DOI:
10.1039/D3QM00131H
(Research Article)
Mater. Chem. Front., 2023,
7, 2454-2463
Red-shift emission and rapid up-conversion of B,N-containing electroluminescent materials via tuning intramolecular charge transfer†
Received
12th February 2023
, Accepted 20th March 2023
First published on 22nd March 2023
Abstract
Boron (B) and nitrogen (N)-based polycyclic aromatic hydrocarbons (PAHs) have been demonstrated as promising materials for building efficient thermally activated delayed fluorescent (TADF) emitters in blue and green regions, while red emission materials based on B,N systems are rare. Hence, to achieve a red-shifted emission peak over 600 nm by simply modifying the core of B,N-PAHs is a rewarding and challenging task. In this work, we demonstrate the para-D–π–B strategy implementation of modulating the predominance of locally excited (LE)/charger transfer (CT) states by introducing peripheral electron-donating units in a boron-carbazole containing backbone (BNCz) to develop four TADF emitters, BN-TC, BN-AC, BN-PXZ and BN-PZ. Due to the effect of different donor strengths on the excited states of these materials, we obtain full-color emission and a high photoluminescence quantum yield (ΦPL) of nearly 100%. Notably, the device employing BN-PZ as a dopant exhibits orange-red emission with an electroluminescence (EL) peak at 612 nm. Meanwhile, this compound realizes very fast reverse intersystem crossing (RISC) with a rate constant (kRISC) of 1.8 × 106 s−1, resulting in a device with a high external quantum efficiency (EQE) of 25.0% and low efficiency roll-off at high brightness.
1. Introduction
Organic light-emitting diodes (OLEDs) are now the star product in the full-color display and solid-state lighting fields. The internal quantum efficiency (IQE) of OLEDs based on pure organic TADF emitters is up to 100%, as 75% of the triplet excitons can be transferred from the lowest triplet (T1) excited state to the lowest singlet (S1) excited state by the reverse intersystem crossing process.1–3 In order to achieve an efficient RISC process, a small singlet–triplet splitting energy (ΔEST) between S1 and T1 in TADF materials is the critical point, which requires the overlap between the highest occupied molecular orbitals (HOMO) and the lowest unoccupied molecular orbitals (LUMO) to be as small as possible.4–9 The most widespread TADF molecular design strategy is the use of highly distorted donor–acceptor (D–A) structures.10–13 The twisted D–A framework accompanied by the intramolecular charge transfer (ICT) characteristic allows TADF materials to easily exhibit full-color emission, which is essential for color displays, white lighting, imaging applications, etc.14–16
In 2016, Hatakeyama and co-workers developed novel PAHs containing boron (B) and nitrogen (N) atoms. This B,N-based TADF material features outstanding blue emission with a high ΦPL and small ΔEST.17 B,N-based TADF materials emitting in blue and green regions are currently booming, owing to great efforts that have been made to modify and innovate this B,N-based core through different strategies. Duan et al. and Wang et al. both introduced acceptors at the para-carbon position of B-substituted phenyl rings (para-A–π–B), which could modulate the LUMO distribution and excited state of the molecule to red-shift the emission wavelength, resulting in efficient green OLEDs.18,19 Chou and co-workers reported a series of B,N-based TADF materials with green emission via the design approach of introducing sulfur atoms into the B,N-based core.20,21 Yasuda's group and Yang's group obtained highly-efficient yellow devices by a peripheral decoration strategy on the B,N-based core (para-D–π–N and peri-D–π–N).22,23 Recently, Duan and co-workers reported deep-red B,N-based TADF materials via the design approach of the para-B–π–B method.24 Regrettably, on the one hand, B,N-based TADF emitters in longer wavelength regions with emission peaks exceeding 600 nm remain scarce, while on the other hand, the up-conversion rates of these TADF materials are still limited to a magnitude order of 104 s−1, leading to severe triplet–triplet annihilation (TTA) and triplet–polaron annihilation (TPA). Hence, developing new schemes for obtaining red-shifting B,N-containing PAHs with high up-conversion rates is in urgent demand for producing efficient and low roll-off red TADF-OLEDs.
Herein, we propose and practice a novel strategy of modulating the HOMO level and predominance of locally excited (LE)/charger transfer (CT) states by introducing a donor. In detail, we introduce donors with different HOMO energy levels at the para-position of the B atom in the BNCz core,25,26 as shown in Fig. 1, namely, BN-TC, BN-AC, BN-PXZ and BN-PZ. It is worth noting that BNCz is not only an MR-TADF molecule,18,27,28 but it can also act as an acceptor in the D–A skeleton. When increasing the interaction strength of the donors, the excited state properties gradually tend from LE towards CT. As a result, we have succeeded in achieving full-color emissions from 464 nm to 634 nm in toluene through donor engineering. These TADF emitters exhibit high ΦPLs of nearly 100% and a remarkably elevated RISC rate (106 s−1) is obtained for BN-PZ. The corresponding blue and green devices achieve maximum external quantum efficiencies (EQE) of over 20%. Notably, the BN-PZ-based OLEDs display orange-red emission peaking at 612 nm, and a high maximum EQE of 25.0% with significantly low efficiency roll-off. A remarkable EQE of 17.6% at 1000 cd m−2 is the highest value reported for sensitizer-free TADF OLEDs with EL peaks over 610 nm so far. This work confirms that modulating the predominance of the LE/CT state is an effective method to realize red-shifted emission in B–N-containing PAHs.
