DOI:
10.1039/D4FO00204K
(Paper)
Food Funct., 2024,
15, 4051-4064
Nutritional profiling, fiber content and in vitro bioactivities of wheat-based biscuits formulated with novel ingredients†
Received
12th January 2024
, Accepted 24th March 2024
First published on 27th March 2024
Abstract
This study evaluated the nutritional profile and fiber content of innovative formulations of wheat-based biscuits enriched with chia seeds, carob flour and coconut sugar. The in vitro antioxidant, cytotoxic, anti-inflammatory and antimicrobial activities were also investigated to understand the potential health advantages of the incorporation of these new ingredients. The novel biscuits demonstrated significant improvements in protein and mineral content, with increases of 50% and 100% in chia biscuits, and up to 20% and 40% in carob biscuits, respectively. Fiber also notably increased, particularly in samples containing 10% carob flour, which increased four times as compared to wheat-based samples. The new ingredients exhibited antibacterial and antifungal activity, particularly against Yersinia enterocolitica (minimum inhibitory concentration 1.25 mg mL−1 in coconut sugar) and Aspergillus fumigatus (minimum inhibitory concentration/minimum fungicidal concentrations 2.5/5 mg mL−1 in chia seeds). However, the final biscuits only displayed antifungal properties. Carob flour and chia seeds had a remarkably high capacity to inhibit the formation of TBARS and promoted greater antioxidant activity in biscuit formulations, with EC50 values decreasing from 23.25 mg mL−1 (control) to 4.54 mg mL−1 (15% defatted ground chia seeds) and 1.19 mg mL−1 (10% carob flour). Only chia seeds exhibited cellular antioxidant, anti-inflammatory and cytotoxic activity, attributes that were lost when seeds were added into the biscuits. These findings highlight the potential health benefits of these ingredients, particularly when incorporated in new wheat-based formulations.
1. Introduction
The use of alternative ingredients in traditional food formulations is a common practice that results in novel and appealing organoleptic experiences while maintaining or improving a healthy and nutritious balanced food. Increased consumer awareness of healthy nutrition imposes the need of a new market of innovative products that provide nutritional benefits and promote good health. In addition, the incorporation of health-promoting components into bakery products with a focus on circular economy and sustainable food production systems is also being explored.1 The bakery industry has responded to this demand by developing a wide range of products that incorporate alternative ingredients. These products often include the addition or substitution of wheat, either partially or completely, with seeds (chia, sesame, quinoa), legumes (lentils, chickpeas), vegetables (apple, tomato), spices (cinnamon, cloves) different types of cereals (spelt, rye) and pseudocereals (buckwheat). This has resulted in a variety of baked goods, many of them suitable for consumers with gluten intolerance and celiac disease, while also providing added nutritional benefits.2,3 The trend of incorporating alternative ingredients in bakery products is particularly noticeable in the biscuit industry. Traditional wheat-flour biscuits made with sugars and fats are being replaced with innovative recipes aimed at providing consumers with low-sugar, gluten-free, high-fiber, and mineral-enriched alternatives, among other health benefits.4
Chia seed (Salvia hispanica L.) is characterized by its high content of dietary fiber (18–40%) and polyunsaturated fatty acids, especially from the ω-3 group, with α-linolenic acid being the most representative (60% of the total fatty acids). Additionally, it is a source of proteins (15–24%), carbohydrates (26–41%), and minerals (4–6%).5,6 Compared to traditional crops such as wheat, corn, rice, and oats, chia has a higher content of protein, fiber, and lipids. Chia also boasts bioactive compounds with strong antioxidant activity, including gallic, caffeic, chlorogenic, cinnamic, or ferulic acid.6 It also shows a high water absorption capacity, resulting in a clear gel known as chia mucilage. These properties make chia a functional food with remarkable nutritional characteristics, ideal for use in the food industry as a foam-stabilizing agent, suspending agent, emulsifier, adhesive, or binder with water-holding capacity.5 As such, incorporating chia into certain food formulations is desirable from both a technological and nutritional perspective.7–9
Carob flour is obtained from the carob pod of the leguminous tree Ceratonia siliqua L., by dehulling and deseeding the pulp, which is then oven dried and mechanically milled.10 Despite carob pulp being mainly used for animal feed and the production of sugar syrup,11 it has recently been recognized as an important source of nutrients and essential elements for human metabolism, healthy growth and disease prevention, such as dietary fiber, polyphenols and minerals, while having low amounts of fat.12 Moreover, it is gluten-free, which makes carob flour an excellent ingredient for developing new food products with enhanced nutritional value and a suitable alternative for people with celiac disease or gluten intolerance.13–15 For this reason, carob flour is increasingly being used in bakeries,12 particularly in Mediterranean countries that are facing issues related to climate change.
Reducing the sugar content is another issue for new food formulations. In 2015, the World Health Organization (WHO) recommended that the consumption of added sugars should be reduced to less than 10% of total caloric intake.16 Cereals and their by-products are the main sources of added sugar, accounting for 19.1% of the total dietary sugar intake.17 Therefore, it is crucial to consider alternatives to sucrose, such as carob flour and other sweeteners (i.e. coconut sugar), which can be added to cereal-based foods and can provide healthier alternatives to combat obesity and other diet-related health issues.18,19
In recent years, several investigations have been focused on cereal-based foods formulated with chia and carob flour and there is no information for coconut sugar. The majority of these studies have assessed the psychochemical attributes and consumer acceptability of the final products, including textural and sensorial analysis.8,9,14,15,20,21 Nevertheless, the researches of the nutritional changes in novel formulations containing carob, chia and coconut sugar are limited or even nonexistent. In this context, the aim of this study was to evaluate the nutritional profile and the fiber content of new formulations of wheat-based biscuits enriched with novel ingredients such as chia seeds, carob flour, and coconut sugar. The goal was designing a cereal-based product that offers added nutritional and physiological values respect to the traditional wheat-based biscuit. Different biological activities in vitro, including antioxidant, cytotoxic and anti-inflammatory were also assessed to determine the health-enhancing effects of the reformulated food. Additionally, the antimicrobial activity of novel ingredients was further investigated to set more insight into their potential use as food preservatives and additives aimed at enhancing the safety and shelf life of biscuits. The findings from this research will provide valuable knowledge regarding the health properties of chia seeds, carob flour and coconut sugar, highlighting their potential applications in the food industry.
2. Materials and methods
2.1. Standards and reagents
Ampicillin, methicillin and streptomycin were acquired from Fischer Scientific (Janseen Pharmaceutical, Belgium) and ketoconazole was provided by Frilabo (Porto, Portugal). Ellipticine, dexamethasone, quercetin, fatty acid methyl ester (FAME) reference standard mixture 37 (standard 47885-U), trolox (6-hydroxy-2,5,7,8-tetramethylchroman-2-carboxylic acid) and thiobarbituric acid were obtained from Sigma-Aldrich (St. Louis, MO, USA). Porcine (Sus scrofa) brains were obtained from animals slaughtered at officially licensed premises. Analytical grade reagents were used for all experiments. Sulfuric acid (98%), n-hexane (95%), petroleum ether, methanol, ethanol and toluene were purchased from Fischer Scientific (Leicestershire, UK). Roswell Park Memorial Institute medium (RPMI 1640), Dulbecco's modified Eagle's medium, fetal bovine serum, Hank's balanced salt solution (HBSS) and trypsin–EDTA (ethylenediaminetetraacetic acid) were provided by Hyclone (Logan, Utah, USA). Malt extract broth and Muller-Hinton broth were from Biolab® (Budapest, Hungary) while blood agar (Sheep blood 7%) was from LiofilChemsrl (Roseto d. Abruzzi (TE), Italy). 2′,7′-Dihydrodichlorofluorescein diacetate, 2,2′-azobis(2-amidinopropane) dihydrochloride (AAPH), tris(hydroxymethyl) aminomethane, trichloroacetic acid, dimethyl sulfoxide (DMSO), lipopolysaccharide, and sodium nitrate were also from Sigma-Aldrich (St. Louis, MO, USA). p-Iodonitrotetrazolium chloride and sodium sulfate were provided by Panreac Applichem (Barcelona, Spain). Water was treated through a Milli-Q water purification system (TGI Pure Water Systems, Greenville, SC, USA).
2.2. Ingredients
Black chia seeds (Salvia hispanica L.) originating from Mexico and certified for organic cultivation were purchased from a local market (Madrid, Spain) (Lot C-O-AD18-3-V1, importer EcoAndes Import Export, S.L.). These seeds were subjected to different physical–chemical treatments and the following samples were obtained: (1) ground chia seed (GC): whole seeds were ground using an Ultra-turrax (IKA, T10 basic, Boutersem, Belgique) to obtain a homogeneous mixture and passed through a mesh size sieve of 0.80 mm; (2) defatted ground chia seed (DGC): ground chia seeds were washed twice with hexane (1
:
10, w/v) and left to dry for 20 hours at room temperature. Carob flour, coconut sugar, wheat flour and other food-grade ingredients (white sugar, salt and sunflower oil) were purchased from local supermarkets (Madrid, Spain).
2.3. Preparation of biscuits
Fourteen biscuits were prepared according to the recipe described in the AACC (American Association of Cereal Chemists) method 10–5422 (ESI Table 1†). Control biscuit was formulated with wheat flour (130 g), white sugar (35 g), distilled water (30 g), sunflower oil (26 g), sodium bicarbonate (0.8 g), ammonium bicarbonate (0.4 g) and salt (1 g).
- Ground chia biscuits (GC): wheat flour was replaced by ground chia seed, with percentages in the final weight of 5% (GC5), 10% (GC10) and 15% (GC15).
- Defatted ground chia biscuits (DGC): wheat flour was replaced by defatted ground chia seed, with percentages in the final weight of 5% (DGC5), 10% (DGC10) and 15% (DGC15).
- Carob flour biscuits (CF): wheat flour was replaced by carob flour, with percentages in the final weight of 1% (CF1), 5% (CF5) and 10% (CF10).
- Coconut sugar biscuit (CS): 100% of white sugar was replaced by coconut sugar (CS100).
