Development of a portable, microwell-based, smartphone-assisted colorimetric device to measure the activities of anaerobic digestion†
Received
9th September 2023
, Accepted 14th November 2023
First published on 14th November 2023
Abstract
Anaerobic digestion (AD) is a complex process that can be severely impacted by a range of toxicants found in wastewater, leading to system failure. To prevent this, monitoring key process indicators is crucial, but current methods have limitations in terms of response time and reliability. To address this challenge, we propose an innovative solution for ex situ monitoring of biotoxicity in AD using a paper-microwell-based and smartphone-assisted colorimetric analytical device. This device utilizes resazurin reduction by anaerobic sludge as a biological indicator, which reflects the health of anaerobic microbial consortia with a short response time. The device is fabricated through plasma treatment of chromatography paper, integrated with resazurin, and requires no external power source. To evaluate the device's efficiency, we conducted tests to measure and differentiate the toxicity of three types of chlorophenols, namely pentachlorophenol, 2,4-dichlorophenol, and 4-chlorophenol, after loading them into AD for a duration of 30 min. This paper-microwell-based analytical device demonstrated its ability to identify the presence of toxicants and provide a quick response time, making it a promising technology for monitoring toxicity incidents in real-time in AD processes. With its low cost, portability, and reliability, this innovative technology has the potential to contribute to the prevention of system failure caused by toxicants in AD processes. The analytical greenness metric approach (AGREE) proposes this paper-based analytical device as a greener alternative for measuring anaerobic digestion activities compared to traditional methods such as biochemical methane potential and chemical oxygen demand.
Environmental significance
Our proposed paper-microwell-based and smartphone-assisted colorimetric analytical device presents significant environmental implications for anaerobic digestion process, which is considered as one of the core parts of today's sustainable development technology for the centralized treatment of organic waste and the ability to reduce the escape of greenhouse gases into the atmosphere. Anaerobic digestion, a complex wastewater treatment process, is vulnerable to toxicants that can result in system failure. Current monitoring methods have limitations in terms of response time and reliability. Our innovative solution addresses this challenge by enabling in situ monitoring of biotoxicity in anaerobic digestion. The device utilizes resazurin reduction by anaerobic sludge as a biological indicator, reflecting the health of microbial consortia with a short response time. By successfully differentiating the toxicity of specific chlorophenols, the device demonstrates its ability to identify toxicants and provide a real-time response. With its low cost, portability, and reliability, this technology has the potential to prevent system failure caused by toxicants, enhancing the environmental performance of the anaerobic digestion process.
|
1. Introduction
In the context of carbon neutrality, anaerobic digestion (AD) is considered as one of the core parts of today's sustainable development technology for the centralized treatment of organic waste and the ability to reduce the escape of greenhouse gases into the atmosphere.1 AD comprises three typical steps, namely hydrolysis, acetogenesis and methanogenesis.2 The microorganisms involved in these steps are mutually dependent,3 and toxicants, such as heavy metals, organic pollutants, and antibiotics, present at inhibitory concentrations in wastewater can inhibit the growth and activity of specific microbial populations, leading to a decrease in biogas production and even system failure.4 The complexity of anaerobic sludge presents a challenge for monitoring and controlling the anaerobic digestion process.
Parameters such as volatile acids (VFAs), chemical oxygen demand (COD-soluble and total), gas flow and composition, pH, microbial ecology, and generic microbial toxicity are crucial for AD performance and can be correlated to control.5 However, monitoring these parameters post-event or with measurement delays can lead to difficulties in preventing system failure. Therefore, there is a need for quick measurement of key process indicators to detect toxicity in advance. Several bioassays have been developed for toxicity detection in AD, such as the luminescent bacteria toxicity assay,6 titration bioassay,7 and biosensors.8 However, most of these methods have slow response times to toxicants,9 making them unreliable for real-time monitoring of overload and toxicity incidents in AD processes.10
Resazurin reduction assay is a nontoxic biological indicator11 that has multipurpose application,12 including in mammalian cell,13 fungi,14 and bacteria15 viability assays. It has the potential to reflect the health of anaerobic microbial consortium with short response times.16 While the addition of anaerobic sludge samples with resazurin has been tested in a microplate reader to measure the activity of anaerobic digestion,16–19 it has been found that this instrument is unsuitable for ex situ monitoring of biotoxicity in AD. Although a portable resazurin reduction-based biosensor device has been proposed for anaerobic biotoxicity detection, additional driving forces, such as various pumps, make the portability of this instrument not so convenient.20,21
Paper-based analytical devices have gained widespread popularity due to their convenience,22 low cost,23 and versatility.24,25 In this study, we present a paper-microwell-based and smartphone-assisted colorimetric analytical device for resazurin reduction by anaerobic sludge as a portable method for measuring biotoxicity in AD. The paper microwell was fabricated with plasma treatment on chromatography paper. Integrated with resazurin, this device was successfully applied to measure the biotoxicity of pentachlorophenol (PCP), 2,4-dichlorophenol (2,4-DCP) and 4-chlorophenol (4-CP) to AD. The simplicity and low cost of fabrication, coupled with the flexibility and versatility of the format, make this device an attractive and easy-to-use analytical tool for portable screening methods for anaerobic activity measures.
