A dominant contribution to light absorption by methanol-insoluble brown carbon produced in the combustion of biomass fuels typically consumed in wildland fires in the United States†
Received
10th August 2021
, Accepted 21st December 2021
First published on 22nd December 2021
Abstract
The light-absorption properties of brown carbon (BrC) are often estimated using offline, solvent-extraction methods. However, recent studies have found evidence of insoluble BrC species that are unaccounted for in solvent extraction. In this work, we produced carbonaceous aerosol particles from the combustion of three biomass fuels (pine needles, hickory twigs, and oak foliage). We utilized a combination of online and offline measurements and optical calculations to estimate the mass fractions and contribution to light absorption by methanol-soluble BrC (MSBrC), methanol-insoluble BrC (MIBrC), and elemental carbon (EC). Averaged over all experiments, the majority of the carbonaceous aerosol species were attributed to MSBrC (90% ± 5%), while MIBrC and EC constituted 9% ± 5% and 1% ± 0.5%, respectively. The BrC produced in all experiments was moderately absorbing, with an imaginary component of the refractive index (k) at 532 nm ranging between 0.01 and 0.05. However, the k values at 532 nm of the MSBrC (0.004 ± 0.002) and MIBrC (0.211 ± 0.113) fractions were separated by two orders of magnitude, with MSBrC categorized as weakly absorbing BrC and MIBrC as strongly absorbing BrC. Consequently, even though MSBrC constituted the majority of the aerosol mass, MIBrC had a dominant contribution to light absorption at 532 nm (72% ± 11%). The findings presented in this paper provide support for previous reports of the existence of strongly absorbing, methanol-insoluble BrC species and indicate that relying on methanol extraction to characterize BrC in biomass-burning emissions would severely underestimate its absorption.
Environmental significance
Organic aerosol produced from biomass burning is an important player in the radiative balance in the atmosphere. However, there are major challenges associated with characterizing the optical properties of light-absorbing organic aerosol, or brown carbon, which hinder accurate representation of its interaction with solar radiation in climate calculations. Here, we show that conventional techniques that rely on extracting brown carbon in methanol to characterize its optical properties can severely underestimate brown carbon light absorption. Even though the methanol-insoluble fraction constituted less than 10% of the brown carbon mass on average in our experiments, it contributed more than 70% of the total mid-visible light absorption. Accounting for methanol-insoluble brown carbon will enhance the representation of biomass-burning aerosol in climate calculations.
|
Introduction
Combustion sources produce different species of light-absorbing particles that perturb the radiative balance in the atmosphere.1 Black carbon (BC), the most absorbing of those species, is one of the three most potent contributors to radiative forcing, along with carbon dioxide and methane.2 Other light-absorbing species, known as brown carbon (BrC),3,4 absorb light less efficiently than BC, yet exert significant radiative forcing,5–11 with estimates assigning it up to 50% of light absorption at short wavelengths and 25% of total radiative forcing by absorbing particles.5,6
The light-absorption properties of BrC, described by the imaginary component of the refractive index, k, vary greatly.12 Values of k at mid-visible wavelengths of different BrC species have been reported between 10−4 and 10−1, spanning several orders of magnitude.13–18 At the same time, the wavelength dependence of BrC absorption is also highly variable, with stronger wavelength dependence exhibited by the less absorbing BrC.13,14,19–23 While BrC was originally thought to be solely produced by low-temperature, smoldering biomass combustion, more recent works have identified BrC species emitted from higher-temperature biomass combustion13,19–21,24 as well as the combustion of liquid fossil fuels.18,22,25 In addition, the operational definitions of BrC have expanded to include strongly absorbing, non-volatile, and refractory species.12,14,18,26
Thus, the umbrella term BrC covers a range of organic species with widely varying light-absorption and physicochemical properties. This broad range of properties causes a large uncertainty associated with the effect of BrC on the radiative balance in the atmosphere.12 In particular, the majority of climate models that represent BrC absorption use a singular set of parameters (i.e., k values) to represent the various light-absorbing organic species due to the difficulty of including a more comprehensive representation. This can underestimate the direct radiative effect of BrC by skewing towards the less-absorbing species, partly due to a dated understanding of BrC that excludes absorption at longer wavelengths. An effective representation of BrC in climate models must thus reduce the complexity associated with representing thousands of species while, at the same time, effectively capture the relevant light-absorption and physicochemical properties.
In recent years, parameterizations and categorizations have been introduced to facilitate this outcome. Saleh et al. (2014)19 showed that light-absorption properties of BrC emitted from biomass burning can be parameterized as a function of the emissions' relative BC and OA content. Those parameterizations have been implemented in some models, yielding a better agreement between model predictions and observations.8 More recent classifications of BrC have also been proposed based on their physicochemical properties. Corbin et al. (2019)18 divided BrC into soluble BrC and tar BrC, defined by their solubility or insolubility, respectively, in any of the commonly used solvents such as water, methanol, and acetone. Corbin et al.'s categorization of BrC further includes physicochemical properties characteristic of each category, such as light-absorption properties, volatility, and molecular size. Hettiyadura et al. (2021)23 reported the existence of BrC chromophores, containing nonpolar and less-polar PAHs, that were found only in an “oily” fraction of tar condensates. Those oil-specific compounds were overall less volatile and more viscous than other BrC components. Saleh (2020)12 presented a classification based on light-absorption properties, dividing BrC into 4 bins spanning the 4 orders of magnitude covered by mid-visible k values of BrC reported in the literature. Saleh's light-absorption-based BrC classification also highlights that more absorbing BrC species tend to be less volatile and less soluble in water and organic solvents, and to have larger molecular sizes.
The distinction drawn by Corbin et al.18 between soluble and insoluble BrC can be further extended to distinguish soluble species of BrC. Indeed, numerous studies have found that water-soluble BrC is less absorbing than methanol-soluble BrC.27–29 Further, Cheng et al. (2020)17 showed that some BrC produced from the combustion of single-molecule fuels was insoluble in methanol but soluble in dichloromethane (DCM), with the DCM-soluble species being more light absorbing than the methanol-soluble species. Cheng et al.17 also found that a significant fraction of some BrC samples could be insoluble in both. Those insoluble BrC species create a disagreement between the light-absorption properties retrieved via solvent-extraction methods and those retrieved in online measurements. In fact, Shetty et al. (2019)30 found that the light-absorption properties of biomass-burning particles retrieved using solvent-extraction methods and those retrieved from online measurements could differ by up to a factor of 10, with the discrepancy increasing with increasing elemental carbon (EC) content. Higher EC content is correlated with stronger light absorption by biomass-burning BrC,13,19 thus the findings of Shetty et al.30 are consistent with the aforementioned association between light absorption and solubility in water and organic solvents.