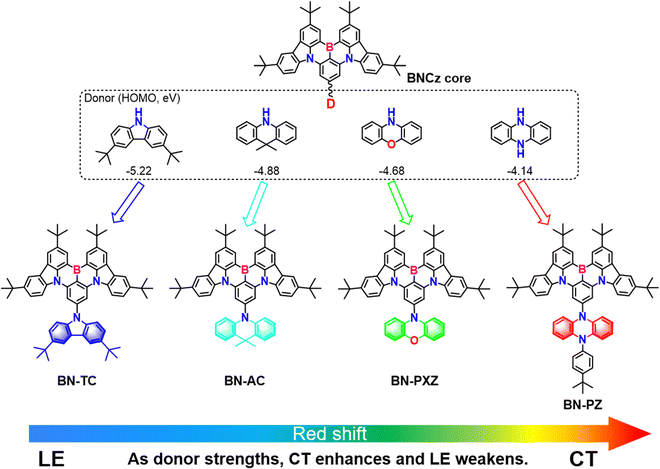 |
| Fig. 1 Design for full-color TADF emitters based on boron–nitrogen embedded polycyclic aromatic hydrocarbon as well as chemical structures of BNCz, BN-TC, BN-AC, BN-PXZ and BN-PZ. | |
2. Results and discussion
2.1. Molecular design and synthesis
Three designed boron-carbazole containing emitters, namely, BN-AC, BN-PXZ and BN-PZ, were synthesized by a nucleophilic aromatic substitution reaction and tandem electrophilic arene borylation (Experimental Section and Scheme S1 in the ESI†). These emitters have been characterized by 1H NMR, 13C NMR, and time of flight mass spectrometry (Fig. S1–S9, ESI†). All final products were purified by temperature-gradient sublimation under vacuum after recrystallization and the purity requirements of fabrication for OLEDs were achieved by vacuum deposition. Thermogravimetric analysis (TGA) and differential scanning calorimetry (DSC) indicated the excellent thermal stabilities of BN-AC, BN-PXZ and BN-PZ, with 5% weight-loss decomposition temperatures (Td) of 440 °C, 453 °C and 454 °C, respectively, and glass transition signals (Tg) of 253 °C, 268 °C and 271 °C, respectively (Fig. S10, ESI†). Such excellent thermal stability of these emitters is consistent with their rigid configurations, which fulfills the requirement of thermal evaporation. The single crystal structure of BN-PZBN-PZ was obtained from a toluene/CH2Cl2 mixed solution, as shown in Fig. S11 and S12 (ESI†) (CCDC number: 2241686†), revealing a highly distorted molecular geometry with a large dihedral angle of 84.9° between the B,N-core and phenazine. The twisted D–A structure and the strongest PZ donor could strengthen the ICT state, enabling the simultaneous realization of long-wavelength emission.
2.2. Theoretical calculations
To understand the effect of different para-donor units of the backbone on molecular geometry and optoelectronic properties, density functional theory (DFT) and time-dependent DFT (TD-DFT) calculations were performed using the B3LYP functional with the 6-31G(d) basis set in the Gaussian 16 program. The frontier orbital distributions and energy levels of these compounds are depicted in Fig. 2. The dihedral angles of the twisted D–A structure are estimated to be 52°, 84°, 74° and 77° for BN-TC, BN-AC, BN-PXZ and BN-PZ, respectively. The large dihedral angles are beneficial for enhancing the ICT character and lowering ΔEST. BN-TC shows a delocalized HOMO–LUMO distribution, whereas the HOMO and LUMO orbitals of BN-AC, BN-PXZ and BN-PZ are separated. In addition, the energy gap (Eg), S1 and T1 all decrease as the donor strength increases, which could cause spectral red-shifts. They also exhibit large oscillator strengths (f) of 0.3912, 0.4139, 0.4163 and 0.3444, indicating a fast-radiative decay and high ΦPL.
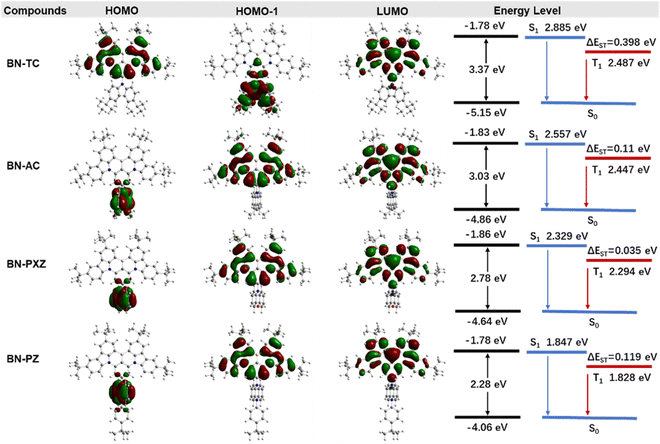 |
| Fig. 2 Distributions of frontier molecular orbitals, calculated energy gaps and energy-level diagrams for the singlet and triplet excited states of BN-TC, BN-AC, BN-PXZ and BN-PZ. | |
Furthermore, the natural transition orbitals (NTOs) of S1 and triplet (Tn) excited states of these materials were calculated and are shown in Fig. 3. The S1 excited states of all three molecules show completely separated “holes” and “particles” respectively located on the donor and BNCz core, but their Tn excited states are distinguishing. For BN-AC, the ΔEST between the S1 and T1 excited state is 0.110 eV, while ΔEST between the S1 and second triplet (T2) excited state is 0.010 eV, which seems small enough to establish a feasible RISC channel. However, the S1 and T2 excited states have highly similar CT features, which forbids spin–orbital coupling (SOC) between them, and therefore invalidates T2 → S1 RISC.28 In addition, the simulated SOC matrix element (
) between the LE-predominant T1 excited state and S1 excited state of BN-AC reaches 0.436 cm−1 (
), which is one order of magnitude higher than that between T2 and S1 (
). The T2 excited state of BN-PXZ is mainly centralized on the BNCz core, corresponding to LE-predominance. Despite a smaller energy gap between its T1 and S1, T1 can also not support RISC to S1, because its CT feature induced vanishing of SOC. Meanwhile, SOC of the S1 → T1 excitation sharply decreases by several times, compared to that of S1 → T2 excitation (
,
). The T1 excited state of BN-PZ exhibits a similar “hole” and “particle” distribution to S1, while T2 is unable to establish a transition channel with S1, due to an unbridgeable energy gap. In a word, both BN-AC and BN-PXZ simultaneously contain CT-predominant S1 and LE-predominant Tn excited states, while BN-PZ only has the CT feature, demonstrating that excited states convert from LE-predominant to CT-predominant with increasing donor strength, in which a limit that determines the role of the BN core as an emitter or an acceptor probably exists. More specifically, on connecting a para-donor with a shallower HOMO energy level than that of PXZ, the molecule will exhibit a CT-predominant excited state, while the BNCz core will act as an acceptor. In this case, we predict that BN-AC and BN-PXZ should exhibit narrow-band emissions combined with the CT feature, while BN-PZ is identical to common D–A type molecules with broad emission.
