DOI:
10.1039/D2VA00126H
(Critical Review)
Environ. Sci.: Adv., 2022,
1, 662-679
A review on global energy use patterns in major crop production systems
Received
11th June 2022
, Accepted 10th September 2022
First published on 13th September 2022
Abstract
This study presents the energy assessment of 49 different crops in India, Bangladesh, China, Malaysia, the Philippines, Indonesia, Iran, Turkey, Australia, Nigeria and Thailand. According to the Food and Agricultural Organization (FAO), these crops were selected from the Indicative Crop Classification (ICC) i.e. cereals, vegetables, fruits, oilseeds, legumes/pulses, fiber and tobacco. The data regarding energy consumption of these crops were collected from articles published between 2001 and 2021. The amounts of energy utilized in diversified crop operations such as tillage, sowing, interculture, fertilization, irrigation, chemical applications, harvesting, threshing and transportation have been discussed. The energy input in terms of direct (manual and animal energy and fuel/diesel) and indirect (fertilizers, pesticides, machinery and seed energy) energy sources for various cropping systems is presented here. Various researchers have extensively examined different operations or sources utilizing more than recommended energy worldwide. In several countries, farmers are in practice utilizing more fertilizers and pesticides than their permitted levels. The energy consumption pattern in wheat production showed Turkey (35
737 MJ ha−1) to be the highest user and Australia (10
900 MJ ha−1) to be the lowest energy user. In rice production, Iran (64
158 MJ ha−1) applied substantially higher while the Philippines applied (12
800 MJ ha−1) slightly lower energy in contrast to other countries, whereas, in millet production, India (7000 MJ ha−1) was the highest and Nigeria (3283 MJ ha−1) was the lowest energy consumer. However, in overall crop production, sugarcane was the highest energy utilizing crop (148
020 MJ ha−1), while wheat (259
000 MJ ha−1) was the highest energy-generating crop. The energy ratio, specific energy and energy productivity of various crops varied between 0.76–29.4, 0.15–26.73 MJ kg−1 and 0.04–6.67 kg MJ−1, respectively.
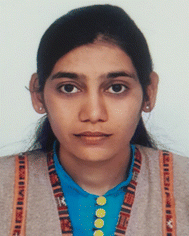 Raveena Kargwal | Dr. Raveena Kargwal completed her Doctor of Philosophy in Processing and Food Engineering from the College of Agricultural Engineering and Technology, Chaudhary Charan Singh Haryana Agricultural University, Hisar (Haryana), in 2021. Currently, she is a Senior Research Fellow, Department of Renewable & Bio Energy Engineering, CCSHAU Hisar, Haryana (India). She has published 11 research papers in various NAAS rated international peer-reviewed journals, 9 book chapters, and 8 articles. She has attended national/international conferences and also attended an international workshop under the SPARC project, MHRD, Govt. of India. |
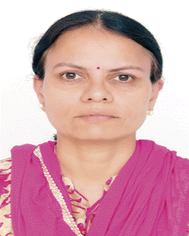 Yadvika | Dr. Yadvika is an Associate Professor in the Department of Renewable & Bio Energy Engineering, College of Agricultural Engg. & Technology, CCSHAU, Hisar, Haryana (India). She has completed her graduation from the same college and PhD from the Centre for Rural Development, IIT Delhi. Her research interests are biogas technology, biofuels, vermicomposting, energy auditing of agriculture & agro-based industries, densification of crop residues, solar drying, solar refrigeration etc. |
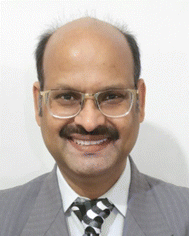 Anil Kumar | Dr. Anil Kumar completed his Doctor of Philosophy from the Centre for Energy Studies, Indian Institute of Technology Delhi, India, in 2007. He is an Associate Professor, Mechanical Engineering Department and Additional Coordinator, Centre for Energy and Environment, Delhi Technological University. He has published 173 papers in international peer-reviewed journals and 80 papers in international/national conference proceedings. He has received more than 5753 + citations with a 41 h-index (Google Scholar) and 3368 + citations with a 32 h-index (SCOPUS). His areas of specialization are energy technology, renewable energy, solar energy applications, and environmental issues. Dr Kumar has visited countries, namely the UK, Thailand, and Malaysia. |
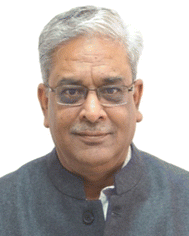 Mukesh Kumar Garg | Dr. Mukesh Kumar Garg completed his Doctor of Philosophy from the Indian Agricultural Research Institute, New Delhi. He started his career at Haryana Agricultural University, Hisar as an Assistant Professor in 1985. Dr Garg has been involved in design, development and field evaluation of various pieces of post harvest machinery/processing equipment, namely feed block machines, single pot solar cookers, aonla pricking machines, petha (ash gourd) pricking machines, machines for mixing wheat straw with molasses, etc. Dr Garg has authored three books and also published about 90 research papers, 40 popular articles, 13 practical/training manuals, 10 book chapters, 4 extension leaflets and 1 patent. |
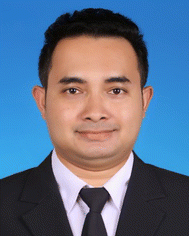 Issara Chanakaewsomboon | Dr. Issara Chanakaewsomboon is working as an instructor at the Faculty of Environmental Management, Prince of Songkla University, Thailand. He is also working as a director at the Sustainable Innovation Center (SIC-PSU). He has experience in twelve industrial service projects. He is currently on the Editorial Board of the Journal of Energy, Environmental & Chemical Engineering, published by the Science Publishing Group. He has published articles in various peer-reviewed journals and completed his PhD in Chemical Engineering from Prince of Songkla University, Thailand. Specialties: sustainable engineering, high value-added products from agro-wastes, biofuel technology, and chemical engineering. |
Environmental significance
This study would provide valuable information to farmers and policymakers on a global scale, allowing them to recommend appropriate changes in agricultural practices that would result in substantial energy savings in agriculture production. It will also provide valuable information to farmers and decision-makers, emphasizing the importance of energy management in crop production. The amount of energy input and output differed by geographical location and crop type. Conventional energy consumption in agricultural processing has enhanced environmental damage in recent decades.
|
1. Introduction
In today's modern era, energy plays a strategic role in the economic development of any country. Energy is the most critical input in the agricultural crop production system, ultimately leading to the economic development of any society and country. Proper energy conservation measures, reasonable management, and minimization of energy losses at various steps have become highly influential in developing countries because of the ever-increasing population with substantial energy demands and depleting natural resources. An agricultural farm is both a consumer and producer of energy. The quest for energy and resource conservation agro-techniques is increasing.1 Energy consumption in agricultural production has increased more than in many other areas of the global economy. It is because agricultural production has been more mechanized. Fertilizers and chemicals have become more widely used to boost crop yields, and machinery has become more widely used to boost fieldwork productivity.2
Energy analysis is a fundamental and essential requirement for executing any well-defined energy management program.3 Assessment of energy use patterns in crop production is necessary to efficiently use available natural resources, properly manage/conserve energy, and minimize losses during different unit operations. It would help minimize energy costs and waste without affecting production and quality. Thus, energy auditing of crop production would attempt to harmonize energy consumption and its application. It would also distinguish significant energy-consuming operations and sources.4 Based on operations and sources, energy use plans of different cropping systems such as cereals (wheat, rice, barley, millet, sorghum, and corn), pulses (soybean and green gram), cash crops (cotton and sugarcane), oilseeds (groundnut) and horticultural crops (apples, grapes, tomato, eggplant, potato, chili, and cucumber) have been evaluated by various researchers. Variations have been observed for a similar type of crop in different regions of the world. The energy input and output in wheat production ranged between 10
900–35
737 MJ ha−1 and 37
906–1
00
346 MJ ha−1 while in the case of rice, it ranged between 13
616–64
158 MJ ha−1 and 46
200–2
34
393 MJ ha−1.5–9
It is perceived that the energy use pattern in crop cultivation also varies according to the sources of energy, climatic conditions, geographical location, types of crop, etc. The role of these critical factors in the energy requirements of a particular crop in terms of energy input and output should be assessed. There is an urgent need to identify energy-intensive unit operations in different crop production practices.
