DOI:
10.1039/D2EN00801G
(Critical Review)
Environ. Sci.: Nano, 2023,
10, 62-71
Biological effects of AgNPs on crop plants: environmental implications and agricultural applications
Received
30th August 2022
, Accepted 11th November 2022
First published on 15th November 2022
Abstract
The varied use and disposal of silver nanoparticles (AgNPs) has allowed their presence in reclaimed water and sewage sludge, making them possibly available in agricultural soils. Furthermore, research advances have highlighted the agricultural application of AgNPs as alternative pesticides. As such, it is imperative to understand the phytotoxicity of AgNPs to evaluate their environmental implications. Although this is a subject of intense investigation, most of the reported results come from environmentally irrelevant concentrations. An analysis of the publications using relevant concentrations demonstrates that AgNPs may provide several benefits to crop plants. In addition, data show that one of the plant responses to AgNP exposure is the production of reactive oxygen species (ROS), which depending on the concentration may cause a variety of effects in plants. Excess ROS may damage tissues and interrupt biological functions, while low ROS may trigger beneficial responses. Data available are discussed and based on the evidence, it is hypothesized that the biological effects of AgNPs in plants may be attributed to ROS activation. In addition, the ROS generation mechanisms and molecular mechanisms of plant responses to AgNPs are discussed. Finally, the risk assessment of the application of AgNPs in agriculture, as well as the research questions to fill the knowledge gaps, is depicted in this review.
Environmental significance
In this review article, we highlight the possibility of the overestimation of AgNPs' phytotoxicity due to the use of environmentally irrelevant concentrations used in previous reports. We propose that the phytotoxicity of AgNPs depends on the level of reactive oxygen species (ROS) that AgNPs produced, and appropriate doses of ROS stimulate the growth of plants, while over-accumulated ROS inhibit plant growth. In addition, ROS generated via catalysis by AgNPs can act as signaling molecules, which may activate systematic defense response and subsequently promote the stress resistance ability of plants. As such, low doses of AgNPs may have potential applications as nanobiostimulants in agriculture.
|
Introduction
Silver (Ag) is a precious metal that has been used by, practically, all ancient cultures. It has had application in jewelry, coinage, rituals, and medicine. Silver was the main antibacterial agent before the appearance of antibiotics.1 One of the first written works documenting the medicinal properties of silver is the Compendium of Materia Medica (
), a Chinese medical text dating back to 1518–1593. There have been many historical applications of Ag in medicine. For instance, silver nitrate (AgNO3) was used to prevent gonorrheal eye infections in newborns,2 while Ag foils were used to prevent infections in surgical wounds.3 The medical use of Ag diminished rapidly after the discovery of antibiotics in the late 1920s.4 However, the indiscriminate use of antibiotics has resulted in the antibiotic resistance crisis that has forced medicine to seek alternative treatments. The rapid development of nanotechnology, and the application of nanomaterials in the medical area, seems to have opened the door for the rebirth of Ag as an antimicrobial agent.
Silver nanoparticles (AgNPs) are composed of clusters of atoms ranging between 1 and 100 nm in size. The excellent antibacterial properties of AgNPs allow their application in medicine, food packaging, food additives, and water disinfection. Other applications include textiles, electronics, household appliances, cosmetics, and sprays.5 In recent decades, AgNPs have received considerable attention as nanopesticides for the control of plant-associated pathogens such as insects, fungi, bacteria, and viruses.6,7 To gain the safe and sustainable use of AgNPs in agricultural production, the assessment of their environmental implications has to keep pace with the AgNPs' application.8
Crop plants are one of the essential building blocks of ecosystems, and the understanding of plant–nanoparticle interactions is an indispensable part of risk assessment.9 Furthermore crop plants are the most important source of food, which supply mankind with rich sources of energy, proteins, vitamins, and minerals. Wheat, rice, maize, pearl millet, and sorghum provide over half of the world's food calories.10 As such, understanding the impacts of AgNPs on crop plants is of critical importance for the evaluation of their potential risks on agriculture and human health.11
It is estimated that 450 t per year of AgNPs are used worldwide,12 most of which ends up in agricultural soils.13,14 The annual input of silver to agricultural soils due to the use of reclaimed sludge and water was estimated to be 1.2 × 10−3 to 9.68 μg kg−1.15 As such, the chance for crop plants to interact with AgNPs will increase correspondingly.16 For food security and food safety concerns, some issues have to be addressed, concerning the use of AgNPs in agriculture: 1) the effects on plant growth; 2) the effects on yield; 3) the bioaccumulation of Ag in edible tissues and its transfer to human via food chain; and 4) the impacts of AgNP exposure on the nutritional qualities of crop plants (Fig. 1).
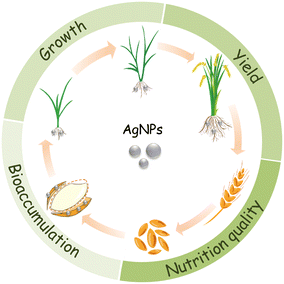 |
| Fig. 1 Schematic illustration of the main effects of AgNPs on crop plants. | |
Actually, the abovementioned concerns have been in place for a long time, and one of the earliest publications regarding the phytotoxicity of AgNPs dates back to 2007.17 To date, tremendous research efforts have been made on these concerns, but several gaps still exist in current knowledge regarding the environmental risk of the use of AgNPs on crop plants. Therefore, the aim of the present review is to provide an overview of the current understanding about the environmental risk of AgNPs. This paper begins discussing the current knowledge and gaps regarding the phytotoxicity of AgNPs on crop plants. Subsequently, the hypothesis that AgNPs trigger biological effects due to the production of excess reactive oxygen species (ROS) molecules is discussed. In this regard, the mechanism of ROS production and molecular mechanisms used by plants to deal with are explored. Finally, this paper offers a fresh look at future directions on the environmental risk assessment of AgNPs.
General review on the phytotoxicity of AgNPs
A literature search in Web of Science Knowledge using the keywords “silver nanoparticle” and “plant” resulted in 129 hits for the span 2012–2022. After abstracts' inspection, 80 peer-reviewed journal articles were identified as phytotoxicity studies. Among them, 71 studies reported plant exposure via root and 9 articles described foliar exposure results.