2. Materials and method
2.1 Chemicals and materials
Alkyl ketene dimer (AKD) 90%, heptane, and beef extract powder were purchased from Dieckmann, HK. Resazurin sodium salt (90%) was purchased from J&K Scientific, China. PCP, 2,4-DCP, 4-CP were purchased from Sigma-Aldrich, HK. Whatman 1 chromatography paper (WCP) was purchased from Whatman, HK. COD analyzer was purchased from Hach. The plasma cleaner was from Harrick Plasma, PDC-32G-2. The metal mask was designed by AutoCAD 2019 and manufactured by Taobao. The seed anaerobic sludge was collected from Tai Po Sewage Treatment Works and was cultured in an anaerobic reactor operated at ∼30 °C as the seed for the toxicity test. The anaerobic reactor was fed weekly with a synthetic medium and acetic acid to give a final COD of around 1 g L−1 with volatile suspended solids around 5 g L−1.16 The pH value was regulated to around 7 with sodium hydroxide. The anaerobic sludge granule size distribution was analyzed by Mastersizer 2000 Particle Size Analyzer with Hydro 2000SM (AWM2002) Dispersion Unit. The surface morphology and pore size of WCP were characterized by a SEM (scanning electron microscope, Quattro S, Thermo Fisher).
2.2 Fabrication of the paper-microwell-based and smartphone-assisted colorimetric analytical device
In consideration of the greater sensitivity offered by chromatography paper compared to other types of filter paper, as reported by Sun et al.,26 this study opted to use chromatography paper, which was subsequently cut into 6 × 12 cm rectangles. Plasma etching was selected to form the microwell with the hydrophobic barrier.27 The paper was soaked into the AKD-heptane solution at 0.6 g L−1 for 2 min. After the heptane evaporated, the hydrophobic coating was cured at 105 °C for 40 min. A piece of metal mask with a pre-hollowed out microwell array (around 5 mm in diameter for each well) was then utilized to cover chromatography paper tightly and placed it into the chamber of plasma cleaner. The radio frequency of the plasma cleaner was set as 18 watts with 0.55 torr pressure and the treated time was 10 seconds. Finally, the plasma-treated paper was soaked into a 0.1 mM resazurin solution for 5 min in a dark environment, dried in a desiccator for subsequent trials.
The black anaerobic sludge samples can significantly influence the accuracy of colorimetric outcomes. While the view from the backside of the testing papers can partially eliminate the colored effects compared to the top view (Fig. 1). The black box was designed for better colorimetric identification, encompassing two removable side plates as two shelves for holding smartphone and testing paper, surrounding controllable white LED to ensure the same surrounding light conditions, and two gas ports to create an oxygen-free environment by pumping nitrogen gas. The schematic structure is shown in Fig. 1, where the same camera settings in iPhone 11 pro max (optical components, e.g., 1080 p HD at 30 fps, no exposure adjustment) was used to record the video from the backside of the testing papers to eliminate the black color effects. The distance (20 cm) between the cellphone and testing chromatography paper was fixed.
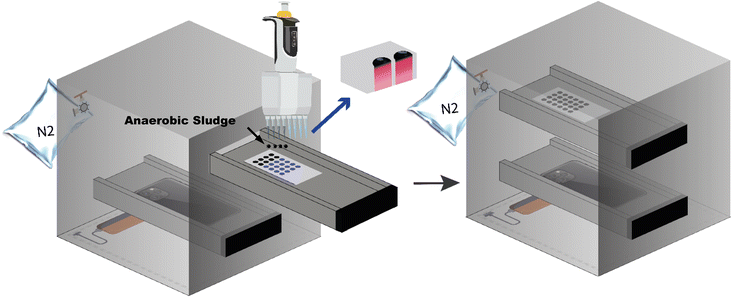 |
| Fig. 1 The design of black box for colorimetric identification on paper-microwell-based and smartphone-assisted analytical device. | |
2.3 Colorimetric identification
To study the activity of anaerobic sludge, the sample was titrated at the center of the circular zone of each microwell and a 50 min video for the change of color on the back of test chromatography paper was recorded by iPhone 11 pro max in the black box mentioned above. Footages were captured every 10 min in the recorded video and imported into the app named Color Picker,‡ by which the recorded color of a desired spot (3 × 3 pixels at 72 dpi) was digitized to hue values.28 ΔHue, the values deviated from the original hue (time 0), of three desired spots in three parallel microwells were calculated to digitize the color change, representing triplicate test. Meantime, the same experiment but with autoclaved feeding medium was carried out as an illumination control to this image-based detection. All experiments above need to be carried out at the same time to neutralize the effect of illumination by comparing to the control.
To determine the optimal condition of anaerobic sludge for microwell analysis, amounts of 5, 10, 15 and 20 microliters sludge sample, each volume with non, twice, four time, six times and eight time-dilution, and only medium diluted with DI water, were studied by the method mentioned above with the prepared paper-microwell-based analytical device. The complete process, encompassing the sampling of anaerobic sludge from the anaerobic digester to the act of dripping it onto the microwell, is efficiently conducted within a timeframe of no more than one minute. Throughout this procedure, utmost care is taken to minimize any contact with air, ensuring minimal exposure and preserving the integrity of the anaerobic environment.
To form the relationship between ΔHue values and resorufin ratios, a series of resazurin/resorufin mixture solutions with different ratios of resorufin (from 0–1) were prepared in autoclaved anaerobic sludge. The optimal volume (decided above) of this autoclaved anaerobic sludge with resazurin/resorufin was dropped onto each microwell to ensure the liquid per unit is the same as actual experiment for sludge activity measurement. Hue values were read out by app Color Picker as mentioned before.