This work aims to find and critically analyze global energy consumption patterns in different crop production systems such as cereals, cash crops, horticultural crops, oilseed crops etc. The findings of this work will help researchers and policymakers identify the dominant energy-intensive/wasting operations. It would also assist extension specialists in suggesting appropriate procedures and energy sources to producers (farmers and processors) to optimize the input energy and reduce the energy losses. Therefore, it will lead to large-scale minimization of energy losses and aid society in conserving vital natural resources for future generations.
2. Assessment of energy consumption
Agricultural scientists worldwide have established a direct linkage between energy use per hectare and crop yield.10,11 Mechanization in various operations ensured increased input energy with decreased operational costs. In most crops, hoeing and weeding have notably been perceived as the most labor-consuming operations along with harvesting, resulting in an enhanced cost of operation.10
On an agricultural farm, energy is exploited in different forms, such as manual, mechanical, chemical, and electrical energy. Energy sources have also been classified into different categories, as given in Table 1.9 Different types of unit operations carried out on any agricultural field are presented in Fig. 1.
Table 1 Types of energy and their sources
Types of energy |
Energy sources |
Ref. |
Direct energy |
Humans, animals, petrol, diesel, electricity, and irrigation water from canals |
9
|
Indirect energy |
Seeds, farmyard manure, fertilizers, chemicals, and machinery |
Renewable energy |
Humans, animals, seeds, farmyard manure, and canal water |
Non-renewable energy |
Petrol, diesel, electricity, chemicals, fertilizers, and machinery |
Commercial energy |
Petrol, diesel, electricity, chemicals, fertilizers, seeds, and machinery |
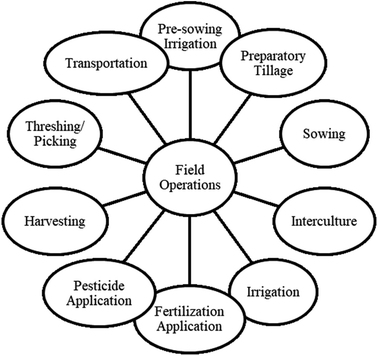 |
| Fig. 1 Different field operations in any crop production system. | |
The quantum of energy consumption in any crop production and distribution network needs to be significantly optimized to cater to the needs of the ever-proliferating global population and attain societal and fiscal objectives. An accurate energy source at a precise time and location in modern-day agriculture is required for the unified management of natural resources.
2.1 Cereal crops
Cereals are the primary source of energy in a vegetarian diet. Accessibility of energy from cereal crops depends on the type of grains consumed. Important cereal crops that are used in human food on a daily basis are paddy, wheat, sorghum, millet, barley, and maize. The global production of cereals in 2020–21 was 2796 million tonnes (MT) and consumption was 2657.1 million tonnes.12 Maize is an important crop as a staple food in many countries. Maize is widely cultivated throughout the world and has the highest production among all the cereal crops with 1125 million tonnes (MT), followed by rice (505 MT), wheat (775.8 MT), barley (159.54 MT), and sorghum (62.05 MT).12
2.2 Pulses
Developing countries use pulses as a staple food for rural and urban populations, whereas developed countries use them as major cash crops. The essential pulses consumed globally are beans (Phaseolus vulgaris L.), lentil (Lens culinaris L.), chickpea (Cicer arietinum L.) and soybean.13
2.3 Oilseed
Major oilseed crops grown in different geographical locations mainly include rapeseed, mustard, peanut, sunflower seed, cottonseed, palm kernel, canola, copra etc. Oilseed crops include both annual (generally called oilseeds) as well as perennial plants whose seeds, nuts, or fruits are either consumed directly as food or crushed to get oil (used by the food, oleochemical, biofuel and other industries). Some crops (fiber crops) contain oilseed and fiber and are harvested from the same plant, such as coconut, kapok fruit, cotton, linseed, and hempseed. Table 2 provides data on primary oilseed production in 2020–21.
Table 2 Major oilseed crop production in the worlda14
Rank |
Different oilseeds |
Production in year 2020–21 (million metric tons) |
Source: https://www.statista.com/statistics/267271/worldwide-oilseed-production-since-2008/.
|
1 |
Soybeans |
362.05 |
2 |
Rapeseed |
68.87 |
3 |
Peanuts |
47.79 |
4 |
Sunflower seed |
49.46 |
5 |
Cottonseed |
41.80 |
6 |
Palm kernel |
19.96 |
7 |
Copra |
5.75 |
2.4 Cash crops
Cash crops are grown explicitly for being sold to earn cash/money from the market. Cotton, sugarcane, tobacco, jute, and oilseeds are cash crops grown in India, while cassava, tea, coffee, etc., are cash crops grown in other parts of the world. India is the leading country in cotton production (6205 thousand metric tonnes), followed by China (5987 thousand metric tonnes) and the USA (4555 thousand metric tonnes) during 2018–19.15
2.5 Horticultural crops
The most popular horticultural crops are tomatoes, bananas, watermelon, and apples.12 Horticultural crops are significant components of a healthy diet. Fruits and vegetables have good nutritional and medicinal values due to their high proteinaceous and fiber content, including various vitamins and minerals. China was the first fruit and vegetable producing country with 588.26 million tonnes (MT), followed by India (132.03 MT) and the USA (29.99 MT) in the year 2019.
2.6 Indices of energy analysis
Energy use efficiency, energy productivity, energy ratio, and net energy gain are indices of energy analysis. These indices/parameters are determined from eqn (1)–(4).16,17 Energy coefficients are used to calculate the energy indicators (Table 3).
Table 3 Energy equivalents used in agricultural energy systems
Energy source |
Unit |
Energy equivalent (MJ per unit) |
Ref. |
Human labour
|
Man |
h |
1.96 |
18
|
Woman |
h |
1.75 |
18
|
Child |
h |
0.98 |
18
|
![[thin space (1/6-em)]](https://www.rsc.org/images/entities/char_2009.gif) |
Animal
|
Bullock |
Pair hour |
14.07 (body weight above 450 kg) |
19
|
10.10 (body weight 350–450 kg) |
![[thin space (1/6-em)]](https://www.rsc.org/images/entities/char_2009.gif) |
Fuel
|
Diesel |
L |
56.31 |
18
|
Agricultural machinery |
h |
62.7 |
18
|
Tractor |
h |
10.95 |
18
|
Farm yard manure |
kg |
0.3 |
20
|
![[thin space (1/6-em)]](https://www.rsc.org/images/entities/char_2009.gif) |
Fertilizer
|
Nitrogen |
kg |
60.6 |
21
|
Phosphorus |
kg |
11.1 |
22
|
Potash/Potassium |
kg |
6.70 |
22
|
![[thin space (1/6-em)]](https://www.rsc.org/images/entities/char_2009.gif) |
Chemical application
|
Fungicide |
kg |
181.9 |
23
|
Insecticide |
kg |
101.9 |
24
|
Seed |
kg |
14.7 |
|
Electricity |
kWh |
11.93 |
25
|
Water |
m3 |
1.02 |
23
|
2.6.1 Energy use efficiency.
Energy use efficiency or the energy ratio is determined as the output (yield) and input energy (direct and indirect energy) from the crop production system. After converting the yield into energy, the output is calculated by multiplying it with its energy equivalent.16,17 |  | (1) |
2.6.2 energy productivity.
Energy productivity (kg MJ−1) is defined as the total amount of harvested products divided by the total energy consumed by harvested products:16,17 |  | (2) |
2.6.3 Net energy gain.
Net energy gain (MJ ha−1) is defined as the difference between output and input energy.17 | Net energy gain (MJ ha−1) = Energy output (MJ ha−1) − Energy input (MJ ha−1) | (3) |
2.6.4 Specific energy.
Specific energy (MJ kg−1) is defined as the amount of energy spent to produce a unit of marketable product.16,17 |  | (4) |
2.7 Mechanization index
Mechanization is defined as the percentage of machinery energy to the sum of human, animal and machinery energies.26 |  | (5) |
3. Energy use patterns in different crop production systems
Indices of energy analysis such as energy use efficiency, energy productivity, energy ratio, and net energy gain were calculated from eqn (1)–(4). Fig. 2 shows the share of different crops' direct and indirect energy sources. Direct energy sources varied from 40–45%, while indirect energy sources were found to vary between 55–60%. The source-wise and operation-wise energy input–output of various crops in different countries are also discussed in this section.
