Effects of UVR exposure on the gut microbiota of mice and humans
Received
11th November 2019
, Accepted 3rd January 2020
First published on 6th January 2020
Abstract
Many alterations to the skin microbiome by exposure to UV radiation (UVR) have been postulated and may contribute to the ability of UVR phototherapy to regulate skin inflammatory diseases. Very recently, an effect of sub-erythemal narrowband UVB radiation (311 nm) on the gut microbiome of healthy individuals was reported. The relative abundance of Firmicutes and Proteobacteria increased in faecal samples of those receiving three exposures to narrowband UVB radiation; the Bacteroidetes phyla were reduced by UVB. In mice chronically exposed to sub-erythemal broadband UVR, similar faecal changes in Firmicutes and Bacteroidetes have been reported. Murine studies have allowed a further dissection of the relative ability of UVR and dietary vitamin D to modulate the gut microbiome by analysis of relative bacterial abundance in mice with similar 25-hydroxy vitamin D levels obtained by UVR exposure or from their diet, respectively. The studies of mice recovering from colitis suggested that dietary vitamin D could stimulate greater faecal abundance of Rikenellaceae, whilst exposure to UVR was necessary for changes to the abundance of Lachnospiraceae and Desulfovibrionaceae. Both human and murine studies report that multiple exposures to sub-erythemal UVR can increase the diversity of the gut microbiome, which in turn may be beneficial to the health of the host.
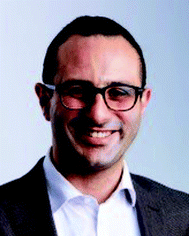 Simon Ghaly | Dr Ghaly is head of the inflammatory bowel disease unit at St Vincent's hospital, Sydney and conjoint lecturer at the School of Medicine, UNSW Sydney. He recently completed his doctoral studies examining the effect of vitamin D and ultraviolet radiation in both murine models of colitis and in human inflammatory bowel disease. His ongoing research efforts focus on the relevance of vitamin D to gut mucosal immunity, as well as the role of diet and microbiome in inflammatory bowel disease. |
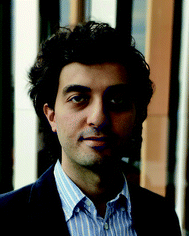 Nadeem O. Kaakoush | Dr Kaakoush is a Scientia Fellow, and Head of the Host-Microbiome Interactions group in the School of Medical Sciences at the University of New South Wales. He completed his doctoral studies in the area of host-microbial interactions. In 2011, he was awarded a NHMRC Early Career Fellowship to investigate the microbiome in inflammatory bowel diseases. He then received a Cancer Institute NSW Career Development fellowship to investigate the microbiome in upper gastrointestinal cancers. His current research focuses on the influence of host and environmental factors on microbiome dynamics, and how these changes relate to an array of gastrointestinal diseases. |
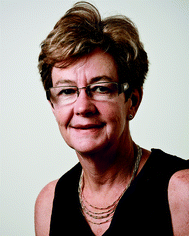 Prue H. Hart | Prue Hart is a Principal Research Fellow, Telethon Kids Institute, Perth, Australia and Adjunct Professor, University of Western Australia. Her research interests include cellular immunology and inflammation control. Prue runs a trial of UVB phototherapy for people with their first demyelinating disease, an early form of multiple sclerosis. This trial follows 20 years of basic research investigating the mechanisms by which UV radiation is immunomodulatory. |
Introduction
UV irradiation stimulates immunoregulatory pathways in skin. Importantly, immunoregulation occurs in response to sub-erythemal UV radiation (UVR), and extends beyond the site of skin irradiation. In humans and mice, responses to antigens applied to non-irradiated skin sites are reduced, and as used for treatment of inflammatory skin conditions, narrowband UVB phototherapies are under investigation for treatment of systemic autoimmune conditions, for example an early form of multiple sclerosis.1 The mechanisms of UVR-induced immunoregulation have been recently reviewed.2–4
Several other systemic effects of exposure to sub-erythemal UVR have been recognised. By stimulating the release of stored NO in the skin, UVR exposure has been shown to modulate blood pressure.5 In addition, by a similar NO-dependent pathway and independently of the actions of vitamin D, UVR exposure has been shown to reduce hallmarks of the metabolic syndrome in mice exposed to a high-fat diet.6 Even when already-obese mice were exposed to UVR, there was a significant reduction in fasting insulin levels and extent of hepatic steatosis.7
This perspective summarises findings whereby exposure of the skin to UVR may have another systemic effect, namely an effect on the gut microbiome. The effects of UVR exposure on skin microbiota and consequent systemic effects are first reviewed before discussion of the effects of skin UVR exposure on gut microbiota. As proposed for UVR-regulation of immunity, UVR exposure may regulate the gut microbiota both by a vitamin D-dependent mechanism (and thus replicated by dietary vitamin D) and potentially a vitamin D-independent pathway. Studies that compared the effect in mice of oral vitamin D with that of chronic sub-erythemal UV irradiation of skin will be discussed and compared with outcomes recently reported for changes in the gut microbiota of humans after three full body exposures to sub-erythemal narrowband UVR.8
UVR and the skin microbiome
In a two-way interaction, the immune system tolerates skin commensal bacteria and the immune system keeps the skin microbiota in check. The numbers of FoxP3+ T regulatory cells are significantly higher in skin than in blood; these cells are maintained, in part, by the short chain fatty acids produced by skin bacteria.9 However, UVR exposure, even sub-erythemal doses, may disrupt the skin microbiota by effects on (a) the organisms themselves such as by stimulating DNA mutations or changes to their energy source, (b) the biochemistry or architecture of the skin (oedema, altered permeability of a broken or proliferating epidermal barrier), and (c) on the skin resident cells and their immune responses to the microbial antigens. In response to UVR exposure, several antimicrobial peptides that can activate both innate and adaptive immune responses are produced in human skin.10
Further evidence that the skin microbiome and the inflammatory and immune systems are in a functional equilibrium follows studies of the response to UVR by mice housed under germ-free (GF) compared with under specific-pathogen-free (SPF) conditions.11 When given a single dose of erythemal UVR, there was a greater inflammatory response in the SPF mice. This suggested that there may be a lack of development and maturation of the immune system in GF mice during the neonatal period12 or that the skin microbiome enhances the innate immune pathways stimulated by UVR exposure and, by inference, may facilitate protective responses to other foreign insults. When UVR-induced suppression of a contact hypersensitivity response was examined, the extent of suppression of the recall immune response was smaller in the SPF mice, suggesting that the microbiome protects the host and encourages homeostasis.11 These studies focussed on the role of the skin microbiome when present or absent; further experiments are required to determine the effect of UVR on modulating the diversity and individual components of the skin microbiome and consequent inflammatory and immune responses.