In this paper, we present further evidence of biomass-burning BrC that is insoluble in methanol. We use a combination of online and offline measurements to apportion the biomass-burning BrC into methanol-soluble and methanol-insoluble fractions and retrieve the light-absorption properties of each fraction. Doing so, we show that even though the majority of the BrC was methanol-soluble, the light absorption was dominated by the methanol-insoluble BrC.
Methods
Experimental procedure
We burnt dead pine needles, hickory twigs, and dead oak foliage inside a 7.5 m3 environmental chamber. These fuels are commonly consumed in wildfires and prescribed burns in the Southeastern United States.31,32 The fuels were dried inside an oven at 60 °C for 24 hours to reduce their moisture content. Approximately 50 g of each fuel was burned inside an environmental chamber. The fuels were allowed to burn inside the chamber for tens of seconds up to a minute. We then performed online measurements and collected filter samples for offline measurements over a period of several hours.
A scanning mobility particle sizer (SMPS, TSI 3882) continuously measured the particle size distribution in the range of 10–500 nm. We used a photoacoustic spectrometer (Multi-PAS III)33 to measure the absorption coefficient (babs, Mm−1) of the aerosol at 3 wavelengths: 422 nm, 532 nm, and 782 nm. As described in the following sections, these online measurements were used to retrieve the aerosol light-absorption properties.
We collected particles on two filter trains at a flow rate of 5 SLPM for offline analysis. One consisted of a sole 47 mm Quartz (Q) filter (Pall Inc., Tissuquartz 2500), the other of a 47 mm Teflon (PTFE) filter (0.2 microns, Sterlitech Corporation, PTU024750) followed by a Quartz behind Teflon filter (QBT). We targeted a total particle mass loading of 300 μg on the Quartz and Teflon filters, estimated from the sampling flowrate and total particle mass concentration obtained from SMPS measurements. Depending on the particle concentration in the environmental chamber, we collected the filter samples for several hours until the target loading was approximately reached. The Quartz and QBT filters were used to determine the mass fractions of methanol-soluble BrC (MSBrC), methanol-insoluble BrC (MIBrC), and EC, and the PTFE filter was used to determine the light-absorption properties of MSBrC, as elaborated in the subsequent sections.
Mass apportionment
The procedure to apportion the particle mass into fractions of MSBrC, MIBrC, and EC is illustrated in Fig. 1. We immersed a 1.5 cm2 punch of the Quartz filter in 3 ml of methanol for 24 hours in a process of passive extraction, i.e., without sonication. This process minimizes the physical extraction of methanol-insoluble species from the Quartz filter, while also preserving the integrity of the filter for the subsequent analysis.30,34 We performed the extraction in the dark at 4 °C in order to minimize photolysis-induced reactions that could lead to destruction of BrC chromophores35 (photobleaching). After 24 hours, the Quartz punch was removed and dried using a stream of clean, dry air. We then used an organic carbon – elemental carbon (OCEC) analyzer (Sunset Laboratory Inc, Portland, OR, USA, Model 5 L) running the NIOSH-870 protocol (see ESI Table S1†) to determine the residual total carbon (TC) mass on the filter punch after extraction (TCQ,residual). As further elaborated below, TCQ,residual corresponds to TC of the methanol-insoluble species, including both MIBrC and EC: | TCQ,residual = OCMIBrC + EC | (1) |
Here, OCMIBrC and EC were obtained from the OCEC analyzer measurements of the residual carbonaceous material on the Quartz punch after extraction. The OCEC analyzer divides the analyte into OC and EC depending on the temperature and conditions at which they desorb during the analysis. It also identifies pyrolyzed OC, which corresponds to organic species that become pyrolyzed during the initial heating phase, resisting volatilization in the oxygen-deficient phase and appearing instead with the EC.36,37 In eqn (1), OCMIBrC includes both the non-pyrolyzed and the pyrolyzed OC reported by the OCEC analyzer. An implicit assumption in eqn (1) is that all the carbon in MIBrC is detected as OC in the OCEC analyzer. In reality, it is possible that some strongly absorbing, refractory BrC is mistakenly classified as EC by thermal-optical measurements.18,22,38 Thus, OCMIBrC could be underestimated and EC overestimated in the analysis.