Impacts of AgNPs on plant growth via root and foliar exposure
From the analysis of root exposure studies, the following facts can be deduced: first, about 82% (58 out of 71) of the articles describe experiments performed in artificial growth media including nutrient solution, agar, or sand. Very likely, in these media, the AgNPs will interact directly with the root tissue causing specific effects. However, natural soil is a more sophisticated system where the AgNPs will undergo dissolution, adsorption, aggregation, fixation, and transformation. Therefore, the soil has a buffering capacity that can modulate the toxicity of nanoparticles.18 Lee et al.19 revealed that growth media effect the phytotoxicity of AgNPs on crop plants. As such, the data obtained for the above-mentioned media cannot represent the real environmental risk. Second, 18% (13 out of 71) of the studies were performed in real soil; but, unfortunately, the exposure concentrations of AgNPs varied from 100 μg kg−1 to 2000 mg kg−1, which are higher than the predicted Ag concentration in real soil (5.33 × 10−6 ∼ 7.4 μg kg−1).15 In a recent review, Kulikova suggests that the exposure dose should not exceed a realistic concentration (10 μg kg−1 soil) of AgNPs.15 Up to date, only in a few studies, the plants were exposed to environmentally realistic concentrations of AgNPs. It is noteworthy that in recent studies, researchers have started to use a relatively low exposure dose (0.1–1 mg kg−1).20–22 Under a low exposure dose, AgNPs have been found to have either no toxic effects, or even positive/stimulatory effects on plant growth. For instance, Wang et al.23 revealed that AgNPs (1–10 mg kg−1), entering the soil via the application of sludge, pose low risk to wheat (Triticum aestivum L. cv. Sunbrook). Liu et al.24 found that AgNPs at 1 mg kg−1 soil did not impact wheat growth and yield. In a recent study, Singh and Kumar22 found that exposure of Ag2O NPs (1 and 10 mg kg−1 soil–sludge) did not show any significant reduction in spinach (Spinacia oleracea) growth, compared to control. In a more recent study, Yan et al.25 have found that AgNPs, at 1 mg kg−1, promoted rice growth and significantly increased rice yield by 42.3%, compared with the control. Taken together, the results indicate that AgNPs at a low exposure dose (<1 mg kg−1) are non-toxic to crop plants. Therefore, we can state that AgNPs at environmentally realistic concentrations (<10 μg kg−1) have limited negative impacts on crop plants. Compared to root exposure, foliar exposure has been less documented in AgNPs' phytotoxicity studies.26–29 Only 11% of the studies (9 out of 80) describe experiments where the AgNPs were exposed to plants via leaves. The leaf is an important exposure pathway for products that control pests via contact. However, a number of pests have shown resistance to traditional pesticides, which opens a door for the use of alternative products. AgNPs show promising potential to replace traditional pesticides, given their ability to control plant pathogens including insects, weeds, bacteria and fungi.30 It is predicted that AgNP-based nanopesticides will soon be on the market to be widely applied in agriculture. Different from root exposure in which the soil acts as a buffering medium, foliar exposure will result in the direct deposit of AgNPs on the leaf surface, facilitating the uptake and subsequent translocation to other tissues. Li et al.27 compared the effects of exposure pathways (foliar vs. root) on the bioaccumulation of AgNPs in soybean (Glycine max (L.) Merr.) and rice (Oryza sativa L.). They demonstrated that foliar exposure led to 17–200 times more Ag bioaccumulation than root exposure. Larue et al.28 found that AgNPs can be internalized inside lettuce (Lactuca sativa L.) leaves after foliar exposure, and a classical washing process inefficient to remove Ag from edible tissues. By using μXRF and ToF-SIMS, they revealed that foliar sprayed AgNPs were embedded in the leaf cuticle and/or in cuticular waxes present on its surface, as well as in the sub-stomatal chamber. Taken together, for leafy vegetables, especially if the leaf surface is hydrophilic, nanoparticulate Ag can easily accumulate in edible leaves. In the future, more phytotoxicity studies via foliar exposure are needed to investigate the phytotoxicity and bioaccumulation of AgNPs on crop plants. It should be noted that foliar spray implies that a portion of the product will go directly to the soil and water, representing a contamination source.
Internalization of AgNPs/Ag and the potential risks of entering into food chain
In addition to the phytotoxicity concern, whether AgNPs or Ag can bioaccumulate in the edible tissues of crop plants and enter the food chain is another concern. Thus far, reports about the bioaccumulation of AgNPs mainly focus on vegetables, especially leafy vegetables such as lettuce31 and spinach.22 Previous reports have shown that Ag can accumulate in edible tissues of leafy vegetables either via root exposure or foliar exposure. For example, Wu et al.32 reported that Ag can be taken up by hydroponically cultivated lettuce (L. sativa L.). By using inductively coupled plasma-mass spectrometry (ICP-MS), Ag (1 mg kg−1) was detected in the leaves of lettuce exposed to 0.75 mg L−1 AgNPs, which can be further transferred to lettuce's consumer snails. In contrast, Li et al.33 planted three vegetable plants (chili (Capsicum annuum L.), lettuce (L. sativa L.) and radish (Raphanus sativus)) in the soil amended with the AgNP-containing sludge (10.4 ± 2.1 mg kg−1) for 52–71 days, and found that Ag was not accumulated in the edible tissues of all the tested plants. As we mentioned before, soil is a more complex system, AgNPs may undergo adsorption and fixation with soil minerals and organic matters, which will reduce the bioavailability of AgNPs to plants. Different from root exposure, foliar exposure easily results in NP accumulation in leaves. Li et al.33 found that foliar spray of AgNPs at 10 mg L−1 resulted in Ag accumulation in younger leaves (45 mg kg−1 fresh weight) and flowers of lettuce (L. sativa L.). In another AgNP–lettuce study, Larue et al.28 not only observed that AgNPs were trapped in lettuce leaves, but also revealed the biotransformation of AgNPs in lettuce cells, such as oxidation of AgNPs and complexation of Ag+ with thiol-containing molecules.
Cereals crops such as wheat, maize, rice, and sorghum are major staple foods for the most population. Compared to leafy vegetables, current understanding on the bioaccumulation of Ag in grains of cereal crops is very limited, because the end period of experiments hardly waits for the fulfilment of the plant life cycles. Liu et al.24 found that applying 1 mg kg−1 AgNPs to the soil at the seedling stage of wheat did not induce Ag bioaccumulation in wheat grains, and there was no significant enrichment in root, stem and leaf tissues. In a recent study, Yan et al.34 have found that exposure of rice seedlings to 1 mg kg−1 AgNPs via root irrigation did not result in Ag accumulation in rice grains, while 10 mg kg−1 AgNPs resulted in Ag accumulation in rice husk. From these reports, it can be concluded that the risks for low soil Ag concentration entering cereal grains are generally limited. Thus far, knowledge regarding the Ag accumulation in soybean and maize seeds is still missing. It is strongly recommended that future studies cover the life cycle of these plants to determine the possible accumulation of Ag in the edible portion. Similar recommendation applies for studies using foliar spray of AgNPs in cereals plants.
It has to be pointed out that the above-mentioned studies used ICP-MS to quantify the Ag content in plant tissues after acid digestion, whether the detected Ag was in nanoparticulate form or ionized silver remains unknown. From the view of toxicology, AgNPs and ionized Ag may induce different metabolic changes when entering into cells. Recently, researchers have started to use advanced single particle-inductively coupled plasma-mass spectrometry (sp-ICP-MS) technique for the quantitative analysis of NP internalization in plant tissues.35 Bao et al.35 cultivated 2-week-old seedlings of Arabidopsis in a nutrient solution with 0.02 mg L−1 of AgNPs for two weeks. After exposure to AgNPs for 2 weeks, the size distribution of AgNPs in leaves was determined by sp-ICP-MS after enzymatic digestion. They found that the size of AgNPs in roots and leaves was centered at around 20.7 nm, which evidenced that plant can uptake and bioaccumulate nanoparticulate Ag.