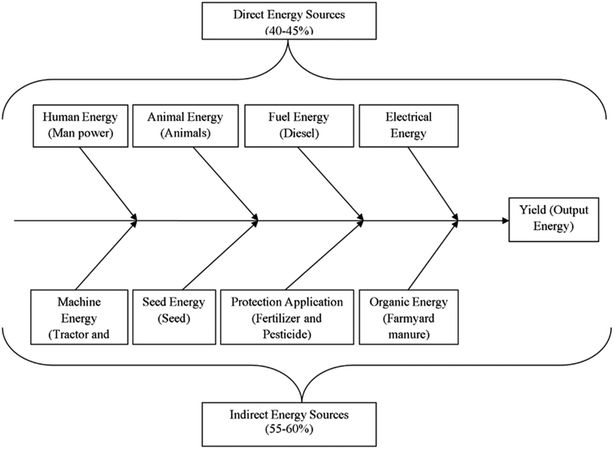 |
| Fig. 2 Share of direct and indirect energy sources. | |
3.1 Cereal crops
Energy use patterns in different cereal crop production systems are discussed in this section:
3.1.1 Wheat.
In Australia, the total energy consumption and output for wheat production were 10
900 MJ ha−1 and 100
346 MJ ha; hence the total energy output for wheat production was around ten times the total energy input with an overall energy efficiency of 9.21%. Chemical fertilizers contributed 47% of the total energy input consumed on wheat-growing farms.5 In some parts of India, wheat is grown under rainfed conditions (without fertilizers and irrigation). This cropping system was studied, and energy consumption was reported to be 2736.46 MJ ha−1 in mechanized and 1774.88 MJ ha−1 in traditional operations.10
Wheat production in different agro-climatic regions of central India consumed 14
345 MJ ha−1 of energy while producing 56
595 MJ ha−1. Out of the total energy input, the share of chemical fertilizers (31.1%) was more than that of diesel (20.5%) and electricity (24.2%). Indirect energy (50.4%) was slightly higher than direct energy (49.6%).17,18 In Turkey, the total energy input in wheat production was 25
876.29 MJ ha−1, leading to an enhanced energy output of 76
990.96 MJ ha−1 with an energy ratio of 2.97. The share of chemical fertilizers was 43.84% of the total energy input, followed by diesel fuel (13.07%) and electricity (11.10%). Other energy inputs were wheat seed (15.06%), irrigation water (13.93%), machinery (1.39%), transportation (0.92%), chemicals (0.48%) and human labour (0.20%). The energy ratio (EUE), energy productivity, specific energy, and net energy in wheat production were 2.97, 0.20 kg MJ−1, 4.94 MJ kg−1, and 51
114.67 MJ ha−1, respectively.27 The total energy consumed by different operations such as tillage, sowing, interculture, irrigation, harvesting, and threshing was 17
159.5 MJ ha−1 in wheat cultivation. The energy ratio, specific energy, productivity, and net energy were 2.21, 7.18 MJ kg−1, 0.14 kg MJ−1, and 20
746.5 MJ ha−1. Major energy-consuming operations in wheat were diesel (44.61%), chemical fertilizers (23.54%), irrigation water (10.58%), seeds (10.11%), machinery (9.86%), chemicals (0.92%) and labour (0.38%).28 The energy output was 84
427.33 MJ ha−1. For wheat production, energy use efficiency, specific energy, energy productivity, and net energy were 2.36, 8.96 MJ kg−1, 0.112 kg MJ−1, and 48
690.20 MJ ha−1, respectively.6 Irrigation water as an energy input was 14.5% greater in wheat under the cotton-wheat system (4093 ± 133 MJ ha−1) than that under the rice-wheat (3576 ± 124 MJ ha−1) cropping system in north-western India. Other energy sources did not play any significant role in energy input. In India, almost 65% of the total surveyed farms were energy-inefficient. Nitrogen fertilizers and excess electricity used for lifting underground water contributed heavily to energy input. A total of 12% of energy input could be saved by optimizing the use of freely available groundwater and N-fertilizers.29
The high energy input in wheat production in Portugal could be substantially reduced with efficient use of resources by adopting no-tillage, reduced use of P2O5, and increased use of irrigation. Among these three options, better results could be expected with no-tillage with reduced energy consumption (45%), reduced GHG emissions (30%), and lowered costs (8%) per ton of wheat produced. The increment in profit was about 24% for no-tillage, 3% for reduced P2O5, and 4% for irrigation.30
3.1.2 Paddy.
The energy requirements and economic analysis of paddy production were carried out in Australia. In paddy cultivation, energy consumption and production were 24
116.40 MJ ha−1 and 16
1586 MJ ha−1, with an energy ratio/energy use efficiency of 6.70. Chemical fertilizers consumed 43% of the total energy inputs in paddy growing farms.5 The energy use pattern was studied under different tillage operations in Bangladesh at the Bangladesh Rice Research Institute (BRRI). Paddy grown using power tillers consumed (15
751 MJ ha−1) and produced (147
842 MJ ha−1) more energy than that grown using bullock farming systems (13
781 MJ ha−1 and 127
632 MJ ha−1).7 The total energy input and output (grain + straw) ranged between 19
000 and 26
700 MJ ha−1 and 121
000–1
25
000 MJ ha−1, respectively.31 The effect of irrigation on double-rice cropping patterns was studied in low land areas of the Philippines. The energy input was compared among farmers’ fields, triple-rice systems & diversified rice-based systems and it ranged from 12
400–13
100 MJ ha−1, 12
800–22
800 MJ ha−1 and 15
300–30
900 MJ ha−1, respectively. Out of the total Agricultural Energy Index (AEI), nitrogenous fertilizers and commercial fuel accounted for greater than 60% and 75% in farmers’ as well as experimental fields.8 Energy-exergy analysis conducted in different paddy growing areas of Iran viz. Guilan, Golestan, and Mazandaran showed significant differences in total energy input with agronomical practices such as crop rotation, transplantation time, and land preparation. The total energy input in Golestan province was the highest (64
158.78 MJ ha−1) among the three provinces considered for the study, and that was due to higher diesel consumption (46.44%).24 Energy inputs and outputs were determined in rice crops in Malaysia and yield, total energy input, energy output/input ratio, and energy intensity were 7625 kg ha−1, 16
440 MJ ha−1, 7.76, and 2.16 MJ kg−1. China, India, and Thailand consumed more energy to grow paddy than Malaysia. Major energy consumption was due to conventional energy sources [chemical manure (60%), diesel (17%), pesticides (4%), and machinery (3%)]. The share of renewable resources such as seed, human labor, and organic fertilizers was 15, 0.25, and 0.22%. The B
:
C ratio was 1.37.32 The transplanting method was studied in Raipur, Chhattisgarh (India), on the energy requirements of the rice production system. The total energy consumed by different operations was 13
615.94 MJ ha−1. Fertilizers consumed almost 60% of the total energy used (i.e. 7706.75 MJ ha−1), while intercultural operations consumed the minimum energy. The cost of cultivation was 500 USD/ha and the cost/benefit ratio was 1.96.33 Consumption of energy in paddy cultivation by small, medium and large farmers ranged between 32
400–36
700 MJ ha−1. 60% energy was contributed by direct and 40% energy was contributed by indirect sources in gross energy consumption. However, conventional and non-conventional energy sources contributed 92% and 8% to the total energy input.34 Higher energy use efficiency (by 19%) and higher energy productivity (by 24.8%) were reported in the case of farmers' practices (FPs) as compared to simplified and reduced-input practices (SRIPs). Specific energy reduced from 3.76 MJ kg−1 (FP) to 3.01 MJ kg−1 (SRIPs), indicating a significant enhancement in EUE and reduction in energy utilization with the adoption of more innovative agronomic practices such as SRIPs.9 Varied rice growing approaches were studied in the Indo-Gangetic Plains of India. Since the direct sowing method was found to be better in terms of energy efficiency, it should be suggested to farmers to save water and energy and reduce global warming potential. The global warming potential from rice cultivation has been reported as 467, almost 169% more than from other major cereal crops, thus, becoming a major cause of concern.35
In the western coastal regions of India, different sources contributed 53.64% indirect and 46.16% direct energy during rice production. Diesel (82%) and fertilizers (49%) had the maximum share among direct and indirect energy inputs. There was a substantial difference in the cost/input (19.99 Rs per MJ) and cost/output (0.83 Rs per MJ). The share of conventional energy (72.86%) was about 2.6 times that of non-conventional (27.14%). Irrigation (46%) had a significant share in alternate energy, followed by human resources (30%). The output was contributed almost equally by seeds (52%) and straw (48%). The net energy gain, energy use efficiency, energy productivity, specific energy, and water productivity were 61
738.52 MJ ha−1, 2.22, 1.16 kg MJ−1, 0.86 MJ kg−1, and 9.33 kg m−3. Energy utilization for rice production by small (32
417.7 MJ ha−1), medium (36
471.61 MJ ha−1), and large (36
742.85 MJ ha−1) farmers showed that electricity had the highest share in all operations and farm sizes. Direct energy (60%) used was more than indirect energy (40%). Renewable energy (8%) had a bare minimum share in comparison to non-renewable energy sources (92%).34
