Nano-pelargonidin modulates p53/PARP/HSP 90/XRCC1 signaling axis to combat cypermethrin-induced genotoxicity and metabolic dysfunction in fish: a molecular docking predicted in vivo approach†
Received
21st December 2023
, Accepted 2nd March 2024
First published on 19th March 2024
Abstract
Pesticides and other products used in agricultural practices often find their way into nearby waterbodies through agricultural run-offs, contributing to pollution and affecting non-target organisms, especially edible fish, posing a risk to human health. In this study, an attempt is made to address this global issue by formulating a possible nano-phyto-product that can combat the genotoxic and metabolic effects of pesticides in fish models. Thus, poly-lactide-co-glycolide (PLGA)-encapsulated nano-pelargonidin (NPG) was synthesized and characterized via AFM, FESEM, DLS, and CD methods. An in silico molecular docking study was conducted to identify the signalling proteins that interact with the phyto-core-compound pelargonidin (PG) to predict the effectiveness of NPG in combating pesticide-induced toxicity. Thereafter, the predicted signalling proteins, p53, PARP, and HSP 90, were tracked in Oreochromis mossambicus to investigate the possible effect of NPG in combating cypermethrin (CM)-induced genotoxicity-associated dysfunctions, such as ROS generation, alteration in MMP, micronucleus formation, and histopathological changes. Overall, the results revealed that pre-treatment with negatively charged PLGA-encapsulated NPG (∼64 nm) showed protective efficacy against CM-induced genotoxicity by modulating the activity of biological macromolecules such as DNA-repair proteins and stress proteins, which was corroborated by molecular docking predictions. Thus, the present strategy appears to be a cost-effective method for combating pesticide-induced toxicity issues quite effectively to restore environmental harmony and protect non-target organisms, like fish.
Environmental significance
Cypermethrin is an agrochemical that induces toxicity in aquatic organisms, especially fish, when leeched out into water bodies, causing severe damage in living cells. To address this issue, a nanotechnology-based drug-delivery technique was adopted in this present work. Nanotechnology has recently been used in drug designing in conjunction with developments in this subject, demonstrating the prevalence of its importance in a variety of domains for enhancing the activity of each therapeutic component against various illnesses. Pelargonidin (PG), an active phyto-ingredient, has been reported to have anti-oxidant, anti-inflammatory, anticarcinogenic, and antigenotoxic activities. Therefore, we aimed to increase the preventive ability of PG by using nanotechnology-based polymeric PLGA nanoencapsulation to provide preventive efficacy against cypermethrin-induced-toxicity in a fish biosystem in vivo.
|
1. Introduction
Environmental pollution, particularly in waterbodies, generated by pesticide contamination from agricultural run-offs, is a global problem impacting several health issues in non-target aquatic organisms, including fish, and indirectly affecting mankind. Organophosphates, carbamates, chlorinated hydrocarbons, pyrethroids, and nicotinoids are the main groups of insecticides that are known to pose a threat to the long-term survival of important ecosystems.1 Bioindicators, such as fish, provide a comprehensive view of the state of the environment over time, providing an indication of aquatic pollution levels.2 Fish are extremely susceptible to the contaminating effects of pyrethroids in water bodies. The health of fish can be seriously affected after exposure to cypermethrin (CM), a well-known type II pyrethroid, since it drastically disrupts several physiological and biochemical processes by modulating different signalling cascades in fish.3–5 Therefore, it is imperative to take prompt action to counter or avoid the harmful effects of these pesticides related to trophic level-oriented bioaccumulation and biomagnification resulting in serious health complications.
To address this particular issue, a nanotechnology-based drug-delivery technique was adopted in this work. Nanotechnology has recently been used as a drug-design tool in a variety of domains for enhancing the activity of therapeutic components against various illnesses.6 Multiple studies on the synthesis and use of ecologically benign nanoparticles of various sizes utilizing phyto-products have reignited interest in nano-science owing to their many modalities and activities.7–9 As reported, PLGA (poly-lactide-co-glycolide), a non-toxic, biodegradable polymer (approved by FDA) is a well-known nanocarrier of drug components that can extend the persistence of newly designed drugs in the body by suspending the release of an inner drug component, which eventually improves the bioavailability, biocompatibility and prevent premature breakdown of the designed drug in the biological environment.10–26 Nanotechnology presents an opportunity for designing nanoparticle-mediated drug-delivery systems that can address the precise challenges of optimum drug delivery in a multiple-fold decreased dose through a cost-effective strategy.27,28
In this research work, plant-derived chemicals were preferred over synthetic molecules due to their non-toxicity/limited toxicity, low cost, and ease of availability. Phytocompounds have previously been utilized by various researchers to combat pesticide-induced toxicity in fish and other animals29–36 and are reported to provide enormous health advantages. Phytocompounds are now routinely employed in lifestyle care products, including in vegetables, medications, healthcare beverages, and cosmetics.37–39 They have been shown to have powerful biological actions against stress and its related damage in biological macromolecules, such as DNA breakage, protein expression modification, and tissue obliteration. The phytocompound pelargonidin (PG), a natural anthocyanidin, has been reported to exhibit vaso-protective,40 anti-inflammatory,41 anti-carcinogenic,42 anti-obesity,43 and anti-diabetic44,45 activities by rescuing cells and tissues from cytotoxicity and genotoxicity caused by several toxicants, both in its phytochemical form and nano-encapsulated forms.16–49 On the other hand, several toxicological investigations of xenobiotics have found a correlation with the impairment of key DNA repair proteins, such as p53, PARP, HSP 90, and XRCC1, which promote dysfunction of signalling cascades and ultimately lead to genetic instability.18,24,33,39 Therefore, an approach based on nanotechnology was exploited in the present study to address the issue of cypermethrin (CM) pesticide-induced genotoxicity in a tilapia fish model, whereby the phytocompound PG was encapsulated in PLGA to check whether the formulated nano-pelargonidin (NPG) would play a beneficial role in inhibiting the progression and intense complications that may develop in fish when exposed to the toxic chemical pesticide CM.
In order to increase the potential therapeutic efficacy of the nanoparticles, the identification of the potential target of the inner core phytocompound becomes necessary, which could allow predicting the signalling proteins that are involved in the “switch on” (activation of a concerned protein) or “switch off” (de-activation of a concerned protein) process for the overall mechanism of the events involved in the toxicity by participating in modulatory pathway inhibition/initiation in a precise manner by binding to different protein motifs. In the in silico predictions, investigations were therefore conducted to determine whether the phytocompound PG encapsulated in PLGA was equally effective in modulating those proteins in a living fish model as well.
Thus, the objectives of the present study were: (i) to identify the proteins that interact with PG by performing a molecular docking study, (ii) to formulate and characterize the PLGA-encapsulated nano-pelargonidin (NPG), (iii) to determine CM's persistence capability in water, (iv) to check whether NPG shows any protective role against CM-induced toxicity in tilapia fish (in vivo), and (v) to corroborate the in silico predicted biomolecules and their interactive biofactors involved in combating CM-induced genotoxicity and associated cellular dysfunction.
2. Method and materials
2.1 Materials
The analytical grade reagents and solvents utilized in this research work were all obtained from Sigma-Aldrich and other reputable companies. These are presented in the text in accordance with how they were used in the tests.
2.2 Photolytic reaction of cypermethrin
First, 5 mg of cypermethrin was dissolved in 2 μL of acetone followed by adding 1 mL of water to perform the reaction. This reaction mixture was placed under sunlight in the presence of an oxygen atmosphere for 7 days. After the 7 days of reaction, the final product was evaporated in a desiccator and then underwent FTIR and 1H NMR analyses for obtaining information on the chemical structure of the product together with the crude cypermethrin.
2.3 Preparation of nano-pelargonidin through PLGA encapsulation
The optimal conditions were followed to create nano-pelargonidin (NPG), which was encapsulated in poly-lactide-co-glycolide (PLGA) using a typical solvent displacement approach with minor modification.16,50
2.4 Characterization of NPG through atomic force microscopy imaging
The shape and surface of the synthesized NPG were determined by atomic force microscopy (AFM) imaging, which was performed in amplitude and tapping modes and using WSxM 5.0 Develop 7.0 software, which is free software for scanning probe microscopy, for image processing and data acquisition to obtain 2-dimensional (2D) and 3-dimensional (3D) plots of the synthesized nanoparticles. By referring to the established technique, the effectiveness of pelargonidin's encapsulation and its ability to load into the PLGA were assessed,13,51 according to the given equation as stated below:
2.5 Field emission scanning electron microscopy and dynamic light scattering analyses
Newly synthesized NPG in powder form was placed on a carbon conductive tap for the field emission scanning electron microscopy (FESEM) analysis of the size and morphology together with EDX spectroscopy. The size distribution of the nanoparticles and zeta potential were estimated by dynamic light scattering (DLS) study using a Nano-ZS instrument (for measurement of the particle size ranging from sub-nanometres to several micrometres in diameter), as well as the zeta potential (Malvern, UK), following standard protocol according to ref. 6.
2.6 Determination of stability of NPG
Using spectrophotometry, the stability profile of NPG over time was determined at 508 nm as the λmax wavelength of PG. The nanoparticles were dissolved in water, and their absorbance was recorded at this specific wavelength starting at 0 h and continuing for 60 h.52
2.7 Assessment of the thermal denaturation
Using a SHIMADZU UV-1700 spectrophotometer equipped with a temperature programmer to regulate the rate of temperature change during the denaturation experiment, the thermal denaturation (Tm) profile of DNA was acquired. The sample mixture included 20 μM of the drug and 4.00 mM of DNA concentration. The data chart recorded the temperature and absorbance variations between the sample cuvette and the reference cuvette (which were identical to one another except for the absence of DNA) at the wavelength of 260 nm.
