DOI:
10.1039/D2SU00069E
(Tutorial Review)
RSC Sustain., 2023,
1, 2148-2161
A comprehensive review on removal of environmental pollutants using a surfactant based remediation process
Received
6th October 2022
, Accepted 9th July 2023
First published on 10th July 2023
Abstract
Surfactants are considered amphiphilic substances, having excellent adsorption and association capabilities, offering them the potential for a variety of techniques designed to eliminate pollution and preserve the natural world. Surfactants are not only used to remove the oil-leakage menace but are equally important in removing several pollutants such as heavy metals, dyes, industrial wastes, several pesticides, pharmaceutical wastes, and other toxic compounds. These pollutants have a very alarming impact on human health and on the ecosystem as a whole. Traditional remediation processes are inadequate, tedious, or limited by technology and expense. Surfactants are good at removing these pollutants from diverse media. In this review, a comprehensive and systematic analysis is presented for the remediation of these pollutants using surfactants. We aim to provide the most recent and accurate information on the use of surfactants to help eliminate a varied range of toxic agents from waste water, including volatile organic compounds, personal care products, pharmaceutical effluents, dyes, pesticides, and petroleum hydrocarbons. Authors have also discussed why these pollutants occur and how surfactants might help reduce them. Different forms of surfactants provide a wide range of possibilities, as demonstrated by both laboratory studies and real-world procedures. Among surfactants, bio-surfactants and blended surfactants are superior and warrant consideration. For example, more research into developing and deploying novel bio-surfactants has the potential to improve the effectiveness, efficiency, and economy of wastewater treatment systems.
Sustainability spotlight
This review provides the most recent and accurate information on the use of micelles and surfactants to help remove a wide range of toxic agents from water and waste, including volatile organic compounds, pesticides, and petroleum hydrocarbons. The literature review leads to the conclusion that large-scale decontamination of wastewater can benefit from the use of micelles-assisted water and soil treatment technologies.
|
1. Introduction
Industrialization is typically blamed as the main cause of hazardous waste leakage into aquatic environments, despite the fact that it is inextricably linked to a nation's economy. Effluents released from the industries are absorbed into groundwater, surface water, and subsurface soils. Industrial effluent degrades water quality and constitutes a serious hazard to people and aquatic life because it contains harmful substances such as metals, VOCs (volatile organic compounds), insecticides, colour pollutants, medications, and personal care products.1–4 Traditional wastewater treatment techniques include ultrafiltration (UF) with adsorptive adsorption, reverse osmosis, solvent extraction, ion exchange, and electrochemistry.4,5 Environmentally friendly surfactant-based approaches have drawn a lot of interest for removing pollutants from a variety of media, the reason being their eco-friendliness, elevated efficacy in removing pollutants, flexibility, and dependence on “green chemistry” concepts.6–9
The escalation of industrialization has resulted in a shift in global focus towards the ecosystem and human health, both of which are experiencing detrimental effects due to the surge in environmental pollution. The absence of a definitive solution to the pollution issue can be attributed to its constantly evolving nature. The degradation of air, water, and soil pollutants persists, resulting in notable health concerns for the global populace. Fine particulate matter (PM) in the air, for instance, raises the chance of developing a number of serious illnesses. As reported by the WHO in 2019, nearly 900
000 people died from diarrhea-related causes in 2016, more than 470
000 of whom were children. The presence of heavy metals and persistent organic pollutants (POPs) in contaminated soil poses potential health hazards, including metal poisoning and endocrine-disrupting effects. These risks are particularly significant during early developmental stages when growth and maturation are ongoing.10 The issue of environmental pollution is believed to be the predominant factor contributing to health risks, and as such, warrants further investigation in identifying the root cause of persistent human ailments.
Oil is a chief source of energy in the world and is considered to be an important parameter for national economies.11 Oil spills have been the main causes of ocean pollution due to the fast expansion of offshore oil exploration and maritime traffic. The United States Environmental Protection Agency, or US EPA, estimated that the country's total oil leaks were around 2500 million gallons.12 According to estimates from Finland, there are also over 23
000 contaminated locations where oil spills pose a serious threat.13 The fact that 35% of Canada's subterranean reservoir systems are spilling is quite worrying.14 Iran has been facing significant oil pollution issues because of its 8.58 percent ownership of the world's total oil reserves, enormous oil output, and extensive pipeline network.14 Saturates, aromatics, and heteroatoms are among the many types of chemicals found in oil.15 Aromatic chemicals, which have 2–6 member aromatic rings, are mutagenic, cause physical or functional defects in human embryos known as teratogenic, and various carcinogenic compounds are lethal to humans.15 Soil and groundwater that have been polluted by oil must be cleaned up as quickly as feasible.
Surfactant-enhanced remediation (SER) is widely used for the cleanup of many pollutants and oil-contaminated land and aquifers, for example, because it can reduce the effects of drifting and allow for faster recovery.16–19In situ surfactant dislodging and ex situ surfactant cleansing are the two main types of SER.14,19 Surfactants make it easier for solid-phase (through adsorption) oil pollutants to be removed and help NAPL (non-aqueous phase liquids) to dissolve. This increases their ability to move into the water phase or their ability to work with water phase mitigation agents or microorganisms. Surfactants also lower a solution's surface tension (the force between air and water) and interfacial tension (the force between the aqueous and organic phases).20,21
The action mechanisms being mobilization and solubilization, applications such as in the formation of surfactant solutions and surfactant effervescence or foams, inducing components such as their ability to penetrate, kinds of surfactants, contaminants, and amounts of surfactants, and discharge procedure techniques have all been summarized in several review papers.22,23 It's important to note that their capacity to form micelles in water makes hydrophobic pollutants like polychlorinated biphenyls (PCBs) and polycyclic aromatic hydrocarbons (PAHs) easier to dissolve.24 In the current review article, the authors have made an effort to provide a summary of the numerous classes of surfactants, their uses, environmental occurrences, pollution, and toxicological consequences, as well as several methods used to eradicate soil and water pollution through some environmentally friendly techniques based on surfactants. In addition to this, the authors have also included a few techniques that are based on surfactants that are used to combat soil and water pollution. The usage of surfactant-based remediation processes, which are employed for the removal of dangerous substances from the environment, was the subject of a research study that was conducted not too long ago. This is despite the growth in the number of publications that are connected to surfactants.
2. Literature survey and selection criteria
Recent years have seen the discovery of emerging contaminants in the environment and wastewater.25,26 These pollutants are substances that are created during the manufacturing of various goods, have unidentified impacts on the surrounding environment and human robustness, and are not covered by the majority of environmental laws across the globe.27 The majority of chemicals are found in pharmaceuticals, personal care products (PCPs), and chemicals that mess with hormones.28 The fact that these compounds might hurt the environment has led to a lot of research into how to get rid of them from water.