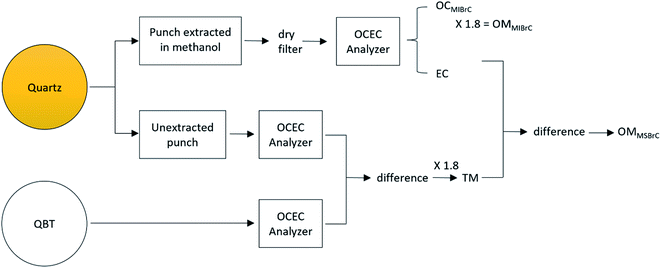 |
| Fig. 1 Flowchart summarizing the procedure for apportioning the carbonaceous particle mass into methanol-soluble BrC (MSBrC), methanol-insoluble BrC (MIBrC), and EC (see eqn (1)–(5)). | |
In order to determine the methanol-soluble OC (OCMSBrC) fraction, we used the same OCEC analysis procedure to determine the TC mass on an unextracted Quartz filter punch (TCQ) and on a QBT filter punch (TCQBT). Since the QBT filter only collected adsorbed vapor species, the difference between TCQ and TCQBT corresponds to the TC in the particle phase,39 including MSBrC, MIBrC, and EC:
| TCQ − TCQBT = OCMSBrC + OCMIBrC + EC | (2) |
Then, OCMSBrC can be obtained from eqn (1) and (2) as:
| OCMSBrC = (TCQ − TCQBT) − TCQ,residual | (3) |
We converted OCMSBrC and OCMIBrC to organic-mass basis (OMMSBrC and OMMIBrC) assuming OM/OC of 1.8, which is typical for biomass-combustion emissions.40–42 As shown in Table S2 in the ESI,† assuming OM/OC of 1.5–2 did not have a significant effect on the retrieved light-absorption properties. The fractions of MSBrC, MIBrC, and EC in the particles were then obtained as:
| fMSBrC = OMMSBrC/TM; fMIBrC = OMMIBrC/TM; fEC = EC/TM | (4) |
Where TM is the total mass of carbonaceous species:
| TM = OMMSBrC + OMMIBrC + EC | (5) |
Retrieving aerosol light-absorption properties
We retrieved the imaginary component of the refractive index of the BrC aerosol (kBrC,aerosol) at 422 nm and 532 nm using optical closure.13,19,43,44 In brief, we used Mie calculations to constrain the kBrC,aerosol that, coupled with measured particle size distributions, best reproduced the measured babs at that wavelength. We calculated the wavelength dependence, wBrC,aerosol, assuming that kBrC,aerosol exhibits a power-law dependence on wavelength: | wBrC,aerosol = log(k422,BrC,aerosol/k532,BrC,aerosol)/log(532/422) | (6) |
The Mie calculations assumed a BrC real component of the refractive index equal to 1.6.12 To account for absorption by EC, we applied several assumptions. First, we assumed that EC and BrC were externally mixed. We also assumed that the EC particles were spherical (which is inherent in Mie calculations) and that their size distribution had the same shape as that of BrC. Therefore, the size distributions measured by the SMPS were split between EC and BrC based on the EC/OM values obtained from the OCEC analyzer measurements. Finally, we used an EC complex refractive index of 1.85 + 0.71i.45 We note that because the EC fraction was small in these experiments (EC/OC < 0.05), these simplifying assumptions had a small effect on the retrieved kBrC,aerosol as discussed in Section S2 in the ESI.†
Light-absorption apportionment
As summarized in Fig. 2, we employed a combination of online and offline measurements and Mie calculations to retrieve the imaginary component of the refractive indices of methanol-soluble BrC (kMSBrC) and methanol-insoluble BrC (kMIBrC). First, we used the particles collected on the PTFE filters to determine the light-absorption properties of MSBrC following the procedure of Cheng et al. (2020).17 For the extraction of the PTFE filters, we immersed each filter in 5 ml of methanol inside a glass vial and sonicated for 15 minutes. In preliminary experiments, we confirmed that sonication for longer times (up to 30 minutes) had no observable effect on extraction efficiency. Unlike with the passive extraction used with the Quartz filters, sonication can physically extract some of the methanol-insoluble species. To remove the methanol-insoluble particles from the methanol solution, we filtered the methanol extracts through 13 mm PTFE (0.2 microns, Sterlitech Corporation, PTU021350) using a glass vial with a metal luer lock tip.
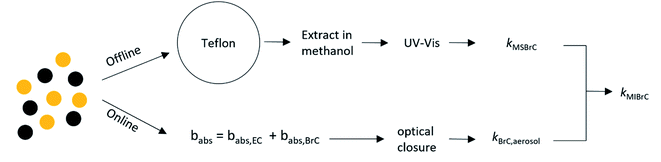 |
| Fig. 2 Flowchart showing the light-absorption apportionment procedure (see eqn (7)–(9)). | |
We measured the MSBrC concentration in the solutions using the OCEC analyzer. To do so, we pipetted 300 μl onto 1.5 cm2 punches of prebaked Quartz filters and dried the filters using a stream of clean, dry air. Because methanol is relatively volatile, it evaporates rapidly under the stream of air, leaving behind the BrC. We then retrieved the total carbon mass on the punch running the NIOSH-870 protocol on the OCEC analyzer. We used the measured mass to estimate the BrC concentration in the solutions, CMSBrC. As before, we assumed OM/OC = 1.8.
We used a UV-vis Spectrophotometer (Agilent, Cary 60) to measure the UV-vis absorbance of the extracts in the 200–800 nm range at a 1 nm resolution. We then converted the measured absorbance to light-absorption properties of MSBrC using the relation between kMSBrC and the absorption coefficient (α, cm−1):
Here,
α is calculated from the UV-vis measurements using
| α(λ) = ln(10)A(λ)ρ/(CMSBrCL) | (8) |
where
A is the measured absorbance,
ρ is the density of the extracts (assumed to be 1.2 g cm
−3),
L (1 cm) is the optical path length, and
CMSBrC is the concentration of MSBrC in the solution. Although the absorption coefficients
α and
babs have similar units (length
−1), they express different physical quantities.
babs represents the total absorption cross section of the aerosol per unit volume of air and thus depends on the aerosol concentration and size distribution, whereas
α is a material property that is directly related to
k (
eqn (7)).
We retrieved kMIBrC based on the assumption that MIBrC and MSBrC were well-mixed in the BrC aerosol and that kBrC,aerosol is a volume-weighted average of kMSBrC and kMIBrC. Therefore:
| kMIBrC = (kBrC,aerosol − kMSBrCfMSBrC/(fMSBrC + fMIBrC)) × (fMSBrC + fMIBrC)/fMIBrC | (9) |
where,
kMSBrC is obtained from the UV-vis measurements,
kBrC,aerosol is obtained from the optical closure analysis, and the fractions (
fMSBrC and
fMIBrC) are obtained from the mass apportionment analysis.