- Carob flour and coconut sugar biscuits: wheat flour was replaced by carob flour, with percentages in the final weight of 1% (CF1), 5% (CF5), and 10% (CF10), and 100% of white sugar was replaced by coconut sugar (CS100).
The total amount of solids in the dough remained the same. Ingredients were thoroughly mixed and the dough was rolled out to disks with the diameter of 6.5 cm and the thickness of 2 mm, and baked at 180 °C for 22 minutes in a convention oven (Memmert UFE 400, Germany).
2.4. Proximal nutritional composition
The proximal composition was determined in ingredients and biscuits separately. For this purpose, the official analysis methodologies AOAC (Association of Official Analytical Chemists) were used.23 Moisture was determined gravimetrically by desiccation in an oven at 105 °C to constant weight, according to AOAC 984.25. The crude protein (N × 6.25) was evaluated using the macro-Kjeldahl method (AOAC 991.02). Fat content was determined by Soxhlet extraction with petroleum ether according to AOAC 989.05. The total dietary fiber (TDF) content was determined by the AOAC 985.29 method, using the Kit TDF100A (Sigma-Aldrich Chemie GmbH, Buchs, Switzerland). Total mineral content was determined as ash by incineration at 550 °C (AOAC 935.42). Results are expressed as g/100 g of fresh sample. Total carbohydrates (including fiber) were calculated by difference. Energy was estimated using the following conversion factors: 4 kcal g−1 for proteins and carbohydrates (excluding fiber) and 9 kcal g−1 for fat and results were expressed in kcal/100 g.24
2.5. Analysis of soluble sugars
Soluble sugars were analyzed according to Pinela et al.25 Samples were spiked with the internal standard melezitose (25 mg mL−1) and extracted for 90 min with ethanol/water 80
:
20 (v/v) at 80 °C. The analysis was performed in a high-performance liquid chromatography (HPLC) system coupled to a refractive index (RI) detection. Chromatographic separation was achieved with a Eurospher 100-5 NH2 column (4.6 × 250 mm, 5 μm, Knauer). The mobile phase was acetonitrile/water 70
:
30 (v/v). Soluble sugars were identified by comparison with pure standards and quantified based on the internal standard concentration. The results were expressed in g/100 g fresh sample.
2.6. Analysis of fatty acids profile
Fatty acid methyl esters (FAMEs) were prepared from oils derived from the determination of fat content in both ingredients and biscuits. The transesterification reaction was carried out by mixing the oils (500 μL) with a catalytic solution of methanol, sulfuric acid, and toluene (2
:
1
:
1, v/v/v) (5 mL). The mixture was incubated overnight at 50 °C and 600 rpm. After that, phase separation was achieved by adding 3 mL of water and 3 mL of diethyl ether to the mixture and vortexing for 30 seconds. The FAMEs were recovered from the upper layer and mixed with sodium sulfate. The samples were then filtered using 0.22 μm nylon filters and diluted 1/10 in diethyl ether and stored at −20 °C until further analysis.
FAMEs analysis was conducted using a gas chromatograph (GC) equipped with a flame ionization detector (FID), a split/splitless injector, and a capillary column (30 m × 0.32 mm ID × 0.25 μm, Macherey-Nagel). The identification of fatty acids was performed by comparing the relative retention times of FAME peaks obtained from the oil extracted from samples with a reference standard FAME mixture. The specific details of the chromatography separation and determinations were previously described by Reis et al.26 The results were expressed in relative percentage (%).
2.7. Evaluation of in vitro biological activities
For the extract preparation, 1 g of both ingredients and biscuits was used. Firstly, samples were macerated at room temperature with ethanol/water solution (80
:
20, v/v; 30 ml). The hydroethanolic extract was filtered using a filter (Whatman No. 4) and the retentate was extracted again using the same procedure. The ethanolic fraction of the extracts was evaporated under reduced pressure in a rotary evaporator (Büchi R-210, Flawil, Switzerland) and the aqueous fraction was frozen and lyophilized (Freezone 4.5, Labconco, Kansas City, MO, USA). The lyophilized extracts were re-dissolved: (i) in culture medium at 20 mg mL−1 for the evaluation of antimicrobial activity; (ii) in distilled water at 8 mg mL−1 for the evaluation of cytotoxic and anti-inflammatory activity and at 2 mg mL−1 for cellular antioxidant activity evaluation; and (iii) in a hydroethanolic solution (ethanol/water; 80
:
20, v/v) at 200 mg mL−1 for TBARS inhibition assay. Stock solutions of lyophilized extracts were diluted successively to obtain the appropriate range of working concentrations.
2.7.1. Antimicrobial activity.
Methods described by Pires et al.27 and Heleno et al.28 were employed for antimicrobial assays. Eight bacterial strains were tested for antibacterial activity. Enterobacter cloacae (ATCC 49741), Escherichia coli (ATCC 25922), Pseudomonas aeruginosa (ATCC 9027), Salmonella enterica serotype Enteritidis (ATCC 13076), Yersinia enterocolitica (ATCC 8610), Bacillus cereus (ATCC 11778), Listeria monocytogenes (ATCC 19111) and Staphylococcus aureus (ATCC 11632). Two fungal strains were tested for antifungal activity: Aspergillus fumigatus (ATCC 204305) and Aspergillus brasiliensis (ATCC 16404). Commercial strains of these microorganisms were acquired from Frilabo (Porto, Portugal). Streptomycin and ampicillin were used as a positive control for antibacterial assays, except for S. aureus, for which methicillin was employed. Ketoconazole was selected as positive control for the antifungal assays.
Gram-positive bacteria (B. cereus, L. monocytogenes, S. aureus) were incubated in fresh blood agar containing 7% sheep blood, while Gram-negative bacteria (E. cloacae, E. coli, P. aeruginosa, S. enterica, Y. enterocolitica) were incubated in Muller Hilton agar. Both types of bacteria were incubated at 37 °C for 24 hours to maintain the exponential growth phase. Bacterial suspensions were prepared at a concentration of 1.5 × 106 CFU mL−1. Malt agar plates were used for the micromycetes (fungal strains) and they were incubated at 25 °C for 72 hours. After that, fungal spores were recovered from the agar surface using sterile 0.85% saline solution containing 0.1% Tween 80 (v/v). Fungal spore suspensions were adjusted to a concentration of 1.0 × 105 UFC mL−1.
Results were presented as minimum inhibitory concentration (MIC), minimum bactericidal concentration (MBC) and minimum fungicidal concentrations (MFC) and expressed in mg mL−1.
2.7.2. Antioxidant activity.
For the antioxidant activity evaluation, two different in vitro assays were used: the cell-based assays of thiobarbituric acid reactive substances (TBARS) formation inhibition and the cellular antioxidant activity (CAA). The capacity of the extracts to inhibit the formation of TBARS was assessed according to Lockowandt et al.29 using porcine brain tissue as oxidizable biological substrates. Trolox was used as a positive control. The results were expressed as EC50 values (mg mL−1), which translate the extract concentration responsible for 50% of antioxidant capacity.
For the cellular antioxidant activity assessment, the method described by de la Fuente et al.30 was followed. Extracts at various concentrations (ranging from 500 to 2000 μg mL−1) were prepared in H2O. The extracts were incubated with a murine macrophage cell line (RAW 264.7); obtained from the European Collection of Authenticated Cell Cultures (ECACC) at 37 °C for 1 hour. After incubation, the medium was discarded, and the cells were washed twice with 100 μL of HBSS. Then, 100 μL of AAPH (600 μM) was added to the cells. The reaction was carried out using a plate reader Biotek FLX800 (Bio-Tek Instruments, Inc., Winooski, VT, USA) with fluorescence filters set at an excitation wavelength of 485 nm and an emission wavelength of 535 nm. Fluorescence values were recorded every 5 minutes over a period of 1 hour, and the differences in the areas under the curve (AUC) were calculated. Quercetin standard was used as a positive control. The CAA values were determined using the equation
, where
represents the integrated area under the sample fluorescence versus time curve, and
represents the integrated area from the control curve. The results were expressed as a percentage of inhibition of the oxidation reaction.
2.7.3. Anti-inflammatory activity.
Both ingredient and biscuit extracts at concentrations of 6.25–400 μg mL−1 (in water) were tested for the determination of anti-inflammatory activity. Briefly, the stimulation of murine macrophages RAW 264.7 cell line was measured by means of nitric oxide (NO) production by using the Griess Reagent System kit (Promega, WI, USA), as previously described by Souilem et al.31 Dexamethasone was used as positive control and the results were expressed as IC50 values, in μg mL−1.
2.7.4. Cytotoxic activity.
Both ingredient and biscuit extracts at concentrations of 6.25–400 μg mL−1 (in water) were used for the determination of cytotoxic activity against different human tumor cell lines: gastric adenocarcinoma, AGS; colon adenocarcinoma, CaCo-2; breast adenocarcinoma, MCF-7; and non-small cell lung cancer, NCI-H460. AGS and Caco-2 cell lines were obtained from ECACC, whereas the MCF-7 and NCI-H460 cell lines were purchased from the Leibniz-Institute DSMZ (Deutsche Sammlung von Mikroorganismen und Zellkulturen GmbH, Braunschweig, Germany). Elipticine was selected as positive control, and the results were expressed as GI50 values, in μg mL−1. Cells were treated according to the protocol described by Mandim et al.32 The sulforhodamine B (SRB) colorimetric assay (Sigma-Aldrich, St. Louis, MO, USA) described by de la Fuente et al.30 was applied.
2.8. Statistical analysis
All determinations were carried out in triplicate, and results were expressed as the mean ± standard deviation (SD). Statistical analysis was performed by using Statgraphics Centurion XV (Herndon, VA, USA). Firstly, to meet the requirements for the performance of analysis of variance (ANOVA) the normality of residuals and homoscedasticity were assessed by conducting the Saphiro-Wilk's and Levene's tests, respectively. Then, one-way ANOVA was carried out to evaluate statistical differences between treatments, followed by Tukey's HSD post hoc test. Statistical differences with respect to positive controls were evaluated by student's t-test. Differences were considered to be significant at p < 0.05.