Illegal substances, genetically engineered organisms, nanoscale materials, agrochemicals, industrial compounds, micro-sized plastic particles, domestic chemicals, surface-active agents, organic solvents, and phthalate esters are among several instances that deviate from these classifications yet are acknowledged as noteworthy pollutants.29 The list of substances includes antimicrobials, disinfectants, flame retardants, perfluorinated compounds, processed sugars, hormones, pharmaceuticals, X-ray contrast media, and fire extinguishers.30–32
Due to specific technological and budgetary constraints, conventional cleanup methods are either ineffective, laborious, or constrained. Surfactants are useful for removing hazardous heavy metal ions and organic pollutants from various media due to their excellent pollutant-removal effectiveness and environmental friendliness.4 Surface-active agents are another name for surfactants. They are substances with sections that are both water-loving (hydrophilic, generally polar) and water-repelling (hydrophobic, generally lipophilic) and have the ability to lower interfacial energy as well as remove soluble and insoluble components from water.33 Polychlorinated biphenyls (PCBs) and polycyclic aromatic hydrocarbons (PAHs) can be dissolved in water because of their potential to form micelles in aqueous solutions.24 The hydrophilic and hydrophobic components of anionic, cationic, amphoteric, and nonionic surfactants differ depending on the type of surfactant, giving rise to a variety of features and functions.34 Amphoteric surfactants have both positive and negative charges in their hydrophilic moieties, making them a hybrid of cationic and anionic surfactants.34 Surfactant function and application types are greatly influenced by their structural makeup. Their level of ionization and critical micelle concentration (CMC) depend on the availability of groups that don't like water and counterions. Colloidal objects are stabilized by hydrophilic polar groups' attraction for polar solvents like water, whereas the availability of groups that don't like water and counter ions influences the extent of ionization and critical micelle concentration.35
A number of goods, including beauty products for hair, include more than one kind of surface-active substance. Typically, just one type of surfactant is used for a variety of tasks, including the production of fluoropolymers, the formulation of pesticides, and soil remediation (Fig. 1). Wastewater treatment plant (WWTP) effluents, sludge, surface waters, sediments, groundwater, and drinking water are only some of the places these compounds can be found because of their general use and the technological constraints of wastewater treatment and management. Main discharge sources that might cause surfactants to enter waterways are: discharges from residential and treatment facilities, rainy runoff, and industrial and municipal fluids.28
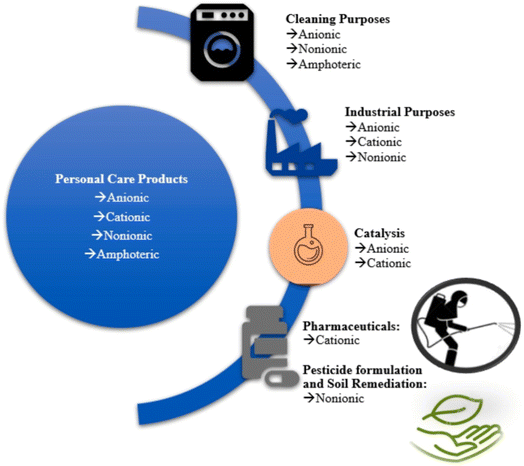 |
| Fig. 1 Various surfactant-enhanced remediation (SER) methods for oil-contaminated soil and groundwater are described.36 | |
Several further analyses have examined various features of surfactants over the last five years. Recent developments and applications of these substances have been covered in earlier research,33,37,38 as well as the use of surface-active substances in the treatment of wastewater39 and the separation of substances by means of pressure-driven membrane separation.39 Other studies have investigated the use of physical techniques for surfactant removal, such as adsorption,40 the impact of cleaning agents on natural habitats and wastewater treatment techniques,41 and the existence of surfactants in marine ecosystems42 and plants,43 as well as ways to deal with surfactant bioremediation.44
3. Action and performance investigation methods of surfactants
SER of oil-tainted surfaces and aquifers has been the subject of many studies around the world over the past 20 years (Fig. 1). For various kinds of surfactants, like anionic, cationic, amphoteric, biosurfactants, and gemini, there have been many ideas about how they work and how to improve their productivity. With the number of publications each year rapidly rising during the past ten years, the publication of connected articles is on the rise. Applications for oil-contaminated site cleanup have indicated that SER is gaining popularity.
3.1. Action mechanisms of surface active agents
There are two types of surfactants: natural (derived from plants, microbes, etc.) and synthetic (made artificially). Subunits or monomers of surfactant molecules are distributed in solution at low surfactant concentrations. The amphiphilic characteristic of surfactants allows monomers to be organized in a specific sequence at the air–water (A/W) and oil–water (O/W) interfaces, reducing surface tension and interfacial tension. As the concentration increases, evenly distributed surfactant subunits are mostly swamped at the surface, and micelles begin to form when hydrophobic groups attract each other.45Fig. 2 shows the mechanism of removal of pollutants using surfactant.
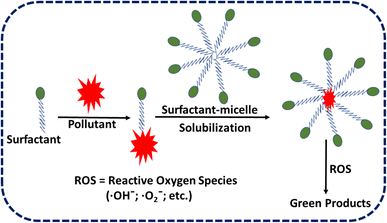 |
| Fig. 2 Image depicting the mechanism of removal of pollutants using surfactant. | |
The critical micelle concentration (CMC) is the minimum amount of surfactant needed for micelles to form.46 The introduction of surfactant molecules into water results in a reduction of the interfacial tension between air and water. At low concentrations, the molecules undergo adsorption on the surface. However, as the concentration increases, the surfactant molecules migrate towards the bulk phase. Once the interface reaches full saturation with the molecules, the forces become imbalanced and begin to act upon the molecules, leading to a reduction in surface tension values. Upon surpassing the point of saturation, the molecules present in the bulk phase initiate the formation of micelles, resulting in a significant reduction in surface tension to its minimum level. Fig. 3 shows that the surface tension of air over water and the oil–water interfacial tension in the solution keep dropping, and upon reaching their CMC marks, they usually settle at the lowest level.47,48
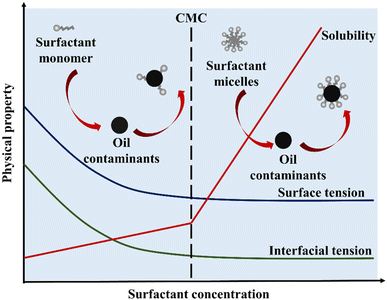 |
| Fig. 3 The effect of surfactant concentration on surface tension, interfacial tension, and the solubility enhancement factor for oil impurities, adapted from ref. 48b. | |
3.2. Performance evaluation methods for surfactants
People have come up with various ways to assess and describe the performance of various surfactants. Surfactant qualities are often described in terms of CMC, hydrophile–lipophile balance (HLB), surface tension, and interfacial tension. A lower CMC value indicates that more micelles can form from the same amount of surfactant. This means that the solubilizing capacity is better, less surfactant is used, and the expense of cleaning up is less.49,50
Like CMC, reduced interfacial and surface tensions mean that a surface-active substance works better. The hydrophile–lipophile balance is a method of calculating how much a surfactant with a value between 3 and 6 completely dissolves in the NAPL phase and forms water-in-oil emulsions. Elevated hydrophile–lipophile balance surfactants with values between 8 and 18 completely dissolve in water and make oil-in-water (O/W) emulsions51 as shown in Fig. 4. Choosing surfactants with the right HLB amount can make it easier to clean up oil-contaminated surfaces and water. But surfactants with HLB values equal to 10 could indeed make the surface tension decrease and make it easier to move NAPL.52
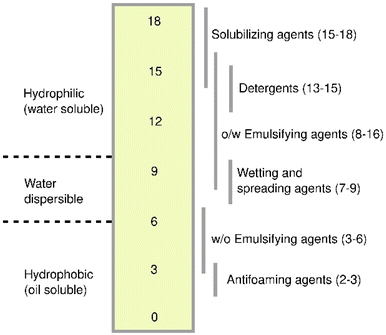 |
| Fig. 4 Image depicting the HLB variation for the surfactants. | |
4. Classification of surfactants
Depending on the polarity of the head group, surfactant compounds are classified into five different groups. Non-ionic surfactants, which don't contain a charge on their head group and are hence more susceptible to solvents rich in electrolytes, are one of these types. Fig. 5 shows the typical structure of five distinct types of surfactants, including: (1) anionic surfactants, which have a negative charge on their head group and are widely used in the detergent industry; (2) cationic surfactants, which possess a positive charge on their head group and are employed as disinfectants and preservatives; (3) non-ionic surfactants, which have no polar head group and these are used as wetting agents, emulsifiers and industrial cleaners; (4) zwitterionic surfactants, which have a head group made up of both positive and negative charges and these are employed in emulsion polymerization, oil recovery, and textile processing; (5) gemini surfactants, which are a different kind of surfactant molecule with two polar heads and two nonpolar tails that are connected by a spacer to produce a dimer-like structure and are used in oilfields as they have good thermal stability, and another class is biosurfactants that possess certain special properties and have found application in the food industry, bioremediation and biotechnology. Fig. 5 depicts the typical structure of the five different kinds of surfactants on the basis of the polar head group.
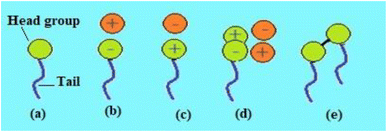 |
| Fig. 5 Types of surfactant: (a) non-ionic, (b) anionic, (c) cationic, (d) zwitterionic and (e) gemini surfactants.53 | |
4.1. Anionic surfactants
Since they are used in the majority of commercial and domestic applications, anionic surface-active substances are the most well-known and often used type of surface-active substance. In aqueous solutions, anionic surfactants' polar head group maintains a negative charge. As seen in Fig. 6, the most prevalent anionic polar head groups are sulfonates, carboxylates, sulfates, and phosphates. Sulfonates have mostly been used in the petroleum sector for the purpose of enhanced oil recovery (EOR). Due to their biodegradability and environmentally benign effects, green and natural anionic surfactants made from natural resources, like surfactants derived from esterification of vegetable oils,54,55 have become more popular and been used in a variety of sectors over the past several decades.
