Determination of the isotopic composition of copper in a certified reference material HICU-1 by MC-ICP-MS using gravimetric isotope mixture calibration†
Received
28th June 2023
, Accepted 15th August 2023
First published on 21st August 2023
Abstract
Isotopic analysis by mass spectrometry relies on certified reference materials (CRMs) in order to correct instrumental isotopic fractionation (aka mass bias). Currently, SRM976 copper isotopic standard from the National Institute of Standards and Technology (NIST) remains the sole internationally recognized reference material for copper isotope ratio. However, the SRM976 material is no longer available and new copper isotopic CRMs are needed to serve as reference points for decades to come. In this study, we report an independent and fully calibrated isotope ratio measurement of a high-purity copper certified reference material, HICU-1, at the National Research Council Canada (NRC). MC-ICP-MS was employed for the isotope ratio measurements which were calibrated using the gravimetric isotope mixture approach involving two near-pure enriched copper isotopes. Copper isotope ratio n(63Cu)/n(65Cu) = 2.2413 ± 0.0009 (95% CI, k = 2) was established in the NRC HICU-1 and its isotopic composition has been shown to be nearly identical to that of NIST SRM976.
1. Introduction
High-precision isotopic analysis of copper plays an important role in the study of various geological, biological and chemical processes1–5 with applications to geoscience,6,7 environmental3,8 and medical9,10 sciences. Copper isotope ratio measurements are typically performed using either thermal ionization mass spectrometry (TIMS) or multiple collector-inductively coupled plasma mass spectrometer (MC-ICP-MS). Copper is recognized as an analytically unfavorable element to be measured by conventional TIMS because of its poor ionization efficiency as a result of its high first ionization potential.11 The commercialization of MC-ICP-MS in the early 1990s has made high-precision measurements of Cu isotope ratios more efficient due to its simple sample introduction system, high ionization efficiency and sensitivity.12 However, MC-ICP-MS exhibits significantly larger instrumental isotopic fractionation (mass bias) than TIMS, and proper corrections are crucial. To achieve this correction, one typically relies on isotopic CRMs.
IUPAC recognizes13 the best measurement of copper isotopic composition which was based on TIMS measurement from the 1960s14 associated with a certified reference material SRM976 produced by the National Institute of Standards and Technology (NIST). SRM976 is no longer available and new independent CRMs are therefore needed. In this vein, a new CRM (GBW04624) has been produced by the National Institute of Metrology China15 based on the use of enriched Cu isotopes for calibration. Meanwhile, the National Research Council Canada (NRC) has also produced a candidate Cu isotopic CRM (HICU-1).16 The aim of this study is to document the development of a new primary realization of the isotopic composition of copper independent of SRM976.
2. Experimental section
2.1. Instrumentation
A Neptune Plus (Thermo Fisher Scientific, Bremen, Germany) MC-ICP-MS was used for Cu isotope ratio measurements, and was operated in a low mass resolution mode. MC-ICP-MS is equipped with nine Faraday cups, a quartz dual cyclonic spray chamber and a PFA self-aspirating nebulizer (Elemental Scientific, Omaha, NE, USA) at a flow rate of 50 μL min−1. The instrument was tuned daily for high sensitivity while maintaining flat-top square peak and stable signal. The gain calibration of the Faraday cups was performed weekly to ensure the normalization of their efficiencies. Rotating amplifiers was chosen during the measurements, which effectively cancels out all amplifier calibration biases and improves measurement precision. Typical operating conditions are presented in Table 1.