A breakdown in the tolerance of the immune system with the skin microbiota has been implicated in the pathogenesis of several human skin conditions. Dysbiosis of the skin microbiome with reduced diversity and fewer commensal bacteria producing protective antimicrobial peptides has been implicated in atopic dermatitis. Exposure to narrowband UVR phototherapy has been shown to reduce the abundance of Staphylococcus aureus in skin and improve disease severity (for review3). In psoriasis, a dysfunctional inflammatory immune response to commensal skin microbiota develops and repeated exposure to narrowband UVB is a common successful treatment (for review3). In a recent study of patients with chronic plaque psoriasis,13 there was a significantly lower abundance of the phylum Firmicutes and the genus Staphylococcus in lesional skin compared to non-lesional skin. Furthermore, there were bacterial changes including a reduction in the abundance of Pseudomonas after narrowband UVB therapy of treatment responders.13 However, it remains unknown whether any changes in the skin microbiome are a cause or product of the condition, and similarly if the success of UVR exposure is due primarily to immunosuppression or changes to the microbiome.
The gut microbiome
Collective studies suggest the gut microbiota and the products they produce are master regulators of tissue health and immunity, even in remote organs of the body.14 The gut microbiota play a homeostatic role by regulating food metabolism, intestinal barrier integrity and colonisation by pathogenic organisms. The gut microbiome is commonly dominated by the phyla Firmicutes and Bacteroidetes, with other taxa within Proteobacteria, Actinobacteria and Fusobacteria and lesser known phyla providing additional microbial diversity. It is difficult to define what constitutes a healthy gut microbiota given that geographical differences exist within the diversity of the microbiome even in one individual. However, at the population level, healthy individuals appear to cluster into gut microbiome enterotypes that are driven by evolution with the host and environmental factors such as diet.15 These enterotypes are often dominated by specific bacterial genera such as Bacteroides, Prevotella or Faecalibacterium, that seem to specialise in the degradation of different forms of carbohydrates derived from animals or plants.15 While the phylum Firmicutes comprises a large array of taxa that can range from anti-inflammatory species to potent pathogens, in the context of the commensal gut microbiota, families within Firmicutes such as Lachnospiraceae and Ruminococcaceae are generally considered health promoting owing to their capacity to anaerobically ferment carbohydrates into short chain fatty acids.16
In early life, the gut microbiota have been implicated in the development of the mucosal and systemic immune systems.12 In adult life, the gut microbiota continue to maintain immune homeostasis and can contribute to both susceptibility and protection from autoimmune and other chronic diseases.14 Gut microbiome perturbations have been implicated in the pathogenesis of inflammatory bowel diseases, atopic asthma, behavioural disorders, obesity and type 2 diabetes, cardiovascular disease and autoimmune diseases such as rheumatoid arthritis and multiple sclerosis (for review14).
UVR and the human gut microbiome
Changes to the gut microbiome following skin exposure to UVR is a novel concept. The first report of modulation of the human intestinal microbiome by skin exposure to narrowband UVB has just been published.8 Participants in the study (all female, n = 21) received full-body UVR; they were exposed to approximately 70% of a minimum erythemal dose of narrowband UVR (311 nm) three times over a single week between February and April (i.e. towards the end of winter) in Vancouver, Canada. The participants were divided into those who had taken vitamin D supplements in the previous 3 months (mean average supplementation in 9 participants of 1389 IU vitamin D per day). Most of the supplemented group had 25(OH)D3 levels before phototherapy of >75 nmol L−1.8 The non-supplemented group (n = 11) were defined as vitamin D-insufficient at the beginning of the study with serum 25-hydroxy vitamin D3 (25(OH)D3) levels of <75 nmol L−1.