2.8 Circular dichroism spectroscopy
Circular dichroism (CD) spectra were obtained to monitor the changes in the structural integrity of DNA in the range of 200–650 nm wavelength using a 1 cm path length of rectangular quartz cuvette (Jasco spectropolarimeter; model PC controlled J-815; Jasco International Co. Ltd; equipped with a temperature controller and thermal programmer PFO 425L/15). The standard DNA concentration for conducting this experiment was 1 mM. The generated CD spectra were obtained from interaction of the drug with native calf thymus DNA at 37 °C and compared with that of the CD spectrum of only calf thymus DNA.
2.9 Ligand and protein preparation for the molecular docking
The relevant proteins of this study were p53, PARP, and HSP 90. The protein–ligand docking study was performed with the compound pelargonidin. The software programs ChemDraw Ultra 8.0 and Chem3D Ultra 8.0 were used for the ligand molecule preparation optimization. Argus Lab 4.0.1 was used for the docking of ligand inside the receptor active cleft. ChemDraw Ultra 8.0 and Chem3D Ultra 8.0 were used for constructing the 2D and 3D structures of pelargonidin. The 3D form was then energy minimized for attaining the proper geometry. HSP 90, PARP, and p53 were all downloaded from Protein Data Bank with PDB IDs of 1YET,533GJW,54 and 1YC5,55 respectively. The water molecules were deleted from the downloaded protein, and hydrogen atoms were added to further prepare it for appropriate interaction. The Argus Lab 4.0.1 dock engine was used to dock the ligand into the protein's functional cavity. The active cleft of binding was identified using the co-crystallized molecules of geldanamycin (HSP 90), A968427 (p53), and nicotinamide (PARP). The protein–ligand complex with the lowest interaction energy was chosen as the predictive score for further research after flexible docking. Utilizing Discovery Studio, which aids in identifying important amino acid residues and the suitable bonds linking proteins with the ligands, protein–ligand interaction studies were carried out. The predictive accuracy of docking studies depends on the preparation of the ligand and protein structures and the scoring function used. The Argus Lab 4.0.1 dock engine simulation AScore, as a scoring function obtained while the ligand and receptor are flexible, can allow generating many conformer complexes of the ligand–protein.
2.10 Experimental design
Disease-free, hormone-free, fresh tilapia fish (Oreochromis mossambicus) were purchased from a fish farm near Kalyani. All animal procedures were performed in accordance with the CPCSEA guidelines for the care and use of laboratory animals of Kalyani University and approved by the animal ethics committee of IAEC of the University of Kalyani, 892/GO/Re/S/01/CPCSEA (Proposal no: KU/29-03-23/06). All the fish were acclimatized to the experimental environment and then transferred to an experimental aquarium for conducting the experiments. Nine healthy fish were selected for each experimental group, including the control group, with the groups described below.
Control.
The fish were fed with commercial fish dry pellets.
CM-treated.
The fish received CM at a dose of 0.05 μL g−1 b.w. (a suitable sub-lethal dose selected previously through a range finding trial) through intramuscular injection. Their feeding was stopped for 24 h before the exposure to CM.
Pre-treated PG + CM.
PG was administered to the fish as a pre-treatment at a dose of 0.5 mg g−1 b.w. for 7 days, followed by the subcutaneous injection of CM at a dose of 0.05 μL g−1 b.w. Feeding was stopped for 24 h prior to the exposure to CM.
Pre-treated NPG I + CM.
This group was fed with NPG at a dose of 0.5 mg g−1 b.w. for 7 days, followed by the subcutaneous injection of CM at a dose of 0.05 μL g−1 b.w. Feeding was stopped for 24 h prior to the exposure to CM.
Pre-treated NPG II + CM.
The tilapia fish received NPG at a dose of 1 mg g−1 b.w. for 7 days, followed by the subcutaneous injection of CM at a dose of 0.05 μL g−1 b.w. Feeding was stopped for 24 h prior to the exposure to CM.
2.11 Determination of cell viability in the PG-treated group
After the end of the 7 days of experiments, hepatic tissue from all the experimental groups, including the control, was dissected out of the fish and directly perfused in cold PBS buffer. Then, a trypan blue exclusion assay was performed to estimate the percentage of live cells as per the standard protocol in ref. 18.
2.12 Determination of the histopathological changes
After dissecting the liver, kidney, spleen, muscle, testis, and gill tissue from all the fish in the experimental groups, including the control group, they were fixed in neutral buffer formalin and embedded into a paraffin block. Next, 6 μM of paraffin sections were processed in upgraded and downgraded alcohol series, and after that, counterstained with haematoxylin and eosin. The stained tissue sections were observed under a compound microscope for analysing any changes in the tissue architecture.39
2.13 Analysis of the reactive oxygen species generation from hepatic cell suspensions
Hepatocytes were isolated in PBS buffer and fixed in 4% paraformaldehyde for analysing reactive oxygen species (ROS) production after adding DCFDA-H2 dye. After 30 min of incubation at room temperature, the ROS level was estimated by fluorospectroscopy at 485 nm (excitation)/535 nm (emission) wavelength as per the standardized protocol in ref. 18, 24 and 56.
2.14 Analysis of the ROS, nuclear condensation, and DNA damage in histological sections of hepatic tissue
Histological fixed hepatic tissue sections from the control and experimental groups of fish were deparaffinized with xylene and afterwards were hydrated through a downgraded series of alcohol concentrations followed by water. The sections were then stained with H2DCF-DA in a dark condition for 30 min at room temperature. After the dye was cleaned off through water from the sections and then, the samples were counterstained with Hoechst dye for 15 min at 4 °C. After further washing the tissues with water, they were incubated with propidium iodide (PI) for an additional 15 min at 4 °C, and examined under a confocal microscope (Carl Zeiss LSM 800) at 10X magnification.
2.15 Estimation of the anti-oxidative enzyme profiles
The modulations of the anti-oxidative enzymes functions, such as catalase (CAT), superoxide dismutase (SOD), lipid peroxidase (LPO), and total thiol (reduced GSH), were estimated using the hepatic tissue collected from all the experimental groups along with the control group. Briefly, hepatic tissue was homogenized into cold PBS buffer and centrifuged at 10
464 g for 30 min to collect the tissue suspension and then stored at 80 °C, with standard protocols followed, like in ref. 57 for estimating LPO, ref. 58 for determining SOD activity, ref. 59 for sensing any possible differences in CAT activity, and ref. 60 to determine the reduced glutathione content in hepatic tissue.
2.16 Determination of the hepatic biomarker enzymes
We analysed the levels of alkaline phosphatase, acid phosphatase, and lactate dehydrogenase from the hepatic tissue suspensions by following the standard protocols in ref. 61 and 62 respectively.
2.17 Determination of the serum glucose level
Following the established procedure for the GOD-POD glucose kit (Autospan),12,63 blood serum from the control and each experimental group of tilapia fish was separated to determine the blood glucose level.
2.18 Analysis of the DNA fragmentation data
The SNET technique was used to extract DNA from the hepatic, muscle, and spleen tissue of the control and experimental groups.64 By applying 1% ethidium bromide in an agarose gel electrophoresis, the degree of DNA damage was assessed.
2.19 Estimation of micronucleus formation in blood
Following anaesthesia, blood was drawn from the caudal vein of the control and experimental groups and applied as a smear on a grease-free slide. The smear was air-dried and fixed with Carnoy's fixative solution with the normal technique.24 The slides were stained with Giemsa solution after fixation, and then observed under a compound microscope (Labomed Lx 300) under 40× magnification. Each set of 1000 erythrocytes underwent micronucleus test analysis.
2.20 Analysis of the mitochondrial membrane potential
Isolated cell suspensions from the hepatic tissue samples were incubated with rhodamine 123 for 15 min at 4 °C to estimate the mitochondrial membrane potential (MMP) in the different experimental groups, including the control. Changes in the MMP level were measured by fluorospectroscopy at 480 nm (excitation)/535 nm (emission) wavelength as per the standard protocol in ref. 12.
2.21 Determination of the ATPase level
We analysed the level of ATPase enzyme in hepatic tissues from all the experimental groups, including the control, using the standard methodology to determine the functions of the ATPase enzyme.65
2.22 Detection of the calcium ions by a complexometric titration method
Hepatic tissue suspensions of the different experimental groups, including the control group, were titrated with disodium salt of EDTA solution in the presence of an indicator (EBT, Eriochrome black T) to estimate the level of calcium ions following the standard protocol in ref. 13 and 66.
2.23 Immunofluorescence study of p53, PARP, HSP 90, XRCC1 proteins in hepatic tissue
We conducted an immunofluorescence study using anti-p53, PARP, HSP 90, and XRCC1 proteins (Santa Cruz Biotechnology, Inc., USA), and then, FITC-tagged secondary antibodies (Sigma, USA) were used in hepatic tissue, and the samples were observed under a confocal microscope (Carl Zeiss LSM 800) under 10× magnification.17,24 The intensity of protein expression was measured using ImageJ software by using the same macros plugin for maintaining the same pixel size to form the heatmap.
2.24 Analytical statistics
Following the completion of three independent experiments and the necessary computation of all the mean and S.E. values, the data from several trials were analysed using Student's t-test and one-way ANOVA.
3. Results
3.1 Characterization of cypermethrin under sunlight reaction
The interaction of cypermethrin with O2 was investigated through recording 1H NMR (Fig. 1) and FTIR spectra for the crude compound and that after 7 days reaction with O2. In the 1H NMR spectra, small downfield chemical shift values for the protons of Ha, Hd, and
by 0.09, 0.076, and 0.065 ppm, respectively, were observed, indicating there was almost no interaction with cypermethrin under the experimental conditions (Fig. 1). The FTIR spectrum of cypermethrin showed major bands at 2924, 2854, and 1742 cm−1, which remained unchanged upon interaction (Fig. S1†). Thus both the 1H NMR and FTIR spectra revealed that cypermethrin could not take part in interactions.