Table 1 MC-ICP-MS operating conditions
Instrument settings |
Radio-frequency power |
1180 W |
Plasma gas flow rate |
16.0 L min−1 |
Auxiliary gas flow rate |
1.00 L min−1 |
Sample gas flow rate |
1.010 L min−1 |
Sampler cone orifice (H, Ni) |
1.1 mm |
Skimmer cone orifice (Ni) |
0.8 mm |
Lens settings |
Optimized for high and stable analyte signal while maintaining a flat top peak |
![[thin space (1/6-em)]](https://www.rsc.org/images/entities/char_2009.gif) |
Data acquisition parameters
|
Faraday cup configuration |
C (63Cu), H1 (65Cu); or L3 (63Cu), L2 (65Cu), C (67Zn), H2 (69Ga), H3 (71Ga) |
Mass resolution |
Low |
Signal integration time |
2.097 s |
Numbers of integrations, cycles, and blocks |
1, 7, 8; or 1, 5, 10 |
2.2. Reagents and solutions
High-purity nitric acid was obtained by a sub-boiling distillation system (Milestone Inc., Shelton, CT, USA) of reagent grade feedstocks (Fisher Scientific, Ottawa, ON, Canada). The deionized water (DIW, 18.5 MΩ cm) was obtained from a Milli-Q ion exchange system (Sigma-Aldrich, Oakville, ON, Canada). All labware was soaked in 5% HNO3 solution for a couple of days and then rinsed with DIW, then dried in a class-10 fume hood prior to use.
Near-pure separated copper isotopes in a metallic form (99.8% 63Cu and 99.7% 65Cu, designated as materials A and B, respectively) were obtained from Trace Sciences International (Richmond Hill, Ontario, Canada). Chemical purity of copper in both isotopically enriched materials was determined by glow discharge mass spectrometry (GDMS) at NRC. Afterwards, these two near-pure copper metals were cleaned with 10% HNO3, rinsed with DIW water, and dried in a class-10 fume hood before their quantitative dissolution in a few mL of high purity HNO3. Gravimetric dilution was performed to yield mass fractions of w(Cu, A) = 2061.98 mg kg−1 and w(Cu, B) = 1947.72 mg kg−1. Working solutions of copper enriched isotope materials of w(Cu, A1) = 3.9859 mg kg−1, w(Cu, A2) = 4.0012 mg kg−1, w(Cu, B1) = 3.9973 mg kg−1, and w(Cu, B2) = 3.9985 mg kg−1, were gravimetrically prepared by serial dilution of their stock solutions in 2% HNO3, respectively.
Gallium metal isotopic reference material, SRM994 was purchased from National Institute of Standards and Technology (Gaithersburg MD, USA). A 1500 μg g−1 stock solution of SRM994 was prepared by quantitative dissolution of Ga in warm concentrated nitric acid and then diluted with DIW. A 3200 μg g−1 stock solution of NIST SRM976 Cu isotopic material was prepared in 5% HNO3, which is used as delta zero material. NIST SRM994 Ga was added to the individual HICU-1 copper solutions and the bracketing standard (SRM976) as the internal standard for the evaluation of homogeneity of HICU-1.16 Note that other internal standards such as Ni isotopes can also be used, as Ni isotopes are closer in mass to the Cu isotopes. Because the major isotope 58Ni has an interference of 58Fe, therefore, 60Ni (26.2%) and the minor isotope of 62Ni (3.63%) are normally used. However, the isotope ratio measurement involving a minor isotope generally produce poorer precision as compared to that of more abundant Ga isotopes (60.1% for 69Ga and 39.7% for 71Ga). Thus, Ga was selected as the internal standard in the study.
2.3. Sample preparation and analysis using gravimetric mixture model
Twelve individual stock solutions (labelled Cu1 to Cu12) of HICU-1 were prepared from 12 units of HICU-1 metal rods. The copper rods, each weighing approx. 0.9 g, were cleaned with 10% HNO3 for 5 min, rinsed with DIW and dried with argon. The cleaned copper pins were weighed, individually dissolved in a few mL HNO3 with mild heating, and diluted with deionized water to result in a nominal mass fraction w(Cu) = 8000 mg kg−1. A mixed stock solution (labelled Cumix) was prepared by blending equal aliquots (2 g each) of Cu1 to Cu12 solutions and this solution was used to establish the isotopic composition of HICU-1.