3.1.3 Maize.
When maize was produced in southern India, energy consumption and productivity were 16
701.61 MJ ha−1 and 5766.50 kg ha−1. Chemical fertilizers (55%) and organic manure (FYM, 17.48%) consumed the highest input energy, followed by diesel (14.33%). Indirect (77.37%) and non-renewable (72.42%) energy sources contributed more than 70% to the input energy pool. Simultaneously, direct (22.63%) and renewable (27.58%) energy sources shared more than 20% of input energy. Energy production from straw was 55
447.08 MJ ha−1, with a productivity of 4435.76 kg ha−1.36
Corn silage cultivation was carried out on the Mediterranean coast of Turkey using five tillage treatments (minimum, ridge, band, conventional and no-tillage). Minimum tillage was better than others in terms of yield (49
743.3 kg ha−1), energy ratio (8.78), productivity (2.12 kg MJ−1), and profit (7.78). The total energy input in non-tillage and ridge tillage was 21
505.44 MJ ha−1 and 23
779.95 MJ ha−1.37
3.1.4 Barley.
Barley was a low energy (2175 MJ ha−1) consuming crop when its energy use and economic analysis were studied in Australia. The total energy output and energy ratio for barley production were 64
314 MJ ha−1 and 8.21. Chemical fertilizers accounted for 29% of the overall energy input.5
3.1.5 Millets.
Energy utilization patterns were evaluated in five different categories of farmers (based on their farm sizes) in millet production in Nigeria. The total energy input varied between 1700–6000 MJ ha−1, while output varied between 2300–13
100 MJ ha−1, indicating the effect of practices adopted by different farmers on energy use.38 To examine the energy-exergy analysis, five different tillage treatments were tried in rainfed pearl millet cultivation at Hisar, Haryana (India). “No-tillage” treatment showed the lowest energy output/input ratio due to the lowest grain yield, while “low tillage with two intercultural operations” showed the highest ratios of both output–input and B–C, indicating the necessity of this treatment for moisture conservation and weed management, leading to optimum grain yields. This treatment also resulted in an energy output increase of 39.6 MJ (maximum) per unit of energy input added. It was nearly followed by another treatment of low tillage + one interculture + Atrazine spray. An average energy input of 7000 MJ ha−1 was observed for pearl millet production.39 Pearl millet production was studied for three categories of farmers in Nigeria. The energy output (3156 MJ ha−1) of small farmers was less than their input energy (3945 MJ ha−1). There was a difference of around 20% in the input energy of medium (4845 MJ ha−1) and large farmers (6090 MJ ha−1). However, their output energy was observed to have no significant difference (12
597 MJ ha−1 and 12
789 MJ ha−1). The primary direct energy sources were animal draft, manual labor, and fuel energy, while farmyard manure, pesticides, seed, and machinery were the primary indirect energy sources.40 Energy consumption and production were 4785.52 MJ ha−1 and 29
400.00 MJ ha−1 analyzed at the university field in Haryana (India). The maximum share in the total energy input was fertilizers (56%), followed by fossil fuel (29%) and human resources (12%). The energy use efficiency was 6.12.41
3.1.6 Sorghum.
The data of different energy-consuming operations for sorghum cultivation were obtained from the CRSF farm, Maharashtra (India). In the mechanized and traditional methods, energy inputs were 3555.52 MJ ha−1 and 2675.28 MJ ha−1. In a mechanized method, seedbed preparation (1178.7 MJ ha−1) was the most energy-intensive operation, followed by interculture (1114 MJ ha−1), residue management (552.8 MJ ha−1), harvesting + threshing (451.84 MJ ha−1) and sowing (258.18 MJ ha−1). While in the case of the traditional method, seedbed preparation (1040.48 MJ ha−1) consumed the highest energy followed by interculture (787.44 MJ ha−1), harvesting + threshing (451.84 MJ ha−1), residue management (251.2 MJ ha−1) and sowing (144.32 MJ ha−1).10
3.2 Leguminous/pulse crops
Energy use patterns in different pulse crop production systems are discussed here:
3.2.1 Chickpea.
In Iran, the energy input and output for chickpea production were 4826 MJ ha−1 and 14
663 MJ ha−1. The maximum share of energy consumed is by diesel fuel (72%), followed by seed (13%) and machinery (8%), respectively. The energy ratio and specific energy were 3.04 and 7.55 MJ kg−1.42
3.2.2 Soybean.
An Indian study showed that traditional soybean crop farming used more operational energy inputs (615.06 MJ ha−1) than the mechanized system (498.39 MJ ha−1). Also, the energy cost of traditional farming was four times higher than that of mechanized farming.43 The total energy output for soybean production was more in mechanized operation (3417.28 MJ ha−1) than in the traditional one (2655.36 MJ ha−1).10 Total input (18
026.50 MJ ha−1) and output energy (71
228.86 MJ ha−1) were quite high when economic and sensitivity analysis of soybean production was performed at Kordkuy, Iran. The significant share of petroleum fuel was 66.67%. Fertilizers and irrigation contributed 14.32% and 6.18% while energy use efficiency was 4.62.44
3.2.3 Green gram.
The energy input in mechanized farming (3130.72 MJ ha−1) was higher than that in the traditional method (2680.78 MJ ha−1). However, the operational cost was lower in the mechanized method (Rs. 5147 per ha) as compared to the traditional method (Rs. 8407.5 per ha).10
3.2.4 Alfalfa.
In alfalfa agro ecosystems, the total energy intake and output were 49
689.59 and 240
072.7 MJ ha−1, respectively. Diesel fuels (43.1 percent), electricity (24.36 percent), and nitrogen fertilizers (12.2%) all had the greatest percentage of input energy (12.2 percent). Energy consumption efficiency, energy productivity, specific energy, and net energy were likewise found to be 4.83, 0.27, 3.68, and 190
383.11 MJ ha−1, respectively. The total energy input was determined to be 10.24 percent renewable and 89.76 percent non-renewable.45
3.3 Oilseed crops
Energy use patterns in different oilseeds crop production systems are discussed in this section:
3.3.1 Groundnut.
During groundnut cultivation studied in Iran, the total output energy (79
252.02 MJ ha−1) was almost four times higher than the input energy (20
164.36 MJ ha−1). Diesel fuel (43.51%) played a significant role in energy consumption, followed by chemical fertilizers (29.11%).46
3.3.2 Sunflower.
In Iran, total energy consumption and output for sunflower production were 22
945 MJ ha−1 and 49
791 MJ ha−1. Direct and indirect energy contributions in sunflower production were found to be 57.94% and 42.06% of the total energy input. The energy ratio, energy productivity, and specific energy were 2.17, 0.079 kg MJ−1 and 12.52 MJ kg−1, respectively.47
3.3.3 Canola.
The energy input found in canola production was 30
889 MJ ha−1 studied in Iran. The total energy output, energy ratio, energy productivity, and a specific energy for canola production were 89
578 MJ ha−1, 2.9, 0.12 kg MJ−1, and 8.27 MJ kg−1. Fertilization was the highest energy-consuming operation, followed by electricity and diesel energy.47