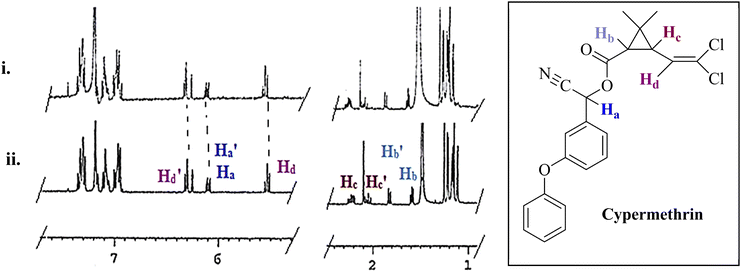 |
| Fig. 1 Partial 1H NMR (CDCl3, 400 MHz) of cypermethrin after 7 days (i) and cypermethrin (ii) upon passing O2. Inset, structure of cypermethrin; a–d refer to different protons. | |
3.2 Characterization of PLGA-encapsulated nano-pelargonidin
The biosynthesized PLGA-encapsulated NPG was fully characterized by AFM, FESEM, and DLS techniques, validating the size of the synthesized nanoparticles was below 100 nm. The morphological characteristic of NPG was examined by AFM, which demonstrated its spherical shape, while the subsequent 2D and 3D views of the AFM images showed the mean diameter of NPG was 64 ± 0.05 nm, having a smooth surface without any cracks or holes (ESI† S2a–c). As observed from the 2D view from fast Fourier transformation (FFT) in the AFM study, NPG also showed a uniform spatial frequency of the topographic planar signal (ESI† S2d). The spherical shape morphology of NPG determined by AFM study was further confirmed by FESEM study. From the analysis of the EDX spectra, only intense peaks of carbon and oxygen were found, confirming the purity of the newly synthesized NPG (ESI† S2e and f). Through the DLS study, as mentioned above the size distribution analysis of PLGA-encapsulated NPG revealed that the newly synthesized nanoparticles were below 100 nm and zeta potential value was −17 mV with a polydispersity index (PDI) of 0.616. The encapsulation efficacy (EE%) of PG in the NPG capsule was calculated to be 87.57%, representing a high loading capacity of pelargonidin within PLGA to create nano-encapsulated PG.
3.3 Determination of the thermal denaturation
The Tm data revealed that CT-DNA alone (without PG or NPG) had a melting temperature (Tm) of 82.5 ± 0.5 °C, and increased to 87.5 ± 0.5 °C and 90.0 ± 0.5 °C, respectively, upon the separate introduction of PG and NPG with CT-DNA (ESI† S2g).
3.4 Analysis of the CD spectra
From the CD spectra, it could be observed that CT DNA showed a positive band at 283 nm for base stacking and one negative band at 237 nm due to a helical change, which were typical characteristics indicating the B-form of CT DNA. After that, CT-DNA could interact with PG and NPG and as a result the molar ellipticity of CT-DNA significantly increased. However, in comparison to PG binding with CT-DNA, NPG showed a slightly higher molar ellipticity at a 10-fold reduced concentration than PG (ESI† S2h).
3.5 Stability of NPG
From the spectroscopic data, it was observed that the stability of NPG was maintained from 0 h to 60 h. According to the findings, the PLGA encapsulation enabled PG to remain stable over a sufficient time scale to facilitate good drug delivery in the body system (Fig. 2).
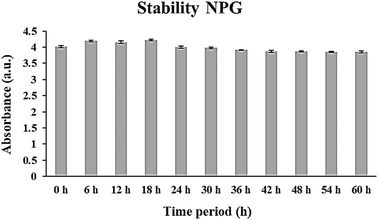 |
| Fig. 2 Assessment of the stability of NPG over a time period. | |
3.6
In silico docking studies for estimating the drug–protein interactions
The optimal poses of the PG-p53, PG-PARP, and PG-HSP 90 complexes displayed docking scores of −5.71, −8.19, and −9.05 kcal mol−1, respectively. These docking values were considered the lowest energy of interaction for the ligand fitting into the active pocket of the protein with maximum flexibility. Fig. 3 shows the best binding pose of the protein–ligand complex. The multiple linkages that hold the proteins to the ligand, i.e., hydrogen bonds, disulfide bonds, hydrophobic bonds, and electrostatic interaction, were responsible for the process of the effective interaction. Table 1 lists the essential amino acid residues required for biological function. The crucial amino acids linking the protein–ligand complex are shown in Fig. 3.
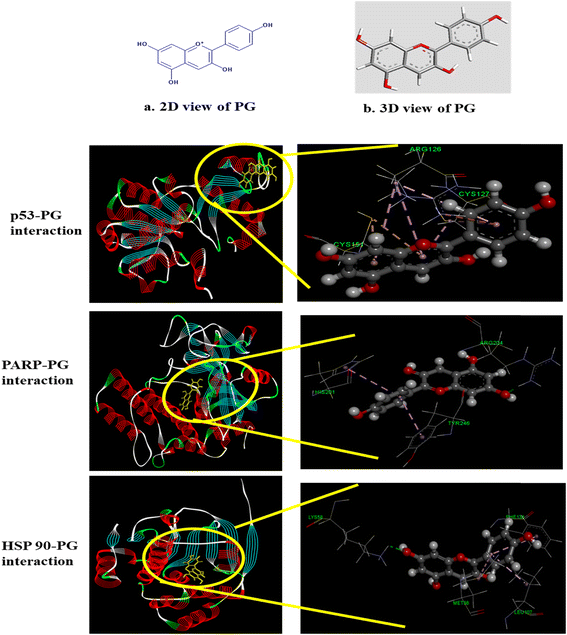 |
| Fig. 3 Analysis of docking interactions between the p53–pelargonidin complex, PARP–pelargonidin complex, and HSP 90–pelargonidin complex with crucial amino acid residues. | |
Table 1 Crucial amino acid residues linking proteins with pelargonidin
Protein–ligand complexes |
PDB ID of proteins |
Docking score (kcal mol−1) |
Amino acid residues |
Effective linkages |
PG-p53 |
1YC5
|
−5.71 kcal mol−1 |
Cys151 |
Disulfide bonding |
Arg126 |
Electrostatic interaction |
Arg126 and Cys127 |
Hydrophobic interaction |
PG-PARP |
3GJW
|
−8.19 kcal mol−1 |
ARG204 |
Hydrogen bonding |
HIS201 and TYR246 |
Hydrophobic interaction |
PG-HSP90 |
1YET
|
−9.05 kcal mol−1 |
LYS58 |
Hydrogen bonding |
MET98, LEU107 PHE138 |
Hydrophobic interaction |
3.7 Analysis of the cell cytotoxicity in the PG- and NPG-treated groups
Tilapia fish pre-treated with PG and NPG prior to CM administration showed a significant reduction in CM-induced % cytotoxicity of hepatic cells by enhancing the % of viable cells when compared with the group treated with CM alone (Fig. S3†).
3.8 Analysis of the histopathology of PG- and NPG-treated different tissues
Histopathological analysis of hepatic tissue confirmed that the pre-treatment of the fish with PG and NPG prior to the exposure to CM could sufficiently maintain the histological architecture, middle positioned nucleus, their compactness, etc., as these were nearly the same as those of the control group. The CM-treated group, on the other hand, showed necrosis, a loss of compactness, disruption of hepatopancreases, and abnormality in the position of the nucleus, which were not found in the pre-treated PG and NPG + CM groups (Fig. S4A–E†). The CM-treated kidney tissue showed glomerular detachment from the epithelial lining, and peritubular oedema (Fig. S4F–J†), while the spleen exhibited hemosiderin deposition and an aggregation of melano-macrophages, and a significant reduction in white pulp that was not observed in the pre-treated-NPG-groups (Fig. S4K–O†). The CM-treated gill tissue showed a large vacuole in the primary lamellae, a curling of the secondary lamellae, reduced interlamellar space, and degenerated secondary lamellae (Fig. 4A–D), while a degenerated and split muscle fibre, and vacuolated and degenerated muscle tissue were observed following treatment with CM (Fig. 4E–H). However, the fish pre-treated with NPG followed by CM exposure did not show any significant histological changes in gill and muscle tissues. Moreover, the brain tissue in the CM-treated group showed nuclear abnormalities and a loss of compactness, which was not observed in the pre-treated NPG groups followed by CM treatment (Fig. 4I–L). Histological analysis of testis tissue of the CM-treated group showed a reduction in the size of the seminiferous tubules, vacuolation, necrosis in spermatocytes, and damage to the histological architecture. However, the pre-treated NPG groups were able to maintain the proper functioning structure of the testes similar to in the control group after exposure to CM (Fig. 4M–P).
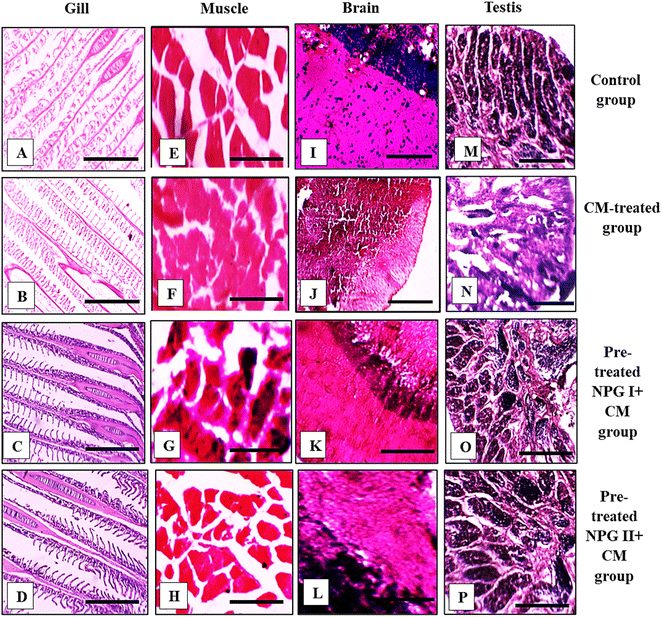 |
| Fig. 4 Histopathological changes were analysed in different tissues, such as the gills (A–D), muscles (E–H), brains (I–L), and testes (M–P) obtained from the experimental groups, including the control set. Scale of images = 4.5 nm. | |
3.9 Determination of ROS generation from hepatic cell suspensions
The overall data showed that the fish pre-treated with PG, NPG I, and NPG II followed by exposure to CM could prevent extreme intracellular ROS generation in hepatic tissue when compared to the group treated with CM alone (Fig. S5†).