2.4 Toxicity of chlorophenols for anaerobic digestion
30 min after feeding the seed reactor, 40 mL of fresh anaerobic sludge was mixed with 40 mL of autoclaved synthetic medium in a 100 mL serum bottle to achieve a final volatile suspended solids (VSS) concentration of 2.5 g L−1. The serum bottles were then purged with nitrogen gas for 1 minute and sealed with a rubber stopper. To investigate the effects of chlorophenols on anaerobic digestion, specific amounts of PCP, 2,4-DCP, and 4-CP stock solutions were added to the serum bottles to yield concentrations of 10 mg L−1 for each chlorophenol, while a control experiment without chlorophenols was also set up. Triplicate experiments were conducted for each test in an incubating shaker at 150 rpm and 37 °C. The experiment lasted seven days.
In order to evaluate the developed paper-microwell-based analytical device for detecting the possible toxicity on anaerobic digestion, the optimal volume of anaerobic sludge from the above experiment setup at day 0, 1, 2, 3, 4, 5, 6, and 7 was dropped onto microwell paper to analyze the anaerobic sludge activity to investigate the effects of three chlorophenols on anaerobic digestion. It should be pointed out that day 0 refers to 30 min after addition of chlorophenols in serum bottles at the beginning of the experiment. While the gas volume was measured using calibrated wetted glass syringes, and the composition of the gas analyzed using a gas-chromatograph (GC-Shimadzu GC 2010) equipped with a TCD for determining biochemical methane potential (BMP). The chemical oxygen demand (COD) was analyzed by taking a 0.1 mL sludge sample, diluting it to 0.2 mL with the medium, and injecting it into pre-prepared digestion solution in Hach vials with a detection range of 0–1500 mg O2 per L.
2.5 Data analysis
The reduction of resazurin in anaerobic system can be described as:
,19 where k1 and k2 are the reaction rate constant for resazurin to resorufin and resorufin to dihydroresorufin, respectively, M1 and M2 are metabolites such as NADH etc.29 According to our previews study,26 this whole reaction process can be described by a pseudo-first order model: |  | (1) |
By fitting ΔHue values over time to eqn (1), k1 and k2 were obtained to show the kinetics of reduction of resazurin to resorufin and resorufin to dihydroresorufin, respectively. While the pseudo-first-order rate constant for resazurin to resorufin, k1, was used as a “toxicity indicator” to identify toxicants to anaerobic digestion. The values of k1 for the effect of anaerobic sludge activity on resazurin reduction process were then compared using a one-way ANOVA with Tukey test.
2.6 The analytical greenness metric (AGREE)30
The analytical greenness metric (AGREE) introduces a pioneering approach to evaluating greenness by incorporating the twelve principles of green analytical chemistry, i.e. 12 SIGNIFICANCE principles, as the input criteria. The final assessment result in AGREE is derived from the summation of evaluations for each of the 12 input variables. These assessments are then interpreted and converted into a score ranging from 0 to 1 on a scale. A higher score indicates a greener analytical method. By utilizing AGREE, we have assessed the eco-friendliness of three techniques: paper-based microwell, BMP, and COD, specifically in their analysis of anaerobic digestion activities.
3. Results and discussion
According to Chen et al.,31 it is suggested that the bio-reduction of resazurin to resorufin by living cells can only occur intracellularly through the process of resazurin-adsorption, resazurin-diffusion into cell, resazurin-reduction in cell and resorufin-diffusion out. Anaerobic granular sludge is characterized by a multi-layer structure, with dominant microbial communities present from the inner to the outer layer, protected by extracellular polymers secreted by microorganisms in the outer layer to withstand external adverse conditions.32 Our previous study has shown that the reduction of resazurin by anaerobic sludge was associated with granules rather than the liquid phase.16 Therefore, although the reduction of resazurin in anaerobic sludge can be complex, the fundamental mechanism still involves adsorption, diffusion, reduction to product, and diffusion of product. The filter paper microwell used in this study combines the reaction (resazurin reduction) and separation (reaction products and granules). The size distribution of anaerobic sludge granules and the structure of the filter paper (as shown in Fig. 2) ensure successful separation of the resazurin reduction product, and the anaerobic sludge granules themselves do not interfere with the colorimetric analysis.
