. Kofinas, ACS Macro Lett., 2014, 3, 249–254 CrossRef CAS PubMed.
- L. Ren, R. Ozisik, S. P. Kotha and P. T. Underhill, Macromolecules, 2015, 48, 2593–2602 CrossRef CAS.
- Kenry and C. T. Lim, Prog. Polym. Sci., 2017, 70, 1–17 CrossRef CAS.
- L. S. Shores, S. H. Kelly, K. M. Hainline, J. Suwanpradid and J. H. Collier, Front. Immunol., 2020, 11, 1855 CrossRef CAS PubMed.
- A. Hemati Azandaryani, K. Derakhshandeh and E. Arkan, Int. J. Polym. Mater., 2018, 67, 677–685 CrossRef CAS.
- L. Martinez-Ortega, A. Mira, A. Fernandez-Carvajal, C. R. Mateo, R. Mallavia and A. Falco, Pharmaceutics, 2019, 11, 14 CrossRef CAS PubMed.
- M. D. Fontes, A. B. Meneguin, A. Tercjak, J. Gutierrez, B. S. F. Cury, A. M. dos Santos, S. J. L. Ribeiro and H. S. Barud, Carbohydr. Polym., 2018, 179, 126–134 CrossRef CAS PubMed.
- A. H. Azandaryani, K. Derakhshandeh and E. Arkan, Int. J. Polym. Mater. Polym. Biomater., 2018, 67, 677–685 CrossRef.
- M. Toriello, M. Afsari, H. K. Shon and L. D. Tijing, Membranes, 2020, 10, 204 CrossRef CAS PubMed.
- M. Shinkawa, K. Motai, K. Eguchi, W. Takarada, M. Ashizawa, H. Masunaga, N. Ohta, Y. Hayamizu and H. Matsumoto, Membranes, 2021, 11, 389 CrossRef CAS PubMed.
- X. Zhang and Y. Lu, Polym. Rev., 2014, 54, 677–701 CrossRef CAS.
- V. A. Kornev, E. A. Grebenik, A. B. Solovieva, R. I. Dmitriev and P. S. Timashev, Comput. Struct. Biotechnol. J., 2018, 16, 488–502 CrossRef CAS PubMed.
- D. Gabriel, T. Mugnier, H. Courthion, K. Kranidioti, N. Karagianni, M. C. Denis, M. Lapteva, Y. Kalia, M. Moller and R. Gurny, J. Controlled Release, 2016, 242, 16–24 CrossRef CAS PubMed.
- E. He, H. Li, X. Li, X. Wu, K. Lei and Y. Diao, Int. J. Mol. Sci., 2022, 23, 3798 CrossRef CAS PubMed.
- A. M. Fernandez-Romero, F. Maestrelli, S. Garcia-Gil, E. Talero, P. Mura, A. M. Rabasco and M. L. Gonzalez-Rodriguez, Int. J. Mol. Sci., 2021, 22, 13566 CrossRef CAS PubMed.
- R. Oliveira, D. Silva, S. Mota, J. Garrido, E. Garrido, J. Lobo and I. Almeida, Appl. Sci., 2022, 12, 3260 CrossRef CAS.
- K. Rana, T. Pani, S. K. Jha, D. Mehta, P. Yadav, D. Jain, M. K. Pradhan, S. Mishra, R. Kar, B. R. G, A. Srivastava, U. Dasgupta, V. S. Patil and A. Bajaj, Nanoscale, 2022, 14, 3834–3848 RSC.
- M. S. Freag, A. S. Torky, M. M. Nasra, D. A. Abdelmonsif and O. Y. Abdallah, Nanomedicine, 2019, 14, 931–954 CrossRef CAS PubMed.
- J. R. Madan, S. Khobaragade, K. Dua and R. Awasthi, Dermatol. Ther., 2020, 33, e13370 CrossRef CAS PubMed.
- P. Tripathi, A. Kumar, P. K. Jain and J. R. Patel, Int. J. Biol. Macromol., 2018, 120, 1322–1334 CrossRef CAS PubMed.
- M. I. Asad, D. Khan, A. U. Rehman, A. Elaissari and N. Ahmed, Nanomaterials, 2021, 11, 3433 CrossRef CAS PubMed.
- Q. Li, F. Li, X. Qi, F. Wei, H. Chen and T. Wang, Colloids Surf., B, 2020, 195, 111246 CrossRef CAS PubMed.
- S. Doppalapudi, A. Jain, D. K. Chopra and W. Khan, Eur. J. Pharm. Sci., 2017, 96, 515–529 CrossRef CAS PubMed.
- M. Walunj, S. Doppalapudi, U. Bulbake and W. Khan, J. Liposome Res., 2020, 30, 68–79 CrossRef CAS PubMed.
- K. L. Mao, Z. L. Fan, J. D. Yuan, P. P. Chen, J. J. Yang, J. Xu, D. L. ZhuGe, B. H. Jin, Q. Y. Zhu, B. X. Shen, Y. Sohawon, Y. Z. Zhao and H. L. Xu, Colloids Surf., B, 2017, 160, 704–714 CrossRef CAS PubMed.
- J. H. Lee, Y. S. Jung, G. M. Kim and J. M. Bae, Br. J. Dermatol., 2018, 178, e24–e25 CAS.
- Y. W. Yew, C. Z. Y. Phuan, X. Zhao, E. S. T. Tan, W. S. Chong and H. L. Tey, Ann. Acad. Med. Singapore, 2022, 51, 16–23 Search PubMed.
- M. Nasr, S. Abdel-Hamid, N. H. Moftah, M. Fadel and A. A. Alyoussef, Curr. Drug Delivery, 2017, 14, 426–432 CrossRef CAS PubMed.
- R. I. El-Gogary, M. H. Ragai, N. Moftah and M. Nasr, Expert Opin. Drug Delivery, 2021, 18, 1523–1532 CrossRef CAS PubMed.
- A. Ormerod, A. Winfield, A. Priprem, R. Moody, N. Graham and C. Moran, J. Dermatol. Treat., 1992, 2, 133–136 CrossRef PubMed.
- B. Kumar, K. Sandhu and I. Kaur, J. Dermatol., 2004, 31, 798–801 CrossRef CAS PubMed.
- M. F. Ali, M. Salah, M. Rafea and N. Saleh, Med. Sci. Monit., 2008, 14, Pi66–Pi74 Search PubMed.
- T. Patel, T. Bhutani, K. L. Busse and J. Koo, Cutis, 2011, 88, 149–154 Search PubMed.
Footnote |
† These authors contributed equally to this work. |
|
This journal is © The Royal Society of Chemistry 2022 |
Click here to see how this site uses Cookies. View our privacy policy here.