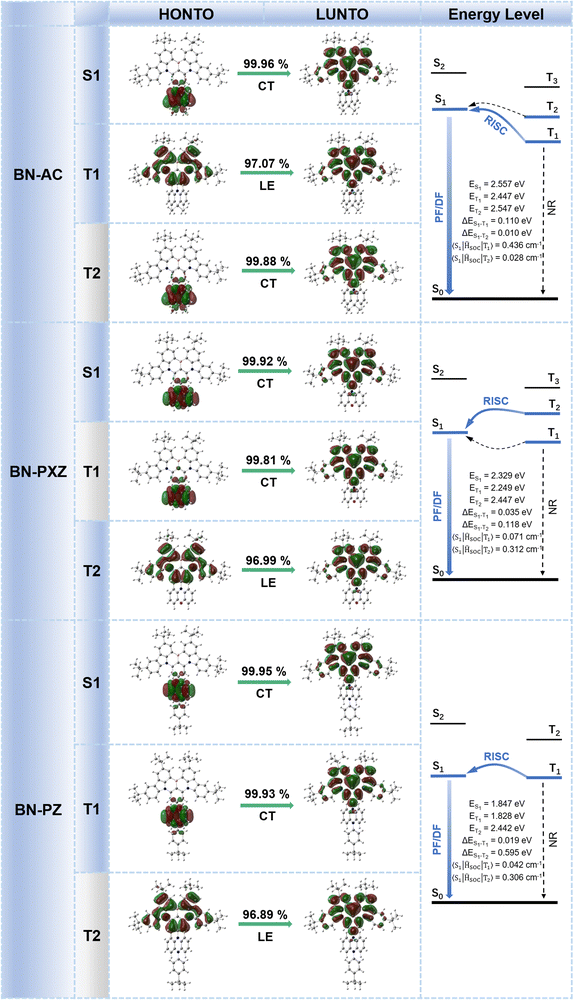 |
| Fig. 3 Energy-level diagrams and related NTOs for the singlet and triplet excited states of BN-AC, BN-PXZ and BN-PZ. Transition energies for S1 and Tn (n = 1, 2) and the SOC matrix elements were calculated at the B3LYP/6-31G(d) levels of theory, respectively. | |
2.3. Photophysical properties
The photophysical spectroscopic properties of BN-AC, BN-PXZ and BN-PZ are shown in Fig. 4, and the key data are summarized in Table 1. As predicted by DFT calculations, the UV absorption of these materials shows a strong MR characteristic peak at ∼464 nm, which originates from the B,N-containing framework. Due to the shallowest HOMO energy level of the donor PZ, BN-PZ has an additional low energy ICT absorption band at 490–550 nm (Fig. S13, ESI†) and exhibits red emission with a peak at 634 nm in a dilute toluene solution (Fig. 4b). The S1 and T1 energy values are obtained from the onset wavelength of low-temperature fluorescence and phosphorescence spectra, based on which the ΔEST values are calculated to be 0.14 eV, 0.12 eV and 0.03 eV for BN-AC, BN-PXZ and BN-PZ, respectively. Such a tiny ΔEST can benefit efficient recycling of the triplet excitons via the rapid RISC process. The PL spectra of BN-AC and BN-PXZ in dilute toluene are almost identical, resulting from the similar emissions in solution originating from their LE states, but the curve of BN-PXZ in the region over 510 nm is relatively higher than that of BN-AC, which is consistent with the stronger CT state of BN-PXZ (Fig. 4b). The solvent polarity-dependent emission behaviors of emitters were studied using the Lippert–Mataga model, in which the X-axis stands for the solvent polarity factor f, and the Y-axis represents Stokes’ shift (Fig. S14 and Table S2, ESI†). The UV-vis absorption spectrum of BN-AC and BN-PXZ showed a slight change within a scope of 5 nm as the solvent polarity increased, indicating a negligible dipolar change in the S0 state with the change in solvent polarity. The dipole moment of S1 excitons can be estimated from the fitted line of experimental points. Their two-section lines corresponded to the two different exciton states with two distinguishable small and large dipole moments in low-polarity and high-polarity solvents, respectively. In the case of low-polarity solvents, the S1 excitons of BN-AC and BN-PXZ behaved as LE states with small dipole moments of 4.47 D and 7.54 D, respectively, while their S1 excitons exhibited large dipole moments of 14.23 D and 14.02 D, respectively, in high-polarity solvents, which clearly illustrated a considerable contribution of ICT character in the excited state. Compared with the other emitters, BN-PZ exhibits only CT-dominant emission in both low- and high-polarity solvents, demonstrating the ICT characteristic expected from molecular design. In addition, BN-PZ exhibits a wider photoluminescence (PL) spectrum than the others, resulting from dominance of the CT state rather than the LE state, which is consistent with the theory of spontaneous emission that the full width at half maximum (FWHM) is proportional to the square of the emission peak wavelength.29 When doped in a 3,3′-bis(carbazol-9-yl)biphenyl (mCBP) host, all three materials display slightly broadened spectra due to π–π interactions (Fig. 4c). It is noted that, due to the host–guest interaction, the CT state also participates in the emission in the doped film, in which BN-PXZ exhibits a red-shifted shoulder peak in the region over 510 nm. Moreover, the ΦPL values in mCBP doped films are measured to be 94%, 97% and 98% for BN-AC, BN-PXZ and BN-PZ, exhibiting PL emission at 484 nm, 486 nm and 610 nm, respectively.
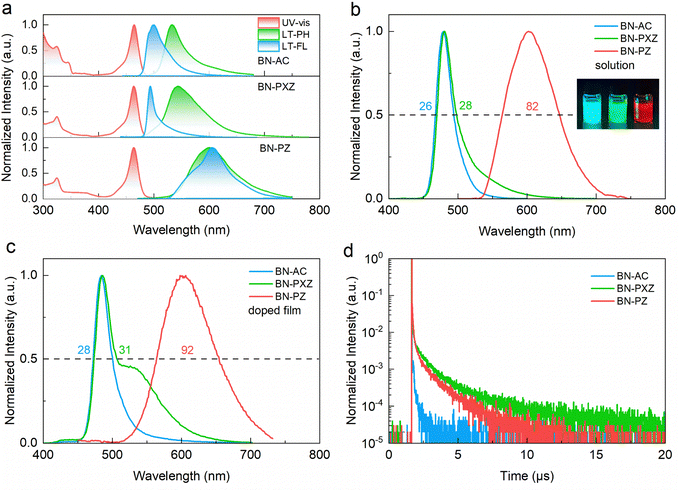 |
| Fig. 4 (a) UV-vis absorption (Abs., in 1 × 10−5 M toluene), steady-state fluorescence (LT-FL, 77 K in neat films), and phosphorescence (LT-Ph, 77 K in neat films) spectra. (b) Photoluminescence spectra in toluene (1 × 10−5 M). (c) Photoluminescence spectra in 15 wt% doped films (host: mCBP). (d) Transient photoluminescence decay profiles (doped films in vacuo). | |
Table 1 Physical properties of BNCz, BN-TC, BN-AC, BN-PXZ and BN-PZ
Compounds |
λ
abs
(nm) |
λ
em
(nm) |
FWHMa (nm) |
Stokes shifta (nm) |
E
S1/ET1/ΔESTc (eV) |
HOMO/LUMOd (eV) |
T
d
(°C) |
In toluene, 1.0 × 10−5 M.
λ
em measured in the 15%-doped film in mCBP host.
E
S1 and ET1 were determined from the onsets of LTFL and LTPh, respectively; ΔEST = ES1 – ET1.
HOMO levels were determined from the onset of the oxidation curves, and LUMO levels were calculated by using the HOMO levels and Eg.
Measured from thermal gravimetric analysis (TGA).