3.3.4 Rapeseed.
In rapeseed production it was discovered that the reduced tillage approach maximized energy efficiency in rapeseed farming. In comparison to other inputs in all applications, the energy input for fertilizers was 7242.50 MJ ha−1. For each application, rapeseed cultivation's energy output/input ratios were determined to be 7.30, 7.55, and 7.24, respectively.28
3.3.5 Sesame.
The energy input was found to be 10
020 in sesame production in the area of the province of Antalya, Turkey. The total output, net energy, input–output ratio and energy productivity for sesame production were obtained as 12
000 MJ ha−1, 1980 MJ ha−1, 1.8 and 1.76 kg MJ−1.48
3.3.6 Peanut.
In Iran, total energy utilization, energy output and average yield of peanut production were obtained as 19
248.0, 8720.68 MJ ha−1 and 3488.39 kg h−1, respectively. Chemical fertilization and diesel fuel were the highest energy-consuming operations. The energy input–output ratio was calculated to be 4.53.49
3.4 Fiber/cash crops
Energy use patterns in different fiber/cash crop production systems are given as follows:
3.4.1 Cotton.
The energy input in traditional farming (696.76 MJ ha−1) was higher than that in mechanized farming (507.67 MJ ha−1) in India.43 In comparison, the energy cost was four times higher in the traditional system than in the mechanized one. The total energy input was 4336.41 MJ ha−1 in mechanized and 4284.28 MJ ha−1 in the traditional system. Though there was an increase in the energy input due to mechanization, the cost of operation decreased.10 The energy input and output were 17
747.06 MJ ha−1 and 67
260 MJ ha−1 in commercial cotton cultivation in India. Energy consumption included fertilizers (5350.74 MJ ha−1), irrigation (4252.50 MJ ha−1), machinery (3814.17 MJ ha−1), diesel fuel (2947.83 MJ ha−1), humans (1128.12 MJ ha−1) and seeds (253.70 MJ ha−1). Energy productivity, specific energy, energy ratio, and net energy were 0.32 kg MJ−1, 3.11 MJ kg−1, 3.79 and 49
512.94 MJ ha−1, respectively.50,59 In Iran, energy expenditure for cotton cultivation was 31
237 MJ ha−1, in which fertilizers, diesel, and machinery had the major share. Greenhouse gas generation (GHG) due to the machine, fossil fuel, and water application was 1195 kg CO2eq. per ha. Energy productivity was 0.11 kg MJ−1 and energy use efficiency was 1.85. The contribution of direct energy (40%) was less than that of indirect energy (60%). As observed in the case of other crops, renewable energy (29%) had a lower share than non-renewable energy sources (71%).51
3.4.2 Cassava.
In cassava production, the energy input and output were 8571.26 MJ ha−1 and 55
776 MJ ha−1, whereas productivity was 9960.00 kg ha−1. Indirect and direct sources consumed energy at 78.67% and 21.33%, respectively, during cassava production. The highest energy input was by fertilizers (64.0%), followed by diesel fuel (19.50%). Energy productivity and energy ratio were 1.18 MJ kg−1 and 7.1, respectively.52
3.4.4 Sugarbeet.
In sugar beet production, the energy input and output were determined to be 34
201.75 and 285
600 MJ ha−1, respectively. Chemical fertiliser energy accounts for 41.97 percent, diesel fuel energy for 21.16 percent, irrigation for 11.97 percent, electricity for 11.96 percent, human labour energy for 6.47 percent, equipment energy for 5.53 percent, seed energy for 0.61 percent, and chemical energy for 0.33 percent. The energy input–output ratio and net energy were found to be 8.35 and 251
398.25 MJ ha−1, respectively.55
3.5 Nut crops
Energy use patterns in different nut crops such as walnuts, hazelnuts and pistachios are discussed here. The energy consumption pattern of walnut crops was studied in Turkey. Energy consumption was found to be 23
992.54 MJ ha−1 and the output was 14
679.52 MJ ha−1. The highest energy was utilized in chemical application (74.40%) for walnut production, while the lowest was found in farmyard manure (0.18%). The input–output ratio of walnut production was found to be 0.61.56 In the cases of hazelnuts and pistachios, the total energy consumption was 2862.62 MJ ha−1 and 54305 MJ ha−1 while energy output was 11
255.00 MJ ha−1 and 61
827.0 MJ ha−1, respectively.57,58 The highest energy utilizing operation of a pistachio orchard was electricity, whereas it was diesel fuel (33.84%) for hazelnut production.
3.6 Horticultural crops
Energy use patterns in different horticultural crops are discussed here:
3.6.1 Fruits.
Energy use patterns in apples and grapes grown in two different provinces of Iran viz. Tehran and Hamadan were studied. The energy utilized in growing apples was 42
819.25 MJ ha−1. Diesel fuel (21.88%) was the most energy-consuming in different operations, followed by compost (17.66%) and electricity (13.09%). Net energy, specific energy, and energy ratio were 7038.18 MJ ha−1, 2.06 MJ kg−1, and 1.16.59 The total energy input (68
928 MJ ha−1) and output (148
380 MJ ha−1) for grape production were comparatively higher than those for apple production. Grape production also resulted in a higher energy ratio (2.27), specific energy (3.76 MJ kg−1), and net energy (79
452 MJ ha−1). In comparison, energy productivity (0.28 kg MJ−1) was slightly lower than for apple production (0.49 kg MJ−1). The contribution of indirect energy was 75%, while that of direct energy was 25%.60
Energy utilization in lemon production was the maximum among the three citrus fruits {orange (60
949.69 MJ ha−1), lemon (62
977.87 MJ ha−1), and mandarin (48, 838.17 MJ ha−1)} in Antalya province, Turkey. Nitrogenous fertilizers (49.68%) had a higher total energy input segment, followed by diesel (30.79%). The energy ratio of lemon (1.06) was slightly lower than that of orange (1.25) and mandarin (1.17). Only 3.74% of the energy sources used was renewable, compared to 95.90% of the non-renewable ones. Orange production had a maximum benefit-cost ratio of 2.37, followed by lemon, indicating it as the most remunerative option for growers among the three citrus fruits.61 In the case of sweet cherry, direct and indirect energy shares were 34.48% and 54.91% of the total input energy. The maximum energy-consuming operations were found in chemical applications (45.35%), especially nitrogen with 38.05%.62 Banana was the fruits' highest direct energy consuming crop (51
560.1 MJ ha−1). The total input and output of banana production were 51
560.1 and 98
024.88 MJ ha−1, respectively. Electricity has the highest share (27.55%) of the total energy input.63
3.6.2 Vegetables.
The total energy input for greenhouse cucumber production in Iran was 148
836.76 MJ ha−1. Diesel fuel (41.94%) and chemical fertilizers (19.69%) were the highest energy inputs. Fertilizers (39%), fossil fuel (21%), seeds (14.9%), irrigation (7.5%), and compost (6.4%) consumed enormous energy during potato production.64 Average yield and energy consumption were 28
613.7 kg ha−1 and 92
296.3 MJ ha−1. However, the energy use efficiency, energy productivity, and specific energy were 1.1, 0.3 kg MJ−1, and 3.2 MJ kg−1.60,74 The average yield for glasshouse tomato production was 25
025.4 kg ha−1 in the fall and 22
392.9 kg ha−1 in summer. Overall energy consumption was higher in the fall (81
362.2 MJ ha−1) than in summer (63
023.2 MJ ha−1).16,20 Total input energy and yield were 13
911 MJ ha−1 and 21
290 kg ha−1 for eggplant production in Iran. Energy use efficiency, energy productivity, net energy, and energy intensity were 9.03, 1.53 kg MJ−1, 111
701.33 MJ ha−1, and 5.19 MJ per $.65 In Indonesia, gross energy consumption in tomato cultivation in a greenhouse (GH) (47
620 MJ ha−1) was less than that in an open field (OF) (49
010 MJ ha−1). The total input energy requirements of GH chili {medium (41
550 MJ ha−1) and highland (58
840 MJ ha−1)} and lettuce (24
540 MJ ha−1) were slightly more than those of chili grown in open farms {medium (41
040 MJ ha−1) and highland (57
940 MJ ha−1)} and lettuce (23
870 MJ ha−1). The energy use efficiency ratio was greater in GH (0.45–0.85) than in open farm vegetable cultivation for different crops such as chili and tomato (0.17–0.52). However, this ratio was twice as high in OF lettuce as in the greenhouse one.66
3.7 Tobacco
The energy input for tobacco production was determined to be 25
523.32 MJ ha, while the energy output was calculated to be 840 MJ ha. In tobacco production, energy consumption efficiency, productivity, specific energy, and net energy were determined to be 0.03, 0.04 kg MJ, 24.31 MJ kg, and −24683.32 MJ ha, respectively. Direct and indirect energy shares were found to be 47.16% and 52.84%, respectively.
