DOI:
10.1039/C8CC09751H
(Communication)
Chem. Commun., 2019,
55, 1915-1918
Synthesis of dihydroquinolinones via iridium-catalyzed cascade C–H amidation and intramolecular aza-Michael addition†
Received
9th December 2018
, Accepted 18th January 2019
First published on 18th January 2019
Abstract
An iridium-catalyzed annulation of chalcones with sulfonyl azides via cascade C–H amidation and intramolecular aza-Michael addition was developed, affording a variety of 2-aryl-2,3-dihydro-4-quinolones in moderate to good yields. This reaction features easy operation, readily available starting materials, and the cascade formation of two C–N bonds in one pot.
Quinolones are a class of compounds with a broad spectrum of antibiotic properties.1 In particular, 2-aryl-2,3-dihydro-4-quinolones, namely azaflavanones, have attracted more attention due to their unique bioactivities such as antimalarial activity and cytotoxic activity against a panel of human tumor cell lines and potent cross-species microRNA inhibitors.2 Besides, they also serve as key intermediates in the synthesis of many biologically active natural products.3 Thus the synthesis of quinolones from readily available starting materials is highly desirable. Traditionally, the construction of the 2,3-dihydro-4-quinolone skeleton could be achieved via intermolecular Fries-type rearrangement of aryl β-lactams catalyzed by Brønsted or Lewis acids,4 PPA-promoted intramolecular Friedel–Crafts acylation of 3-(arylamino)propanoic acids or esters,5 Michael addition of 2-alkenoylanilines in the presence of appropriate bases,6 and palladium-catalyzed intermolecular cyclizations.7 In the case of 2-aryl-2,3-dihydroquinolin-4-ones, the condensation of o-aminoacetophenones and aryl aldehydes in the presence of certain organocatalysts was frequently employed (Scheme 1, eqn (1)).8 In addition, intramolecular aza-Michael addition of o-aminochalcones was also utilized in the successful construction of this structure under the catalysis of transition-metals9 or organocatalysts (Scheme 1, eqn (2)).10 However, throughout the abovementioned approaches, either intermolecular or intramolecular reactions, odorous and vulnerable arylamines were used as essential substrates with limited substrate scopes. As a result, more general, clean, and efficient methods are required for the direct construction of 2-aryl-2,3-dihydro-4-quinolone derivatives from readily available starting materials.
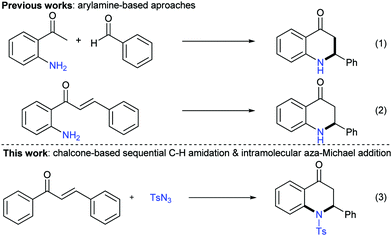 |
| Scheme 1 Approaches for the synthesis of 2-aryl-2,3-dihydro-4-quinolones. | |
Direct C–H amidation was proved to be a convenient approach for C–N bond formation with high atom-economy.11 In 2012, Chang pioneered direct C–H amidation employing sulfonyl azides as the N-sources with gaseous nitrogen as a clean by-product.12 Subsequently, Chang's13 and other groups14,15 deeply demonstrated organic azide-involved direct C–H amidation as a reliable and efficient method for the construction of C–N bonds. Additionally, chalcones, which can be conveniently synthesized from aryl aldehydes and 1-arylethanones via Claisen–Schmidt condensation, are an important class of compounds that exhibit a broad range of biological activities (anticancer, antidiabetic, anti-HIV, and anti-inflammatory).16 The β-H activation or ortho-C–H bond activation of chalcones was selectively achieved under transition-metal catalyzed conditions.17 However, sequential ortho-C–H bond activation and aza-Michael addition in one pot has never been reported. In this paper, we aimed to accomplish the annulation of chalcones with sulfonyl azides via ortho-C–H bond amidation and then to fulfill the aza-Michael addition in one pot for the construction of 2-aryl-2,3-dihydro-4-quinolones with high atom- and step-economy (Scheme 1, eqn (3)).
