DOI:
10.1039/D0QO00640H
(Review Article)
Org. Chem. Front., 2020,
7, 2815-2841
Organoselenium chemistry-based polymer synthesis
Received
28th May 2020
, Accepted 20th July 2020
First published on 20th July 2020
Abstract
Due to their unique properties, selenium-containing polymers have become one of the research focuses of polymer materials in recent years. First, selenium can transform among various states of selenoxide, selenone, and selenium free radicals, and then be restored under these conditions, which endows these polymers with multiresponsive behaviors, including responses to redox changes, light, radiation, etc. Second, these polymers show characteristic properties. Selenium-containing polymers exhibit unique multiple optical–electrical characteristics, including high photoconductivity, anisotropic thermoconductivity, high piezoelectric and thermoelectric responses, and a high refractive index. Based on these various properties, selenium-containing polymers are regarded as excellent functional polymer materials. Therefore, it is meaningful to synthesize selenium-containing polymers with various structures. This paper principally gives a summary of the organic reactions of organoselenium compounds and reviews the research on the synthesis of selenium-containing polymers via different methods, such as the use of selenium-containing monomers and their polymerization, selenium-containing initiators for polymerization, selenium-containing mediators for controlled polymerization and selenium-containing molecules for the postmodification of polymers. Moreover, applications of selenium-containing polymers are described, including their use as intrinsic high refractive index polymeric materials, oxidative- and reductive-responsive materials, dynamic exchanging polymers, selective metal adsorbents, and catalysts.
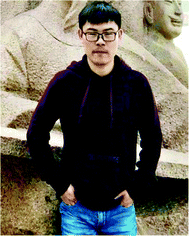 Qilong Li | Qilong Li was born in Jiangsu, China in 1993. He obtained his master's degree at Soochow University in 2015. Currently, he is a Ph.D. candidate who is interested in the synthesis of selenium-containing polymers under the supervision of Prof. Jian Zhu at the same university. |
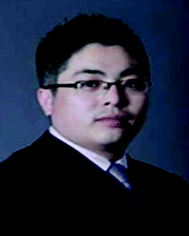 Xiangqiang Pan | Xiangqiang Pan obtained his BS degree in materials chemistry from the Huaihai Institute of Technology in 2005 and Ph.D. degree in organic chemistry from Soochow University in 2011. He is an associate professor at Soochow University. He is interested in the development of new synthetic methodologies for sulfur and selenium polymers, and new controlled/living radical polymerization processes. |
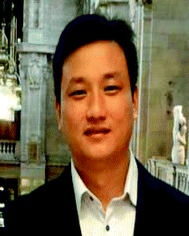 Zhengbiao Zhang | Zhengbiao Zhang received his BS degree, master's degree, and Ph.D. degree in 1997, 2000, and 2007, respectively, from Soochow University under the supervision of Prof. Xiulin Zhu. Since 2000, he has worked as an Assistant Professor (2000), Lecturer (2003), Associate Professor (2009) and Professor (2013) in Soochow University. His current research interests include the precision synthesis of polymers with well-defined molecular weights, architecture, tacticity and sequences; structure/property (function) relationship of precision polymers; recyclable and biomimetic polymers; and design and synthesis of functional polymers. |
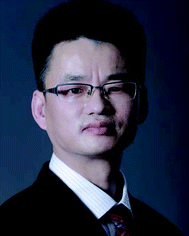 Jian Zhu | Jian Zhu received his BS degree, master's degree and Ph.D. degree in 1995, 1998, and 2004, respectively, from Soochow University under the supervision of Professor Xiulin Zhu. Since 1998, he has worked as an Assistant Professor (1998), Lecturer (2001), Associate Professor (2006) and Professor (2013) in Soochow University. His current research interests include controlled radical/cationic polymerizations and functional selenium-containing polymers. |
1. Introduction
Selenium (Se) was discovered in nature and named in 1817 by the Swedish chemist Jöns Jacob Berzelius.1,2 In the periodic table, it is classified into group VIA, along with O, S, Te and Po, as an amorphous semiconductor. Se exists in inorganic and organic forms. Inorganic selenium often has a cyclic structure with eight selenium atoms (Se8, red selenium). However, its organic form is a linear state when monomeric Se8 undergoes ring opening polymerization in the solid state at a wide range of temperatures below its melting temperature (gray selenium). Although selenium was discovered very early, it was rarely researched over the next hundred years.3,4 Inorganic selenium ushered in great developments in the middle of the twentieth century. This form of selenium was applied to ceramics, glass, metallurgy, and petrochemical engineering. In particular, inorganic selenium has been used in photoelectric devices, semiconductor devices, solar cells, infrared photoconductive materials and other advanced technologies in recent years.5–9 Organoselenium compounds have been known since 1847, when Wöhler and Siemens reported the first synthesis of ethyl selenol.10 However, organic selenium has had a sluggish research evolution because of its terrible smell, photosensitivity, frangibility to oxidation and low stability. A series of organic selenium compounds were synthesized in 1973 and they represented a milestone in the development of organic selenium chemistry.11 It is worth mentioning that Sharpless and Lauer reported a mild method for transforming epoxide to allyl alcohol in the same year.12 Diphenyl diselenide was oxidized in hydrogen peroxide and then selenoxide was formed, which generated the β elimination reaction to obtain unsaturated double bonds. This reaction enabled further development of organic selenium chemistry. After 1973, some common organic selenium reagents were commercialized. Based on these commercial materials and synthetic methods, different organic selenium compounds have been explored and have become important intermediates for the further synthesis of olefin and heterocyclic compounds.13–15 Several years later, organic selenium underwent a progression from molecules to polymers. Selenium-containing polymers were reported by Heitz and his partners in 1976.16 In their study, some selenide-styrene monomers were synthesized and then polymerized. It is worth noting that organic selenium compounds show excellent redox properties and biological enzymatic responsiveness, as well as antineoplastic,17–20 antiviral,21,22 and antioxidative23,24 activities, among other biological characteristics. Nonetheless, selenium molecules still have some drawbacks, such as toxicity, volatility and instability,25 which limit their usefulness in medicines. Therefore, selenium-containing polymers have been considered to avoid these drawbacks. Additionally, selenium-containing polymers can exhibit other outstanding characteristics, such as responsive behaviors under light and radiation stimuli, a high refractive index, and dynamic exchange.26,27 Hence, increasing efforts have been made in recent years to study the synthesis and application of selenium-containing polymers in the fields of both polymer chemistry and polymeric materials.
Here, this minireview gives a brief summary of the organic reactions of organoselenium and focuses on the synthesis of selenium-containing polymers using different methods. More specifically, these methods include using selenium-containing monomers (Se monomers) and their polymerization, selenium-containing initiators (Se-initiators) for polymerization, selenium-containing mediators (Se-mediators) for controlled polymerization and selenium-containing molecules (Se-molecules) for the postmodification of polymers. Moreover, the characteristics and applications of selenium-containing polymers are described, including being applied as high refractive index polymers, being applied in oxidative- and reductive-responsive materials, being applied as polymers with a dynamic covalent bond, and being applied as adsorbents and catalysts.
2. Organic reactions of selenium compounds
As mentioned above, organoselenium compounds have been developed for several decades. Over this period, various organoselenium compounds have been synthesized using different methods. In detail, selenium functional groups can be introduced through electrophilic, nucleophilic, or radical reactions. These approaches generally combine chemo-, regio-, and stereoselectivities with mild experimental conditions.
An electrophilic selenium reaction was discovered in the late 1950s. Electrophilic organoselenium compounds provided synthetic chemists with useful and powerful reagents (Scheme 1A). Seleno-functionalization of olefins represents an important method for the rapid introduction of vicinal functional groups, often with a concomitant formation of rings and stereocenters. The synthesis of electrophilic organoselenium compounds was thus developed, including the addition reaction of double bonds, the addition reaction involving oxygen/nitrogen/carbon-centered nucleophiles, the addition reaction involving chiral nucleophiles or substrates, selenocyclizations, double cyclization reactions, etc. Nucleophilic selenium reactions were utilized in the 1970s (Scheme 1B). Selenium nucleophiles (RSe−) are used in many types of functional-group transformations. Nucleophilic selenium reagents include important intermediates, such as selenols and selenolates, inorganic regents, and organic reagents with a variety of Se–M (M = metal) chemical bonds. In addition, selenium compounds are used in a wide variety of synthetic radical reactions as radical precursors (Scheme 1C). Various methods of synthesizing selenium radical precursors have been described, such as homolytic substitution, selenide building block and solid-phase synthesis, seleno group transfers onto alkenes and alkynes, PhSeH in radical reactions, and the catalysis of stannane-mediated reactions. The organic reactions related to organoselenium compounds have already been well summarized in different studies.28,29 Based on these organic reactions of organoselenium compounds, selenium-containing polymers have been synthesized to develop new functional polymeric materials.
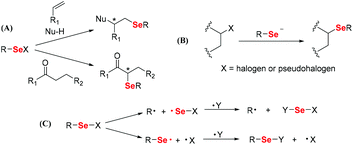 |
| Scheme 1 The reactivity of electrophilic, nucleophilic and radical reactions of organoselenium compounds.28,29 (A) Electrophilic reactions; (B) nucleophilic reactions; and (C) radical reactions. | |
3. Synthesis of selenium-containing polymers using different methods
3.1. Se monomers and their polymerization
Polymers are formed by the polymerization of monomers. Therefore, it is possible to prepare selenium-containing polymers via the direct polymerization of selenium-containing monomers (Se monomers). In addition, the selenium content in polymers is directly related to the selenium content of monomers, offering the most convenient way to control this content in polymers. Free radical polymerization, step polymerization and ring-opening polymerization are thus used to polymerize different Se monomers.
3.1.1. Free radical polymerization of Se monomers.
Vinyl monomers are commonly used monomers that can be polymerized through free radical polymerization. Therefore, selenium-containing moieties were introduced into vinyl monomers, followed by polymerization. Early investigations showed that these monomers are distinguished by the type of selenide moieties connecting the double bond of ethylene, e.g., direct or indirect. In 1972, Okamoto et al. reported that benzoyl peroxide (BPO) cannot initiate the polymerization of phenyl vinyl selenide when phenylselenide is directly connected to the double bond,30 although the corresponding sulfide readily polymerizes.31 The authors obtained a double bond addition product, methylphenylselenium dibenzoate, which was produced by the rearrangement of a tetravalent selenium compound, phenylvinylselenium dibenzoate. The reason was found to be the strong interaction of the selenium atom with free radicals.32,33 In subsequent years, Yuki and his coworkers focused on the synthesis and free radical polymerization of selenide-containing vinyl monomers with selenide moieties indirectly connected with double bonds (Scheme 2).25,34 In 1996,25 the authors synthesized p-methylselenostyrene and p-phenylselenostyrene. These two monomers can be polymerized through free radical polymerization initiated by azobisisobutyronitrile (AIBN) (showing 73% and 62% conversions, respectively) or BPO (showing 38% and 54% conversions, respectively) (Scheme 2A). These number-averaged molecular weights of polymers were in a range of 20 kg mol−1 to 150 kg mol−1. Later, in 1999, they reported the copolymerization of p-phenylselenomethyl styrene (St) with styrene, and the polymer yield remained below 10%.34 Polymers with phenylseleno groups could be further used to initiate radical polymerization of methyl methacrylate (MMA) under a high-pressure mercury lamp at room temperature via homolytic cleavage of the phenylseleno group to form graft copolymers (Scheme 2B). The polymerization was mainly initiated via a carbon-centered radical. The selenium-centered radical reversibly terminated the polymerization. However, the grafting polymerization resulted in a poor structure control, partially due to the strong interaction of selenium atoms with free radicals. After these reports, the free radical polymerization of selenide-containing vinyl monomers has rarely been investigated over the next two decades.
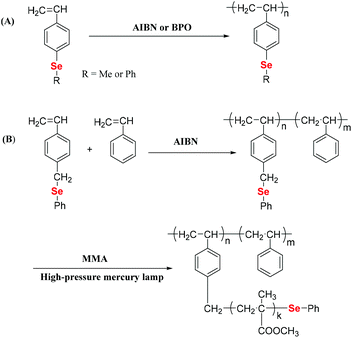 |
| Scheme 2 Free radical polymerization of Se monomers. (A) The free radical polymerization of p-methylselenostyrene and p-phenylselenostyrene. Reproduced with permission from ref. 25. Copyright 1996 Hüthig & Wepf Verlag, Zug. (B) The free radical copolymerization of p-phenylselenomethylstyrene with styrene. Reproduced with permission from ref. 34. Copyright 1999 Springer Nature. | |
In 2019, Zhu et al. reported the copolymerization of (p-phenseleno)styrene (PhSeSt) with phenyl maleimide (Scheme 3A) in a reversible addition–fragmentation chain transfer (RAFT) polymerization by using AIBN as the initiator and 2-cyanoprop-2-yl dithionaphthalenoate (CPDN) as the RAFT agent.35 This polymer shows multiple peaks in the size-exclusion chromatography (SEC) curve, indicating a loss of living radical polymerization, partially due to the side reactions caused by the phenylselenide moiety.36–38 Alternatively, Zhu and his coworkers investigated the copolymerization of phenylselenide-substituted maleimide with St, which maintained a conversion lower than 50% (Scheme 3B and C).35,39 These selenium-containing polymers showed excellent thermostability and had a high refractive index up to 1.968 at 633 nm. In another work reported by Börner and Lutz,40 the authors synthesized a sequence-controlled styrene/maleimide controlled radical polymerization platform, which was utilized to design polymers containing protected selenol fragments at precisely defined positions in the polymer chain. Then, the obtained selenium-containing polymer could be cyclized and allowed to undergo single-chain folding. This strategy offered a method for more complex macromolecule folding. In general, this is an effective method to obtain selenium-containing polymers via free radical polymerization of Se monomers. However, there is still a lack of examples of free radical polymerization routes due to the strong interaction of selenium atoms with free radicals.
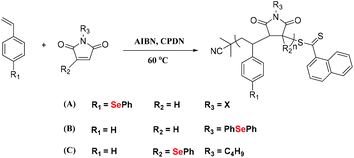 |
| Scheme 3 (A) The alternating copolymerization of (p-phenseleno)styrene (PhSeSt) and phenyl maleimide. Adapted with permission from ref. 35. Copyright 2019 The Royal Society of Chemistry; (B) and (C) the investigation of copolymerization of St with maleimide modification by the phenylseleno group at different positions. Adapted with permission from ref. 35. Copyright 2019 The Royal Society of Chemistry; adapted with permission from ref. 39. Copyright Open Access. | |
3.1.2. Stepwise polymerization of Se monomers.
Stepwise polymerization is an important tool for the preparation of polymers. Most selenium-containing polymers in the literature have been synthesized via step polymerization of Se monomers. Prince and Bremer investigated the polymerization of dibromomethane and sodium selenide. The corresponding polymer was obtained as a white powder in 1967,41 and the selenium content in the obtained polymer reached 85 wt%. Three years later, Kroll et al. reported many kinds of selenium-containing polymers, including Se-polyester, Se-polyurea and Se-polyurethane.42 These selenium-containing polymers were synthesized by the step polymerization of bis(2-hydroxyethyl)selenides or bis(2-aminoethyl)selenides with different diisocyanate, paraphthaloyl chloride, anhydride and epoxy compounds. In 2005, a selenium-containing moiety was introduced into a polyimide (PI) by Turgay.43 The general processes of synthesizing these selenium-containing PIs are the two-step polycondensation of a diamine and dianhydride, followed by thermal imidization or one-step polycondensation of the diamine and dianhydride, and finally their chemical imidization.44–48 Ueda et al. reported the synthesis of selenophene-containing PIs to increase the refractive index (RI) of polymers (Scheme 4A).49 Selenophene-containing diamine was used as the selenium-functionalized monomer for the synthesis of the PIs. The obtained PIs showed RIs as high as 1.760 at 633 nm. Zhu and his coworkers synthesized selenide-containing PIs (Scheme 4B) with selenide directly connected in the main chain.50 Bis(p-aminophenyl)selenide (BAPSe) diamine and 1,1′-bis(4-(3,4-dicarboxylbenzoyloxy)phenyl)selenide dianhydride (BDPSD) were employed as monomers, which allow the coexistence of selenide with both the diamine and dianhydride. Therefore, these PIs show higher selenium contents and RIs than the selenophene-containing PIs in Ueda's work. PIs with RIs as high as 1.968 at 633 nm were obtained in such a manner. However, these aromatic PIs showed poor solubility, which limited their processability for their potential applications. Furthermore, the authors aimed to increase the solubility of the previously reported aromatic PIs by introducing a trifluoromethyl group (CF3), which has a large free volume.51 The CF3-containing diamine monomer bis(3-trifluoromethyl-4-aminophenyl)selenide (BTFAPS) was synthesized, and 4,4′-(hexafluoroisopropylidene)diphthalic anhydride (6FDA) was chosen as the dianhydride (Scheme 4C).52 Then, two-step polycondensation was performed. As a result, these PIs exhibited better solubility and higher RIs up to 1.831 at 633 nm. In addition to Zhu's work, You et al. reported selenide-containing PIs that can enhance the refractive indices in the visible and near-infrared regions (Scheme 4D).53 These works indicate that the introduction of chalcogen atoms in the polyimide backbone is a promising method for improving the refractive index of thermally stable optical materials, specifically those employed in advanced sensor and imaging applications.