3.10 Analysis of ROS, nuclear condensation, and DNA damage in histological sections of hepatic tissue
According to the confocal microscopic examinations (Fig. 5), only the CM-treated group produced more ROS than the pre-treated PG and NPG + CM groups. CM treatment promoted the generation of free radicals in hepatic tissue, as demonstrated by staining the tissue with the fluorescent dye 2′,7′-dichlorodihydrofluorescein diacetate (H2DCFDA). The fluorescence intensities of Hoechst and PI dye were reduced in the PG and NPG pre-treated groups, indicating that PG and NPG could suppress ROS-induced DNA damage and nuclear condensation more effectively than CM treatment alone.
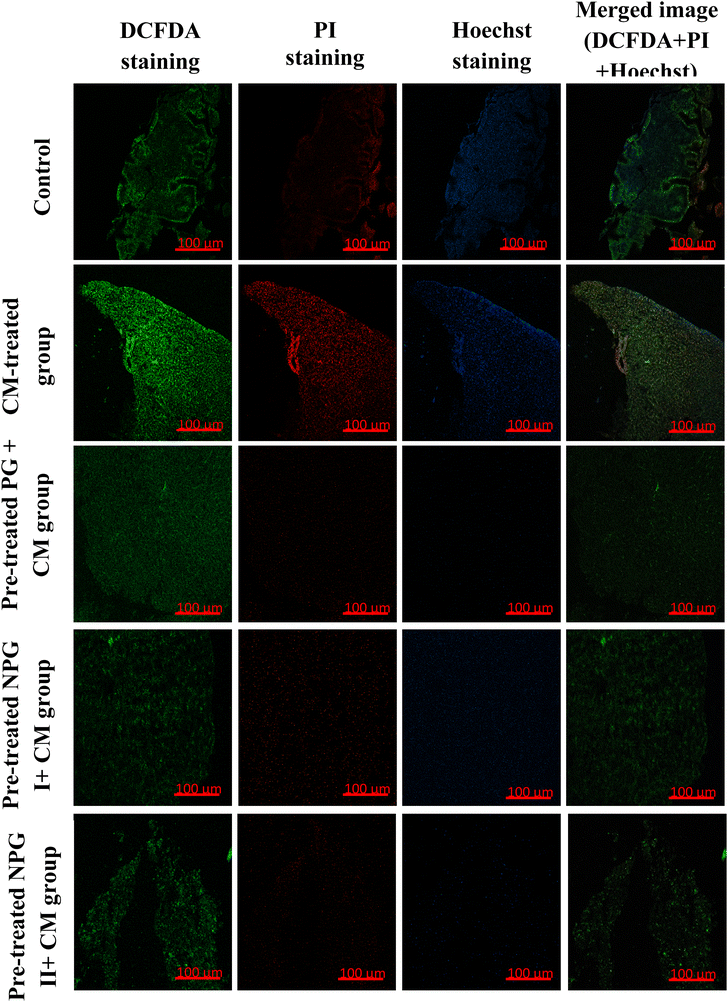 |
| Fig. 5 Fluorescence study of ROS generation, DNA damage, and nuclear condensation in the hepatic tissues of the PG- and NPG-treated groups impacted by CM-induced toxicity. | |
3.11 Determination of the anti-oxidative enzyme profile in hepatic tissue
A significant increase was observed in the catalase activity and levels of SOD and total thiol enzymes in the pre-treated PG and NPG groups, when compared with the group with CM treatment alone. The pre-treated NPG I group showed higher levels of catalase, SOD, and total thiol enzymes than the NPG II-treated group. The level of LPO enzyme was observed to be elevated in the CM-treated group when compared with the untreated control group and pre-treated NPG groups (Fig. 6).
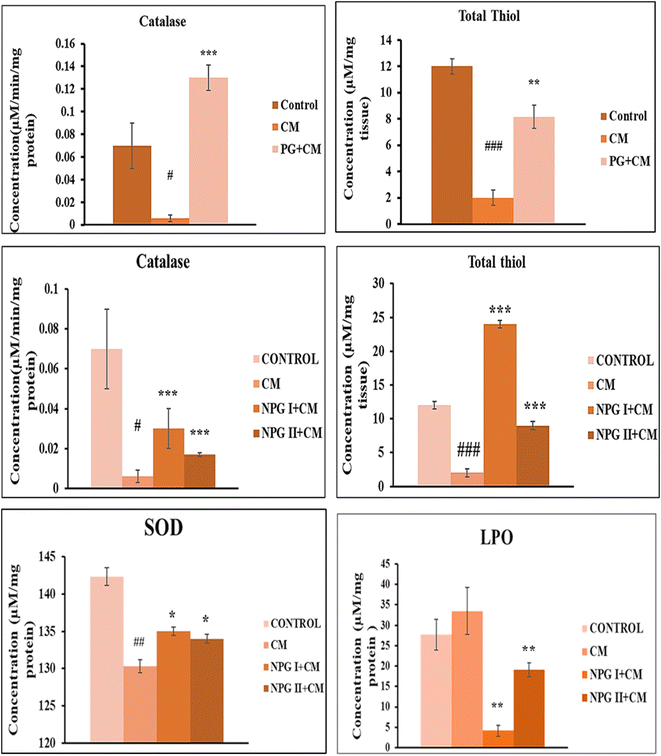 |
| Fig. 6 Analysis of the anti-oxidative enzymes profiles of all experimental groups of tilapia fish. ###p < 0.001 vs. control, ##p < 0.01 vs. control, #p < 0.05 vs. control, ***p < 0.001 vs. CM, **p < 0.01 vs. CM, *p < 0.05 vs. CM. | |
3.12 Analysis of the hepatic enzymes level
Active biomarker enzymes were induced during the onset of hepatotoxicity after exposure to CM. The elevated acid phosphatase and LDH levels were restricted in the pre-treated PG and NPG groups prior to CM exposure. Nevertheless, alkaline phosphatase was inhibited by CM treatment and was found to be near to normal values in the pre-treated NPG groups (Fig. S6†).
3.13 Analysis of the DNA fragmentation assay in tilapia fish
Fig. S7† shows the results of the gel electrophoresis investigation of DNA fragmentation in the tilapia fish liver, spleen, and brain tissues. In contrast to the control and other experimental groups, the CM-treated fish group had an expanded smearing of extracted genomic DNA from the hepatic, spleen, and brain tissues. However, the fish pre-treated with NPG I and NPG II and then given CM treatment had decreased DNA band smearing that was nearly identical to the control group.
3.14 Analysis of micronucleus formation in blood
When compared to the control group, the fish exposed to the pesticide CM exhibited a substantial increase in the amount of micronucleus formation in the blood. In contrast to the CM-treated group, the findings (Table S1†) from the pre-treated NPG I + CM and NPG II + CM groups showed a decrease in micronucleus development.
3.15 Determination of serum blood glucose level
According to the GOD-POD research, the fish that had been pre-treated with NPG I and NPG II before being exposed to CM displayed blood glucose levels that were nearly normal, as opposed to the elevated levels shown in the fish that had been treated with CM (Fig. S8†).
3.16 Estimation of the mitochondrial membrane potential
As compared to the control group of tilapia fish, the MMP level was significantly decreased in the CM-treated group, indicating the depolarization of the mitochondrial membrane state. However, the pre-treated PG and NPG groups showed an upsurge of rhodamine 123 intensity, which indicated that NPG was able to re-establish the hyperpolarized mode of the mitochondrial membrane potential. In this case, NPG I was revealed to be a more potent candidate for maintaining the MMP level compared to NPG-II (Fig. 7).
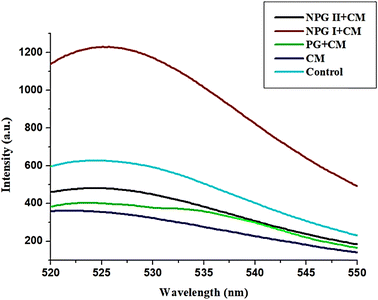 |
| Fig. 7 Analysis of the mitochondrial membrane potential in the control, CM-treated, and PG + CM, NPG I + CM, and NPG II + CM treated groups. | |
3.17 Quantitative assessment of ATPase activity
A significantly increased level of ATPase enzyme was observed in the CM-treated group compared to the control group, which indicated that the ATP level subsided after exposure to CM. However, the pre-treated PG and NPG groups could efficiently increase the ATP level in hepatic tissue, and, as a result, a reduction in ATPase enzyme was observed in the nano-treated groups (Fig. S9†).
3.18 Estimation of the intracellular calcium level
Compared to the CM-treated group, the pre-treated NPG groups showed a significantly higher level of intracellular calcium in hepatic tissue after CM exposure. It was observed that NPG I was more efficient in restoring the calcium level than pre-treatment with NPG II (Fig. S10†).