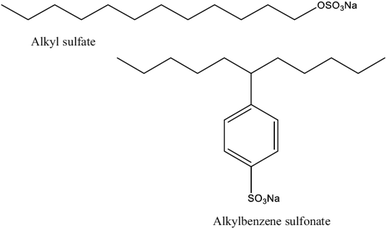 |
| Fig. 6 Structures of some anionic surfactants.53 | |
4.2. Cationic surfactants
In water, this family of surface-active substances with a positive constituent separate into an anionic portion that is frequently of the halogen type and an amphiphilic cationic part. A large majority of this group of cationic surfactants is made up of nitrogen derivatives, like quaternary ammonium salts and salts of fatty amines with one or more long alkyl chains that typically come from natural sources.56,57 Because high-pressure hydrogenation is needed during their production, cationic surfactants are often more expensive than anionic surfactants. Due to their high price, they were only used in areas where there was no less expensive alternative, such as (1) as bactericides and (2) as cationic substances that adsorb on anionic substrates to produce hydrophobic effects, which have enormous importance for business in areas like stopping corrosion. Fig. 7 displays several of the cationic surfactants.
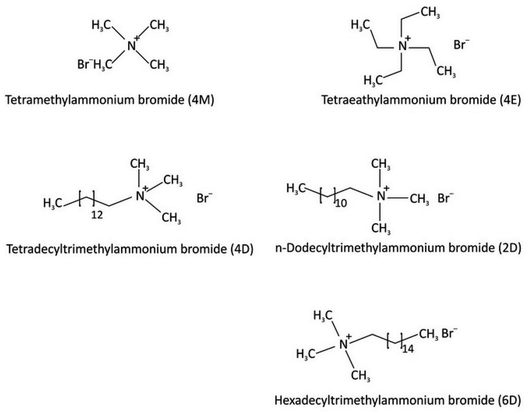 |
| Fig. 7 A number of cationic surfactant structures.57b | |
4.3. Non-ionic surfactants
With a market share of almost 45%, these surfactants account for the majority of the second-most important step in manufacturing. In aqueous solutions like water, alcohol, ether, etc., non-ionic surfactants do not dissociate. By poly-condensing ethylene oxide, which produces hydrophilic polyethylene glycol chains, the majority of polyethoxylated nonionics have been created. Sugar head groups have been used in nonionic surfactants because they have been shown to be less hazardous, taking into account the principles of green chemistry and important characteristics of surfactant biodegradability. Lipophilic groups such as alkylbenzene or the alkyl chain of fatty acids are frequently used. Polymeric chains made from the polycondensation of polyether and propylene oxide as a lipophilic group have also become more important in business. These chains are often called poly-EO-poly-PO copolymers.58aFig. 8 displays several of the nonionic surfactants.
 |
| Fig. 8 The chemical compositions of a few nonionic surfactants.58b | |
4.4. Amphoteric surfactants or zwitterionic surfactants
Another type of surfactant known as zwitterionic surfactants exhibits electrical neutrality as a result of the existence of positively and negatively charged polar head groups in the hydrophilic moiety. The quaternary salt of ammonia is typically the cationic component, whereas the phosphate and sulfonate groups are responsible for the anionic component. The zwitterionic surfactants are used in a variety of cosmetics and home goods.59 Their unique features are due to the doubly polarized head group. The surface area per molecule is improved by a greater charge separation between the molecules, which improves the activity of the surfactant. Examples of common zwitterionic surfactants are betaines and carboxybetaines.60aFig. 9 displays a few of the zwitterionic surfactants.
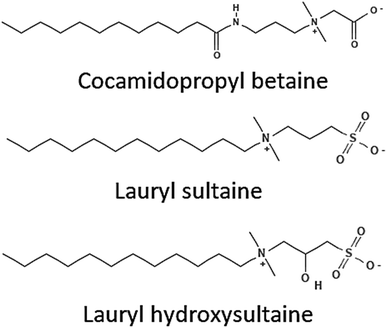 |
| Fig. 9 Structures of some zwitterionic surfactants.53 | |
4.5. Gemini surfactants
A surfactant's structure and design are crucial in establishing its qualities and range of applications. In 1991 and 1993, Menger and his research team found a productive class of surfactants known as gemini or dimeric surfactants.60b,c Gemini surfactants have a different structure than traditional surfactants, which have a head that doesn't like water and a tail that does. Instead, they have two groups that attract both water and oil and are separated by a chain. The length of the chain with a gap (or spacer chain) can range from 2 to 7 methylene groups, with flexible or stiff entities. The water-loving and water-hating properties of the surface-active substances are enhanced by the presence of two polar heads, increasing their total efficacy. A number of gemini surfactants are shown in Fig. 10.
 |
| Fig. 10 Gemini surfactants with cationic structures.60d | |
4.6. Biosurfactants
In light of growing ecological concerns, stricter environmental regulations, and advancements in biotechnology, biosurfactants have emerged as a promising substitute for synthetic surfactants in the market. This is due to their biodegradable nature, low toxicity, and cost-effectiveness.60e Biosurfactants are made from things that are renewable in nature, like vegetation and bacteria.61,62 During the biochemical process of making biosurfactants, microorganisms add oxygen or polar atoms to the carbon–hydrogen bonds of molecules that look like hydrocarbons.45 Nonionic di- or polysaccharide compounds, anionic or cationic amino acids, or peptide-derived products normally make up the hydrophilic groups, whereas hydroxylated, saturated, and/or unsaturated fatty acids and/or water-repelling (or hydrophobic) peptides typically constitute the hydrophobic groups.45,63 Thus, many biosurfactants are recognized as non-ionic or anionic, whereas some are cationic.48b Biosurfactants have a molecular structure that has both hydrophilic and hydrophobic groups (amphiphilic) and lowers surface tension. This lets microbes spread on materials that don't mix with water. Fig. 11 depicts the various sources from which biosurfactants have been derived and then used for further application in various industries.
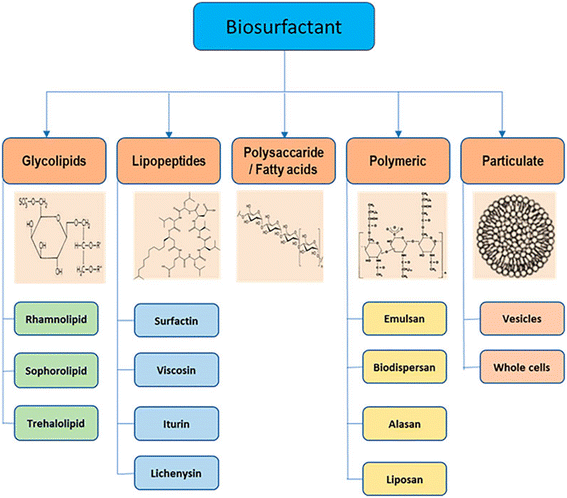 |
| Fig. 11 Some biosurfactants and their sources.65 | |
The main source of rhamnolipid, a well-known biosurfactant, is Pseudomonas aeruginosa.62 A rhamnose or two fragments make up the hydrophilic group, whereas up to 3 hydroxyl fatty acids (varying from 8 to 22 carbon chains long in each) form the hydrophobic group.63 Surfactants having HLB values of 22–24 are classified as rhamnolipids, which are very hydrophilic surfactants.63
Oil spills have a terrible impact on the marine ecosystem and species. Chemically produced surfactants were considered inappropriate for clean-up since they had been shown to be hazardous to aquatic life.64 Biosurfactants' ability to foam makes them useful in a variety of industries, including lowering oil viscosity and using them as detergents to treat petroleum storage containers. The use of biosurfactants in oil recovery processes has drawn the attention of a number of scientists. Although their cost is typically higher than that of chemical surfactants, making EOR less economically attractive, biosurfactants outperform their chemical counterparts in terms of ecological suitability, health effects, and the possibility of being produced from renewable sources.
5. Factors affecting the performance of surfactants
The effectiveness of surfactant-enhanced remediation (SER) depends on many things, like the concentration and/or quantity of surfactants, the temperature, the pH, the amount of inorganic salts, the physicochemical characteristics of the medium, and the quantity and type of oil spillage. These things will affect SER's ability to stop drifting and recover.