3. Results and discussion
3.1. Proximal composition of ingredients and biscuit formulations
The proximal composition of the ingredients is presented in Table 1, including (1) wheat flour and white sugar as control (traditional ingredients), (2) chia seeds and carob flour as wheat flour partial replacers and (3) coconut sugar as white sugar replacer. In comparison to wheat flour, ground chia seeds presented significantly higher levels of proteins, fats, dietary fiber, minerals and soluble sugars, while exhibiting lower total carbohydrate content. The primary fatty acid detected in wheat flour was linoleic acid (C18:2n6), accounting for 52.3% of the lipid profile. In contrast, ground chia seeds were mainly composed of linolenic acid (C18:3n3), with a significantly lower contribution from linoleic acid (C18:2n6). These values align with findings from previous studies.20 Upon the defatting process, the fat content notably decreased from 32.03% in ground seeds to 8.21% in the defatted samples, which resulted in an increase in the levels of most other components. In agreement with Nassef et al.,20 significant differences (p < 0.05) were observed in the content of all chemical compositions among ground chia seeds, defatted ground chia seeds, and wheat flour. In the case of carob flour, the most prominent feature was its high fiber and soluble sugar content. Additionally, it showed higher levels of minerals but lower fat content compared to wheat flour, as previously reported by other authors.13 Carob flour also had a lower protein content compared to wheat; however, it has been described that it contains higher levels of certain essential amino acids such as lysine, histidine, valine and threonine than wheat.13 This characteristic makes carob a high-quality protein ingredient with important nutritional value. The predominant fatty acid in carob was oleic acid (C18:1n9), again with a significantly lower contribution of the linoleic acid as compared with wheat flour, in accordance with observations from Youssef et al.33 Regarding sweeteners, unlike white sugar, which primarily consists of soluble sugars, coconut sugar presented protein, fiber and minerals. It had a minimal fat content, with the predominant fatty acid being palmitic acid (C16:0).
Table 1 Proximate composition and energy value of novel ingredients (chia seeds, carob flour and coconut sugar) as compared with traditional ones (wheat flour and white sugar)
|
Control |
Wheat flour replacers |
Control |
Sugar replacer |
|
Wheat flour |
Ground chia seed |
Defatted ground chia seed |
Carob flour |
White sugar |
Coconut sugar |
Results are mean ± standard deviation (n = 3). nd: not detected. Different letters in the same line indicate significant differences between samples (p < 0.05). |
Proximate composition (g/100 g fresh sample) |
Protein |
9.47 ± 0.31c |
21.69 ± 0.25e |
18.06 ± 0.14d |
5.28 ± 0.07b |
nd |
0.92 ± 0.44a |
Fat |
1.16 ± 0.01b |
32.03 ± 0.42d |
8.21 ± 0.06c |
0.42 ± 0.01ab |
nd |
0.20 ± 0.06a |
Total saturated fat |
0.30 ± 0.01b |
8.36 ± 0.08d |
2.49 ± 0.06c |
0.13 ± 0.01a |
nd |
0.11 ± 0.01a |
C6:0 (%) |
nd |
1.10 ± 1.01b |
0.40 ± 0.01a |
nd |
nd |
nd |
C8:0 (%) |
nd |
0.50 ± 0.20a |
0.70 ± 0.10a |
nd |
nd |
nd |
C14:0 (%) |
0.20 ± 0.10a |
nd |
nd |
0.40 ± 0.01b |
nd |
nd |
C16:0 (%) |
23.10 ± 0.50a |
17.60 ± 2.40a |
21.30 ± 1.20a |
25.30 ± 3.80a |
nd |
46.70 ± 0.01b |
C18:0 (%) |
1.60 ± 0.01a |
6.90 ± 0.40bc |
8.01 ± 0.40c |
5.40 ± 0.80b |
nd |
7.80 ± 0.10c |
Total unsaturated fat |
0.83 ± 0.03c |
22.93 ± 1.15e |
5.39 ± 0.07d |
0.26 ± 0.02b |
nd |
0.09 ± 0.01a |
C18:1n9 (%) |
16.01 ± 0.80b |
11.70 ± 0.30a |
13.30 ± 0.60a |
42.80 ± 0.40d |
nd |
34.01 ± 0.40c |
C18:2n6 (%) |
52.30 ± 0.10c |
18.60 ± 0.30b |
18.20 ± 0.50b |
16.20 ± 4.30ab |
nd |
8.90 ± 0.60a |
C18:3n3 (%) |
3.40 ± 0.10a |
41.30 ± 3.80a |
34.20 ± 2.40b |
2.70 ± 1.30a |
nd |
nd |
Total carbohydrates |
79.41 ± 0.38c |
36.19 ± 0.04a |
61.33 ± 0.25b |
87.70 ± 0.03d |
99.97 ± 0.00f |
94.12 ± 0.36e |
Fiber |
3.41 ± 0.42b |
25.60 ± 0.31c |
31.23 ± 0.42d |
35.80 ± 0.51e |
nd |
1.30 ± 0.06a |
Soluble sugars |
0.50 ± 0.06a |
0.93 ± 0.04a |
0.79 ± 0.06a |
30.86 ± 0.26b |
104.41 ± 0.69d |
95.46 ± 1.48c |
Fructose |
nd |
0.14 ± 0.02a |
0.11 ± 0.03a |
9.04 ± 0.13c |
nd |
2.42 ± 0.06b |
Glucose |
nd |
nd |
nd |
2.80 ± 0.05b |
nd |
4.12 ± 0.14c |
Sucrose |
0.50 ± 0.06a |
0.79 ± 0.01a |
0.68 ± 0.03a |
19.02 ± 0.09b |
104.41 ± 0.69d |
88.92 ± 1.29c |
Moisture |
9.44 ± 0.00f |
5.54 ± 0.00d |
7.31 ± 0.00e |
4.41 ± 0.00c |
0.03 ± 0.00a |
3.62 ± 0.00b |
Total mineral content |
0.52 ± 0.06a |
4.54 ± 0.12d |
4.99 ± 0.05e |
2.19 ± 0.03c |
nd |
1.14 ± 0.05b |
Energy value (kcal/100 g) |
352.32 ± 0.16b |
417.44 ± 2.58c |
266.53 ± 0.06a |
232.48 ± 1.61a |
399.89 ± 0.01b |
376.76 ± 0.06b |
Novel formulations of biscuits were prepared with partial or total replacement of traditional ingredients (wheat flour and white sugar) by innovative ingredients (chia seeds, carob flour and coconut sugar) (ESI Table 1†). Chia biscuits were formulated with both defatted and non-defatted ground chia seeds in a range between 5 and 15% of the final weight. Despite chia seeds are allowed as an ingredient in baked goods at concentrations below 10%,34 experiments were conducted with percentages as high as 15% to gain a deeper understanding of the nutritional composition and in vitro bioactivities of the biscuits formulated with these seeds. Similarly, carob flour was added to the biscuits in a range between 1 and 10% of the final weight. As expected, the addition of chia led to an increase in protein, fat, fiber, and mineral content, while concurrently reducing total carbohydrate content without modifying the energy value of the final product (Table 2). This change resulted in an improved nutritional profile when compared to the control sample. The rise in fiber and mineral content was particularly pronounced when defatted seeds were incorporated, resulting in a slight uptick in fat content when they were added at percentages of 10 and 15%. In the case of chia biscuits, the increase in fat content resulted in a positive outcome since chia is abundant in polyunsaturated fatty acids, primarily omega-3 fatty acids (C18:3n3, linolenic acid). These fatty acids are considered essential because the human body cannot synthesize them and they must be obtained through dietary sources.7 At this point, it is important to mention that an increase in unsaturated fatty acids could promote lipid oxidation during storage. Therefore, although the chemical modifications of biscuit during storage are not within the scope of the present study, it is relevant to carefully assess the potential impact of lipid oxidations throughout the shelf life in a risk/benefit context. Previous studies conducted by our research group reported an acceleration of lipid oxidation in chia-enriched biscuits, particularly due to the increase in polymerization compounds, which suggests a decreased shelf life of the product by promoting rapid rancidity.35 Consistent with the results of this study, several researchers have also documented the increase of proteins, fiber, and fats when chia is included in formulations of bakery products.8,9
Table 2 Proximate composition and energy value of novel biscuits formulated with ground chia seeds (GC), defatted ground chia seeds (DGC), carob flour (CF) and coconut sugar (CS) as compared with traditional biscuits formulated with wheat flour (control)