Effects of AgNP exposure on the nutritional quality of grains or vegetables
The nutritional value of food is given by several components including essential minerals such as iron (Fe) and zinc (Zn), and protein, which are important in human diet.10 Chronic micronutrient deficiency can increase the risk of “hidden hunger”, which is not associated with the lack of food but occurs when the food does not provide sufficient levels of vitamins and minerals.36 Li et al.33 found that AgNPs added to the soil at 10 mg kg−1 significantly decreased the content of K and Mg by 25.0% in the leaves of lettuce. Vittori Antisari et al.37 irrigated basil (Ocimum basilicum L.) with a solution containing AgNPs (100 mg L−1) for four weeks and found that the contents of root Mg and S were significantly decreased. Yang et al.38 exposed wheat plants to different concentrations of AgNPs for 4 months, and found that 2000 mg kg−1 decreased the content of Fe, Cu, and Zn in grains. However, this is an unrealistic concentration that will hardly be found in real life. In a recent study, Yan et al.34 have exposed rice (Liaoxing 1) plants to a relatively low dose (1 and 10 mg kg−1 soil) and found that AgNP exposure at 1 mg kg−1 decreased the content of Fe and Mo by 24.7% and 31.8%, respectively, in grains, while 10 mg kg−1 AgNPs decreased the K content by 19.4% in grains. These reports indicate that AgNP exposure has a negative impact on the nutrition quality of crops, especially mineral nutrients. Nevertheless, Jurkow et al.31 found that foliar application of AgNPs at 40 mg L−1 increased total phenolics content of lettuce grown in hydroponics by 17%. Collectively, both food security and nutrition quality should be considered when evaluating AgNP phytotoxicity.
Phytotoxicity of AgNPs: ROS-driven dose-dependent response
The information contained in most of the 80 analyzed studies suggests that AgNPs cause negative effects on plants, and only a few of them reported positive effects on plant growth. This is in agreement with a previous review article by Yan and Chen, who also observed contradictory reports in AgNP phytotoxicity studies.11 Nevertheless, a large proportion of studies show a common phenomenon: AgNPs/Ag ions trigger the overproduction of reactive oxygen species (ROS) in plants, although the mechanisms for ROS production remain unclear. ROS play dual roles in plant growth and development.39 A small increase in ROS levels activates signaling pathways to initiate biological processes, while at high levels, they cause oxidative stress.40 That means ROS have two faces, namely, redox biology and oxidative stress. As such, the ROS level generated by AgNPs might be a key factor driving the biological effects on plants (positive or negative). Appropriate amounts of ROS (within the threshold level) may stimulate plant growth, while excessive ROS inhibit plant growth by triggering oxidative stress. The biological effects of AgNPs on plants might be a biphasic dose response characterized by stimulation at low doses and inhibition at high doses. The detrimental effects of AgNPs on plants observed in studies are possibly due to the high exposure dose. As summarized in Fig. 2, the inhibitory effects of AgNPs on plants reported in previous studies include generation of oxidative stress,41–44 inhibition of photosynthetic activities,45 and inhibition of plant growth.
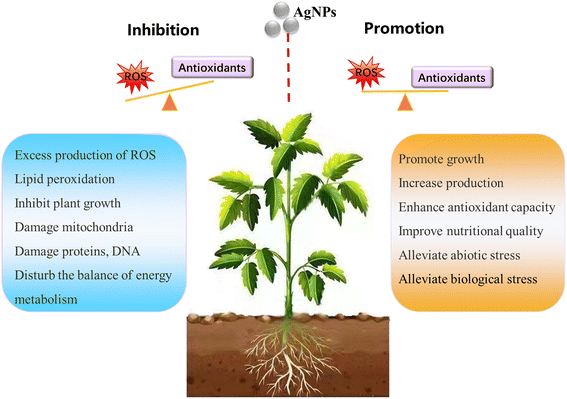 |
| Fig. 2 Schematic representation of the promotion and inhibitory effects of AgNPs on plants. | |
It is noteworthy that a few studies have described the biphasic dose response of AgNPs on crop plants.46–50 For instance, Mirzajani et al.51 found that AgNPs at 30 mg L−1 accelerated root growth of Oryza sativa L., while 60 mg L−1 AgNPs restricted root growth. This dose–response effects seem like “hormesis” effects (Fig. 3), in which low stress generated by ROS induces the adaptive responses; while high stress by ROS inhibits plant growth.52 Previous studies have focused on observing the negative effects of nanomaterials (NMs) on plants under high exposure doses, while few studies paid attention to the stimulatory effects of NMs at low exposure doses.53 This may lead to the overestimation of the toxicity of NMs, and the potential positive effects were likely neglected. Some recent studies have observed the hormesis-like effects of low doses of NMs on plant species.54,55 For example, AgNPs have been found to induce stimulatory effects on the root growth of maize plants. The authors attribute this to hormesis effects.54 In another study, Magaña-López et al.55 found that foliar spray of silica NMs (20 mg L−1) induced hormesis on chili pepper (C. annuum L.) production and protection to cold stress. Gupta et al.56 found the stimulatory effect of bio-synthesized AgNPs on rice (Oryza sativa L., cv. Swarna) during seed germination and seedling growth. They found that AgNP exposure increased the activities of several antioxidant enzymes including catalase (CAT), ascorbate peroxidase (APX) and glutathione reductase (GR), and decreased the amount of lipid peroxidation and H2O2 content. As such, the authors attribute the positive effects of AgNPs on rice seedling growth to the increased redox reaction efficiency. This is a good example that AgNPs, at appropriate concentrations, could act as biostimulants to promote plant growth.