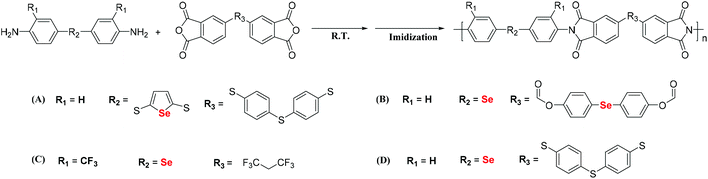 |
| Scheme 4 The structures of selenium-containing polyimides. (A) Selenophene-containing PIs. Adapted with permission from ref. 49. Copyright 2009 Wiley Periodicals, Inc. (B) Some novel selenide-containing PIs that allow selenide to coexist with both diamine and dianhydride. Adapted with permission from ref. 50. Copyright Open Access. (C) CF3-containing PIs. Adapted with permission from ref. 52. Copyright 2019 Elsevier Ltd. (D) Selenide-containing PIs. Adapted with permission from ref. 53. Copyright 2019 American Chemical Society. | |
In addition to the stepwise polymerization of PIs, Zhu and Pan introduced a novel addition reaction of electrophilic selenium reagents and sulfonyl chloride to form a selenium-containing polymer (Scheme 5).54 The obtained polymer display an increased sensitivity to oxidation, pH, and light. Xu and his coworkers have reported several works that are focused on selenide functional polymers.55–63 These selenium-containing polymers were synthesized by a general route. Dihydroxy diselenide/selenide reacted with diisocyanate as the middle Se segment, and then a reactive polymer was added into the oligomer Se segment to form the final selenium-containing polymers. These polymers could self-assemble into micelles and were responsive to redox stimuli. Compared with other redox-responsive aggregates, diselenide-containing block copolymer aggregates were responsive to both oxidants and reductants, even in a solution with a very low concentration under mild conditions. When the reactive polymer or Se segment is replaced by other polymers or segments, it will realize different properties. Chen et al. also reported similar results that showed the highly efficient cytoplasmic delivery of anticancer agents.64 In short, stepwise polymerization shows some advantages in the synthesis of polymers; however, there are still some problems. First, the molecular weight and polymerization processes are not controllable. Thus, the structures of polymers are complicated, which limits their potential applications.
 |
| Scheme 5 The novel addition reaction of electrophilic selenium reagents and sulfonyl chloride. Reproduced with permission from ref. 54. Copyright 2019 The Royal Society of Chemistry. | |
3.1.3. Se monomers in ring-opening polymerization.
In recent years, because of its high efficiency, ring-opening polymerization has become a prominent research area.65–70 In particular, the method is used in the synthesis of polyester materials, including β-butyrolactone, γ-butyrolactone, ε-caprolactone, etc.71,72 Accordingly, some selenium-containing polymers were also prepared in this way with Se monomers. Zhu and Pan focused on this work in 2017 and performed some systematic studies. First, in 2017, they employed γ-butyroselenolactone to react with amine-containing compounds (Scheme 6).73 The amine group was used as a ring-opening agent to realize the ring-opening reaction of γ-butyroselenolactone in order to form selenol. The conversions were approximately 80% and 20% when 15 and 1.5 equiv. of diethylamine were used, respectively. The selenium-containing polymer could be prepared after oxidation of the selenol compound under mild conditions. In their work, several types of polymer architectures were obtained, specifically linear, cyclic, and hyperbranched structures. In addition to the ring-opening reaction of the amine and γ-butyroselenolactone, the alcoholysis of γ-butyroselenolactone has been reported in 2018 (Scheme 6).74 This approach was a convenient and highly efficient reaction protocol for preparing diselenide polyesters, both linear and crosslinked polymers. Then, the authors employed the partial hydrolysis of poly(2-ethyl-2-oxazoline) with free imine in the polymer chain to perform the aminolysis of γ-butyroselenolactone.75 The obtained selenium-containing polymer can be used for waterborne electrospinning, which shows temperature and photoresponsiveness. The crosslinked polyester network was reprocessable due to the characteristic dynamic bond behavior of diselenide.76,77 These strategies could also make use of bio-derived macromolecules, which will be explored in future research. Lang et al. investigated the ring-opening polymerization of a selenium-containing lactone with a macroring via alcoholysis under lipase catalysis.78,79 This synthetic methodology will expand the scope of functional polycarbonates and advance the development of selenic biomaterials in practical applications. Based on γ-butyroselenolactone and selenium-containing lactones with macrorings, Zhang and coworkers performed research on alternating copolymerization of γ-butyroselenolactone with epoxides.80 The alternating degree and regioregularity of copolymers were greater than 99%. The results indicated that the polymer had well-defined structures and selenium in each repeating unit. However, all these works concentrated on the selenium element existing in the main chain of polymers. Li et al. reported ring-opening polymerizations of another selenium-containing lactone that reacted with polyethyleneglycol (PEG) to form a copolymer with the selenium moiety in the side chain of the obtained copolymer.81 In the PB solution, these copolymers formed micelle-like nanoparticles and showed great potential as a novel kind of reactive oxygen species-responsive nanoplatform.
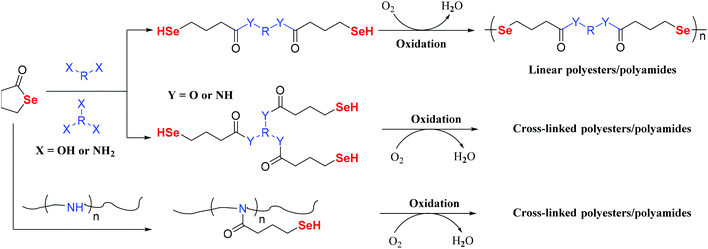 |
| Scheme 6 The ring-opening polymerization of γ-butyroselenolactone via amine or oxhydryl compounds. Adapted with permission from ref. 73 and 75. Copyright 2017 and 2018 American Chemical Society; adapted with permission from ref. 74. Copyright 2018 The Royal Society of Chemistry. | |
The ring-opening polymerization of ε-caprolactone (CL) is a general method for preparing polycaprolactone (PCL).82 The outstanding biodegradable properties of this polymer have attracted much attention.83–86 However, common PCLs tend to crystallize and lack functionalization, which limits their wide applications.87 Thus, modifying PCL to decrease its crystallinity and introduce a functionality is an effective method to improve its performance.88–90 Lang et al. reported the ring-opening polymerization of CL by using dihydroxy diselenide as an initiator.91,92 The obtained selenium-containing polymers showed a good redox response and could self-assemble into micelles to deliver an anticancer drug. In addition, because of the dynamic bond properties of the Se–Se bond, Se-PCL has been proven to be a dynamic polymer in polymer alloys.93 Li et al. described the oxidation and elimination of P(CLSePh-b-PEG) to afford the block copolymer of PEG and unsaturated PCL under very mild conditions with yields in the range of 55–70%.94 Zhu et al. reported the synthesis and direct ring-opening polymerization of phenylseleno-caprolactone (CLSePh) with almost 100% conversion (Scheme 7).95 The carbon–selenium bond in the obtained phenylseleno-caprolactone polymer (PCLSePh) could be oxidatively degraded by m-CPBA to form a new carbon–carbon double bond in the polymer, which offered a way to further modify the PCL-based polymer. The phenylselenide in the side chain could also be used as a macroinitiator to initiate the polymerization of St under UV irradiation, resulting in the graft copolymer of PCL-g-PSt. In addition, the chain structure of the polymer could be adjusted via the copolymerization of CLSePh with CL. Therefore, this work introduced an unsaturated carbon–carbon double bond, which could allow further functionalization of PCL and adjust the properties of this polymer. These high-efficiency ring-opening polymerizations of Se monomers have enriched the types of selenium-containing polymers and can be used in many fields.
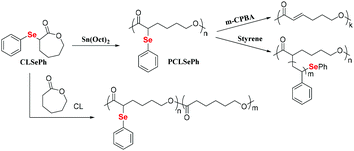 |
| Scheme 7 The ring-opening polymerization of phenylseleno-caprolactone. Adapted with permission from ref. 95. Copyright 2019 The Royal Society of Chemistry. | |
3.2. Se initiators for polymerization
The strength of the Se–Se bond is weaker than that of the S–S bond and C–C bond (Se–Se, 172 kJ mol−1; S–S, 240 kJ mol−1; and C–C, 346 kJ mol−1),96 which results in easier cleavage of the Se–Se bond compared to that of the other two bonds under various stimuli, such as oxidation, reduction and light to form seleno radicals. In some polymerization systems, monomers can be initiated via seleno radicals to obtain Se-terminal polymers. Moreover, the terminal Se (C–Se bond) can be eliminated by a redox reaction. Diphenyl diselenide (DPDSe), the most accessible aryl diselenide, was the first one studied. In 1997, Yuki and his coworkers reported the DPDSe-initiated free radical polymerization of St under irradiation with a high-pressure mercury lamp at room temperature, which showed a maximum conversion of 48%.97 The radical polymerization of methyl methacrylate (MMA) initiated by DPDSe was also realized in 2001,98 and the phenylseleno group, which existed in the terminus of the polymer, was eliminated to form a carbon–carbon double bond. However, the polymerization performance was not very good, e.g., a low monomer conversion (<50%), a low number-average molecular weight (Mn) (<5000) and a wide molecular weight distribution (1.6–2.1). In these polymerizations, DPDSe not only serves as a photoinitiator but also functions as a chain transfer agent.98 In 2000, Yuki employed p-phenylselenomethylstyrene, which was mentioned in section 3.1.1, for homopolymerization under a high-pressure mercury lamp and formed a hyperbranched polystyrene-like polymer.99 However, the detailed polymerization behavior and properties of the polymer are still worth investigating. These excellent works by Yuki and his coworkers illustrate that the cleavage of the Se–Se bond or C–Se bond for forming seleno radicals is an effective method for the polymerization and chain extension of typical vinyl monomers, e.g., St or MMA. However, the reaction conditions still should be optimized. Zhu et al. reported diselenide-mediated controlled radical polymerization under visible light irradiation by optimizing the structure of substituted diphenyl diselenide (Scheme 8).36 The authors synthesized five diselenides with different substituent groups on the phenyl ring and employed them to initiate the polymerization of St. The UV-vis spectrum was used as a key measurement to determine a suitable light source for photopolymerization. After optimizing the substituent structures, the authors found that DmXDSe with methyl substituents at the ortho-position could achieve controlled radical polymerization of St under visible light irradiation with approximately 75% conversion. The molecular weight distribution of the polymer was narrower than 1.30. The polymer showed high chain end fidelity (almost 100%), which could be efficiently converted to the carbon–carbon double bond via oxidation. The authors further expanded the light wavelength of photopolymerization of St from a high-pressure mercury lamp to blue light (460 nm) by using FVPDSe as the mediator. FVPDSe contains two double bonds and a Se–Se bond, which can be simultaneously used as both the polymerization mediator and comonomer.100 Crosslinked selenium-containing polymers have been prepared in a controlled way. Such crosslinked copolymers could be degraded by oxidation due to the selenide-containing crosslinker.
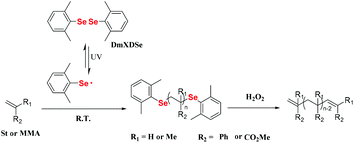 |
| Scheme 8 DmXDSe-initiated radical polymerization of St and MMA and the chain terminal oxidation of the obtained polymer. Adapted with permission from ref. 36. Copyright 2015 The Royal Society of Chemistry. | |
The authors also investigated the synthesis of hyperbranched polystyrene (PS) utilizing p-phenylselenomethylstyrene (they called it phenyl(p-vinylbenzyl)selenide (PVBS)) as an inimer under ultraviolet irradiation (Scheme 9) with approximately 76% conversion.101 Because of the homolysis of the benzyl carbon–selenium bond under UV irradiation,102,103 when the CH2–Se bond of PVBS is cleaved, two radicals are generated. Hyperbranched copolymers and homopolymers have been synthesized by copolymerization with St or homopolymerization of PVBS via self-condensing vinyl polymerization (SCVP).104–107 These reports showed that the photopolymerization of vinyl monomers containing a selenide moiety could be used to prepare graft polymers or hyperbranched polymers. In summary, the key point of the selenium-containing initiator initiating the polymerization is the Se–Se bond or C–Se bond cleavage forming seleno radicals under light, redox control, etc. The process shows a mechanism of an iniferter108–111 and can promote the functionalization of the chain terminal of the polymer for the next reaction; however, the molecular weight distribution of the obtained polymers is still broad (usually >1.3), and the living polymerization is not good.
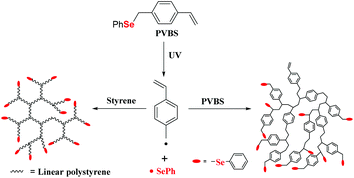 |
| Scheme 9 PVBS as an inimer under UV irradiation to copolymerize with St and homopolymerize itself. Adapted with permission from ref. 101. Copyright 2013 Wiley Periodicals, Inc. | |
3.3. Se mediators for controlled polymerization
Dithiocarbonate compounds as chain transfer agents (CTAs) are added in reversible addition–fragmentation chain transfer (RAFT) polymerization, which is a kind of reversible deactivation radical polymerization (RDRP) that is widely used in the controllable polymerization of vinyl monomers.109,110,112–121 Diselenocarbonate compounds, because of their similar structure to that of dithiocarbonate, were also employed in RAFT polymerization to obtain selenium-containing polymers. In 2008, Lee and his coworkers reported the synthesis of diselenophosphates, named P1 and P2. The authors performed the polymerization of St using these two compounds as the mediators at 110 °C with a thermal initiator (Scheme 10A).122,123 When the polymerization reaction was performed for 5 h, approximately 60% conversion could be achieved. A similar structure of phosphinodithiolate only realized a 0.9% conversion. Compared with the common polymerization of St, the polymerization behavior showed that the molecular weight increased with the conversion. However, the experimental molecular weight deviated greatly from the theoretical molecular weight, and the polymer had a wide molecular weight distribution. This result indicated that P1 and P2 actually worked for a while; however, the process was uncontrollable. Recently, Zhu et al. modified the structure from the substituted phenyl group in P1 and the tertiary butyl group in P2 to a benzyl group (Scheme 10B).124 Similarly, they added P3 into the polymerization system of St at 80 °C with the thermal initiator AIBN. When the polymerization reaction was performed for 7 h, 45.8% conversion could be achieved. The results showed more controllability than that of common radical polymerization. Furthermore, photoinitiation polymerization with UV was used to replace AIBN at room temperature to realize a 42.3% conversion. The polymerization behavior showed that the molecular weight linearly increased with the conversion and that the theoretical molecular weight was close to the actual molecular weight. In addition, the polymerization mechanism was proven to involve an iniferter rather than a RAFT mechanism (Scheme 10C).124 The structural analysis indicated that the selenide moiety existed at the end of the polymer chain and could be further modified. Therefore, X. L. Zhu's group focused on diselenocarbonate compounds and performed some systematic studies. In 2013, a cyclic diselenocarbonate compound (P4) was synthesized and used as a RAFT agent in the polymerization of VAc (Scheme 11A).125 Compared with the analogous dithiocarbonate, the polymerization of VAc showed controllable characteristics in the presence of compound P4. The conversion of polymerization could therefore reach 70%. The polymerization behavior showed that the molecular weight increased linearly with the conversion, but that the polymer showed a wide molecular weight distribution (1.6–2.0). The Se unit also existed at the chain end of the polymer. In the control experiment with dithiocarbonate, the polymerizations did not obey the first-order linear kinetics. Other studies have been reported that attempted to reduce the molecular weight distribution value of the VAc polymer. Noncyclic diselenocarbonate compounds with different leaving groups and similar dithiocarbonate structures were synthesized (Scheme 11B).126 Several of these compounds were added in the polymerization of VAc at 60 °C using AIBN as the initiator. When the polymerization proceeded for 8 h, it could reach approximately 68% conversion. These results illustrated that the two noncyclic diselenocarbonate compounds had the same effect as the corresponding dithiocarbonate for the polymerization behavior of VAc. In addition, the Se unit, which existed at the end of the polymer chain, reacted more easily than the S unit for subsequent modification of the polymer. In later reports, Zhu and his coworkers extended their work to triselenocarbonates. A trithiocarbonate (TTC) was synthesized, and then some or all of the sulfur atoms of TTC were replaced by selenium atoms, resulting in the products P7, P8 and P9 (Scheme 11C).127 The polymerizations of St were performed using these triselenocarbonates as mediators at 60 °C with AIBN as the initiator to realize a maximum 56.4% conversion. The sulfur analog TTC was then employed for a control experiment. The results indicated that these triselenocarbonates worked as RAFT agents and that the polymerization showed living characteristics. In detail, the molecular weight linearly increased with the conversion, and the polymer had a narrow molecular weight distribution. These results show that selenium and sulfur worked in RAFT agents. In addition, the polymerization rate decreased, and the induction period increased with an increasing selenium content. In contrast to previous polymers, in this research the Se unit existed in the middle of the polymer chain based on NMR and UV-vis analyses.