Gravimetric mixtures of materials A and B were prepared by weighing suitable amounts of two sets of solutions containing isotopically enriched copper isotopes A and B. These four solutions are labeled as A1, B1 and A2, B2. The mass fraction of copper in these solutions is as follows:
w(Cu, A1) = 3.9869 mg kg−1, w(Cu, B1) = 3.9977 mg kg−1 |
w(Cu, A2) = 4.0022 mg kg−1, w(Cu, B2) = 3.9989 mg kg−1 |
The resulting mixtures were then diluted with 2% HNO3 to result in a 1.0 mg kg−1 mass fraction of copper. The details are shown in Table 2.
Table 2 Sample preparation using the FGIM mass bias correction model
Set |
Sample ID |
m
A1, g |
m
B1, g |
1 |
A1 |
10.10710 |
|
1 |
B1 |
|
10.14880 |
1 |
AB1-1 |
5.12905 |
5.07330 |
1 |
AB1-b |
5.11060 |
4.98220 |
1 |
AB1-2 |
6.18660 |
4.07940 |
1 |
AB1-3 |
7.01885 |
3.29405 |
1 |
AB1-4 |
8.42985 |
1.75250 |
1 |
AB1-5 |
9.21475 |
1.00635 |
1 |
AB1-6 |
9.94550 |
0.19860 |
1 |
AB1-7 |
4.30325 |
5.69960 |
1 |
AB1-8 |
3.39135 |
6.75905 |
1 |
AB1-9 |
1.73930 |
8.19240 |
1 |
AB1-10 |
0.99895 |
9.26450 |
1 |
AB1-11 |
0.18925 |
9.68420 |
Set |
Sample ID |
m
A2, g |
m
B2, g |
2 |
A2 |
10.03050 |
|
2 |
B2 |
|
10.11650 |
2 |
AB2-1 |
4.98080 |
5.04970 |
2 |
AB2-2 |
5.02330 |
5.05020 |
2 |
AB2-3 |
5.02230 |
5.03380 |
2 |
AB2-4 |
7.00240 |
3.02970 |
2 |
AB2-5 |
7.04850 |
3.04420 |
2 |
AB2-6 |
7.05180 |
3.02980 |
Sample solutions of HICU-1 were prepared by dilution of the working sample solution HICU-1mixw(Cu, HICU-1mix) = 4.00 mg kg−1 in 2% HNO3 to result in a 1.0 mg kg−1 mass fraction of copper. 2% HNO3 was used as a blank. These two sets of test solutions and the sample solutions of HICU-1mix were analyzed by MC-ICP-MS in the following measurement sequences:
The underlined materials serve to monitor the drift during the measurements. In all cases, the maximum observed drift of isotope ratios during a measurement sequence was approx. 3 parts per thousand, and was corrected using polynomial splines.
The two measurement sequences were repeated nine times in total using two sets of standard solutions (Table 1). Since the measurements of pure copper isotopes were involved, a 90 second wash with 2% HNO3 between each solution was employed to efficiently reduce the carryover.
Intensities of copper isotopes obtained from a blank solution of 2% HNO3 were subtracted from all analytical signals. Efforts were also made to match the total mass fraction of copper and the sample matrix (nitric acid levels) across all solutions. To ensure stable signals, the instrument was conditioned for at least 1 h prior to each measurement sequence.
2.4. Sample preparation and analysis for homogeneity test
To assess the homogeneity of the copper isotope ratios in HICU-1, twelve individual units of HICU-1 were randomly selected. Subsequently, twelve individual solutions (Cu1 to Cu12) at w(Cu) = 0.8 mg kg−1 were prepared by diluting their respective stocks in 2% HNO3, followed by spiking with the stock solution of the gallium isotopic standard to yield mass fraction of 1.0 mg kg−1 for gallium. Relative copper isotope ratios (isotope deltas) of the twelve copper standard solutions (Cu1 to Cu12) were then measured against the HICU-1mix solution which was prepared by mixing equal amounts of these twelve solutions. HICU-1mix was also spiked with gallium and we used the combined standard-sample bracketing and internal normalization method (C-SSBIN) to achieve high-precision isotope delta measurements.16 Note that HICU-1 is made from high-purity copper wire and is stored under argon gas. Potential instabilities on the isotopic composition of copper due to long-term storage and transport were therefore deemed to be negligible.