We also quantified the fractional contribution to light absorption by MSBrC, MIBrC, and EC. The fractional contribution by EC was calculated as:
| Xabs,EC = babs,EC/babs | (10) |
where
babs,EC is the absorption coefficient of the EC particles, obtained using Mie calculations and
babs is the total aerosol absorption coefficient measured using the Multi-PAS III. The contributions to absorption by MSBrC and MIBrC were then calculated as:
| Xabs,MSBrC = (1 − Xabs,EC)(kMSBrCfMSBrC/(fMSBrC + fMIBrC))/kBrC,aerosol | (11) |
| Xabs,MIBrC = (1 − Xabs,EC)(kMIBrCfMIBrC/(fMSBrC + fMIBrC))/kBrC,aerosol | (12) |
The uncertainty associated with these calculations is discussed in Section S1 in the ESI.†
Results
Brown carbon aerosol light-absorption properties
Fig. 3 shows the light-absorption properties (k550 and w) of the BrC aerosol plotted against the EC/OM ratios retrieved from the OCEC analyzer. We note that here we use the term ‘BrC aerosol’ to refer to the whole BrC and to indicate that its light-absorption properties were obtained from online measurements in the aerosol phase followed by the subtraction of EC absorption, as described earlier. The individual data points shown correspond to the combustion experiments we conducted with each of the three fuels. On the same figures, we show the parameterizations of k550 and w versus EC/OM (or, equivalently, BC/OA) derived by Saleh et al. (2014),19 based on both internally mixed and externally mixed BC assumptions. For both k550 and w, our data agree with the trends of correlation between the light-absorption properties and EC/OM, with k550 increasing and w decreasing with increasing EC content. The inverse relation between k and w has also been repeatedly established previously for BrC.13,21,46 Notably, the data points from the different combustion experiments follow a similar trend, with no apparent dependence on fuel type. This indicates that the difference in the light-absorption properties of BrC produced in different combustion scenarios is primarily dictated by the different combustion conditions rather than fuel type.14
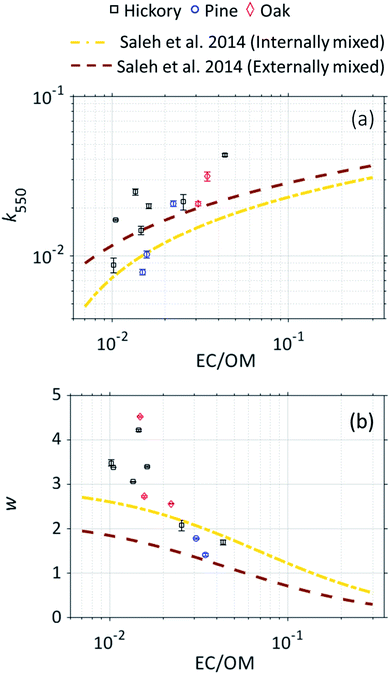 |
| Fig. 3 Light-absorption properties of the BrC aerosol, retrieved using optical closure, as a function of the EC/OM ratio, retrieved from the OCEC analyzer assuming OM/OC = 1.8. (a) The imaginary component of the refractive index at 550 nm (k550) versus EC/OM. (b) The wavelength dependence of the imaginary component of the refractive index (w) versus EC/OM. Also shown are the parameterizations of Saleh et al. (2014)19 with the assumption of internally mixed and externally mixed BC. Error bars represent uncertainty, calculated as described in the ESI.† | |
Both k550 and w values obtained in this study are generally larger than those predicted by the Saleh et al.19 parameterizations. This could be due to true variability, but is also likely due to discrepancies in aerosol light-absorption measurements between the two studies. BrC parameterizations derived from biomass-burning measurements usually involve significant spread in the data points,13,19,47 and while they usually exhibit similar trends, there are large differences between them.12 Because of the relatively small number of data points and limited range of EC/OM in this study, we elect not to report a mathematical fit.
Light-absorption properties of the methanol-soluble and methanol-insoluble brown carbon
Fig. 4 shows the imaginary component of the refractive indices of the MSBrC and MIBrC fractions (kMSBrC and kMIBrC) at 422 nm and 532 nm, plotted against kBrC,aerosol. The figure shows that kMSBrC and kMIBrC are clustered in different ranges. kMSBrC,422 and kMSBrC,532 had average values of 0.015 ± 0.003 and 0.004 ± 0.002, respectively, while kMIBrC,422 and kMIBrC,532 had average values of 0.308 ± 0.161 and 0.211 ± 0.113, respectively. At both wavelengths, MSBrC had a smaller k than the BrC aerosol, while MIBrC had a larger k than the BrC aerosol. In-line with previous reports,17,18,25,30 an important implication of these findings is that relying on methanol extraction can severely underestimate BrC absorption. Furthermore, as kBrC,aerosol increases, kMSBrC is relatively capped, which further indicates that methanol extraction becomes less effective in capturing the light-absorption properties of the BrC particles as a whole as they become more absorbing.17
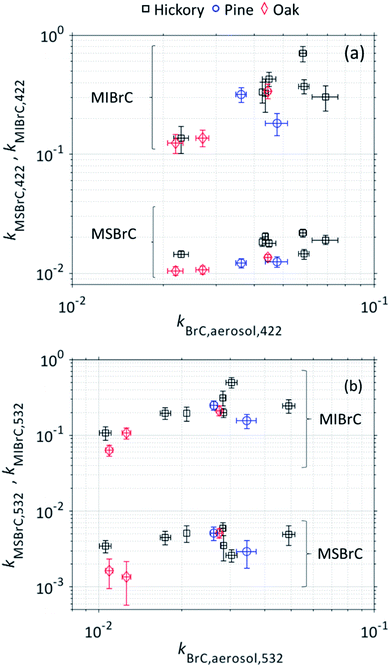 |
| Fig. 4 The imaginary component of the refractive index (k) for the methanol-soluble BrC (MSBrC) and the methanol-insoluble BrC (MIBrC), retrieved from UV-Vis measurements and optical closure, respectively, plotted against k of the BrC aerosol at (a) λ = 422 nm and (b) λ = 532 nm. Error bars represent uncertainty, calculated as described in the ESI.† Numerical values of each data point are given in Table S2 in the ESI.† | |
In Fig. 5, we show the light-absorption properties (k550vs. w) of the BrC aerosol and the MSBrC and MIBrC fractions retrieved in this study. In the backdrop, we show the BrC categories proposed by Saleh (2020)12 along with literature values of biomass-burning BrC k550vs. w retrieved based on methanol extraction (i.e., equivalent to MSBrC in this study). The BrC aerosol produced in this study falls within the moderately absorbing BrC category (M-BrC). However, the fractions that compose it, namely MSBrC and MIBrC, are divided between the weakly absorbing BrC category (W-BrC) and the strongly absorbing category (S-BrC), respectively. The mean MIBrC k550 from all experiments is 2 orders of magnitude larger than MSBrC k550 (kMIBrC,550 = 0.211 ± 0.113; kMSBrC,550 = 0.004 ± 0.002), while MSBrC exhibited a much stronger wavelength dependence (wMIBrC = 1.7 ± 1.1; wMSBrC = 6.3 ± 1.7). The light-absorption properties of MSBrC obtained from our experiments are consistent with those reported for biomass-burning BrC in other works that relied on methanol extraction,21,29,48–51 all of which fall within W-BrC. Those studies investigated emissions from a wide range of biomass fuels, including wood for residential heating/cooking,21 agricultural waste48 (corn stalk), as well as ambient aerosol with strong contributions from residential and agricultural burning.49–51 This indicates that our finding that MSBrC falls within the W-BrC category extends beyond the fuel types investigated in our experiments. Furthermore, previous studies retrieved BrC light-absorption properties from measurements in the aerosol phase, including in emissions from residential wood burning20 and agricultural burning19 (rice straw, hay). These studies reported kBrC values that fall within the M-BrC category,12 and are typically larger than the values from the aforementioned studies that relied on methanol extraction. This suggests that MIBrC is possibly important in emissions from these fuel types.