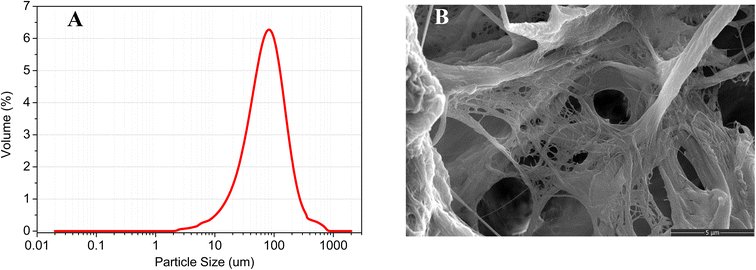 |
| Fig. 2 (A) The anaerobic sludge granular size distribution; (B) the SEM of Whatman Grade 1 chromatography paper. | |
3.1 Volume and dilution of sludge sample for microwell analysis
The volume and concentration of anaerobic sludge can have an effect on the reduction of resazurin. In general, the reduction of resazurin is proportional to the amount of active microorganisms present in the sludge.33 Consequently, a rise in either sludge volume or concentration may result in an upsurge in the population of active microorganisms, leading to a corresponding increase in the rate of resazurin reduction. Nevertheless, it is crucial to acknowledge that the size of the microwell in this study imposes a constraint on the quantity of sludge that can be introduced into a specific volume of resazurin solution. As a result, the volume of sludge sample dispensed onto the microwell plays a pivotal role in determining the mobility of the chemicals under investigation. Additionally, if the sludge is too thick,34 it may limit either the diffusion of resazurin into the sludge or, at the same time, the diffusion of its reduced form back to solution, which can also inhibit the reduction of resazurin. Hence, the density of the sludge sample is another decisive factor in the speed of resazurin reduction. As such, identifying the suitable volume and dilution of the sludge sample for microwell analysis constitutes a primary objective of this study. From Fig. 3A, it is evident that both the original (non-diluted) anaerobic sludge and the twice-diluted sample exhibit comparable rates of color change. This implies that the microorganisms present in the twice-diluted anaerobic sludge are adequate to reduce the quantity of resazurin present in the microwell, while the microorganisms in the original anaerobic sludge exceed the required amount. The degree of color change reflects the sensitivity of the colorimetric method used. As depicted in Fig. 3A, a higher sensitivity to color change was observed for a particular dilution of anaerobic sludge with a larger sample volume within 50 min. Therefore, based on these findings, the optimal conditions for future studies were determined to be a twice dilution and 20 microliters of sludge sample.
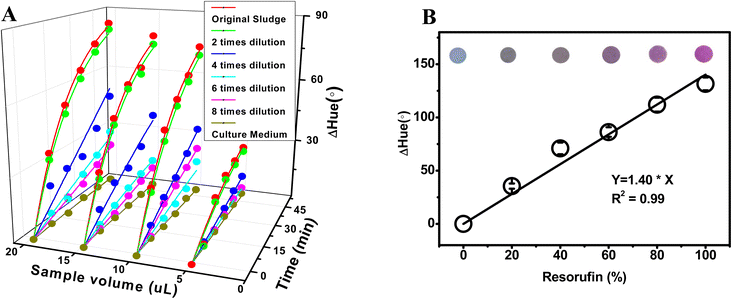 |
| Fig. 3 (A) The effect of sludge sample volume and condensity on speed of color change due to resazurin reduction by anaerobic sludge; (B) the correlation between ΔHue value and resorufin ratio in resorufin/resazurin mixture on Whatman Grade 1 chromatography paper. | |
Moreover, the correlation between ΔHue value and resorufin ratio during reaction process on microwell in 20 microliters of sludge sample was investigated. A linear relationship between ΔHue value and resorufin ratio was obtained (ΔHue = 1.40 × [resorufin%], R2 = 0.99 in Fig. 3B), suggesting that the color change can be linearly indicated the concentration of the resorufin.
3.2 Paper-microwell-based analytical device for investigating the effect of chlorophenols on digestion activity
The resazurin reduction by the anaerobic sludge was measured out by the paper-microwell-based analytical device to evaluate the activity of anaerobic digestion in each setup. The videos of resazurin reduced by anaerobic sludge on the microwells were sped up by a factor of 600 (details can be found in Videos S1†). The typical capture from the video of day 0 presenting the color change on the paper microwell at 50 min was showed in Fig. 4A (frontside and backside of microwell, respectively), in which the degree of color pink in original sludge (column a) microwell is the highest, while the pink color is difficult to read out in PCP spiked system (column d) same as the autoclaved sludge (column e). With the app of Color Picker in smartphone, the color due to resazurin reduction on the paper microwell at 0, 10, 20, 30, 40 and 50 min were digitized to hue values which can differentiate one color family from others. ΔHue, the values deviated from the original hue (time 0 of microwell experiment) were calculated to digitize the color change. The color change kinetics by anaerobic sludge at day 0 (30 min after spiking chlorophenols) was highlighted in Fig. 4B, and day 1–7 after feeding were summarized in Fig. 4C. By fitting ΔHue values over time to eqn (1), the pseudo-first-order rate constants k1 and k2 were obtained and summarized in Table 1.
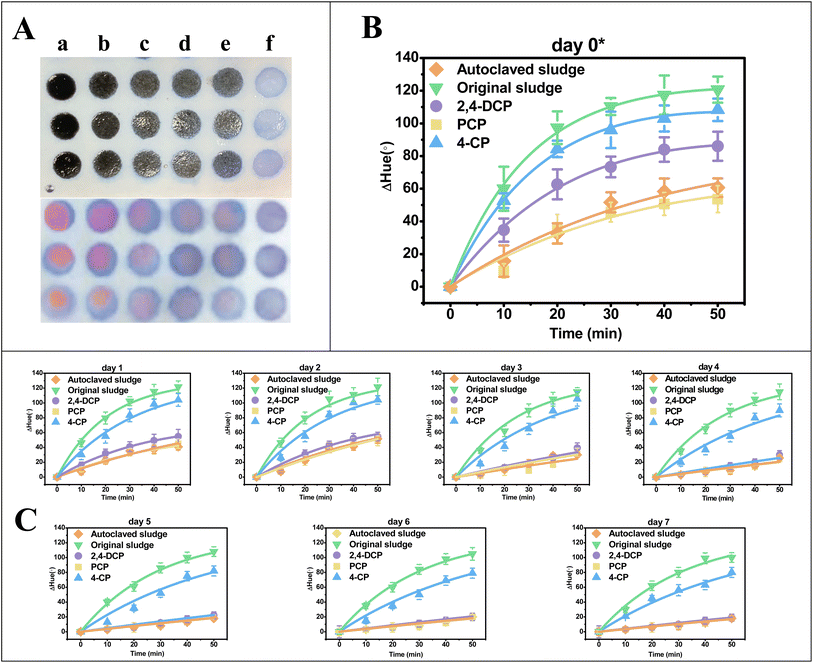 |
| Fig. 4 (A) Kinetics ΔHue recorded by microwell due to resazurin reduction by anaerobic sludge at day 0 after chlorophenols spiked for 30 min; (B) the typical color change recorded on frontside (upper) and backside (lower) of the microwell after 50 min with original sludge (column a), 4-CP spiked (column b), DCP spiked (column c), PCP spiked (column d), autoclaved sludge (column e), and feeding medium (column f); (C) kinetics of ΔHue recorded by microwell due to resazurin reduction by anaerobic sludge at day 1–7. | |
Table 1 Fitting results for k1 and k2 for resazurin reduction by anaerobic sludge on microwell fabricated from chromatography paper
Sampling time (day) |
Treatment |
k
1
(min−1) |
k
2
(min−1) |
R
2
|
k
1 and k2 are the pseudo-first-order rate constants for the conversion of resazurin to resorufin and resorufin to dihydroresorufin, respectively. This is expressed as the mean ± standard deviation of three parallel data sets.
