DOI:
10.1039/C4RA11773E
(Paper)
RSC Adv., 2015,
5, 248-254
Natural peptide anti-glycation effect in the presence of Aloe vera phenolic components on human serum albumin
Received
4th October 2014
, Accepted 12th November 2014
First published on 13th November 2014
Abstract
The Maillard reaction, non-enzymatic glycation after a complex series of reactions that involve reducing-sugars and proteins, produces a large number of end-products that are known as advanced glycation end-products (AGEs). AGEs are related to the pathogenesis of many diseases such as diabetes, inflammatory arthritis and cataracts. Today there is an increasing demand for natural AGE inhibitors for curing diabetes that have fewer side effects compared to synthetic drugs. The aim of this study was to explore the anti-glycation effect of aloin, in the presence and absence of casein-derived peptides, on human serum albumin (HSA). UV-visible and fluorescence spectroscopy were used to explore the number of free lysine residues, AGE formation and fibril formation during the HSA glycation. According to trinitrobenzenesulfonic acid and fluorescamine assay results, the presence of aloin and peptides reduced the number of glucose-attached lysine residues. Moreover AGE specific fluorescence and thioflavin T fluorescence decreased in the presence of aloin and peptides, indicative of a decrease in the formation of AGEs and fibrils respectively during the glycation of HSA. According to this study, aloin and camel casein derived peptides showed a synergic anti-glycation effect and inhibited the formation of fibrils and AGEs during the HSA glycation. This effect can be related to the antioxidant activity of aloin–peptide complex.
Introduction
It has been reported that 366 million people had diabetes in 2011, and it is estimated that this number will increase to 552 million by 2030.1 In diabetes, either insulin deficiency or insulin resistance triggers an abnormal increase in blood glucose, known as hyperglycemia. High blood glucose concentrations can cause a number of harmful health effects, such as an increased risk of heart disease, strokes, kidney disease, and blindness.2–4 The long-term effects of diabetes mostly can be caused by protein glycation.
Protein glycation is a non-enzymatic reaction that starts with the nucleophilic attack of a reducing sugar with primary amine groups on proteins to form a Schiff base.5 A slow rearrangement of this intermediate product can create more stable Amadori products, or ketoamine. Furthermore, modifications of these glycation products, such as rearrangement, oxidation, polymerization and cleavage, produces irreversible conjugates known as advanced glycation end products (AGEs).6
Serum albumin, with its long half-life (about 21 days) and high concentrations in the circulatory system, is highly sensitive to glycation.7 High concentrations of glycated albumin in cases of diabetes mellitus can trigger many metabolic disorders such as retinopathy, nephropathy, neuropathy and coronary artery disease.8,9 Today there is an increasing demand for plant based drugs for diabetes that are natural, less toxic, and cost-effective.10 Previous studies have shown that Aloe vera gel and phytosterols derived from Aloe vera gel are useful for the treatment of type 2 diabetes mellitus.11
Aloe vera L. (syn.: Aloe barbadensis Miller; Hindi: Ghikanvar; AV), belongs to the family Liliaceae (sub-family of the Asphodelaceae), native to North Africa and cultured in warm climatic areas.12 Aloe vera (AV) has a high content of phenolic compounds, glycosides (aloins), 1,8-dihydroxyanthraquinone derivatives (aloe-emodin), beta-1,4 acetylated mannan, mannose-phosphate and alprogen glucoprotein.13 Leaf exudates and mucilaginous gel of aloe have anti-inflammatory,14 anticancer,15 antioxidant,16 cytoprotective, cardiac stimulatory and immunomodulatory activities.17 They have also been reported to have antidiabetic and hypoglycemic properties.18–20 Little is known about the aloe ingredients responsible for all of these physiological effects and the concentrations required to exert their biological activities.
The major anthraquinones in aloe plants are aloin and aloe-emodin, and comprise 0.1 to 21.5% dry weight in the leaf exudate of 68 aloe species.21 Apparently aloin and aloe-emodin are responsible for the reported anti-inflammatory effects of aloe.22
Aloin, also known as barbaloin, is a C-glycoside derivative of an anthraquinone.23 An esterase secreted by intestinal microflora hydrolyzes the orally administrated aloin.24 Hydrolyzed C-glycoside forms aloe-emodin, an anthrone which is further auto-oxidized to the quinine. Aloin and aloe-emodin have laxative, antibacterial, antiviral, and anticancer effects.25–27
Milk protein-derived bioactive peptides have been used frequently in food additives for the formulation of functional foods.28 The bioactive peptides derived from milk proteins display various functionalities such as antioxidant activities, anticancer activities, reduction of blood pressure (ACE) and mineral binding activities.29
Camel milk proteins differ from those in bovine milk, which has a direct effect on their functional and biological properties.30 Caseins are the major proteins of bovine and camel milk and β-CN constitutes about 65% of total camel caseins.31
The results of our previous study showed that a mixture of aloin and casein derived peptides showed a very high antioxidant activity in a synergistic manner as compared to each component alone.32 Some studies have recently demonstrated that antioxidants, such as vitamin C and E, reduce in vitro and in vivo protein glycation.33–35 The aim of this study was to explore the anti-glycation effect of aloin in the presence and absence of casein derived peptides.