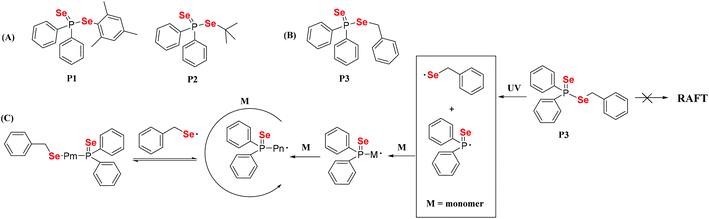 |
| Scheme 10 (A) The structures of diselenophosphates P1 and P2. Reproduced with permission from ref. 122. Copyright 2008 Elsevier Ltd; (B) and (C) the structure of diselenophosphate P3 and the mechanism of the polymerization of St with P3 under UV irradiation. Reproduced with permission from ref. 124. Copyright 2012 Wiley Periodicals, Inc. | |
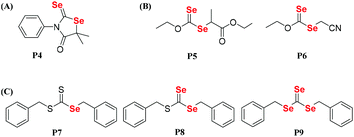 |
| Scheme 11 The chemical structures of (A) P4. Reproduced with permission from ref. 125. Copyright 2013 Wiley Periodicals, Inc.; (B) P5 and P6. Reproduced with permission from ref. 126. Copyright 2013 Wiley Periodicals, Inc.; (C) P7, P8 and P9. Reproduced with permission from ref. 127. Copyright 2013 Wiley Periodicals, Inc. | |
In general, vinyl monomers have been divided into highly active monomers and low-activity monomers, and different active monomers exhibit different polymerization behaviors. In order to obtain low molecular weight distribution polymers, RAFT polymerization of these monomers should be mediated with different RAFT agents. Therefore, a universal RAFT agent would be valuable. In 2009, Rizzardo et al. reported their works.128–131 The universal RAFT agents were dithiocarbonate compounds, and they worked well in different polymerization systems by adjusting the pH value. In addition to the universal dithiocarbonate RAFT agents, Zhu and his coworkers developed universal diselenocarbonate RAFT agents (Scheme 12).132 First, organoselenium compounds with different types of atoms (N, O, and S) adjacent to the selenocarbonyl group were synthesized and used as mediators in the polymerization of St and VAc. This work showed that P6 could control the polymerization. Based on P6, the authors synthesized P12, P13 and P14, which worked well with St, VAc, MMA, MA, BA, PFS, NVC, and NIPAM monomers. Their conversions were 42.0%, 27.5%, 50.9%, 93.9%, 75.2%, 64.0%, 22.1% and 37.5%, respectively. As a result, P14 was chosen as a universal diselenocarbonate RAFT agent for further research. Then, a compound with a structure similar to that of P14 was synthesized to control the polymerization of St for further reaction with some functional groups.133,134 In addition to monofunctional diselenocarbonates, difunctional diselenocarbonates were synthesized by Zhu and his coworkers and used to control the polymerization of vinyl monomers.135 The Se unit existed at the two ends of the polymer chain, and the ends reacted to form a cyclic diselenide polymer. In addition to RAFT agents, diselenocarbonates were used in ATRP as pseudohalogens. Zhu et al. also presented some studies regarding these agents. In their reports, Cu[SeC(Se)N(C4H9)]2 was added as an activator into the ICAR-ATRP of an MMA monomer to control the polymerization,136 which could bring about a maximum conversion of 58.7%. In contrast, even though the catalyst concentration was decreased to 1.9 ppm, the polymerization with [Cu(SC(S)N(C4H9)2)2] could be conducted at 65 °C with a 44.6% monomer conversion in 290 min. They then, for the first time, reported the introduction of alkyl diethyldiselenocarbamate as an initiator for the ATRP system and obtained polymers end-functionalized with an organoselenium structure (Scheme 13).137 Compound P16 could control the polymerization of St with a low molecular weight distribution and a Se unit at the end of the polymer could be obtained. In conclusion, diselenocarbonates as chain transfer agents have contributed to live/control radical polymerizations, including RAFT polymerization and ATRP. The polymerization behavior showed that the molecular weight increased linearly with the conversion, the theoretical molecular weight was similar to the actual molecular weight, and the polymer had a narrow molecular weight distribution. These polymers obtained had end-functionalized organoselenium structures that could be used for postmodification.
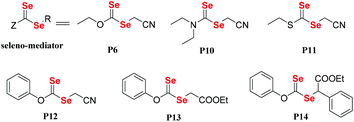 |
| Scheme 12 The chemical structures of Se compounds as RAFT agents. Reproduced with permission from ref. 132. Copyright 2013 The Royal Society of Chemistry. | |
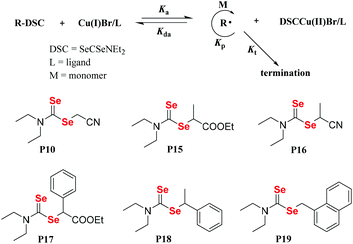 |
| Scheme 13 The structures of diselenocarbamates and the proposed polymerization mechanism for ATRP with the alkyl diethyldiselenocarbamate/CuBr/ligand system. Adapted with permission from ref. 137. Copyright 2015 Wiley Periodicals, Inc. | |
3.4. Se molecules for postmodification of polymers
Here, postmodification indicates that Se-molecules reacted directly with functionalized polymers to form selenium-containing polymers. This method tends to involve organic reactions rather than polymerization. Zhu and his coworkers focused on preparing selenium-containing functional polymers. In 2015, they reported Br-terminal polystyrene synthesized by reacting ATRP with Na2Se2 to form diselenide polystyrene.138 The obtained selenium-containing polymer had redox responsiveness and was an efficient protecting ligand for Au nanoparticles because of the susceptibility of the Se–Se bond. Then, a selenide-functionalized exchange resin was employed to react with the polymer precursor synthesized via live radical polymerization to prepare a selenium-containing polymer (Scheme 14).139 These selenium-containing polymers could have two-arm, four-arm and cyclic structures, depending on the polymer precursor, and the selenide-functionalized exchange resin could be recycled. In addition to the redox responsiveness of the Se–Se bond, it is usually used as a dynamic covalent bond to prepare self-healing materials. Some studies have been reported by Zhang and Xu. Additionally, dynamic selenium-containing polymers are another research field.140–143 The use of Se–Se, Se–S and Se–N as dynamic covalent bonds has been reported in these studies. These polymers were used as self-healing or shape memory materials. In 2017, Zhu et al. reported that a polyurethane prepolymer reacted with p-aminophenyl diselenide (DADPDSe) as a crosslinker to obtain crosslinked diselenide polyurethane (Scheme 15).77 The resulting materials not only exhibit faster self-healing properties than the corresponding disulfide polymers but also show the ability to be processed at temperatures as low as 100 °C. For high refractive index polymers, the authors employed a glycidyl methacrylate polymer as a precursor, and then postmodification was performed by the reaction between the pendent epoxy group and PhSeZnCl (Scheme 16).144 Moreover, the present route enabled control of the polymer's molecular weight and the concentration of selenium-containing structures, which in turn enabled the characteristic ability of adjustable RI and Abbe's number of final polymers. Similarly, Xing et al. used PEG-b-PMAA as a polymer precursor to react with different dihydroxy diselenides.145 These obtained selenium-containing polymers exhibited redox dual-responsive degradation. In brief, postmodification was used to synthesize functional selenium-containing polymers for further application.
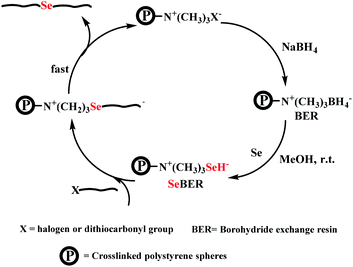 |
| Scheme 14 The diagrammatic representation of selenium-functionalized ion-exchange resin reacting with a polymer precursor. Adapted with permission from ref. 139. Copyright 2017 The Royal Society of Chemistry. | |
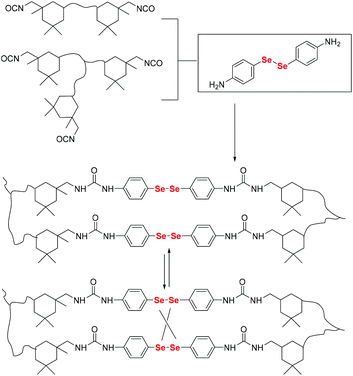 |
| Scheme 15 Synthetic procedure for obtaining a diselenide-containing poly(urea-urethane) elastomer. Adapted with permission from ref. 77. Copyright 2017 The Royal Society of Chemistry. | |
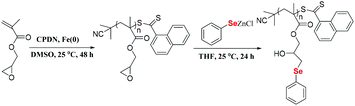 |
| Scheme 16 The synthesis of selenide-containing polymers by postmodification of Se-molecules. Reproduced with permission from ref. 144. Copyright 2016 Elsevier B.V. | |
4. Applications of selenium-containing polymers
The unique properties of selenium give selenium-containing polymers characteristic properties. First, selenium-containing polymers are proven to be good candidates for fabricating intrinsic high refractive index polymeric materials, due to the high molar refraction of the selenium group.146 Second, selenium-polymers exhibit multiple response behaviors, especially in redox responsiveness. Due to the reversible multiple structural variations from selenide to selenoxide or selenone, and then being restored to these conditions,55 they were employed as reversible sensors. Furthermore, selenium-containing polymers, which had Se–Te, Se–Se, Se–S or Se–N, were used as self-healing polymers, shape memory materials, etc., because of their weak bond energy, which is regarded as a dynamic covalent bond.76,77 The structural variation from the selenium functional group to selenium free radicals96 was used to modify the structures of the polymers. In addition to these works, selenium-containing polymers were also applied as adsorbents of heavy metal elements and catalysts. Therefore, selenium-containing polymers are regarded as excellent building blocks for functional polymer materials.
4.1. Intrinsic high refractive index polymers
Synthetic polymers with intrinsic high refractive indices (RI) are very useful in optical applications, such as advanced display devices, various lenses, optical waveguides, and diffractive gratings.147–152 RI as one of the characteristics of materials can be formulated with the Lorentz–Lorenz equation.153
In this equation, n is the RI value and [R] is the molar refractive index.
Based on this equation, polymeric materials with an intrinsic high RI can be developed by introducing moieties with a high molar refraction and a low molar volume. Selenium, an analogue of sulfur in the chalcogen group, possesses a much higher molar refraction ([R]), even compared to sulfur, i.e., [R] = 11.17 for selenide and [R] = 7.69 for sulfur.146 Such a property makes selenium-containing polymers excellent building blocks of high RI polymers. In 2009, Ueda et al. synthesized selenophene-containing PIs with a high RI of 1.759 at 633 nm,154 and they have reported many works on sulfur-containing polymers with a high RI. However, there has been no in-depth research after the report on selenophene-containing PIs. A few years later, Zhu and his coworkers expanded the structures and applications of high RI selenium-containing polymers. In 2014, Zhu et al. investigated the synthesis of hyperbranched polystyrene (PS) utilizing phenyl(p-vinylbenzyl)selenide (PVBS) as an inimer under ultraviolet irradiation (Scheme 9).101 The obtained polymer showed an RI value of 1.697 at 589 nm, which can be adjusted by simply changing the selenium content in the polymers. Then, they synthesized selenide-containing polymers by postmodification of Se-molecules (Scheme 16).144 When the content of selenium reached its maximum, the polymer had an RI value of 1.719 at 589 nm. Moreover, it could have an adjustable RI and Abbe's number for the final polymers. Among the various polymers with high RI values, selenide-containing polyimides (PIs) have received much attention due to their excellent thermal stability, low dielectric constant, and outstanding mechanical properties.155,156 In Zhu's work, novel selenide-containing PI films were designed and synthesized (Scheme 4B).50 Increasing the selenium content resulted in an increase in the RI. When selenium was present in both the diamine and dianhydride repeating units, it showed the highest RI of 1.968 at 633 nm, which is the highest intrinsic RI of a polymer ever reported. These PIs with a high RI could be used as antireflection coatings, showing almost no change in the surface morphology and providing a low reflectivity value of approximately 11% at 850 nm. However, these normal aromatic PIs show poor solubility, which limits their processability. Although aliphatic PIs show better solubility, they demonstrate poor thermostability and it is difficult to use them under special conditions.157–160 Based on these considerations, the authors aimed to increase the solubility of aromatic PIs by introducing a trifluoromethyl group (CF3) (Scheme 4C),52 which has physiochemical characteristics such as a large free volume, excellent electronegativity, a small atomic radius, and a low molar polarizability, and endows the CF3-containing PIs with some desirable properties.161 It was determined that introducing selenide could increase the RI, resulting in the highest RI value of 1.844 at a 633 nm wavelength and the lowest reflectivity of 17% at an 850 nm wavelength; thus, the antireflection coating and trifluoromethyl groups could give a prominent result to improve the solubility of PIs (Fig. 1).52
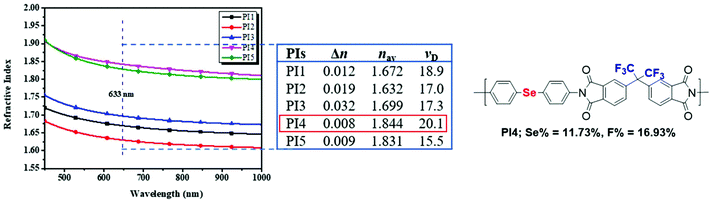 |
| Fig. 1 Refractive index curves of five polyimide films and the structure of PI4. Adapted with permission from ref. 52. Copyright 2019 Elsevier Ltd. | |
Because of the poor structural control of PIs prepared by general polycondensation, N-substituted phenyl maleimide polymers are somewhat multipurpose materials that show some good properties similar to PIs.162 Maleimides were employed as monomers to synthesize the polymers via RAFT polymerization in the pendent group (Scheme 3B and C),35 which helps realize dissolution, structure control and higher RIs. The influence factors of refractive indices (RIs) to polymers, such as the different substituent elements, molecular weights, and maleimide and selenium contents, were investigated in detail (Fig. 2). A higher molecular weight and selenium content brought about higher RI values on this basis. The maximum RI value is 1.869 at 633 nm, when it has an equal molar ratio of SePMI and PhSeSt. Based on the reversible transformation of selenide and selenoxide or selenone in a redox solution, they built cycles of RI with redox and exhibited a good redox responsibility of RI. This will be used as a potential RI responsive redox sensor. In addition to Zhu's works, You et al. reported selenide-containing PIs that can enhance the refractive indices in the visible and near-infrared regions (Scheme 4D),53 and they exhibited a refractive index of 1.778 at 637 nm. Recently, Tang and his coworkers synthesized selenium-containing polymers using a multicomponent polymerization which shows high efficiency, requires mild reaction conditions and is atom economical,163,164 where thin films fabricated by spin-coating exhibited high refractive indices of up to 1.806 at 550.0 nm.165 In summary, high efficiency could be achieved by increasing the RI of polymers by introducing a selenium group, and these high RI polymers will show positive results in the optical fields.