|
Control |
GC5 |
GC10 |
GC15 |
DGC5 |
DGC10 |
DGC15 |
Proximate composition (g/100 g fresh sample) |
Protein |
5.98 ± 0.32a |
7.50 ± 0.10cde |
8.30 ± 0.04ef |
9.16 ± 0.03f |
7.32 ± 0.08bcde |
7.98 ± 0.20de |
7.94 ± 0.24de |
Fat |
14.55 ± 0.02ab |
16.51 ± 0.08de |
18.15 ± 0.23f |
20.51 ± 0.39g |
14.75 ± 0.24ab |
15.67 ± 0.05cd |
16.61 ± 0.01e |
Total saturated fat |
1.82 ± 0.07a |
2.15 ± 0.06b |
3.86 ± 0.18c |
5.84 ± 0.05e |
1.93 ± 0.03a |
2.04 ± 0.04ab |
4.72 ± 0.05d |
C6:0 (%) |
nd |
nd |
0.50 ± 0.10a |
1.90 ± 0.80b |
nd |
nd |
4.10 ± 1.70c |
C8:0 (%) |
nd |
nd |
0.20 ± 0.10a |
0.70 ± 0.20b |
nd |
nd |
0.80 ± 0.10b |
C14:0 (%) |
0.10 ± 0.01a |
0.10 ± 0.01a |
0.10 ± 0.01 |
0.20 ± 0.01b |
0.10 ± 0.01a |
0.10 ± 0.01a |
0.20 ± 0.01b |
C16:0 (%) |
8.50 ± 0.20a |
8.70 ± 0.20a |
14.01 ± 0.30b |
17.60 ± 1.30c |
8.80 ± 0.40a |
8.60 ± 0.01a |
14.30 ± 0.80c |
C18:0 (%) |
4.10 ± 0.10a |
4.20 ± 0.01a |
6.50 ± 0.10c |
8.10 ± 0.40d |
4.20 ± 0.01a |
4.30 ± 0.20a |
9.01 ± 0.40d |
Total unsaturated fat |
12.41 ± 0.21bc |
14.03 ± 0.79d |
13.57 ± 0.21c |
11.83 ± 0.20b |
12.51 ± 0.23bc |
13.26 ± 0.68c |
10.45 ± 0.23a |
C18:1n9 (%) |
29.80 ± 0.10bc |
27.30 ± 0.01a |
37.01 ± 0.40d |
37.10 ± 0.50d |
28.60 ± 0.30abc |
28.60 ± 0.31abc |
24.40 ± 0.90e |
C18:2n6 (%) |
55.30 ± 0.20d |
52.90 ± 0.01d |
33.20 ± 1.20c |
24.10 ± 3.30b |
53.10 ± 0.01d |
51.01 ± 1.01d |
34.10 ± 2.20a |
C18:3n3 (%) |
0.20 ± 0.01a |
4.80 ± 0.10c |
4.60 ± 0.20c |
4.00 ± 0.70bc |
3.10 ± 0.01b |
5.01 ± 0.31c |
4.40 ± 0.10a |
Total carbohydrates |
76.25 ± 0.39g |
73.00 ± 0.37cde |
70.44 ± 0.39b |
67.26 ± 0.47a |
74.18 ± 0.02def |
72.94 ± 0.12cd |
71.99 ± 0.17bc |
Fiber |
2.63 ± 0.06a |
5.80 ± 0.31c |
8.13 ± 0.53f |
10.83 ± 0.66h |
7.47 ± 0.64e |
9.57 ± 0.95gh |
13.73 ± 1.35j |
Soluble sugars |
19.21 ± 0.01abcd |
18.90 ± 0.10abcd |
19.45 ± 0.15bcd |
18.61 ± 0.02abcd |
18.15 ± 0.78abc |
18.69 ± 0.89abcd |
19.03 ± 0.81abcd |
Fructose |
nd |
nd |
nd |
nd |
nd |
nd |
0.14 ± 0.01a |
Glucose |
nd |
nd |
nd |
nd |
nd |
nd |
0.20 ± 0.01a |
Sucrose |
19.21 + 0.01c |
18.90 ± 0.10abc |
19.45 ± 0.15c |
18.61 ± 0.02abc |
18.15 ± 0.78abc |
18.69 ± 0.89abc |
18.69 ± 0.80abc |
Moisture |
2.10 ± 0.00b |
1.29 ± 0.01a |
1.36 ± 0.00a |
1.05 ± 0.01a |
2.34 ± 0.01b |
1.68 ± 0.01ab |
1.24 ± 0.00a |
Total mineral content |
1.11 ± 0.05ab |
1.69 ± 0.19bcd |
1.75 ± 0.21bcd |
2.01 ± 0.05cd |
1.40 ± 0.14abc |
1.73 ± 0.27bcd |
2.22 ± 0.06d |
Energy value (kcal/100 g) |
449.40 ± 0.14c |
447.39 ± 0.37c |
445.78 ± 0.34c |
447.00 ± 1.71c |
428.92 ± 1.73b |
426.47 ± 0.84b |
414.33 ± 0.17a |
|
Control |
CF1 |
CF5 |
CF10 |
CS100 |
CF1-CS100 |
CF5-CS100 |
CF10-CS100 |
Results are mean ± standard deviation (n = 3). nd: not detected. Different letters in the same line indicate significant differences between samples (p < 0.05). |
Proximate composition (g/100 g fresh sample) |
Protein |
5.98 ± 0.32a |
6.53 ± 0.21abc |
6.65 ± 0.09abc |
6.26 ± 0.34ab |
7.10 ± 0.04abcd |
6.92 ± 0.07abcd |
6.58 ± 0.00abc |
7.06 ± 0.45abcd |
Fat |
14.55 ± 0.02ab |
14.64 ± 0.08ab |
14.80 ± 0.12ab |
14.26 ± 0.01a |
14.20 ± 0.08a |
14.56 ± 0.14ab |
15.09 ± 0.12bc |
14.99 ± 0.00abc |
Total saturated fat |
1.82 ± 0.07a |
1.93 ± 0.08a |
1.95 ± 0.05a |
1.89 ± 0.01a |
1.92 ± 0.04a |
2.02 ± 0.03ab |
2.28 ± 0.05b |
2.01 ± 0.06ab |
C6:0 (%) |
nd |
nd |
nd |
nd |
nd |
nd |
nd |
nd |
C8:0 (%) |
nd |
nd |
nd |
nd |
nd |
nd |
nd |
nd |
C14:0 (%) |
0.10 ± 0.01a |
0.1 ± 0.0a |
0.1 ± 0.0a |
0.1 ± 0.0a |
0.1 ± 0.0a |
0.1 ± 0.0a |
0.1 ± 0.0a |
0.1 ± 0.0a |
C16:0 (%) |
8.50 ± 0.20a |
8.6 ± 0.3a |
8.4 ± 0.1a |
8.4 ± 0.2a |
8.8 ± 0.5a |
8.9 ± 0.2a |
9.2 ± 0.1a |
8.7 ± 0.1a |
C18:0 (%) |
4.10 ± 0.10a |
4.5 ± 0.0a |
4.7 ± 0.1ab |
4.8 ± 0.1ab |
4.6 ± 0.2ab |
4.9 ± 0.3ab |
5.8 ± 0.3bc |
4.6 ± 0.3ab |
Total unsaturated fat |
12.41 ± 0.21bc |
12.32 ± 0.31bc |
12.47 ± 0.27bc |
12.02 ± 0.15b |
11.94 ± 0.07b |
12.14 ± 1.01b |
12.42 ± 0.21bc |
12.59 ± 0.24bc |
C18:1n9 (%) |
29.80 ± 0.10bc |
30.6 ± 0.2c |
30.5 ± 0.0c |
30.5 ± 0.0c |
30.5 ± 0.4c |
30.5 ± 0.3c |
28.3 ± 0.1ab |
27.7 ± 0.2a |
C18:2n6 (%) |
55.30 ± 0.20d |
53.4 ± 0.2d |
53.6 ± 0.4d |
53.6 ± 0.5d |
53.3 ± 0.6d |
52.6 ± 0.1d |
53.8 ± 0.5d |
56.0 ± 0.2d |
C18:3n3 (%) |
0.20 ± 0.01a |
0.2 ± 0.0a |
0.2 ± 0.0a |
0.2 ± 0.0a |
0.3 ± 0.1a |
0.3 ± 0.1a |
0.2 ± 0.0a |
0.3 ± 0.0a |
Total carbohydrates |
76.25 ± 0.39g |
76.19 ± 0.36g |
75.98 ± 0.31fg |
75.02 ± 0.49fg |
74.98 ± 0.16fg |
75.09 ± 0.13fg |
74.79 ± 0.12efg |
75.18 ± 0.48fg |
Fiber |
2.63 ± 0.06a |
2.70 ± 0.06a |
5.09 ± 0.02b |
9.11 ± 0.14g |
2.86 ± 0.10a |
3.93 ± 0.34b |
6.12 ± 0.01d |
11.34 ± 0.05i |
Soluble sugars |
19.21 ± 0.01abcd |
19.37 ± 0.45abcd |
20.08 ± 0.26cd |
20.90 ± 0.69d |
16.74 ± 0.01a |
17.30 ± 0.31ab |
18.07 ± 0.22abc |
18.11 ± 0.09abc |
Fructose |
nd |
nd |
0.20 ± 0.00b |
0.66 ± 0.02d |
0.37 ± 0.01c |
0.47 ± 0.02c |
0.73 ± 0.00d |
1.15 ± 0.01e |
Glucose |
nd |
nd |
0.18 ± 0.02a |
0.25 ± 0.01b |
0.58 ± 0.01c |
0.58 ± 0.05c |
0.75 ± 0.04d |
1.04 ± 0.02e |
Sucrose |
19.21 + 0.01c |
19.37 ± 0.45a |
19.70 ± 0.25c |
19.98 ± 0.71c |
15.79 ± 0.00a |
16.26 ± 0.24ab |
16.59 ± 0.25ab |
15.91 ± 0.06a |
Moisture |
2.10 ± 0.00b |
1.80 ± 0.00ab |
1.43 ± 0.00ab |
3.24 ± 0.02c |
2.67 ± 0.01bc |
2.18 ± 0.01b |
2.27 ± 0.02b |
1.19 ± 0.00a |
Total mineral content |
1.11 ± 0.05ab |
0.84 ± 0.05a |
1.15 ± 0.11ab |
1.20 ± 0.014ab |
1.04 ± 0.04ab |
1.24 ± 0.08ab |
1.27 ± 0.00ab |
1.58 ± 0.03bcd |
Energy value (kcal/100 g) |
449.40 ± 0.14c |
449.50 ± 0.42c |
441.87 ± 0.70c |
416.06 ± 0.09a |
442.42 ± 1.31c |
441.45 ± 0.07c |
437.25 ± 0.70bc |
419.49 ± 0.01ab |
The addition of carob flour fundamentally resulted in a significant increment of the fiber content in biscuits, particularly at substitutions level of 5% and 10% (Table 2). Babiker et al.14 described that a complete replacement of wheat flour with carob flour in cookies led to a significant rise in protein content (from 8.94% to 13.49%) and a decrease in fat content (from 14.20% to 12.30%). However, a 50% substitution with carob flour only exerted residual changes in the composition. In the present study, the protein content reached 9.24%, and the fat content was 14.00%, which closely resembled the levels in the control sample. Despite the proximate composition of the biscuits with carob was very similar to the control biscuit, the notable increase in fiber, even with just 1% incorporation of carob, suggests an improvement for health in this new formulation. This is particularly significant given the well-documented health benefits associated with increased fiber intake. Research, such as the recent review by Ioniţă-Mîndrican et al.,36 has linked higher fiber consumption to various positive health outcomes, including reduced risk of diabetes, obesity, constipation, as well as a lowered risk of coronary heart diseases and stroke. The nutritional composition of the biscuits made with carob flour and coconut sugar was remarkably similar to those made with common sugar. However, there was a slight increase in protein, mineral and fiber content in the former, suggesting a mild additional improvement in the nutritional profile compared to the control sample. Given the low-fat content of the flours used, there was not a significant change in the lipid profile that warrants further comment (Table 2).