Materials and methods
Camel casein preparation
Camel milk was skimmed via heating to 37 °C and immediate centrifugation (5000g, 15 min). After adjustment of the pH of the skimmed milk to 4.6 with 1 N HCl and mixing at 35 °C for 30 min, caseins (CNs) were precipitated and separated from whey proteins by centrifugation (5860g, 60 min, 4 °C). After thorough washing with distilled water, caseins were lyophilized and stored at −20 °C until use. Camel β-CN was purified using batch fractionation on diethylaminoethyl (DEAE)–cellulose following a modified purification procedure.36
Preparation of casein hydrolysate samples
Two different peptides were produced; peptide 1: hydrolysation of camel β-CN with chymotrypsin was carried out at pH 7.8, an enzyme/substrate (E/S) ratio of 1/100 (w/w), 37 °C for 2 h; peptide 2: hydrolysation of camel β-CN with pepsin was carried out at pH 2.0 at an E/S ratio of X/100 (w/w) for 30 min. Further hydrolysation with trypsin and chymotrypsin was done at pH 7.8, an E/S ratio of 1/100 (w/w), 37 °C for 2 h. At the end of the hydrolysis, proteases were inactivated by heating the reaction mixture for 15 min at 85 °C. CN hydrolysates were separated by centrifugation for 15 min at 1680g, at 4 °C, using ultrafiltration (UF) membranes (Amicon Ultra-15, Millipore, cutoff of 10, 5, and 3 kDa). An o-phthaldialdehyde- (OPA-) based spectroscopic assay was used to measure the peptide concentration using a spectrophotometer (Shimadzu, model UV-3100, Kyoto, Japan) which was explained before.37
Preparation of advanced glycation end-products (AGEs)
AGEs were prepared by incubating 0.6 μM of human serum albumin (HSA) without and with glucose (16.5 mg ml−1 or 300 mg dl−1), aloin (0.25 mg ml−1), peptide (0.5 mg ml−1) in PBS (pH 7.4) containing sodium azide (0.1 mM) under sterile conditions at 37 °C up to 42 days. The proteins were dialysed against PBS, sterile-filtered with a 0.2 μm Millipore filter and stored at −20 °C. Protein concentrations were determined using a bicinchoninic acid assay.38
Quenching of tryptophan and 1-anilino-8-naphthalene sulfonic acid (ANS) fluorescence
Fluorescence spectra were collected with a Cary-Eclipse spectrophotometer. Tryptophan emission spectra were obtained in the range of 300 to 450 nm after excitation at 285 nm.
ANS–protein complex emission fluorescence spectra were collected in the range of 350 to 700 nm with an excitation wavelength of 470 nm after addition of 5 μl 1-anilino-8-naphthalene sulfonic acid (ANS) (0.2 mM) to 500 μl albumin samples (0.2 mg ml−1) and 5 min incubation.
2,4,6-Trinitrobenzene sulfonic acid (TNBS) assay
The TNBS assay is a sensitive method for determining the primary free amino groups in proteins. TNBSA reacts with a protein’s free amino groups to form trinitrophenolic derivatives as chromogenic products. The presence of trinitrophenolic derivatives was detected at an absorption wavelength of 335 nm. The final concentration of all samples was 0.2 mg ml−1. Valine was used as standard.39
Determination of lysine modifications
Fluorescamine was used to determine the number of reacted lysines, as described in detail previously.40 Briefly, 5 μl protein solution (1 mg ml−1), 100 μl of 100 mM Na2HPO4, 45 μl water, and 50 μl fluorescamine reagent (1 mM fluorescamine in acetonitrile) were mixed. After incubation in the dark for 10 min, fluorescence was collected at excitation/emission wavelengths of 390/490 nm.
AGE analysis
Fluorescence of the samples was collected at the excitation and emission maxima of 350 and 450 nm, respectively, versus an un-incubated blank containing the protein, sugar and inhibitor.
Determination of fibrillar state with thioflavin T
The fibrillar state of modified HSA was determined with thioflavin T as previously described.41 Briefly, 30 μl protein solution (approx. 1 mg ml−1) was mixed with 100 μl thioflavin T reagent (10 μM thioflavin T in 100 mM phosphate buffer, pH 7.0) and fluorescence was collected at excitation/emission wavelengths of 450/490 nm. Fresh HSA solution (1 mg ml−1) and PBS were used as controls.