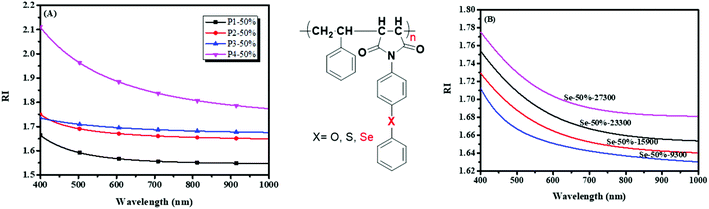 |
| Fig. 2 (A) Refractive index curves of four polymers with different substituent elements at different wavelengths; (B) refractive index curves of six polymers with different monomer ratios at different wavelengths. Adapted with permission from ref. 35. Copyright 2019 The Royal Society of Chemistry. | |
4.2. Multiresponsive materials
Selenium-containing polymers showed multiresponsive behavior with different stimuli, such as photo, irradiation, and redox reaction. Among these stimuli, the redox stimulus, e.g., oxidative- and reductive-responsiveness, was considered as the most interesting factor due to the biology and chemistry of the selenium element and the existing reactive oxygen species in the human body,166–169 based on reversible multiple structural variations from selenide to selenoxide or selenone, and then being restored under these conditions.55 Selenocysteine (Sec), known as a typical selenium source, has been widely discovered in glutathione peroxidases (GPx),170 and is involved in important biochemical processes of antioxidation.171 Lu and his coworkers172 synthesized selenium-containing polymers, called P(EGx-SeHC), which were prepared by the ring-opening polymerization of EGx-SeHC derived from selenohomocysteine (SeHC) and oligoethylene glycol. Selenohomocysteine (SeHC) is a derivative of Sec and has an N-carboxyanhydride group, which is often used to synthesize polypeptides (Fig. 3).172 The obtained polymers are promising protein mimics due to their peptidic backbones and they show helical conformations. Because of the redox character of Sec in cells, P(EGx-SeHC) showed a similar redox responsivity. The results showed that P(EGx-SeHC) could be oxidized with 20 mM H2O2 for 1 h in water at room temperature, compared to the oxidation of homocysteine derived polypeptides,173 which normally require 300 mM H2O2, 1% acetic acid and 6 h at 38 °C and are then completely restored with 30 mM dithiothreitol in 5 h. The redox process is proven to be the structural variation of selenide–selenoxide–selenide via NMR. Meanwhile, with the change in the structural variation, according to circular dichroism (CD) spectroscopy, the conformations of the polymer undergo correspondingly rapid and reversible helix–coil–helix transitions. Compared to the measurement method of CD spectroscopy in transitions of conformations, a fluorescent probe was simpler and more direct. Jing et al. synthesized selenium-containing fluorescent micromolecules with an endoplasmic reticulum (ER) structure which is irreplaceable in eukaryotic cells.174 The selenium-containing fluorescent micromolecules showed a reversible structural variation of selenide–selenoxide–selenide and exhibited emission–quenching–emission of fluorescence using a photoinduced electron transfer (PET) effect under a HClO/GSH redox cycle. Of course, other similar works have also been reported by some researchers, and most of these works focused on fluorescent micromolecules.175,176
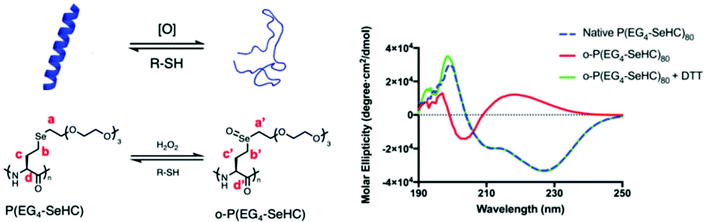 |
| Fig. 3 Oxidation and reduction-induced helix–coil transition of P(EG4-SeHC) and its spectroscopy after H2O2 oxidation followed by DTT reduction. Adapted with permission from ref. 172. Copyright 2019 The Royal Society of Chemistry. | |
On the other hand, the drug delivery and release of selenium-containing polymers by the redox response have received extensive attention. Due to selenoxide and selenone having better hydrophilicity than selenium, the self-assembly behaviors of selenide-containing polymers can be regulated by the change in redox conditions. Xu and his coworkers have reported a series of studies. In 2010, they reported an amphiphilic block copolymer with selenide moieties in the main chain where the copolymer was loaded with Doxorubicin (DOX) (Fig. 4A).177 The copolymer could undergo self-assembly to form micelles, and then it underwent a structural disassembly and a controlled release of loaded DOX in 0.1% H2O2. X-ray photoelectron spectroscopy (XPS) measurements showed a transformation from selenide to selenone, which brought about a change from the hydrophilic to hydrophobic character. Considering its mild oxidation conditions and the release of DOX, this selenium-containing block copolymer is expected to be further employed as a smart drug vehicle for the loading and release of drugs in physiological environments. After the report regarding these selenide moieties in the main chain of the polymer, they developed an amphiphilic block graft polymer PEO-b-PAA-Se with selenide moieties in the side chain (Fig. 4B).178 The micelle of the polymer which was formed via self-assembly could disassemble into fragments and release Nile red loaded under mild conditions of 0.1% H2O2, and 77Se NMR showed a transformation from selenide to selenoxide. Furthermore, when the authors added 0.5 wt% vitamin C as a reductant, these fragments quickly assembled to micelles because they were restored from selenoxide to selenide. The process demonstrated that the reversible redox could act as a potential biomaterial in drug delivery and release. Moreover, some other analogous works reported by Xu and his coworkers can be found in these literature reports.55–63
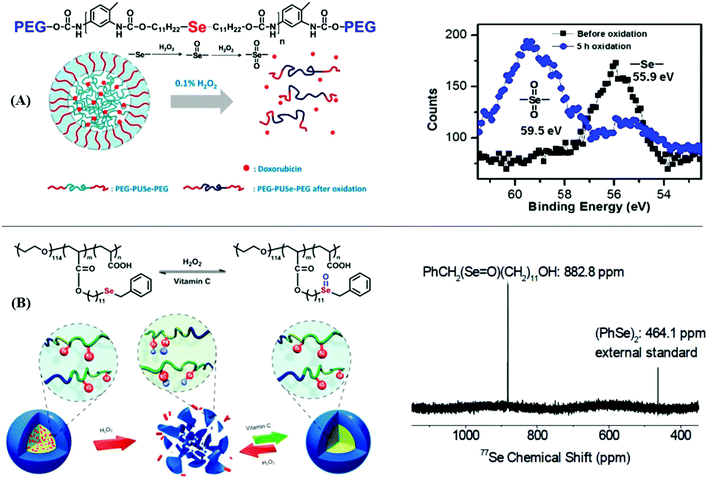 |
| Fig. 4 The drug delivery and release of selenium-containing polymers via redox response. (A) The process of self-assembly and structural disassembly of an amphiphilic block copolymer with selenide moieties in the main chain to form micelles and its XPS measurement showing a transformation from selenide to selenone. Reproduced with permission from ref. 177. Copyright 2010 The Royal Society of Chemistry; (B) the process of self-assembly and structural disassembly of amphiphilic block graft polymer PEO-b-PAA-Se with selenide moieties on the inside chain and its 77Se NMR, which showed a transformation from selenide to selenoxide. Reproduced with permission from ref. 178. Copyright 2012 The Royal Society of Chemistry. | |
Zhu et al. introduced the redox responsiveness of selenium polymers into high RI polymers to prepare responsive-RI redox sensors. These polymer structures and their high RI are reviewed in Schemes 3 and 4 and section 4.2.35,52 In the case of maleimide polymers, the maximum RI value is 1.869 at 633 nm, which is observed with an equal molar ratio of SePMI and PhSeSt. Its RI could change from 1.869 to 1.768 after being oxidized by 30% H2O2, and then being restored to 1.859 (Fig. 5).35 A similar case was taken in the reported selenide-containing polyimide.52 The RI reversible changes were realized via the chemical structural transformation of selenide–selenoxide–selenide in the redox agents, because of the difference in the molar refractive indices of selenide and selenoxide. Therefore, these polymers could be potentially used as responsive-RI redox sensors. Beside these studies, there were sporadic reports on the redox chemistry of selenium, such as reversible-tuning Krafft temperature and the reversible sol–gel transition.179,180
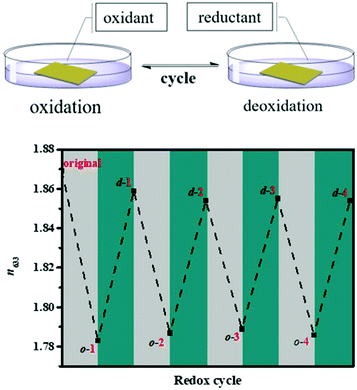 |
| Fig. 5 The RI (633 nm) changes of P4–50% during many redox cycles (o represents oxidized, d represents deoxidized and the number represents the redox time). Reproduced with permission from ref. 35. Copyright 2019 The Royal Society of Chemistry. | |
4.3. Dynamic covalent bonds
Dynamic covalent bonds are a series of chemical covalent bonds that can undergo reversible cleavage and formation under some applicable conditions, such as temperature, light, stress, etc.181,182 Obviously, these different conditions can be employed to realize relevant responsive behaviors. The key step is the control of a reversible balance, including dynamic and thermodynamic balances.183 Therefore, it is an effective route to build responsive polymers by introducing dynamic covalent bonds to some polymers. Such examples of dynamic covalent bonds in early research are summarized as imine bonds,184–186 interesterification,187–189 Diels–Alder (D–A) bonds,190–193 diselenide (S–S) bonds,194–196etc. Soon afterwards, selenide-containing (Se–X) bonds were expected to be used as dynamic covalent bonds if they had a lower bond energy than an S–S bond (S–S bond: 240 kJ mol−1).96
Analogous to the S–S bond, Xu and his coworkers paid attention to Se–Se bonds, because the Se–Se bond possesses a lower bond energy (Se–Se bond: 172 kJ mol−1; S–S bond: 240 kJ mol−1
96). In the work,76 the Se–Se bond was proven to be a novel dynamic covalent bond via a model reaction of the molecule with two symmetrical diselenide compounds under visible light (380–780 nm) or heating (70 °C)(Fig. 6A). The exchange process could be easily traced by 1H NMR and 77Se NMR, and the selenide radical was seized by 2,2,6,6-tetramethylpiperidine-1-oxyl (TEMPO). After that, they expanded the Se–Se bond to a polymer. Two diselenide-linked polystyrenes (PS–Se–Se–PS) (Mw = 90 kDa and Mw = 8 kDa) (Fig. 6B) were synthesized and used for a model exchange reaction of the polymer. A new PS–Se–Se–PS with a 49 kDa molecular weight (the sum of half of 90 kDa and half of 8 kDa) was obtained. The result showed that Se–Se can be employed as a dynamic covalent bond in the polymer and that wavelengths above 600 nm are sufficient to induce the exchange reaction. On the basis of this result, in 2015, Xu et al. reported a self-healing diselenide-containing polyurethane elastomer under visible light.140 The self-healing process was realized by the exchange reaction of the Se–Se bond in the polymer. They obtained different contents of diselenide elastomers via polymerization of different molar ratios of toluene diisocyanate (TDI), polytetramethyleneglycol (PTMG) and di-(1-hydroxyundecyl) diselenide (DiSe). A visualization method was taken up to demonstrate these self-healing properties. When the obtained elastomer bulk was cut into two small pieces and two sections were put together lightly, after lighting for 24 h with a lamp, the rebuilt elastomer could bear a maximum hanging weight of 200 g. Simultaneously, the strain–stress curves were used to quantificationally explain the effect of self-healing by mechanical stretching of the healed elastomer. The optimal result showed healing up to 72% of the uncut material after lighting for 48 h with the lamp. However, on replacing the lamp with a 457 nm 0.6 W laser, the time needed for healing was greatly shortened from 48 h to only 30 min and the breaking stress could reach 84% of the uncut material. The research provided guidance for the application of diselenide-containing polymers as self-healing materials. Therewith, Zhu and his coworkers also reported some works on diselenide self-healing materials. In 2017, they reported a postmodification reaction of a polyurethane prepolymer and p-aminophenyl diselenide or p-aminophenyl disulfide as a crosslinker to obtain a crosslinked diselenide or disulfide polyurethane.77 The obtained polyurethane bulk was cut into two small pieces and two sections were put together lightly as well. According to their self-healing property measurements, after 30 min, for the healing samples based on diselenide, 56% of the initial material was recovered; however, only 28% was recovered for the disulfide material. When the time was extended to 24 h, the recovery of the diselenide material was already 76%, while the recovery of the disulfide material only reached 43% (Fig. 7A). It should be noted that the self-healing processes were conducted without heating and special lighting. Moreover, the diselenide polyurethanes showed better reprocessability and recyclable features than disulfide polyurethanes when they were placed in plates at 100 °C with pressure (Fig. 7B). Computational and experimental investigations showed the ability of aromatic diselenides to exchange much faster than aromatic disulfides. Of course, the conclusion was matched with density functional theory (DFT) analysis results. The report not only demonstrates self-healing properties but also shows that a diamino crosslinker is an important component in cross-linked polymers. After that, in 2019, they synthesized a novel diamino diselenide crosslinker via the ring opening reaction of γ-butyroselenolactone and postmodification reaction with prepared polyurethane.197 The obtained polymer also showed better self-healing properties (almost 100%) without heating, a catalyst, special lighting or reprocessing. Other researchers expanded it to waterborne polyurethanes and the materials showed good performance.198
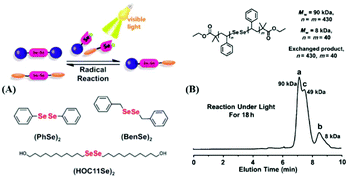 |
| Fig. 6 A visible-light-induced diselenide exchange reaction. (A) Three model compounds used in the diselenide exchange; (B) exchange reaction between two different PS–Se–Se–PS. Reproduced with permission from ref. 76. Copyright 2014 Wiley-VCH Verlag GmbH & Co. KGaA, Weinheim. | |
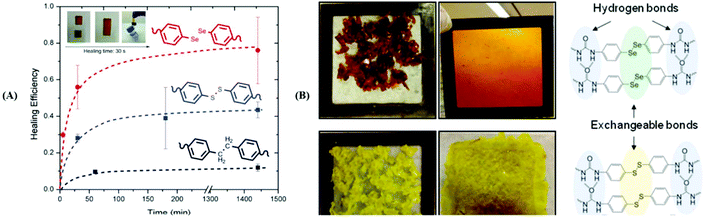 |
| Fig. 7 (A) Healing efficiency values for aromatic diselenide, disulfide and dimethylene based systems (inset: the photographic sequence of a healing process of diselenide polyurethane); (B) reprocessing of the diselenide polyurethane (up) and disulfide polyurethane (down) at 100 °C. Reproduced with permission from ref. 77. Copyright 2017 The Royal Society of Chemistry. | |
Beside their use as self-healing materials, diselenide polymers have been used in shape memory materials and other materials because of the stress–relaxation of the Se–Se bond in the dynamic exchange process. In 2017, Xu et al. reported visible light-induced shape memory polymers based on diselenides.142 The obtained polymers had different contents of diselenide via polymerization of different molar ratios of diphenylmethane diisocyanate (MDI), PTMG, DiSe and glycerol. On the grounds of measurement data, the shape memory behavior of the polymers could be adjusted by altering their thermal properties, and they showed higher contents of diselenide, which indicates better stress–relaxation and better properties (Fig. 8A). In addition, differing from thermosetting polymers, the obtained polymers exhibited plasticity under visible light. The authors combined the shape memory feature and plasticity to enable a periodic transformation between a temporary shape and the permanent shape by visible light-induced (Fig. 8B). More vividly, they employed a commercial projector as the light source to pattern the obtained materials, which had been stretched and they projected an image because the stress of the irradiated parts was relaxed and bulged to show the patterning of the material (Fig. 8C). Of course, other researchers reported some similar works,199,200 which will not be explained in detail here. Based on the shape memory property of the Se–Se bond, Xu et al. recently reported tunable structural color patterns that employed the visible-light-responsive dynamic diselenide.201 In their work, they prepared multiple complex patterns via a white LED and light from a commercial projector. Moreover, these patterns showed a high resolution. The key points of the work were the birefringence phenomenon and the stress–relaxation of stretched selenium-containing polymers under polarized light. Furthermore, according to Xu's report, the dynamic Se–Se bond has been researched in cancer therapy.202 The mechanism of action was deemed to disrupt the antioxidant system in cancer cells via the formation of Se–Se bonds inhibiting the activity of the enzyme irreversibly. Some detailed works have been reported. First, two small molecule model reactions were conducted to prove that the Se–N bond in a synthesized small molecule could attack Se in the Se–S bond in thioredoxin reductase (TrxR) to form a new Se–Se bond in a physiological environment; then the amphiphilic block copolymer micelles with a small molecule Se–N bond were reacted with TrxR. The results demonstrated a novel pathway for anticancer research, focusing on the mechanism of selenium-containing dynamic covalent bonds.
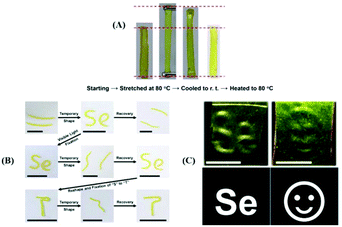 |
| Fig. 8 (A) Visual illustration of the shape memory behavior; (B) process of the shape memory behavior and visible light-induced reshaping of the permanent shape; (C) patterning of the synthesized materials utilizing a commercial projector as the light source. Reproduced with permission from ref. 142. Copyright 2017 American Chemical Society. | |
In addition to the Se–Se bond, the Se–S bond,203 Se–N bond,141 and Se–Te bond were researched as dynamic covalent bonds because of their weak bond energies (Se–S bond: 203 kJ mol−1, Se–N bond: 193 kJ mol−1 and Se–Te bond: 28 kJ mol−1).96 Some systematic works have been reported by Xu and his coworkers. The Se–S bond was formed via the exchange reaction of the S–S bond and Se–Se under UV, and it could revert to the S–S bond and Se–Se bond when it was irradiated with visible light.204 The Se–N bond could be eliminated by heating or adding acid after its formation via the reaction of phenylselenium bromide and pyridine derivatives.205 Similarly, the Se–Te bond was prepared by a metathesis reaction between diselenides and ditellurides, which could be manipulated with the presence or absence of visible light.206
In particular, due to their weak bond energies, alkyl C–Se bonds were also regarded as dynamic covalent bonds under various stimuli, such as oxidation, reduction and light, to form seleno radicals, which were applied in the chain structure modification of polymers. The chain structures of the polymers are considered to have a crucial relationship with their properties. Some changes in the properties of polymers should adjust their chain structures, such as chain growth and modification of the configuration. Selenium-containing polymers could realize the adjustment of chain structures under mild stimulation, especially under light, based on a structural variation from the selenium functional group to the selenium free radicals. As described in Scheme 2B,34 the obtained selenium-containing polymer could be further used to initiate the radical polymerization of MMA under light irradiation to cause chain growth by hemolytic cleavage of the CH2–Se bond to form a selenium free radical. The polymerization was mainly initiated by a carbon-centered radical and a selenium-centered radical that could reversibly terminate the polymerization. A similar work reported the synthesis of a block polymer of St and MMA using PSt obtained from diselenide mediated polymerization as a macroiniferter.207 Specifically, according to Zhu's report,135 when the polymerization was mediated by difunctional diselenocarbonates (Se-mediators), the Se unit existed in the two ends of the polymer chain. After the aminolysis reaction, the terminal Se units of both chain ends were converted to selenol, and then the selenol spontaneously underwent a high-efficiency coupling reaction to generate the multiblock or monoblock diselenide-containing cyclic polymer depending on the polymer concentration (Fig. 9). These studies indicated that selenium-containing polymers were good precursors for further chain structure modification because of the weak bond energy of Se–C and Se–Se.
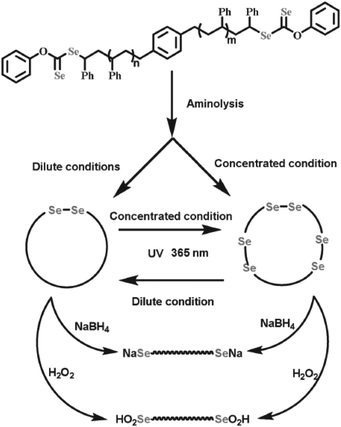 |
| Fig. 9 The process of generating a diselenide-containing cyclic polymer via aminolysis reaction of difunctional diselenocarbonates in the two ends of the polymer chain and terminal selenol coupling reaction. Reproduced with permission from ref. 135. Copyright 2016 WILEY-VCH Verlag GmbH & Co. KGaA, Weinheim. | |
In summary, polymers with these dynamic covalent bonds showed some analogical features with diselenide-containing polymers. Thus, it can be seen that dynamic covalent bonds can endow the polymer materials with some intelligently responsive characters and make it meaningful to seek novel dynamic covalent bonds with other unique types of responsiveness.
4.4. Adsorbents of heavy metal elements
With the progress of chemistry and electronics, abundant heavy metal elements (HMEs), including Au, Cu, Hg, Cr, Cd, Pb, etc., have been discharged into water and soil. HMEs are deemed unhealthy to flora and fauna and polluted water destroys soil grown fruits. To solve these problems, scientists developed selenium-containing materials as sorbents to adsorb these HMEs, according to Pearson's Hard and Soft, Acids and Bases (HSAB) principle.208–210 In the principle, soft Lewis acids will preferentially react with soft Lewis bases, and hard Lewis acids more easily react with hard Lewis bases. As a result, selenium-containing materials that are deemed as soft bases are excellent sorbents to these HMEs, which are regarded as soft acids.