2.5. Possible spectral interferences
The isotopically enriched copper materials and the HICU-1 constitute high-purity copper metals. As such, the potential spectral interferences of 23Na40Ar+, 23Na216O1H, 23Na218O1H+, 47Ti16O+, 46Ti16O1H +, 49Ti16O+, 48Ti16O1H+, 126Te++, 130Te++ and 130Ba++ on 63Cu and 65Cu isotopes from matrix elements are negligible, since Ti, Na and Te contents are less than 200 ng g−1 in the solid materials (Table S1†), which do not affect Cu isotope ratios at parts-per-thousand level of precision.
3. Results and discussion
3.1. Chemical purity of enriched copper isotopes
Crucial to the isotope ratio measurements using the FGIM model is the chemical purity of the near-pure isotopes used. Efforts were made to procure high-purity copper isotopes which were produced in a similar fashion. The chemical purity of both enriched copper materials was determined using high resolution Glow Discharge Mass Spectrometry (GDMS) at the NRC. GDMS is an ultra-sensitive method is capable of interrogation solids directly and able to provide SI traceable impurity determination in metals.17,18 Elemental impurities in the enriched coper materials A (63Cu), B (65Cu) and HICU-1 (ref. 19) are presented in Table S1 (ESI†). Iron and lead were found to constitute the majority of impurities (see Table S1†) in these enriched materials. Purity values of w(Cu, A) = 0.999 76(19) kg kg−1 (uc, k = 1) and w(Cu, B) = 0.999 90(5) kg kg−1 (uc, k = 1) were obtained for both enriched materials from the analysis of trace element levels.
3.2. Isotope ratio correction factor
Traditional implementation of the gravimetric mixture method requires, at minimum, measurements of all (N − 1) isotope amount ratios in all N pure materials and in (N − 1) independent gravimetric mixtures of any two enriched materials.12,20 Since copper has only two stable isotopes, it suffices to measure the isotope ratio (r63/65) in the two enriched isotope materials (A and B) and a single gravimetric mixture of the two (AB) in order to derive the isotope ratio correction factor K63/65: |  | (1) |
Here, m63 and m65 are nuclide masses of two isotopes of copper; mA and mB are the masses of enriched materials of A and B used to prepare the mixture solution AB. From the isotope ratio correction factor, the mass bias corrected isotope ratio R63/65 in the sample (HICU-1) can be obtained using the following equation: | RHICU-1,63/65 = K63/65rHICU-1,63/65 | (2) |
Although a single mixture of AB is needed to derive the K63/65, multiple mixtures of AB (Table 2), with isotope ratios r63/65 ranging from 0.02 to 45, were prepared and characterized in order to investigate the stability of K63/65 correction factor estimates over this isotope ratio interval. While the isotope ratio correction factors (K63/65) vary between the measurement sequences, Fig. 1 shows that the estimates of K63/65 remain largely constant in the r63/65 isotope ratio interval from approx. 0.8 to 2. Nevertheless, the estimates of K63/65 exhibit a small, yet persistent, trend with r63/65.