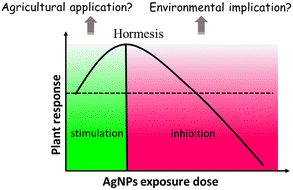 |
| Fig. 3 U-shaped dose–response curve of AgNP hormesis effects, which exhibit low dose stimulation and high dose inhibition. | |
Apart from the negative effects, some studies observed positive/stimulatory effects of AgNPs on crop plants, which could be applied in agriculture. Particularly, a growing number of studies have shown that seed priming with AgNPs promoted seed germination and seedling development. For instance, Mahakham et al.57 found that rice aged seeds primed with AgNPs at 10 and 20 mg L−1 significantly increased the germination rate by 18.5% and 29.6%, respectively. Zhou et al.58 showed that AgNP-priming at 20 and 40 mg L−1 significantly accelerated seed germination and seedling development of Chinese cabbage (Brassica campestris L.). Acharya et al.59 found that onion (Allium cepa L.) seeds primed with AgNPs at 31.3 mg L−1 increased seed germination and seedling emergence. Similar results have been found in a variety of plant species including Zea mays L., Trigonella foenum-graecum L., and A. cepa L.60 These studies have showed that AgNPs can be used as priming agents to increase the germination performance of crops. Recently, some studies have shown that AgNP priming not only can promote germination, but also can increase the yield. Zhou et al.58 showed that priming of cabbage seeds with 40 mg L−1 of AgNPs significantly increased the yield by 44.3%. Meanwhile, AgNP priming increased the Fe content in cabbage leaves by 23.8%. Acharya et al.59 revealed that Riverside and Maxima watermelon (Citrullus lanatus) seeds primed with AgNPs (31.3 mg L−1) showed significantly increased yields by 31.6% and 35.6%, respectively. Cabbage is a leafy vegetable with a relatively short life cycle. Thus far, whether seed priming with AgNPs can increase the yield of main crops including wheat, rice, and maize remains unknown. Given the low dose of AgNPs used during priming (10–40 mg L−1), AgNP-based seed priming could be an ecofriendly approach to support the sustainable development of agriculture. The mechanisms by which AgNP priming promotes germination remain largely unknown. We speculate that AgNP-generated ROS, as stress signal molecules, can trigger stress responses, inducing the changes in metabolome and transcriptome. These molecular changes may contribute to the promotion of germination and seedling establishment. Recently, Acharya et al.59 have employed 1H nuclear magnetic resonance (NMR) and liquid chromatography coupled with mass spectrometry (LC-MS) based on metabolomics to explore the metabolome profile changes in onion seeds after AgNP priming. From the changes in metabolic imprint, they elucidated the mechanisms through which AgNP priming improved the seed performance. They revealed that AgNP seed priming treatment decreased the content of hormones and growth regulators such as abscisic acid and cis-(+)-12-oxo-phytodienoic acid, while increasing germination-related stimulators such as γ-aminobutyric acid and zeatin. This study sheds light on the mechanistic analyses of nano seed priming. Omics, especially metabolomics and transcriptomics, will be a powerful tool to get a holistic understanding of the molecular responses of seeds to AgNP stimulation.
In addition to improving seed germination and yield under non-stressed conditions, AgNP seed priming has been shown to increase the stress resistance or tolerance of plants. Salt stress is one of the major abiotic stresses endured by crops. Mohamed et al.61 showed that seed priming with AgNPs (215, 539 and 1079 mg L−1) for 24 h increased the shoot fresh and dry weight of normal and salt-stressed (8.77 g L−1 of NaCl) wheat seedlings (T. aestivum L.). The authors also observed that AgNP priming increased the activities of key antioxidant enzymes and decreased the malondialdehyde (MDA) and H2O2 contents in leaves.61 This indicates that priming with AgNPs alleviated the salt stress through boosting the antioxidant defense system of wheat. Similarly, Khan et al.62 demonstrated that seed priming with AgNPs (1.08, 2.16 and 3.24 g L−1) enhanced the height, yield, and photosynthesis of pearl millet (Pennisetum glaucum L.) grown under salt conditions (7.01, 8.77 g L−1 of NaCl). Similarly, it was observed that the increased superoxide dismutase (SOD) and catalase (CAT) activities reduced the H2O2 and MDA contents in AgNP-primed seeds. In addition to alleviating salt stress, AgNP-priming has been shown to increase the tolerance to low temperature stress of plants. For instance, Prażak et al.63 demonstrated that at chill temperatures, the germination rate of green bean (Phaseolus vulgaris L.) seeds treated with AgNPs at 0.25, 1.25 and 2.5 mg L−1 was remarkably increased. Especially, low concentrations of AgNPs (0.25, 1.25 mg L−1) augmented the average seedling height, fresh and dry weight, and net photosynthesis. Collectively, low levels of AgNP-generated ROS play a positive role in plant growth and stress tolerance, which have potential applications in agriculture.
Mechanistic insights into the biological effects of AgNP-induced ROS on plant
Regardless of the positive or negative effects of AgNPs on plants, it is important to understand the molecular mechanisms underlying the biological effects of AgNPs on plant life. Numerous studies have evidenced that AgNP exposure triggers the generation of ROS (Fig. 4). However, it was unclear if ROS are generated by AgNPs themselves or dissolved Ag ions. Some recent experiments have shown that under in vitro conditions, the ROS generator is AgNPs, instead of Ag ions. He et al.64 found that H2O2 can mediate oxidation of zero-valent AgNPs under acidic conditions (pH 3.0, 3.5, 4.0), generating hydroxyl radicals (˙OH) and Ag(I). In contrast, Ag+ did not exert significant activity for generating ˙OH in the presence of H2O2. By using electron spin resonance (ESR), Li et al.65 also demonstrated that AgNPs can directly produce ˙OH in the presence of H2O2, while Ag ions did not catalyze the production of free radicals. The formation of ˙OH by AgNPs occurs via a mechanism similar to the Fenton reaction (Fig. 5), in which AgNPs act as the Fenton-like reagent:
Ag + H2O2 + H+ = Ag+ + ˙OH + H2O |
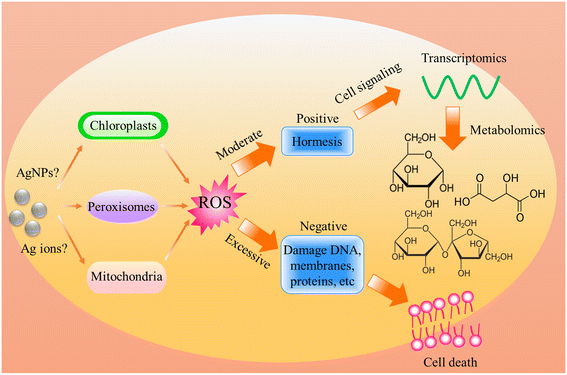 |
| Fig. 4 Schematic diagram of the biological effects of AgNP-induced ROS on plants, highlighting the positive and negative effects caused by moderate and excessive ROS respectively. | |
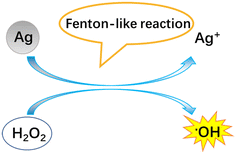 |
| Fig. 5 Schematic diagram of the Fenton-like reaction of AgNPs to generate hydroxyl radicals. | |
It is important to point out that the two above-mentioned reports were from in vitro experiments. In cells, the environment (pH, matrix, and molecules) is complicated, and whether nanoparticulate Ag has Fenton-like effects and catalyze the generation of ˙OH in cells remains unknown. In addition, some in vivo experiments showed that Ag ions also triggered the generation of ROS. For example, Huang et al.66 found that Ag ions at 10 μg L−1 induced a significant ROS increase in cyanobacteria (Nostoc sphaeroides). In addition, Ag ion and AgNP exposure induced very similar metabolic changes in N. sphaeroides, and the antioxidant related metabolites (polyphenols and ascorbic acid) were significantly decreased. Taken together, it is still debated if ROS are generated from AgNPs themselves or dissolved Ag ions. More studies, especially in vivo experiments are needed to clarify the ROS generation mechanism by AgNPs.