Faecal samples were collected both before UVR exposure and in the 3 days following the third UVR exposure. Faecal microbiota were examined by sequencing of the V6–V8 segment of the 16S ribosomal RNA gene. An increase in alpha diversity and shift in beta diversity of the gut microbiota was observed only in those who had not been recently supplemented with vitamin D.8 There was no change with UVR of the gut microbiota of the recently supplemented group. At the phylum level, three exposures to UVR significantly increased the relative abundance of Firmicutes and significantly reduced that of Bacteroidetes (Table 1). For the less dominant taxa, a significant increase was identified in the relative abundance of Proteobacteria. There was no significant change for Verrucomicrobia.8 At the family level, the largest effect of UVR was an enrichment of Lachnospiraceae with its abundance correlating positively with UVR-induced increases in serum 25(OH)D3. The authors concluded that UVR-induced vitamin D was responsible for the effects of UVR on the increased diversity of the gut microbiota.8
Table 1 Changes in relative abundance of bacterial phyla in faecal samples from humans and mice exposed to UV radiation. Faecal samples from females only were examined in both studies. A minimum erythemal dose (MED) is that amount of UVR that simulates a just perceptible reddening of the skin after 24 hours. Shading emphasises similar findings between humans and mice. All changes were observed in mice not given DSS at any time
UVR and the murine gut microbiome
Studies in C57BL/6 mice were designed to compare the effect of chronic sub-erythemal UVR exposure with that of oral vitamin D on the gut microbiome. This comparison was relevant and may inform on whether any changes to the gut bacterial microbiota due to UVR can be replicated by dietary vitamin D.
Six-week old mice were fed one of three different diets for 5 weeks (n = 10 mice per group).17,18 These diets were labelled as vitamin D-high (D++)(10
000 IU kg−1 vitamin D3, 0.5% calcium), vitamin D-sufficient (D+)(2280 IU kg−1 vitamin D3, 1% calcium) and vitamin D-insufficient (D−)(0 IU kg−1 vitamin D3, 2% calcium). The diets were tolerated well, no physiological dysfunction was observed and serum calcium levels were not significantly different between groups of mice.18 After 4 weeks, the mice stayed on their respective vitamin D-containing diets but half of each group of mice were UV-irradiated and the other half mock-irradiated once daily for 4 days, and then twice weekly for another 5 weeks.17 The protocol involved irradiation of shaved dorsal skin with 1 kJ m−2 broadband UVR (290–400 nm); for C57BL/6 mice using this irradiation source, ∼2 kJ m−2 is an erythemal dose of UVR.19 The mock-irradiated mice were shaved and handled in a very similar manner but were not placed under the UVB-emitting lamps (Philips TL UV-B, Eindhoven, the Netherlands).
The methodology for murine faecal microbiota analysis, 16S rRNA amplicon sequencing, was similar to that reported above in the study of human faeces.17,18 Irrespective of vitamin D diet or time (sampling after 6 and 14 exposures to UVR), there was no difference in alpha- or beta-diversity in faecal samples from UV-irradiated mice compared to the corresponding non-irradiated mice, probably due to the small number of mice. However, when all groups were analysed as having been UV-irradiated, or not irradiated, there was a significant difference in overall beta-diversity with UV irradiation, independent of the effect of vitamin D.17 With UVR exposure, there was a shift from the phylum Bacteroidetes to the phyla Deferribacteres and Firmicutes (Table 1). At the genus level, amongst the organisms enriched with UVR exposure was Coprococcus, a member of the family Lachnospiraceae within the phylum Firmicutes, and one well recognised as beneficial to good gut health by production of the anti-inflammatory molecule, butyrate. In addition, butyrate can upregulate the expression of the vitamin D receptor on intestinal epithelial cells.20 It is less easy to ascribe functions to other bacteria significantly increased in the faecal pellets from UV-irradiated mice; these included members of the genera Mucispirillum, Clostridium and Desulfovibrio.17 Their role in altering the function and equilibrium of the gut microbiota with host physiology must await further scientific investigations of not only the bacteria but also the molecules they produce.
An effect of chronic sub-erythemal UVR on the faecal microbiota was also detected in mice with inflammatory colitis induced by the chemical, dextran sodium sulphate (DSS). The mice in this experiment were fed throughout on the vitamin D-deficient diet. When examined by PERMANOVA, there were significant differences in the overall beta-diversity of the faecal microbiota.17 With UVR, there was again an overall shift from phylum Bacteroidetes to phylum Firmicutes, and an increase in phylum Verrucomicrobia,17 again suggesting general beneficial effects of UV irradiation of skin.
Vitamin D diets and the murine gut microbiome
The faecal microbiome of the mice on the three different vitamin D diets was analysed by protocols identical to those used to examine faecal pellets from the UV-irradiated mice.18 After 5 weeks on the diets,18 there were no differences with increasing vitamin D in the species richness as measured by Chao1. However, Shannon's diversity in the D++ mice was significantly reduced compared with the D-mice; global microbial composition was also found to be significantly different across the groups. When individual taxa were examined, there was a significant correlation between four taxa (>0.1% average relative abundance) and serum 25(OH)D3 levels.18 For increased vitamin D intake, the relative abundance of Paulidibacter, Bacteroidales S24-7 and Sutterella increased whilst that of Coprococcus decreased. In fact, the mice on the high D++ diet had a more similar faecal pro-inflammatory overall microbiota composition to mice with bowel inflammation due to administration of DSS. Of particular note, Sutterella spp increased in relative abundance in D++ mice with bowel inflammation and supports the finding of enriched Sutterella spp in humans with inflammatory bowel diseases.21 In summary, the effects of chronic sub-erythemal UV irradiation on the mouse gut microbiome were very different to those measured in mice fed a diet supplemented with extra vitamin D. The gut microbiome from UV-irradiated mice was enriched with the anti-inflammatory Firmicutes, in particular Coprococcus. In contrast, Coprococcus spp were reduced in mice fed a high vitamin D diet, and there was an increased relative abundance of pro-inflammatory bacteria, suggesting a negative effect of the D++ diet.