Results
Quantification of primary amino groups by TNBSA
Trinitrobenzenesulfonic acid (TNBSA) is used for the quantification of primary amino groups. In the determination of the available lysine in glycated proteins, the distinction between reductive alkylation and Maillard-type condensation necessitates quantitative evaluation of non-alkylated lysines in protein hydrolysate, compared to results with the TNBSA method.39 The presence of aloin and peptide 2 reduced the number of attached lysines (Fig. 1).
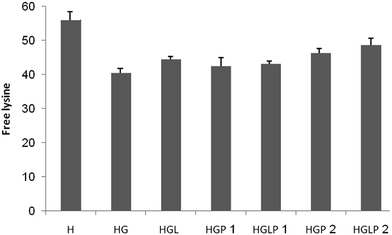 |
| Fig. 1 Number of free lysine residues for HSA samples which were incubated for 42 days in 50 mM phosphate buffer, pH 7.4 at 37 °C with (H) no additives (control), (HG) 16.5 mM glucose, (HGL) 16.5 mM glucose and 0.25 mg ml−1 aloin, (HGP1) 16.5 mM glucose and 0.5 mg ml−1 peptide 1, (HGLP1) 16.5 mM glucose and 0.25 mg ml−1 aloin and 0.5 mg ml−1 peptide 1, (HGP2) 16.5 mM glucose and 0.5 mg ml−1 peptide 2, (HGLP2) 16.5 mM glucose and 0.25 mg ml−1 aloin and 0.5 mg ml−1 peptide. | |
Determination of the number of non-free lysine residues in HSA
Fig. 2 shows the percentages of reacted lysine residues in samples incubated for 42 days using fluorescamine. Determination of the number of non-free lysine residues in HSA after incubation with physiological concentrations of glucose for 42 days, when AGE formation is expected, showed approximately 28 out of the 58 lysine residues in HSA were not free. The presence of peptides reduced the number of non-free lysine residues and the presence of aloin–peptide lowered this number even more.
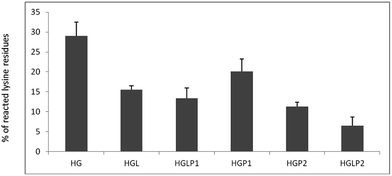 |
| Fig. 2 Percentages (%) of reacted lysine residues compared to control HSA; samples were incubated for 42 days in 50 mM phosphate buffer, pH 7.4 at 37 °C with (HG) 16.5 mM glucose, (HGL) 16.5 mM glucose and 0.25 mg ml−1 aloin, (HGP1) 16.5 mM glucose and 0.5 mg ml−1 peptide 1, (HGLP1) 16.5 mM glucose and 0.25 mg ml−1 aloin and 0.5 mg ml−1 peptide 1, (HGP2) 16.5 mM glucose and 0.5 mg ml−1 peptide 2, (HGLP2) 16.5 mM glucose and 0.25 mg ml−1 aloin and 0.5 mg ml−1 peptide 2. | |
Intrinsic fluorescence changes of HSA
A useful tool to follow the formation of AGEs is the study of the intrinsic fluorescence of proteins. As shown in previous studies the intrinsic protein fluorescence decreases in the presence of Glc and AGE-specific fluorescence changes are related to Glc concentration.40 The results of our study (Fig. 3) showed that glycation of HSA with Glc decreased the intrinsic fluorescence of HSA (excitation/emission 280/350 nm). The presence of aloin and peptides reduced the glycated protein fluorescence changes, with the aloin–peptide 2 mixture being the most effective component (Fig. 4).
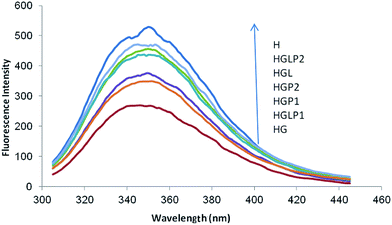 |
| Fig. 3 Intrinsic fluorescence curve of HSA samples which were incubated for 42 days in 50 mM phosphate buffer, pH 7.4 at 37 °C with (H) no additives (control), (HG) 16.5 mM glucose, (HGL) 16.5 mM glucose and 0.25 mg ml−1 aloin, (HGP1) 16.5 mM glucose and 0.5 mg ml−1 peptide 1, (HGLP2) 16.5 mM glucose and 0.25 mg ml−1 aloin and 0.5 mg ml−1 peptide 1, (HGP2) 16.5 mM glucose and 0.5 mg ml−1 peptide 2, (HGLP2) 16.5 mM glucose and 0.25 mg ml−1 aloin and 0.5 mg ml−1 peptide 2. Excitation/emission 280/350 nm. | |
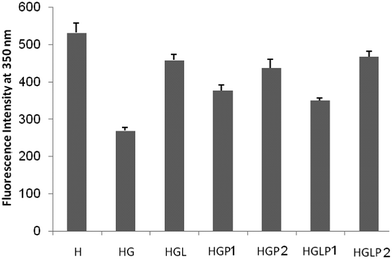 |
| Fig. 4 Intrinsic fluorescence changes at maximum wavelength (350 nm) of HSA samples which were incubated for 42 days in 50 mM phosphate buffer, pH 7.4 at 37 °C with (H) no additives (control), (HG) 16.5 mM glucose, (HGL) 16.5 mM glucose and 0.25 mg ml−1 aloin, (HGP1) 16.5 mM glucose and 0.5 mg ml−1 peptide 1, (HGLP2) 16.5 mM glucose and 0.25 mg ml−1 aloin and 0.5 mg ml−1 peptide 1, (HGP2) 16.5 mM glucose and 0.5 mg ml−1 peptide 2, (HGLP2) 16.5 mM glucose and 0.25 mg ml−1 aloin and 0.5 mg ml−1 peptide 2. | |