Fig. 1 depicts the proximal composition and fiber content of both the control biscuit and the new formulations containing chia, carob, and coconut sugar. The formulations with 10% chia and carob were specifically chosen for comparison under similar conditions, adhering to the maximum allowable concentration of chia in baked products.34 As mentioned before, the novel biscuits exhibited notable improvements in protein and mineral content (up to 50%), and substantially there was a rise in fiber when compared to traditional wheat-based biscuits, particularly in samples containing carob flour (up to four times). These findings underscore the potential health advantages associated with the incorporation of these new ingredients into the reformulated biscuits.
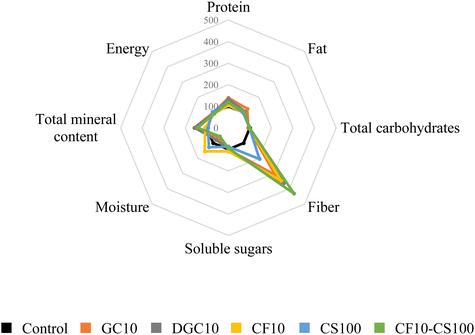 |
| Fig. 1 Proximal composition and fiber content of the control biscuit and the new formulations containing ground chia seeds (GC), defatted ground chia seeds (DGC) and carob flour (CF) at 10% and coconut sugar (CS) at 100%. | |
3.2. Bioactivities of ingredients and biscuit formulations
3.2.1. Antimicrobial activity.
Tables 3 and 4 display the results obtained for the antimicrobial activity of the extracts derived from ingredients and biscuit formulations. Results of antibacterial activity are expressed as MIC and MBC, while those of antifungal activity are expressed as MIC and MFC. Regarding ingredients (Table 3), wheat exhibited the lowest antibacterial effect, only inhibiting the growth of E. Cloacae and P. aeruginosa at concentrations of 20 mg mL−1. Chia seeds, carob flour, white sugar and coconut sugar presented antibacterial activity against almost all bacteria tested, with coconut sugar being particularly noteworthy for its inhibition of the growth of Y. enterocolitica by at 1.25 mg mL−1, followed by carob flour at 2.5 mg mL−1 and chia seeds at 5 mg mL−1. Similar results were observed in antifungal activity for all the ingredients against A. brasiliensis. However, chia seeds and white sugar exhibited the highest fungicidal effect on A. fumigatus. This finding suggests a positive impact, especially considering the abundance of this toxigenic microorganism in cereal crops, particularly in maize, wheat and barley.37 The antimicrobial activity may be linked to the nutritional composition of both ingredients and biscuits, where phenolic compounds could have had a relevant role. These compounds have the capability to interact with lipids and proteins in the cell membrane, making it not to be semipermeable and causing the leakage of ions and other cellular components. Consequently, this disrupts the metabolism within bacterial cells, ultimately leading to cell death.38 In this context, several studies have demonstrated that compounds like gallic and protocatechuic acids possess inhibitory effect of Y. enterocolitica growth, suggesting their potential application as food preservatives to extend the shelf life of foods.39,40 In a similar way, ferulic acid has been reported to have antifungal action.41
Table 3 Antibacterial (MIC/MBC, mg mL−1) and antifungal activity (MIC/MFC, mg mL−1) of extracts obtained from novel ingredients (chia seeds, carob flour and coconut sugar) as compared with traditional ones (wheat flour and white sugar)
|
Wheat |
GC |
DGC |
CF |
WS |
CS |
Positive controls |
|
nt: not tested. Results are mean ± standard deviation (n = 3). MIC: minimum inhibitory concentration; MBC: minimal bactericidal concentration. MBC: minimal fungicidal concentration. WF: Wheat flour. GC: Ground chia seed. DGC: defatted ground chia seed. CF: carob flour. WS: White sugar. CS: Coconut sugar. |
Antibacterial activity |
|
|
|
|
Streptomycin |
Ampicillin |
|
Gram-negative bacteria |
E. cloacae |
20/>20 |
20/>20 |
20/>20 |
20/>20 |
>20/>20 |
>20/>20 |
0.007/0.007 |
0.15/0.15 |
E. coli |
>20/>20 |
20/>20 |
>20/>20 |
20 />20 |
20/>20 |
20/>20 |
0.01/0.01 |
0.15/0.15 |
P. aeruginosa |
20/>20 |
>20/>20 |
>20>20 |
20/>20 |
20/>20 |
20/>20 |
0.06/0.06 |
0.63/0.63 |
S. enterica |
>20/>20 |
20/>20 |
20/>20 |
10/>20 |
20/>20 |
20/>20 |
0.007/0.007 |
0.15/0.15 |
Y. enterocolitica |
>20/>20 |
5/>20 |
5/>20 |
2.5/>20 |
10/>20 |
1.25/>20 |
0.007/0.007 |
0.15/0.15 |
Gram-positive bacteria |
B. cereus |
>20/>20 |
>10/>20 |
>10/>20 |
10/>20 |
>20/>20 |
>20/>20 |
0.007/0.007 |
nt/nt |
L. monocytogenes |
>20/>20 |
>10/>20 |
>10/>20 |
20/>20 |
20/>20 |
20/>20 |
0.007/0.007 |
0.15/0.15 |
S. aureus |
>20/>20 |
20/>20 |
20/>20 |
10/>20 |
10/>20 |
20/>20 |
0.007/0.007 |
0.15/0.15 |
Antifungal activity |
|
|
|
|
Ketoconazole |
|
|
|
A. brasiliensis |
10/>20 |
10/>20 |
10/>20 |
10/>20 |
10/>20 |
10/>20 |
0.06/0.125 |
— |
A. fumigatus |
2.5/20 |
2.5/5 |
2.5/5 |
2.5/20 |
2.5/5 |
5/20 |
0.5/1 |
— |
Table 4 Antifungal activity (MIC/MBC, mg mL−1) of extracts obtained from novel biscuits formulated with ground chia seeds (GC), defatted ground chia seeds (DGC), carob flour (CF) and coconut sugar (CS) as compared with those from traditional ones (control: formulated with wheat flour and white sugar)
|
|
Ground chia seed |
Defatted ground chia seed |
Carob flour |
CS 100% |
Carob flour + coconut sugar 100% |
Positive control |
|
Control |
GC5 |
GC10 |
GC15 |
DGC5 |
DGC10 |
DGC15 |
CF1 |
CF5 |
CF10 |
CS100 |
CF1-CS100 |
CF5-CS100 |
CF10-CS100 |
Ketoconazole |
Results are mean ± standard deviation (n = 3). MIC: minimum inhibitory concentration; MBC: minimal bactericidal concentration. |
A. brasiliensis
|
10/20 |
10/20 |
10/20 |
10/20 |
10/20 |
10/20 |
5/10 |
5/10 |
10/20 |
10/20 |
10/20 |
10/20 |
10/>20 |
2.5/20 |
0.06/0.125 |
A. fumigatus
|
1.25/2.5 |
2.5/5 |
5/10 |
2.5/5 |
2.5/5 |
2.5/5 |
2.5/5 |
2.5/5 |
5/20 |
5/20 |
5/20 |
5/20 |
10/>20 |
2.5/20 |
0.5/1 |
In a recent revision concerning plants utilized as antimicrobials, it has been elucidated that Salvia species are notably abundant in various bioactive compounds, including terpene derivatives, essential oils, phenolic compounds, flavonoids, and tannins. These compounds have been closely associated with a range of bioactive properties, including antimicrobial activities, ascribed to Salvia species.42 In this sense, previous research by Kobus-Cisowska et al.43 reported the antimicrobial activity of ethanolic extracts from chia seeds. Consistent with our findings, ground chia seeds have shown antibacterial potential against L. monocytogenes, P. aeruginosa, and E. coli. This activity is primarily attributed to the high content of chlorogenic, ferulic, and protocatechuic acids, with greater extraction efficiency achieved when the seeds are ground. Similarly, antimicrobial properties have been previously described in carob samples.44 Recently, Djebari et al.45 evaluated the biological activities of extracts obtained from different edible parts of carob tree, including pulp and gum. Their study demonstrated that these extracts could inhibit the growth of pathogenic bacteria at concentrations of 20 mg mL−1, and completely halt bacterial growth at higher concentrations (>50 mg mL−1). The authors concluded that extracts from carob pulp and gum show promise as alternatives to synthetic additives in the medicinal industry. In this context, they may serve as potential antioxidant agents and preservatives that combat bacterial contamination. In the case of carob, the antimicrobial effect can be mainly attributed to the high content of phenolic acids, gallotannins, and flavonoids.46 While there is limited information in the literature regarding the antimicrobial activity of sugar samples, certain studies have demonstrated that other coconut-derived products, such as coconut water47 and virgin coconut oil,48 are capable of inhibiting the growth of microorganisms such as Salmonella typhimurium and Staphylococcus aureus.
None of the novel formulations exhibited antibacterial activity against the tested microorganisms as compared with wheat-based biscuits, with the exception of biscuits CF10-CS100, which inhibited the growth of B. cereus at concentration of 20 mg mL−1 (data not shown). However, all biscuits displayed antifungal activity (Table 4). Among them, CF10-CS100 highlighted for its inhibition against A. brasiliensis followed by the biscuit containing 15% defatted chia seeds (DGC15). In contrast, the control sample exhibited the highest inhibition against A. fumigatus, which proved to be the most sensitivity to all the extracts. Based on the literature, it is anticipated that the activity against microorganisms would diminish or even vanish with heat treatment. Xu et al.49 noted significant reductions in the fungistatic activity of flaxseed when exposed to high temperatures. Similar decreases in antimicrobial properties have been documented in other heat-treated products, including honey.50 At this point, it is worth considering the possible potential antimicrobial effect of Maillard reaction compounds generated during the heat treatment. In this regard, Diaz-Morales et al.51 reported the antimicrobial properties of melanoidins isolated from various bakery products, including bread and biscuits, suggesting their capacity to enhance food shelf-life and safety. These authors also noted that the antimicrobial properties of melanoidins can vary, influenced by both the microorganism species and the type of melanoidin. This variability could explain the differences observed in the present study for each biscuit, since the development of the products of the Maillard reaction and consequently its activity could depend on the composition of the ingredients and the amount incorporated to the formulations. Previous studies conducted by our research group has indicated that incorporating defatted flour into biscuit formulations led to a substantial rise in Maillard reaction products, including acrylamide and furan compounds.52 Furthermore, research by Turfani et al.13 highlighted an increase in the browning of wheat bread enriched with carob flour. This was attributed not only to the color of the added flour but also to a higher occurrence of the Maillard reaction during baking, a consequence of elevated lysine content in carob as compared with wheat.