Are UVR-induced changes to the faecal microbiome associated with vitamin D?
We were uncertain whether it was valid to compare the effect of dietary vitamin D with that of UVR and expect a similar effect if vitamin D were the active intermediary after UV-irradiation of skin, particularly if serum 25(OH)D3 levels differed significantly. The D++, D+ and D− mice had respective levels of 25(OH)D3 after 5 weeks on the diets of 101 ± 5 nmol L−1 (mean ± SD), 41 ± 2 nmol L−1 and 12 ± 4 nmol L−1.18 The induction of 25(OH)D3 by UVR was small and the increases were not significant for mice fed a D+ or D++ diet.18 Comparisons were only made between D+ and D− mice because the D++ diet stimulated considerable gut inflammation as described above. Thus, in the next analysis, the microbiome in faecal pellets from mice with similar serum 25(OH)D3 levels were examined.15 In one group, mice had attained a mean 25(OH)D3 level of 40 nmol L−1 solely from a diet supplemented with 2280 IU kg−1 vitamin D3. The other group of mice had received no vitamin D in their diet but had been UV-irradiated (1 kJ m−2) on their shaved back on 6 occasions (daily on 4 consecutive days and then twice weekly for one week). With UV-irradiation, their 25(OH)D3 levels had increased from 6 nmol L−1 at baseline, to 40 nmol L−1. It was hypothesised that if the UVR-effects on the microbiota (see above) were due to UVR-induced vitamin D3, the composition and diversity of the microbiome in the two groups of mice would be similar. This study was completed in mice recovering from DSS-colitis; it was proposed that the preconditioning by the UVR or vitamin D prior to DSS, and the continuing UVR and diet, would have controlled the microbiota post-DSS. However, there were significant differences in overall beta-diversity between the groups.17 An examination of significant differences in taxa showed that Rickenellaceae, S45_7 and Akkermansia were dominant in mice receiving vitamin D from their diet; dominance was defined as a linear discriminant analysis score >3.5 and a p < 0.05.17Actinomycetales, Mucispirillum, Lactobacillus, Methylibium, Flexispira, Enterobacteriaceae and Photobacterium were significantly dominant in UV-irradiated mice on vitamin D-deficient diets (Table 2). Further experimentation is required to understand potential consequences of these relative changes in gut bacteria. However, the results suggest that UVR and dietary vitamin D may have different effects on the gut microbiome.
Table 2 Differential abundance of bacteria in faecal samples. In both the human and mouse studies, linear discriminant analysis scores were calculated for individual taxa that significantly change in relative abundance. In the human studies, UVR-induced changes were detected only for those that had not been supplemented with vitamin D over the previous 3 months; relative changes pre- and post-UVR exposure in those individuals are shown. In the murine studies, the comparison is between mice that had similar serum 25(OH)D3 levels after UVR exposure or after inclusion of vitamin D in their diet. These mice were recovering from DSS-induced colitis
Phylum |
Family |
Genus |
Relative changes in bacterial abundance after UVR exposure by non-vitamin D-supplemented individuals (narrowband UVR, 3 exposures of ∼0.7 MED in 1 week) |
Changes in bacterial abundance in mice with similar 25(OH)D levels from UVR exposure or diet |
UVR exposure in absence of dietary vitamin D: Vitamin D-deficient mice exposed to broadband UVR (4 daily exposures, then 2 exposures in 1 week, all ∼0.5 MED) |
Dietary vitamin D: Mice receiving vitamin D by diet, no UVR exposure |
Firmicutes
|
Lachnospiraceae
|
|
↑ |
|
|
Firmicutes
|
Lachnospiraceae
|
Coprococcus
|
|
↑ |
|
Firmicutes
|
Clostridiales vadin BB60 group
|
Clostridiales vadin BB60 group
|
↑ |
|
|
Firmicutes
|
Clostridia Family XIII
|
Family XIII AD3011group
|
↑ |
|
|
Firmicutes
|
Lactobacillaceae
|
Lactobacillus
|
|
↑ |
|
Firmicutes
|
Clostridiaceae
|
Clostridiaceae_unclassified
|
|
↑ |
|
Firmicutes
|
Clostridiaceae
|
Clostridium
|
|
↑ |
|
Bacteroidetes
|
Rikenellaceae
|
Alistipes
|
↑ |
|
↑ |
|
Rikenellaceae_unclassified
|
Bacteroidetes
|
S24_7
|
|
|
|
↑ |
Bacteroidetes
|
Marinifilaceae
|