To achieve our goals, we started to screen the reaction conditions for the cascade amidation and aza-Michael addition of chalcone (1a) with tosyl azide (2a) using Rh(III) as the catalyst and AgSbF6 as the additive (Table 1, entry 1). However, only a trace amount of product was detected, even after we switched the catalyst to Ru(II) (Table 1, entry 2). To our delight, the desired product 2-phenyl-1-tosyl-2,3-dihydroquinolin-4(1H)-one (3aa) was obtained in 26% yield when catalyzed by [Cp*IrCl2]2 (Table 1, entry 3). Encouraged by this result, other reaction parameters were then extensively investigated to further optimize the reaction. For example, AgNTf2 was a better silver source than AgBF4 (Table 1, entries 4 and 5). Other solvents such as DCM, toluene, acetonitrile, and methanol were tested, and the results indicated that DCM was the best choice (Table 1, entries 6–9). Increasing the temperature to 120 °C resulted in a higher yield (Table 1, entry 6). The yields increased remarkably after adding one equivalent amount of acid. Product 3aa was isolated in 77% yield in the presence of pivalic acid (Table 1, entry 10).
Table 1 Screening the optimized reaction conditionsa
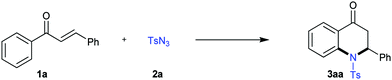
|
Entry |
Catalyst |
Additive |
Solvent |
Yieldb (%) |
Reaction conditions: 1a (0.2 mmol), 2a (0.4 mmol), catalyst (5 mol%), additive (20 mol%) in solvent (2 mL) at 100 °C for 12 h.
At 120 °C.
Adding HOAc (1 equiv.), 120 °C.
Adding PivOH (1 equiv.), 120 °C.
|
1 |
[Cp*RhCl2]2 |
AgSbF6 |
DCE |
Trace |
2 |
[Ru(p-cymene)Cl2]2 |
AgSbF6 |
DCE |
Trace |
3 |
[Cp*IrCl2]2 |
AgSbF6 |
DCE |
26 |
4 |
[Cp*IrCl2]2 |
AgNTf2 |
DCE |
30 |
5 |
[Cp*IrCl2]2 |
AgBF4 |
DCE |
Trace |
6 |
[Cp*IrCl2]2 |
AgNTf2 |
DCM |
38 (49)b |
7 |
[Cp*IrCl2]2 |
AgNTf2 |
Toluene |
0 |
8 |
[Cp*IrCl2]2 |
AgNTf2 |
MeCN |
Trace |
9 |
[Cp*IrCl2]2 |
AgNTf2 |
MeOH |
7 |
10 |
[Cp*IrCl2]2 |
AgNTf2 |
DCM |
63c (77)d |
After the establishment of the optimized reaction conditions, the substrate scope of this cascade amidation and aza-Michael addition reaction was investigated as listed in Fig. 1. As expected, chalcones with different substituents on aryls adjacent to alkenyl have little influence on the efficiency of this reaction, leading to the corresponding 2-aryl-2,3-dihydro-4-quinolone products in good yields (3aa–3ka, 85–65% yields). Electron donating groups, such as methyl, tert-butyl, and phenyl, or electron withdrawing groups, such as halo, nitro, and methoxycarbonyl, at either the meta- or para-position of phenyls, were all well tolerated. Next, the substituent influences on aryls adjacent to the carbonyls of chalcones were tested. Methyl, halo, and methoxycarbonyl at the para-position of phenyl all reacted smoothly to generate the products in good yields (3la–3qa, 74–55% yields). The tolerance of halo produced opportunities for further derivations (3ea–3ha, 3ma–3pa, and 3ua). In particular, iodo remains innocent under this Ir-catalyzed condition to produce 3pa in 55% yield. Notably, steric hindrance had a negative influence on this reaction, for chalcones with ortho-substituents generated the products in lower yields (3ra & 3sa). In order to investigate the selectivity of this reaction, meta-substituted substrates 1t and 1u were subjected to the reaction, leading to the corresponding products in moderate yields with excellent regioselectivities (3ta & 3ua). In addition, if the β-aryl was replaced with alkyl (1v), 2-alkyl-2,3-dihydroquinolin-4(1H)-one (3va) could be obtained in moderate yield. Other sulfonyl azides, such as benzenesulfonyl azide, 4-methoxybenzenesulfonyl azide, and 4-chlorobenzenesulfonyl azide, were also proved to be efficient N-sources, leading to the corresponding dihydroquinolin-4(1H)-ones in good to moderate yields (3ab–3ad).