3.2.2. Antioxidant, anti-inflammatory and cytotoxic activity.
The results of the antioxidant activity of the extracts of both ingredients and biscuit formulations are presented in Fig. 2 and Table 5. Fig. 2 displays the results obtained in the TBARS assay, where higher antioxidant activities are characterized by lower EC50 values. Porcine brain cells were selected due to their high content in polyunsaturated fatty acids. Carob flour and chia seeds exhibited a high capacity to inhibit the formation of TBARS, with EC50 values ranging from 0.18 mg mL−1 to 0.20–0.25 mg mL−1, respectively. Higher values were detected in coconut sugar (31.72 mg mL−1), while white sugar did not present any activity. The addition of these ingredients to the biscuit formulations promoted greater antioxidant activity compared to the control biscuit extracts, reaching EC50 values up to 5.57 and 4.54 mg mL−1 when 15% ground chia seeds and defatted ground chia seeds were incorporated in the biscuits, respectively. Concentrations were even lower when samples were formulated with 10% carob flour (1.19 mg mL−1), decreasing up to 0.66 mg mL−1 when white sugar was replaced by coconut sugar.
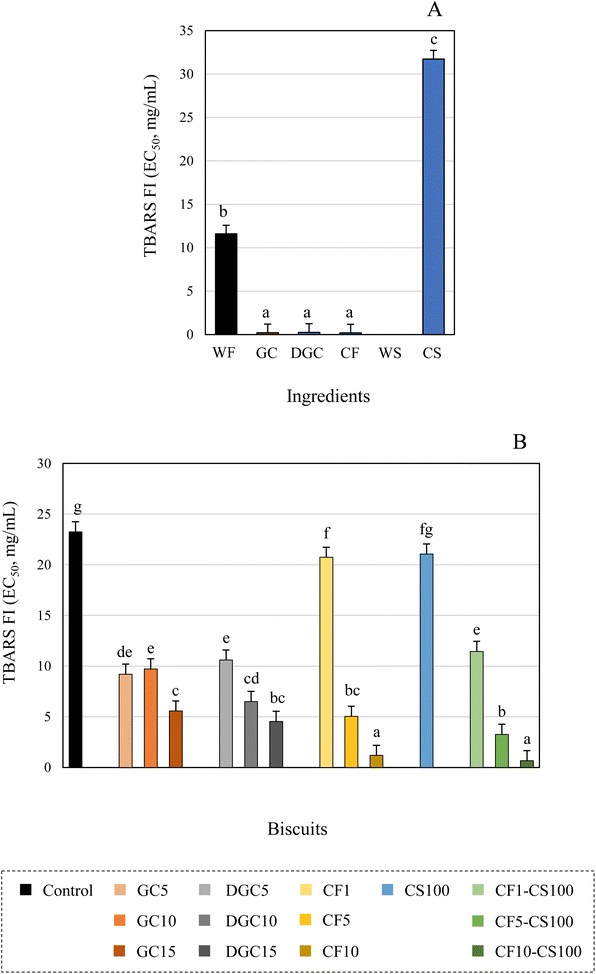 |
| Fig. 2 Antioxidant activity of extracts obtained from ingredients (A) (WF: wheat flour, GC: ground chia, DGC: defatted ground chia, CS: coconut sugar) and novel biscuit formulations (B) (TBARS assay). Different letters in ingredients or formulations indicate significant differences between samples (p < 0.05). | |
Table 5 Antioxidant, anti-inflammatory and cytotoxic activities of extracts obtained from ground chia seeds and defatted ground chia seeds
|
Ground chia seed |
Defatted ground chia seed |
Cellular antioxidant activity: quercetin: 95 ± 5% oxidation inhibition at 0.3 μg mL−1. Anti-inflammatory activity: IC50 values for dexamethasone: 6.3 ± 0.4 μg mL−1 (RAW 264.7). Cytotoxic activity: IC50 values for ellipticine: 1.23 ± 0.03 μg mL−1 (AGS), 1.21 ± 0.02 μg mL−1 (Caco-2), 1.02 ± 0.02 μg mL−1 (MCF-7), 1.01 ± 0.01 μg mL−1 (NCI-H460). Different letters in each row correspond to significant differences among extracts of chia seeds (p < 0.05). |
Cellular antioxidant activity (oxidation inhibition % at 2 mg mL−1) |
RAW 264.7 |
20% |
62% |
Anti-inflammatory activity (IC50 μg mL−1) |
RAW 264.7 |
18.9 ± 1.7 |
>400 |
Cytotoxic activity (GI50 μg mL−1) |
AGS |
202.1 ± 4.2a |
191.8 ± 6.7a |
Caco-2 |
130.4 ± 11.9a |
382.4 ± 8.2b |
MCF-7 |
220.7 ± 6.9a |
323.4 ± 2.2b |
NCI-H460 |
298.3 ± 10.0b |
175.5 ± 14.3a |
The high antioxidant capacity of chia seeds has been reported by other authors, mainly associated with the high content of polyphenolic compounds and bioactive peptides.53,54 Recently, Mas et al.54 reported the ability of chia polyphenols to reduce lipid peroxidation. Coelho et al.53 described that protein hydrolysates from defatted chia seeds have potential in vitro and in vivo antioxidant capacity and can effectively inhibit lipid oxidation in food models. In agreement with affirmations of Mas et al.,8 the supplementation of biscuits with chia seeds could be recommended to improve antioxidant properties of foods even when heat treated. These authors reported that the addition of 10% defatted chia seeds in cookies increased the polyphenol content and the in vitro antioxidant capacity of the formulations. Carob flour has also been noted to enhance the antioxidant capacity of various bakery products. Several studies, have demonstrated that the antioxidant power, measured by FRAP (Ferric Reducing Antioxidant Power) was notably elevated in bread made from blends of wheat flour and carob flours, especially unrefined carob flours at substitutions of 10% or more. Similarly, assays like ABTS (2,2′-azino-bis-3-ethylbenzthiazoline-6-sulphonic acid), DPPH (2,2-diphenyl-1-picrylhydrazyl) and HPS (Hydrogen peroxide scavenging) have indicated an increased antioxidant capacity in muffins supplemented with carob powder.13 This enhancement was linked to a greater contribution of total phenolic compounds and total flavonoids content in the baked goods.15 In addition to the composition of the innovative ingredients, it is essential to consider the contribution of Maillard reaction compounds generated during baking to the enhancement of the antioxidant capacity in the biscuit samples, as previously reported in other bakery products.18
In contrast to the antioxidant activity observed in the TBARS assay, for all the ingredients and biscuit formulations, only chia seeds exhibited cellular antioxidant, anti-inflammatory and cytotoxic activity (Table 5). The highest concentration tested (2 mg mL−1) inhibited the oxidation reaction generated in the RAW macrophages by 20% in the extracts from ground chia seed and by 62% in those prepared from defatted ground chia seeds. Regarding the anti-inflammatory activity, the concentration of extract required to inhibit 50% NO production was from 18.9 μg mL−1 in ground chia seeds whereas defatted samples did not present activity at the maximum concentration tested (> 0.4 mg mL−1). The anti-inflammatory effect of chia seed could be due to the presence of lipids in this matrix and particularly to the high content of polyunsaturated fatty acids, which have been previously associated with anti-inflammatory activity by acting as competitor substrates for the inhibition oxidation of arachidonic acid.55 High levels of polyunsaturated fatty acids in oils obtained from sea bass and bream head oils have been associated with the inhibition of NO in LPS-stimulated macrophage cells.30 Regarding the cytotoxic activity, the colon cancer cells (Caco-2) and the breast cancer cells (MFC-7) exhibited significantly higher susceptibility to the ground chia seeds (GI50 = 130.4 μg mL−1 and 220.7 μg mL−1, respectively) as compared with defatted ground chia seeds at the tested concentrations (GI50 = 382.4 μg mL−1 and 323.4 μg mL−1, respectively). In contrast, defatted samples inhibited to a significant greater extent the proliferation of lung cancer cells (NCI-H460) (GI50 = 175.5 μg mL−1vs. 298.3 μg mL−1 in ground chia seeds), whereas similar growth inhibition effects of stomach cancer cell lines (AGS) were observed for both samples (GI50 range: 191.8–202.1 μg mL−1). Again, the fat content of the seeds could be considered as responsible for this activity, since previous works have revealed the antiproliferative effect of omega-3 fatty acids against colon and lung cancer cells.56,57 Together with the fat content, other components of the seeds such as peptides could also be involved in cellular inhibition, which would explain the activity in the defatted ground chia seed.58
4. Conclusions
The partial replacement of wheat flour with ground chia seeds and carob flour and the total replacement of white sugar with coconut sugar in biscuit formulations enhanced the protein, mineral and particularly the fiber content. Additionally, the incorporation of chia seeds resulted in elevated levels of omega-3 fatty acids, which are essential for the human health. The new ingredients exhibited antibacterial and antifungal activity, particularly against Yersinia enterocolitica and Aspergillus fumigatus, although the final biscuits only displayed antifungal properties. The remarkable ability of chia seeds and carob flour to inhibit the formation of TBARS remained in the biscuits and the antioxidant capacity was even increased when white sugar was replaced by coconut sugar. Chia seeds were the only ingredient displaying cellular antioxidant, anti-inflammatory, and cytotoxic activities, which were not observed in the biscuit formulations. The results of this study emphasize the significant health benefits associated with the incorporation of these innovative ingredients into traditional biscuits. Moreover, they underscore the potential applications of these ingredients in the food industry, particularly when integrated into new cereal-based formulations.