Odoribacter
|
↑ |
|
|
Actinobacteria
|
Coriobacteriaceae
|
Colinsella
|
↑ |
|
|
Actinobacteria
|
Actinomycineae
|
Actinomycetales_unclassifed
|
|
↑ |
|
Deferribacteres
|
Deferribacteraceae
|
Mucispirillum
|
|
↑ |
|
Proteobacteria
|
Desulfovibrionaceae
|
Bilophila
|
↑ |
↑ |
|
Desulfovibrionaceae.unclassified
|
Proteobacteria
|
Unassigned
|
Methylibium
|
|
↑ |
|
Proteobacteria
|
Helicobacteraceae
|
Flexispira
|
|
↑ |
|
Proteobacteria
|
Enterobacteriaceae
|
Enterobacteriaceae_unclassified
|
|
↑ |
|
Proteobacteria
|
Vibrionaceae
|
Photobacterium
|
|
↑ |
|
Verrucomicrobia
|
Akkermansiaceae
|
Akkermansia
|
|
|
↑ |
Comparison of the effects of UVR on the human and murine gut microbiome
Table 1 demonstrates that the effects of repeated sub-erythemal UVR exposure on the gut microbiota at the phyla level were similar in humans and mice. For these data, humans were exposed to narrowband UVR 3 times over 7 days, whilst mice received 6 broadband UVR exposures over 11 days. In subsequent studies comparing the effects of UVR on human and murine microbiota, differentially abundant bacterial families were examined by Linear Discriminant analysis (Table 2). Importantly, the studies of mice with similar serum 25(OH)D3 levels obtained by vitamin D supplementation or UVR exposure, respectively, allowed us to suggest which gut bacteria may be altered in relative abundance in humans in response to dietary vitamin D (e.g. Rikenellaceae) and those altered by exposure to UVR (e.g. Lachnospiraceae, Desulfovibrionaceae).
Mechanism of UVR regulation of the gut microbiome
There are no reports of gut microbial species expressing the vitamin D receptor. Dietary vitamin D requires absorption and hydroxylation to the active vitamin D metabolite, 1,25(OH)2D; this metabolite may then interact with intestinal epithelial cells and other gut lining cells such as dendritic cells.22 Studies of commensals added to GF mice have shown that the microbiota, through controlling fibroblast growth factor levels, can regulate vitamin D metabolism.23 In contrast, UVR-induced vitamin D, if it is responsible for the effects of UVR, would be systemic and by compartmentalisation, may have lesser access to gut-lining cells that help to control the diversity and prevalence of gut microbiota.
Even after avoiding the pro-inflammatory effects of high dose vitamin D supplementation (D++) on the gut microbiota, skin UVR exposure was associated with a gut microbiome in mice recovering from DSS-induced colitis different to that measured in mice made vitamin D-sufficient by dietary means.17 This may reflect UVR-induced changes to components of the immune system, and subsequent immune cell-microbiota crosstalk. In addition, soluble products from cells of the skin or draining lymph nodes after UVR exposure (for review2–4) may modulate the function of intestinal epithelial cells; these cells are important intermediaries in the two-way interactions between gut microbiota and the cells in and behind the intestinal wall.24 In D+ mice receiving chronic sub-erythemal UVR (6 irradiations over 11 days), serum IL-17 levels were reduced (p = 0.05) compared with mice receiving the same diet but not irradiated.17 In the comparison of mice attaining their vitamin D via their diet (D+UVR−) or by UVR exposure (D−UVR+), proximal colon expression of CYP27B1, the enzyme responsible for 25(OH)D to 1,25(OH)2D conversion, was 4-fold greater in the UV-irradiated mice.17 In addition, IL-1β mRNA levels were reduced 5-fold.17 These changes suggest less inflammation in the circulation and gut wall of UV-irradiated mice and that the reduced inflammation may facilitate greater diversity of the microbiota as seen in the UV-irradiated mice. There is evidence that high-affinity microbiota-specific IgA are important in controlling diverse, mucosa-associated bacterial communities;25 T follicular helper cells in germinal centres of gut lymph nodes are important to class switch recombination and somatic recombination for IgA production.26 UVR exposure has been associated with reduced development of T follicular helper cells in mice.27 Further experimentation is required to determine if there are changes to IgA levels in the gut of UV-irradiated mice.