As the concentration of surfactants rises, the effectiveness of remediation often rises as well. As the surfactant concentration rises, the surface/interfacial tension falls, and micelles form until it reaches the CMC point, increasing the oil solubility. Because the number of micelles that can form an association due to repulsive forces between head groups is limited, increasing the concentration results in a greater number of micelles.63 So, as the concentration of surfactants goes up, so does their ability to make hydrophobic hydrocarbons more soluble. According to Zhou et al.,66a saponin and Tween 80 concentrations had linearly positive correlations with phenanthrene solubility (WSR = 0.0113 and 0.0312, respectively). Additionally, Lai et al.18 discovered a favorable correlation between rhamnolipids and surfactant content in a range of 0–0.2 percent and the effectiveness of TPH removal from soil. Olasanmi and Thring66b noted that rhamnolipid content increased from 100 to 500 mg L−1 along with the effectiveness of TPH removal from soil. There are, however, some conflicting accounts. The greatest TPH removal effectiveness from soil was attained with 0.004 percent of the surfactants lecithin, aescin, and tannin, and as the surfactant concentration increased, this efficiency decreased.48 It seems that the primary mobilization mechanism that those surfactants perform is that instead of the interfacial tension decreasing, it now increases when the adsorption deficit rises beyond the CMC.67 The enhanced adsorption of organic pollutants in the land caused by the adsorbed surfactants will decrease the effectiveness of remediation.49 As a result, the type of surfactant influences its ideal concentration.
How well and how much it costs to remove oil depends on how much surfactant is issued (i.e., the ratio of water to soil, the dosage, or the time it takes to clean up). As the injection volume of surfactant solution increased, the level of residual pollutants in soil columns steadily dropped.19,50 With a fixed washing time of 24 hours, the removal efficiency of benz[a]anthracene using a soil mixture increased from 25% to 80% as the water/soil concentration increased from 4
:
1 to 36
:
1. Its crude oil level in spilled oil was reduced by employing a synthetic biosurfactant, SWPUEN-1, even as the water/soil proportion and cleaning duration grew and eventually stabilized, exceeding 2.5
:
1 and 120 min, respectively.20 There was no discernible difference in the remediation efficiency for lower and upper oil-polluted sites after 1 day and 7 days of biosurfactants and surfactants in cleaning (with a water/soil proportion of 2
:
1).18 All of this shows that sufficient solution and washing time were required to remove the oil droplets and then for surfactants to remove the oil layer. These outcomes further demonstrate the speed of SER.
The elimination of oil pollutants can be improved by raising the temperature. When the temperature went from 20 °C to 40 °C, the discharge efficiency improvements of aromatic and aliphatic oil pollutants in distinct fragments of land went from 62.41 percent to 81.12 percent to 76.47 percent to 92.34 percent and 72.93 percent to 77.55 percent to 85.25 percent to 92.39 percent, respectively.14 According to research, increasing the temperature (to between 35 and 65 °C) is more successful than lengthening the washing duration for removing TPH from oil-aged soil.46 According to ANOVA findings, heat had the biggest influence, and 23.5 °C was the ideal temperature for TPH extraction from petroleum soil by biosurfactants.66b In a similar manner, another study showed that the best temperature for removing oil from soil with SDS/Tween 80 blended surfactant was 35 °C. As the temperature goes from 25 to 65 °C, the oil removal rate first goes up and then goes down.66c Every surfactant does have a range of temperatures where it works best. When the temperature goes up, the thermal movement of molecules happens faster. This makes the oil less viscous and less sticky, which makes it easier to separate and dissolve.
Studies revealed that anionic surfactants were much more successful in eliminating contaminants when the pH was higher. The percentage removal of both the aromatic and aliphatic portions of petroleum in land by SDS improved as the pH rose from 5.76 to 8.14, respectively, from 45.45 percent to 65.19 percent to 70.38 percent to 87.70 percent and from 65.51 percent to 72.01 percent to 80.75 percent to 83.14 percent.14 SDS was most effective at cleaning up gasoline spills when the pH was between 8 and 10; when the pH reached 11, the high ion concentration limited its efficacy.46 In an alkaline climate, soil electronegativity rises, which reduces anionic surfactant adsorption loss because electrical repulsion is increased. Nonionic biosurfactants, of which saponin is one, are less sensitive to pH variations. However, because glucuronic acid is included in the hydrophilic group of saponin, it functions better in an acidic environment.21 At pH 8.0, the MSR of phenanthrene through saponin is around 28% of what it is at pH 4.0. This is because saponin has a CMC value that goes up when the pH goes up.21 As pH goes up, the net charge will go up, and indeed the repulsive electrostatic impact will become stronger, which apparently makes CMC go up. The performance of surfactants is significantly influenced by salts (or ionic strength). Most of the time, sodium salts are used mostly as an additive to decrease the critical micelle concentration as well as adsorption losses, which increases the hydrophobic basic quantity of micelles.20,21 According to Wei et al.,20 the amount of combined Triton X-100/SDBS blended surfactants decreased from 15.34 to 11.07 mg g−1, and the desorption efficacy of pyrene increased from 40% to over 50% when the saltwater concentration varied from 0 to 1.84 wt percent.
Further research discovered that when MgCl2 and CaCl2 were added, the cleaning effectiveness of benz[a]anthracene through the SDS/Tween 80 combination in land was reduced66c because calcium ions and magnesium ions precipitated anionic agents.66d NaCl, Na2SO4, and notably Na2CO3 increased the effectiveness for pollutants being removed.66c Additionally, Na2SiO3 used to have a greater enhancing impact, increasing the effectiveness of TPH removal from soil by nearly 20%.66e To create bigger micelles that aid in the dissolving of oil, sodium ions will squeeze the electrical double layer (or EDL) and lessen the electrostatic repulsive forces of anionic micelles.63 According to Zhou et al.,66 this electrostatic attraction between the hydrophilic groups in saponin diminished as the concentration of Na+ increased. Additionally, by precipitating Ca2+ and Mg2+, SiO32−, and/or CO32−, you provide alkaline conditions and therefore lessen the dissolution losses of anionic surfactant. As was already noted, anionic surfactants benefit from an alkaline environment.
The effectiveness of remediation is affected by crystallite size (measured by specific surface area, or SSA), cation exchange capacity (CEC), porous structure shape, the amount of fine fractions, and the amount of organic matter (OM). The sequence of the effects of soil parameters was CEC > OM > fine fraction concentration > SSA, according to research employing Triton X-100 to effectively eliminate pollutants in 12 common lands in China (Li et al., 2020). Other research looked at five various soil types and discovered a strong association between CEC, clay concentration, and organic matter content, with correlation values of 0.90, 0.83, and 0.43, respectively.66d Almost all emphasized how closely the CEC concentration and Triton X-100 adsorption loss are connected. The base layer of the entrapped Trion X-100 is thought to be kept on the soil surface's hydrophilic (charged) areas, forming an uneven multi-layer of the entrapped Trion X-100.66d,f Strong autocorrelations were seen between CEC and OM, soil texture, and SSA. As a result, these four variables decrease the effectiveness of remediation and result in a loss of surfactant adsorption.
6. Waste water pollution and its effects
Natural organic matter (NOM), dissolved organic matter (DOM), nutritional ions (phosphate and nitrate), personal care items, medicines, volatile organic compounds (VOCs), and petroleum hydrocarbons are only some of the contaminants that have polluted the DOM. The quality of the water is ruined by these poisonous compounds, rendering it unusable for irrigation and home needs. The possible application of micelles and surfactants in the treatment of wastewater was the main focus of this review. Drinking water may be made from the water below and on the surface of a pool or stream. Therefore, pollutants, both organic and inorganic, might be present in the geographic regions through which water travels. Similar to this, man-made substances or the presence of microbes can taint the water. Until recently, a number of human activities, including sewage treatment, industry, and agricultural runoff, had caused water contamination. The components produced as a result of microbial activity's transformation and the process of decaying organic matter are included in the NOM. These pollutants may exist as dissolved and undissolved organic matter (DOM), which may be differentiated using different separation methods. The term “DOM” refers to organic compounds that may readily flow through a 0.45 m filtration membrane, while particulate organic matter (POM) refers to the material that was left behind on the membrane. The DOM is usually made up of materials from the land and soil, organic chemicals made in a lab, and phytoplankton and algae, among other things. The optical clarity of water is also decreased by the presence of DOM in groundwater due to an increase in turbidity. On the other hand, wastewater also contains a sizable amount of inorganic contaminants such as phosphate, nitrate, and heavy metal ions. The wastewater treatment techniques now in use can only get rid of a tiny portion of these contaminants. When compared to irrigation water from other sources, the level of these major nutrients in treated sewage is still on the higher side. Pesticides have major health risks and are the cause of water pollution in agricultural regions. Similar to this, petrochemicals and hydrocarbons are also prevalent in wastewater as contaminants that pose serious threats to both human and aquatic health.4
Another category of contaminants, known as “emerging pollutants” (EPs), also contributes significantly to water contamination. EPs can develop either spontaneously or artificially during the production of several substances that are not routinely checked for environmental effects. Novel sources of EPs may be created during the preparation of new compounds and after their disposal, respectively. The main sources of these toxins are wastewater treatment plants, large sources of air pollution, waste from crops or animals, and the processes used to make them. These can be divided into several categories, including industrial chemicals, insecticides, dyes, medicines, and byproducts of disinfection. But it is expected that their presence will have a big negative effect on both people and the environment.