3.19 Analysis of the expression levels of p53, PARP, HSP 90, and XRCC1 stress proteins by immunofluorescence study in hepatic tissue
According to the immunofluorescence investigation, the pre-treated NPG I and NPG II fish groups that were then exposed to CM had higher expression levels of the proteins p53, PARP, and XRCC1 in their hepatic tissue than the fish groups that had just been exposed to CM. By preventing its function as a molecular chaperon to start stress-induced cellular to organismal dysfunctioning, the NPG pre-treatment could lower the expression level of HSP 90 protein in hepatic tissue compared to the CM-treated group, thereby controlling the normal cell level homoeostasis. Fig. 8a presents visual representations of the immunofluorescence investigations for the levels of HSP 90, p53, PARP, and XRCC1 protein expression in the liver tissue of all the experimental groups of tilapia fish. For a better understanding of the interaction between these signalling proteins in several experimental groups of tilapia fish, the intensity profiles of the p53, PARP, and HSP 90 protein expression levels were statistically depicted as a heatmap (Fig. 8b).
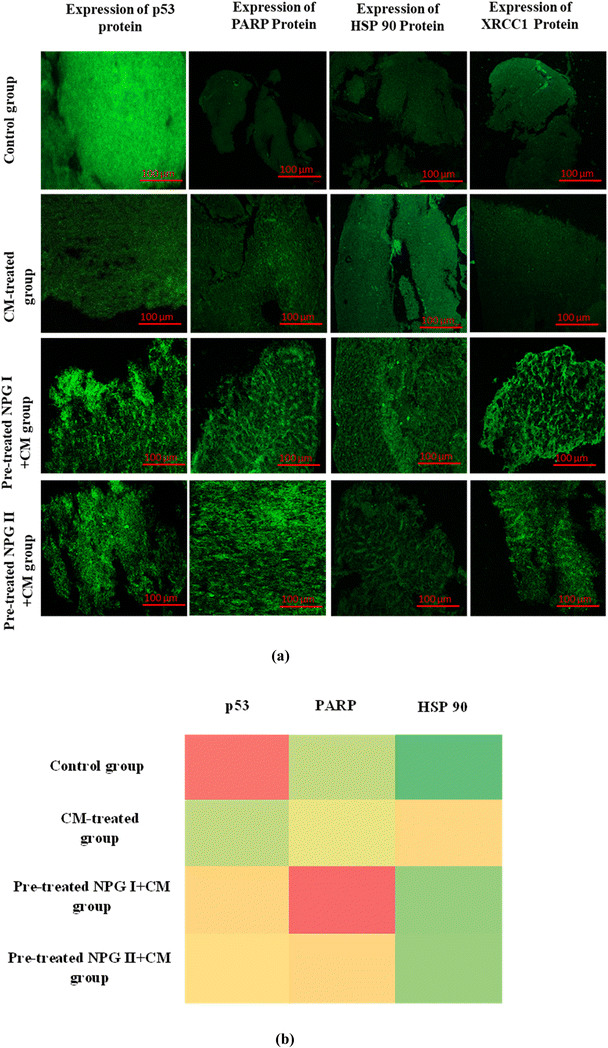 |
| Fig. 8 (a) Immunofluorescence study of p53, PARP, HSP 90, and XRCC1 protein expression levels in the hepatic tissue of all the experimental groups of tilapia fish. (b) Heatmap image illustrating the levels of p53, PARP, and HSP 90 protein expression in the tilapia fish liver tissue. Here, the p53, PARP, and HSP 90 proteins, which are key players in avoiding DNA damage, are shown in a schematic illustration of the DNA repair pathway with red denoting greater value, yellow denoting intermediate value, and green denoting lower value. | |
4. Discussion
Pesticides are widely used for agricultural and non-agricultural purposes worldwide, affecting both target and non-target organisms whenever they get exposed to these chemicals. However, the bioaccumulation and biotransformation of pesticides can lead to an overall toxic effect of the pesticides on secondary consumers, which needs to be addressed urgently. The importance of the present research work emphasizes delineating the issue of non-target organism toxicity by deploying the prospect of nanotechnology coupled with the goodness of phytocompounds to protect the health of fish and their nutritional value when consumed.
A phytochemical-based environmentally friendly biodegradable polymeric nanoparticle was synthesized using pelargonidin as the core ingredient. The nano-pelargonidin (NPG) had an average size of ∼64 nm with a spherical shape and smooth surface, possessing a negative zeta potential. All these qualities along with their good dispersal index and entrapment efficacy make NPG ideal for targeted drug delivery to tissues. The interaction of drugs with DNA is a major aspect of pharmacology and is crucial for understanding the route of drug effectiveness for developing more potential drugs with precisely tailored medications with fewer side effects. The results obtained from both Tm profile and CD spectral analyses clearly indicated that NPG interacted with CT-DNA on a higher scale than PG. The intensification of both the positive and negative bands of DNA clearly indicated resilient intercalation and stabilization of the right-handed B conformation of DNA.67 As per earlier reports, the bioavailability of NPG in different tissues was spectrophotometrically detected, where NPG was found to be more available in tissues in relation to PG, suggesting a high penetrability of NPG at 6 h post-treatment. The potential accumulation of the nanoparticles in different tissues indicated that the core phytochemical PG was absorbed in tissues without structural degradation. This strongly advocates the beneficial role of PLGA as a nanocarrier that ultimately governs the tissue penetration and overall distribution of the drug in the nano-form.18 Further, the stability curve determined that the nanoparticle suspension maintained NPG as stable without deteriorating over a range of time intervals, and as a result, it demonstrated an enhanced bioavailability and biodistribution of the drug in different tissues. NPG with support from the polymeric platform demonstrated enhanced drug efficacy and protective action against CM-induced toxicity. Therefore, NPG might function by interfering in cell divisional activities and potentially delaying cytotoxicity; NPG may also activate signalling factors to initiate DNA protection, thereby potentially preventing cells from undergoing the process of apoptosis.
Cypermethrin (CM), a widely used 4th generation pyrethroid for crop pest management, has negative effects on the aquatic environment, especially fish, which are very sensitive to this pesticide. The NMR and FTIR data of cypermethrin revealed that cypermethrin was unchanged from its actual structural and chemical form in water bodies after reacting with sunlight and oxygen at the end of the photoreduction reaction. Therefore, CM could accelerate the toxic impact, which was observed as a dysfunctioning of hepatic enzymes, histopathological alteration, ROS-mediated mitochondrial damage, and genotoxicity of fish via oxidative stress generation. ROS-mediated detrimental effects on DNA and cell nuclei were observed in group treated with CM alone, where a substantial level of DNA damage and nuclear condensation was seen, leading to the apoptosis of hepatic tissue. The remarkable cellular alterations observed in the CM-exposed tilapia fish were a depolarization of the mitochondrial membrane potential, inhibition of ATP and calcium production, micronucleus formation, and modulation of the DNA repairing signalling proteins, such as p53, PARP, HSP 90, and XRCC1. CM can modulate p53-mediated DNA repairing signalling with enhanced HSP 90 protein expression, which can thereby inhibit polymerase enzyme activity by impeding XRCC1 activation. DNA may be directly linked to CM-induced ROS generation, where free radicals can readily interact with DNA bases to break DNA double strands and reduce the ability of DNA-restoring proteins to self-repair against DNA fragmentation, which causes apoptosis in brain, spleen, and liver cells. Thus, the goal of nanoparticle-based drug-delivery system to maximize medication effectiveness while minimizing cytotoxicity was achieved with scientific validation of the dose and cost-effectiveness.
Tilapia pre-treated with NPG exhibited better inhibition against CM-induced cytotoxic effects in hepatic cells, enriching the overall nutritional importance of these consumable fish by maintaining an optimum balance in the physiology of the cells, cellular biochemistry, sub-cellular functioning, as well as survival rate. Histopathological examination, an essential biomarker for future inquiry at the sub-cellular level, can assist in estimating the risk factor of environmental toxicants for monitoring environmental health. Here, the fish pre-treated with PG and NPG provided superior protection to the tissue architecture against pesticide-induced harm. The CM-treated fish had elevated blood glucose levels, which were inhibited or controlled to a greater extent by PG and NPG pre-treatment, suggesting that stress protein elevation may block glycogen catabolism by modulating the catecholamine hormones essential for insulin secretion and glucose homeostasis.
An excessive accumulation of hazardous substances in the hepatic tissue produces a variety of enzymatic alterations. Due to adaptive reaction to the cytotoxic and genotoxic impacts of CM in hepatic tissue, alkaline phosphatase plays an essential role in transferring the metabolites across membranes, the transphosphorylation reaction, and may alter the glycogen content by the activation of phosphorylase enzymes that are involved in glycogen synthesis. Pre-treatment with NPG in fish could significantly promote the synthesis of glycogen by inactivating phosphorylase enzymes, and reducing the acid phosphatase level, which is involved in the breakdown of glycogen for getting the needed energy to overcome the stress imparted by CM. Moreover, the acid phosphatase level in hepatic tissue was found to be decreased in the NPG-treated group because NPG could prevent the activity of this lysozyme enzyme that is involved in the hydrolysis of ester linkages between the phosphate esters. LDH, an oxidoreductase class of enzyme, helps in cellular respiration by converting the lactate to pyruvate, which is a reversible process with the reduction of NAD+ to NADH and vice versa. CM-induced hepatoxicity requires energy for cell survival in that stress condition and as a result, LDH was found to be highly activated for meeting the energy demand in a short period of time by anaerobic respiration, which might have a detrimental effect on the fish body. In contrast, the LDH activity was near to normal in the nanoparticles-treated group, because the nano-phytochemical could prevent the interrupted cellular respiration by the epithelium and also the necrosis promoted by disruption of the membrane permeability, which was shown in the fish exposed to CM alone.