Although ROS generation mechanism remains unclear, it can be confirmed that ROS are generated upon exposure to AgNPs. Hydrogen peroxide and hydroxyl radicals are produced predominantly in plant cells during photosynthesis, photorespiration, and respiration processes.67 Hydroxyl radicals are the most potent, yet short-lived of the ROS radicals.68 On the one hand, ˙OH is acknowledged to play signaling roles in plant cells, while on the other hand, excess of ˙OH induces destruction in cells.68 Appropriate amounts of ˙OH can activate the immune response, and thereby enhance the adaptation to stress. This might be the reason for the above-mentioned plant resilience enhancement by AgNP priming under stress conditions. In several studies, it has been observed that there is upregulation of defense-related genes in plants exposed to AgNPs. For instance, Nair et al.69 found that AgNP exposure (0, 0.2, 0.5 and 1 mg L−1) to rice seedlings increased ROS level and up-regulated genes related to oxidative stress tolerance, e.g., FSD1, MSD1, CSD1, CSD2, CATa, CATb, CATc, APXa and APXb. Li et al.70 found that AgNPs (5 mg L−1) triggered the up-regulation of detoxification-related genes (GSH1 and GST) in lotus (Nelumbo nucifera). Noori et al.44 revealed that exposure of tomato (Lycopersicon esculentum) to AgNPs at 10–30 mg L−1 induced upregulation of defense-related genes, including ethylene-inducing xylanase (EIX), peroxidase 51 (POX), and phenylalanine ammonia lyase. In addition to inducing the changes at gene level, AgNP exposure has been found to induce proteomics changes. Mirzajani et al.71 found that rice seedlings exposed to 60 mg AgNPs per L depicted proteomics alteration, upregulation of proteins involved in oxidative stress tolerance, and Ca2+ regulation and signaling. At metabolic level, some studies also found that AgNP exposure triggered the up- or down-regulation of antioxidant defense-related metabolites. For example, Zhang et al.72 found that AgNP foliar exposure at 40 mg per plant up-regulated the level of ferulic acid, ethyl cinnamate, cis-2-hydroxycinnamic acid, and 1,2,4-benzenetriol in cucumber leaves, which are associated with ROS scavenging. This suggests that AgNPs can induce antioxidant defense responses. When the generated ROS are within the threshold value, the plant is not damaged, but can be positively stimulated. Conversely, when ROS cannot be eliminated timely, damage occurred. It is possible that the negative effects reported in some studies were due to the excess ROS. Hossain et al.73 found that AgNPs (50 mg L−1) lead to severe oxidative burst in soybean seedlings. Abdel-Aziz et al. found that AgNPs adversely affected the chloroplast ultrastructure of broad bean (Vicia faba L.).74 By injecting AgNPs (100 mg L−1) into Vicia faba leaves, Falco et al. found that AgNPs lead to excessive production of ROS, resulting in a decrease in the photochemical efficiency of photosystem II (PSII) and an increase in non-photochemical quenching.75 However, this is a very high concentration, considering the environmental relevant concentrations of AgNPs. Studies are needed to determine the threshold concentrations at which AgNPs begin to produce excess ROS.
Conclusions and future perspectives
The literature shows that AgNPs, depending on the concentration, mode of application, and plant species, impact different physiological–biochemical functions in plants. In general, most of the previous studies focused on observing the toxic effects on plants under high doses of AgNPs, ignoring the promising phyto-stimulatory effects at ambient concentrations. An analysis of the literature allows us to predict that AgNPs, at environmentally realistic concentrations, have limited negative impacts on crop plants, and the risks for soil Ag to reach the edible portions are low. However, it has to be pointed out that we need more data regarding the phytotoxicity and bioaccumulation of AgNPs in crop plants including rice, wheat, maize, and soybean grown in real soil and cultivated for the whole life cycle, to make an accurate conclusion about the environmental and health implications of AgNPs. It is worth mentioning that at environmentally relevant concentrations (ppb level), AgNPs or Ag may be non-detectable in plant tissues using current analytical methods. Applying advanced analytical instruments with low detection limits is critical for detecting the threshold concentrations of AgNPs inducing toxic effects. In addition, AgNPs have been found to have even positive/stimulatory effects on plant growth, which have potential applications in agriculture. Further studies are required to determine the mechanism of ROS generation, actual exposed form and threshold of ROS that could stimulate plant growth to help better agricultural applications of AgNPs. Overall, the analysis of available literature leaves no doubt that there are additional aspects still unclear concerning the biological effects of AgNPs on crop plants. For instance, what are the exposure concentrations causing benefits in certain groups of plants? What are the possible mechanisms for ROS generation? What are the best applications of AgNPs in agricultural production? Finally, given the costs of silver, cost–benefit analysis of the use of AgNPs in agriculture is mandatory.
Conflicts of interest
There are no conflicts to declare.
Acknowledgements
This work was funded by National Natural Science Foundation of China under 21876081 and 21906081. Any opinions, finding, and conclusions or recommendations expressed in this material are those of authors and do not necessarily reflect the views of National Science Foundation of China or the United States National Science Foundation.
References
- J. W. Alexander, History of the medical use of silver, Surg. Infect., 2009, 10(3), 289–292 CrossRef.
- Credé, Die Verhütung der Augenentzündung der Neugeborenen, Arch. Gynaekol., 1881, 17(1), 50–53 CrossRef.
- S. Silver, T. Phung le and G. Silver, Silver as biocides in burn and wound dressings and bacterial resistance to silver compounds, J. Ind. Microbiol. Biotechnol., 2006, 33(7), 627–634 CrossRef CAS PubMed.
- J. A. Lemire, J. J. Harrison and R. J. Turner, Antimicrobial activity of metals: mechanisms, molecular targets and applications, Nat. Rev. Microbiol., 2013, 11(6), 371–384 CrossRef CAS.
- E. Rezvani, A. Rafferty, C. McGuinness and J. Kennedy, Adverse effects of nanosilver on human health and the environment, Acta Biomater., 2019, 94, 145–159 CrossRef CAS.
-
N. Konappa, S. Krishnamurthy, U. C. Arakere, S. Chowdappa, R. Akbarbasha and N. S. Ramachandrappa, Chapter 12 - Nanofertilizers and nanopesticides: Recent trends, future prospects in agriculture, in Advances in Nano-Fertilizers and Nano-Pesticides in Agriculture, ed. S. Jogaiah, H. B. Singh, L. F. Fraceto and R. D. Lima, Woodhead Publishing, 2021, pp. 281–330 Search PubMed.
- S. Mansoor, I. Zahoor, T. R. Baba, S. A. Padder, Z. A. Bhat, A. M. Koul and L. Jiang, Fabrication of Silver Nanoparticles Against Fungal Pathogens, Front. Nanotechnol., 2021, 3, 679358 CrossRef.
- P. A. Holden, J. P. Schimel and H. A. Godwin, Five reasons to use bacteria when assessing manufactured nanomaterial environmental hazards and fates, Curr. Opin. Biotechnol., 2014, 27, 73–78 CrossRef CAS PubMed.
- A. M. Santos-Espinoza, D. González-Mendoza, V. M. Ruiz-Valdiviezo, M. C. Luján-Hidalgo, F. Jonapa-Hernández, B. Valdez-Salas and F. A. Gutiérrez-Miceli, Changes in the physiological and biochemical state of peanut plants (Arachis hypogaea L.) induced by exposure to green metallic nanoparticles, Int. J. Phytorem., 2021, 23(7), 747–754 CAS.