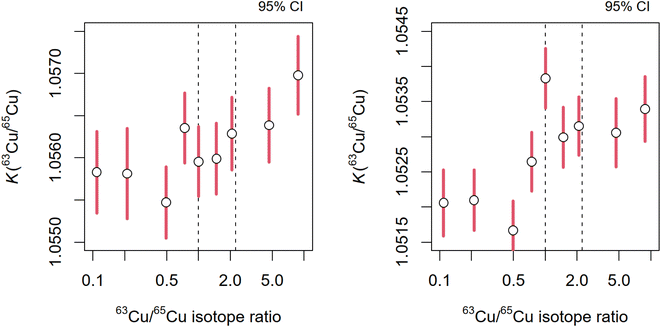 |
| Fig. 1 The estimates of instrumental isotope ratio correction factors (K63/65) from two measurement sequences (set 1) depending on the isotope ratio of copper (r63/65) in the gravimetric mixtures of materials A and B (Table 1). Two dotted vertical lines correspond to r63/65 = 1 : 1 (best measurements) and r63/65 = 2.24 (natural copper). | |
The dependency of K63/65 on r63/65, however weak, creates a conceptual problem on how to select the appropriate K63/65 value to be applied to natural copper samples. In 1994, Henrion introduced the concept of exact matching isotope ratio to 1
:
1 to reduce potential biases in isotope dilution results.21 Similar effects have also been noted in more complex isotope dilution models.22 Taken together, these observations suggest that gravimetric mixture model might also exhibit a more robust performance when only isotope mixtures with 1
:
1 isotope ratios are employed.23 Although we find no significant difference in the estimates of K63/65 from AB mixtures with r63/65 = 1 and r63/65 = 2.24 (natural copper), nevertheless, we rely on AB mixtures having isotopic composition r63/65 ≈ 1.00 ± 0.05 (AB1-1, AB1-2, and AB2-1, AB2-2, AB2-3; see Table 2) to derive the K63/65 for isotope ratio of copper in HICU-1.
3.3. Copper isotope ratio in HICU-1
Copper isotope ratio in HICU-1, R63/65(HICU-1), is calculated using eqn (1) and (2) from the measurements of two sets performed over the course of four months. Fig. 2 shows the results from individual measurement sequences when 1
:
1 mixtures were utilized for calibration. It is evident that the estimates of R63/65 remain constant to within ±0.1 part per thousand despite the large variability (approx. ±1 parts per thousand) in the isotope ratio correction factors. The latter exhibit larger variability due to different plasma conditions.
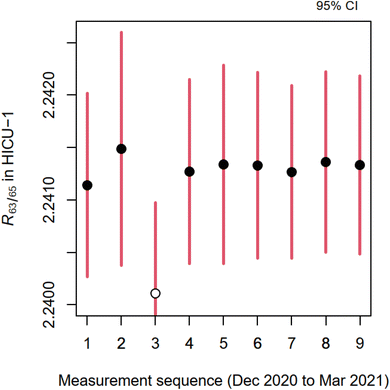 |
| Fig. 2 Copper isotope ratio estimates in HICU-1 from nine measurement sequences spanning from December 2020 to March 2021. Error bars correspond to 95% confidence intervals. Results for the measurement sequence nr. 3 are not used as they exhibit a large (0.2%) drift in the observed isotope ratios during the measurement sequence. | |
The uncertainty associated with the R63/65(HICU-1) was evaluated using the Monte Carlo method.24 This approach involves probabilistic modelling of all input data and their interrelationships which produces the estimates of correction factors. Uncertainty evaluation of the isotope ratio correction factor, K63/65, considered contributions from (1) the chemical purity of both enriched copper materials, (2) their masses and dilution factors to obtain primary stock solutions, (3) the subsequent dilution and mixing these solutions to obtain blends AB, (4) repeatability of isotope ratio measurements, (5) isotope ratio drift correction with splines, and (6) uncertainty of the atomic masses of copper isotopes. Uncertainties associated with the aforementioned components were modelled using Gaussian distributions and a single Monte Carlo step provided isotope ratios of R63/65(HICU-1) from all set 1 measurements and isotope ratios of R63/65(HICU-1) from set 2 measurements. The results from both sets were indistinguishable and the resulting estimate of R63/65(HICU-1) was obtained by combining (not averaging) the results from both sets.
We believe that the combined uncertainty associated with R63/65(HICU-1) accounts for every aspect that reasonably contributes to the uncertainty of the measurement, including the homogeneity contribution in HICU-1. The isotopic homogeneity of copper in HICU-1 was assessed by analysis of twelve individual HICU-1 units, which were measured (n = 5) relative to Cumix (made from mixing these 12 units). Standard deviation of 60 isotope delta values was 0.015‰.