Fluorescence analysis is an excellent method for evaluating the formation of ROS. Here, H2DCFDA was oxidized quickly to 2′,7′-dichlorofluorescein (DCF), a highly fluorescent compound with an emission peak at about 526 nm, after binding with free radicals produced by CM exposure. Considering the amount of free radicals produced in the cellular/tissue milieu, H2DCFDA was directly proportional to DCF. However, modification of the stress proteins, such as HSP 90, occurs when pesticides repeatedly generate ROS, which causes tissue damage and total cell death. The present study demonstrated that the pelargonidin-based nano-formulation could effectively capture free radicals, exhibiting adequate anti-oxidant activity after being exposed to CM.
In this study, the assessment of ROS generation corroborated well with the activity of the anti-oxidant enzymes and thus strongly verified that pre-treatment with NPG could modulate the anti-oxidative mechanism and other signalling pathways in the hepatic tissue of tilapia fish for regulating intracellular ROS generation induced by CM, thereby controlling the overall hepatotoxicity. It was clearly observed in ROS-induced oxidative stress that the LPO level increased in the CM-treated group caused by ferroptosis, which was triggered by oxidative attack on the lipids to produce MDA. The pre-treatment with NPG could diminish the peroxidation process of lipid molecules that were involved in the disruption of membrane construction, elucidating subsequent changes in flexibility and permeability, changes in ion transport, and the suppression of metabolic processes. The largest intracellular low molecular weight non-protein thiol molecule is known to be a primary defence anti-oxidant enzyme that can modulate ROS-induced lipid peroxidation. This total thiol level was decreased by CM treatment, resulting in an increased generation of free radicles, which were observed to be much lower in the pre-treated NPG group. For the metalloenzyme group, SOD could dismutase the reactive superoxide anion free radical (O2−) to form molecular oxygen and H2O2, the main substrate for catalase enzyme. Haem-containing enzyme catalase could oxidize H2O2 and rapidly break down into oxygen and water to maintain cellular redox homeostasis. PLGA-encapsulated NPG could increase the total thiol, SOD, and CAT enzyme levels and also inhibit the LPO level to regulate the ROS and ROS-mediated toxicity. A ROS-mediated detrimental effect was observed on DNA and the nuclei in the group treated with CM alone. The substantial level of DNA damage was caused by oxidized nucleoside bases of DNA, which increased the rate of oxidation of DNA, while nuclear condensation was seen in the fluorescence study, leading to the apoptosis of CM-treated hepatic tissue.
CM-induced ROS trafficking within the mitochondria could be a positive-feedback process, resulting in increased ROS generation that could be spread throughout the cell and cause noticeable mitochondrial and cellular injury. PLGA-encapsulated NPG could regulate the oxidative phosphorylation for producing a significant amount of ATP through electron-transport chains after being exposed to CM, which was disrupted in the CM-treated group. Along with maintaining the ATPase enzyme activity, both PG and NPG could prevent the depolarization of the mitochondrial membrane potential, which helped in opening calcium-gated ions channel for maintaining the cytosolic calcium homeostasis. PG and NPG could maintain the polarization of the mitochondrial membrane, which assisted mitochondria in absorbing more calcium ions and channelling them to produce energy as a form of ATP to facilitate cell metabolism, survival, and proliferation.
To the best of our knowledge, this study was the first to demonstrate how PG and NPG could help prevent pesticide-induced genotoxicity by modulating p53, PARP, XRCC1, and HSP 90 protein expression in a tilapia fish model. From the confocal images showing the protein expression, it could be stated that NPG-activated PARP proteins in hepatic tissue inhibited CM-induced genotoxicity through DNA fragmentation, resulting in a concerted activation of the p53 protein, thereby initiating the auto-repair mechanism at the site of DNA damage. Moreover, the stress protein HSP 90 was overexpressed in the CM-treated group, which was responsible for suppressing the activity of the XRCC1 protein at the site of DNA repair. Pre-treatment with NPG could diminish the stress condition by modulating the expression of HSP 90, which could facilitate the activation of downstream signalling of PARP, whereby the XRCC1 protein could efficiently bind with DNA polymerase enzyme for adding nucleosides at the breakage site of DNA strands to prevent DNA fragmentation. The NPG-fed fish showed a greater control over micronucleus formation than the CM-fed fish, which might be due to abnormal p53 and PARP expressions induced by CM. This synergistic interaction between p53 and PARP and antagonistic interaction between HSP 90 and XRCC1 were interplayed at the molecular level after the nanoparticles application, which could be considered as a drug response against pesticide-induced toxicity in a fish model that could aid better fish health and a better quality of fish protein and nutrients. From the overall data, it could be concluded that the molecular docking-based prediction perfectly corroborated with the protective activity of NPG, whereby it showed a greater preventive role against pesticide-exposed fish, thus making humans less vulnerable from consuming such fish.
Recent advancements in the field of nanotechnology in medication design highlights the widespread significance of nanotechnology-based drug delivery for boosting the efficacy of individual therapeutic components against a range of diseases, as was shown here in light of the fact that PLGA-mediated nano-drug delivery demonstrated several advantages, including the capacity to retain large amounts of the drug component, potentiating suspended release, easy intake in targeted tissue without hampering cellular architecture, and improved bioavailability and biocompatibility. It has been stated that the active component, pelargonidin (PG) possesses anti-inflammatory, anti-carcinogenic, anti-oxidant, and anti-genotoxic properties by being loaded within a PLGA nano shell, which offers preventive effectiveness against cypermethrin-induced toxicity in fish biosystem. The significance of this present research work should therefore enhance the usage of phytochemicals targeting specific proteins and the use of advanced nanotechnology tools to minimize or reduce the side effects of drugs for use in a cost-effective dose and thereby restore a synchronization between the environment and mankind in an eco-friendly manner.
5. Conclusion
Nanotechnology-based therapeutic strategies using the nano-formulated pelargonidin drug could restrict the harmful effects of environmental toxicants in fish and human population. This present research work shows the links between phytochemical and advanced nanotechnology to allow minimizing or completely reducing the side effects of drugs in a much lower dose of drug, which would directly lower the overall treatment cost. Overall, the findings resoundingly demonstrate that by using the nano-formulated pelargonidin drug, targeted protein-based therapeutic strategies could more effectively limit the negative effects of environmental toxicants in fish and the human population consuming them. This present work could re-establish a synchronisation between the environment and mankind in a sustainable way. This research work suggests the implementation of nano-drugs by using phytochemicals as a core constituent that would pave a new insight in the arena of pharmacology and support the interested pharma industry to manufacture nano-based anti-pesticidal drugs for the dual purpose of better serving the aquaculture industry and safeguarding the health concerns of humankind.
Author contributions
Conceptualization: PS and AS; data curation: PS, SD, RD; funding acquisition: AS; investigation: AS, SN, and DT; software: RD and SN for in silico study; supervision: AS; validation: AS, SN, DT, and ARKB; roles/writing – original draft: PS; and writing – review & editing: AS, SN, DT, and ARKB.
Conflicts of interest
There are no conflicts to declare.
Acknowledgements
The authors would like to express their gratitude to DST SERB (Grant No.: ECR/2017/000355/LS dated 15.11.2017) and UGC, New Delhi for the UGC-BSR grant awarded to Dr. Asmita Samadder which was used for this research study. The authors would also like to thank the UGC DAE, Kolkata centre for DLS instrumentation. The authors would also like to thank the University of Kalyani for giving a Personal Research Grant which was partly utilised in this work. PS, SD, and RD were grateful to UGC for their UGC SRF fellowship. The authors are grateful to their respective institutes for providing the essential research infrastructure.
References
- B. Chakraborty, Effect of pesticide and heavy metal toxicants on fish and human health, J. Crop Weed, 2023, 19(1), 1–7 CrossRef
.
- C. Plessl, E. O. Otachi, W. Körner, A. Avenant-Oldewage and F. Jirsa, Fish as bioindicators for trace element pollution from two contrasting lakes in the Eastern Rift Valley, Kenya: spatial and temporal aspects, Environ. Sci. Pollut. Res., 2017, 24, 19767–19776 CrossRef CAS PubMed
.
-
S. Aziz, M. Mumraiz, F. Latif and M. Sarfraz Ali, Cypermethrin-Induced Reproductive Toxicity in Zebrafish: Biochemical and Molecular Perspective, in Xenobiotics in Aquatic Animals: Reproductive and Developmental Impacts, Springer Nature Singapore, Singapore, 2023 May 11, pp. 123–142 Search PubMed
.
- P. Gupta, A. Mahapatra, A. Suman and R. K. Singh, In silico and in vivo assessment of developmental toxicity, oxidative stress response & Na+/K+-ATPase activity in zebrafish embryos exposed to cypermethrin, Ecotoxicol. Environ. Saf., 2023, 251, 114547 CrossRef CAS PubMed
.
- F. Huang, Q. Liu, S. Xie, J. Xu, B. Huang, Y. Wu and D. Xia, Cypermethrin induces macrophages death through cell cycle arrest and oxidative stress-mediated JNK/ERK signaling regulated apoptosis, Int. J. Mol. Sci., 2016, 17(6), 885 CrossRef PubMed
.
- C. A. Lamey, N. Moussa, M. W. Helmy, M. Haroun and S. A. Sabra, Simultaneous encapsulation of dasatinib and celecoxib into caseinate micelles towards improved in vivo anti-breast cancer efficacy with reduced drug toxicity, J. Drug Delivery Sci. Technol., 2023, 87, 104807 CrossRef CAS
.
- A. Narmani, R. Jahedi, E. Bakhshian-Dehkordi, S. Ganji, M. Nemati, R. Ghahramani-Asl, K. Moloudi, S. M. Hosseini, H. Bagheri, P. Kesharwani and A. Khani, Biomedical applications of PLGA nanoparticles in nanomedicine: Advances in drug delivery systems and cancer therapy, Expert Opin. Drug Delivery, 2023, 20, 937–954 CrossRef CAS PubMed
.