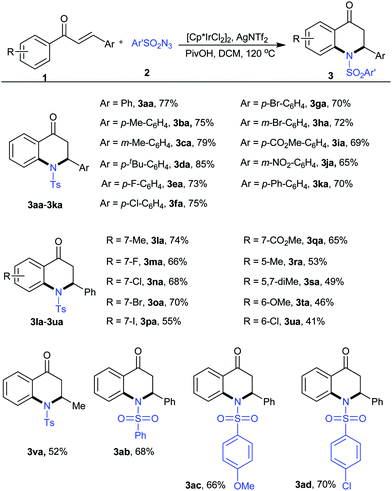 |
| Fig. 1 Scope of the Ir-catalyzed cascade amidation and aza-Michael addition reactions.a aReaction conditions: 1 (0.2 mmol), 2 (0.4 mmol), [Cp*IrCl2]2 (5 mol%), AgNTf2 (20 mol%), PivOH (1 equiv.) in DCM (2 mL) at 120 °C for 12 h, isolated yields. | |
Interestingly, if NaOAc was employed in the model reaction instead of pivalic acid, only ortho-amidation occurred, and no cyclisation product was detected at all. Other chalcones with different substituents all gave the amidation products in good to moderate yields in the presence of AgSbF6 as the silver source (Fig. 2, 4a–4f). This result was similar to Chang's.17f
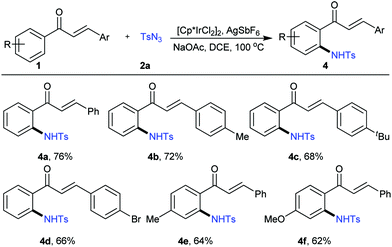 |
| Fig. 2 Scope of the Ir-catalyzed cascade amidation and aza-Michael addition reactions.a aReaction conditions: 1 (0.2 mmol), 2a (0.4 mmol), [Cp*IrCl2]2 (5 mol%), AgSbF6 (20 mol%), NaOAc (1 equiv.) in DCE (2 mL) at 100 °C for 12 h, isolated yields. | |
Control experiments were conducted to investigate the mechanism of this reaction as listed in Scheme 2. Product 3aa could be isolated in 89% yield from 4a catalyzed by [Cp*IrCl2]2 in the presence of AgNTf2 (Scheme 2, eqn (a)). However, no product was formed in the absence of any transition-metal catalysts (Scheme 2, eqn (b)). The cyclisation could occur under the catalysis of either [Cp*IrCl2]2 or AgNTf2, while the yields were lower than that under the catalysis of the Ir–Ag system (Scheme 2, eqn (c) & (d) vs. eqn (a)). These results indicated that the amidation product 4a was the reaction intermediate and the metal-catalyst was essential for the aza-Michael addition step. Besides, AgNTf2 may play an important role in the deprotonation process of 4a to form the sulfamide anion with enhanced nucleophilicity for the following aza-Michael addition.
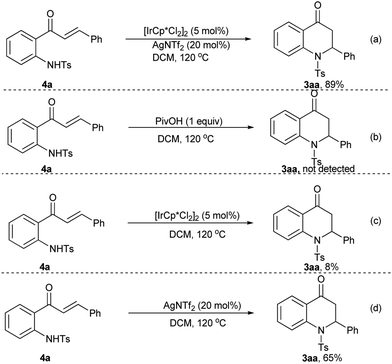 |
| Scheme 2 Mechanistic studies. | |
According to the mechanistic experiments and literature reports,13,15 a possible mechanistic pathway is proposed in Scheme 3. First, the treatment of a dimeric iridium species with AgNTf2 and PivOH generates the active Ir(III) catalyst, which induces the ortho-C–H bond cleavage of 1a to produce cyclometalated Ir(III) complex A. Subsequently, the coordination of the tosyl azide to A forms Ir-species B. The following migratory insertion of B leads to intermediate C with the release of byproduct N2. Then, protodemetalation of C provides another Ir-coordinated intermediate, D. Finally, intramolecular nucleophilic 1,4-addition of D gives compound E and regenerates the active Ir complex. Product 3aa was formed from E after enol–keto tautomerization.
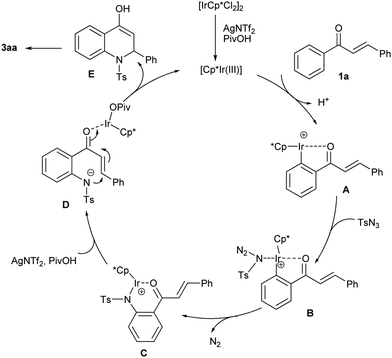 |
| Scheme 3 Proposed mechanism. | |
In summary, we have developed a cascade ortho-C–H amidation and intramolecular aza-Michael addition with chalcones and sulfonyl azides. A variety of 2-aryl-2,3-dihydro-4-quinolones with different substituents on aryls were obtained in moderate to good yields under standard conditions. This reaction features easy operation, readily available starting materials, and the cascade formation of two C–N bonds in one pot. This procedure represents an effective tool for the convenient synthesis of pharmaceutically promising heterocyclic compounds.