The mean coefficients were calculated by fitting three replicates to eqn (1) (n = 6, critical R2 = 0.707, p = 0.05). a, b, c, d: significant difference labels. Turkey test in Anova (α = 0.05) was conducted on k1 of the experimental and control groups.
|
0 |
PCP |
0.0935 ± 0.006 a |
0.0307 ± 0.013 |
0.937 |
2,4-DCP |
0.1529 ± 0.005 b |
0.0298 ± 0.002 |
0.996 |
4-CP |
0.1916 ± 0.008 c |
0.0128 ± 0.007 |
0.998 |
Original sludge |
0.2199 ± 0.009 d |
0.0300 ± 0.006 |
0.998 |
Autoclaved sludge |
0.0915 ± 0.008 a |
0.0514 ± 0.002 |
0.971 |
1 |
PCP |
0.0806 ± 0.002 |
0.0679 ± 0.003 |
0.943 |
2,4-DCP |
0.0874 ± 0.004 |
0.0457 ± 0.005 |
0.937 |
4-CP |
0.1247 ± 0.007 |
0.0165 ± 0.004 |
0.996 |
Original sludge |
0.1588 ± 0.008 |
0.0184 ± 0.006 |
0.981 |
Autoclaved sludge |
0.0715 ± 0.005 |
0.0178 ± 0.002 |
0.967 |
2 |
PCP |
0.0804 ± 0.006 |
0.0196 ± 0.005 |
0.910 |
2,4-DCP |
0.0887 ± 0.005 |
0.0334 ± 0.003 |
0.902 |
4-CP |
0.1253 ± 0.006 |
0.0103 ± 0.004 |
0.940 |
Original sludge |
0.1486 ± 0.004 |
0.0111 ± 0.006 |
0.955 |
Autoclaved sludge |
0.0727 ± 0.004 |
0.0141 ± 0.005 |
0.959 |
3 |
PCP |
0.0545 ± 0.005 |
0.0315 ± 0.004 |
0.772 |
2,4-DCP |
0.0577 ± 0.003 |
0.0113 ± 0.003 |
0.890 |
4-CP |
0.1139 ± 0.005 |
0.0100 ± 0.001 |
0.901 |
Original sludge |
0.1403 ± 0.006 |
0.0113 ± 0.002 |
0.927 |
Autoclaved sludge |
0.0494 ± 0.002 |
0.0151 ± 0.002 |
0.921 |
4 |
PCP |
0.0444 ± 0.007 |
0.0104 ± 0.002 |
0.812 |
2,4-DCP |
0.0499 ± 0.006 |
0.0226 ± 0.004 |
0.889 |
4-CP |
0.1012 ± 0.010 |
0.0099 ± 0.001 |
0.927 |
Original sludge |
0.1355 ± 0.005 |
0.0100 ± 0.001 |
0.972 |
Autoclaved sludge |
0.0453 ± 0.005 |
0.0331 ± 0.005 |
0.806 |
5 |
PCP |
0.0449 ± 0.003 |
0.0262 ± 0.006 |
0.801 |
2,4-DCP |
0.0462 ± 0.009 |
0.0226 ± 0.005 |
0.861 |
4-CP |
0.0984 ± 0.010 |
0.0100 ± 0.001 |
0.909 |
Original sludge |
0.1300 ± 0.007 |
0.0100 ± 0.002 |
0.973 |
Autoclaved sludge |
0.0429 ± 0.005 |
0.0330 ± 0.005 |
0.774 |
6 |
PCP |
0.0437 ± 0.002 |
0.0262 ± 0.004 |
0.807 |
2,4-DCP |
0.0442 ± 0.002 |
0.0226 ± 0.005 |
0.861 |
4-CP |
0.0964 ± 0.008 |
0.0100 ± 0.001 |
0.914 |
Original sludge |
0.1273 ± 0.008 |
0.0100 ± 0.001 |
0.968 |
Autoclaved sludge |
0.0423 ± 0.009 |
0.0341 ± 0.006 |
0.803 |
7 |
PCP |
0.0432 ± 0.008 |
0.0262 ± 0.005 |
0.774 |
2,4-DCP |
0.0423 ± 0.003 |
0.0226 ± 0.003 |
0.914 |
4-CP |
0.0949 ± 0.005 |
0.0100 ± 0.002 |
0.929 |
Original sludge |
0.1243 ± 0.009 |
0.0170 ± 0.004 |
0.970 |
Autoclaved sludge |
0.0408 ± 0.007 |
0.0341 ± 0.006 |
0.838 |
Based on the results presented in Fig. 4 and Table 1, it was observed that the k1 value of the autoclaved sludge at day 0 was 0.0915 min−1, indicating that there was still slight microbial activity present even after being autoclaved. This result is consistent with a previously reported phenomenon.35 Moreover, the k1 value for the treatment with spiked PCP was 0.0935 min−1, which was not significantly different from the autoclaved setup. When comparing the k1 values among treatments with PCP (0.0935 ± 0.006 min−1), 2,4-DCP (0.1529 ± 0.005 min−1), 4-CP (0.1916 ± 0.008 min−1), and the original sludge (i.e., positive control, 0.2199 ± 0.009 min−1), a significant difference was observed. This suggests different levels of anaerobic activity among these treatments and indicates that the toxicity of PCP, 2,4-DCP, and 4-CP for anaerobic digestion can be detected at day 0. Based on the k1 values obtained, the order of toxicity for the three types of chlorophenols studied is PCP > 2,4-DCP > 4-CP.