3.8 Multi-crop production systems
Energy usage analysis of major cereal cropping systems worldwide, i.e., rice-wheat, has been studied and compared with other multi-cropping systems under varied climatic conditions. One such study was carried out in India, where the energy requirements of six cropping systems viz. rice–pea–wheat–greengram, maize–pea–wheat, rice–wheat, rice–mustard–greengram, soybean–wheat and pigeonpea–wheat were compared. Energy usage in rice–pea–wheat–greengram (65
052 MJ ha−1) was more than that in the traditional rice-wheat (50
264 MJ ha−1) system followed by maize-pea–wheat (46
031 MJ ha−1), rice–mustard–greengram (43
614 MJ ha−1) and soybean–wheat (30
859 MJ ha−1). The pigeon pea–wheat system (29
015 MJ ha−1) had minimum energy utilization. In all the cropping systems, chemical fertilizers played a major role in energy consumption followed by water application, machinery, chemical application, human resources, and seed.66 The energy input and output in rice-wheat cropping patterns were found to be 59
152 MJ ha−1 and 164
784 MJ ha−1 whereas a 61
913 MJ ha−1 energy input and 163
272 MJ ha−1 output were found in the rice–maize cropping system.1 The energy ratio of rice–wheat and rice–maize cropping systems was 3.06 and 2.98, respectively.
Energy-exergy analysis of rice–wheat with other cropping systems was compared on flat bed (FB) and raised bed (RB) systems. The rice–potato–wheat cropping system emerged as the highest consumer {75
697 (RB)-77
601 MJ ha−1 (FB)} as well as producer {218
065 (RB)-2
22
836 MJ ha−1 (FB)} of energy. Rice–wheat–sesbania {47
830 (RB)-48
770 MJ ha−1 (FB)} closely followed rice-wheat–greengram {47
482 (RB)-48
414 MJ ha−1 (FB)} in terms of input energy, though, there was approximately a 78% difference in their output energy. The rice–wheat system recorded the lowest output energy {151
862 (RB)-1
56
085 MJ ha−1 (FB)}. Input energy in the flatbed was higher than that in the raised bed.67 Seven year-long studies suggested that agricultural conservation practices were adopted for conserving natural resources, such as zero tillage (ZT) and permanent bed (PB), and compared to traditional tillage (CT) with various maize cropping systems (maize-wheat-mungbean) they emerged as a sustainable option to achieve better biomass productivity, bio-energy yield and energy-use efficiency in India and South Asia. Both ZT and PB fields utilized less energy for water application (16.8–22.9%) and land preparation (49.7–51.5%) and resulted in notably elevated net energy (14.8–18.9%) and energy production (10.6–14.5%). The energy use-efficiency (13.4–17.1%), biomass productivity (9.9–14.1%), and bio-energy yield (17.3–19.8%) were also markedly higher in CA. The maize-wheat–mungbean (MWMb) cropping system was recommended as a better option than a conventional rice-based cropping pattern in the Indo-Gangetic Plains, India. This cropping system, along with agricultural conservation practices with better management of nutrients, would help reduce the large-scale menace of straw burning, energy, and nutritional scarcity and help to reclaim the ever-dwindling biomass productivity and water tables.68 Energy assessment was conducted on maize–wheat–greengram cultivated conventionally and using conservation practices. Zero tillage (89
170 MJ ha−1) consumed 8% less energy than conventional on a flatbed, while on a raised bed, it conserved 91% energy in field preparation and 38% in irrigation. Zero tillage resulted in greater energy use efficiency and productivity, leading to an overall higher output of 387
235 MJ ha−1.69
Energy consumption for maize, rainfed rice, irrigated rice, cassava and wet-season soybean varied from 9790–12
790, 10
090–13
110, 1790–18
490, 4950–9130 and 5210–10
030 MJ ha−1. The dry-season soybean was the minimum, ranging between 5310–7860 MJ ha−1. Material and physical energy inputs accounted for 62% and 38%. Fertilizer energy consumed the highest energy, followed by seeds, pesticides, and herbicides. The physical energy input was more than 97.8% of mechanical power sources.
The energy ratio for different crops varied between 0.76–29.4. Dry and wet-season soybeans had lower energy use efficiency (2.0–3.7), followed by rainfed and irrigated rice. Sugarcane had the maximum efficiency of energy use (9.3–10.1), followed by maize and cassava.54 Conservation agriculture (CA) practices such as zero-till (ZT) with residue management (RM) improved the grain yield of pearl millet (22.3%) and mustard (24.5%) compared to conventional till (CT) without residues, which ultimately resulted in a better net revenue of 1270 US$/ha. Crop residue mulching consumed remarkably higher energy (72.3–87.1%) out of the total consumption. The average energy input, output, and yield of corn in India and Indonesia were found to be 21
270 MJ ha−1, 25
298 MJ ha−1 and 1721 kg ha−1, while in the case of the USA they were 34
448 MJ ha−1, 1
38
180 MJ ha−1 and 9400 kg ha−1.70
Analysis of energy intensiveness and energy use efficiency (energy ratio) of agricultural practices in Bangladesh (1990–2005) showed that total energy production increased from 69
873 to 82
080 MJ ha−1 (14.8% increase) with enhanced energy consumption from 17
940 to 27
100 MJ ha−1 (33% increase). The simultaneous enhancement in energy intensity was 45.67–54.47 MJ per US$. Energy use efficiency for various crops declined (3.97–3.03) with an increased energy input, indicating a faster increase in energy consumption than production. The mechanization index improved from 64% to 78%.71
3.9 Energy consumption and production of major cereal crops
Fig. 3(a) and (b) depict Turkey (30
806 MJ ha−1) as the intensive user of input energy in wheat as compared to the other two countries {India (14
345 MJ ha−1) and Australia (10
900 MJ ha−1)}. However, Australia with 100
346 MJ ha−1 produced a markedly 19.5% extra output energy as compared to Turkey (80
708 MJ ha−1) and India (56
595 MJ ha−1).5,6,17,27,28
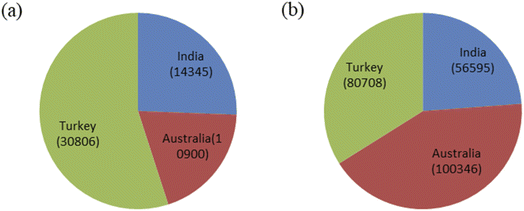 |
| Fig. 3 (a) and (b): Country-wise energy input and output (MJ ha−1) in wheat production. | |
Fig. 4(a) and (b) indicate that, though energy utilization for rice cultivation in Iran (60
524 MJ ha−1)24 was 41% higher than that in India (35
210 MJ ha−1), its energy production was 73% lower, showing the inefficient use of energy for rice production in Iran.32 Though India (35
210 MJ ha−1) and China (34
544 MJ ha−1) had a 1.8% difference in their energy inputs for rice production. Still, there was a 39% difference in their energy outputs depicting the effect of geographical location as well as excessive use of energy by China.9 Similarly, the Philippines (17
755 MJ ha−1) and Malaysia (16
440 MJ ha−1) differed by 7.4% in their energy inputs. In comparison, the energy output of Malaysia (127
643 MJ ha−1)32 was almost 45% more than that of the Philippines (70
922 MJ ha−1).8 Bangladesh was the lowest consumer of energy with 15
751 MJ ha−17 but emerged as a more prominent energy producer than Malaysia (127
643 MJ ha−1)17 and the Philippines (70
922 MJ ha−1). The energy output of Bangladesh (56
595 MJ ha−1) was 13.7% and 52% higher energy than that of Malaysia (127
643 MJ ha−1)32 and the Philippines (70, 922 MJ ha−1).8 Iran emerged as the highest energy user among all the countries and Bangladesh has the lowest consumption of energy for rice production (Fig. 4(a) and (b)).