- S. S. Myers, A. Zanobetti, I. Kloog, P. Huybers, A. D. B. Leakey, A. J. Bloom, E. Carlisle, L. H. Dietterich, G. Fitzgerald, T. Hasegawa, N. M. Holbrook, R. L. Nelson, M. J. Ottman, V. Raboy, H. Sakai, K. A. Sartor, J. Schwartz, S. Seneweera, M. Tausz and Y. Usui, Increasing CO2 threatens human nutrition, Nature, 2014, 510(7503), 139–142 CrossRef CAS.
- A. Yan and Z. Chen, Impacts of Silver Nanoparticles on Plants: A Focus on the Phytotoxicity and Underlying Mechanism, Int. J. Mol. Sci., 2019, 20(5), 1003 CrossRef CAS PubMed.
- E. McGillicuddy, I. Murray, S. Kavanagh, L. Morrison, A. Fogarty, M. Cormican, P. Dockery, M. Prendergast, N. Rowan and D. Morris, Silver nanoparticles in the environment: Sources, detection and ecotoxicology, Sci. Total Environ., 2017, 575, 231–246 CrossRef CAS PubMed.
- A. Keller and A. Lazareva, Predicted Releases of Engineered Nanomaterials: From Global to Regional to Local, Environ. Sci. Technol. Lett., 2013, 1, 65–70 CrossRef.
- V. Bolaños-Benítez, F. McDermott, L. Gill and J. Knappe, Engineered silver nanoparticle (Ag-NP) behaviour in domestic on-site wastewater treatment plants and in sewage sludge amended-soils, Sci. Total Environ., 2020, 722, 137794 CrossRef.
- N. A. Kulikova, Silver Nanoparticles in Soil: Input, Transformation, and Toxicity, Eurasian Soil Sci., 2021, 54(3), 352–365 CrossRef CAS.
- A. E. Pradas del Real, V. Vidal, M. Carrière, H. Castillo-Michel, C. Levard, P. Chaurand and G. Sarret, Silver Nanoparticles and Wheat Roots: A Complex Interplay, Environ. Sci. Technol., 2017, 51(10), 5774–5782 CrossRef CAS PubMed.
- B. Nowack and T. D. Bucheli, Occurrence, behavior and effects of nanoparticles in the environment, Environ. Pollut., 2007, 150(1), 5–22 CrossRef CAS.
- B. Ahmed, A. Rizvi, K. Ali, J. Lee, A. Zaidi, M. S. Khan and J. Musarrat, Nanoparticles in the soil–plant system: a review, Environ. Chem. Lett., 2021, 19(2), 1545–1609 CrossRef CAS.
- W.-M. Lee, J. I. Kwak and Y.-J. An, Effect of silver nanoparticles in crop plants Phaseolus radiatus and Sorghum bicolor: Media effect on phytotoxicity, Chemosphere, 2012, 86(5), 491–499 CrossRef CAS PubMed.
- P. Wang, N. W. Menzies, P. G. Dennis, J. Guo, C. Forstner, R. Sekine, E. Lombi, P. Kappen, P. M. Bertsch and P. M. Kopittke, Silver Nanoparticles Entering Soils via the Wastewater-Sludge-Soil Pathway Pose Low Risk to Plants but Elevated Cl Concentrations Increase Ag Bioavailability, Environ. Sci. Technol., 2016, 50(15), 8274–8281 CrossRef CAS.
- G. Liu, M. Zhang, Y. Jin, X. Fan, J. Xu, Y. Zhu, Z. Fu, X. Pan and H. Qian, The Effects of Low Concentrations of Silver Nanoparticles on Wheat Growth, Seed Quality, and Soil Microbial Communities, Water, Air, Soil Pollut., 2017, 228(9), 348 CrossRef.
- D. Singh and A. Kumar, Binary mixture of nanoparticles in sewage sludge: Impact on spinach growth, Chemosphere, 2020, 254, 126794 CrossRef CAS PubMed.
- P. Wang, N. W. Menzies, P. G. Dennis, J. Guo, C. Forstner, R. Sekine, E. Lombi, P. Kappen, P. M. Bertsch and P. M. Kopittke, Silver Nanoparticles Entering Soils via the Wastewater–Sludge–Soil Pathway Pose Low Risk to Plants but Elevated Cl Concentrations Increase Ag Bioavailability, Environ. Sci. Technol., 2016, 50(15), 8274–8281 CrossRef CAS PubMed.
- G. Liu, M. Zhang, Y. Jin, X. Fan, J. Xu, Y. Zhu, Z. Fu, X. Pan and H. Qian, The Effects of Low Concentrations of Silver Nanoparticles on Wheat Growth, Seed Quality, and Soil Microbial Communities, Water, Air, Soil Pollut., 2017, 228(9), 348 CrossRef.
- X. Yan, Z. Y. Pan, S. Chen, N. B. Shi, T. H. Bai, L. Q. Dong, D. M. Zhou, J. C. White and L. J. Zhao, Rice exposure to silver nanoparticles in a life cycle study: effect of dose responses on grain metabolomic profile, yield, and soil bacteria, Environ. Sci.: Nano, 2022, 9(6), 2195–2206 RSC.
- J. Wu, G. Wang, M. G. Vijver, T. Bosker and W. J. G. M. Peijnenburg, Foliar versus root exposure of AgNPs to lettuce: Phytotoxicity, antioxidant responses and internal translocation, Environ. Pollut., 2020, 261, 114117 CrossRef CAS.
- C. C. Li, F. Dang, M. Li, M. Zhu, H. Zhong, H. Hintelmann and D. M. Zhou, Effects of exposure pathways on the accumulation and phytotoxicity of silver nanoparticles in soybean and rice, Nanotoxicology, 2017, 11(5), 699–709 CrossRef CAS.
- C. Larue, H. Castillo-Michel, S. Sobanska, L. Cécillon, S. Bureau, V. Barthès, L. Ouerdane, M. Carrière and G. Sarret, Foliar exposure of the crop Lactuca sativa to silver nanoparticles: evidence for internalization and changes in Ag speciation, J. Hazard. Mater., 2014, 264, 98–106 CrossRef CAS PubMed.
- J. Wu, G. Wang, M. G. Vijver, T. Bosker and W. Peijnenburg, Foliar versus root exposure of AgNPs to lettuce: Phytotoxicity, antioxidant responses and internal translocation, Environ. Pollut., 2020, 261, 114117 CrossRef CAS PubMed.
- D. Wang, N. B. Saleh, A. Byro, R. Zepp, E. Sahle-Demessie, T. P. Luxton, K. T. Ho, R. M. Burgess, M. Flury, J. C. White and C. Su, Nano-enabled pesticides for sustainable agriculture and global food security, Nat. Nanotechnol., 2022, 17(4), 347–360 CrossRef CAS PubMed.
- R. Jurkow, R. Pokluda, A. Sękara and A. Kalisz, Impact of foliar application of some metal nanoparticles on antioxidant system in oakleaf lettuce seedlings, BMC Plant Biol., 2020, 20(1), 290 CrossRef CAS PubMed.