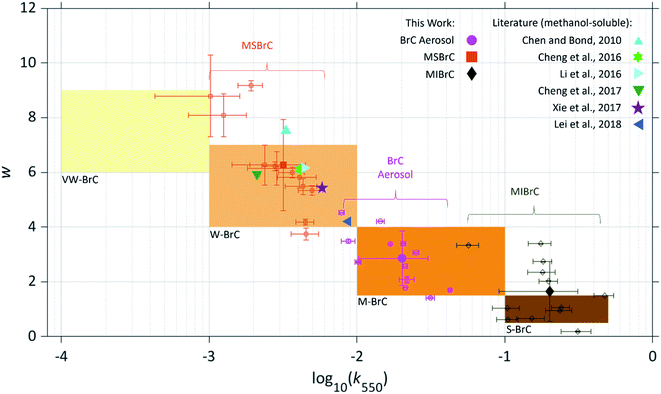 |
| Fig. 5 Light-absorption properties of the BrC aerosol and the MSBrC and MIBrC fractions produced in this work, shown in log10(k550) – w space. The shaded rectangles represent the BrC categories suggested by Saleh (2020).12 Open circles, squares, and rhombi represent individual data points from each experiments and filled markers represent the average values retrieved for the categories of BrC aerosol, MSBrC, and MIBrC. To avoid cluttering, we did not include different markers for each fuel type in this figure. The figure also includes the average values of biomass-burning k550vs. w reported in or calculated from previous studies that utilized methanol extraction.24,29,48–51 Error bars represent uncertainty, calculated as described in the ESI.† | |
The stronger light absorption of MIBrC compared to MSBrC reported here and in other works17,18,25 confirms that MSBrC cannot be used to represent the light-absorption properties of BrC aerosols as a whole. The methanol-insoluble fraction must be accounted for in order to arrive at an accurate representation of absorption by BrC. The light-absorption properties of the MIBrC in our experiments span a similar range to that suggested by Corbin et al. (2019)18 for marine-engine exhaust, as well as other reports of strongly absorbing BrC that have been referred to using different terminologies, including refractory BrC,14 intermediate absorber (between BrC and BC),52 BrC associated with extremely low volatility organic compounds (ELVOCs),19 and brown carbon spheres.53
Mass fractions and contribution to absorption
Fig. 6 shows the mass fractions of MSBrC (fMSBrC), MIBrC (fMIBrC), and EC (fEC) in the carbonaceous aerosol, averaged over all the combustion experiments. MSBrC constituted by far the largest fraction (90% ± 5%), while MIBrC and EC constituted 9% ± 5% and 1% ± 0.5%, respectively. This is consistent with previous studies that have reported methanol extraction efficiencies of >90%.29,48 Indeed, these high extraction efficiencies by methanol have led those studies to assume that methanol effectively extracts all the organics in biomass-burning particle emissions. While this assumption is justified when the purpose is to study the chemical composition of the OA (e.g., to investigate OA formation pathways in biomass burning), it is not when the purpose is to quantify BrC light absorption.
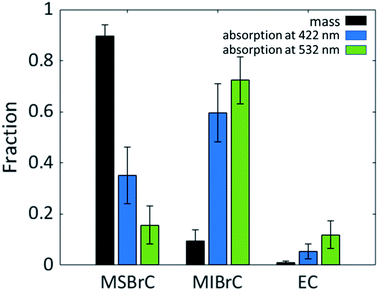 |
| Fig. 6 Mass fractions of MSBrC, MIBrC, and EC averaged over all combustion experiments and their corresponding fractional contribution to total aerosol absorption at 422 nm and 532 nm. Error bars represent uncertainty, calculated as described in the ESI.† | |
Also shown in Fig. 6 are the estimated contributions to absorption by each of the MSBrC, MIBrC, and EC fractions, averaged over all experiments. Despite constituting the majority of the particles by mass, the MSBrC contributed 35% ± 11% and 16% ± 7% of the total absorption at 422 nm and 532 nm, respectively. In contrast, the MIBrC contributed 60% ± 11% and 72% ± 10% at 422 nm and 532 nm, respectively, and the EC fraction contributed 5% ± 3% and 12% ± 5% at 422 nm and 532 nm, respectively. It is worth noting that the relative differences between the contributions to absorption at 422 nm and 532 nm between MSBrC, MIBrC, and EC is a reflection of the differences in the wavelength dependence of their absorption. As shown in Fig. 5, wMSBrC and wMIBrC were 6.3 ± 1.7 and 1.7 ± 1.1, respectively, while wEC was assumed to be zero.
Due to the limited number of experiments in this study, we were not able to investigate the source of variability in the relative abundance of MSBrC and MIBrC across experiments. Following from previous reports on BrC absorption being correlated with combustion conditions,13,14,19 we expect that combustion conditions will also dictate the relative abundance of MSBrC and MIBrC. Specifically, we expect that the MIBrC fraction would be relatively small in smoldering combustion and would increase as combustion conditions become more flaming, leading to an overall increase in BrC absorption.