Author contributions
M. M., F. J. M., L. B. and E. P. developed the study design, methodology and research. M. M., C. C., T. C. S. P. P. and R. C. C. performed the data collection. M. M. carried out the main analysis with supervision from T. C. S. P. P., R. C. C. and E. P. M. M. wrote the first and final drafts of the manuscript. F. J. M., C. C., T. C. S. P. P., R. C. C., L. B. and E. P. critically reviewed the manuscript. All authors contributed to the article and approved the submitted version.
Conflicts of interest
The authors declare that they have no known competing financial interests or personal relationships that could have appeared to influence the work reported in this paper.
Acknowledgements
This work was supported by the Community of Madrid and European funding from FSE and FEDER programs (project S2018/BAA-4393, AVANSECAL-II-CM). The authors are grateful to the Foundation for Science and Technology (FCT, Portugal) for financial support by national funds FCT/MCTES CIMO (UIDB/00690/2020 and UIDP/00690/2020) and SusTEC (LA/P/0007/2020); national funding by FCT, P.I., through the institutional scientific employment program-contract for L. Barros and R. Calhelha contracts, through the individual scientific employment program-contract for E. Pereira (2021.03908.CEECIND). C. Caleja are grateful to the VIIAFOOD—Plataforma de Valorização, Industrialização e Inovação comercial para o AgroAlimentar project for her contract (No. C644929456-00000040), a project supported under the PRR (https://www.recuperarportugal.gov.pt; accessed in 22 July 2023), and financed by the European Union/Next Generation EU. project for her contract.
References
- A. Nicolosi, V. R. Laganà and D. Di Gregorio, Habits, health and environment in the purchase of bakery products: consumption preferences and sustainable inclinations before and during COVID-19, Foods, 2023, 12, 1661, DOI:10.3390/foods12081661
.
- C. S. Birch and G. A. Bonwick, Ensuring the Future of Functional Foods, Int. J. Food Sci. Technol., 2019, 54, 1467–1485, DOI:10.1111/ijfs.14060
.
- A. Torbica, M. Belović and J. Tomić, Novel breads of non-wheat flours, Food Chem., 2019, 282, 134–140, DOI:10.1016/j.foodchem.2018.12.113
.
- M. Mesías, F. J. Morales and C. Delgado-Andrade, Acrylamide in biscuits commercialised in Spain: A view of the Spanish market from 2007 to 2019, Food Funct., 2019, 10, 6624–6632, 10.1039/C9FO01554J
.
-
D. Orona-Tamayo, M. E. Valverde and O. Paredes-López, Chia-The new golden seed for the 21st century: nutraceutical properties and technological uses, in Sustainable Protein Sources, ed. S. R. Nadathur, J. P. D. Wanasundara and L. Scanlin, Academic Press, San Diego, CA, USA, 2017, pp. 265–281 Search PubMed
.
- B. Kulczyński, J. Kobus-Cisowska, M. Taczanowski, D. Kmiecik and A. Gramza-Michałowska, The chemical composition and nutritional value of chia seeds—current state of knowledge, Nutrients, 2019, 11, 1242, DOI:10.3390/nu11061242
.
- L. Costantini, L. Lukšic, R. Molinari, I. Kreft, G. Bonafaccia, L. Manzi and N. Merendino, Development of gluten-free bread using tartary buckwheat and chia flour rich in omega-3 fatty acids and flavonoids as ingredients, Food Chem., 2014, 165, 232–240, DOI:10.1016/j.foodchem.2014.05.095
.
- A. L. Mas, F. I. Brigante, E. Salvucci, N. B. Pigni, M. L. Martinez, P. Ribotta, D. A. Wunderlin and M. V. Baroni, Defatted chia flour as functional ingredient in sweet cookies. How do Processing, simulated gastrointestinal digestion and colonic fermentation affect its antioxidant properties?, Food Chem., 2020, 316, 126279, DOI:10.1016/j.foodchem.2020.126279
.
- G. Adamczyk, E. Ivanišová, J. Kaszuba, I. Bobel, K. Khvostenko, M. Chmiel and N. Falendysh, Quality Assessment of Wheat Bread Incorporating Chia Seeds, Foods, 2021, 10, 2376, DOI:10.3390/foods10102376
.
- A. K. Yousif and H. M. Alghzawi, Processing and characterization of carob powder, Food Chem., 2000, 69, 283–287, DOI:10.1016/S0308-8146(99)00265-4
.
- M. D. Pettit and J. M. Pinilla, Production and purification of a sugar syrup from carob pods, LWT–Food Sci. Technol., 1995, 28, 145–152, DOI:10.1016/S0023-6438(95)80027-1
.
- A. Gioxari, C. Amerikanou, I. Nestoridi, E. Gourgari, H. Pratsinis, N. Kalogeropoulos, N. K. Andrikopoulos and A. C. Kaliora, Carob: A Sustainable Opportunity for Metabolic Health, Foods, 2022, 11, 2154, DOI:10.3390/foods11142154
.
- V. Turfani, V. Narducci, A. Durazzo, V. Galli and M. Carce, Technological, nutritional and functional properties of wheat bread enriched with lentil or carob flours, LWT–Food Sci. Technol., 2017, 78, 361–366, DOI:10.1016/j.lwt.2016.12.030
.
- E. E. Babiker, M. M. Özcan, K. Ghafoor, F. Al Juhaimi, A. A. M. Ahmed and I. A. Almusallam, Physico-chemical and bioactive properties, fatty acids, phenolic compounds, mineral contents, and sensory properties of cookies enriched with carob flour, J. Food Process. Preserv., 2020, 44, e14745, DOI:10.1111/jfpp.14745
.
- L. Červenka, M. Frühbauerová and H. Velichová, Functional properties of muffin as affected by substituting wheat flour with carob powder, Potr. Slovak. J. Food Sci., 2019, 13, 212–217, DOI:10.5219/1033
.
-
WHO (World Health Organization), Guideline: Sugars Intake for Adults and Children. World Health Organization (WHO), Geneva, Switzerland, 2015 Search PubMed
.
- E. Ruiz and G. Varela-Moreiras, Adequacy of the dietary
intake of total and added sugars in the Spanish diet to the recommendations: ANIBES study, Nutr. Hosp., 2017, 34, 45–52, DOI:10.20960/nh.1571
.
- Y. Shen, G. Chen and Y. Li, Bread characteristics and antioxidant activities of Maillard reaction products of white pan bread containing various sugars, LWT–Food Sci. Technol., 2018, 95, 308–315, DOI:10.1016/j.lwt.2018.05.008
.
-
Z. Szakály and M. Kiss, in Developing Sustainable and Health Promoting Cereals and Pseudocereals, ed. M. Rakszegi, M. Papageorgiou and J. M. Rocha, Academic Press, 2023, ch 20, pp. 467–488 Search PubMed
.
- S. L. Nassef, G. S. El-Hadidy and A. S. Abdelsattar, Impact of defatted chia seeds flour addition on chemical, rheological, and sensorial properties of toast bread, Egypt. J. Agric. Sci., 2022, 73, 55–66, DOI:10.21608/ejarc.2023.174785.1008
.
- F. Saitta, A. Apostolidou, M. Papageorgiou, M. Signorelli, I. Mandala and D. Fessas, Influence of carob flour ingredients on wheat-based systems, J. Cereal Sci., 2023, 111, 103655, DOI:10.1016/j.jcs.2023.103655
.
-
AACC (American Association of Cereal Chemists), Approved Methods of the American Association of Cereal Chemists, Association of Cereal Chemists, St Paul, Minnesota, 2000 Search PubMed
.
-
AOAC (Association of Official Analytical Chemists), Official methods of analysis of AOAC international, Association of Official Analytical Chemists, Maryland, 20th edn, 2016 Search PubMed
.
-
European Parliament & Council of the European Union, Regulation (EU) No 1169/2011 of the European Parliament and of the Council of 25 October 2011 on the provision of food information to consumers, amending Regulations (EC) No 1924/2006 and (EC) No 1925/2006 of the European Parliament and of the Council, and repealing Commission Directive 87/250/EEC, Council Directive 90/496/EEC, Commission Directive 1999/10/EC, Directive 2000/13/EC of the European Parliament and of the Council, Commission Directives 2002/67/EC and 2008/5/EC and Commission Regulation (EC) No 608/2004. O.J.E.U., L 304, 18-63, 2011.
- J. Pinela, J. C. M. Barreira, L. Barros, S. C. Verde, A. L. Antonio, A. M. Carvalho, M. B. P. P. Oliveira and I. C. F. R. Ferreira, Suitability of gamma irradiation for preserving fresh-cut watercress quality during cold storage, Food Chem., 2016, 206, 50–58, DOI:10.1016/j.foodchem.2016.03.050
.
- F. S. Reis, L. Barros, A. Martins and I. C. F. R. Ferreira, Chemical composition and nutritional value of the most widely appreciated cultivated mushrooms: an inter-species comparative study, Food Chem. Toxicol., 2012, 50, 191–197, DOI:10.1016/j.fct.2011.10.056
.
- T. C. S. P. Pires, M. I. Dias, L. Barros, R. C. Calhelha, M. J. Alves, M. B. P. P. Oliveira and I. C. F. R. Ferreira, Edible flowers as sources of phenolic compounds with bioactive potential, Food Res. Int., 2018, 105, 580–588, DOI:10.1016/j.foodres.2017.11.014
.
- S. A. Heleno, I. C. F. R. Ferreira, A. P. Esteves, A. Ćirić, J. Glamočlija, A. Martins and M. J. R. P. Queiroz, Antimicrobial and demelanizing activity of Ganoderma lucidum extract, p-hydroxybenzoic and cinnamic acids and their synthetic acetylated glucuronide methyl esters, Food Chem. Toxicol., 2013, 58, 95–100, DOI:10.1016/J.FCT.2013.04.025
.