- C. Li, Z. Wang, H. Lei and D. Zhang, Recent progress in nanotechnology-based drug carriers for resveratrol delivery, Drug Delivery, 2023, 30(1), 2174206 CrossRef PubMed
.
- A. K. Rashwan, N. Karim, Y. Xu, J. Xie, H. Cui, M. R. Mozafari and W. Chen, Potential micro-/nano-encapsulation systems for improving stability and bioavailability of anthocyanins: An updated review, Crit. Rev. Food Sci. Nutr., 2023, 63(19), 3362–3385 CrossRef CAS PubMed
.
- A. Samadder, D. Chakraborty, A. De, S. S. Bhattacharyya, K. Bhadra and A. R. Khuda-Bukhsh, Possible signaling cascades involved in attenuation of alloxan-induced oxidative stress and hyperglycemia in mice by ethanolic extract of Syzygium jambolanum: drug-DNA interaction with calf thymus DNA as target, Eur. J. Pharm. Sci., 2011, 44(3), 207–217 CrossRef CAS PubMed
.
- A. Samadder, J. Das, S. Das and A. R. Khuda-Bukhsh, Dihydroxy-isosteviol-methyl-ester, an active biological component of Pulsatilla nigricans, reduces arsenic induced cellular dysfunction in testis of male mice, Environ. Toxicol. Pharmacol., 2012, 34(3), 743–752 CrossRef CAS PubMed
.
- A. Samadder, S. Das, J. Das, A. Paul and A. R. Khuda-Bukhsh, Ameliorative effects of Syzygium jambolanum extract and its poly (lactic-co-glycolic) acid nano-encapsulated form on arsenic-induced hyperglycemic stress: a multi-parametric evaluation, J. Acupunct. Meridian Stud., 2012, 5(6), 310–318 CrossRef PubMed
.
- A. Samadder, J. Das, S. Das, A. De, S. K. Saha, S. S. Bhattacharyya and A. R. Khuda-Bukhsh, Poly (lactic-co-glycolic) acid loaded nano-insulin has greater potentials of combating arsenic induced hyperglycemia in mice: some novel findings, Toxicol. Appl. Pharmacol., 2013, 267(1), 57–73 CrossRef CAS PubMed
.
- A. Samadder, S. Das, J. Das and A. R. Khuda-Bukhsh, Relative efficacies of insulin and poly (lactic-co-glycolic) acid encapsulated nano-insulin in modulating certain significant biomarkers in arsenic intoxicated L6 cells, Colloids Surf., B, 2013, 109, 10–19 CrossRef CAS PubMed
.
- A. Samadder and A. R. Khuda-Bukhsh, Nanotechnological approaches in diabetes treatment: A new horizon, World J. Transl. Med., 2014, 3(2), 84–95 CrossRef
.
- A. Samadder, S. K. Abraham and A. R. Khuda-Bukhsh, Nanopharmaceutical approach using pelargonidin towards enhancement of efficacy for prevention of alloxan-induced DNA damage in L6 cells via activation of PARP and p53, Environ. Toxicol. Pharmacol., 2016, 43, 27–37 CrossRef CAS PubMed
.
- A. Samadder, D. Tarafdar, S. Abraham, K. Ghosh and A. Khuda-Bukhsh, Nano-pelargonidin protects hyperglycemic-induced L6 cells against mitochondrial dysfunction, Planta Med., 2017, 83(05), 468–475 CrossRef CAS PubMed
.
- A. Samadder, D. Tarafdar, R. Das, A. R. Khuda-Bukhsh and S. K. Abraham, Efficacy of nanoencapsulated pelargonidin in ameliorating pesticide toxicity in fish and L6 cells: Modulation of oxidative stress and signalling cascade, Sci. Total Environ., 2019, 671, 466–473 CrossRef CAS PubMed
.
- S. Das, J. Das, A. Samadder, S. S. Bhattacharyya, D. Das and A. R. Khuda-Bukhsh, Biosynthesized silver nanoparticles by ethanolic extracts of Phytolacca decandra, Gelsemium sempervirens, Hydrastis canadensis and Thuja occidentalis induce differential cytotoxicity through G2/M arrest in A375 cells, Colloids Surf., B, 2013, 101, 325–336 CrossRef CAS PubMed
.
- S. Das, J. Das, A. Samadder, A. Paul and A. R. Khuda-Bukhsh, Strategic formulation of apigenin-loaded PLGA nanoparticles for intracellular trafficking, DNA targeting and improved therapeutic effects in skin melanoma in vitro, Toxicol. Lett., 2013, 223(2), 124–138 CrossRef CAS PubMed
.
- J. Das, A. Samadder, S. Das, A. Paul and A. R. Khuda-Bukhsh, Nanopharmaceutical approach for enhanced anti-cancer activity of betulinic acid in lung-cancer treatment via activation of PARP: interaction with DNA as a target, J. Pharmacopuncture, 2016, 19(1), 37–44 CrossRef PubMed
.
- J. Das, A. Samadder, J. Mondal, S. K. Abraham and A. R. Khuda-Bukhsh, Nano-encapsulated chlorophyllin significantly delays progression of lung cancer both in in vitro and in vivo models through activation of mitochondrial signaling cascades and drug-DNA interaction, Environ. Toxicol. Pharmacol., 2016, 46, 147–157 CrossRef CAS PubMed
.
- S. S. Bhattacharyya, S. Paul, A. De, D. Das, A. Samadder, N. Boujedaini and A. R. Khuda-Bukhsh, Poly (lactide-co-glycolide) acid nanoencapsulation of a synthetic coumarin: cytotoxicity and bio-distribution in mice, in cancer cell line and interaction with calf thymus DNA as target, Toxicol. Appl. Pharmacol., 2011, 253(3), 270–281 CrossRef CAS PubMed
.
- P. Sow, S. Dey, R. Dey, A. Majumder, S. Nandi, M. Bera and A. Samadder, Poly lactide-co-glycolide encapsulated nano-curcumin promoting antagonistic interactions between HSP 90 and XRCC1 proteins to prevent cypermethrin-induced toxicity: An in silico predicted in vitro and in vivo approach, Colloids Surf., B, 2022, 220, 112905 CrossRef CAS PubMed
.
- A. Kumari, R. Singla, A. Guliani and S. K. Yadav, Nanoencapsulation for drug delivery, EXCLI J., 2014, 13, 265–286 Search PubMed
.
-
F. B. Schappo, L. S. Assunção, C. D. F. Ribeiro and I. L. Nunes, Poly (Lactic-Co-glycolic Acid)(PLGA) Nanoparticles for Drug Delivery, 1st edn, 2023, pp. 477–506 Search PubMed
.
- J. K. Patra, G. Das, L. F. Fraceto, E. V. Campos, M. D. Rodriguez-Torres, L. S. Acosta-Torres, L. A. Diaz-Torres, R. Grillo, M. K. Swamy, S. Sharma and S. Habtemariam, Nano based drug delivery systems: recent developments and future prospects, J. Nanobiotechnol., 2018, 16(1), 1–33 CrossRef PubMed
.
- S. Dey, A. Samadder and S. Nandi, Exploring current role of nanotechnology used in food processing industry to control food additives and their biochemical mechanisms, Curr. Drug Targets, 2022, 23(5), 513–539 CrossRef CAS PubMed
.
- P. Bhattacharjee, A. Borah and S. Das, Quercetin-induced amelioration of deltamethrin stress in freshwater teleost, Channa punctata: Multiple biomarker analysis, Comp. Biochem. Physiol., Part C: Toxicol. Pharmacol., 2020, 227, 108626 CAS
.
- M. Abdel-Tawwab and H. S. Hamed, Antagonistic effects of dietary guava (Psidium guajava) leaves extract on growth, hemato-biochemical, and immunity response of cypermethrin-intoxicated Nile tilapia, Oreochromis niloticus, fingerlings, Aquaculture, 2020, 529, 735668 CrossRef CAS
.
- K. Eronat and D. Sağır, Protective effects of curcumin and Ganoderma lucidum on hippocampal damage caused by the organophosphate insecticide chlorpyrifos in the developing rat brain: Stereological, histopathological and immunohistochemical study, Acta Histochem., 2020, 122(7), 151621 CrossRef CAS PubMed
.
- G. Albasher, T. Albrahim, N. Alsultan, S. Alfaraj, M. S. Alharthi, R. B. Kassab and A. E. Abdel Moneim, Red beetroot extract mitigates chlorpyrifos-induced reprotoxicity associated with oxidative stress, inflammation, and apoptosis in rats, Environ. Sci. Pollut. Res., 2020, 27, 3979–3991 CrossRef CAS PubMed
.
- A. Samadder, S. Das, B. Pal, S. Das, A. Mandal, P. Biswas, S. Ghosh, S. H. Mandal, P. Sow, R. Das and S. Biswas, First report on chlorophyllin to protect mammalian and fish muscle cells from pesticide toxicity via activation of p53 and PARP, Aquac. Fish., 2021, 6(4), 393–399 CrossRef
.
- A. Samadder, S. Dey, P. Sow, R. Das, S. Nandi, J. Das, B. Bhattacharjee, A. Chakrovorty, M. Biswas and P. Guptaroy, Phyto-chlorophyllin prevents food additive induced genotoxicity and mitochondrial dysfunction via cytochrome c mediated pathway in mice model, Comb. Chem. High Throughput Screening, 2021, 24(10), 1618–1627 CrossRef CAS PubMed
.
- S. Dey, I. Nagpal, P. Sow, R. Dey, A. Chakrovorty, B. Bhattacharjee, S. Saha, A. Majumder, M. Bera, N. Subbarao and S. Nandi, Morroniside interaction with poly (ADP-ribose) polymerase accentuates metabolic mitigation of alloxan-induced genotoxicity and hyperglycaemia: a molecular docking based in vitro and in vivo experimental therapeutic insight, J. Biomol. Struct. Dyn., 2023, 8, 1–18 Search PubMed
, just accepted.