Importantly, even though MIBrC constitutes an order-of-magnitude smaller fraction of the carbonaceous aerosol than MSBrC, it contributes a dominant fraction of the total absorption. These findings are consistent with previous reports of a dominant contribution to absorption by insoluble BrC produced from heavy fuel oil combustion18,25 and indicate that methanol-extraction techniques are inadequate at quantifying light absorption by biomass-burning BrC. In addition to its association with differences in light-absorption properties, solubility in methanol is also expected to be associated with other physicochemical properties, including volatility and molecular size.14,18 Furthermore, larger molecular size BrC species have been shown to be more resistant to decay in absorption due to photobleaching upon aging in the atmosphere.54 Consequently, in addition to MIBrC being more light-absorbing than MSBrC, it is also expected to be less volatile, possibly less susceptible to photobleaching, and therefore have a longer lifetime in the atmosphere.
Conclusions
In this work, we report the existence of a methanol-insoluble BrC (MIBrC) fraction produced in biomass combustion that is significantly more light absorbing than the methanol-soluble BrC (MSBrC) fraction. These findings contribute to the growing body of literature on the association between solubility and the light-absorption properties of BrC produced in biomass combustion,30 as well as controlled combustion of single-molecule fuels17 and marine engines.18,25 In concordance with previous studies,29,48 methanol was efficient at extracting the organic matter produced in our biomass-burning experiment, where MSBrC constituted 90% ± 5% of the total carbonaceous species. However, considering this high methanol extraction efficiency as an indication that MSBrC is representative of the overall BrC is misleading. Our results show that relying on methanol extraction to constrain the light-absorption properties of biomass-burning BrC results in a severe misrepresentation of these properties, leading to an order-of-magnitude underestimation of BrC light absorption at mid-visible wavelengths.
Author contributions
Khairallah Atwi: methodology, formal analysis, investigation, data curation, validation, visualization, writing – original draft, writing – review & editing. Zezhen Cheng: methodology, writing – original draft. Omar El Hajj: investigation, writing – original draft. Charles Perrie: investigation, writing – original draft. Rawad Saleh: conceptualization, methodology, writing – original draft, writing – review & editing, supervision, project administration, funding acquisition.
Conflicts of interest
There are no conflicts to declare.
Acknowledgements
Financial support was provided by the National Science Foundation, Division of Atmospheric and Geospace Sciences (AGS-1748080).
Notes and references
- T. C. Bond, S. J. Doherty, D. Fahey, P. Forster, T. Berntsen, B. DeAngelo, M. Flanner, S. Ghan, B. Kärcher and D. Koch, Bounding the role of black carbon in the climate system: A scientific assessment, J. Geophys. Res.: Atmos., 2013, 118, 5380–5552 CAS.
-
R. K. Pachauri, M. R. Allen, V. R. Barros, J. Broome, W. Cramer, R. Christ, J. A. Church, L. Clarke, Q. Dahe and P. Dasgupta, Climate Change 2014: Synthesis Report. Contribution of Working Groups I, II and III to the Fifth Assessment Report of the Intergovernmental Panel on Climate Change, IPCC, 2014 Search PubMed.
- A. Laskin, J. Laskin and S. A. Nizkorodov, Chemistry of atmospheric brown carbon, Chem. Rev., 2015, 115, 4335–4382 CrossRef CAS PubMed.
- M. Andreae and A. Gelencsér, Black carbon or brown carbon? The nature of light-absorbing carbonaceous aerosols, Atmos. Chem. Phys., 2006, 6, 3131–3148 CrossRef CAS.
- Y. Zhang, H. Forrister, J. Liu, J. Dibb, B. Anderson, J. P. Schwarz, A. E. Perring, J. L. Jimenez, P. Campuzano-Jost and Y. Wang, Top-of-atmosphere radiative forcing affected by brown carbon in the upper troposphere, Nat. Geosci., 2017, 10, 486–489 CrossRef CAS.
-
X. Wang, C. Heald, D. Ridley, J. Schwarz, J. Spackman, A. Perring, H. Coe, D. Liu and A. Clarke, Exploiting Simultaneous Observational Constraints on Mass and Absorption to Estimate the Global Direct Radiative Forcing of Black Carbon and Brown Carbon, 2014 Search PubMed.
- Y. Feng, V. Ramanathan and V. R. Kotamarthi, Brown carbon: a significant atmospheric absorber of solar radiation?, Atmos. Chem. Phys., 2013, 13, 8607–8621 CrossRef.
-
H. Brown, X. Liu, Y. Feng, Y. Jiang, M. Wu, Z. Lu, C. Wu, S. Murphy and R. Pokhrel, Radiative Effect and Climate Impacts of Brown Carbon with the Community Atmosphere Model (CAM5), Report 1680-7375, Lawrence Berkeley National Laboratory (LBNL), Berkeley, CA (United States), 2018 Search PubMed.
- R. Saleh, M. Marks, J. Heo, P. J. Adams, N. M. Donahue and A. L. Robinson, Contribution of brown carbon and lensing to the direct radiative effect of carbonaceous aerosols from biomass and biofuel burning emissions, J. Geophys. Res.: Atmos., 2015, 120, 10285–10296 Search PubMed.
- R. J. Park, M. J. Kim, J. I. Jeong, D. Youn and S. Kim, A contribution of brown carbon aerosol to the aerosol light absorption and its radiative forcing in East Asia, Atmos. Environ., 2010, 44, 1414–1421 CrossRef CAS.
- M. Z. Jacobson, Effects of biomass burning on climate, accounting for heat and moisture fluxes, black and brown carbon, and cloud absorption effects, J. Geophys. Res.: Atmos., 2014, 119, 8980–9002 CAS.
- R. Saleh, From Measurements to Models: Toward Accurate Representation of Brown Carbon in Climate Calculations, Curr. Pollut. Rep., 2020, 1–15 Search PubMed.
- C. D. McClure, C. Y. Lim, D. H. Hagan, J. H. Kroll and C. D. Cappa, Biomass-burning-derived particles from a wide variety of fuels–Part 1: Properties of primary particles, Atmos. Chem. Phys., 2020, 20, 1531–1547 CrossRef CAS.
- R. Saleh, Z. Cheng and K. Atwi, The brown–black continuum of light-absorbing combustion aerosols, Environ. Sci. Technol. Lett., 2018, 5, 508–513 CrossRef CAS.