Isotope delta value δ(65Cu) = −0.04 ± 0.03‰ (95% CI) in HICU-1 relative to NIST SRM976 was obtained in our laboratory recently,16 thus demonstrating that the two materials are nearly identical in their isotopic composition of copper which, in turn, facilitates the replacement of SRM976 with HICU-1.
3.4. Isotopic abundances and atomic weight of copper in NRC HICU-1
Isotopic abundances and atomic weight of copper in HICU-1 were calculated as derived quantities from the corresponding isotope ratio, R63/65(HICU-1) = 2.2413 ± 0.0009 (95% CI, k = 2), using standard expressions25,26 and the atomic masses of copper isotopes listed in the 2016 Atomic Mass Evaluation report.27 The resulting isotopic composition is summarized in Table 3. The molar mass, M(Cu), and the atomic weight of copper, Ar(Cu), are related as M(Cu) = Ar(Cu)Mu, where Mu is the molar mass constant, Mu = 1 g mol−1 with negligible uncertainty.
The uncertainty of the derived quantities, listed in Table 3, was propagated from the corresponding isotope ratio, R63/65(HICU-1), using Monte Carlo method as implemented in the R package CIAAWconsensus.26
Table 3 Isotopic composition of copper in the NRC HICU-1 isotopic CRM
Quantity |
Value |
Expanded uncertainty, k = 2 |
Unit |
Atomic weight, Ar(Cu) |
63.54607 |
0.00018 |
1 |
Molar mass, M(Cu) |
63.54607 |
0.00018 |
g mol−1 |
Isotopic abundance, x(63Cu) |
0.69148 |
0.00009 |
mol mol−1 |
Isotopic abundance, x(65Cu) |
0.30852 |
0.00009 |
mol mol−1 |
Isotope ratio, n(63Cu)/n(65Cu) |
2.2413 |
0.0009 |
mol mol−1 |
Isotope ratio, n(65Cu)/n(63Cu) |
0.44617 |
0.00018 |
mol mol−1 |
Isotope delta, δSRM976(65Cu) |
−0.04‰ |
0.03‰ |
SRM976 |
Our measurements provide an independent estimate of copper isotope ratio not only in the NRC CRM HICU-1 but also in the long-standing NIST SRM976. Combining the copper isotope ratio in HICU-1 with isotope delta measurements between HICU-1 and SRM976 yields the following isotope ratio value in NIST SRM976 copper isotopic standard: R63/65 = 2.2412 ± 0.0009 (95% CI, k = 2). While the uncertainty achieved in this study is comparable to the similar recent study from NIM China, our estimates differ significantly (see Table 4). In light of these unexplained discrepancies, it will be useful to have an international comparison of copper isotope ratio measurements in order to address the measurement comparability of CRM producers, as it was recently done for the copper isotope delta measurements in high-purity materials (CCQM Pilot Study P213).28
Table 4 Estimates of copper isotope ratio, R63/65, in the NIST SRM976 copper reference material
Laboratory/year |
Value |
Expanded uncertainty, k = 2 |
NIST USA/1964 |
2.2440 |
0.0021 |
NIM China/2022 |
2.2448 |
0.0008 |
NRC Canada/2023 |
2.2412 |
0.0009 |
4. Conclusions
In this work, we have performed an independent, primary measurement of copper isotope ratio in NRC Certified Reference Material HICU-1. For this, we employed near-pure copper isotopes and their gravimetric mixtures in order to calibrate the MC-ICP-MS for instrumental isotopic fractionation. The isotopic composition of NRC HICU-1 is nearly identical to that of NIST SRM976, with isotope delta value δ(65Cu) = −0.04 ± 0.03‰ (95% CI, k = 2) in HICU-1 relative to NIST SRM976. These characteristics would render HICU-1 a suitable “delta-zero” reference material to replace the now-exhausted SRM976 for decades to come. Although the uncertainty of copper isotope ratio in this work is comparable to the only other independent effort to establish the isotopic composition of copper by NIM China, the isotope ratio values differ significantly (6-sigma) which emphasize the need for additional comparative studies to establish isotopic composition of elements in reference materials.