- L. Lockowandt, J. Pinela, C. L. Roriz, C. Pereira, R. M. V. Abreu, R. C. Calhelha, M. J. Alves, L. Barros, M. Bredol and I. C. F. R. Ferreira, Chemical features and bioactivities of cornflower (Centaurea cyanus L.) capitula: The blue flowers and the unexplored non-edible part, Ind. Crops Prod., 2019, 128, 496–503, DOI:10.1016/j.indcrop.2018.11.059
.
- B. de la Fuente, J. Pinela, R. C. Calhelha, S. A. Heleno, I. C. F. R. Ferreira, F. J. Barba, H. Berrada, C. Caleja and L. Barros, Sea bass (Dicentrarchus labrax) and sea bream (Sparus aurata) head oils recovered by microwaveassisted extraction: Nutritional quality and biological properties, Food Bioprod. Process., 2022, 136, 97–105, DOI:10.1016/j.fbp.2022.09.004
.
- S. Souilem, Â. Fernandes, R. C. Calhelha, J. C. M. Barreira, L. Barros, F. Skhiri, A. Martins and I. C. F. R. Ferreira, Wild mushrooms and their mycelia as sources of bioactive compounds: antioxidant, anti-inflammatory and cytotoxic properties, Food Chem., 2017, 230, 40–48, DOI:10.1016/j.foodchem.2017.03.026
.
- F. Mandim, S. A. Petropoulos, J. Pinela, M. I. Dias, K. D. Giannoulis, M. Kostić and L. Barros, Chemical composition and biological activity of cardoon (Cynara cardunculus L. var. altilis) seeds harvested at different maturity stages, Food Chem., 2022, 369, 130875, DOI:10.1016/j.foodchem.2021.130875
.
- M. K. E. Youssef, M. M. El-Manfaloty and H. M. Ali, Assessment of proximate chemical composition, nutritional status, fatty acid composition and phenolic compounds of carob (Ceratonia Siliqua L.), Food Public Health, 2013, 3, 304–308, DOI:10.5923/j.fph.20130306.06
.
-
EC (European Commission), 2013, Commission implementing decision of 22.1.2013 authorising an extension of use of Chia (Salvia hispanica) seed as a novel food ingredient under Regulation (EC) No 258/97 of the European Parliament and of the Council, O.J.E.U., L21, 34–35.
- M. Mesías, F. Holgado, G. Márquez-Ruiz and F. J. Morales, Risk/benefit considerations of a new formulation of wheat-based biscuit supplemented with different amounts of chia flour, LWT–Food Sci. Technol., 2016, 73, 528–535, DOI:10.1016/j.lwt.2016.06.056
.
- C. B. Ioniţă-Mîndrican, K. Ziani, M. Mititelu, E. Oprea, S. M. Neacşu, E. Moroşan, D. E. Dumitrescu, A. C. Roşca, D. Drăgănescu and C. Negrei, Therapeutic benefits and dietary restrictions of fiber intake: A state of the art review, Nutrients, 2022, 14, 2641, DOI:10.3390/nu14132641
.
- A. Alkuwari, Z. U. Hassan, R. Zeidan, R. Al-Thani and S. Jaoua, Occurrence of mycotoxins and toxigenic fungi in cereals and application of yeast volatiles for their biological control, Toxins, 2022, 14, 404, DOI:10.3390/toxins14060404
.
- S. Petti and S. Scully, Polyphenols, oral health and disease: A review, J. Dent., 2009, 37, 413–423, DOI:10.1016/j.jdent.2009.02.003
.
- L. Tian, J. Fu, M. Wu, S. Liao, X. Jia, J. Wang, S. Yang, Z. Liu, Z. Liu, Z. Xue, Y. Wang, H. Li and G. Gong, Evaluation of gallic acid on membrane damage of Yersinia enterocolitica and its application as a food preservative in pork, Int. J. Food Microbiol., 2022, 374, 109720, DOI:10.1016/j.ijfoodmicro.2022.109720
.
- M. Wu, L. Tian, J. Fu, S. Liao, H. Li, S. Gai and G. Gong, Antibacterial mechanism of Protocatechuic acid against Yersinia enterocolitica and its application in pork, Food Control, 2022, 133, 108573, DOI:10.1016/j.foodcont.2021.108573
.
- H. Yan, X. Meng, X. Lin, N. Duan, Z. Wang and S. Wu, Antifungal activity and inhibitory mechanisms of ferulic acid against the growth of Fusarium graminearum, Food Biosci., 2023, 52, 102414, DOI:10.1016/j.fbio.2023.102414
.
-
R. M. Abdel-Massih and M. E. Beyrouthy, in Medicinal Plants as Anti-infectives, ed. F. Chassagne, Academic Press, 2022, ch 2, pp. 59–101 Search PubMed
.
- J. Kobus-Cisowska, D. Szymanowska, P. Maciejewska, D. Kmiecik, A. Gramza-Michałowska, B. Kulczyński and J. Cielecka-Piontek, In vitro screening for acetylcholinesterase and butyrylcholinesterase inhibition and antimicrobial activity of chia seeds (Salvia hispanica), Electron. J. Biotechnol., 2019, 37, 1–10, DOI:10.1016/j.ejbt.2018.10.002
.
- S. B. Ayache, F. S. Reis, M. I. Dias, C. Pereira, J. Glamočlija, M. Soković, E. B. Saafi, I. C. F. R. Ferreira, L. Barros and L. Achour, Chemical characterization of carob seeds (Ceratonia siliqua L.) and use of different extraction techniques to promote its bioactivity, Food Chem., 2021, 351, 129263, DOI:10.1016/j.foodchem.2021.129263
.
- S. Djebari, M. Wrona, C. Nerín, O. Djaoudene, S. Guemouni, A. Boudria and K. Madani, Phenolic compounds profile of macerates of different edible parts of carob tree
(Ceratonia siliqua L.) using UPLC-ESI-Q-TOF-MS: Phytochemical screening and biological activities, Fitoterapia, 2024, 172, 105696, DOI:10.1016/j.fitote.2023.105696
.
- I. J. Stavrou, A. Christou and C. P. Kapnissi-Christodoulou, Polyphenols in carobs: A review on their composition, antioxidant capacity and cytotoxic effects, and health impact, Food Chem., 2018, 269, 355–374, DOI:10.1016/j.foodchem.2018.06.152
.
- S. Beristaín-Bauza, A. Martínez-Niño, A. P. Ramírez-González, R. Ávila-Sosa, H. Ruíz-Espinosa, I. I. Ruiz-López and C. E. Ochoa-Velasco, Inhibition of Salmonella Typhimurium growth in coconut (Cocos nucifera L.) water by hurdle technology, Food Control, 2018, 92, 312–318, DOI:10.1016/j.foodcont.2018.05.010
.
- D. C. Widianingrum, C. T. Noviandi and S. I. O. Salasia, Antibacterial and immunomodulator activities of virgin coconut oil (VCO) against Staphylococcus aureus, Heliyon, 2019, 5, e02612, DOI:10.1016/j.heliyon.2019.e02612
.
- Y. Xu, C. Hall and C. Wolf-Hall, Fungistatic activity of heat-treated flaxseed determined by response surface methodology, J. Food Sci., 2008, 73, M250–M256, DOI:10.1111/j.1750-3841.2008.00806.x
.
- M. Majkut, J. Kwiecińska-Piróg, E. Wszelaczyńska, J. Pobereżny, E. Gospodarek-Komkowska, K. Wojtacki and T. Barczak, Antimicrobial activity of heat-treated Polish honeys, Food Chem., 2021, 343, 128561, DOI:10.1016/j.foodchem.2020.128561
.
- N. Diaz-Morales, M. Ortega-Heras, A. M. Diez-Maté, M. L. Gonzalez-SanJose and P. Muñiz, Antimicrobial properties and volatile profile of bread and biscuits melanoidins, Food Chem., 2022, 373, 131648, DOI:10.1016/j.foodchem.2021.131648
.
- M. Mesías, P. Gómez, E. Olombrada, F. Holgado and F. J. Morales, Risk/Benefit Evaluation of Chia Seeds as a New Ingredient in Cereal-Based Foods, Int. J. Environ. Res. Public Health, 2022, 20, 5114, DOI:10.3390/ijerph20065114
.
- M. S. Coelho, S. de Araujo Aquino, J. M. Latorres and M. M. Salas-Mellado, In vitro and in vivo antioxidant capacity of chia protein hydrolysates and peptides, Food Hydrocoll., 2019, 91, 19–25, DOI:10.1016/j.foodhyd.2019.01.018
.
- A. L. Mas, R. I. Bonansea, M. E. Fernandez, J. M. Kembro, M. C. Labaque, D. A. Wunderlin and M. V. Baroni, Dietary supplementation with chia polyphenols alleviates oxidative stress and improves egg nutritional quality in Japanese quails under heat stress, J. Therm. Biol., 2023, 111, 103421, DOI:10.1016/j.jtherbio.2022.103421
.
- P. C. Calder, Polyunsaturated fatty acids and inflammation, Biochem. Soc. Trans., 2005, 33, 423–427, DOI:10.1042/BST0330423
.
- P. Ahangar, M. Sam, V. Nejati and R. Habibian, Treatment of undifferentiated colorectal cancer cells with fish-oil derived docosahexaenoic acid triggers caspase-3 activation and apoptosis, J. Cancer Res. Ther., 2016, 12, 798–804, DOI:10.4103/0973-1482.157326
.
- Y. Yin, C. Sui, F. Meng, P. Ma and Y. Jiang, The omega-3 polyunsaturated fatty acid docosahexaenoic acid inhibits proliferation and progression of non-small cell lung cancer cells through the reactive oxygen species mediated inactivation of the PI3K/Akt pathway, Lipids Health Dis., 2017, 16, 87, DOI:10.1186/s12944-017-0474-x
.
- A. León Madrazo and M. R. Segura Campos, In silico prediction of peptide variants from chia (S. hispanica L.) with antimicrobial, antibiofilm, and antioxidant potential, Comput. Biol. Chem., 2022, 98, 107695, DOI:10.1016/j.compbiolchem.2022.107695
.
|
This journal is © The Royal Society of Chemistry 2024 |
Click here to see how this site uses Cookies. View our privacy policy here.