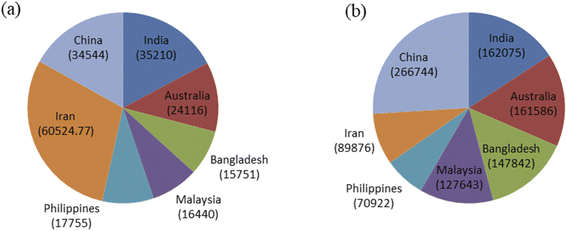 |
| Fig. 4 (a) and (b): Country-wise energy input and output (MJ ha−1) in rice production. | |
3.10 Global average variation in energy input-output and energy indices of crop production
Fig. 5(a) and 5(b) show the average consumption and production of energy in different crops across the globe. Country-wise data regarding energy input–output, net energy, energy productivity, specific energy and energy ratio of cereal, pulses, oilseed and cash crops, are given in Table 4. Energy use was the highest in sugarcane (148
020 MJ ha−1)18 production in cereal crops followed by rice (29
191 MJ ha−1),8,24,32 corn (23
779 MJ ha−1),37 groundnut (20
164 MJ ha−1),46 soybean (18
026 MJ ha−1),10,43,44 cotton (17
747 MJ ha−1),10,43,50 barley (7830 MJ ha−1),7 millet (5081 MJ ha−1) 38–40, sorghum (3555 MJ ha−1) and greengram (3130 MJ ha−1),10 respectively. The energy output was two times more than energy input in all crops except sugarcane, which was lower than energy consumption.
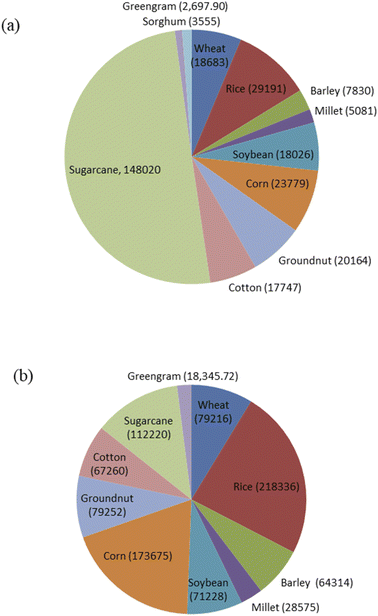 |
| Fig. 5 (a) and (b): Global average variation in input and output energy (MJ ha−1) of different crops. | |
Table 4 Global variation in energy use patterns of different crops
Crops |
Country |
Input energy (MJ ha−1) |
Output energy (MJ ha−1) |
Net energy (MJ ha−1) |
Energy ratio |
Energy productivity (kg MJ−1) |
Specific energy (MJ kg−1) |
Ref. |
Wheat |
Australia |
10 900.80 |
10 0346.40 |
89 445.20 |
9.21 |
1.61 |
0.62 |
5
|
India |
52 400.00 |
259 000.00 |
206 000 |
5.00 |
0.16 |
6.25 |
29
|
14 345.00 |
56 595.00 |
42 210 |
3.95 |
0.27 |
3.7 |
17
|
Turkey |
25 876.29 |
76 990.96 |
51 114.06 |
2.97 |
0.20 |
4.94 |
27
|
17 159.50 |
37 906.00 |
20 746.5 |
2.21 |
0.14 |
7.18 |
28
|
35 737.13 |
84 427.33 |
48 690.2 |
2.36 |
0.112 |
8.96 |
6
|
Rice |
Australia |
24 116.40 |
16 1586.00 |
137 469.6 |
6.70 |
1.48 |
0.68 |
5
|
Bangladesh |
15 751.00 (tiller farming) |
147 842.00 |
132 127 |
9.38 |
0.30 |
3.32 |
7
|
13 781.00 (bullock farming) |
127 632.00 |
113 851 |
9.26 |
0.29 |
3.34 |
15 751.00 |
71 589 |
55 838 |
4.54 |
0.30 |
3.23 |
32
|
Malaysia |
16 440.00 |
127 643.00 |
111 203 |
7.76 |
0.46 |
2.16 |
32
|
Philippines |
12 800–22 800 (triple-rice system) |
71 600–10 4400 |
58 800–81 600 |
4.94 |
0.15 |
6.46 |
8
|
15 300–30 900 (diversified rice system) |
46 200–72 600 |
30 900–41 700 |
2.57 |
0.19 |
5.32 |
Iran |
64 158.78 (Golestan) |
89 491.00 |
25 332.22 |
1.39 |
0.064 |
15.62 |
24
|
62 383.99 (Mazandaran) |
88 179.00 |
25 795.01 |
1.41 |
0.059 |
16.94 |
55 031.53 (Guilan) |
91 958.00 |
36 926.47 |
1.67 |
0.070 |
14.28 |
Thailand |
20 471 |
67 291.4 |
46 820.40 |
3.28 |
0.22 |
4.44 |
32
|
India |
13 616.00 |
174 953.00 |
161 337 |
2.34 |
6.27 |
12.84 |
33
|
9257.00 |
39 675.30 |
30 418.3 |
4.28 |
0.29 |
3.43 |
32
|
32 417.17 (small farmers) |
119 040.60 |
140 835.20 |
5.36 |
0.22 |
4.55 |
34
|
36 471.61 (medium farmers) |
167 290.00 |
130 994.80 |
4.61 |
0.19 |
5.25 |
36 742.85 (large farmers) |
145 813.00 |
109 282.90 |
3.99 |
0.16 |
6.33 |
35 605.00 (puddled transplanted rice) |
156 662.00 |
120 171.00 |
4.40 |
0.20 |
6.40 |
72
|
7832.00 (direct seeded rice) |
57 173.60 |
45 403.00 |
7.30 |
0.30 |
4.10 |
China |
31 703.00 |
119 040.00 |
87 337.00 |
3.75 |
0.25 |
3.91 |
32
|
China |
34 545.00 (FP) |
26 6745.0 (FP) |
232 200 |
7.74 |
0.27 |
3.76 |
9
|
25 441.5 (SRIP) |
234 393.0 (SRIP) |
208 951.5 |
|
0.33 |
3.01 |
Barley |
Australia |
7830.00 |
64 314.00 |
56 484.00 |
8.21 |
1.29 |
0.78 |
5
|
Millet |
Nigeria |
3283.00 |
6943.00 |
3660 |
2.02 |
6.67 |
0.15 |
38
|
|
4960.00 |
9514.00 |
4554.00 |
1.84 |
0.28 |
3.57 |
40
|
India |
7000.00 |
69 269.80 |
22 400.00 |
9.63 |
0.26 |
3.72 |
39
|
|
4785.52 |
29 400.00 |
24 614.48 |
6.14 |
0.41 |
2.39 |
41
|
Sorghum |
Ukraine |
11 256.00 |
125 860.00 |
114 604.00 |
11.18 |
0.33 |
3.02 |
73
|
Corn |
Turkey |
23 779.96 |
17 3675.04 |
149 895.08 |
7.30 |
2.12 |
0.89 |
38
|
Corn |
Iran |
68 928.00 |
148 380.00 |
79 452 |
2.27 |
0.28 |
3.76 |
74
|
Chickpea |
Iran |
4826 |
14 663 |
9837.00 |
3.04 |
0.13 |
7.55 |
42
|
Iran |
18 026.50 |
71 228.86 |
52 202.36 |
4.62 |
0.16 |
9.86 |
44
|
Green gram |
India |
2697.90 |
18 345.72 |
15 647.82 |
6.80 |
0.26 |
3.8 |
75
|
Alfalfa |
Iran |
49 689.59 |
240 072.7 |
190 383.11 |
4.83 |
0.27 |
3.68 |
45
|
Groundnut |
Iran |
20 164.36 |
79 252.02 |
59 087.66 |
3.50 |
0.21 |
4.74 |
46
|
Sunflower |
Iran |
22 945 |
49 791 |
26 846.00 |
2.17 |
0.08 |
12.52 |
47
|
Sesame |
Turkey |
10 020.0 |
12 000 |
1980 |
1.80 |
0.56 |
1.78 |
48
|
Soybean |
Iran |
27 376.5 |
78 493.1 |
51 116.6 |
3.10 |
0.11 |
9.30 |
25
|
Peanuts |
Iran |
19 248.0 |
87 209.7 |
67 961.6 |
4.50 |
0.18 |
5.52 |
49
|
Rapeseed |
Turkey |
10 485.0 |
76 570.3 |
66 085.2 |
7.30 |
0.20 |
4.89 |
28
|
Coconut |
Turkey |
17 553.00 |
515 208 |
4 97 655.0 |
29.4 |
1.17 |
0.85 |
2
|
Canola |
Iran |
30 889 |
89 578 |
58 689.00 |
2.90 |
0.12 |
8.27 |
47
|
Cotton |