More than 700 hazardous substances, including their metabolites and transformation products, have been identified by the WHO as EPs in marine ecosystems. Additionally, the presence of heavy metals and dyes raises severe questions about the water's purity. Mercury (Hg), copper (Cu), arsenic (As), and chromium (Cr) found in water are major pollutants that have been linked to a number of diseases, such as hyperkeratosis, peripheral vascular disease, and various kinds of cancer.24,68a Significant turbidity and coloring in water are also caused by the presence of manganese and iron in higher amounts.68bFig. 12 depicts the spread of different pollutants' life cycles.
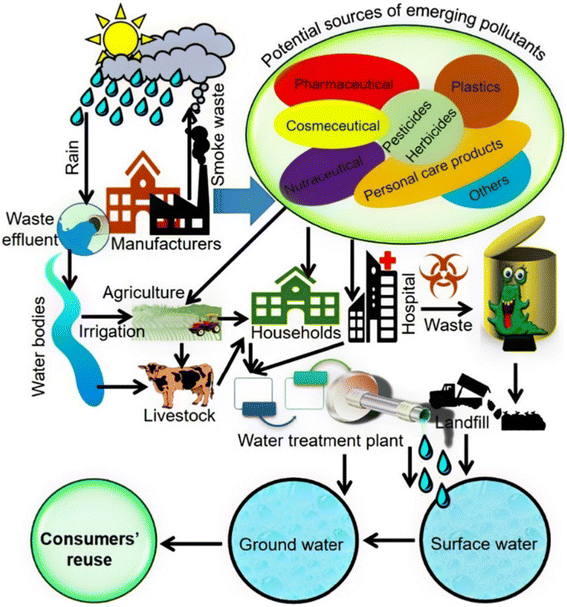 |
| Fig. 12 Image showing the spread and life cycle of new pollutants, from where they come from to where they end up. Possible sources and routes of pollution of ground and surface water by ECs.68c | |
7. Removal of pollutants using surface active agents
Surface-active substances are made up of molecules that have both a head and a tail and are amphiphiles. Polar and nonpolar species are strongly attracted to these molecules. They function in solvents by decreasing their surface tension when they build up on the surface and acting as a conduit between the liquid and the air. While there is no micellar structure below CMC, the micellar aggregates above CMC come in a variety of sizes and forms. The employment of surfactants as a means of eliminating moieties can be used to remove a variety of pollutants of rising concern, including VOCs, medicines and chemicals intended for use on oneself, hazardous metals, organic pollutants, colors, insecticides, and hydrocarbons derived from crude oil. It has already been established that several surfactants may remove these pollutants. Mao et al.69 have employed surfactants in soil remediation. On the other side, Palmer and Hatley39 discussed the use of surfactants in waste water treatment. Tween Crew, BS-400, and 80 Gold were used by Rodriuez-Escales et al.70 to remove a combination of pyrene, phenanthrene, fluorene, and anthracene. For soils containing less than 15% of fine materials, the research team looked at a clearance range of 57 to 99 percent; however, soils containing more than 20% of fine materials had lower removal rates. It was shown that just a few of the mixture's PAHs may interact with the surfactant. As a result, raising the surfactant concentration may not always result in more PAH elimination. The elimination of PAHs is better when biodegradation and desorption are used together as opposed to methods such as “pump and treat,” which divide cleanup and soaking up. Similar surface-active substances were also employed for soil desorption of pyrene with various particle sizes. Surfactant and material addition rates should be tightly correlated with one another.
7.1. Removal of volatile organic components
Pollutants that are known to cause infertility, respiratory problems, and mutations include VOCs such as ethylbenzene, benzene, xylene, toluene, polychloroethylenes, polychloromethanes, and polychloroethanes. A challenging category of solvents comprises chlorinated organic compounds, namely trichloroethylene (TCE) and tetrachloroethylene (PCE). The compounds in question are frequently utilised and exhibit characteristics such as limited solubility in water, low potential for biodegradation, and a density exceeding that of water.71 VOCs are created by a wide variety of biological and abiotic processes. By inciting a number of photochemical processes, they are also to blame for the development of smog.72 Numerous businesses continue to use and produce VOCs despite knowledge of their negative impacts on the environment and the health of living things. Decontaminating polluted soil and wastewater is crucial to preventing VOCs from evaporating into the atmosphere. There are several techniques used to provide a VOC-free environment, including biological, physical, and chemical ones.73 The surfactant-based absorption approach for VOCs is the most effective removal technique being considered out of all the alternatives.
7.2. Removal of pharmaceuticals and personal care products by surfactants
A sizable category of developing contaminants consists of pharmaceuticals and personal care products (PPCPs).74 Due to their wide environmental footprint, high production, and high consumption, these items are significant. They might permeate into the soil and travel to water reservoirs if they are improperly removed from waste water.75 PPCPs are biologically active substances that may accumulate and remain in living things, causing major health risks as well as being hazardous to the environment. Since they have estrogenic actions, they are also known as endocrine disruptors.76a Pharmaceuticals include drugs with more than 3000 distinct active ingredients that are used to treat ailments in both people and animals.76b Either the original molecules of pharmaceuticals or their metabolites are released into the environment. A broad variety of chemicals, including parabens, triclosan, benzophenones, and bisphenols, are also included in personal-care items. Detergents, plastics, cleaners, toothpaste, sunscreens, shampoos, conditioners, lotions, preservatives, and a few more items that are discharged into the environment unaltered all include them.76a Due to their slow disintegration, traditional treatment facilities were unable to effectively remove PPCPs from sewage, necessitating the deployment of advanced technologies.75 Surfactants have been demonstrated to be effective in removing PPCPs. The necessity of incorporating surfactants into emulsion-liquid membranes (ELM) to remove PPCPs from wastewater is growing.
This technology provides the foundation for selective permeability of solutes via membranes. It was made up of emulsion globules with the aqueous phase as the exterior phase and an internal phase confined inside a membrane phase that only allowed certain solutes from the internal phase to pass through. Surfactants in ELM play a role in improving the stability of the emulsion to prevent membrane rupture. Surfactants also prevent emulsion leakage.76c,d Daas and his colleague76d used an emulsion-liquid membrane with SPAN 80 as a surfactant, hexane (diluent), and Na2CO3 to remove more than 90% of ketoprofen and ibuprofen from mineral, pure, and seawater (internal phase). An inner aqueous solution of potassium chloride and the surfactant Span 80 were used in a different investigation to remove paracetamol with ELM.77 SPAN 80 (sorbitan monooleate) has been shown in numerous studies to be the most effective surface-active substance for removing PPCPs using the ELM method.77–79 The optimal roles for kerosene and hexane were in the membrane phase or as diluents along with surfactants.
7.3. Toxic metal removal using surfactants
Heavy metals in the water supply can have harmful, long-lasting consequences on humans, animals, plants, aquatic life, and microbes. The primary industries responsible for heavy metal contamination are those that produce fertilizer, pesticides, leather, pharmaceuticals, and metals. The adulteration of heavy metals is also a result of weathering, erosion, and fuel combustion.80,81 The United States Environmental Protection Agency (USEPA) lists thirteen distinct metals as the most persistent pollutants.82 Metals such as Pb, Cu, Zn, As, Cr, Ni, Cd, and Hg are examples. These metals are very poisonous and carcinogenic, and they are also easy to dissolve in water and mobile, both free and bound. Metal pollution hinders the biodegradation of organic pollutants, which has an impact on both the ecological and physiological factors of microorganisms and lowers the sustainability of soil quality.82 Researchers have explored the utilization of surfactants in getting rid of heavy metals using a variety of techniques, including soil-washing, extraction, desorption, and phytoremediation. Surfactants have shown capability in the ultrafiltration process as well. This capacity may be improved by pretreating the membrane with surfactants or biosurfactants to remove metal ions. Similar to regular carbon, surfactant-modified activated carbon showed that it could remove metal ions 2–4 times better than regular carbon.83 Biosurfactants are used more and more to get rid of metals because they have great qualities like low toxicity, strong biocompatibility, improved degradation, outstanding improvement in foaming, and stability across a broad pH and temperature range.