3.3 Comparing to traditional digestion activity measurement through COD and BMP
The activity of anaerobic digestion is traditionally measured through monitoring biochemical methane potential,36–38 and chemical oxygen demand (COD) is a critical parameter for evaluating specific methanogenic activity and biochemical methane potential.39–41 In this study, both biogas production and COD removal were used to investigate the impact of three chlorophenols (4-CP, 2,4-DCP, and PCP) on anaerobic digestion. Fig. 5A shows that the positive control (i.e., original sludge) produces biogas at the fastest rate, while the negative control (i.e., autoclaved sludge) produces biogas at the slowest rate. The biogas (methane) cumulative rates of the systems spiked with 4-CP, 2,4-DCP, and PCP are different, indicating that the effects of these compounds on anaerobic digestion differ, with toxicity sequence of PCP > 2,4-DCP > 4-CP. Fig. 5B demonstrates that the COD removal ability of microorganisms in anaerobic digestion is similar to the biogas cumulative rates, with PCP > 2,4-DCP > 4-CP. This suggests that 4-CP exhibits lower toxicity, while PCP shows higher inhibition to anaerobic digestion. The toxicity sequence of these three chlorophenols obtained through traditional methods is consistent with the literature.4Fig. 5A indicates that the traditional BMP method requires two days to distinguish the toxicity differences among 4-CP, 2,4-DCP, and PCP on anaerobic digestion. By measuring COD removal 2 days after chlorophenols spiked (Fig. 5B), the toxicity of 4-DCP and PCP on anaerobic digestion could be identified and differentiated, however, this method was unfortunately incapable of detecting the toxicity of 4-CP on anaerobic digestion at that time. The k1 values in Table 1 derived from ΔHue of different treatments measured by the paper-microwell-based analytical device were plotted against experiment scale in Fig. 5C, in which the difference in toxicity of 4-CP, 2,4-DCP, and PCP on anaerobic digestion can be detect at the beginning when the chlorophenols were just spiked.
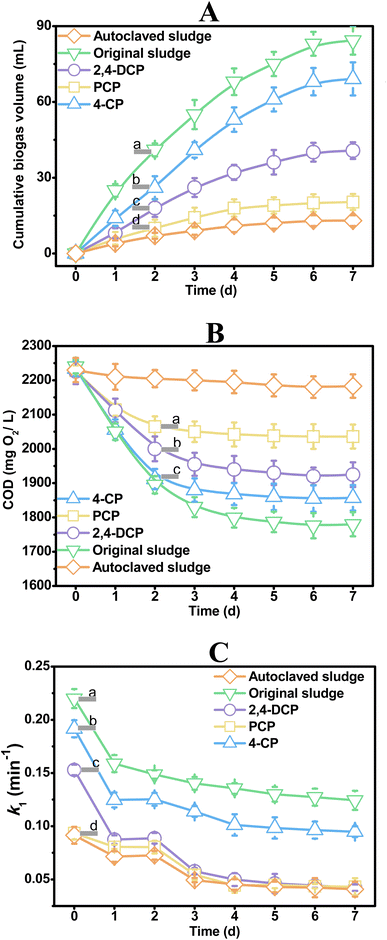 |
| Fig. 5 The cumulative biogas (A) and chemical oxygen demand removal (B) during 7 days anaerobic digestion with 4-CP, DCP and PCP spiked, and (C) kinetics (presented as k1) of ΔHue due to resazurin reduction by anaerobic sludge in 7 days after feeding indicates the activity of anaerobic digestion affected by spiked chlorophenols. a, b, c, d: significant difference labels. | |
The paper-microwell-based and smartphone-assisted analytical device developed in this study is based on colorimetry and may not be as sensitive as traditional detection methods for anaerobic digestion activity measurement, such as fluorescence-based technology. But as a rapid monitoring method, its economy and portability make it an excellent alternative for real-time rapid screening on the spot. This technology has the potential to revolutionize the way we monitor anaerobic digestion activity, making it more accessible and affordable for researchers and practitioners in this field.