ANS fluorescence
The quenching of tryptophan fluorescence in modified glycated HSA reflected a local unfolding around these residues (Fig. 5). Other than the structural modification, a partial opening of hydrophobic pockets was also reported for HSA glycated in vitro for 21 days with glucose.42 The stronger affinity of proteins for the anilinonaphtalene-8-sulfonate (ANS) probe is related to a higher exposure of hydrophobic sites to the solvent. However, a longer incubation (more than 21 days) of albumin with glucose apparently inhibits penetration of ANS into the hydrophobic domains of the protein, which is attributed to the formation of the molten globule-like state and amyloid nanofibrils after 3 weeks and 20 weeks of incubation with glucose, respectively.42,43
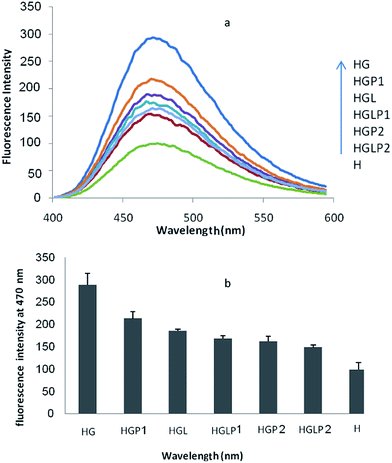 |
| Fig. 5 (a) ANS fluorescence curve and (b) fluorescence intensity at 470 nm in the presence of HSA which was incubated for 42 days in 50 mM phosphate buffer, pH 7.4 at 37 °C with (H) no additives (control), (HG) 16.5 mM glucose, (HGL) 16.5 mM glucose and 0.25 mg ml−1 aloin, (HGP1) 16.5 mM glucose and 0.5 mg ml−1 peptide 1, (HGLP1) 16.5 mM glucose and 0.25 mg ml−1 aloin and 0.5 mg ml−1 peptide 1, (HGP2) 16.5 mM glucose and 0.5 mg ml−1 peptide 2, (HGLP2) 16.5 mM glucose and 0.25 mg ml−1 aloin and 0.5 mg ml−1 peptide 2. | |
AGE-specific fluorescence
AGE-specific fluorescence has been described previously and it is widely used for the detection of AGEs and for following the onset of AGE formation in vitro and in vivo.40,44 In order to show the effect of AGEs on the changes of fluorescence intensity of HSA the data in this part are expressed as percentages of fluorescence intensity changes compared to control HSA (Fig. 6 and 7) The fluorescence intensity of the AGEs increased at 342/440 and 381/460 nm compared to the control HSA. Fig. 6 and 7 show the lower fluorescence intensity of AGEs in the presence of aloin and peptides, with the most efficient one being aloin–peptide 2 complex.
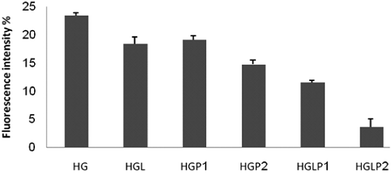 |
| Fig. 6 Percentages of fluorescence intensity changes at 342 nm compared to control HSA. Samples were incubated for 42 days in 50 mM phosphate buffer, pH 7.4 at 37 °C with (HG) 16.5 mM glucose, (HGL) 16.5 mM glucose and 0.25 mg ml−1 aloin, (HGP1) 16.5 mM glucose and 0.5 mg ml−1 peptide 1, (HGLP1) 16.5 mM glucose and 0.25 mg ml−1 aloin and 0.5 mg ml−1 peptide 1, (HGP2) 16.5 mM glucose and 0.5 mg ml−1 peptide 2, (HGLP2) 16.5 mM glucose and 0.25 mg ml−1 aloin and 0.5 mg ml−1 peptide 2. | |
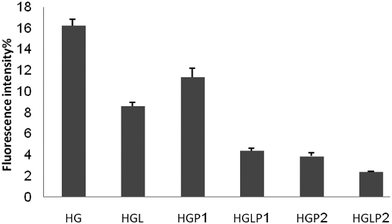 |
| Fig. 7 Percentages of fluorescence intensity changes compared to control HSA at 381 nm. Samples were incubated for 42 days in 50 mM phosphate buffer, pH 7.4 at 37 °C with (HG) 16.5 mM glucose, (HGL) 16.5 mM glucose and 0.25 mg ml−1 aloin, (HGP1) 16.5 mM glucose and 0.5 mg ml−1 peptide 1, (HGLP1) 16.5 mM glucose and 0.25 mg ml−1 aloin and 0.5 mg ml−1 peptide 1, (HGP2) 16.5 mM glucose and 0.5 mg ml−1 peptide 2, (HGLP2) 16.5 mM glucose and 0.25 mg ml−1 aloin and 0.5 mg ml−1 peptide 2. | |
Determination of the fibrillar state of incubated proteins with thioflavin T
Thioflavin T binds to specific sites of amyloid fibrils, namely cross-structures, so it can detect significant changes in protein structure.45 Formation of amyloid fibrils in HSA glycated with glucose, fructose, and ribose was formerly described by Sattarahmady et al.43 In their experiment they found an increase in thioflavin T fluorescence with increasing incubation time in the presence of constant glucose 6-phosphate concentration. Our results showed that the presence of aloin and peptides decreased the formation of fibrils during the glycation of HSA (Fig. 8).