- A. T. Lambe, C. D. Cappa, P. Massoli, T. B. Onasch, S. D. Forestieri, A. T. Martin, M. J. Cummings, D. R. Croasdale, W. H. Brune and D. R. Worsnop, Relationship between oxidation level and optical properties of secondary organic aerosol, Environ. Sci. Technol., 2013, 47, 6349–6357 CrossRef CAS PubMed.
- K. M. Updyke, T. B. Nguyen and S. A. Nizkorodov, Formation of brown carbon via reactions of ammonia with secondary organic aerosols from biogenic and anthropogenic precursors, Atmos. Environ., 2012, 63, 22–31 CrossRef CAS.
- Z. Cheng, K. Atwi, O. E. Hajj, I. Ijeli, D. A. Fischer, G. Smith and R. Saleh, Discrepancies Between Brown Carbon Light-absorption Properties Retrieved from Online and Offline Measurements, Aerosol Sci. Technol., 2020, 1–15 Search PubMed.
- J. C. Corbin, H. Czech, D. Massabò, F. B. de Mongeot, G. Jakobi, F. Liu, P. Lobo, C. Mennucci, A. A. Mensah and J. Orasche, Infrared-absorbing carbonaceous tar can dominate light absorption by marine-engine exhaust, npj Clim. Atmos. Sci., 2019, 2, 1–10 CrossRef CAS.
- R. Saleh, E. S. Robinson, D. S. Tkacik, A. T. Ahern, S. Liu, A. C. Aiken, R. C. Sullivan, A. A. Presto, M. K. Dubey and R. J. Yokelson, Brownness of organics in aerosols from biomass burning linked to their black carbon content, Nat. Geosci., 2014, 7, 647 CrossRef CAS.
- N. K. Kumar, J. C. Corbin, E. A. Bruns, D. Massabó, J. G. Slowik, L. Drinovec, G. Močnik, P. Prati, A. Vlachou and U. Baltensperger, Production of particulate brown carbon during atmospheric aging of residential wood-burning emissions, Atmos. Chem. Phys., 2018, 18, 17843–17861 CrossRef CAS.
- M. Xie, G. Shen, A. L. Holder, M. D. Hays and J. J. Jetter, Light absorption of organic carbon emitted from burning wood, charcoal, and kerosene in household cookstoves, Environ. Pollut., 2018, 240, 60–67 CrossRef CAS PubMed.
- Z. Z. Cheng, K. Atwi, T. Onyima and R. Saleh, Investigating the dependence of light-absorption properties of combustion carbonaceous aerosols on combustion conditions, Aerosol Sci. Technol., 2019, 53, 419–434 CrossRef CAS.
- A. P. S. Hettiyadura, V. Garcia, C. Li, C. P. West, J. Tomlin, Q. He, Y. Rudich and A. Laskin, Chemical Composition and Molecular-Specific Optical Properties of Atmospheric Brown Carbon Associated with Biomass Burning, Environ. Sci. Technol., 2021, 55, 2511–2521 CrossRef CAS PubMed.
- M. Xie, M. D. Hays and A. L. Holder, Light-absorbing organic carbon from prescribed and laboratory biomass burning and gasoline vehicle emissions, Sci. Rep., 2017, 7, 1–9 CrossRef PubMed.
- Z. Bai, L. Zhang, Y. Cheng, W. Zhang, J. Mao, H. Chen, L. Li, L. Wang and J. Chen, Water/Methanol-Insoluble Brown Carbon Can Dominate Aerosol-Enhanced Light Absorption in Port Cities, Environ. Sci. Technol., 2020, 54, 14889–14898 CrossRef CAS PubMed.
- N. Shetty, P. Beeler, T. Paik, F. J. Brechtel and R. K. Chakrabarty, Bias in Quantification of Light Absorption Enhancement of Black Carbon Aerosol Coated with Low Volatility Brown Carbon, Aerosol Sci. Technol., 2021, 1–16 Search PubMed.
- R.-J. Huang, L. Yang, J. Shen, W. Yuan, Y. Gong, J. Guo, W. Cao, J. Duan, H. Ni and C. Zhu, Water-Insoluble Organics Dominate Brown Carbon in Wintertime Urban Aerosol of China: Chemical Characteristics and Optical Properties, Environ. Sci. Technol., 2020, 54, 7836–7847 CrossRef CAS PubMed.
- R. Satish and N. Rastogi, On the use of brown carbon spectra as a tool to understand their broader composition and characteristics: a case study from crop-residue burning samples, ACS Omega, 2019, 4, 1847–1853 CrossRef CAS PubMed.
- Y. Chen and T. Bond, Light absorption by organic carbon from wood combustion, Atmos. Chem. Phys., 2010, 10, 1773–1787 CrossRef CAS.
- N. J. Shetty, A. Pandey, S. Baker, W. M. Hao and R. K. Chakrabarty, Measuring light absorption by freshly
emitted organic aerosols: optical artifacts in traditional solvent-extraction-based methods, Atmos. Chem. Phys., 2019, 19, 8817–8830 CrossRef CAS.
- M. Zheng, G. R. Cass, J. J. Schauer and E. S. Edgerton, Source apportionment of PM2. 5 in the southeastern United States using solvent-extractable organic compounds as tracers, Environ. Sci. Technol., 2002, 36, 2361–2371 CrossRef CAS PubMed.
- P. M. Fine, G. R. Cass and B. R. Simoneit, Chemical characterization of fine particle emissions from the fireplace combustion of woods grown in the southern United States, Environ. Sci. Technol., 2002, 36, 1442–1451 CrossRef CAS PubMed.
- D. A. Fischer and G. D. Smith, A portable, four-wavelength, single-cell photoacoustic spectrometer for ambient aerosol absorption, Aerosol Sci. Technol., 2018, 52, 393–406 CrossRef CAS.
- S. M. Phillips and G. D. Smith, Spectroscopic comparison of water-and methanol-soluble brown carbon particulate matter, Aerosol Sci. Technol., 2017, 51, 1113–1121 CrossRef CAS.