Mechanism of vitamin D regulation of the gut microbiome
There is considerably more known on mechanisms by which dietary vitamin D may influence the gut microbiota. Observational human studies have suggested some beneficial, although inconsistent, associations between vitamin D and the gut microbiome.28 In one study of young healthy adults, there was an inverse correlation between the relative abundance of Coprococcus and serum 25(OH)D levels.29 The relationship survived correction against age, sex, season and BMI, but not for inflammatory markers.29 In murine studies, researchers have generally studied the effects of vitamin D-sufficiency versus vitamin D-insufficiency on gut microbiota and their interaction with the host immune system. In the cecum of vitamin D-sufficient mice, higher numbers of members of the genus Clostridium and Bacteroides have been reported,30 with a significant, linear correlation between the numbers of Clostridium XIVa and XVIII and the frequency of colonic RORγt/FoxP3+ T regulatory cells.30 In turn, FoxP3+ T cells can regulate IgA selection and facilitate diversification of bacterial species responsible for immune homeostasis.25
Colonic transfer studies from vitamin D-sufficient to -deficient mice and administration of 1,25(OH)2D3 to vitamin D-deficient mice have suggested that the impact of dietary vitamin D on stimulating changes to the immune system may be greatest in early life at the time of immune system development, and may be less relevant to the studies discussed above in which the mice were 6 weeks old before administration of the vitamin D diets. Studies of a conditional VDR knockout mouse have also shown an important role for vitamin D binding to the vitamin D receptor in intestinal epithelial cells for control of intestinal homeostasis by regulating intestinal autophagy and the microbiome.31 More specifically, in a mouse model of colitis, expression of low levels of the vitamin D receptor in intestinal epithelial cells correlated with decreased expression of the autophagy gene, ATG16L1. Administration of the bacterial product butyrate increased expression of both factors and in turn, less microbiota-associated gut inflammation.31
Impact of changes by UVR and vitamin D on the gut microbiome
The relevance and impact of UVR and dietary vitamin D on changes to the gut microbiota were examined in mice with colitis induced by the chemical DSS. DSS-induced colitis is driven by immune cells responding to pro-inflammatory signals following disturbance in the gut epithelial barrier. With a pro-inflammatory gut microbial profile after 5 weeks on a high vitamin D-supplemented diet, it was not surprising that more severe colitis was measured in the mice fed a high vitamin D diet for 5 weeks prior to DSS administration.18 In our experimental conditions, however, mice fed the vitamin D-deficient diet were not more susceptible to DSS-colitis than mice fed a vitamin D-sufficient diet. UVR exposure also had no effect on the initiation or resolution of DSS-induced colitis.17 These results raise the question of the suitability of the DSS-colitis model to examine the effect of changes to the gut microbiome that are less dramatic than those measured in D++ mice. In future experiments, models of colitis driven by immunological dysfunction should be investigated as they may be more susceptible to lesser but significant changes in microbial diversity and abundance.
Gut back to skin communication
The signalling between UV-irradiated skin and the gut may be two-directional. As a consequence of UVR-modulation of the gut microbiome, molecular products of altered gut bacteria may modify the physiological properties of skin. The gut-skin axis has been covered in several reviews whereby metabolites from the gut microbiota are skin accessible,32,33 and they may be relevant for treatment of skin diseases like atopic dermatitis. These products may include short chain fatty acids from carbohydrate metabolism (e.g. butyrate, propionate) and tryptophan and lipid catabolites (e.g. indole and conjugated linoleic acid, respectively).14,32 Further studies are required to examine feedback by products of the gut microbiota altered by UVR exposure or dietary vitamin D and their ability to regulate the integrity of the skin barrier and skin immunity.
Conclusions
Chronic sub-erythemal UVR exposure of the skin of humans and mice can increase alpha diversity of the gut microbiome. At the phyla level, changes in humans exposed to narrowband UVB radiation, and in mice exposed to broadband UVR, showed a relative increase in the Firmicutes
:
Bacteroidetes ratio (Table 1). The impact of these changes has been difficult to interpret. The gut microbiome changes induced by UVR in mice were associated with reduced circulating IL-17, and a marker suggesting less inflammation in the colon. However, the relevance of the microbiota changes requires more experimentation. Many autoimmune diseases have now been associated with a misbalance of homeostatic processes controlling gut bacteria;14,34 it may be rewarding to study the comparative effects of vitamin D supplementation by diet and by UVR exposure on models of these diseases. Future studies should be better controlled than those reviewed in this perspective, and with increased power due to larger numbers of participants and mice. At least in mice, the amount of vitamin D in the diet can be better controlled than in humans where vitamin D status may be influenced by vitamin D supplementation, differences in diet and casual sun exposure. Narrowband UVB radiation (311 nm) was used in the human trial, broadband UVR (290–400 nm) in the mouse studies. Comparative studies must be better co-ordinated for use of similar radiation; future studies may investigate the modulatory effects of other wavelengths.
If UVR exposure can induce a gut microbiome with increased diversity and relative abundance of the phylum Firmicutes, it is important to know if dietary vitamin D can replicate these changes and thus, similar benefits be obtained without sun exposure Alternatively, if the benefits are by vitamin D-independent mechanisms, exposure to UVR may be required. In the studies reviewed in the perspective, there was no evidence that mice receiving vitamin D from their diet have a gut microbiome similar to that of mice obtaining their vitamin D by UVR exposure. In contrast, the authors of the study of humans exposed to narrowband UVB concluded that UVR-induced vitamin D3 was responsible for the microbiome changes. Studies of UV-irradiated mice deficient in the vitamin D receptor, or CYP27B1, and therefore unable to make the active form of vitamin D, will help in the future to determine if UVR-induced vitamin D contributes to the mechanisms by which UVR exposure can modulate the gut microbiome. It is possible that in a manner similar to the ability of UVR to regulate immunity,2,3 there may be multiple pathways by which UVR regulates the gut microbiome, including those dependent on, and others independent of, vitamin D synthesis.
Conflicts of interest
There are no conflicts to declare.
Acknowledgements
The authors acknowledge an GESA-Ferring IBD Clinician Establishment Award to SG.
References
- P. H. Hart, R. M. Lucas, D. R. Booth, W. M. Carroll, D. Nolan, J. M. Cole, A. P. Jones and A. G. Kermode, Narrowband UVB phototherapy for clinically isolated syndrome: A trial to deliver the benefits of vitamin D and other UVB-induced molecules, Front. Immunol., 2017, 8, 3 CrossRef PubMed
.