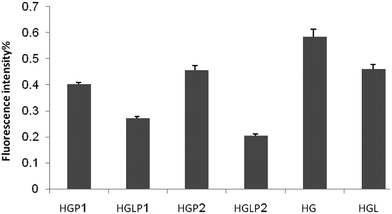 |
| Fig. 8 Percentages of fluorescence intensity changes of thioflavin T at 490 nm compared to control HSA. Samples were incubated for 42 days in 50 mM phosphate buffer, pH 7.4 at 37 °C with (HG) 16.5 mM glucose, (HGL) 16.5 mM glucose and 0.25 mg ml−1 aloin, (HGP1) 16.5 mM glucose and 0.5 mg ml−1 peptide 1, (HGLP1) 16.5 mM glucose and 0.25 mg ml−1 aloin and 0.5 mg ml−1 peptide 1, (HGP2) 16.5 mM glucose and 0.5 mg ml−1 peptide 2, (HGLP2) 16.5 mM glucose and 0.25 mg ml−1 aloin and 0.5 mg ml−1 peptide 2. | |
Discussion
Non-enzymatic glycation or browning, which is known as the Maillard reaction, is a complex series of reactions that involve reducing-sugars and α-amines of proteins, producing a large number of end-products that are known as advanced glycation end-products (AGEs). AGEs are related to the pathogenesis of diabetes and neurological diseases such as Alzheimer’s disease, vascular stiffening, atherosclerosis, osteoarthritis, inflammatory arthritis and cataracts.46 Therefore, AGE inhibitors and AGE breakers are potential therapeutics for curing diseases. The underlying mechanism of AGE inhibitors is based on the attenuation of glycoxidation and/or oxidative stress by the sequestration of metal ions, reactive 1,2-dicarbonyl compounds, and reactive oxygen and reactive nitrogen species.46
Aminoguanidine (AG),47 aspirin,48 vitamin B6,49 quercetin50 etc., are inhibitors of the Maillard reaction.51 Some natural inhibitors of AGEs formation have been reported to date, most of which are anthraquinones and flavonoids with IC50 values ranging from 90–200 mM.52 In a recent study on the structural requirements of flavonoids for formation of advanced glycation end-products (AGEs), it was shown that various flavonoids with strong AGE formation inhibitory activity tended to exhibit strong scavenging activity for 1,1-diphenyl-2-picrylhydrazyl and superoxide anion radicals.52
In a different study on the anti-glycation activity of anthraquinones from the seeds of Cassia tora on AGEs–BSA formation, emodin showed a stronger inhibitory activity (IC50 values of 118) than the well known glycation inhibitor aminoguanidine (IC50 value of 961 mM). The inhibitory effect of emodin on AGEs–BSA formation in the assay was reconfirmed by a specific AGEs–ELISA (absorbance of 450 nm).53 According to this study, aloin and camel casein derived peptides showed a synergic inhibitory effect on AGE formation. This effect can be attributed to the antioxidant activity of aloin–peptide complex which was reported recently.32
Conclusion
There are an increasing number of reports on bioactive peptides from milk hydrolysates in the literature. Nature amazingly encapsulates and preserves bioactive peptides within the parent protein to be released and activated safely by gastrointestinal enzymes. Previous studies have shown that camel milk derived bioactive peptides have different functionalities toward human health. In this study it was shown that the use of camel milk bioactive peptides in combination with aloin inhibited AGE production effectively and could be introduced as a nutraceutical food. These kinds of functional foods could be used effectively to replace or reduce the amounts of synthetic drugs used for diabetes.