In these selenium-containing materials, selenium nanoparticles (Nano Se) were easier to employ, because of their high specific surface area. In 2011, Bai et al. reported a work of removing Cu2+ from wastewater using Nano Se.211 In their work, a good adsorption effect to Cu2+ was shown and the maximum value was 0.89 g g−1. With the process of adsorption, they obtained Cu2Se, which is a crucial semiconducting material. In addition to this report, Jain and his coworkers focused on the effluent treatment and the recovery of HMEs. The main studies were on the adsorption of Zn2+, Cu2+, Cd2+ and others via the biogenic elemental selenium nanoparticles (BioSeNPs), which were produced by the reduction of selenite (SeO32−) in the presence of anaerobic granules from a full-scale upflow anaerobic sludge blanket reactor treating wastewater from a paper mill. In detail, first, the maximum adsorption capacity observed was 0.06 g g−1 for Zn2+ when the pH was as low as 3.9.212 Second, the adsorption capacity of Cu2+ reached 78% at pH 5.2.213 Third, the maximum adsorption capacity observed was 176.8 mg of Cd2+ adsorbed per gram of BioSeNPs.214 These studies can be exploited to develop a novel heavy metal removal process based on BioSeNPs.
Analogous to Nano Se, selenium-containing polymers have been developed as adsorbents of HMEs in recent years. Zhu et al. synthesized selenium-containing phenolic resin spheres (Se–RS) with a controlled selenium content through the extended Stöber method (Fig. 10A).215 The Se–RS exhibited an adsorption capacity of Au3+ and Pd2+, existed in an aqueous solution, and had maximum Au3+ and Pd2+ uptake values of 25.17 mmol g−1 and 2.19 mmol g−1, respectively (Fig. 10B).215 Even with a 0.4 ppb concentration of Au3+ in solution, the Se–RS worked well. Meanwhile, the adsorbed Au3+ takes the form Au0, which is noble via the reduction reaction of Se–RS (Fig. 10C).215 This indicates that Se–RS could be used in the treatment of electronic waste sewage to effectively transform Au3+ to gold, which will not only recycle water pollutants, but also bring about economic benefits. After that, in 2020, Xu et al. employed a novel selenium-containing polyurethane to extract gold from electronic wastewater, where the maximum adsorption capacity was 0.802 g per gram of Se-polyurethane.216 Based on the results, first the authors designed the equipment by combining a plastic tube filled with a synthesized polymer and a peristaltic pump to simulate the dynamic extraction process in industrial treatment (Fig. 11A).216 The result was further characterized by ICP-MS. 99.99% of Au3+ was extracted with this system within 5 min, exhibiting a high throughput and reliable effectiveness. Then, in order to obtain bulk gold, they improved the route by preparing Se-foam via crosslinking and foaming technology (Fig. 11B).216 Of course, compared to the dynamic extraction process, the Se-foam is more convenient for operation and transportation. In conclusion, selenium-containing materials showed the expected adsorption properties for HMEs due to their role as soft bases, and they are expected to make a few contributions against HEM pollution and resource depletion.
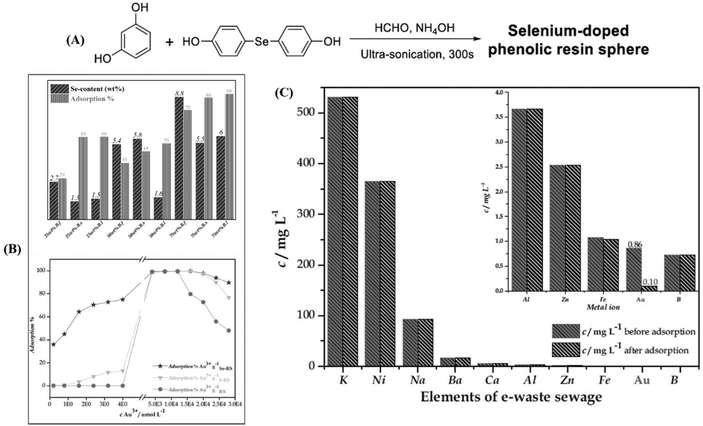 |
| Fig. 10 (A) Synthetic route of selenium-doped phenolic resin spheres; (B) the adsorption efficiency of Se–RS for Pd2+ and the adsorption behavior of Se–RS, RS and RS for Au3+ (initial ion concentration = 1.0 mmol L−1, pH = 2, volume of solution = 10 cm3, weight of dry sphere = 0.010 g); (C) gold extraction from electronic waste sewage (volume of solution = 10 cm3, pH = 5, weight of dry sphere = 0.010 g). Reproduced with permission from ref. 215. Copyright 2019 Elsevier B.V. | |
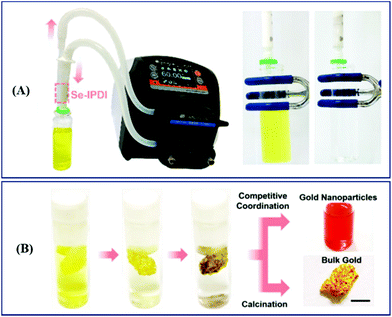 |
| Fig. 11 (A) The equipment for simulating the dynamic extraction process in industrial treatment and the color change of the Au3+ solution before and after treatment with the system; (B) procedure of gold extraction with Se-foam and posttreatments by competitive coordination or calcination. Reproduced with permission from ref. 216. Copyright 2020 WILEY-VCH Verlag GmbH & Co. KGaA, Weinheim. | |
4.5. Application as catalysts
Catalysts play a crucial role in most chemical and biological reactions.217,218 In general, transition metals are broadly studied, because these heterogeneous catalysts are easy to process and separate after the reactions.219 However, selenium-based catalysts have also been given much attention, including organoselenium compounds, polymer-loaded selenium agents and selenium-containing polymers, due to their better catalytic activity and selectivity. In the past few years, some studies involving organoselenium compounds as catalysts have been reported.220–227 Often, organoselenium catalysts could be separated through filtration, distillation or extraction, depending on the physical states of the products.228 Under some conditions, these organoselenium compounds will not work well and will have to be recycled. As a result, polymer-loaded selenium agents and selenium-containing polymers were considered as better techniques to be used as catalysts.
With the techniques of using polymer-loaded selenium agents, in 2006 Barrero et al. took solid-supported selenyl bromide, which is commercially available as a catalyst, for halogenation of the terminal isopropylidene units of different acyclic polyolefinic polyprenoids regioselectively, and the best yield of up to 96% was obtained.229 In addition to this work, Detty and his coworkers focused on a gel loaded with selenium as the catalyst. In 2008, they reported that 4-(hydroxymethyl)phenyl benzyl selenoxide as a catalyst was sequestered in xerogel, which was formed by 3-aminopropyltriethoxysilane/tetraethoxysilane to catalyze the bromination of organic substrates, such as 4-pentenoic acid, 3,5-dihydroxybenzoic acid, 1,3,5-trimethoxybenzene, N-phenylmorpholine, and N,N-dimethylaniline.230 The result showed 23 times the effects compared to its molecules, and it could be separated through filtration and reuse. Some years later, they extended the work to tetraethoxysilane xerogel with diselenide as a catalyst for the reaction from unsaturated olefin acid to bromolactone.231 Its main source as a catalyst was selenic acid, which is formed by the oxidation of H2O2, and using X-ray photoelectron spectroscopy (XPS), its +4 oxidation state was verified. Similarly, the catalyst could be reused and an approximate catalytic rate was determined. Because of the commonality of the oxidation of selenium with H2O2, Yu et al. wanted to design and prepare a polymer resin-supported organoselenium catalyst, as they expected its industrial potential.232 In their work, they found that selenium on polymers exhibited a hexavalent state, which was different from that of the selenium micromolecule. When a polymer resin-supported organoselenium catalyst was used to catalyze the oxidation reaction of cyclohexene with H2O2 in water to form trans-1,2-cyclohexanediol, which was an important industrial intermediate, the catalytic process showed that the high-valent (+6) selenium species were reduced to divalent selenium (+2). The reaction showed a 98% yield under optimal conditions (only 1 mol% catalyst). Beside the high efficiency, the polymer resin-supported organoselenium catalyst could be recycled and reused at least 5 times without deactivation. Compared with selenium micromolecules, especially RSeSeR,225,228 divalent selenium is more active, which means it could be reoxidized by air, and the reaction needs no excess H2O2. In addition to polymer-loaded selenium agents, selenium-containing polymers were expected to show a better catalytic performance and recyclability, but there were few reports. Recently, Pich and his coworkers combined poly(N-vinylcaprolactam) (PVCL) and a diselenide cross-linker to form a selenium-containing microgel to catalyze the reaction of acrolein (Fig. 12A).219 Obviously, the catalytic system combined the advantages of the microgel, which could swell, and diselenide, which could break the Se–Se bond. Its catalytic process mechanism was that acrolein and other reagents entered into the three-dimensional network of the microgel when it swelled in the solution, and diselenide was oxidized to form selenic acid, which could catalyze acrolein to obtain acrylic acid (AA) and methyl acrylate (MA) (Fig. 12B).219 Then, with the formation of selenic acid, the degree of crosslinking declined and the product could return from the microgel. Of course, the content of AA and MA depended on the reaction solvent. In summary, the selenium species were employed as catalysts in many reactions, and they worked well. Specifically, selenium-containing polymers with a better catalytic performance and recyclability will be the goal for realizing universality under different conditions, due to the multiple structures of polymers.
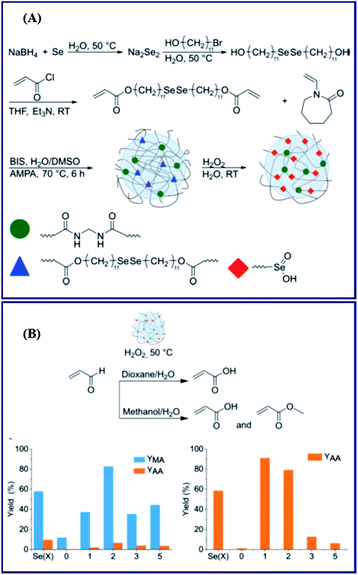 |
| Fig. 12 The synthesis route of selenium containing PVCL microgels and it catalyzing the oxidation reaction of acrolein with H2O2, resulting in AA and MA. Reproduced with permission from ref. 219. Copyright 2019 WILEY-VCH Verlag GmbH & Co. KGaA, Weinheim. | |
5. Conclusion and outlook
In summary, this paper gave a brief overview of the organic reactions of organoselenium and summarized works on the synthesis of selenium-containing polymers using different methods. More specifically, these methods involve Se monomers and their polymerization, Se-initiators for polymerization, Se-mediators for controlled polymerization and Se-molecules for the postmodification of polymers. The obtained selenium-containing polymers showed outstanding and unique properties, including redox characteristics, high refractive indices, dynamic responsiveness and the characteristics of adsorption and catalysis which have been widely used in different fields, especially in the fabrication of bio-based and high performance materials based on their multi-responsive properties.
In addition to the reported research, there are certainly still some meaningful and challenging studies to perform on selenium-containing polymers. First, the amount, structure and position of selenium containing moieties in these polymers would dominantly determine their properties. Thus, a further understanding of the chemistry of the synthesis of selenium containing polymers with a precise control of the polymer structure, especially the amount, structure and position of selenium moieties in polymers, is still one of these research studies focused in this field. Second, the excellent multiple and sensitive responsiveness of selenium containing polymers to different stimuli makes them unique candidates for the fabrication of a wide range of functional materials. New application fields, such as oxidant and reductant sensors, photonic crystals, responsive gels etc., could be explored. Last but not least, the widely explored catalysis based on selenium compounds in organic chemistry could be developed further by introducing a related structure into the polymer for improving its catalytic efficiency and endowing it with the recycling property.
Conflicts of interest
The authors declare no conflict of interest.
Acknowledgements
This work was supported by the National Natural Science Foundation of China (No. 21871200 and 21925107), the Priority Academic Program Development (PAPD) of Jiangsu Higher Education Institutions, the Educational Commission of Jiangsu Province of China (17KJA150008) and the Program of Innovative Research Team of Soochow University.
References
- J. Berzelius, Ueber das selenium, J. Chem. Phys., 1817, 21, 342 Search PubMed.
- R. Boyd, Selenium stories, Nat. Chem., 2011, 3, 570 CrossRef CAS PubMed.
-
J. C. Bailar, Comprehensive inorganic chemistry, Pergamon Press, Oxford, 1973 Search PubMed.
-
F. A. Cotton and G. Wilkinson, Advanced inorganic chemistry, John Wiley&Sons, 4th edn, 1980 Search PubMed.
- K. A. Ann Mary, N. V. Unnikrishnan and R. Philip, Ultrafast optical nonlinearity in nanostructured selenium allotropes, Chem. Phys. Lett., 2013, 588, 136–140 CrossRef CAS.
- K. A. Ann Mary, N. V. Unnikrishnan and R. Philip, Cubic to amorphous transformation of Se in silica with improved ultrafast optical nonlinearity, RSC Adv., 2015, 5, 14034–14041 RSC.
- M. Nyk, D. Wawrzynczyk, J. Szeremeta and M. Samoc, Spectrally resolved size-dependent third-order nonlinear optical properties of colloidal CdSe quantum dots, Appl. Phys. Lett., 2012, 100, 041102 CrossRef.
- I. L. Bolotin, D. J. Asunskis, A. M. Jawaid, Y. Liu, P. T. Snee and L. Hanley, Effects of surface chemistry on nonlinear absorption, scattering, and refraction of PbSe and PbS nanocrystals, J. Phys. Chem. C, 2010, 114, 16257–16262 CrossRef CAS.
- A. D. Lad, P. P. Kiran, G. R. Kumar and S. Mahamuni, Three-photon absorption in ZnSe and ZnSe/ZnS quantum dots, Appl. Phys. Lett., 2007, 90, 133113 CrossRef.
- F. Wöhler and C. Siemens, Ueber das selenmercaptan, Ann. Chem., 1847, 61, 360 CrossRef.
- D. L. J. Clive, Modern organoselenium chemistry, Tetrahedron, 1978, 34, 1049–1132 CrossRef CAS.
- K. B. Sharpless and R. F. Lauer, Mild procedure for the conversion of epoxides to allylic alcohols. first organoselenium reagent, J. Am. Chem. Soc., 1973, 40, 2697–2699 CrossRef.
- T. Wirth, Organoselenium chemistry in stereoselective reactions, Angew. Chem., Int. Ed., 2000, 39, 3740–3749 CrossRef CAS PubMed.
- D. M. Freudendahl, S. A. Shahzad and T. Wirth, Recent advances in organoselenium chemistry, Eur. J. Org. Chem., 2009, 1649–1664 CrossRef CAS.
- S. A. Shahzad and T. Wirth, Fast synthesis of benzofluorenes by selenium-mediated carbocyclizations, Angew. Chem., Int. Ed., 2009, 48, 2588–2591 CrossRef CAS PubMed.
- R. Michels, M. Kato and W. Heitz, Polymere reagenzien, 5. polymere selenreagenzien, Makromol. Chem., 1976, 177, 2311–2320 CrossRef CAS.
- J. A. Sokoloski, O. L. Blair and A. C. Sartorelli, Alterations in glycoprotein synthesis and guanosine triphosphate levels associated with the differentiation of HL-60 leukemia cells produced by inhibitors of inosine 5′-phosphate dehydrogenase, Cancer Res., 1986, 46, 2314–2319 CAS.
- Z. Parandoosh, R. K. Robins, M. Belei and B. Rubaleava, Tiazofurin and selenazofurin induce depression of cGMP and phosphatidylinositol pathway in L1210 leukemia cells, Biochem. Biophys. Res. Commun., 1989, 164, 869–874 CrossRef CAS PubMed.
- T. J. Boritzki, D. A. Berry, J. A. Besserer, P. D. Cook, D. W. Fry, W. R. LeoPold and R. C. Jackson, Biochemical and antitumor activity of tiazofurin and its selenium analog (2-β-d-ribofuranosyl-4-selenazolecarboxamide), Biochem. Pharmacol., 1985, 34, 1109–1114 CrossRef CAS PubMed.
- A. Bhattacharya, S. Turowski, I. D. S. Martin, A. Rajput, Y. M. Rustum, R. M. Hoffman and M. Seshadri, Magnetic resonance and fluorescence-protein imaging of the anti-angiogenic and anti-tumor efficacy of selenium in an orthotopic model of human colon cancer, Anticancer Res., 2011, 31, 387–394 CAS.
- R. W. Sidwell, J. H. Huffman, E. W. Call, H. Alaghamandan, P. D. Cook and R. K. Robins, Activity of selenazofurin against influenza A and B viruses in vitro, Antimicrob. Agents Chemother., 1985, 28, 375–377 CrossRef CAS PubMed.
- J. J. Kris, J. A. North, R. A. Mckernan, B. K. Murray, P. G. Canonico, J. W. Huggins, P. C. Srivastava and R. K. Robins, Broad-spectrum antiviral activity of 2-beta-D-ribofuranosylselenazole-4-carboxamide, a new antiviral agent, Antimicrob. Agents Chemother., 1983, 24, 353–361 CrossRef PubMed.
- R. Prabhakar, K. Morokuma and D. G. Musaev, Peroxynitrite reductase activity of selenoprotein glutathione peroxidase: a computational study, Biochemistry, 2006, 45, 6967–6977 CrossRef CAS PubMed.
- B. K. Sarma and G. Mugesh, Glutathione peroxidase (GPx)-like antioxidant activity of the organoselenium drug ebselen: unexpected complications with thiol exchange reactions, J. Am. Chem. Soc., 2005, 127, 11477–11485 CrossRef CAS PubMed.
- T. Ando, T. S. Kwon, A. Kitagawa, T. Tanemura, S. Kondo, H. Kunisada and Y. Yuki, Synthesis and free radical polymerization of p-methylseleno- and p-phenylselenostyrenes, Macromol. Chem. Phys., 1996, 197, 2803–2810 CrossRef CAS.
- K. Tanaka and A. Saitoh, Optical nonlinearities of Se-loaded zeolite (ZSM-5): a molded nanowire system, Appl. Phys. Lett., 2009, 94, 241905 CrossRef.
- R. Ke, X. Zhang, S. Zhang, S. Li, C. Mao, H. Niu, J. Song and Y. Tian, Self-catalytic polymerization of water-soluble selenium/polypyrrole nanocomposite and its nonlinear optical properties, Phys. Chem. Chem. Phys., 2015, 17, 27548–27557 RSC.