- P. H. Hart and M. Norval, Ultraviolet radiation-induced immunosuppression and its relevance for skin carcinogenesis, Photochem. Photobiol. Sci., 2018, 17, 1872–1884 RSC
.
- P. H. Hart, M. Norval, S. N. Byrne and L. E. Rhodes, Exposure to ultraviolet radiation in the modulation of human diseases, Annu. Rev. Phytopathol., 2019, 14, 55–81 CrossRef CAS PubMed
.
- J. J. Bernard, R. L. Gallo and J. Krutmann, Photoimmunology: how ultraviolet radiation affects the immune system, Nat. Rev. Immunol., 2019, 19, 688–701 CrossRef CAS PubMed
.
- D. Liu, B. O. Fernandez, A. Hamilton, N. N. Lang, J. M. C. Gallagher, D. E. Newby, M. Feelisch and R. B. Weller, UVA irradiation of human skin vasodilates arterial vasculature and lowers blood pressure independently of nitric oxide synthase, J. Invest. Dermatol., 2014, 134, 1839–1846 CrossRef CAS PubMed
.
- S. Geldenhuys, P. H. Hart, R. Endersby, P. Jacoby, M. Feelisch, R. B. Weller, V. Matthews and S. Gorman, Ultraviolet radiation suppresses obesity and symptoms of metabolic syndrome independently of vitamin D in mice fed a high-fat diet, Diabetes, 2014, 63, 3759–3769 CrossRef CAS PubMed
.
- N. Fleury, M. Feelisch, P. H. Hart, R. B. Weller, J. Smoothy, V. B. Matthews and S. Gorman, Sub-erythemal ultraviolet radiation reduces metabolic dysfunction in already overweight mice, J. Endocrinol., 2017, 233, 81–92 CAS
.
- E. S. Bosman, A. Y. Albert, H. Lui, J. P. Dutz and B. A. Vallance, Skin exposure to narrow band ultraviolet (UVB) light modulates the human intestinal microbiome, Front. Microbiol., 2019, 10, 2410 CrossRef PubMed
.
- A. Schwarz, A. Bruhs and T. Schwarz, The short-chain fatty acid sodium butyrate functions as a regulator of the skin immune system, J. Invest. Dermatol., 2017, 137, 855–864 CrossRef CAS PubMed
.
- S. Felton, F. Navid, A. Schwarz, T. Schwarz, R. Glaser and L. E. Rhodes, Ultraviolet radiation-induced upregulation of antimicrobial proteins in health and disease, Photochem. Photobiol. Sci., 2013, 12, 29–36 RSC
.
- V. K. Patra, K. Wagner, V. Arulampalam and P. Wolf, Skin microbiome modulates the effect of ultraviolet radiation on cellular response and immune function, iScience, 2019, 15, 211–222 CrossRef CAS PubMed
.
- M. Tanaka and J. Nakayama, Development of the gut microbiota in infancy and its impact on health in later life, Allergol. Int., 2017, 66, 515–522 CrossRef CAS PubMed
.
- M. Assarsson, A. Duvetorp, O. Dienus, J. Soderman and O. Seifert, Significant changes in the skin microbiome in patients with chronic plaque psoriasis after treatment with narrowband ultraviolet B, Acta Derm.-Venereol., 2018, 98, 428–436 CrossRef CAS PubMed
.
- J. Durack and S. V. Lynch, The gut microbiome: Relationships with disease and opportunities for therapy, J. Exp. Med., 2019, 216, 20–40 CrossRef CAS PubMed
.
- P. I. Costea, F. Hildebrand, M. Arumugam, F. Bäckhed, M. J. Blaser, F. D. Bushman, W. M. de Vos, S. D. Ehrlich, C. M. Fraser, M. Hattori, C. Huttenhower, I. B. Jeffery, D. Knights, J. D. Lewis, R. E. Ley, H. Ochman, P. W. O'Toole, C. Quince, D. A. Relman, F. Shanahan, S. Sunagawa, J. Wang, G. M. Weinstock, G. D. Wu, G. Zeller, L. Zhao, J. Raes, R. Knight and P. Bork, Enterotypes in the landscape of gut microbial community composition, Nat. Microbiol., 2018, 3, 8–16 CrossRef CAS PubMed
.
- A. D. Truax, L. Chen, J. W. Tam, N. Cheng, H. Guo, A. A. Koblansky, W. C. Chou, J. E. Wilson, W. J. Brickey, A. Petrucelli, R. Liu, D. E. Cooper, M. J. Koenigsknecht, V. B. Young, M. G. Netea, R. Stienstra, R. B. Sartor, S. A. Montgomery, R. A. Coleman and J. P. Ting, The inhibitory innate immune sensor NLRP12 maintains a threshold against obesity by regulating gut microbiota homeostasis, Cell Host Microbe, 2018, 24, 364–378.e6 CrossRef CAS PubMed
.
- S. Ghaly, N. O. Kaakoush, F. Lloyd, L. Gordon, C. Forest, I. C. Lawrance and P. H. Hart, Ultraviolet irradiation of skin alters the faecal microbiome independently of vitamin D in mice, Nutrients, 2018, 10(8), 1069 CrossRef PubMed
.