- J. Wu, T. Bosker, M. G. Vijver and W. J. G. M. Peijnenburg, Trophic Transfer and Toxicity of (Mixtures of) Ag and TiO2 Nanoparticles in the Lettuce–Terrestrial Snail Food Chain, Environ. Sci. Technol., 2021, 55(24), 16563–16572 CrossRef CAS PubMed.
- M. Li, H.-l. Liu, F. Dang, H. Hintelmann, B. Yin and D. Zhou, Alteration of Crop Yield and Quality of Three Vegetables upon Exposure to Silver Nanoparticles in Sludge-Amended Soil, ACS Sustainable Chem. Eng., 2020, 8(6), 2472–2480 CrossRef CAS.
- X. Yan, Z. Pan, S. Chen, N. Shi, T. Bai, L. Dong, D. Zhou, J. C. White and L. Zhao, Rice exposure to silver nanoparticles in a life cycle study: effect of dose responses on grain metabolomic profile, yield, and soil bacteria, Environ. Sci.: Nano, 2022, 9(6), 2195–2206 RSC.
- D. Bao, Z. G. Oh and Z. Chen, Characterization of Silver Nanoparticles Internalized by Arabidopsis Plants Using Single Particle ICP-MS Analysis, Front. Plant Sci., 2016, 7, 32 Search PubMed.
- R. De La Torre-Roche, J. Cantu, C. Tamez, N. Zuverza-Mena, H. Hamdi, I. O. Adisa, W. Elmer, J. Gardea-Torresdey and J. C. White, Seed Biofortification by Engineered Nanomaterials: A Pathway To Alleviate Malnutrition?, J. Agric. Food Chem., 2020, 68(44), 12189–12202 CrossRef CAS PubMed.
- L. Vittori Antisari, S. Carbone, S. Bosi, A. Gatti and G. Dinelli, Engineered nanoparticles effects in soil-plant system: Basil (Ocimum basilicum L.) study case, Appl. Soil Ecol., 2018, 123, 551–560 CrossRef.
- J. Yang, F. Jiang, C. Ma, Y. Rui, M. Rui, M. Adeel, W. Cao and B. Xing, Alteration of Crop Yield and Quality of Wheat upon Exposure to Silver Nanoparticles in a Life Cycle Study, J. Agric. Food Chem., 2018, 66(11), 2589–2597 CrossRef CAS.
- A. Gandin, P. Dizengremel and Y. Jolivet, Integrative role of plant mitochondria facing oxidative stress: The case of ozone, Plant Physiol. Biochem., 2021, 159, 202–210 CrossRef CAS PubMed.
- M. Schieber and N. S. Chandel, ROS Function in Redox Signaling and Oxidative Stress, Curr. Biol., 2014, 24(10), R453–R462 CrossRef CAS PubMed.
- X. Zou, P. Li, Q. Huang and H. Zhang, The different response mechanisms of Wolffia globosa: Light-induced silver nanoparticle toxicity, Aquat. Toxicol., 2016, 176, 97–105 CrossRef CAS PubMed.
- P. Cvjetko, A. Milošić, A.-M. Domijan, I. Vinković Vrček, S. Tolić, P. Peharec Štefanić, I. Letofsky-Papst, M. Tkalec and B. Balen, Toxicity of silver ions and differently coated silver nanoparticles in Allium cepa roots, Ecotoxicol. Environ. Saf., 2017, 137, 18–28 CrossRef CAS PubMed.
- R. Kaveh, Y.-S. Li, S. Ranjbar, R. Tehrani, C. L. Brueck and B. Van Aken, Changes in Arabidopsis thaliana Gene Expression in Response to Silver Nanoparticles and Silver Ions, Environ. Sci. Technol., 2013, 47(18), 10637–10644 CrossRef CAS PubMed.
- A. Noori, T. Donnelly, J. Colbert, W. Cai, L. A. Newman and J. C. White, Exposure of tomato (Lycopersicon esculentum) to silver nanoparticles and silver nitrate: physiological and molecular response, Int. J. Phytorem., 2020, 22(1), 40–51 CrossRef CAS PubMed.
- D. Dewez, V. Goltsev, H. M. Kalaji and A. Oukarroum, Inhibitory effects of silver nanoparticles on photosystem II performance in Lemna gibba probed by chlorophyll fluorescence, Curr. Plant Biol., 2018, 16, 15–21 CrossRef.
- H. M. H. Salama, Effects of silver nanoparticles in some crop plants, Common bean (Phaseolus vulgaris L.) and corn (Zea mays L.), Int. Res. J. Biotechnol., 2012, 3(10), 190–197 Search PubMed.
- N. Gruyer, M. Dorais, C. Bastien, N. Dassylva and G. Triffault-Bouchet, Interaction between silver nanoparticles and plant growth, Acta Hortic., 2014, 1037, 795–800 CrossRef.
- M. Hasan, K. Mehmood, G. Mustafa, A. Zafar, T. Tariq, S. G. Hassan, S. Loomba, M. Zia, A. Mazher, N. Mahmood and X. Shu, Phytotoxic Evaluation of Phytosynthesized Silver Nanoparticles on Lettuce, Coatings, 2021, 11(2), 225 CrossRef CAS.
- F. Casillas-Figueroa, M. E. Arellano-García, C. Leyva-Aguilera, B. Ruíz-Ruíz, R. Luna Vázquez-Gómez, P. Radilla-Chávez, R. A. Chávez-Santoscoy, A. Pestryakov, Y. Toledano-Magaña, J. C. García-Ramos and N. Bogdanchikova, Argovit™ Silver Nanoparticles Effects on Allium cepa: Plant Growth Promotion without Cyto Genotoxic Damage, Nanomaterials, 2020, 10(7), 1386 CrossRef CAS.
- F. Yanık and F. Vardar, Assessment of silver nanoparticle-induced morphological, biochemical and physiological alterations in wheat roots, Ann. Bot., 2019, 9, 83–94 Search PubMed.
- F. Mirzajani, H. Askari, S. Hamzelou, M. Farzaneh and A. Ghassempour, Effect of silver nanoparticles on Oryza sativa L. and its rhizosphere bacteria, Ecotoxicol. Environ. Saf., 2013, 88, 48–54 CrossRef CAS.
- E. Agathokleous, M. Kitao and E. J. Calabrese, Hormesis: A Compelling Platform for Sophisticated Plant Science, Trends Plant Sci., 2019, 24(4), 318–327 CrossRef CAS PubMed.
- E. Agathokleous, Z. Feng, I. Iavicoli and E. J. Calabrese, The two faces of nanomaterials: A quantification of hormesis in algae and plants, Environ. Int., 2019, 131, 105044 CrossRef CAS.
- W. M. A. Sillen, S. Thijs, G. R. Abbamondi, R. De La Torre Roche, N. Weyens, J. C. White and J. Vangronsveld, Nanoparticle treatment of maize analyzed through the metatranscriptome: compromised nitrogen cycling, possible phytopathogen selection, and plant hormesis, Microbiome, 2020, 8(1), 127 CrossRef CAS.