We thank the National Natural Science Foundation of China (21602086 and 21672028), and the Jiangsu Key Laboratory of Advanced Catalytic Materials & Technology (BM2012110) for financial support. Wang acknowledges the Postgraduate Research & Practice Innovation Program of Jiangsu Province (SJCX18_1000) for financial support.
Conflicts of interest
There are no conflicts to declare.
Notes and references
-
A. S. Wagman and M. P. Wentland, Comprehensive Medicinal Chemistry II, ed. J. B. Taylor and D. J. Triggle, Elsevier, Amsterdam, 2006, vol. 7, pp. 567–596 Search PubMed
.
-
(a) Y. Xia, Z.-Y. Yang, P. Xia, K. F. Bastow, Y. Tachibana, S.-C. Kuo, E. Hamel, T. Hackl and K.-H. Lee, J. Med. Chem., 1998, 41, 1155 CrossRef CAS PubMed
;
(b) S.-X. Zhang, J. Feng, S.-C. Kuo, A. Brossi, E. Hamel, A. Tropsha and K.-H. Lee, J. Med. Chem., 2000, 43, 167 CrossRef CAS
;
(c) S. Chandrasekhar, S. N. C. V. L. Pushpavalli, S. Chatla, D. Mukhopadhyay, B. Ganganna, K. Vijeender, P. Srihari, C. R. Reddy and M. J. Ramaiah, Bioorg. Med. Chem. Lett., 2012, 22, 645 CrossRef CAS PubMed
.
-
(a) D. Ma, C. Xia, J. Jiang and J. Zhang, Org. Lett., 2001, 3, 2189 CrossRef CAS PubMed
;
(b) D. Ma, C. Xia, J. Jiang, J. Zhang and W. Tang, J. Org. Chem., 2003, 68, 442 CrossRef CAS PubMed
.
-
(a) K. W. Anderson and J. J. Tepe, Org. Lett., 2002, 4, 459 CrossRef CAS PubMed
;
(b) S. Kano, T. Ebata and S. Shibuya, J. Chem. Soc., Perkin Trans. 1, 1980, 2105 RSC
;
(c) S. Kano, S. Shibuya and T. Ebata, Heterocycles, 1981, 15, 1011 CrossRef CAS
;
(d) R. G. Schmidt, E. K. Bayburt, S. P. Latshaw, J. R. Koenig, J. F. Daanen, H. A. McDonald, B. R. Bianchi, C. Zhong, S. Joshi, P. Honore, K. C. Marsh, C.-H. Lee, C. R. Faltynek and A. Gomtsyan, Bioorg. Med. Chem. Lett., 2011, 21, 1338 CrossRef CAS PubMed
.
-
(a) Y. Gong and K. Kato, J. Fluorine Chem., 2004, 125, 767 CrossRef CAS
;
(b) J. Song, L. M. Jones, G. E. Chavarria, A. K. Charlton-Sevcik, A. Jantz, A. Johansen, L. Bayeh, V. Soeung, L. K. Snyder, S. D. Lader Jr., D. J. Chaplin, M. L. Trawick and K. G. Pinney, Bioorg. Med. Chem. Lett., 2013, 23, 2801 CrossRef CAS PubMed
;
(c) R. L. Jarvest, J. M. Berge, V. Berry, H. F. Boyd, M. J. Brown, J. S. Elder, A. K. Forrest, A. P. Fosberry, D. R. Gentry, M. J. Hibbs, D. D. Jaworski, P. J. O’Hanlon, A. J. Pope, S. Rittenhouse, R. J. Sheppard, C. Slater-Radosti and A. Worby, J. Med. Chem., 2002, 45, 1959 CrossRef CAS PubMed
.
-
(a) N. G. Kundu, J. S. Mathnty, P. Das and B. Das, Tetrahedron Lett., 1993, 34, 1625 CrossRef CAS
;
(b) J. Donnelly and D. Farrell, Tetrahedron, 1990, 46, 885 CrossRef CAS
;
(c) A. B. Richard and N. J. Baskar, Heterocycl. Chem., 2011, 48, 613 CrossRef
.