Literature.19,28,29
|
BNCz
|
467 |
481 |
44 |
14 |
2.66/2.53/0.13 |
−5.40/−2.85 |
— |
BN-TC
|
460 |
464 |
22 |
4 |
2.64/2.50/0.14 |
−5.46/−2.85 |
— |
BN-AC
|
464 |
479 (484)b |
26 |
14 |
2.59/2.45/0.14 |
−5.33/−2.74 |
447 |
BN-PXZ
|
464 |
480 (486)b |
28 |
14 |
2.57/2.45/0.12 |
−5.14/−2.55 |
458 |
BN-PZ
|
464 |
634 (610)b |
82 |
70 |
2.34/2.31/0.03 |
−4.58/−1.99 |
466 |
To further investigate the photophysical properties of these emitters, the transient PL spectra of the doped films in mCBP host matrix were recorded. As depicted in Fig. 4d, BN-AC, BN-PXZ and BN-PZ exhibited a significantly delayed fluorescence behavior owing to the small ΔEST values, showing prompt fluorescence lifetimes (τp)/delayed fluorescence lifetimes (τd) of 3.8 ns/9.7 μs, 8.9 ns/1.7 μs and 39.4 ns/1.2 μs, respectively. Thereinto, the BN-AC-based doped film displayed shorter τp compared to the tens of nanoseconds for BN-PZ, which is consistent with the gradual decreasing proportion of the prompt fluorescence component from BN-AC to BN-PZ (Table S3, ESI†). Moreover, the short τd value of about 1 μs of the BN-PZ is conducive to the rapid RISC process. Furthermore, by incorporating the large rate constants of singlet radiative decay, the concentration quenching of triplet excitons and efficiency roll-off in electroluminescent devices can be effectively alleviated at high brightness.27,30 The temperature dependence of transient decay in the doped films clearly shows that the delayed fluorescence (DF) component increased progressively alone with rising temperature, indicating the TADF process in the molecules (Fig. S15, ESI†).
To understand the S1 and T1 exciton dynamic processes of these TADF emitters in doped films, the rate constants, such as singlet radiative decay rate constants (kr), nonradiative decay rate constants (knr), internal conversion rate constants (kIC), intersystem crossing rate constants (kISC), and reverse intersystem crossing rate constants (kRISC) are estimated according to the formulas provided in the literature and summarized in the Table S3 (ESI†).31–33 Resulting from the highly rigid planar parent core BNCz unit, the kr values of these emitters are over 107 s−1. Benefiting from the increasing ICT character and small ΔEST, the kRISC value of BN-PZ is in the range of 106 s−1, which is much higher than those of most of the reported BNCz-based TADF emitters, which suggests an efficient T1 → S1 exciton up-conversion and high utilization of excitons.
2.4. Electroluminescent device performance
The EL properties of these TADF emitters were further investigated with the device structures of indium tin oxide (ITO)/1,4,5,8,9,11-hexaazatriphenylenehexacarbonitrile (HATCN, 10 nm)/di-[4-(N,N-ditolyl-amino)-phenyl] cyclohexane (TAPC, 60 nm)/3,3′-di(carbazol-9-yl)biphenyl (mCBP, 10 nm)/mCBP:x wt% emitters (20 nm)/1,3,5-tri(m-pyrid-3-ylphenyl)benzene (TmPyPB, 45 nm)/LiF (1 nm)/Al (100 nm). Herein, ITO and Al were used as the anode and cathode, respectively; HATCN and LiF served as the hole- and electron-injecting layers, respectively; TAPC and TmPyPB played the role of hole- and electron-transporting layers, respectively; mCBP acted as an electron blocking layer (EBL) and the host to form an emitting layer (EML). The corresponding energy level diagrams of each layer are illustrated in Fig. 5a. For the emitting layer, the optimized dopant concentration is 5 wt% for both BNCz and BN-TC, and 15 wt% for the other three TADF emitters (Fig. S16, ESI†). The current density–voltage–luminance (J–V–L) characteristics of these devices are shown in Fig. 5b and the key data of the devices are summarized in Table 2. The OLEDs based on BN-AC and BN-PXZ display attractively narrow EL emissions, with emission peaks at 484 nm and 482 nm, FWHM values of 27 nm and 25 nm, high maximum EQE values of 23.4% and 24.4%, and CIE coordinates of (0.10, 0.31) and (0.23, 0.43), respectively (Fig. 5c and 5d). Expectedly, the presence of a shoulder peak in the EL spectrum of BN-PXZ catches our attention. One explanation attributes this type of shoulder peak to dimer emission;18,31 however, there is no shoulder peak in the EL spectrum of BN-AC with a similar conformation and dihedral angle. Hence, we propose a more plausible explanation: both LE state emission and relatively weak CT state emission exist in the emission of BN-PXZ, so a shoulder peak appears. And this is consistent with the NTO simulation results and PL spectra. Besides, the EL spectra of BN-AC, BN-PXZ and BN-PZ are highly stable under different operating voltages without emission shifting, and the device performances of BNCz and BN-TC are essentially the same as those reported in the literature. (Fig. S17 and S18, ESI†).26,27 More importantly, the BN-PZ-based OLEDs exhibit prominent EL performances with a high maximum luminance (Lmax) of 44210 cd m−2 and orange-red emission with a peak at 612 nm, which is in agreement with the PL spectra in doped films. Without using TADF-sensitizing fluorescence (TSF) technology, this device achieves a maximum EQE (EQEmax) as high as 25.0%. Furthermore, the BN-PZ-based device displays significantly alleviated efficiency roll-off of 15.2% and 29.6% with EQE values of 21.2% and 17.6% in 100 and 1000 cd m−2 (Fig. 5c and Table 2), respectively. In comparison with most sensitizer-free TADF OLEDs in the emission region over 610 nm, the BN-PZ-based device exhibits state-of-the-art EQE in 1000 cd m−2 and a lower efficiency roll-off, which can be attributed to the suppressed TTA and TPA in EML at high brightness due to its higher kRISC (Fig. S19 and Table S4, ESI†).4,14,16,34–59 Although the orange-red device displays high EQE and low efficiency roll-off, we expect to find a suitable TADF sensitizer that will be employed to further improve device efficiency and stability.