Acknowledgements
The support of the University of Tehran, Center of Excellence in Biothermodynamics (CEBiotherm), Iran National Science Foundation (INSF), Iran National Elites Foundation (INEF), UNESCO Chair on Interdisciplinary Research in Diabetes (University of Tehran, Tehran, Iran), and the Iran Society of Biophysical Chemistry is gratefully acknowledged.
References
- D. R. Whiting, L. Guariguata, C. Weil and J. Shaw, IDF Diabetes Atlas: Global estimates of the prevalence of diabetes for 2011 and 2030, Diabetes Res. Clin. Pract., 2011, 94, 311–321 CrossRef PubMed.
- G. Basta, G. Lazzerini, M. Massaro, T. Simoncini, P. Tanganelli, C. Fu, T. Kislinger, D. M. Stern, A. M. Schmidt and R. De Caterina, Advanced glycation end products activate endothelium through signal-transduction receptor RAGE: a mechanism for amplification of inflammatory responses, Circulation, 2002, 105, 816–822 CrossRef CAS.
- J. W. Hartog, A. A. Voors, S. J. Bakker, A. J. Smit and D. J. van Veldhuisen, Advanced glycation end-products (AGEs) and heart failure: pathophysiology and clinical implications, Eur. J. Heart Failure, 2007, 9, 1146–1155 CrossRef CAS PubMed.
- A. Goldin, J. A. Beckman, A. M. Schmidt and M. A. Creager, Advanced glycation end products sparking the development of diabetic vascular injury, Circulation, 2006, 114, 597–605 CrossRef CAS PubMed.
- P. Thornalley, A. Langborg and H. Minhas, Formation of glyoxal, methylglyoxal and 3-deoxyglucosone in the glycation of proteins by glucose, Biochem. J., 1999, 344, 109–116 CrossRef CAS.
- J. Anguizola, R. Matsuda, O. S. Barnaby, K. S. Hoy, C. Wa, E. DeBolt, M. Koke and D. S. Hage, Review: Glycation of human serum albumin, Clin. Chim. Acta, 2013, 425, 64–76 CrossRef CAS PubMed.
- A. Guerin-Dubourg, A. Catan, E. Bourdon and P. Rondeau, Structural modifications of human albumin in diabetes, Diabetes Metab., 2012, 38, 171–178 CAS.
- C. G. Schalkwijk, N. Ligtvoet, H. Twaalfhoven, A. Jager, H. G. T. Blaauwgeers, R. O. Schlingemann, L. Tarnow, H. H. Parving, C. D. A. Stehouwer and V. W. M. Van Hinsbergh, Amadori albumin in type 1 diabetic patients: Correlation with markers of endothelial function, association with diabetic nephropathy, and localization in retinal capillaries, Diabetes, 1999, 48, 2446–2453 CrossRef CAS.
- M. P. Cohen, Intervention strategies to prevent pathogenetic effects of glycated albumin, Arch. Biochem. Biophys., 2003, 419, 25–30 CrossRef CAS PubMed.
- J. K. Grover, S. Yadav and V. Vats, Medicinal plants of India with anti-diabetic potential, J. Ethnopharmacol., 2002, 81, 81–100 CrossRef CAS.
- M. Tanaka, E. Misawa, Y. Ito, N. Habara, K. Nomaguchi, M. Yamada, T. Toida, H. Hayasawa, M. Takase and M. Inagaki, Identification of five phytosterols from Aloe vera gel as anti-diabetic compounds, Biol. Pharm. Bull., 2006, 29, 1418–1422 CAS.
- E. R. Rodriguez, J. D. Martin and C. D. Romero, Aloe vera as a functional ingredient in foods, Crit. Rev. Food Sci. Nutr., 2010, 50, 305–326 CrossRef CAS PubMed.
- J. H. Hamman, Composition and applications of Aloe vera leaf gel, Molecules, 2008, 13, 1599–1616 CrossRef CAS PubMed.
- A.-I. A. O. E. From and A. V. Gel, Aloe vera and gibberellin. Anti-inflammatory activity in diabetes, J. Am. Podiatr. Med. Assoc., 1989, 79, 24–26 Search PubMed.
- H. El-Shemy, M. Aboul-Soud, A. Nassr-Allah, K. Aboul-Enein, A. Kabash and A. Yagi, Antitumor properties and modulation of antioxidant enzymes activity by Aloe vera leaf active principles isolated via supercritical carbon dioxide extraction, Curr. Med. Chem., 2010, 17, 129–138 CrossRef CAS.
- S. Rajasekaran, K. Sivagnanam and S. Subramanian, Antioxidant effect of Aloe vera gel extract in streptozotocin-induced diabetes in rats, Pharmacol. Rep., 2005, 57, 90–96 Search PubMed.
- S.-A. Im, Y.-R. Lee, Y.-H. Lee, M.-K. Lee, Y. I. Park, S. Lee, K. Kim and C.-K. Lee, In vivo evidence of the immunomodulatory activity of orally administered Aloe vera gel, Arch. Pharm. Res., 2010, 33, 451–456 CrossRef CAS PubMed.