-
(a) K. Okuro and H. Alper, J. Org. Chem., 2012, 77, 4420 CrossRef CAS PubMed
;
(b) R. Grigg, A. Liu, D. Shaw, S. Suganthan, D. E. Woodall and G. Yoganathan, Tetrahedron Lett., 2000, 41, 7125 CrossRef CAS
;
(c) T. Nemoto, T. Fukuda and Y. Hamada, Tetrahedron Lett., 2006, 47, 4365 CrossRef CAS
.
-
(a) V. Chandrasekhar, K. Vijeender and C. Sridhar, Tetrahedron Lett., 2007, 48, 4935 CrossRef
;
(b) K. Kanagaraj and K. Pitchumani, J. Org. Chem., 2013, 78, 744 CrossRef CAS PubMed
;
(c) H. Zheng, Q. Liu, S. Wen, H. Yang and Y. Luo, Tetrahedron: Asymmetry, 2013, 24, 875 CrossRef CAS
;
(d) R. P. Pandit, K. Sharma and Y. R. Lee, Synthesis, 2015, 3881 CAS
;
(e) G.-F. Pan, L. Su, Y.-L. Zhang, S.-H. Guo and Y.-Q. Wang, RSC Adv., 2016, 6, 25375 RSC
;
(f) X. Liu and Y. Lu, Org. Biomol. Chem., 2010, 8, 4063 RSC
.
-
(a) M. Castaing, S. L. Wason, B. Estepa, J. F. Hooper and M. C. Willis, Angew. Chem., Int. Ed., 2013, 52, 13280 CrossRef CAS PubMed
;
(b) R. Shintani, T. Yamagami, T. Kimura and T. Hayashi, Org. Lett., 2005, 7, 5317 CrossRef CAS PubMed
;
(c) B.-L. Lei, C.-H. Ding and X.-L. Hou, J. Am. Chem. Soc., 2009, 131, 18250 CrossRef CAS PubMed
;
(d) R. P. Pandit, K. Sharma and Y. R. Lee, Synthesis, 2015, 3881 CAS
;
(e) A. Gorepatil, P. Gorepatil, M. Gaikward, D. Mhamane, A. Phadkule and V. Ingle, Synlett, 2018, 235 CAS
.
-
(a) K. Saito, Y. Moriya and T. Akiyama, Org. Lett., 2015, 17, 3202 CrossRef CAS PubMed
;
(b) S. Cheng, L. Zhao and S. Yu, Adv. Synth. Catal., 2014, 356, 982 CrossRef CAS
;
(c) X. Liu and Y. Lu, Org. Lett., 2010, 12, 5592 CrossRef CAS PubMed
;
(d) M. Chelghoum, M. Bahnous, A. Bouraiou, S. Bouacida and A. Belfaitah, Tetrahedron Lett., 2012, 53, 4059 CrossRef CAS
.
-
(a) K. Shin, H. Kim and S. Chang, Acc. Chem. Res., 2015, 48, 1040 CrossRef CAS PubMed
;
(b) Y. Park, Y. Kim and S. Chang, Chem. Rev., 2017, 117, 9247 CrossRef CAS PubMed
;
(c) M.-L. Louillat and F. W. Patureau, Chem. Soc. Rev., 2014, 43, 901 RSC
;
(d) T. Ramirez, B. Zhao and Y. Shi, Chem. Soc. Rev., 2012, 41, 931 RSC
.
- J. Y. Kim, S. H. Park, J. Ryu, S. H. Cho, S. H. Kim and S. Chang, J. Am. Chem. Soc., 2012, 134, 9110 CrossRef CAS PubMed
.
-
(a) J. Ryu, K. Shin, S. H. Park, J. Y. Kim and S. Chang, Angew. Chem., Int. Ed., 2012, 51, 9904 CrossRef CAS PubMed
;
(b) H. Hwang, J. Kim, J. Jeong and S. Chang, J. Am. Chem. Soc., 2014, 136, 10770 CrossRef CAS PubMed
;
(c) J. Ryu, J. Kwak, K. Shin, D. Lee and S. Chang, J. Am. Chem. Soc., 2013, 135, 12861 CrossRef CAS PubMed
;
(d) T. Kang, Y. Kim, D. Lee, Z. Wang and S. Chang, J. Am. Chem. Soc., 2014, 136, 4141 CrossRef PubMed
;
(e) H. Kim, G. Park, J. Park and S. Chang, ACS Catal., 2016, 6, 5922 CrossRef
;
(f) Y. Kim, J. Park and S. Chang, Org. Lett., 2016, 18, 1892 CrossRef CAS PubMed
.