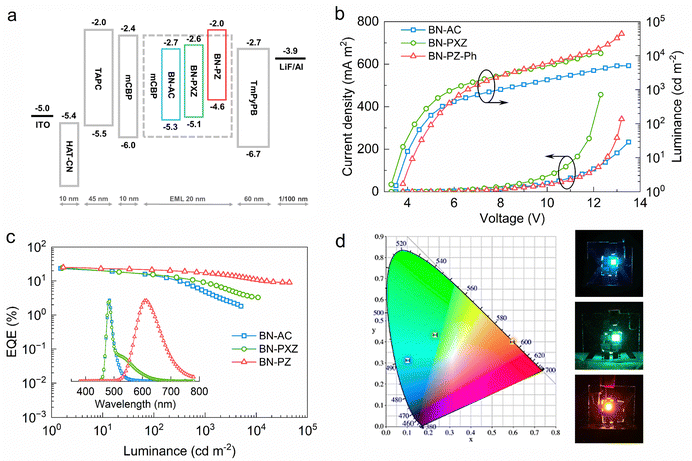 |
| Fig. 5 (a) Device structure and energy level diagram of the materials used. (b) Current density and luminance versus voltage (J–V–L). (c) External quantum efficiency versus luminance (EQE-L) curves of the devices (inset: EL spectra of the devices). (d) The color coordinates of the devices on the CIE 1931 color space and the photographs showing the emission color of the 15 wt%-doping devices. | |
Table 2 The device performances of BNCz, BN-TC, BN-AC, BN-PXZ and BN-PZ
Compounds |
V
ON
(V) |
λ
EL
(nm) |
FWHMc (nm) |
L
max
(cd m−2) |
CEmaxe (cd A−1) |
PEmaxf (lm W−1) |
EQEg (%) |
CIEh (x, y) |
Turn-on voltage at 1 cd m−2.
EL maximum wavelength.
Full width at half maximum.
Maximum luminance.
Maximum current efficiency.
Maximum power efficiency.
Maximum external quantum efficiency and values at 100 and 1000 cd m−2.
Value at 100 cd m−2.
|
BNCz
|
3.8 |
490 |
32 |
5017 |
34.3 |
29.0 |
21.1/13.0/5.9 |
(0.13, 0.41) |
BN-TC
|
4.1 |
474 |
37 |
4448 |
28.2 |
21.6 |
21.2/8.7/4.2 |
(0.13, 0.20) |
BN-AC
|
3.5 |
484 |
27 |
5089 |
35.1 |
31.5 |
23.4/15.9/5.5 |
(0.10, 0.31) |
BN-PXZ
|
3.3 |
482 |
25 |
11710 |
51.2 |
48.7 |
24.4/15.5/8.2 |
(0.23, 0.43) |
BN-PZ
|
3.8 |
612 |
104 |
44210 |
34.9 |
29.2 |
25.0/21.2/17.6 |
(0.60, 0.40) |
3. Conclusions
In conclusion, we propose a novel strategy of introducing a shallow HOMO-energy donor unit at the para-carbon position in the core of B,N-PAHs, effectively enabling red-shifted emission. Benefiting from a novel strategy of modulating the HOMO level and predominance of the LE/CT state, these TADF materials exhibit full-color emission and ΦPL values of nearly 100%. The OLEDs based on these dopants achieve high EQEs of over 20%. Owing to the strong ICT character and faster RISC (kRISC > 106 s−1), the orange-red device utilizing BN-PZ as an emitter shows a maximum EQE of 25% with an emission peak at 612 nm, and attains a high maximum Lmax of 44210 cd m−2 and low efficiency roll-off with a remarkable EQE of 17.6% in 1000 cd m−2, which is the highest value reported for sensitizer-free TADF OLEDs with EL peaks over 610 nm so far. This work has provided a facile and effective strategy to modulate the LE/CT state of B,N-containing PAHs for efficient and stable red TADF-OLEDs.
4. Experimental section
Synthesis
Detailed synthetic procedure and general analysis of the materials are described in the ESI.†
Device fabrication and measurements
Devices were fabricated at a vacuum level lower than 1 × 10−4 Pa for both organic and metal layers. ITO coated glasses with a sheet resistance of 15 Ω square−1 were used as the substrate and cleaned with ultra-purified water and organic solvents, and then irradiated in UV-ozone for 15 min. Organic materials were evaporated to ITO at a rate of about 1 Å s−1, while LiF and Al were evaporated at rates of around 0.1 and 5 Å s−1, respectively. The intersection of ITO and the metal electrodes gave a 10 mm2 active device area.
Conflicts of interest
The authors declare that they have no known competing financial interests or personal relationships that could have appeared to influence the work reported in this paper.
Acknowledgements
The authors acknowledge the financial support from the National Key R&D Program of China (no. 2022YFE0206100), the Science and Technology Development Fund (FDCT), Macau SAR (no. 0008/2022/AMJ), the National Natural Science Foundation of China (no. 62274117, 62075061), the Jiangsu Provincial department of Science and Technology (no. BZ2022054), the Science and Technology Innovation Plan Of Shanghai Science and Technology Commission (no. 22520760600), the Bureau of Science and Technology of Suzhou Municipality (no. SYC2022144), and the Collaborative Innovation Center of Suzhou Nano Science & Technology.
References
- H. Uoyama, K. Goushi, K. Shizu, H. Nomura and C. Adachi, Highly efficient organic light-emitting diodes from delayed fluorescence, Nature, 2012, 492, 234–238 CrossRef CAS PubMed
.
- Y. Tao, K. Yuan, T. Chen, P. Xu, H. Li, R. Chen, C. Zheng, L. Zhang and W. Huang, Thermally Activated Delayed Fluorescence Materials Towards the Breakthrough of Organoelectronics, Adv. Mater., 2014, 26, 7931–7958 CrossRef CAS PubMed
.
- Z. Yang, Z. Mao, Z. Xie, Y. Zhang, S. Liu, J. Zhao, J. Xu, Z. Chi and M. P. Aldred, Recent advances in organic thermally activated delayed fluorescence materials, Chem. Soc. Rev., 2017, 46, 915–1016 RSC
.
- J.-X. Chen, W.-W. Tao, W.-C. Chen, Y.-F. Xiao, K. Wang, C. Cao, J. Yu, S. Li, F.-X. Geng, C. Adachi, C.-S. Lee and X.-H. Zhang, Red/Near-Infrared Thermally Activated Delayed Fluorescence OLEDs with Near 100% Internal Quantum Efficiency, Angew. Chem., Int. Ed., 2019, 58, 14660–14665 CrossRef CAS PubMed
.
- Y.-Z. Shi, K. Wang, S.-L. Zhang, X.-C. Fan, Y. Tsuchiya, Y.-T. Lee, G.-L. Dai, J.-X. Chen, C.-J. Zheng, S.-Y. Xiong, X.-M. Ou, J. Yu, J.-S. Jie, C.-S. Lee, C. Adachi and X.-H. Zhang, Characterizing the Conformational Distribution in an Amorphous Film of an Organic Emitter and Its Application in a “Self-Doping” Organic Light-Emitting Diode, Angew. Chem., Int. Ed., 2021, 60, 25878–25883 CrossRef CAS PubMed
.
- L. Zhou, H. Wang, Y.-Z. Shi, X.-C. Fan, J.-X. Chen, K. Wang, J. Yu and X.-H. Zhang, Controlling the conjugation extension inside acceptors for enhancing reverse intersystem crossing of red thermally activated delayed fluorescence emitters, Chem. Eng. J., 2022, 440, 135775 CrossRef CAS
.