- A. Okyar, A. Can, N. Akev, G. Baktir and N. Sütlüpinar, Effect of Aloe vera leaves on blood glucose level in type I and type II diabetic rat models, Phytother. Res., 2001, 15, 157–161 CrossRef CAS PubMed.
- S. Yongchaiyudha, V. Rungpitarangsi, N. Bunyapraphatsara and O. Chokechaijaroenporn, Antidiabetic activity of Aloe vera L. juice. I. Clinical trial in new cases of diabetes mellitus, Phytomedicine, 1996, 3, 241–243 CrossRef CAS.
- B. Vogler and E. Ernst, Aloe vera: a systematic review of its clinical effectiveness, Br. J. Gen. Pract., 1999, 49, 823–828 CAS.
- B.-E. Van Wyk, M. V. R. Van Oudtshoorn and G. Smith, Geographical variation in the major compounds of Aloe ferox leaf exudate, Planta Med., 1995, 61, 250–253 CrossRef CAS PubMed.
- M.-Y. Park, H.-J. Kwon and M.-K. Sung, Evaluation of aloin and aloe-emodin as anti-inflammatory agents in Aloe by using murine macrophages, Biosci., Biotechnol., Biochem., 2009, 73, 828–832 CrossRef CAS PubMed.
- D. Saccù, P. Bogoni and G. Procida, Aloe exudate: characterization by reversed phase HPLC and headspace GC-MS, J. Agric. Food Chem., 2001, 49, 4526–4530 CrossRef PubMed.
- Q.-M. Che, T. Akao, M. Hattori, K. Kobashi and T. Namba, Isolation of a human intestinal bacterium capable of transforming barbaloin to aloe-emodin anthrone, Planta Med., 1991, 57, 15–19 CrossRef CAS PubMed.
- D. S. Alves, L. Pérez-Fons, A. Estepa and V. Micol, Membrane-related effects underlying the biological activity of the anthraquinones emodin and barbaloin, Biochem. Pharmacol., 2004, 68, 549–561 CrossRef CAS PubMed.
- T. Hatano, M. Kusuda, K. Inada, T.-o. Ogawa, S. Shiota, T. Tsuchiya and T. Yoshida, Effects of tannins and related polyphenols on methicillin-resistant Staphylococcus aureus, Phytochemistry, 2005, 66, 2047–2055 CrossRef CAS PubMed.
- A. Y. Esmat, C. Tomasetto and M.-C. Rio, Cytotoxicity of a natural anthraquinone (aloin) against human breast cancer cell lines with and without ErbB-2: Topoisomerase II-alpha coamplification, Cancer Biol. Ther., 2006, 5, 97–103 CrossRef CAS.
- P. J. Huth, D. K. Layman and P. H. Brown, Foreword, J. Nutr., 2004, 134, 961S CAS.
- R. Nagpal, P. Behare, R. Rana, A. Kumar, M. Kumar, S. Arora, F. Morotta, S. Jain and H. Yadav, Bioactive peptides derived from milk proteins and their health beneficial potentials: an update, Food Funct., 2011, 2, 18–27 CAS.
- H. El-Hatmi, J.-M. Girardet, J.-L. Gaillard, M. H. Yahyaoui and H. Attia, Characterisation of whey proteins of camel Camelus dromedarius milk and colostrum, Small Ruminant Res., 2007, 70, 267–271 CrossRef PubMed.
- S. Kappeler, Z. Farah and Z. Puhan, 5′-Flanking regions of camel milk genes are highly similar to homologue regions of other species and can be divided into two distinct groups, J. Dairy Sci., 2003, 86, 498–508 CrossRef CAS.
- F. Ghamari, S. M. Ghaffari, M. Salami, F. Moosavi-Movahedi, F. Farivar, A. Johari, A. A Saboury, J. M. Chobert, T. Haertlé and A. A Moosavi-Movahedi, Synergic study of glucosidase inhibitory action of aloin and its antioxidant activity with and without camel casein and its peptides, Protein Pept. Lett., 2013, 20, 607–612 CrossRef CAS.
- J. A. Vinson and T. B. Howard, Inhibition of protein glycation and advanced glycation end products by ascorbic acid and other vitamins and nutrients, J. Nutr. Biochem., 1996, 7, 659–663 CrossRef CAS.
- A. Ceriello, Hyperglycaemia: the bridge between non-enzymatic glycation and oxidative stress in the pathogenesis of diabetic complications, Diabetes, Nutr. Metab., 1999, 12, 42–46 CAS.
- V. Jakuš, M. Hrnčiarová, J. Čársky, B. Krahulec and N. Rietbrock, Inhibition of nonenzymatic protein glycation and lipid peroxidation by drugs with antioxidant activity, Life Sci., 1999, 65, 1991–1993 CrossRef.