-
P. Sow and A. Samadder, Nanotechnological Approach in Combating Pesticide Induced Fish Toxicity: Pros and Cons Controversy, in Proceedings of the Zoological Society, Springer India, 2021, pp. 1–15 Search PubMed
.
- A. S. Ribeiro, M. Estanqueiro, M. B. Oliveira and J. M. Sousa Lobo, Main benefits and applicability of plant extracts in skin care products, Cosmetics, 2015, 2(2), 48–65 CrossRef CAS
.
- S. Yoo, K. Kim, H. Nam and D. Lee, Discovering health benefits of phytochemicals with integrated analysis of the molecular network, chemical properties and ethnopharmacological evidence, Nutrients, 2018, 10(8), 1042 CrossRef PubMed
.
- P. Sow, R. Das, S. Dey, R. Dey, M. Biswas, S. Nandi, D. Tarafdar, A. Samadder and A. R. Khuda-Bukhsh, Natural curcumin prevents cypermethrin induced toxicity in fish via p53 protein modulation, J. Global Trends Pharm. Sci., 2021, 12, 1955–1972 Search PubMed
.
- W. Li, G. Zhang, S. Tan, C. Gong, Y. Yang, M. Gu, Z. Mi and H. Y. Yang, Polyacylated anthocyanins derived from red radishes protect vascular endothelial cells against palmitic acid-induced apoptosis via the p38 MAPK pathway, Plant Foods Hum. Nutr., 2022, 77(3), 412–420 CrossRef CAS PubMed
.
- E. R. Rashed, T. El-Hamoly, M. M. El-Sheikh and M. A. El-Ghazaly, Pelargonidin ameliorates reserpine-induced neuronal mitochondrial dysfunction and apoptotic cascade: a comparative in vivo study, Drug Chem. Toxicol., 2023, 46(3), 462–471 CrossRef CAS PubMed
.
- Z. Tian, C. Sun and J. Liu, Pelargonidin inhibits vascularization and metastasis of brain gliomas by blocking the PI3K/AKT/mTOR pathway, J. Biosci., 2022, 47(4), 64 CrossRef CAS PubMed
.
- S. Wang, Q. Du, X. Meng and Y. Zhang, Natural polyphenols: a potential prevention and treatment strategy for metabolic syndrome, Food Funct., 2022, 13(19), 9734–9753 RSC
.
- R. Dey, S. Nandi and A. Samadder, Pelargonidin mediated selective activation of p53 and parp proteins in preventing food additive induced genotoxicity: an in vivo coupled in silico molecular docking study, Eur. J. Pharm. Sci., 2021, 156, 105586 CrossRef CAS PubMed
.
- D. T. Gaikwad, S. P. Bansode, D. P. Mali, G. H. Wadkar, V. T. Pawar and F. A. Tamboli, Promising Discovery of Alpha Amylase Enzyme Inhibitors from Terminalia arjuna for Antidiabetic Potential, Technology, 2022, 12(3), 1020–1024 Search PubMed
.
- S. K. Abraham, N. Schupp, U. Schmid and H. Stopper, Antigenotoxic effects of the phytoestrogen pelargonidin chloride and the polyphenol chlorogenic acid, Mol. Nutr. Food Res., 2007, 51(7), 880–887 CrossRef CAS PubMed
.
- N. Khandelwal and S. K. Abraham, Intake of anthocyanidins pelargonidin and cyanidin reduces genotoxic stress in mice induced by diepoxybutane, urethane and endogenous nitrosation, Environ. Toxicol. Pharmacol., 2014, 37(2), 837–843 CrossRef CAS PubMed
.
- N. Khandelwal and S. Abraham, Protective effects of common anthocyanidins against genotoxic damage induced by chemotherapeutic drugs in mice, Planta Med., 2014, 80(15), 1278–1283 CrossRef CAS PubMed
.
- S. Dhivya, N. Khandelwal, S. K. Abraham and K. Premkumar, Impact of anthocyanidins on mitoxantrone-induced cytotoxicity and genotoxicity: an in vitro and in vivo analysis, Integr. Cancer Ther., 2016, 15(4), 525–534 CrossRef CAS PubMed
.
- H. P. Fessi, F. Puisieux, J. P. Devissaguet, N. Ammoury and S. Benita, Nanocapsule formation by interfacial polymer deposition following solvent displacement, Int. J. Pharm., 1989, 55, R1–R4 CrossRef CAS
.
- I. Horcas, R. Fernández, J. M. Gomez-Rodriguez, J. W. Colchero, J. W. Gómez-Herrero and A. M. Baro, WSXM: A software for scanning probe microscopy and a tool for nanotechnology, Rev. Sci. Instrum., 2007, 78(1), 013705 CrossRef CAS PubMed
.
- S. Haldar, G. Vijaykumar, L. Carrella, S. Batha, G. T. Musie and M. Bera, Inorganic Phosphate and Arsenate within New Tetranuclear Copper and Zinc Complexes: Syntheses, Crystal Structures, Magnetic, Electrochemical, and Thermal Studies, ACS Omega, 2017, 2(4), 1535–1549 CrossRef CAS PubMed
.
- C. E. Stebbins, A. A. Russo, C. Schneider, N. Rosen, F. U. Hartl and N. P. Pavletich, Crystal structure of an Hsp90–geldanamycin complex: targeting of a protein chaperone by an antitumor agent, Cell, 1997, 89(2), 239–250 CrossRef CAS PubMed
.
- J. Miyashiro, K. W. Woods, C. H. Park, X. Liu, Y. Shi, E. F. Johnson, J. J. Bouska, A. M. Olson, Y. Luo, E. H. Fry and V. L. Giranda, Synthesis and SAR of novel tricyclic quinoxalinone inhibitors of poly (ADP-ribose) polymerase-1 (PARP-1), Bioorg. Med. Chem. Lett., 2009, 19(15), 4050–4054 CrossRef CAS PubMed
.
- J. L. Avalos, K. M. Bever and C. Wolberger, Mechanism of sirtuin inhibition by nicotinamide: altering the NAD+ cosubstrate specificity of a Sir2 enzyme, Mol. Cell, 2005, 17(6), 855–868 CrossRef CAS PubMed
.
- K. Ghosh, D. Tarafdar, A. Samadder and A. R. Khuda-Bukhsh, Piperazine-based simple structure for selective sensing of Hg2+ and glutathione and construction of logic circuit mimicking INHIBIT gate, New J. Chem., 2013, 37, 4206–4213 RSC
.
- H. Ohkawa, N. Ohishi and K. Yagi, Assay for lipid peroxides in animal tissues by thiobarbituric acid reaction, Anal. Biochem., 1979, 95(2), 351–358 CrossRef CAS PubMed
.
- P. Kakkar, B. Das and P. N. Viswanathan, A modified spectrophotometric assay of superoxide dismutase, Indian J. Biochem. Biophys., 1984, 21(2), 130–132 CAS
.
- A. Maehly, Chance B The assay of catalases and peroxidases, Methods Biochem. Anal., 1954, 1, 357–424 CAS
.
- J. Sedlak and R. H. Lindsay, Estimation of total, protein-bound, and nonprotein sulfhydryl groups in tissue with Ellman's reagent, Anal. Biochem., 1968, 25(1), 192–205 CrossRef CAS PubMed
.
- P. R. Kind and E. King, Estimation of plasma phosphatase by determination of hydrolysed phenol with amino-antipyrine, J. Clin. Pathol., 1954, 7(4), 322–326 CrossRef CAS PubMed
.
- D. Weisshaar, E. Gossrau and B. Faderl, Normal ranges of alpha-HBDH, LDH, AP, and LAP as measured with substrate-optimated test charges, Med. Welt, 1975, 26(9), 387–392 CAS
.
- A. Majumder, N. Dutta, S. Dey, P. Sow, A. Samadder, G. Vijaykumar, K. Rangan and M. Bera, A Family of [Zn6] Complexes from the Carboxylate-Bridge-Supported Assembly of [Zn2] Building Units: Synthetic, Structural, Spectroscopic, and Systematic Biological Studies, Inorg. Chem., 2021, 60(23), 17608–17626 CrossRef CAS PubMed
.
- M. M. Chowdhury, A. S. Rahman, L. Nahar, M. Rahman, H. Al Reza and M. S. Ahmed, Efficiency of different DNA extraction methods for fish tissues: a comparative analysis, IOSR J. Pharm. Biol. Sci., 2016, 11(3), 11–15 Search PubMed
.
- R. R. Ishmukhametov, J. B. Pond, A. Al-Huqail, M. A. Galkin and S. B. Vik, ATP synthesis without R210 of subunit a in the Escherichia coli ATP synthase, Biochim. Biophys. Acta, Bioenerg., 2008, 1777(1), 32–38 CrossRef CAS PubMed
.
- S. Sk, A. Majumder, P. Sow, A. Samadder and M. Bera, Exploring a new family of designer copper(II) complexes of anthracene-appended polyfunctional organic assembly displaying potential anticancer activity via cytochrome c mediated mitochondrial apoptotic pathway, J. Inorg. Biochem., 2023, 243, 112182 CrossRef CAS PubMed
.
- Y. Kong, M. Li, G. Guo, L. Yu, L. Sun, Z. Yin, R. Li, X. Chen and G. Wang, Effects of dietary curcumin inhibit deltamethrin-induced oxidative stress, inflammation and cell apoptosis in Channa argus via Nrf2 and NF-κB signaling pathways, Aquaculture, 2021, 540, 736744 CrossRef CAS
.
|
This journal is © The Royal Society of Chemistry 2024 |