- S. Ghaly, N. O. Kaakoush, F. Lloyd, T. McGonigle, D. Mok, A. Baird, B. Klopcic, L. Gordon, S. Gorman, C. Forest, R. Bouillon, I. C. Lawrance and P. H. Hart, High dose vitamin D supplementation alters faecal microbiome and predisposes mice to more severe colitis, Sci. Rep., 2018, 8, 11511 CrossRef PubMed
.
- P. H. Hart, M. A. Grimbaldeston, G. J. Swift, A. Jaksic, F. P. Noonan and J. J. Finlay-Jones, Dermal mast cells determine susceptibility to ultraviolet B-induced systemic suppression of contact hypersensitivity responses in mice, J. Exp. Med., 1998, 187, 2045–2053 CrossRef CAS PubMed
.
- T. Gaschott, O. Werz, A. Steinmeyer, D. Steinhilber and J. Stein, Butyrate-induced differentiation of Caco-2 cells is mediated by vitamin D receptor, Biochem. Biophys. Res. Commun., 2001, 288, 690–696 CrossRef CAS PubMed
.
- M. L. Santoru, C. Piras, A. Murgia, V. Palmas, T. Camboni, S. Liggi, I. Ibba, M. A. Lai, S. Orru, S. Blois, A. L. Loizedda, J. L. Griffin, P. Usai, P. Caboni, L. Atzori and A. Manzin, Cross sectional evaluation of the gut-microbiome metabolome axis in an Italian cohort of IBD patients, Sci. Rep., 2017, 7, 9523 CrossRef PubMed
.
- S. A. Bora, M. J. Kennett, P. B. Smith, A. D. Patterson and M. T. Cantorna, The gut microbiota regulates endocrine vitamin D metabolism through fibroblast growth factor 23, Front. Immunol., 2018, 9, 408 CrossRef PubMed
.
- M. T. Cantorna, K. McDaniel, S. Bora, J. Chen and J. James, Vitamin D, immune regulation, the microbiota, and inflammatory bowel disease, Exp. Biol. Med., 2014, 239, 1524–1530 CrossRef PubMed
.
- A. T. Soderholm and V. A. Pedicord, Intestinal epithelial cells: at the interface of the microbiota and mucosal immunity, Immunology, 2019, 158, 267–280 CrossRef CAS PubMed
.
- S. Kawamoto, M. Maruya, L. M. Kato, W. Suda, K. Atarashi, Y. Doi, Y. Tsutsui, H. Qin, K. Honda, T. Okada, M. Hattori and S. Fagarasan, Foxp3+ T cells regulate immunoglobulin A selection and facilitate diversification of bacterial species responsible for immune homeostasis, Immunity, 2014, 41, 152–165 CrossRef CAS PubMed
.
- K. Honda, TFH-IgA responses keep microbiota in check, Cell Host Microbe, 2015, 17, 144–146 CrossRef CAS PubMed
.
- R. Chacon-Salinas, A. Y. Limon-Flores, A. D. Chavez-Blanco, A. Gonzalez-Estrada and S. E. Ullrich, Mast cell-derived IL-10 suppresses germinal center formation by affecting T follicular helper cell function, J. Immunol., 2011, 186, 25–31 CrossRef CAS
.
- M. Waterhouse, B. Hope, L. Krause, M. Morrison, M. M. Protani, M. Zakrzewski and R. E. Neale, Vitamin D and the gut microbiome: a systematic review of in vivo studies, Eur. J. Nutr., 2019, 58, 2895–2910 CrossRef CAS
.
- R. V. Luthold, G. R. Fernandes, A. C. Franco-de-Moraes, L. G. D. Folchetti and S. R. G. Ferreira, Gut microbiota interactions with the immunomodulatory role of vitamin D in normal individuals, Metabolism, 2017, 69, 76–86 CrossRef CAS PubMed
.
- M. T. Cantorna, Y.-D. Lin, J. Arora, S. Bora, Y. Tian, R. G. Nichols and A. D. Patterson, Vitamin D regulates the microbiota to control the numbers of RORγt/FoxP3+ regulatory T cells in the colon, Front. Immunol., 2019, 10, 1772 CrossRef
.
- S. Wu, Y.-G. Zhang, R. Lu, Y. Xia, D. Zhou, E. O. Petrof, E. C. Claud, D. Chen, E. B. Chang, G. Carmeliet and J. Sun, Intestinal epithelial vitamin D receptor deletion leads to defective autophagy in colitis, Gut, 2015, 64, 1082–1094 CrossRef CAS
.
- C. A. O'Neill, G. Monteleone, J. T. McLaughlin and R. Paus, The gut-skin axis in health and disease: A paradigm with therapeutic implications, Bioessays, 2016, 38, 1167–1176 CrossRef PubMed
.
- J. S. Meisel, G. Sfyroera, C. Bartow-McKenney, C. Gimblet, J. Bugayev, J. Horwinski, B. Kim, J. R. Brestoff, A. S. Tyldsley, Q. Zheng, B. P. Hodkinson, D. Artis and E. A. Grice, Microbiome, 2018, 6, 20 CrossRef PubMed
.
- S. N. Freedman, S. K. Shahi and A. K. Mangalam, The “Gut Feeling”: breaking down the role of gut microbiome in multiple sclerosis, Neurotherapeutics, 2018, 15, 109–125 CrossRef PubMed
.
|
This journal is © The Royal Society of Chemistry and Owner Societies 2020 |
Click here to see how this site uses Cookies. View our privacy policy here.