-
(a) D.-G. Yu, M. Suri and F. Glorius, J. Am. Chem. Soc., 2013, 135, 8802 CrossRef CAS PubMed
;
(b) S. Kim, P. Chakrasali, H. S. Suh, N. K. Mishra, T. Kim, S. H. Han, H. S. Kim, B. M. Lee, S. B. Han and I. S. Kim, J. Org. Chem., 2017, 82, 7555 CrossRef CAS PubMed
;
(c) Q.-Z. Zheng, Y.-F. Liang, C. Qin and N. Jiao, Chem. Commun., 2013, 49, 5654 RSC
;
(d) X. Xiao, G. Jia, F. Liu, G. Ou and Y. Xie, J. Org. Chem., 2018, 83, 13811 CrossRef CAS PubMed
;
(e) D. Intrieri, P. Zardi, A. Caselli and E. Gallo, Chem. Commun., 2014, 50, 11440 RSC
;
(f) V. Lankea and K. R. Prabhu, Chem. Commun., 2017, 53, 5117 RSC
;
(g) Y. Li, Y. Feng, L. Xu, L. Wang and X. Cui, Org. Lett., 2016, 18, 4924 CrossRef CAS PubMed
;
(h) C. Pan, N. Jin, H. Zhang, J. Han and C. Zhu, J. Org. Chem., 2014, 79, 9427 CrossRef CAS PubMed
;
(i) C. Pan, A. Abdukader, J. Han, Y. Cheng and C. Zhu, Chem. – Eur. J., 2014, 20, 3606 CrossRef PubMed
;
(j) M. Bhanuchandra, M. R. Yadav, R. K. Rit, M. R. Kuram and A. K. Sahoo, Chem. Commun., 2013, 49, 5225 RSC
;
(k) B. Zhou, Y. Yang, J. Shi, H. Feng and Y. Li, Chem. – Eur. J., 2013, 19, 10511 CrossRef CAS PubMed
;
(l) V. S. Thirunavukkarasu, K. Raghuvanshi and L. Ackermann, Org. Lett., 2013, 15, 3286 CrossRef CAS
;
(m) B. Sun, T. Yoshino, S. Matsunaga and M. Kanai, Chem. Commun., 2015, 51, 4659 RSC
;
(n) W.-H. Li and L. Dong, Adv. Synth. Catal., 2018, 360, 1104 CrossRef CAS
.
-
(a) M. Maraswami, G. Chen and T.-P. Loh, Adv. Synth. Catal., 2018, 360, 416 CrossRef CAS
;
(b) S. Chen, B. Feng, X. Zheng, J. Yin, S. Yang and J. You, Org. Lett., 2017, 19, 2502 CrossRef CAS
;
(c) L. Xu, L. Wang, Y. Feng, Y. Li, L. Yang and X. Cui, Org. Lett., 2017, 19, 4343 CrossRef CAS PubMed
;
(d) D. Mu, X. Wang, G. Chen and G. He, J. Org. Chem., 2017, 82, 4497 CrossRef CAS PubMed
.
- C. Zhang, W. Zhang, C. Sheng, W. Zhang, C. Xing and Z. Miao, Chem. Rev., 2017, 117, 7762 CrossRef
.
-
(a) T. Yatabe, X. Jin, N. Mizuno and K. Yamaguchi, ACS Catal., 2018, 8, 4969 CrossRef
;
(b) K. Tsuchikama, Y. Kuwata, Y. Tahara, Y. Yoshinami and T. Shibata, Org. Lett., 2007, 9, 3097 CrossRef CAS PubMed
;
(c) K. Tanaka, Y. Otake, A. Wada, K. Noguchi and M. Hirano, Org. Lett., 2007, 9, 2203 CrossRef CAS PubMed
;
(d) M. Bakthadoss and P. V. Kumar, Adv. Synth. Catal., 2018, 360, 2650 CrossRef CAS
;
(e) J.-T. Yu, R. Chen, H. Jia and C. Pan, J. Org. Chem., 2018, 83, 12086 CrossRef CAS PubMed
;
(f) J. Kim and S. Chang, Angew. Chem., Int. Ed., 2014, 53, 2203 CrossRef CAS
.
Footnote |
† Electronic supplementary information (ESI) available. See DOI: 10.1039/c8cc09751h |
|
This journal is © The Royal Society of Chemistry 2019 |
Click here to see how this site uses Cookies. View our privacy policy here.