- J. P. Wong, M. Tsagkaraki, I. Tsiodra, N. Mihalopoulos, K. Violaki, M. Kanakidou, J. Sciare, A. Nenes and R. J. Weber, Atmospheric evolution of molecular-weight-separated brown carbon from biomass burning, Atmos. Chem. Phys., 2019, 19, 7319–7334 CrossRef CAS.
- C. Wu, X. Huang, W. M. Ng, S. M. Griffith and J. Z. Yu, Inter-comparison of NIOSH and IMPROVE protocols for OC and EC determination: implications for inter-protocol data conversion, Atmos. Meas. Tech., 2016, 9, 4547–4560 CrossRef CAS.
- B. Khan, M. D. Hays, C. Geron and J. Jetter, Differences in the OC/EC ratios that characterize ambient and source aerosols due to thermal-optical analysis, Aerosol Sci. Technol., 2012, 46, 127–137 CrossRef CAS.
- J. C. Corbin and M. Gysel-Beer, Detection of tar brown carbon with a single particle soot photometer (SP2), Atmos. Chem. Phys., 2019, 19, 15673–15690 CrossRef CAS.
- R. Subramanian, A. Y. Khlystov, J. C. Cabada and A. L. Robinson, Positive and negative artifacts in particulate organic carbon measurements with denuded and undenuded sampler configurations special issue of aerosol science and technology on findings from the fine particulate matter supersites program, Aerosol Sci. Technol., 2004, 38, 27–48 CrossRef CAS.
- H. S. El-Zanan, D. H. Lowenthal, B. Zielinska, J. C. Chow and N. Kumar, Determination of the organic aerosol mass to organic carbon ratio in IMPROVE samples, Chemosphere, 2005, 60, 485–496 CrossRef CAS PubMed.
- A. C. Aiken, P. F. Decarlo, J. H. Kroll, D. R. Worsnop, J. A. Huffman, K. S. Docherty, I. M. Ulbrich, C. Mohr, J. R. Kimmel and D. Sueper, O/C and OM/OC ratios of primary, secondary, and ambient organic aerosols with high-resolution time-of-flight aerosol mass spectrometry, Environ. Sci. Technol., 2008, 42, 4478–4485 CrossRef CAS PubMed.
- L. Yao, L. Yang, J. Chen, X. Wang, L. Xue, W. Li, X. Sui, L. Wen, J. Chi and Y. Zhu, Characteristics of carbonaceous aerosols: Impact of biomass burning and secondary formation in summertime in a rural area of the North China Plain, Sci. Total Environ., 2016, 557, 520 CrossRef PubMed.
- R. Chakrabarty, H. Moosmuller, L.-W. Chen, K. Lewis, W. Arnott, C. Mazzoleni, M. Dubey, C. Wold, W. Hao and S. Kreidenweis, Brown carbon in tar balls from smoldering biomass combustion, Atmos. Chem. Phys., 2010, 10, 6363–6370 CrossRef CAS.
-
D. A. Lack, J. M. Langridge, R. Bahreini, C. D. Cappa, A. M. Middlebrook and J. P. Schwarz, Brown Carbon and Internal Mixing in Biomass Burning Particles, Proceedings of the National Academy of Sciences, 2012 Search PubMed.
- T. C. Bond and R. W. Bergstrom, Light absorption by carbonaceous particles: An investigative review, Aerosol Sci. Technol., 2006, 40, 27–67 CrossRef CAS.
- D. Sengupta, V. Samburova, C. Bhattarai, E. Kirillova, L. Mazzoleni, M. Iaukea-Lum, A. Watts, H. Moosmüller and A. Khlystov, Light absorption by polar and non-polar aerosol compounds from laboratory biomass combustion, Atmos. Chem. Phys., 2018, 18, 10849–10867 CrossRef CAS.
- Z. Lu, D. G. Streets, E. Winijkul, F. Yan, Y. Chen, T. C. Bond, Y. Feng, M. K. Dubey, S. Liu and J. P. Pinto, Light absorption properties and radiative effects of primary organic aerosol emissions, Environ. Sci. Technol., 2015, 49, 4868–4877 CrossRef CAS PubMed.
- X. Li, Y. Chen and T. C. Bond, Light absorption of organic aerosol from pyrolysis of corn stalk, Atmos. Environ., 2016, 144, 249–256 CrossRef CAS.
- Y. Cheng, K.-b. He, Z.-y. Du, G. Engling, J.-m. Liu, Y.-l. Ma, M. Zheng and R. J. Weber, The characteristics of brown carbon aerosol during winter in Beijing, Atmos. Environ., 2016, 127, 355–364 CrossRef CAS.
- Y. Cheng, K.-b. He, G. Engling, R. Weber, J.-m. Liu, Z.-y. Du and S.-p. Dong, Brown and black carbon in Beijing aerosol: Implications for the effects of brown coating on light absorption by black carbon, Sci. Total Environ., 2017, 599, 1047–1055 CrossRef PubMed.
- Y. Lei, Z. Shen, Q. Wang, T. Zhang, J. Cao, J. Sun, Q. Zhang, L. Wang, H. Xu and J. Tian, Optical characteristics and source apportionment of brown carbon in winter PM2. 5 over Yulin in Northern China, Atmos. Res., 2018, 213, 27–33 CrossRef CAS.
- G. Adler, N. L. Wagner, K. D. Lamb, K. M. Manfred, J. P. Schwarz, A. Franchin, A. M. Middlebrook, R. A. Washenfelder, C. C. Womack and R. J. Yokelson, Evidence in biomass burning smoke for a light-absorbing aerosol with properties intermediate between brown and black carbon, Aerosol Sci. Technol., 2019, 1–33 Search PubMed.
- D. T. Alexander, P. A. Crozier and J. R. Anderson, Brown carbon spheres in East Asian outflow and their optical properties, Science, 2008, 321, 833–836 CrossRef CAS PubMed.
- R. Stevens and A. Dastoor, A review of the representation of aerosol mixing state in atmospheric models, Atmosphere, 2019, 10, 168 CrossRef CAS.
Footnotes |
† Electronic supplementary information (ESI) available. See DOI: 10.1039/d1ea00065a |
‡ Current address: Pacific Northwest National Laboratory, Richland, WA, USA. |
|
This journal is © The Royal Society of Chemistry 2022 |