Conflicts of interest
There are no conflicts of interest to declare.
References
- X. Zhu, R. O'nions, Y. Guo, N. Belshaw and D. Rickard, Determination of natural Cu-isotope variation by plasma-source mass spectrometry: implications for use as geochemical tracers, Chem. Geol., 2000, 163(1–4), 139 CrossRef CAS.
- D. Vance, C. Archer, J. Bermin, J. Perkins, P. J. Statham, M. C. Lohan, M. J. Ellwood and R. A. Mills, The copper isotope geochemistry of rivers and the oceans, Earth Planet. Sci. Lett., 2008, 274(1–2), 204 CrossRef CAS.
- M. Bigalke, S. Weyer, J. Kobza and W. Wilcke, Stable Cu and Zn isotope ratios as tracers of sources and transport of Cu and Zn in contaminated soil, Geochim. Cosmochim. Acta, 2010, 74(23), 6801 CrossRef CAS.
- P. Telouk, A. Puisieux, T. Fujii, V. Balter, V. P. Bondanese, A. P. Morel, G. Clapisson, A. Lamboux and F. Albarede, Copper isotope effect in serum of cancer patients. A pilot study, Metallomics, 2015, 7(2), 299 CrossRef CAS PubMed.
- V. Balter, A. N. Costa, V. P. Bondanese, K. Jaouen, A. Lamboux, S. Sangrajrang, N. Vincent, F. Fourel, P. Télouk, M. Gigou and et al,
., Natural variations of copper and sulfur stable isotopes in blood of hepatocellular carcinoma patients, Proc. Natl. Acad. Sci. U. S. A., 2015, 112, 982 CrossRef CAS PubMed.
- F. Moynier, F. Albarède and G. F. Herzog, Isotopic composition of zinc, copper, and iron in lunar samples, Geochim. Cosmochim. Acta, 2006, 70(24), 6103 CrossRef CAS.
- F. Moynier, D. Vance, T. Fujii and P. Savage, The Isotope Geochemistry of Zinc and Copper, Rev. Mineral. Geochem., 2017, 82, 543 CrossRef CAS.
- M. Ren, L. Zheng, D. Wang, X. Chen, X. Dong, X. Wei and H. Cheng, Copper isotope ratios allowed for quantifying the contribution of coal mining and combustion to total soil copper concentrations in China, Environ. Pollut., 2022, 308, 119613 CrossRef CAS PubMed.
- P. Télouk, A. Puisieux, T. Fujii, V. Balter, V. P. Bondanese, A.-P. Morel, G. Clapisson, A. Lamboux and F. Albarede, Copper isotope effect in serum of cancer patients. A pilot study, Metallomics, 2015, 7(2), 299 CrossRef PubMed.
- V. Balter, A. Nogueira da Costa, V. P. Bondanese, K. Jaouen, A. Lamboux, S. Sangrajrang, N. Vincent, F. Fourel, P. Télouk, M. Gigou and et al,
., Natural variations of copper and sulfur stable isotopes in blood of hepatocellular carcinoma patients, Proc. Natl. Acad. Sci. U. S. A., 2015, 112(4), 982 CrossRef CAS PubMed.
- F. Moynier, D. Vance, T. Fujii and P. Savage, The Isotope Geochemistry of Zinc and Copper, Rev. Mineral. Geochem., 2017, 82(1), 543 CrossRef CAS.
- L. Yang, S. Tong, L. Zhou, Z. Hu, Z. Mester and J. Meija, A critical review on isotopic fractionation correction methods for accurate isotope amount ratio measurements by MC-ICP-MS, J. Anal. At. Spectrom., 2018, 33(11), 1849 RSC.
- J. Meija, T. B. Coplen, M. Berglund, W. A. Brand, P. De Bièvre, M. Gröning, N. E. Holden, J. Irrgeher, R. D. Loss and T. Walczyk, Isotopic compositions of the elements 2013 (IUPAC technical Report), Pure Appl. Chem., 2016, 88(3), 293 CrossRef CAS.