7.4. Metal contaminated soil remediation by surfactants
Surfactants have a well-known role in soil washing to remove metals. By rinsing the dirt with clean water and various chelating agents, chemical additives, and solvents, toxic metals can be eliminated. The main restrictions are, however, the length of the treatment and the poor availability due to contact with soil particles. Surfactants reduced the amount of time needed and increased the efficacy of the methods used in this situation. The cleaning of dirt using surfactants may comprise ex situ or in situ treatments. Ex situ treatment involves digging up the soil and placing it in the right location, then cleaning the area with a biosurfactant solution. In contrast, in in situ use, soil is first treated with a surface-active substance, then correspondingly complexed with metal ions that have different charges, and finally moved as a result of lower interfacial tension. Micelles employ electrostatic contact, while charged surfactants use an ion exchange process.84 Metals may be recycled and permanently removed from the environment with minimal time investment.85 The use of 11 different types of surfactants has been investigated to remediate soil that has been heavily contaminated with various metals such as Cd, Ni, Cu, As, Zn, and Pb discarded from manufacturing.
7.5. Removal of pesticides by surfactants
The application of pesticides results in eliminating or preventing pests, as well as a great number of other advantages. The contemporary agricultural sector's overuse of pesticides has led to a surge in their concentration levels in water bodies. It is imperative to conduct a comprehensive examination of the influent composition and identify the optimal removal methodology in order to develop a water treatment facility that effectively addresses diverse pesticide types and achieves the highest attainable removal rate.86 They do have downsides, too, most notably hazardous contaminants, which must be disregarded. Because they are poorly soluble in water, many popular pesticides—the name for substances used to kill or control pests—are exceedingly difficult to remove from contaminated soils. So the remediation method, including the use of a surfactant, could be beneficial. Because it only kills plants that are unable to digest it, bentazone is a selective herbicide for this usage. According to one study, three alkyl polyoxyethylene surfactants with the same water-resistant chain but distinct oxyethylene groups were used to study soil that had been contaminated with bentazone with the intention of cleaning the soil.87 The pesticide recovery was improved with these surfactants. The remaining bentazone was deactivated using a photocatalytic process that involved treating wastes with TiO2 particles that were exposed to simulated sunlight for a certain amount of time to break down the bentazone. For removing bentazone from waste materials, Brij was thought to stand out as an efficient surface-active substance with a quick cut in time.87 Due to being resistant to living things, not absorbing well, and being soluble on the soil surface, hexachlorocyclohexane (HCH) bioremediation is constrained. Since the HCH isomers are soluble, bioavailable, and biodeactivated, which are indicators of the surfactant's effectiveness, it is possible to improve HCH biodegradation by utilizing biosurfactants. Rhamnolipid, sophorolipid, and trehalose lipid were among the biosurfactants whose effects were investigated. The solubility of the HCH isomers rises significantly when sophorolipid and rhamnolipid are used, with a maximum yield of the isomers at a concentration of 40 g mL−1, according to the results. In contrast, trehalose-containing lipids produced their greatest amount of solubility at 60 g mL−1. Sophorolipid had the greatest HCH isomer elimination in the soil out of the three surfactants tested.87 A naturally occurring contaminant utilized in pesticides is tributyltin (TBT, an organotin). Contamination needs to be cleaned up due to its hazardous consequences.88
7.6. Removal of petroleum hydrocarbons using surfactants
Some naturally occurring contaminants that are exceedingly difficult to remove include petroleum hydrocarbons (PAHs). They must be completely eliminated from the soil since they are carcinogenic. Despite the fact that petroleum products are an essential component of our energy supply, soil pollution is a result of leaks and unintentional spills. Temporary remedies, such as mechanical and burial approaches, are being used to combat their contamination. For removing petroleum pollutants from soil, surfactants are a viable choice. The hydrocarbon removal efficiency of anionic surfactants is higher than that of non-ionic surfactants. The preferential desorption on distinct hydrocarbons like aliphatic hydrocarbons and larger chain length exhibited greater resistance to degradation compared to other petrol and diesel fuel components.89 Khalladi et al. conducted research on the soil washing of diesel pollution using anionic surfactants and SDS.90 Above an SDS concentration of 8 mM, the effect was deemed suitable. Diesel concentration remained consistent even after a 4 hour soil treatment with a surfactant solution, demonstrating that a precise amount of time is necessary for the surfactant to interact with the soil most effectively. By maintaining the rate at 3.2 mL min−1, 97 percent of the soil pollution from diesel fuel was eliminated. SDS and artificial surfactants continue to be biodegradable. So, the removal capacity of SDS was contrasted with that of biosurfactants such as rhamnolipid and saponin. When rhamnolipid and saponin were compared to SDS, it was found that SDS was better at getting crude oil out of the soil. But the amount of connection between different surfactants and different parts of crude oil can be different. For example, SDS is better at getting rid of aliphatic hydrocarbons, while saponin may be better at dealing with aromatic hydrocarbons.48
8. Present challenges
Here, strenuous efforts are being made to purify wastewater of potentially harmful substances. Traditional water/wastewater remediation methods are restricted in their applicability due to issues like high cost, incompatibility, lack of versatility, and environmental risks associated with the instantaneous removal of various pollutants. Due to their distinct physicochemical features, surfactants are chemicals that are used in a wide variety of consumer and commercial products. They are of major relevance to environmental sustainability and healthy ecosystems as they are adsorbed in the groundwater if used excessively. Some of the surfactants are non-biodegradable and not environmentally friendly and hence, there is a need to search for alternatives that are biodegradable, economically feasible and ecofriendly.
9. Conclusion and future perspectives
Surfactants are now used in many ex situ and in situ cleanup methods, such as pump and treat, air sparging, bioremediation, and in situ chemical oxidation. Surfactants can help oil pollutants that don't dissolve in water move into water, which makes it easier for water-based cleaning agents to get to them. This decreases the consequences of tailing and rebound and makes mitigation work better. Conventional remediation methods have some problems, such as high costs, problems with compatibility, a lack of ability to adapt, and their own environmental threats for the real-time elimination of organic, inorganic, and metal-based pollutants. This makes it hard to use them in a wide range of fields to clean up the environment. Many surfactants have shown promise in decontaminating soil and wastewater from medicines, heavy metals, personal care items, and colors. The employment of remediation technology based on surfactants can readily respond to these accusations while still complying with strict environmental standards. Bio-surfactants, which are environmentally friendly, have gained some application for the purpose, but their high prices make large-scale use difficult. These remediation solutions can meet the systems' needs for integrity and stability in the future to make the environment sustainable for the upcoming generation.
Conflicts of interest
The authors declare that they have no known competing financial interests or personal relationships that could have appeared to influence the work reported in this paper.
Acknowledgements
The authors are grateful to the R&D Cell and School of Basic Sciences & Technology, IIMT University, Meerut, Uttar Pradesh, India for their support.
References
- M. S. Nazir, A. J. Mahdi, M. Bilal, H. M. Sohail, N. Ali and H. M. Iqbal, Sci. Total Environ., 2019, 15(683), 436–444 CrossRef PubMed
.
- L. Liu, M. Bilal, X. Duan and H. M. Iqbal, Sci. Total Environ., 2019, 1(667), 444–454 CrossRef PubMed
.
- N. Ali, A. Khan, M. Bilal, S. Malik, S. Badshah and H. M. Iqbal, Mol, 2020, 6, 226 CrossRef PubMed
.
- T. Rasheed, M. Bilal, A. A. Hassan, F. Nabeel, R. N. Bharagava, L. F. Ferreira, H. N. Tran and H. M. Iqbal, Environ. Res., 2020, 185, 109436 CrossRef CAS PubMed
.
- H. Khan, K. Gul, B. Ara, A. Khan, N. Ali, N. Ali and M. Bilal, J. Environ. Chem. Eng., 2020, 8, 103927 CrossRef CAS
.
- A. Zahid, A. Lashin, U. A. Rana, N. Al-Arifi, I. Ullah, D. D. Dionysiou, R. Qureshi, A. Waseem, H. B. Kraatz and A. Shah, Electrochim. Acta, 2016, 190, 1007–1014 CrossRef CAS
.
- J. Li, Y. Li, Q. Zhang, Y. Song and Z. Wang, Tenside, Surfactants, Deterg., 2020, 57(1), 74–81 CrossRef CAS
.
- S. P. Verma and B. Sarkar, J. Water Process. Eng., 2020, 33, 101048 CrossRef
.