- E. Magaña-López, V. Palos-Barba, N. Zuverza-Mena, M. C. Vázquez-Hernández, J. C. White, R. Nava-Mendoza, A. A. Feregrino-Pérez, I. Torres-Pacheco and R. G. Guevara-González, Nanostructured mesoporous silica materials induce hormesis on chili pepper (Capsicum annuum L.) under greenhouse conditions, Heliyon, 2022, 8(3), e09049 CrossRef PubMed.
- S. D. Gupta, A. Agarwal and S. Pradhan, Phytostimulatory effect of silver nanoparticles (AgNPs) on rice seedling growth: An insight from antioxidative enzyme activities and gene expression patterns, Ecotoxicol. Environ. Saf., 2018, 161, 624–633 CrossRef CAS PubMed.
- W. Mahakham, A. K. Sarmah, S. Maensiri and P. Theerakulpisut, Nanopriming technology for enhancing germination and starch metabolism of aged rice seeds using phytosynthesized silver nanoparticles, Sci. Rep., 2017, 7(1), 1–21 CrossRef CAS.
- X. Zhou, X. Jia, Z. Zhang, K. Chen, L. Wang, H. Chen, Z. Yang, C. Li and L. Zhao, AgNPs seed priming accelerated germination speed and altered nutritional profile of Chinese cabbage, Sci. Total Environ., 2022, 808, 151896 CrossRef CAS.
- P. Acharya, G. K. Jayaprakasha, J. Semper and B. S. Patil, 1H Nuclear Magnetic Resonance and Liquid Chromatography Coupled with Mass Spectrometry-Based Metabolomics Reveal Enhancement of Growth-Promoting Metabolites in Onion Seedlings Treated with Green-Synthesized Nanomaterials, J. Agric. Food Chem., 2020, 68(46), 13206–13220 CrossRef CAS.
- M. Soliman, S. H. Qari, A. Abu-Elsaoud, M. El-Esawi, H. Alhaithloul and A. Elkelish, Rapid green synthesis of silver nanoparticles from blue gum augment growth and performance of maize, fenugreek, and onion by modulating plants cellular antioxidant machinery and genes expression, Acta Physiol. Plant., 2020, 42(9), 1–16 Search PubMed.
- A. K. S. Mohamed, M. F. Qayyum, A. M. Abdel-Hadi, R. A. Rehman, S. Ali and M. Rizwan, Interactive effect of salinity and silver nanoparticles on photosynthetic and biochemical parameters of wheat, Arch. Agron. Soil Sci., 2017, 63(12), 1736–1747 CrossRef CAS.
- I. Khan, S. A. Awan, M. A. Raza, M. Rizwan, R. Tariq, S. Ali and L. Huang, Silver nanoparticles improved the plant growth and reduced the sodium and chlorine accumulation in pearl millet: a life cycle study, Environ. Sci. Pollut. Res., 2021, 28(11), 13712–13724 CrossRef CAS.
- R. Prażak, A. Święciło, A. Krzepiłko, S. Michałek and M. Arczewska, Impact of Ag Nanoparticles on Seed Germination and Seedling Growth of Green Beans in Normal and Chill Temperatures, Agriculture, 2020, 10(8), 312 CrossRef.
- W. He, Y.-T. Zhou, W. G. Wamer, M. D. Boudreau and J.-J. Yin, Mechanisms of the pH dependent generation of hydroxyl radicals and oxygen induced by Ag nanoparticles, Biomaterials, 2012, 33(30), 7547–7555 CrossRef CAS.
- Y. Li, T. Qin, T. Ingle, J. Yan, W. He, J.-J. Yin and T. Chen, Differential genotoxicity mechanisms of silver nanoparticles and silver ions, Arch. Toxicol., 2017, 91(1), 509–519 CrossRef CAS PubMed.
- M. Huang, A. A. Keller, X. Wang, L. Tian, B. Wu, R. Ji and L. Zhao, Low Concentrations of Silver Nanoparticles and Silver Ions Perturb the Antioxidant Defense System and Nitrogen Metabolism in N2-Fixing Cyanobacteria, Environ. Sci. Technol., 2020, 54(24), 15996–16005 CrossRef CAS.
- I. Slesak, M. Libik, B. Karpinska, S. Karpinski and Z. Miszalski, The role of hydrogen peroxide in regulation of plant metabolism and cellular signalling in response to environmental stresses, Acta Biochim. Pol., 2007, 54(1), 39–50 CrossRef CAS.
- S. L. Richards, K. A. Wilkins, S. M. Swarbreck, A. A. Anderson, N. Habib, A. G. Smith, M. McAinsh and J. M. Davies, The hydroxyl radical in plants: from seed to seed, J. Exp. Bot., 2015, 66(1), 37–46 CrossRef CAS.
- P. M. Nair and I. M. Chung, Physiological and molecular level effects of silver nanoparticles exposure in rice (Oryza sativa L.) seedlings, Chemosphere, 2014, 112, 105–113 CrossRef CAS PubMed.
- S. Li, S. Chen, Z. Zhang, Y. Huang, G. Li, Y. Li, X. Deng and J. Li, Short-term exposure to silver nano-particles alters the physiology and induces stress-related gene expression in Nelumbo nucifera, Plant Physiol. Biochem., 2022, 177, 38–45 CrossRef CAS PubMed.
- F. Mirzajani, H. Askari, S. Hamzelou, Y. Schober, A. Römpp, A. Ghassempour and B. Spengler, Proteomics study of silver nanoparticles toxicity on Oryza sativa L, Ecotoxicol. Environ. Saf., 2014, 108, 335–339 CrossRef CAS PubMed.
- H. Zhang, W. Du, J. R. Peralta-Videa, J. L. Gardea-Torresdey, J. C. White, A. Keller, H. Guo, R. Ji and L. Zhao, Metabolomics Reveals How Cucumber (Cucumis sativus) Reprograms Metabolites To Cope with Silver Ions and Silver Nanoparticle-Induced Oxidative Stress, Environ. Sci. Technol., 2018, 52(14), 8016–8026 CrossRef CAS PubMed.
- Z. Hossain, G. Mustafa, K. Sakata and S. Komatsu, Insights into the proteomic response of soybean towards Al2O3, ZnO, and Ag nanoparticles stress, J. Hazard. Mater., 2016, 304, 291–305 CrossRef CAS PubMed.
- H. M. M. Abdel-Aziz and M. Rizwan, Chemically synthesized silver nanoparticles induced physio-chemical and chloroplast ultrastructural changes in broad bean seedlings, Chemosphere, 2019, 235, 1066–1072 CrossRef CAS PubMed.
- W. F. Falco, M. D. Scherer, S. L. Oliveira, H. Wender, I. Colbeck, T. Lawson and A. R. L. Caires, Phytotoxicity of silver nanoparticles on Vicia faba: Evaluation of particle size effects on photosynthetic performance and leaf gas exchange, Sci. Total Environ., 2020, 701, 134816 CrossRef CAS.
Footnote |
† Si Chen and Xin Yan contribute equally to this work. |
|
This journal is © The Royal Society of Chemistry 2023 |
Click here to see how this site uses Cookies. View our privacy policy here.