-
T. Wirth, Organoselenium chemistry-modern developments in organic synthesis, Springer, 1999 Search PubMed.
-
T. Wirth, Organoselenium chemistry-synthesis and reactions, Wiley-VCH, 2012 Search PubMed.
- Y. Okamoto, R. Homsary and T. Yano, Reactions of phenyl vinyl selenide with benzoyl peroxide, Tetrahedron Lett., 1972, 13, 2529–2531 CrossRef.
- M. Price and B. Bremer, Selenium chemistry. II. stereochemistry of vicinal dihalide elimination, J. Org. Chem., 1967, 32, 1655–1656 CrossRef.
- Y. Okamoto, K. L. Chellappa and R. Homsany, Reactions of alkyl phenyl selenide with benzoyl peroxide, J. Org. Chem., 1973, 38, 3172–3175 CrossRef CAS.
- J. F. Lyons, C. H. Schiesser and K. Sutej, Free-radical homolytic substitution at selenium: an efficient method for the preparation of selenophenes, J. Org. Chem., 1993, 58, 5632–5638 CrossRef CAS.
- T. S. Kwon, S. Kondo, K. Takagi, H. Kunisada and Y. YuKi, Synthesis and radical polymerization of p-phenylselenomethylstyrene and applications to graft copolymers, Polym. J., 1999, 31, 483–487 CrossRef CAS.
- Q. Li, K. L. Ng, X. Pan and J. Zhu, Synthesis of high refractive index polymer with pendent selenium-containing maleimide and use as a redox sensor, Polym. Chem., 2019, 10, 4279–4286 RSC.
- C. Ding, C. Fan, G. Jiang, J. Zhang, X. Li, N. Li, X. Pan, Z. Zhang, W. Zhang, J. Zhu and X. Zhu, Diselenide mediated controlled radical polymerization under visible light irradiation: mechanism investigation and α,ω-ditelechelic polymers, Polym. Chem., 2015, 6, 6416–6423 RSC.
- T. S. Kwon, K. Takagi, H. Kunisada and Y. Yuki, Synthesis of diblock copolymers by end functional polystyrene containing phenylseleno groups as polymeric photoiniferterI, J. Macromol. Sci., Part A: Pure Appl. Chem., 2002, 39, 991–1006 CrossRef.
- T. S. Kwon, K. Takagi, H. Kunisada and Y. Yuki, Synthesis of star polystyrene by radical polymerization with 1,2,4,5-tetrakis(p-tert-butylphenyl selenomethyl)benzene as a novel photoiniferter, Eur. Polym. J., 2003, 39, 1437–1441 CrossRef CAS.
- Q. Liu, X. Lv, N. Li, X. Pan, J. Zhu and X. Zhu, Copolymerization of phenylselenide-substituted maleimide with styrene and its oxidative elimination behavior, Polymer, 2018, 10, 321 Search PubMed.
- E. Schué, A. Kopyshev, J. Lutz and H. G. Börner, Molecular bottle brushes with positioned selenols: extending the toolbox of oxidative single polymer chain folding with conformation analysis by atomic force microscopy, J. Polym. Sci., 2020, 58, 154–162 CrossRef.
- M. Prince and B. Bremer, Preparation of hexagonal polyselenomethylene, J. Polym. Sci., Part B: Polym. Lett., 1967, 5, 843–845 CrossRef CAS.
- H. Kroll and E. F. Bolton, The synthesis of selenium condensation polymers using bis(2-hydroxyethyl)selenide and bis(2-aminoethyl)selenide, J. Appl. Polym. Sci., 1970, 14, 2319–2325 CrossRef CAS.
- S. Koytepe, A. Pasahan, E. Ekinci and T. Seckin, Synthesis, characterization and H2O2-sensing properties of pyrimidine-based hyperbranched polyimides, Eur. Polym. J., 2005, 41, 121–127 CrossRef.
- E. Gubbelmans, T. Verbiest, M. V. Beylen, A. Persoons and C. Samyn, Chromophore-functionalised polymides with high-poling stabilities of the nonlinear optical effect at elevated temperature, Polymer, 2002, 43, 1581–1585 CrossRef CAS.
- D. J. Liaw, F. C. Chang, M. K. Leung, M. Y. Chou and K. Muellen, High thermal stability and rigid rod of novel organosoluble polyimides and polyamides based on bulky and noncoplanar naphthalene-biphenyldiamine, Macromolecules, 2005, 38, 4024–4029 CrossRef CAS.
- C. H. Chang, K. L. Wang, J. C. Jiang, D. J. Liaw, K. R. Lee, J. Y. Lai and K. H. Lai, Novel rapid switching and bleaching electrochromic polyimides containing triarylamine with 2-phenyl-2-isopropyl groups, Polymer, 2010, 51, 4493–4502 CrossRef CAS.
- C. Liu, L. Li, Z. Liu, M. Guo, L. Jing, B. Liu, Z. Jiang, T. Matsumoto and M. D. Guiver, Sulfonated naphthalenic polyimides containing ether and ketone linkages as polymer electrolyte membranes, J. Membr. Sci., 2011, 366, 73–81 CrossRef CAS.
- B. J. Jeon, S. W. Cha, M. Y. Jeong, T. K. Lim and J. I. Jin, Synthesis and 2nd order nonlinear optical properties of soluble polyimides bearing nitroazobenzene type chromophore pendants attached in side-on mode, J. Mater. Chem., 2002, 12, 546–552 RSC.
- N. H. You, N. Fukuzaki, Y. Suzuki, Y. Nakamura, T. Higashihara, S. Ando and M. Ueda, Synthesis of high-refractive index polyimide containing selenophene unit, J. Polym. Sci., Part A: Polym. Chem., 2009, 47, 4428–4434 CrossRef CAS.
- Q. Li, J. Zhang, X. Pan, Z. Zhang, J. Zhu and X. Zhu, Selenide-containing polyimides with an ultrahigh intrinsic refractive index, Polymer, 2018, 10, 417 Search PubMed.
- D. P. Erhard, F. Richter, C. B. A. Bartz and H. W. Schmidt, Fluorinated aromatic polyimides as high-performance electret materials, Macromol. Rapid Commun., 2015, 36, 520–527 CrossRef CAS PubMed.
- Q. Li, S. Liu, M. Xu, X. Pan, N. Li, J. Zhu and X. Zhu, Selenide-containing soluble polyimides: high refractive index and redox responsiveness, Eur. Polym. J., 2020, 122, 109358 CrossRef.
- H. Kim, B. C. Ku, M. Goh, H. C. Ko, S. Ando and N. H. You, Synergistic effect of sulfur and chalcogen atoms on the enhanced refractive indices of polyimides in the visible and near-Infrared regions, Macromolecules, 2019, 52, 827–834 CrossRef.
- X. An, W. Lu, M. Pang, Z. Zhang, X. Zhu, J. Zhu and X. Pan, One-pot cascade polymerization based on the addition reactions of electrophilic selenium reagents to alkenes, Polym. Chem., 2019, 10, 574–581 RSC.
- N. Ma, Y. Li, H. Xu, Z. Wang and X. Zhang, Dual redox responsive assemblies formed from diselenide block copolymers, J. Am. Chem. Soc., 2010, 132, 442–443 CrossRef CAS PubMed.
- W. Cao, Y. Li, Y. Yi, S. Ji, L. Zeng, Z. Sun and H. Xu, Coordination-responsive selenium-containing polymer micelles for controlled drug release, Chem. Sci., 2012, 3, 3403–3408 RSC.
- P. Han, S. Li, W. Cao, Y. Li, Z. Sun, Z. Wang and H. Xu, Red light responsive diselenide-containing block copolymer micelles, J. Mater. Chem. B, 2013, 1, 740–743 RSC.
- L. Wang, W. Cao, Y. Yi and H. Xu, Dual redox responsive coassemblies of diselenide-containing block copolymers and polymer lipids, Langmuir, 2014, 30, 5628–5636 CrossRef CAS PubMed.
- W. Zhou, L. Wang, F. Li, W. Zhang, W. Huang, F. Huo and H. Xu, Selenium-containing polymer@metal-organic frameworks nanocomposites as an efficient multiresponsive drug delivery system, Adv. Funct. Mater., 2017, 27, 1605465 CrossRef.
- L. Wang, W. Wang, W. Cao and H. Xu, Multi-hierarchical responsive polymers: stepwise oxidation of a selenium- and tellurium-containing block copolymer with sensitivity to both chemical and electrochemical stimuli, Polym. Chem., 2017, 8, 4520–4527 RSC.
- L. Wang, K. Zhu, W. Cao, C. Sun, C. Lu and H. Xu, ROS-triggered degradation of selenide-containing polymers based on selenoxide elimination, Polym. Chem., 2019, 10, 2039–2046 RSC.
- C. Sun, L. Wang, B. Xianyu, T. Li, S. Gao and H. Xu, Selenoxide elimination manipulate the oxidative stress to improve the antitumor efficacy, Biomaterials, 2019, 225, 119514 CrossRef CAS PubMed.
- J. Xia, P. Zhao, S. Pan and H. Xu, Diselenide-containing polymeric vesicles with osmotic pressure response, ACS Macro Lett., 2019, 8, 629–633 CrossRef.
- Y. Wang, Y. Deng, H. Luo, A. Zhu, H. Ke, H. Yang and H. Chen, Light-responsive nanoparticles for highly efficient cytoplasmic delivery of anticancer agents, ACS Nano, 2017, 11, 12134–12144 CrossRef CAS PubMed.
- H. Li, N. Zhao, C. Ren, S. Liu and Z. Li, Synthesis of linear and star poly(ε-caprolactone) with controlled and high molecular weights via cyclic trimeric phosphazene base catalyzed ring-opening polymerization, Polym. Chem., 2017, 8, 7369–7374 RSC.
- C. Huang, W. Chen, J. Aimi, Y. Huang, S. Venkatesan, Y. Chiang, S. Huang, S. Kuo and T. Chen, Synthesis of well-defined PCL-b-PnBA-b-PMMA ABC-type triblock copolymers: toward the construction of nanostructures in epoxy thermosets, Polym. Chem., 2018, 9, 5644–5654 RSC.
- R. S. Hewawasam, U. L. D. Inush Kalana, N. U. Dharmaratne, T. J. Wright, T. J. Bannin, E. T. Kiesewetter and M. K. Kiesewetter, Bisurea and bisthiourea H-bonding organocatalysts for ring-opening polymerization: cues for the catalyst design, Macromolecules, 2019, 52, 9232–9237 CrossRef CAS.
- E. Vakalopoulou, S. M. Borisov and C. Slugovc, Fast oxygen scavenging of macroporous poly(norbornadiene) prepared by ring-opening metathesis polymerization, Macromol. Rapid Commun., 2020, 1900581 CrossRef CAS PubMed.
- A. Yadav, N. B. Erdal, M. Hakkarainen, B. Nandan and R. K. Srivastava, Cellulose-derived nanographene oxide reinforced macroporous scaffolds of high internal phase emulsion-templated cross-Linked poly(ε-caprolactone), Biomacromolecules, 2020, 21, 589–596 CrossRef CAS PubMed.
- S. Rezaei, A. M. Zolali, A. Jalali and C. B. Park, Novel and simple design of nanostructured, super-insulative and flexible hybrid silica aerogel with a new macromolecular polyether-based precursor, J. Colloid Interface Sci., 2020, 561, 890–901 CrossRef CAS PubMed.
- M. Hong and E. Y. X. Chen, Towards truly sustainable polymers: a metal-free recyclable polyester from biorenewable non-strained γ-butyrolactone, Angew. Chem., Int. Ed., 2016, 55, 4188–4193 CrossRef CAS PubMed.
- A. C. Albertsson and I. K. Varma, Recent developments in ring opening polymerization of lactones for biomedical applications, Biomacromolecules, 2003, 4, 1466–1486 CrossRef CAS PubMed.
- X. Pan, F. Driessen, X. Zhu and F. E. DuPrez, Selenolactone as a building block toward dynamic diselenide-containing polymer architectures with controllable topology, ACS Macro Lett., 2017, 6, 89–92 CrossRef CAS.
- C. Wang, X. An, M. Pang, Z. Zhang, X. Zhu, J. Zhu, F. E. DuPrez and X. Pan, Dynamic diselenide-containing polyesters from alcoholysis/oxidation of γ-butyroselenolactone, Polym. Chem., 2018, 9, 4044–4051 RSC.
- Y. Li, M. Vergaelen, X. Pan, F. Du Prez, R. Hoogenboom and K. De Clerck, In situ cross-linked nanofibers by aqueous electrospinning of selenol-functionalized poly(2-oxazoline)s, Macromolecules, 2018, 51, 6149–6156 CrossRef CAS.
- S. Ji, W. Cao, Y. Yu and H. Xu, Dynamic diselenide bonds: exchange reaction induced by visible light without catalysis, Angew. Chem., Int. Ed., 2014, 53, 6781–6785 CrossRef CAS PubMed.
- X. An, R. H. Aguirresarobe, L. Irusta, F. Ruipérez, J. M. Matxain, X. Pan, N. Aramburu, D. Mecerreyes, H. Sardon and J. Zhu, Aromatic diselenide crosslinkers to enhance the reprocessability and self-healing of polyurethane thermosets, Polym. Chem., 2017, 8, 3641–3646 RSC.
- C. Wei, Y. Zhang, B. Yan, Y. Xu, Z. Du and M. Lang, A versatile strategy to main chain sulfur/selenium-functionalized polycarbonates by macro-ring closure of diols and subsequent ring-opening polymerization, Chem. – Eur. J., 2018, 24, 789–792 CrossRef CAS PubMed.
- C. Wei, Y. Xu, B. Yan, J. Hou, Z. Du and M. Lang, Well-defined selenium-containing aliphatic polycarbonates via lipase-catalyzed ring-opening polymerization of selenic macrocyclic carbonate monomer, ACS Macro Lett., 2018, 7, 336–340 CrossRef CAS.
- C. Zhang, X. Cao and X. Zhang, Metal-free alternating copolymerization of nonstrained γ-selenobutyrolactone with epoxides for selenium-rich polyesters, Macromolecules, 2020, 53, 203–211 CrossRef CAS.
- L. Yu, Y. Yang, F. Du and Z. Li, ROS-responsive chalcogen-containing polycarbonates for photodynamic therapy, Biomacromolecules, 2018, 19, 2182–2193 CrossRef CAS PubMed.
- X. Lou, C. Detrembleur, P. Lecomte and R. Jérôme, Living ring-opening (co)polymerization of 6,7-dihydro-2(5H)-oxepinone into unsaturated aliphatic polyesters, Macromolecules, 2001, 34, 5806–5811 CrossRef CAS.
- M. A. Woodruff and D. W. Hutmacher, The return of a forgotten polymer-polycaprolactone in the 21st century, Prog. Polym. Sci., 2010, 35, 1217–1256 CrossRef CAS.
- J. D. Erndt-Marino, D. J. Munoz-Pinto, S. Samavedi, A. C. Jimenez-Vergara, P. Diaz-Rodriguez, L. Woodard, D. W. Zhang, M. A. Grunlan and M. S. Hahn, Evaluation of the osteoinductive capacity of polydopamine-coated poly(ε-caprolactone) diacrylate shape memory foams, ACS Biomater. Sci. Eng., 2015, 1, 1220–1230 CrossRef CAS.
- S. Lambert and M. Wagner, Environmental performance of bio-based and biodegradable plastics: the road ahead, Chem. Soc. Rev., 2017, 46, 6855–6871 RSC.
- G. I. Peterson, A. V. Dobrynin and M. L. Becker, Biodegradable shape memory polymers in medicine, Adv. Healthcare Mater., 2017, 6, 1700694 CrossRef PubMed.
- E. Malikmammadov, T. E. Tanir, A. Kiziltay, V. Hasirci and N. Hasirci, PCL and PCL-based materials in biomedical applications, J. Biomater. Sci., Polym. Ed., 2018, 29, 863–893 CrossRef CAS PubMed.
- J. P. Latere, P. Lecomte, P. Dubois and R. Jérôme, 2-oxepane-1,5-dione: a precursor of a novel class of versatile semicrystalline biodegradable
(co)polyesters, Macromolecules, 2002, 35, 7857–7859 CrossRef CAS.
- J. Yan, Y. Zhang, Y. Xiao, Y. Zhang and M. Lang, Novel poly(ε-caprolactone)s bearing amino groups: Synthesis, characterization and biotinylation, React. Funct. Polym., 2010, 70, 400–407 CrossRef CAS.
- W. Chen, Y. Zhou, Y. Li, J. Sun, X. Pan, Q. Yu, N. Zhou, Z. Zhang and X. Zhu, Shape-memory and self-healing polyurethanes based on cyclic poly(ε-caprolactone), Polym. Chem., 2016, 7, 6789–6797 RSC.
- C. Wei, Y. Zhang, H. Xu, Y. Xu, Y. Xua and M. Lang, Well-defined labile diselenide-centered poly(ε-caprolactone)-based micelles for activated intracellular drug release, J. Mater. Chem. B, 2016, 4, 5059–5067 RSC.
- C. Wei, Y. Zhang, Z. Song, Y. Xia, H. Xu and M. Lang, Enhanced bioreduction-responsive biodegradable diselenide-containing poly(ester urethane) nanocarriers, Biomater. Sci., 2017, 5, 669–677 RSC.
- J. Liu, X. Ma, Y. Tong and M. Lang, Syntheses of diselenide-containing dynamic polymers and their application in polymer alloys, Appl. Surf. Sci., 2019, 467, 477–485 CrossRef.
- L. Yu, M. Zhang, F. Du and Z. Li, ROS-responsive poly(ε-caprolactone) with pendent thioether and selenide motifs, Polym. Chem., 2018, 9, 3762–3773 RSC.
- J. Wu, C. Ding, D. Xing, Z. Zhang, X. Huang, X. Zhu, X. Pan and J. Zhu, The functionalization of poly(ε-caprolactone) as a versatile platform using ε-(α-phenylseleno) caprolactone as a monomer, Polym. Chem., 2019, 10, 3851–3858 RSC.