- J. Huang, L. Peng, G. Zeng, X. Li, Y. Zhao, L. Liu, F. Li and Q. Chai, Sep. Purif. Technol., 2014, 125, 83–89 CrossRef CAS
.
- R. Mielke, L. Huo, G. Liu, X. Yang, Z. Ahmad and H. Zhong, Chemosphere, 2020, 252, 126620 CrossRef PubMed
.
- C. Hall, P. Tharakan, J. Hallock, C. Cleveland and M. Jefferson, Nature, 2003, 426(6964), 318–322 CrossRef CAS PubMed
.
- C. R. Azevedo, Eng. Failure Anal., 2007, 14, 978–994 CrossRef CAS
.
- H. Talvenmäki, N. Lallukka, S. Survo and M. Romantschuk, Environ. Sci. Pollut. Res., 2019, 26, 34670–34684 CrossRef PubMed
.
- S. Gitipour, M. Hedayati and E. Madadian, Clean: Soil, Air, Water, 2015, 43, 1419–1425 CAS
.
- P. Logeshwaran, M. Megharaj, S. Chadalavada, M. Bowman and R. Naidu, Environ. Technol. Innovation, 2018, 10, 175–193 CrossRef
.
- M. T. Alcántara, J. Gómez, M. Pazos and M. A. Sanromán, J. Hazard. Mater., 2009, 166, 462–468 CrossRef PubMed
.
- M. Davin, A. Starren, M. Deleu, G. Lognay, G. Colinet and M. L. Fauconnier, Chemosphere, 2018, 194, 414–421 CrossRef CAS PubMed
.
- C. C. Lai, Y. C. Huang, Y. H. Wei and J. S. Chang, J. Hazard. Mater., 2009, 167(1–3), 609–614 CrossRef CAS PubMed
.
- G. Pei, Y. Zhu, X. Cai, W. Shi and H. Li, Chemosphere, 2017, 185, 1112–1121 CrossRef CAS PubMed
.
- W. Wei, Z. Ran, H. He, K. Zhou, Z. Huangfu and J. Yu, Chemosphere, 2020, 254, 126854 CrossRef CAS PubMed
.
- W. Zhou, J. Yang, L. Lou and L. Zhu, Environ. Pollut., 2011, 159, 1198–1204 CrossRef CAS PubMed
.
- A. A. Befkadu and C. H. Quanyuan, Pedosphere, 2018, 28, 383–410 CrossRef CAS
.
- A. Karthick, B. Roy and P. Chattopadhyay, J. Environ. Manage., 2019, 243, 187–205 CrossRef CAS PubMed
.
- A. Shah, S. Shahzad, A. Munir, M. N. Nadagouda, G. S. Khan, D. F. Shams, D. D. Dionysiou and U. A. Rana, Chem. Rev., 2016, 116, 6042–6074 CrossRef CAS PubMed
.
- S. Sun, Y. Chen, Y. Lin and D. An, Sci. Total Environ., 2018, 639, 1–7 CrossRef CAS PubMed
.
- S. Mansilla, J. Portugal, J. M. Bayona, V. Matamoros, A. M. Leiva, G. Vidal and B. Pina, J. Environ. Chem. Eng., 2021, 9, 105198 CrossRef CAS
.
- T. Deblonde, C. Cossu-Leguille and P. Hartemann, Int. J. Hyg. Environ. Health, 2011, 214, 442–448 CrossRef CAS PubMed
.
- A. Gogoi, P. Mazumder, V. K. Tyagi, G. T. Chaminda, A. K. An and M. Kumar, Groundw. Sustain. Dev., 2018, 6, 169–180 CrossRef
.
- S. Zandaryaa and D. Frank-Kamenetsky, Water Int., 2021, 46, 195–210 CrossRef
.
- P. Chaturvedi, P. Shukla, B. S. Giri, P. Chowdhary, R. Chandra, P. Gupta and A. Pandey, Environ. Res., 2021, 194, 110664 CrossRef CAS PubMed
.
- A. Majumder and A. K. Gupta, J. Environ. Chem. Eng., 2021, 9, 106496 CrossRef CAS
.
- M. T. Khan, I. A. Shah, I. Ihsanullah, M. Naushad, S. Ali, S. H. Shah and A. W. Mohammad, J. Water Process. Eng., 2021, 41, 101990 CrossRef
.
- S. Polarz, M. Kunkel, A. Donner and M. Schlötter, Chem.–Eur. J., 2018, 24, 18842–18856 CrossRef CAS PubMed
.
- D. Mitru, I. Lucaciu, M. Nita-Lazar, C. I. Covaliu, G. Nechifor, I. C. Moga, B. Marin and I. Paun, Rom. J. Ecol. Environ. Chem., 2020, 2, 210–220 Search PubMed
.
- Z. U. Haq, N. Rehman, F. Ali, N. M. Khan and H. Ullah, J. Mater. Environ. Sci., 2017, 8, 1029–1038 Search PubMed
.
- R. F. Nunes and A. C. Teixeira, Chemosphere, 2022, 300, 134507 CrossRef CAS PubMed
.
- S. M. Shaban, J. Kang and D. H. Kim, Compos. Commun., 2020, 22, 100537 CrossRef
.
- S. P. Chaudhari and R. P. Dugar, J. Drug Delivery Sci. Technol., 2017, 41, 68–77 CrossRef CAS
.
- M. Palmer and H. Hatley, Water Res., 2018, 147, 60–72 CrossRef CAS PubMed
.
- A. A. Siyal, M. R. Shamsuddin, A. Low and N. E. Rabat, J. Environ. Manage., 2020, 254, 109797 CrossRef CAS PubMed
.
- S. A. Mousavi and F. Khodadoost, Environ. Sci. Pollut. Res., 2019, 26, 26439–26448 CrossRef CAS PubMed
.
- F. Xiao, Water Res., 2017, 124, 482–495 CrossRef CAS PubMed
.
- W. De Bruin, Q. Kritzinger, R. Bornman and L. Korsten, Ecotoxicol. Environ. Saf., 2019, 181, 419–427 CrossRef CAS PubMed
.
- D. Cierniak, M. Woźniak-Karczewska, A. Parus, B. Wyrwas, A. P. Loibner, H. J. Heipieper, Ł. Ławniczak and Ł. Chrzanowski, Appl. Microbiol. Biotechnol., 2020, 104, 1–2 CrossRef CAS PubMed
.
-
M. J. Rosen and J. T. Kunjappu, Surfactants and Interfacial Phenomena, John Wiley & Sons, 2012 Search PubMed
.
- M. Cheng, G. Zeng, D. Huang, C. Yang, C. Lai, C. Zhang and Y. Liu, Chem. Eng. J., 2017, 314, 98–113 CrossRef CAS
.
- T. Kobayashi, H. Kaminaga, R. R. Navarro and Y. Iimura, J. Environ. Sci. Health, Part A: Toxic/Hazard. Subst. Environ. Eng., 2012, 47, 1138–1145 CrossRef CAS PubMed
.
-
(a) K. Urum and T. Pekdemir, Chemosphere, 2004, 57, 1139–1150 CrossRef CAS PubMed
;
(b) A. A. Befkadu and C. H. Quanyuan, Pedosphere, 2018, 28, 383–410 CrossRef CAS
.
- W. Zhou and L. Zhu, Environ. Pollut., 2007, 47, 350–357 CrossRef PubMed
.
- W. Zhou and L. Zhu, Water Res., 2008, 42, 101–108 CrossRef CAS PubMed
.
- W. Rongsayamanont, C. Tongcumpou and N. Phasukarratchai, Water, Air, Soil Pollut., 2020, 231, 1–10 CrossRef
.
- G. Javanbakht and L. Goual, Ind. Eng. Chem. Res., 2016, 55, 11736–11746 CrossRef CAS
.
-
N. Saxena and A. Mandal, Natural Surfactants, in Natural Surfactants, Springer, Cham, 2022, pp. 19–24 Search PubMed
.
- N. Saxena, N. Pal, S. Dey and A. Mandal, J. Taiwan Inst. Chem. Eng., 2017, 81, 343–355 CrossRef CAS
.
- S. Kumar, A. Kumar and A. Mandal, AIChE J., 2017, 63, 2731–2741 CrossRef CAS
.
- T. Benvegnu, D. Plusquellec and L. Lemiègre, Polym. Renewable Resour., 2008, 1, 153–178 Search PubMed
.
-
(a) F. Goursaud, M. Berchel, J. Guilbot, N. Legros, L. Lemiègre, J. Marcilloux, D. Plusquellec and T. Benvegnu, Green Chem., 2008, 10, 310–320 RSC
;
(b) B. Rafiei and F. A. Ghomi, J. Crystallogr. Mineral., 2013, 21, 25–32 CAS
.