- M. Zhang, C.-J. Zheng, K. Wang, Y.-Z. Shi, D.-Q. Wang, X. Li, H. Lin, S.-L. Tao and X.-H. Zhang, Hydrogen-Bond-Assisted Exciplex Emitters Realizing Improved Efficiencies and Stabilities in Organic Light Emitting Diodes, Adv. Funct. Mater., 2021, 31, 2010100 CrossRef CAS
.
- Z. Li, D. Yang, C. Han, B. Zhao, H. Wang, Y. Man, P. Ma, P. Chang, D. Ma and H. Xu, Optimizing Charge Transfer and Out-Coupling of A Quasi-Planar Deep-Red TADF Emitter: towards Rec.2020 Gamut and External Quantum Efficiency beyond 30%, Angew. Chem., Int. Ed., 2021, 60, 14846–14851 CrossRef CAS PubMed
.
- C.-C. Yan, X.-D. Wang and L.-S. Liao, Thermally Activated Delayed Fluorescent Gain Materials: Harvesting Triplet Excitons for Lasing, Adv. Sci., 2022, 9, 2200525 CrossRef CAS PubMed
.
- S.-Y. Yang, Z.-Q. Feng, Z. Fu, K. Zhang, S. Chen, Y.-J. Yu, B. Zou, K. Wang, L.-S. Liao and Z.-Q. Jiang, Highly Efficient Sky-Blue π-Stacked Thermally Activated Delayed Fluorescence Emitter with Multi-Stimulus Response Properties, Angew. Chem., Int. Ed., 2022, 61, e202206861 CAS
.
- X. Tang, L.-S. Cui, H.-C. Li, A. J. Gillett, F. Auras, Y.-K. Qu, C. Zhong, S. T. E. Jones, Z.-Q. Jiang, R. H. Friend and L.-S. Liao, Highly efficient luminescence from space-confined charge-transfer emitters, Nat. Mater., 2020, 19, 1332–1338 CrossRef CAS PubMed
.
- Y. Liu, J. Yang, Z. Mao, X. Chen, Z. Yang, X. Ge, X. Peng, J. Zhao, S.-J. Su and Z. Chi, Asymmetric Thermally Activated Delayed Fluorescence Emitter for Highly Efficient Red/Near-Infrared Organic Light-Emitting Diodes, ACS Appl. Mater. Interfaces, 2022, 14, 33606–33613 CrossRef CAS PubMed
.
- H.-Y. Yang, H.-y Zhang, M. Zhang, X.-c Fan, H. Lin, S.-L. Tao, C.-J. Zheng and X.-H. Zhang, Multiplying the efficiency of red thermally activated delayed fluorescence emitter by introducing intramolecular hydrogen bond, Chem. Eng. J., 2022, 448, 137717 CrossRef CAS
.
- Z. Cai, X. Wu, H. Liu, J. Guo, D. Yang, D. Ma, Z. Zhao and B. Z. Tang, Realizing Record-High Electroluminescence Efficiency of 31.5% for Red Thermally Activated Delayed Fluorescence Molecules, Angew. Chem., Int. Ed., 2021, 60, 23635–23640 CrossRef CAS PubMed
.
- J.-L. He, F.-C. Kong, B. Sun, X.-J. Wang, Q.-S. Tian, J. Fan and L.-S. Liao, Highly efficient deep-red TADF organic light-emitting diodes via increasing the acceptor strength of fused polycyclic aromatics, Chem. Eng. J., 2021, 424, 130470 CrossRef CAS
.
- J.-X. Chen, Y.-F. Xiao, K. Wang, D. Sun, X.-C. Fan, X. Zhang, M. Zhang, Y.-Z. Shi, J. Yu, F.-X. Geng, C.-S. Lee and X.-H. Zhang, Managing Locally Excited and Charge-Transfer Triplet States to Facilitate Up-Conversion in Red TADF Emitters That Are Available for Both Vacuum- and Solution-Processes, Angew. Chem., Int. Ed., 2021, 60, 2478–2484 CrossRef CAS PubMed
.
- T. Hatakeyama, K. Shiren, K. Nakajima, S. Nomura, S. Nakatsuka, K. Kinoshita, J. Ni, Y. Ono and T. Ikuta, Ultrapure Blue Thermally Activated Delayed Fluorescence Molecules: Efficient HOMO–LUMO Separation by the Multiple Resonance Effect, Adv. Mater., 2016, 28, 2777–2781 CrossRef CAS PubMed
.
- Y. Zhang, D. Zhang, J. Wei, Z. Liu, Y. Lu and L. Duan, Multi-Resonance Induced Thermally Activated Delayed Fluorophores for Narrowband Green OLEDs, Angew. Chem., Int. Ed., 2019, 58, 16912–16917 CrossRef CAS PubMed
.
- Y. C. L. Xu, C. L. Li, Z. Q. Wang, J. X. Xue, J. N. Wang, Q. Y. Cai and X. L. Wang, Y, Highly Efficient Electroluminescent Materials with High Color Purity Based on Strong Acceptor Attachment onto B-N-Containing Multiple Resonance Frameworks, CCS Chem, 2021, 3, 2077–2091 Search PubMed
.
- X. Wu, J.-W. Huang, B.-K. Su, S. Wang, L. Yuan, W.-Q. Zheng, H. Zhang, Y.-X. Zheng, W. Zhu and P.-T. Chou, Fabrication of Circularly Polarized MR-TADF Emitters with Asymmetrical Peripheral-Lock Enhancing Helical B/N-Doped Nanographenes, Adv. Mater., 2022, 34, 2105080 CrossRef CAS PubMed
.
- M. Yang, S. Shikita, H. Min, I. S. Park, H. Shibata, N. Amanokura and T. Yasuda, Wide-Range Color Tuning of Narrowband Emission in Multi-resonance Organoboron Delayed Fluorescence Materials through Rational Imine/Amine Functionalization, Angew. Chem., Int. Ed., 2021, 60, 23142–23147 CrossRef CAS PubMed
.
- Y. Qi, W. Ning, Y. Zou, X. Cao, S. Gong and C. Yang, Peripheral Decoration of Multi-Resonance Molecules as a Versatile Approach for Simultaneous Long-Wavelength and Narrowband Emission, Adv. Funct. Mater., 2021, 31, 2102017 CrossRef CAS
.
- Y. Zhang, D. Zhang, T. Huang, A. J. Gillett, Y. Liu, D. Hu, L. Cui, Z. Bin, G. Li, J. Wei and L. Duan, Multi-Resonance Deep-Red Emitters with Shallow Potential-Energy Surfaces to Surpass Energy-Gap Law, Angew. Chem., Int. Ed., 2021, 60, 20498–20503 CrossRef CAS PubMed
.