Fig. 6 presents the evaluation of the environmental friendliness of all the methodologies investigated in this study using the AGREE method. The AGREE method employs a color-coded symbol consisting of red, yellow, and green to depict the findings.42 The outer and inner circles in the figure represent the 12 sections, each indicating different aspects of greenness with varying color intensities and scores ranging from 0 to 1.43 Based on the results, the paper-microwell-based and smartphone-assisted colorimetric analytical device demonstrates a commendable greenness score of 0.81. This suggests that this technique is comparatively greener than both BMP, which scored 0.61, and COD, which scored 0.59. These findings provide valuable insights into the environmental performance of the evaluated methodologies and highlight the favorable greenness of the paper-microwell-based and smartphone-assisted colorimetric analytical device.
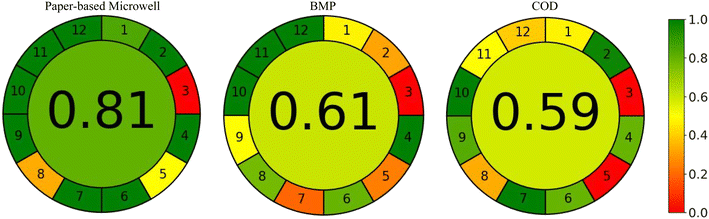 |
| Fig. 6 Results of AGREE analysis for the anaerobic activities measurement methods by paper-based microwell, biochemical methane potential (BMP) and chemical oxygen demand (COD). | |
4. Conclusion
To summarize, this study provides novel insights into the integration of resazurin reduction bioassay with paper-microwell-based and smartphone-assisted analytical device to detect the activity of anaerobic digestion. Resazurin was deposited onto chromatography paper microwell, and the blue resazurin reduced to pink resorufin by anaerobic sludge was recorded in a specially designed black box. Traditional biochemical methane potential and chemical oxygen demand removal methods require at least 2 days to detect the toxicity and distinguish the toxicity differences of PCP, 2,4-DCP, and 4-CP. However, by using the paper-microwell-based and smartphone-assisted analytical device, the color change can be observed in just one hour, indicating the toxicity of PCP, 2,4-DCP, and 4-CP, as well as their differences in toxicity for anaerobic digestion. This approach provides a rapid, cost-effective, and eco-friendly alternative for toxicity detection in anaerobic digestion, and it is the first time it has been explored in this context.
Conflicts of interest
All authors declare no competing interests.
Acknowledgements
The work described in this paper was supported by a grant from the Environment and Conservation Fund (ECF) of Hong Kong SAR, China (ECF 02/2019) and partially by a grant from the Research Grants Council of the Hong Kong SAR, China (UGC/IDS(R)16/19), a grant from Research Grants Council of Hong Kong SAR, China (UGC/FDS16/M(P)05/21), a grant from Hong Kong Metropolitan University Research Grant (PFDS/2022/07), and a grant from Shenzhen Fundamental Research Program (JCYJ20190808181607387).
References
- P. M. V Subbarao, T. C. D' Silva, K. Adlak, S. Kumar, R. Chandra and V. K. Vijay, Environ. Res., 2023, 234, 116286 CrossRef.
- D. C. Stuckey, Bioresour. Technol., 2012, 122, 137–148 CrossRef CAS.
- B. Schink, Microbiol. Mol. Biol. Rev., 1997, 61, 262–280 CAS.
- J. L. Chen, R. Ortiz, T. W. J. Steele and D. C. Stuckey, Biotechnol. Adv., 2014, 32, 1523–1534 CrossRef CAS PubMed.
- P. Worm, F. G. Fermoso, A. J. M. Stams, P. N. L. Lens and C. M. Plugge, Environ. Microbiol., 2011, 13, 1228–1235 CrossRef CAS.
- X. Y. Ma, X. C. Wang, H. H. Ngo, W. Guo, M. N. Wu and N. Wang, Sci. Total Environ., 2014, 468–469, 1–11 CrossRef CAS.
- A. Rozzi, M. C. Tomei, A. C. Di Pinto and N. Limoni, Biotechnol. Bioeng., 1997, 55, 33–40 CrossRef CAS.
- A. Pollice, A. Rozzi, M. C. Tomei, A. C. Di Pinto and G. Laera, Water Res., 2001, 35, 1179–1190 CrossRef CAS PubMed.
- A. Kaur, J. R. Kim, I. Michie, R. M. Dinsdale, A. J. Guwy and G. C. Premier, Biosens. Bioelectron., 2013, 47, 50–55 CrossRef CAS PubMed.
- Z. Liu, J. Liu, S. Zhang, X.-H. Xing and Z. Su, Bioresour. Technol., 2011, 102, 10221–10229 CrossRef CAS PubMed.
- D. A. McKenzie, Int. J. Dairy Technol., 1962, 15, 207–212 CrossRef.
- D. I. Njoku, Q. Guo, W. Dai, J. L. Chen, G. Mao, Q. Sun, H. Sun and Y.-K. Peng, TrAC, Trends Anal. Chem., 2023, 167, 117288 CrossRef CAS.
- V. Mutsenko, A. Barlič, T. Pezić, J. Dermol-Černe, B. Dovgan, B. Sydykov, W. F. Wolkers, I. I. Katkov, B. Glasmacher, D. Miklavčič and O. Gryshkov, Cryobiology, 2019, 91, 104–114 CrossRef CAS PubMed.