-
(a) M. Zhang, J. Gu, X. Zhu, L. Gao, X. Li, X. Yang, Y. Tu and C. Y. Li, Polymer, 2017, 130, 199–208 CrossRef CAS
;
(b) Z. Chu, Y. Feng, X. Su and Y. Han, Langmuir, 2010, 26, 7783–7791 CrossRef CAS PubMed
.
- Z. Chu, Y. Feng, X. Su and Y. Han, Langmuir, 2010, 26, 7783–7791 CrossRef CAS PubMed
.
-
(a) D. W. Tondo, E. C. Leopoldino, B. S. Souza, G. A. Micke, A. C. Costa, H. D. Fiedler, C. A. Bunton and F. Nome, Langmuir, 2010, 26, 15754–15760 CrossRef CAS PubMed
;
(b) F. M. Menger and C. A. Littau, J. Am. Chem. Soc., 1991, 113, 1451–1452 CrossRef CAS
;
(c) F. M. Menger and C. A. Littau, J. Am. Chem. Soc., 1993, 115, 10083–10090 CrossRef CAS
;
(d) M. Pisárčik, J. Jampílek, M. Lukáč, R. Horáková, F. Devínsky, M. Bukovský, M. Kalina, J. Tkacz and T. Opravil, Molecules, 2017, 22, 1794 CrossRef PubMed
;
(e) H. Aboelkhair, P. Diaz and A. Attia, J. Pet. Sci. Eng., 2022, 216, 110796 CrossRef CAS
.
- O. Iglesias, M. A. Sanromán and M. Pazos, Ind. Eng. Chem. Res., 2014, 53, 2917–2923 CrossRef CAS
.
- T. T. Nguyen, N. H. Youssef, M. J. McInerney and D. A. Sabatini, Water Res., 2008, 42, 1735–1743 CrossRef CAS PubMed
.
- R. Jahan, A. M. Bodratti, M. Tsianou and P. Alexandridis, Adv. Colloid Interface Sci., 2020, 275, 102061 CrossRef CAS PubMed
.
- A. P. Karlapudi, T. C. Venkateswarulu, J. Tammineedi, L. Kanumuri, B. K. Ravuru, V. Ramu Dirisala and V. P. Kodali, Petroleum, 2018, 4, 241–249 CrossRef
.
- J. Sharma, D. Sundar and P. Srivastava, Front. Mol. Biosci., 2021, 8, 727070 CrossRef CAS PubMed
.
-
(a) W. Zhou, J. Yang, L. Lou and L. Zhu, Environ. Pollut., 2011, 159, 1198–1204 CrossRef CAS PubMed
;
(b) I. O. Olasanmi and W. T. Ronald, J. Adv. Res., 2020, 21, 79–90 CrossRef CAS PubMed
;
(c) L. Wang, F. Li, Y. Zhan and L. Zhu, Environ. Sci. Pollut. Res., 2016, 23, 14451–14461 CrossRef CAS PubMed
;
(d) P. Wang and A. A. Keller, Environ. Sci. Technol., 2008, 42, 3381–3387 CrossRef CAS PubMed
;
(e) J. Niu, L. Qi, J. Lv and B. Peng, J. Pet. Sci. Eng., 2020, 192, 107350 CrossRef CAS
;
(f) X. Li, B. Wu, Q. Zhang, Y. Liu, J. Wang, D. Xu, F. Li, F. Ma and Q. Gu, Environ. Sci. Pollut. Res., 2020, 27, 23323–23330 CrossRef CAS PubMed
.
- S. Zhang, G. Mao, J. Crittenden, X. Liu and H. Du, Water Res., 2017, 119, 114–125 CrossRef CAS PubMed
.
-
(a) T. Rasheed, M. Bilal, F. Nabeel, M. Adeel and H. M. Iqbal, Environ. Int., 2019, 122, 52–66 CrossRef CAS PubMed
;
(b)
A. Gomez-Caminero, P. D. Howe, M. Hughes, E. Kenyon, D. R. Lewis, M. Moore, A. Aitio, G. C. Becking and J. Ng, Arsenic and Arsenic Compounds, World Health Organization, 2001 Search PubMed
;
(c) T. Rasheed, M. Bilal, F. Nabeel, M. Adeel and H. M. N. Iqbal, Environ. Int., 2019, 122, 52–66 CrossRef CAS PubMed
.
- X. Mao, R. Jiang, W. Xiao and J. Yu, J. Hazard. Mater., 2015, 285, 419–435 CrossRef CAS PubMed
.
- P. Rodríguez-Escales, E. Borràs, M. Sarrà and A. Folch, Water, Air, Soil Pollut., 2013, 224, 1–2 CrossRef
.
- L. M. Vane and E. L. Giroux, J. Chem. Eng. Data, 2000, 45, 38–47 CrossRef CAS
.
- Y. Tu, C. Yang, Y. Cheng, G. Zeng, L. Lu and L. Wang, Bioresour. Technol., 2015, 175, 231–238 CrossRef CAS PubMed
.
- A. Erto and A. Lancia, Chem. Eng. J., 2012, 187, 166–171 CrossRef CAS
.
- H. P. Arp, Environ. Sci. Technol., 2012, 46, 4259–4260 CrossRef CAS PubMed
.
- S. K. Khetan and T. J. Collins, Chem. Rev., 2007, 107, 2319–2364 CrossRef CAS PubMed
.
-
(a) J. M. Brausch and G. M. Rand, Chemosphere, 2011, 82, 1518–1532 CrossRef CAS PubMed
;
(b) S. D. Richardson and T. A. Ternes, Anal. Chem., 2014, 86, 2813–2848 CrossRef CAS PubMed
;
(c) Y. S. Ng, N. S. Jayakumar and M. A. Hashim, J. Hazard. Mater., 2010, 184, 255–260 CrossRef CAS PubMed
;
(d) A. Dâas and O. Hamdaoui, Environ. Sci. Pollut. Res., 2014, 21, 2154–2164 CrossRef PubMed
.
- S. Chaouchi and O. Hamdaoui, Sep. Purif. Technol., 2014, 129, 32–40 CrossRef CAS
.
- S. Chaouchi and O. Hamdaoui, J. Ind. Eng. Chem., 2015, 22, 296–305 CrossRef CAS
.
- S. Chaouchi and O. Hamdaoui, Desalin. Water Treat., 2016, 57, 5253–5257 CrossRef CAS
.
- K. E. Giller, E. Witter and S. P. Mcgrath, Soil Biol. Biochem., 1998, 30, 1389–1414 CrossRef CAS
.
- L. A. Sarubbo, R. B. Rocha Jr, J. M. Luna, R. D. Rufino, V. A. Santos and I. M. Banat, Chem. Ecol., 2015, 31, 707–723 CrossRef CAS
.
- T. R. Sandrin and R. M. Maier, Environ. Health Perspect., 2003, 11, 1093–1101 CrossRef PubMed
.
- I. Xiarchos, D. Doulia, V. Gekas and G. Trägårdh, Sep. Purif. Rev., 2003, 32, 215–278 CrossRef CAS
.
- C. N. Mulligan, Curr. Opin. Colloid Interface Sci., 2009, 14, 372–378 CrossRef CAS
.
- G. Dermont, M. Bergeron, G. Mercier and M. Richer-Laflèche, J. Hazard. Mater., 2008, 152, 1–31 CrossRef CAS PubMed
.
- I. A. Saleh, N. Zouari and M. A. Al-Ghouti, Environ. Technol. Innovation, 2020, 19, 01026 Search PubMed
.
- M. Davezza, D. Fabbri, E. Pramauro and A. B. Prevot, Chemosphere, 2012, 86, 335–340 CrossRef CAS PubMed
.
- L. Mathurasa, C. Tongcumpou, D. A. Sabatini and E. Luepromchai, Int. Biodeterior. Biodegrad., 2012, 75, 7–14 CrossRef CAS
.
- E. Ritoré, B. Coquelet, C. Arnaiz, J. Morillo and J. Usero, Environ. Sci. Pollut. Res., 2022, 1, 1–3 Search PubMed
.
- R. Khalladi, O. Benhabiles, F. Bentahar and N. Moulai-Mostefa, J. Hazard. Mater., 2009, 164, 1179–1184 CrossRef CAS PubMed
.
|
This journal is © The Royal Society of Chemistry 2023 |
Click here to see how this site uses Cookies. View our privacy policy here.