- Y. Liu, C. Li, Z. Ren, S. Yan and M. R. Bryce, All-organic thermally activated delayed fluorescence materials for organic light-emitting diodes, Nat. Rev. Mater., 2018, 3, 18020 CrossRef CAS
.
- W. Yang, W. Ning, H. Jungchi, T. Liu, X. Yin, C. Ye, S. Gong and C. Yang, Polycyclic phenazine-derived rigid donors construct thermally activated delayed fluorescence emitters for highly efficient orange OLEDs with extremely low roll-off, Chem. Eng. J., 2022, 438, 135571 CrossRef CAS
.
- Y. Xu, Z. Cheng, Z. Li, B. Liang, J. Wang, J. Wei, Z. Zhang and Y. Wang, Molecular-Structure and Device-Configuration Optimizations toward Highly Efficient Green Electroluminescence with Narrowband Emission and High Color Purity, Adv. Optical Mater., 2020, 8, 1902142 CrossRef CAS
.
- Y. Xu, C. Li, Z. Li, Q. Wang, X. Cai, J. Wei and Y. Wang, Constructing Charge-Transfer Excited States Based on Frontier Molecular Orbital Engineering: Narrowband Green Electroluminescence with High Color Purity and Efficiency, Angew. Chem., Int. Ed., 2020, 59, 17442–17446 CrossRef CAS PubMed
.
- J. Bian, S. Chen, L. Qiu, R. Tian, Y. Man, Y. Wang, S. Chen, J. Zhang, C. Duan, C. Han and H. Xu, Ambipolar Self-Host Functionalization Accelerates Blue Multi-Resonance Thermally Activated Delayed Fluorescence with Internal Quantum Efficiency of 100%, Adv. Mater., 2022, 34, 2110547 CrossRef CAS PubMed
.
- J.-W. Huang, Y.-C. Hsu, X. Wu, S. Wang, X.-Q. Gan, W.-Q. Zheng, H. Zhang, Y.-Z. Gong, W.-Y. Hung, P.-T. Chou and W. Zhu, Influence of charge transfer strength on emission bandwidth for multiple-resonance emitters via systematically tuning the acceptor–donor assembly, J. Mater. Chem. C, 2022, 10, 7866–7874 RSC
.
- T. Hua, Y.-C. Liu, C.-W. Huang, N. Li, C. Zhou, Z. Huang, X. Cao, C.-C. Wu and C. Yang, High-efficiency and low roll-off deep-blue OLEDs enabled by thermally activated delayed fluorescence emitter with preferred horizontal dipole orientation, Chem. Eng. J., 2022, 433, 133598 CrossRef CAS
.
- X. Cai, J. Xue, C. Li, B. Liang, A. Ying, Y. Tan, S. Gong and Y. Wang, Achieving 37.1% Green Electroluminescent Efficiency and 0.09 eV Full Width at Half Maximum Based on a Ternary Boron-Oxygen-Nitrogen Embedded Polycyclic Aromatic System, Angew. Chem., Int. Ed., 2022, 61, e202200337 CAS
.
- Q. Zhang, B. Li, S. Huang, H. Nomura, H. Tanaka and C. Adachi, Efficient blue organic light-emitting diodes employing thermally activated delayed fluorescence, Nat. Photonics, 2014, 8, 326–332 CrossRef CAS
.
- D. H. Ahn, S. W. Kim, H. Lee, I. J. Ko, D. Karthik, J. Y. Lee and J. H. Kwon, Highly efficient blue thermally activated delayed fluorescence emitters based on symmetrical and rigid oxygen-bridged boron acceptors, Nat. Photonics, 2019, 13, 540–546 CrossRef CAS
.
- T. Chen, C.-H. Lu, C.-W. Huang, X. Zeng, J. Gao, Z. Chen, Y. Xiang, W. Zeng, Z. Huang, S. Gong, C.-C. Wu and C. Yang, Tuning the emissive characteristics of TADF emitters by fusing heterocycles with acridine as donors: highly efficient orange to red organic light-emitting diodes with EQE over 20%, J. Mater. Chem. C, 2019, 7, 9087–9094 RSC
.
- J. Liang, C. Li, Y. Cui, Z. Li, J. Wang and Y. Wang, Rational design of efficient orange-red to red thermally activated delayed fluorescence emitters for OLEDs with external quantum efficiency of up to 26.0% and reduced efficiency roll-off, J. Mater. Chem. C, 2020, 8, 1614–1622 RSC
.
- H. Liu, J. Li, W.-C. Chen, X. Lv, C. Zhou, C.-S. Lee and C. Yang, Efficient Yellow Thermally Activated Delayed Fluorescent
Emitters Based on 3,5-Dicyanopyridine Acceptors, J. Phys. Chem. C, 2020, 124, 25489–25498 CrossRef CAS
.
- F.-M. Xie, P. Wu, S.-J. Zou, Y.-Q. Li, T. Cheng, M. Xie, J.-X. Tang and X. Zhao, Efficient Orange–Red Delayed Fluorescence Organic Light-Emitting Diodes with External Quantum Efficiency over 26%, Adv. Electron. Mater., 2020, 6, 1900843 CrossRef CAS
.
- D. Karthik, Y. H. Jung, H. Lee, S. Hwang, B.-M. Seo, J.-Y. Kim, C. W. Han and J. H. Kwon, Acceptor–Donor–Acceptor-Type Orange–Red Thermally Activated Delayed Fluorescence Materials Realizing External Quantum Efficiency Over 30% with Low Efficiency Roll-Off, Adv. Mater., 2021, 33, 2007724 CrossRef CAS
.
- S. Wang, Z. Cheng, X. Song, X. Yan, K. Ye, Y. Liu, G. Yang and Y. Wang, Highly Efficient Long-Wavelength Thermally Activated Delayed Fluorescence OLEDs Based on Dicyanopyrazino Phenanthrene Derivatives, ACS Appl. Mater. Interfaces, 2017, 9, 9892–9901 CrossRef CAS PubMed
.
- W. Zeng, H.-Y. Lai, W.-K. Lee, M. Jiao, Y.-J. Shiu, C. Zhong, S. Gong, T. Zhou, G. Xie, M. Sarma, K.-T. Wong, C.-C. Wu and C. Yang, Achieving Nearly 30% External Quantum Efficiency for Orange–Red Organic Light Emitting Diodes by Employing Thermally Activated Delayed Fluorescence Emitters Composed of 1,8-Naphthalimide-Acridine Hybrids, Adv. Mater., 2018, 30, 1704961 CrossRef PubMed
.
- S. Kothavale, W. J. Chung and J. Y. Lee, Rational Molecular Design of Highly Efficient Yellow-Red Thermally Activated Delayed Fluorescent Emitters: A Combined Effect of Auxiliary Fluorine and Rigidified Acceptor Unit, ACS Appl. Mater. Interfaces, 2020, 12, 18730–18738 CrossRef CAS PubMed
.