- A. Kauf and R. Kensinger, Purification of porcine β-casein, N-terminal sequence, quantification in mastitic milk, J. Anim. Sci., 2002, 80, 1863–1870 CAS.
- F. C. Church, H. E. Swaisgood, D. H. Porter and G. L. Catignani, Spectrophotometric assay using o-phthaldialdehyde for determination of proteolysis in milk and isolated milk proteins, J. Dairy Sci., 1983, 66, 1219–1227 CrossRef CAS.
- P. Smith, R. I. Krohn, G. Hermanson, A. Mallia, F. Gartner, M. Provenzano, E. Fujimoto, N. Goeke, B. Olson and D. Klenk, Measurement of protein using bicinchoninic acid, Anal. Biochem., 1985, 150, 76–85 CrossRef CAS.
- P. Cayot and G. Tainturier, The quantification of protein amino groups by the trinitrobenzenesulfonic acid method: a reexamination, Anal. Biochem., 1997, 249, 184–200 CrossRef CAS PubMed.
- A. Schmitt, J. Schmitt, G. Münch and J. Gasic-Milencovic, Characterization of advanced glycation end products for biochemical studies: side chain modifications and fluorescence characteristics, Anal. Biochem., 2005, 338, 201–215 CrossRef CAS PubMed.
- J. Misselwitz, S. Franke, E. Kauf, U. John and G. Stein, Advanced glycation end products in children with chronic renal failure and type 1 diabetes, Pediatr. Nephrol., 2002, 17, 316–321 CrossRef PubMed.
- N. Sattarahmady, A. A. Moosavi-Movahedi, F. Ahmad, G. H. Hakimelahi, M. Habibi-Rezaei, A. A. Saboury and N. Sheibani, Formation of the molten globule-like state during prolonged glycation of human serum albumin, Biochim. Biophys. Acta, Gen. Subj., 2007, 1770, 933–942 CrossRef CAS PubMed.
- N. Sattarahmady, A. A. Moosavi-Movahedi, M. Habibi-Rezaei, S. Ahmadian, A. A. Saboury, H. Heli and N. Sheibani, Detergency effects of nanofibrillar amyloid formation on glycation of human serum albumin, Carbohydr. Res., 2008, 343, 2229–2234 CrossRef CAS PubMed.
- M. E. Westwood and P. J. Thornalley, Molecular characteristics of methylglyoxal-modified bovine and human serum albumins. Comparison with glucose-derived advanced glycation endproduct-modified serum albumins, J. Protein Chem., 1995, 14, 359–372 CrossRef CAS.
- M. Krebs, E. Bromley and A. Donald, The binding of thioflavin-T to amyloid fibrils: localisation and implications, J. Struct. Biol., 2005, 149, 30–37 CrossRef CAS PubMed.
- V. P. Reddy and A. Beyaz, Inhibitors of the Maillard reaction and AGE breakers as therapeutics for multiple diseases, Drug Discovery Today, 2006, 11, 646–654 CrossRef CAS PubMed.
- M. Brownlee, H. Vlassara, A. Kooney, P. Ulrich and A. Cerami, Aminoguanidine prevents diabetes-induced arterial wall protein cross-linking, Science, 1986, 232, 1629–1632 CAS.
- R. Huby and J. J. Harding, Non-enzymic glycosylation (glycation) of lens proteins by galactose and protection by aspirin and reduced glutathione, Exp. Eye Res., 1988, 47, 53–59 CrossRef CAS.
- A. A. Booth, R. G. Khalifah and B. G. Hudson, Thiamine pyrophosphate and pyridoxamine inhibit the formation of antigenic advanced glycation end-products: comparison with aminoguanidine, Biochem. Biophys. Res. Commun., 1996, 220, 113–119 CrossRef CAS PubMed.
- Y. Morimitsu, K. Yoshida, S. Esaki and A. Hirota, Protein glycation inhibitors from thyme (Thymus vulgaris), Biosci., Biotechnol., Biochem., 1995, 59, 2018–2021 CrossRef CAS PubMed.
- N. Matsuura, T. Aradate, C. Sasaki, H. Kojima, M. Ohara, J. Hasegawa and M. Ubukata, Screening system for the Maillard reaction inhibitor from natural product extracts, J. Health Sci., 2002, 48, 520–526 CrossRef CAS.
- H. Matsuda, T. Wang, H. Managi and M. Yoshikawa, Structural requirements of flavonoids for inhibition of protein glycation and radical scavenging activities, Bioorg. Med. Chem., 2003, 11, 5317–5323 CrossRef CAS PubMed.
- J. Dae Sik, L. Ga Young, K. Young Sook, L. Yun Mi, K. Chan-Sik, Y. Jeong Lim and J. S. Kim, Anthraquinones from the seeds of Cassia tora with inhibitory activity on protein glycation and aldose reductase, Biol. Pharm. Bull., 2007, 30, 2207–2210 Search PubMed.
|
This journal is © The Royal Society of Chemistry 2015 |