- N. K. Kildaht, Bond energy data summarized, J. Chem. Educ., 1995, 72, 423–424 CrossRef.
- T. S. Kwon, S. Kumazawa, T. Yokoi, S. Kondo, H. Kunisada and Y. Yuki, Living radical polymerization of styrene with diphenyl diselenide as a photoiniferter. synthesis of polystyrene with carbon-carbon double bonds at both chain ends, J. Macromol. Sci., Part A: Pure Appl. Chem., 1997, 34, 1553–1567 CrossRef.
- T. S. Kwon, K. Suzuki, K. Takagi, H. Kunisada and Y. Yuki, Radical polymerization of methyl methacrylate with diphenyl diselenide under thermal or photoirradiation conditions, J. Macromol. Sci., Part A: Pure Appl. Chem., 2001, 38, 591–604 CrossRef.
- K. Takagi, Y. Nishikawa, T. S. Kwon, H. Kunisada and Y. Yuki, Synthesis of branched polystyrene by photopolymerization of selenium-containing styrene monomer, Polym. J., 2000, 32, 970–973 CrossRef CAS.
- W. Lu, X. Pan, Z. Zhang, J. Zhu, N. Zhou and X. Zhu, A degradable cross-linked polymer containing dynamic covalent selenide bond, Polym. Chem., 2017, 8, 3874–3880 RSC.
- J. Lu, N. Zhou, X. Pan, J. Zhu and X. Zhu, Branched polystyrene with high reflex index synthesized from selenium-mediated polymerization, J. Polym. Sci., Part A: Polym. Chem., 2014, 52, 504–510 CrossRef CAS.
- J. Y. C. Chu, D. G. Marsh and W. H. H. Gunther, Photochemistry of organochalcogen compounds. I. Photolysis of benzyl diselenide, J. Am. Chem. Soc., 1975, 97, 4905–4908 CrossRef CAS.
- A. Ouchi and Y. Koga, A facile synthesis of tetra- and dihydronaphthalene derivatives by excimer laser photolysis of 1,2-bis(substituted-methyl)benzenes in the presence of olefins and acetylene, J. Org. Chem., 1997, 62, 7376–7383 CrossRef CAS PubMed.
- S. G. Gaynor, S. Edelman and K. Matyjaszewski, Synthesis of branched and hyperbranched polystyrenes, Macromolecules, 1996, 29, 1079–1081 CrossRef CAS.
-
D. Yan, C. Gao and H. Frey, Hyperbranched polymers: synthesis, properties and applications, John Wiley & Sons, Inc, NJ, 2011 Search PubMed.
- Z. Zhou and D. Yan, A general model for the kinetics of self-condensing vinyl polymerization, Macromolecules, 2008, 41, 4429–4434 CrossRef CAS.
- J. Schmitt, N. Blanchard and J. Poly, Controlled synthesis of branched poly(vinyl acetate)s by xanthate-mediated RAFT self-condensing vinyl (co)polymerization, Polym. Chem., 2011, 2, 2231–2238 RSC.
- T. Otsu, Iniferter concept and living radical polymerization, J. Polym. Sci., Part A: Polym. Chem., 2000, 38, 2121–2136 CrossRef CAS.
- M. Chen, M. Zhong and J. A. Johnson, Light-controlled radical polymerization: mechanisms, methods, and applications, Chem. Rev., 2016, 116, 10167–10211 CrossRef CAS PubMed.
- X. Pan, M. A. Tasdelen, J. Laun, T. Junkers, Y. Yagci and K. Matyjaszewski, Photomediated controlled radical polymerization, Prog. Polym. Sci., 2016, 62, 73–125 CrossRef CAS.
- R. Barbey, L. Lavanant, D. Paripovic, N. Schüwer, C. Sugnaux, S. Tugulu and H. Klok, Polymer brushes via surface-initiated controlled radical polymerization: synthesis, characterization, properties, and applications, Chem. Rev., 2009, 109, 5437–5527 CrossRef CAS PubMed.
- J. Chiefari, Y. K. B. Chong, F. Ercole, J. Krstina, J. Jeffery, T. P. T. Le, R. T. A. Mayadunne, G. F. Meijs, C. L. Moad, G. Moad, E. Rizzardo and S. H. Thang, Living free-radical polymerization by reversible addition-fragmentation chain transfer: the RAFT process, Macromolecules, 1998, 31, 5559–5562 CrossRef CAS.
- G. Moad, E. Rizzardo and S. H. Thang, Living radical polymerization by the RAFT process, Aust. J. Chem., 2005, 58, 379–410 CrossRef CAS.
- A. B. Lowe and C. L. McCormick, Reversible addition-fragmentation chain transfer (RAFT) radical polymerization and the synthesis of water-soluble (co)polymers under homogeneous conditions in organic and aqueous media, Prog. Polym. Sci., 2007, 32, 283–351 CrossRef CAS.
- M. R. Hill, R. N. Carmean and B. S. Sumerlin, Expanding the scope of RAFT polymerization: recent advances and new horizons, Macromolecules, 2015, 48, 5459–5469 CrossRef CAS.
- W. Sang, M. Xu and Q. Yan, Coenzyme-catalyzed electro-RAFT polymerization, ACS Macro Lett., 2017, 6, 1337–1341 CrossRef CAS.
- G. Moad, RAFT polymerization to form stimuli-responsive polymers, Polym. Chem., 2017, 8, 177–219 RSC.
- T. G. McKenzie, Q. Fu, M. Uchiyama, K. Satoh, J. Xu, C. Boyer, M. Kamigaito and G. G. Qiao, Beyond traditional RAFT: alternative activation of thiocarbonylthio compounds for controlled polymerization, Adv. Sci., 2016, 3, 1500394 CrossRef PubMed.
- G. Moad, E. Rizzardo and S. H. Thang, RAFT polymerization and some of its applications, Chem. – Asian J., 2013, 8, 1634–1644 CrossRef CAS PubMed.
- C. Ding, C. Fan, G. Jiang, X. Pan, Z. Zhang, J. Zhu and X. Zhu, Photocatalyst-free and blue light-induced RAFT polymerization of vinyl acetate at ambient temperature, Macromol. Rapid Commun., 2015, 36, 2181–2185 CrossRef CAS PubMed.
- C. Ding, J. Wang, W. Zhang, X. Pan, Z. Zhang, W. Zhang, J. Zhu and X. Zhu, Platform of near-infrared light-induced reversible deactivation radical polymerization: upconversion nanoparticles as internal light sources, Polym. Chem., 2016, 7, 7370–7374 RSC.
- J. Moon, H. Nam, S. Kim, J. Ryu, C. Han, C. Lee and S. Lee, Synthesis of phosphinodiselenoic acid esters and their application as RAFT agents in styrene polymerization, Tetrahedron Lett., 2008, 49, 5137–5140 CrossRef CAS.
- E. Song, Y. Jo, G. Bae, K. Oh, H. M. Jung and S. Lee, Synthesis of phosphinodiselenoic acid ester derivatives and their application in the controlled radical polymerization of styrene, Bull. Korean Chem. Soc., 2009, 30, 2129–2131 CrossRef CAS.
- J. Zeng, J. Zhu, Z. Zhang, X. Pan, W. Zhang, Z. Cheng and X. Zhu, New selenium-based iniferter agent for living free radical polymerization of styrene under UV irradiation, J. Polym. Sci., Part A: Polym. Chem., 2012, 50, 2211–2218 CrossRef CAS.
- Y. Zhan, Z. Zhang, X. Pan, J. Zhu, N. Zhou and X. Zhu, A cyclic selenium-based reversible addition-fragmentation chain transfer agent mediated polymerization of vinyl acetate, J. Polym. Sci., Part A: Polym. Chem., 2013, 51, 1656–1663 CrossRef CAS.
- F. Ma, J. Zhu, Z. Zhang, X. Pan, N. Zhou and X. Zhu, Seleno-containing poly(vinyl acetate) prepared by diselenocarbonates-mediated controlled free radical polymerizations, J. Polym. Sci., Part A: Polym. Chem., 2013, 51, 3159–3165 CrossRef CAS.
- J. Zeng, Z. Zhang, J. Zhu, N. Zhou, Z. Cheng and X. Zhu, Selenium-substituted carbonates as mediators for controlled radical polymerization, J. Polym. Sci., Part A: Polym. Chem., 2013, 51, 2606–2613 CrossRef CAS.
- M. Benaglia, J. Chiefari, Y. K. Chong, G. Moad, E. Rizzardo and S. H. Thang, Universal (switchable) RAFT agents, J. Am. Chem. Soc., 2009, 131, 6914–6915 CrossRef CAS PubMed.
- M. Benaglia, M. Chen, Y. K. Chong, G. Moad, E. Rizzardo and S. H. Thang, Polystyrene-block-poly(vinyl acetate) through the use of a switchable RAFT agent, Macromolecules, 2009, 42, 9384–9386 CrossRef CAS.
- D. J. Keddie, C. Guerrero-Sanchez, G. Moad, R. J. Mulder, E. Rizzardo and S. H. Thang, Chain transfer kinetics of acid/base switchable N-aryl-N-pyridyl dithiocarbamate RAFT agents in methyl acrylate, N-vinylcarbazole and vinyl acetate polymerization, Macromolecules, 2012, 45, 4205–4215 CrossRef CAS.
- D. J. Keddie, C. Guerrero-Sanchez, G. Moad, R. J. Mulder, E. Rizzardo and S. H. Thang, Switchable reversible addition-fragmentation chain transfer (RAFT) polymerization in aqueous solution, N,N-dimethylacrylamide, Macromolecules, 2011, 44, 6738–6745 CrossRef CAS.
- J. Zeng, J. Zhu, X. Pan, Z. Zhang, N. Zhou, Z. Cheng, W. Zhang and X. Zhu, Organoselenium compounds: development of a universal “living” free radical polymerization mediator, Polym. Chem., 2013, 4, 3453–3457 RSC.
- W. Lu, X. An, F. Gao, J. Zhu, N. Zhou, Z. Zhang, X. Pan and X. Zhu, Highly efficient chain end derivatization of selenol-ended polystyrenes by nucleophilic substitution reactions, Macromol. Chem. Phys., 2017, 218, 1600485 CrossRef.
- W. Lu, X. An, J. Zhu, N. Zhou, Z. Zhang, X. Pan and X. L. Zhu, From seleno-mediated radical polymerization to seleno-containing branched polymers and dynamic hydrogel, RSC Adv., 2017, 7, 9773–9779 RSC.
- Z. Cai, W. Lu, F. Gao, X. Pan, J. Zhu, Z. Zhang and X. Zhu, Diselenide-labeled cyclic polystyrene with multiple responses: facile synthesis, tunable size, and topology, Macromol. Rapid Commun., 2016, 37, 865–871 CrossRef CAS PubMed.
- T. Guo, L. Zhang, X. Pan, X. Li, Z. Cheng and X. Zhu, A highly active homogeneous ICAR ATRP of methyl methacrylate using ppm levels of organocopper catalyst, Polym. Chem., 2013, 4, 3725–3734 RSC.
- F. Gao, X. Pan, J. Zhu, Z. Zhang, W. Zhang and X. Zhu, Diselenocarbamates, novel initiators in ATRP of styrene, J. Polym. Sci., Part A: Polym. Chem., 2015, 53, 1927–1933 CrossRef CAS.
- C. Fan, C. Ding, X. Pan, Z. Zhang, J. Zhu and X. Zhu, A straightforward method for preparing well-defined responsive diselenide-containing polymers based on ATRP, Macromol. Rapid Commun., 2015, 36, 903–908 CrossRef CAS PubMed.
- C. Ding, C. Fan, X. Pan, Z. Zhang, J. Zhu and X. Zhu, Selenium borohydride reaction as a versatile platform for the straightforward preparation of selenide-containing topological polymers, Polym. Chem., 2017, 8, 3958–3964 RSC.
- S. Ji, W. Cao, Y. Yu and H. Xu, Visible-light-induced self-healing diselenide-containing polyurethane elastomer, Adv. Mater., 2015, 27, 7740–7745 CrossRef CAS PubMed.
- S. Ji, J. Xia and H. Xu, Dynamic chemistry of selenium: Se-N and Se-Se dynamic covalent bonds in polymeric systems, ACS Macro Lett., 2016, 5, 78–82 CrossRef CAS.
- S. Ji, F. Fan, C. Sun, Y. Yu and H. Xu, Visible light-induced plasticity of shape memory polymers, ACS Appl. Mater. Interfaces, 2017, 9, 33169–33175 CrossRef CAS.
- P. Zhao, J. Xia, M. Cao and H. Xu, Wavelength-controlled light-responsive polymer vesicle based on Se-S dynamic chemistry, ACS Macro Lett., 2020, 9, 163–168 CrossRef CAS.
- H. Jiang, X. Pan, N. Li, Z. Zhang, J. Zhu and X. Zhu, Selenide-containing high refractive index polymer material with adjustable refractive index and Abbe's number, React. Funct. Polym., 2017, 111, 1–6 CrossRef CAS.
- S. Zhai, X. Hu, Y. Hu, B. Wu and D. Xing, Visible light-induced crosslinking and physiological stabilization of diselenide-rich nanoparticles for redox-responsive drug release and combination chemotherapy, Biomaterials, 2017, 121, 41–54 CrossRef CAS.
-
J. G. Speight, Lange's handbook of chemistry, McGraw-Hill, New York, USA, 2005 Search PubMed.
- K. C. Krogman, T. Druffel and M. K. Sunkara, Anti-reflective optical coatings incorporating nanoparticles, Nanotechnology, 2005, 16, 338–343 CrossRef CAS PubMed.
- J. Liu and M. Ueda, High refractive index polymers: fundamental research and practical applications, J. Mater. Chem., 2009, 19, 8907–8919 RSC.
- G. S. Jha, G. Seshadri, A. Mohan and R. K. Khandal, Development of high refractive index plastics, e-Polym., 2007, 7, 1384–1408 Search PubMed.
- D. W. Mosley, G. Khanarian, D. M. Conner, D. L. Thorsen, T. L. Zhang and M. Wills, High refractive index thermally stable phenoxyphenyl and phenylthiophenyl silicones for light-emitting diode applications, J. Appl. Polym. Sci., 2014, 131, 39824–39834 CrossRef.
- K. Kitamura, K. Okada, N. Fujita, Y. Nagasaka, M. Ueda, Y. Sekimoto and Y. Kurata, Fabrication method of double-microlens array using self-alignment technology, Jpn. J. Appl. Phys., 2004, 43, 5840–5844 CrossRef CAS.
- T. Nakamura, H. Fujii, N. Juni and N. Tsutsumi, Enhanced coupling of light from organic electroluminescent device using diffusive particle dispersed high refractive index resin substrate, Opt. Rev., 2006, 13, 104–110 CrossRef.
- H. A. Lorentz, Ueber die Beziehung zwischen der Fortpflanzungsgeschwindigkeit des Lichtes und der Körperdichte, Ann. Phys., 1880, 245, 641–665 CrossRef.
- U. H. You, N. Fukuzaki, Y. Suzuki, Y. Nakamura, T. Higashihara, S. Ando and M. Ueda, Synthesis of high-refractive index polyimide containing selenophene unit, J. Polym. Sci., Part A: Polym. Chem., 2009, 47, 4428–4434 CrossRef.
- R. Okutsu, S. Ando and M. Ueda, Sulfur-containing poly(meth)acrylates
with high refractive indices and high Abbe's numbers, Chem. Mater., 2008, 20, 4017–4023 CrossRef CAS.
- R. Okutsu, Y. Suzuki, S. Ando and M. Ueda, Poly(thioether sulfone) with high refractive index and high Abbe's number, Macromolecules, 2008, 41, 6165–6168 CrossRef CAS.
- D. J. Liaw, F. Chang, M. Leung, M. Chou and K. Muellen, High thermal stability and rigid rod of novel organosoluble polyimides and polyamides based on bulky and noncoplanar naphthalene-biphenyldiamine, Macromolecules, 2005, 38, 4024–4029 CrossRef CAS.
- D. J. Liaw, K. Wang and F. Chang, Novel organosoluble poly(pyridine-imide) with pendent pyrene group: synthesis, thermal, optical, electrochemical, electrochromic, and protonation characterization, Macromolecules, 2007, 40, 3568–3574 CrossRef CAS.
- D. J. Liaw, K. Wang, F. Chang, K. R. Lee and J. La, Novel poly (pyridine imide) with pendent naphthalene groups: synthesis and thermal, optical, electrochemical, electrochromic, and protonation characterization, J. Polym. Sci., Part A: Polym. Chem., 2007, 45, 2367–2374 CrossRef CAS.
- K. Wang, W. Liou, D. J. Liaw and S. Huang, High glass transition and thermal stability of new pyridine-containing polyimides: effect of protonation on fluorescence, Polymer, 2008, 49, 1538–1546 CrossRef CAS.
- D. P. Erhard, F. Richter, C. B. A. Bartz and H. Schmidt, Fluorinated aromatic polyimides as high-performance electret materials, Macromol. Rapid Commun., 2015, 36, 520–527 CrossRef CAS PubMed.
- T. Doi, A. Akimoto, A. Matsumoto, Y. Oki and T. Otsu, Alternating copolymerization of N-(alkyl-substituted phenyl)maleimides with isobutene and thermal properties of the resulting copolymers, J. Polym. Sci., Part A: Polym. Chem., 1996, 34, 2499–2505 CrossRef CAS.
- R. Hu, W. Li and B. Tang, Recent advances in alkyne-based multicomponent polymerizations, Macromol. Chem. Phys., 2016, 217, 213–224 CrossRef CAS.
- R. Kakuchi, Multicomponent reactions in polymer synthesis, Angew. Chem., Int. Ed., 2014, 53, 46–48 CrossRef CAS PubMed.