- H. Wang, B. Zhao, P. Ma, Z. Li, X. Wang, C. Zhao, X. Fan, L. Tao, C. Duan, J. Zhang, C. Han, G. Chen and H. Xu, A red thermally activated delayed fluorescence emitter employing dipyridophenazine with a gradient multi-inductive effect to improve radiation efficiency, J. Mater. Chem. C, 2019, 7, 7525–7530 RSC
.
- Y.-Y. Wang, Y.-L. Zhang, K. Tong, L. Ding, J. Fan and L.-S. Liao, Highly efficient red thermally activated delayed fluorescence materials based on a cyano-containing planar acceptor, J. Mater. Chem. C, 2019, 7, 15301–15307 RSC
.
- J. Fan, J. Miao, N. Li, Y. Zeng, C. Ye, X. Yin and C. Yang, A dual rigid donor and acceptor enabling red thermally activated delayed fluorescence emitters for efficient OLEDs with low efficiency roll-off, J. Mater. Chem. C, 2022, 10, 10255–10261 RSC
.
- K. R. Naveen, S. J. Hwang, H. Lee and J. H. Kwon, Narrow Band Red Emission Fluorophore with Reasonable Multiple Resonance Effect, Adv. Electron. Mater., 2022, 8, 2101114 CrossRef CAS
.
- F.-M. Xie, H.-Z. Li, G.-L. Dai, Y.-Q. Li, T. Cheng, M. Xie, J.-X. Tang and X. Zhao, Rational Molecular Design of Dibenzo[a,c]phenazine-Based Thermally Activated Delayed Fluorescence Emitters for Orange-Red OLEDs with EQE up to 22.0%, ACS Appl. Mater. Interfaces, 2019, 11, 26144–26151 CrossRef CAS PubMed
.
- X. Gong, P. Li, Y.-H. Huang, C.-Y. Wang, C.-H. Lu, W.-K. Lee, C. Zhong, Z. Chen, W. Ning, C.-C. Wu, S. Gong and C. Yang, A Red Thermally Activated Delayed Fluorescence Emitter Simultaneously Having High Photoluminescence Quantum Efficiency and Preferentially Horizontal Emitting Dipole Orientation, Adv. Funct. Mater., 2020, 30, 1908839 CrossRef
.
- J. Li, T. Nakagawa, J. MacDonald, Q. Zhang, H. Nomura, H. Miyazaki and C. Adachi, Highly Efficient Organic Light-Emitting Diode Based on a Hidden Thermally Activated Delayed Fluorescence Channel in a Heptazine
Derivative, Adv. Mater., 2013, 25, 3319–3323 CrossRef CAS
.
- Y. Zou, J. Hu, M. Yu, J. Miao, Z. Xie, Y. Qiu, X. Cao and C. Yang, High-Performance Narrowband Pure-Red OLEDs with External Quantum Efficiencies up to 36.1% and Ultralow Efficiency Roll-Off, Adv. Mater., 2022, 34, 2201442 CrossRef CAS
.
- P. Data, P. Pander, M. Okazaki, Y. Takeda, S. Minakata and A. P. Monkman, Dibenzo[a,j]phenazine-Cored Donor–Acceptor–Donor Compounds as Green-to-Red/NIR Thermally Activated Delayed Fluorescence Organic Light Emitters, Angew. Chem., Int. Ed., 2016, 55, 5739–5744 CrossRef CAS PubMed
.
- J.-X. Chen, K. Wang, C.-J. Zheng, M. Zhang, Y.-Z. Shi, S.-L. Tao, H. Lin, W. Liu, W.-W. Tao, X.-M. Ou and X.-H. Zhang, Red Organic Light-Emitting Diode with External Quantum Efficiency beyond 20% Based on a Novel Thermally Activated Delayed Fluorescence Emitter, Adv. Sci., 2018, 5, 1800436 CrossRef PubMed
.
- Y.-Y. Wang, K.-N. Tong, K. Zhang, C.-H. Lu, X. Chen, J.-X. Liang, C.-K. Wang, C.-C. Wu, M.-K. Fung and J. Fan, Positive impact of chromophore flexibility on the efficiency of red thermally activated delayed fluorescence materials, Mater. Horiz., 2021, 8, 1297–1303 RSC
.
- A. Kumar, H. Y. Shin, T. Lee, J. Jung, B. J. Jung and M. H. Lee, Doubly Boron-Doped TADF Emitters Decorated with ortho-Donor Groups for Highly Efficient Green to Red OLEDs, Chem. – Eur. J., 2020, 26, 16793–16801 CrossRef CAS PubMed
.
- M. Yang, I. S. Park and T. Yasuda, Full-Color, Narrowband, and High-Efficiency Electroluminescence from Boron and Carbazole Embedded Polycyclic Heteroaromatics, J. Am. Chem. Soc., 2020, 142, 19468–19472 CrossRef CAS PubMed
.
- W. Zeng, T. Zhou, W. Ning, C. Zhong, J. He, S. Gong, G. Xie and C. Yang, Realizing 22.5% External Quantum Efficiency for Solution-Processed Thermally Activated Delayed-Fluorescence OLEDs with Red Emission at 622 nm via a Synergistic Strategy of Molecular Engineering and Host Selection, Adv. Mater., 2019, 31, 1901404 CrossRef PubMed
.
- J. Xue, Q. Liang, R. Wang, J. Hou, W. Li, Q. Peng, Z. Shuai and J. Qiao, Highly Efficient Thermally Activated Delayed Fluorescence via J-Aggregates with Strong Intermolecular Charge Transfer, Adv. Mater., 2019, 31, 1808242 CrossRef PubMed
.
- Y. Sun, W. Sun, W. Liu, X. Li, J. Yin and L. Zhou, Efficient Nondoped Pure Red/Near-Infrared TADF OLEDs by Designing and Adjusting Double Quantum Wells Structure, ACS Appl. Electron Mater., 2022, 4, 3615–3622 CrossRef CAS
.
- C. Li, R. Duan, B. Liang, G. Han, S. Wang, K. Ye, Y. Liu, Y. Yi and Y. Wang, Deep-Red to Near-Infrared Thermally Activated Delayed Fluorescence in Organic Solid Films and Electroluminescent Devices, Angew. Chem., Int. Ed., 2017, 56, 11525–11529 CrossRef CAS PubMed
.
- Y.-L. Zhang, Q. Ran, Q. Wang, Y. Liu, C. Hänisch, S. Reineke, J. Fan and L.-S. Liao, High-Efficiency Red Organic Light-Emitting Diodes with External Quantum Efficiency Close to 30% Based on a Novel Thermally Activated Delayed Fluorescence Emitter, Adv. Mater., 2019, 31, 1902368 CrossRef CAS PubMed
.
|
This journal is © the Partner Organisations 2023 |