- W. R. Shields, T. J. Murphy and E. L. Garner, Absolute Isotopic Abundance Ratio and the Atomic Weight of a Reference Sample of Copper, J. Res. Natl. Bur. Stand., 1964, A(68), 589 CrossRef PubMed.
- P. Song, T. Zhou, J. Wang, S. Wang, T. Ren and H. Lu, An improved calibrated mass spectrometry for absolute copper isotope-ratio measurement using two strategies for mass bias correction factor, Anal. Chim. Acta, 2022, 1211, 339905 CrossRef CAS PubMed.
- K. Sullivan, D. Layton-Matthews, M. Leybourne, J. Kidder, Z. Mester and L. Yang, Copper isotopic analysis in geological and biological reference materials by MC-ICP-MS, Geostand. Geoanal. Res., 2020, 44, 349 CrossRef CAS.
- J. Meija, B. Methven and R. E. Sturgeon, Uncertainty of relative sensitivity factors in glow discharge mass spectrometry, Metrologia, 2017, 54(5), 796 CrossRef CAS.
- R. E. Sturgeon, B. Methven, S. N. Willie and P. Grinberg, Assignment of purity to primary metal calibrants using pin-cell VG 9000 glow discharge mass spectrometry: a primary method with direct traceability to the SI international system of units?, Metrologia, 2014, 51(5), 410 CrossRef CAS.
-
B. Methven, I. Pihillagawa Gedara, E. Pagliano, P. Grinberg, L. Yang, J. Meija and Z. Mester, HICU-1: High Purity Copper Certified Reference Material for Copper Mass Fraction and Elemental Impurities, National research Council of Canada, 2020, DOI:10.4224/crm.2020.hicu-1.
- J. Meija, Calibration of isotope amount ratios by analysis of isotope mixtures, Anal. Bioanal. Chem., 2012, 403(8), 2071 CrossRef CAS PubMed.
- A. Henrion, Reduction of systematic errors in quantitative analysis by isotope dilution mass spectrometry (IDMS): an iterative method, Fresenius. J. Anal. Chem., 1994, 350(12), 657 CrossRef CAS.
- E. Pagliano, Z. Mester and J. Meija, Reduction of measurement uncertainty by experimental design in high-order (double, triple, and quadruple) isotope dilution mass spectrometry: application to GC-MS measurement of bromide, Anal. Bioanal. Chem., 2013, 405(9), 2879 CrossRef CAS PubMed.
- S. Tong, J. Meija, L. Zhou, B. Methven, Z. Mester and L. Yang, High-precision measurements of the isotopic composition of common lead using MC-ICPMS: Comparison of calibration strategies based on full gravimetric isotope mixture and regression models, Anal. Chem., 2019, 91(6), 4164 CrossRef CAS PubMed.
-
A. Possolo and J. Meija, Measurement Uncertainty: A Reintroduction, Sistema Interamericano de Metrologia, 2nd edn, 2022 Search PubMed.
- J. Meija and Z. Mester, Uncertainty propagation of atomic weight measurement results, Metrologia, 2008, 45(1), 53 CrossRef CAS.
- J. Meija and A. Possolo, Data reduction framework for standard atomic weights and isotopic compositions of the elements, Metrologia, 2017, 54(2), 229 CrossRef CAS.
- M. Wang, G. Audi, F. Kondev, W. Huang, S. Naimi and X. Xu, The AME2016 atomic mass evaluation (II). Tables, graphs and references, Chin. Phys. C, 2017, 41(3), 030003 CrossRef.
- L. Yang, J. Vogl, J. Mann, R. Kraft, R. Vocke, A. Pramann, J. Eberhardt, O. Rienitz, K.-S. Lee, J. S. Lim and et al, Copper isotope delta measurements in high purity materials: CCQM-P213 pilot study, Metrologia, 2023, 60(1A), 08019 CrossRef.
|
This journal is © The Royal Society of Chemistry 2023 |