- Q. Gao, L. Xiong, T. Han, Z. Qiu, X. He, H. H. Y. Sung, R. T. K. Kwok, I. D. Williams, J. W. Y. Lam and B. Tang, Three-component regio- and stereoselective polymerizations toward functional chalcogen-rich polymers with AIE-activities, J. Am. Chem. Soc., 2019, 141, 14712–14719 CrossRef CAS PubMed.
- E. S. J. Arner, Selenoproteins-what unique properties can arise with selenocysteine in place of cysteine, Exp. Cell Res., 2010, 316, 1296–1303 CrossRef CAS PubMed.
- H. J. Reich and R. J. Hondal, Why nature chose selenium, ACS Chem. Biol., 2016, 11, 821–841 CrossRef CAS PubMed.
- D. Roos and C. C. Winterbourn, Lethal weapons, Science, 2002, 296, 669–671 CrossRef CAS PubMed.
- C. C. Winterbourn, Reconciling the chemistry and biology of reactive oxygen species, Nat. Chem. Biol., 2008, 4, 278–286 CrossRef CAS PubMed.
- M. A. Reeves and P. R. Hoffmann, The human selenoproteome: recent insights into functions and regulation, Cell. Mol. Life Sci., 2009, 66, 2457–2478 CrossRef CAS PubMed.
- J. T. Rotruck, A. L. Pope, H. E. Ganther, A. B. Swanson, D. G. Hafeman and W. G. Hoekstra, Selenium: biochemical role as a component of glutathione peroxidase, Science, 1973, 179, 588–590 CrossRef CAS PubMed.
- G. Wu, C. Ge, X. Liu, S. Wang, L. Wang, L. Yin and H. Lu, Synthesis of water soluble and multi-responsive selenopolypeptides via ring-opening polymerization of N-carboxyanhydrides, Chem. Commun., 2019, 55, 7860–7863 RSC.
- J. R. Kramer and T. J. Deming, Multimodal switching of conformation and solubility in homocysteine derived polypeptides, J. Am. Chem. Soc., 2014, 136, 5547–5550 CrossRef CAS PubMed.
- S. Zang, X. Kong, J. Cui, S. Su, W. Shu, J. Jing and X. Zhang, Revealing the redox status in endoplasmic reticulum by a selenium fluorescence probe, J. Mater. Chem. B, 2020, 8, 2660–2665 RSC.
- S. T. Manjare, Y. Kim and D. G. Churchill, Selenium- and tellurium-containing fluorescent molecular probes for the detection of biologically important analytes, Acc. Chem. Res., 2014, 47, 2985–2998 CrossRef CAS PubMed.
- Z. Lou, P. Li and K. Han, Redox-responsive fluorescent probes with different design strategies, Acc. Chem. Res., 2015, 48, 1358–1368 CrossRef CAS PubMed.
- N. Ma, Y. Li, H. Ren, H. Xu, Z. Li and X. Zhang, Selenium-containing block copolymers and their oxidation-responsive aggregates, Polym. Chem., 2010, 1, 1609–1614 RSC.
- H. Ren, Y. Wu, N. Ma, H. Xu and X. Zhang, Side-chain selenium-containing amphiphilic block copolymers: redox-controlled self-assembly and disassembly, Soft Matter, 2012, 8, 1460–1466 RSC.
- Y. Fan, S. Cai, D. Xu, Q. Sun, X. Liu, Y. Zhang and Y. Fang, Reversible-tuning Krafft temperature of selenium-containing ionic surfactants by redox chemistry, Langmuir, 2020, 36, 3514–3521 CrossRef CAS PubMed.
- K. H. Tan, W. Xu, S. Stefka, D. E. Demco, T. Kharandiuk, V. Ivasiv, R. Nebesnyi, V. S. Petrovskii, I. I. Potemkin and A. Pich, Selenium-modified microgels as bio-inspired oxidation catalysts, Angew. Chem., Int. Ed., 2019, 58, 9791–9796 CrossRef CAS PubMed.
- Y. Y. Chen and G. Liu, Advance in dynamic combinatorial chemistry, Prog. Chem., 2007, 19, 1903–1908 CAS.
- P. T. Corbett, J. Leclaire, L. Vial, K. R. West, J. L. Wietor, J. K. M. Sanders and S. Otto, Dynamic combinatorial chemistry, Chem. Rev., 2006, 106, 3652–3711 CrossRef CAS PubMed.
- S. J. Rowan, S. J. Cantrill, G. R. L. Cousins, J. K. M. Sanders and J. F. Stoddart, Dynamic covalent chemistry, Angew. Chem., Int. Ed., 2002, 41, 898–952 CrossRef PubMed.
- Z. Q. Lei, P. Xie, M. Z. Rong and M. Q. Zhang, Catalyst free dynamic exchange of aromatic Schiff base bonds and its application to self healing and remolding of crosslinked polymers, J. Mater. Chem. A, 2015, 3, 19662–19668 RSC.
- M. Ciaccia, R. Cacciapaglia, P. Mencarelli, L. Mandolini and S. Di Stefano, Fast transimination in organic solvents in the absence of pro ton and metal catalysts. A key to imine metathesis catalyzed by primary amines under mild conditions, Chem. Sci., 2013, 4, 2253–2261 RSC.
- G. H. Deng, F. Y. Li, H. X. Yu, F. Y. Liu, C. Y. Liu, W. X. Sun, H. F. Jiang and Y. M. Chen, Dynamic hydrogels with an environmental adaptive self-healing ability and dual responsive sol-gel transitions, ACS Macro Lett., 2012, 1, 275–279 CrossRef CAS.
- M. Capelot, M. M. Unterlass, F. Tournilhac and L. Leibler, Catalytic control of the vitrimer glass transition, ACS Macro Lett., 2012, 1, 789–792 CrossRef CAS.
- M. Capelot, D. Montarnal, F. Tournilhac and L. Leibler, Metal catalyzed transesterification for healing and assembling of thermosets, J. Am. Chem. Soc., 2012, 134, 7664–7667 CrossRef CAS PubMed.
- A. Demongeot, S. J. Mougnier, S. Okada, C. Soulie-Ziakovic and F. Tournilhac, Coordination and catalysis of Zn2+ in epoxy based vitrimers, Polym. Chem., 2016, 7, 4486–4493 RSC.
- C. Gong, J. Liang, W. Hu, X. Niu, S. Ma, H. Thomas Hahn and Q. Pei, A healable, semitransparent silver nanowire-polymer composite conductor, Adv. Mater., 2013, 25, 4186–4191 CrossRef CAS PubMed.
- G. Fu, L. Yuan, G. Liang and A. Gu, Heat-resistant polyurethane films with great electrostatic dissipation capacity and very high thermally reversible self-healing efficiency based on multi-furan and liquid multi-maleimide polymers, J. Mater. Chem. A, 2016, 4, 4232–4241 RSC.
- M. M. Diaz, G. V. Assche, F. H. J. Maurer and B. V. Melea, Thermophysical characterization of a reversible dynamic polymer network based on kinetics and equilibrium of an amorphous furan-maleimide Diels-Alder cycloaddition, Polymer, 2017, 120, 176–188 CrossRef CAS.
- P. K. Behera, P. Mondal and N. K. Singha, Self-healable and ultrahydrophobic polyurethane-POSS hybrids by Diels-Alder “Click” reaction: a new class of coating material, Macromolecules, 2018, 51, 4770–4781 CrossRef CAS.
- A. Rekondo, R. Martin, A. R. de Luzuriaga, G. Cabanero, H. J. Grande and I. Odriozola, Catalyst free room temperature self-healing elastomers based on aromatic disulfide metathesis, Mater. Horiz., 2014, 1, 237–240 RSC.
- A. R. de Luzuriaga, R. Martin, N. Markaide, A. Rekondo, G. Cabanero, J. Rodriguez and I. Odriozola, Epoxy resin with exchangeable disulfide crosslinks to obtain reprocessable, repairable and recyclable fiber reinforced thermoset composites, Mater. Horiz., 2016, 3, 241–247 RSC.
- A. R. de Luzuriaga, J. M. Matxain, F. Ruiperez, R. Martin, J. M. Asua, G. Cabanero and I. Odriozola, Transient mechanochromism in epoxy vitrimer composites containing aromatic disulfide crosslinks, J. Mater. Chem. C, 2016, 4, 6220–6223 RSC.
- Y. Qian, X. An, X. Huang, X. Pan, J. Zhu and X. Zhu, Recyclable self-healing polyurethane cross-linked by alkyl diselenide with enhanced mechanical properties, Polymer, 2019, 11, 773 Search PubMed.
- W. Fan, Y. Jin, Y. Huang, J. Pan, W. Du and Z. Pu, Room temperature self-healing and reprocessing of diselenide-containing waterborne polyurethanes under visible light, J. Appl. Polym. Sci., 2019, 136, 47071 CrossRef.
- W. Du, Y. Jin, J. Pan, W. Fan, S. Lai and X. Sun, Thermal induced shape memory and self healing of segmented polyurethane containing diselenide bonds, J. Appl. Polym. Sci., 2018, 135, 46326 CrossRef.
- W. Du, Y. Jin, S. Lai, L. Shi, W. Fan and J. Pan, Near-infrared light triggered shape memory and self-healable polyurethane/functionalized graphene oxide composites containing diselenide bonds, Polymer, 2018, 158, 120–129 CrossRef CAS.
- C. Liu, Z. Fan, Y. Tan, F. Fan and H. Xu, Tunable structural color patterns based on the visible-light-responsive dynamic diselenide metathesis, Adv. Mater., 2020, 32, 1907569 CrossRef CAS PubMed.
- S. Pan, J. Yang, S. Ji, T. Li, S. Gao, C. Sun and H. Xu, Cancer therapy by targeting thioredoxin reductase based on selenium-containing dynamic covalent bond, CCS Chem., 2020, 2, 225–235 CrossRef.
- B. Rasmussen, A. Sorensen, H. Gotfredsen and M. Pittelkow, Dynamic combinatorial chemistry with diselenides and disulfides in water, Chem. Commun., 2014, 50, 3716–3718 RSC.
- F. Fan, S. Ji, C. Sun, C. Liu, Y. Yu, Y. Fu and H. Xu, Wavelength controlled dynamic metathesis: A light driven exchange reaction between disulfide and diselenide bonds, Angew. Chem., Int. Ed., 2018, 57, 16426–16430 CrossRef CAS PubMed.
- Y. Yi, H. Xu, L. Wang, W. Cao and X. Zhang, A new dynamic covalent bond of Se-N: Towards controlled self assembly and disassembly, Chem. – Eur. J., 2013, 19, 9506–9510 CrossRef CAS PubMed.
- C. Liu, J. Xia, S. Ji, Z. Fan and H. Xu, Visible-light-induced metathesis reaction between diselenide and ditelluride, Chem. Commun., 2019, 55, 2813–2816 RSC.
- T. S. Kwon, S. Kondo, H. Kunisada and Y. Yuki, Synthesis of polystyrene and poly(methyl methacrylate) each with a phenyl seleno group at terminal chain end by radical polymerization in the presence of benzyl selenide as a photoiniferter, Polym. J., 1998, 30, 559–565 CrossRef CAS.
- R. G. Pearson, Hard and soft acids and bases - the evolution of a chemical concept, Coord. Chem. Rev., 1990, 100, 403–425 CrossRef CAS.
- R. M. LoPachin, D. S. Barber and T. Gavin, Molecular mechanisms of the conjugated α,β-unsaturated carbonyl derivatives: relevance to neurotoxicity and neurodegenerative diseases, Toxicol. Sci., 2008, 104, 235–249 CrossRef CAS PubMed.
- R. M. LoPachin, T. Gavin, D. R. Petersen and D. S. Barber, Molecular mechanisms of 4-hydroxy-2-nonenal and acrolein toxicity: nucleophilic targets and adduct formation, Chem. Res. Toxicol., 2009, 22, 1499–1508 Search PubMed.
- Y. Bai, F. Rong, H. Wang, Y. Zhou, X. Xie and J. Teng, Removal of copper from aqueous solutions by adsorption on elemental selenium nanoparticles, J. Chem. Eng. Data, 2011, 56, 2563–2568 CrossRef CAS.
- R. Jain, N. Jordan, D. Schild, E. D. van Hullebusch, S. Weiss, C. Franzen, F. Farges, R. Hubner and P. N. L. Lens, Adsorption of zinc by biogenic elemental selenium nanoparticles, Chem. Eng. J., 2015, 260, 855–863 CrossRef CAS.
- R. Jain, D. Dominic, N. Jordan, E. R. Rene, S. Weiss, E. D. van Hullebusch, R. Hubner and P. N. L. Lens, Preferential adsorption of Cu in a multi-metal mixture onto biogenic elemental selenium nanoparticles, Chem. Eng. J., 2016, 284, 917–925 CrossRef CAS.
- R. Jain, D. Dominic, N. Jordan, E. R. Rene, S. Weiss, E. D. van Hullebusch, R. Hubner and P. N. L. Lens, Higher Cd adsorption on biogenic elemental selenium nanoparticles, Environ. Chem. Lett., 2016, 14, 381–386 CrossRef CAS.
- Z. Qian, Y. Zhang, X. Pan, N. Li, J. Zhu and X. Zhu, Selenium-doped phenolic resin spheres: ultra-high adsorption capacity of noble metals, React. Funct. Polym., 2019, 142, 223–230 CrossRef CAS.
- Y. Dai, K. Zheng, Y. Tan, W. Xiang, B. Xianyu and H. Xu, Fischesserite-inspired recyclable Se-polyurethanes for selective gold extraction, Adv. Sustainable Syst., 2020, 2000072 CrossRef.
-
G. M. Cooper and R. E. Hausman, The cell: a molecular approach, ASM Press, Washington, D.C. USA, 2007 Search PubMed.
-
J. Hagen, Industrial catalysis: a practical approach, Wiley-VCH, Weinheim, Germany, 3rd edn, 2015 Search PubMed.
- K. H. Tan, W. Xu, S. Stefka, D. E. Demco, T. Kharandiuk, V. Ivasiv, R. Nebesnyi, V. S. Petrovskii, I. I. Potemkin and A. Pich, Selenium-modified microgels as bio-inspired oxidation catalysts, Angew. Chem., Int. Ed., 2019, 58, 9791–9796 CrossRef CAS PubMed.
- D. M. Freudendahl, S. Santoro, S. A. Shahzad, C. Santi and T. Wirth, Green chemistry with selenium reagents: development of efficient catalytic reactions, Angew. Chem., Int. Ed., 2009, 48, 8409–8411 CrossRef CAS PubMed.
- A. Breder and S. Ortgies, Recent developments in sulfur- and selenium-catalyzed oxidative and isohypsic functionalization reactions of alkenes, Tetrahedron Lett., 2015, 56, 2843–2852 CrossRef CAS.
- R. Guo, J. Huang, H. Huang and X. Zhao, Organoselenium-catalyzed synthesis of oxygen- and nitrogen-containing heterocycles, Org. Lett., 2016, 18, 504–507 CrossRef CAS PubMed.
- J. Luo, Z. Zhu, Y. Liu and X. Zhao, Diaryl selenide catalyzed vicinal trifluoromethylthioamination of alkenes, Org. Lett., 2015, 17, 3620–3623 CrossRef CAS PubMed.
- L. Sancineto, C. Tidei, L. Bagnoli, F. Marini, E. J. Lenardao and C. Santi, Selenium catalyzed oxidation of aldehydes: green synthesis of carboxylic acids and esters, Molecules, 2015, 20, 10496–10510 CrossRef CAS PubMed.
- L. Yu, Y. Wu, H. Cao, X. Zhang, X. Shi, J. Luan, T. Chen, Y. Pan and Q. Xu, Facile synthesis of 2-methylenecyclobutanones via Ca(OH)2-catalyzed direct condensation of cyclobutanone with aldehydes and (PhSe)2-catalyzed Baeyer-Villiger oxidation to 4-methylenebutanolides, Green Chem., 2014, 16, 287–293 RSC.
- L. Yu, F. Chen and Y. Ding, Organoselenium-catalyzed oxidative ring expansion of methylenecyclopropanes with hydrogen peroxide, ChemCatChem, 2016, 8, 1033–1037 CrossRef CAS.
- L. Yu, Z. Bai, X. Zhang, X. Zhang, Y. Ding and Q. Xu, Organoselenium-catalyzed selectivity-switchable oxidation of β-ionone, Catal. Sci. Technol., 2016, 6, 1804–1809 RSC.
- L. Yu, J. Ye, X. Zhang, Y. Ding and Q. Xu, Recyclable (PhSe)2-catalyzed selective oxidation of isatin by H2O2: a practical and waste-free access to isatoic anhydride under mild and neutral conditions, Catal. Sci. Technol., 2015, 5, 4830–4838 RSC.
- A. F. Barrero, J. F. Q. del Moral, M. M. Herrador, M. Cortes, P. Arteaga, J. V. Catalan, E. M. Sanchez and J. F. Arteaga, Solid-phase selenium-catalyzed selective allylic chlorination of polyprenoids: facile syntheses of biologically active terpenoids, J. Org. Chem., 2006, 71, 5811–5814 CrossRef CAS PubMed.
- S. M. Bennett, Y. Tang, D. McMaster, F. V. Bright and M. R. Detty, A xerogel-sequestered selenoxide catalyst for brominations with hydrogen peroxide and sodium bromide in an aqueous environment, J. Org. Chem., 2008, 73, 6849–6852 CrossRef CAS PubMed.
- C. M. Gatley, L. M. Muller, M. A. Lang, E. E. Alberto and M. R. Detty, Xerogel-sequestered silanated organochalcogenide catalysts for bromination with hydrogen peroxide and sodium bromide, Molecules, 2015, 20, 9616–9639 CrossRef CAS PubMed.
- Y. Wang, L. Yu, B. Zhu and L. Yu, Design and preparation of a polymer resin supported organoselenium catalyst with industrial potential, J. Mater. Chem. A, 2016, 4, 10828–10833 RSC.
|
This journal is © the Partner Organisations 2020 |
Click here to see how this site uses Cookies. View our privacy policy here.