- S.-J. Li, X. Zhang, X.-H. Wang and C.-Q. Zhao, Eur. J. Med. Chem., 2018, 156, 316–343 CrossRef CAS PubMed.
- W. Dai, B. Inumbra, P. Y. Wong, A. Sarmiento, Y. Yau, J. Han, G. Mao, Y.-K. Peng and J. L. Chen, Biosensors, 2023, 13, 523 CrossRef CAS PubMed.
- J. L. Chen, R. Ortiz, Y. Xiao, T. W. J. Steele and D. C. Stuckey, Water Res., 2015, 75, 123–130 CrossRef CAS PubMed.
- J. L. Chen, T. W. J. Steele and D. C. Stuckey, Sci. Total Environ., 2018, 642, 276–284 CrossRef CAS PubMed.
- J. L. Chen, T. W. J. Steele and D. C. Stuckey, Environ. Sci. Technol., 2016, 50, 11154–11163 CrossRef CAS.
- J. L. Chen, T. W. J. Steele and D. C. Stuckey, Environ. Sci. Technol., 2015, 49, 13463–13471 CrossRef CAS PubMed.
- R. Ortiz, J. L. Chen, D. C. Stuckey and T. W. J. Steele, ACS Appl. Mater. Interfaces, 2017, 9, 13801–13811 CrossRef CAS PubMed.
- R. Ortiz, J. L. Chen, D. C. Stuckey and T. W. J. Steele, Micro Nano Eng., 2019, 2, 92–103 CrossRef.
- J. Hu, S. Wang, L. Wang, F. Li, B. Pingguan-Murphy, T. J. Lu and F. Xu, Biosens. Bioelectron., 2014, 54, 585–597 CrossRef CAS PubMed.
- H. Wang, Y. Li, J. Wei, J. Xu, Y. Wang and G. Zheng, Anal. Bioanal. Chem., 2014, 406, 2799–2807 CrossRef CAS.
- S. Nahavandi, S. Baratchi, R. Soffe, S.-Y. Tang, S. Nahavandi, A. Mitchell and K. Khoshmanesh, Lab Chip, 2014, 14, 1496–1514 RSC.
- D. M. Cate, J. A. Adkins, J. Mettakoonpitak and C. S. Henry, Anal. Chem., 2015, 87, 19–41 CrossRef CAS PubMed.
- Q. Sun, N. F. Y. Tam, J. Han, W. Yung-Kang Peng, Z. Zhu and J. L. Chen, Talanta, 2021, 225, 121966 CrossRef CAS.
- X. Li, J. Tian and W. Shen, Anal. Bioanal. Chem., 2010, 396, 495–501 CrossRef CAS PubMed.
-
H.-C. Lee, Introduction to Color Imaging Science, Cambridge University Press, Cambridge, 2005 Search PubMed.
- J. O'Brien, I. Wilson, T. Orton and F. Pognan, Eur. J. Biochem., 2000, 267, 5421–5426 CrossRef PubMed.
- F. Pena-Pereira, W. Wojnowski and M. Tobiszewski, Anal. Chem., 2020, 92, 10076–10082 CrossRef CAS PubMed.
- J. L. Chen, T. W. J. Steele and D. C. Stuckey, Biotechnol. Bioeng., 2018, 115, 351–358 CrossRef CAS PubMed.
- A. Doloman, H. Varghese, C. D. Miller and N. S. Flann, BMC Syst. Biol., 2017, 11, 69 CrossRef PubMed.
- D. Liu, Environ. Sci. Technol., 1983, 17, 407–411 CrossRef CAS.
- L. van den Berg, S. Toja Ortega, M. C. M. van Loosdrecht and M. K. de Kreuk, Water Res.: X, 2022, 16, 100148 CAS.
- K. Xiao, Z. Yu, K. Pei, M. Sun, Y. Zhu, S. Liang, H. Hou, B. Liu, J. Hu and J. Yang, Front. Environ. Sci. Eng., 2021, 16, 23 CrossRef.
- W. F. Owen, D. C. Stuckey and J. B. Healy Jr, Water Res., 1979, 13, 485–492 CrossRef CAS.
- J. Filer, H. H. Ding and S. Chang, Water, 2019, 11, 921 CrossRef CAS.
- K. Koch, S. D. Hafner, S. Weinrich, S. Astals and C. Holliger, Front. Energy Res., 2020, 8, 63 CrossRef.
- A. Hussain and S. K. Dubey, Appl. Water Sci., 2017, 7, 535–542 CrossRef CAS.
- C. M. W. Harnadek, N. G. H. Guilford and E. A. Edwards, STEM Fellowship J., 2015, 1, 2–5 CrossRef.
- J. N. Meegoda, B. Li, K. Patel and L. B. Wang, Int. J. Environ. Res. Public Health, 2018, 15, 2224 CrossRef CAS PubMed.
- F. Pena-Pereira, M. Tobiszewski, W. Wojnowski and E. Psillakis, Adv. Sample Prep., 2022, 3, 100025 CrossRef.
- D. S. El-Kafrawy, A. H. Abo-Gharam, M. M. Abdel-Khalek and T. S. Belal, Microchem. J., 2022, 176, 107236 CrossRef CAS.
Footnotes |
† Electronic supplementary information (ESI) available. See DOI: https://doi.org/10.1039/d3va00268c |
‡ The specifications are located at https://apps.apple.com/hk/app/color-picker-designer-tools/id1163494239?l=en |
|
This journal is © The Royal Society of Chemistry 2024 |