India |
18 550 |
16 838 |
−1712 |
0.90 |
0.90 |
12.9 |
76
|
Turkey |
17 747.06 |
67 260.00 |
49 512.94 |
3.79 |
0.32 |
3.11 |
50
|
Cassava |
Nigeria |
8571.26 |
55 776 |
47 204.74 |
7.10 |
1.18 |
0.84 |
52
|
Sugarcane |
Iran |
148 020 |
1 12 220 |
35 800.00 |
0.76 |
0.63 |
1.59 |
18
|
Thailand |
14 480 |
134 664 |
1 20 184 |
9.30 |
2.94 |
0.34 |
53
|
India |
11 494 |
44 836.80 |
33 342.80 |
3.90 |
3.05 |
0.32 |
54
|
Sugarbeet |
Turkey |
34 201.75 |
285 600 |
2 51 398.25 |
8.35 |
1.98 |
0.55 |
55
|
Walnut |
Turkey |
19 594.3 |
29 567.1 |
26 728.2 |
1.80 |
0.17 |
16.80 |
56
|
Pistachio |
Iran |
29 437.7 |
24 515.3 |
9972.7 |
0.90 |
0.07 |
26.73 |
58
|
Hazelnut |
Iran |
2862.6 |
11 255 |
8392.4 |
3.9 |
0.16 |
6.36 |
57
|
Tobacco |
Turkey |
25 523.3 |
840 |
−24 683.3 |
0.03 |
0.04 |
24.31 |
55
|
3.11 Global average energy input–output and energy indices of horticultural crops
Fig. 6(a) and (b) show the global average variation in energy input–output of horticultural crops. Table 5 provides country-wise energy input–output as well as energy ratios of horticultural crops. Among the vegetable crops, cucumber (148
897 MJ ha−1)64 had a more significant energy input followed by tomato (72
192 MJ ha−1),16 chili (41
552 MJ ha−1), potato (28
613 MJ ha−1)60 and eggplant (13
911 MJ ha−1).65 Eggplant with the lowest energy input had the highest output of 125
612 MJ ha−1,65 followed by cucumber (95
809 MJ ha−1),64 potato (92
296 MJ ha−1),60 tomato (18
960 MJ ha−1)16 and chili (18
699 MJ ha−1).66 Input energy was more than output energy in cucumber, tomato, and chili production; therefore, production was not profitable. Among fruit crops, the energy ratio of grapes was the highest (2.152). Cultivation of grapes59 had better prospects than that of apples, as apple growers with 42
819 MJ ha−1 of input energy could extract an output of only 49
857 MJ ha−1 leading to an energy ratio of 1.16.60
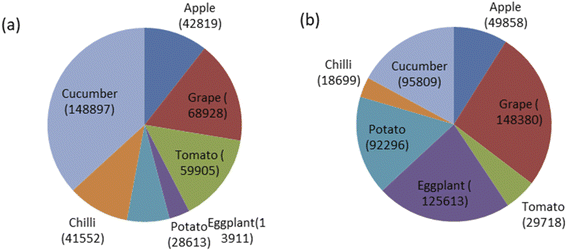 |
| Fig. 6 (a) and (b) Global average variation in input and output energy (MJ ha−1) of horticultural crops. | |
Table 5 Global energy inputs and outputs for different horticultural crops
Crops |
Country |
Input energy (MJ ha−1) |
Output energy (MJ ha−1) |
Net energy (MJ ha−1) |
Energy ratio |
Energy productivity (kg MJ−1) |
Specific energy (MJ kg−1) |
Ref. |
Apple |
Iran |
42 819 |
49 858 |
7039.00 |
1.16 |
0.79 |
1.26 |
59
|
Apple |
Iran |
36 135.16 |
42 278.36 |
6143.20 |
1.17 |
0.49 |
2.05 |
77
|
Apple |
Turkey |
43 404.31 |
65 508.14 |
22 103.83 |
1.51 |
0.63 |
1.59 |
78
|
Apricot |
Turkey |
22 806.2 |
45 968.1 |
23 161.9 |
2.00 |
0.56 |
2.33 |
79
|
Banana |
Turkey |
51 560.1 |
98 024.9 |
4646.8 |
1.90 |
1.00 |
1.00 |
63
|
Cherry |
Turkey |
36 231.1 |
38 001.5 |
1770.4 |
1.10 |
0.42 |
2.56 |
62
|
Grapes |
Iran |
45 213 |
218 654.00 |
174 525.70 |
4.95 |
0.42 |
2.40 |
59
|
Kiwifruit |
Iran |
33 802.8 |
45 039.9 |
11 237.1 |
1.40 |
0.71 |
1.43 |
80
|
Lemon |
Turkey |
62 977.9 |
66 500.0 |
3522.1 |
1.10 |
0.56 |
1.80 |
61
|
Mandarin |
Turkey |
63 169.7 |
58 425.0 |
−4744.7 |
1.00 |
0.51 |
2.04 |
Orange |
Turkey |
48 886.8 |
65 668.8 |
16 782.0 |
1.40 |
0.76 |
1.40 |
Strawberry |
Iran |
805 379.70 |
121 891.33 |
683 488.00 |
0.15 |
0.08 |
12.55 |
81
|
Strawberry |
Iran |
36 822.90 |
55 965.00 |
19 142.60 |
0.48 |
0.25 |
3.96 |
82
|
Peach |
Greece |
42 744.2 |
20 842.4 |
−21 901.9 |
0.50 |
0.26 |
3.90 |
83
|
Pear |
Iran |
172 608.43 |
88 030.30 |
84 466.30 |
0.51 |
0.27 |
3.72 |
84
|
Watermelon |
Iran |
37 103.1 |
59 231.3 |
22 128.2 |
1.60 |
1.74 |
0.69 |
85
|
Potato |
Iran |
28 613 |
92 296 |
63 683.00 |
3.22 |
0.30 |
3.20 |
60
|
Cucumber |
Iran |
148 837 |
95 809 |
53 028.00 |
0.80 |
0.80 |
1.24 |
64
|
Eggplant |
Iran |
13 911 |
125 613 |
1 11 702.00 |
9.02 |
1.53 |
0.65 |
65
|
Tomato |
Turkey |
72 192 |
18 960 |
53 232.00 |
0.25 |
0.36 |
2.81 |
16
|
Pepper |
Iran |
80 253.4 |
80 000 |
−253.4 |
1.00 |
0.80 |
1.25 |
86
|
Garlic |
Iran |
40 307.9 |
26 830.1 |
−13 477.8 |
0.70 |
0.42 |
2.40 |
20
|
Onion |
Morocco |
107 483.0 |
84 269.17 |
23 213.83 |
0.78 |
0.54 |
1.85 |
87
|
Lettuce |
Indonesia |
24 540 |
12 024 |
12 516.00 |
0.49 |
0.33 |
3.07 |
66
|
Tomato |
47 619 |
40 476 |
7143.00 |
0.85 |
1.06 |
0.94 |
66
|
Chilli |
41 552 |
18 699 |
22 853.00 |
0.45 |
0.56 |
1.78 |
66
|
3.12 Different forms of energy
Table 6 summarises the energy form analysis of agricultural production for each categorization. The maximum value of direct energy is found in vegetables (38951.5 MJ ha−1), while the lowest value is found in tobacco (12
036.1 MJ ha−1). In the case of indirect energy, the highest value is found in leguminous crops (37
119.92 MJ ha−1), whereas oilseed crops have the lowest (10
316.06 MJ ha−1). Vegetable crops have the highest renewable energy value (21
341.2 MJ ha−1), whereas oilseed crops have the lowest value (1578.56 MJ ha−1). In the case of non-renewable energy, legumes have the highest value (213
713.84 MJ ha−1), whereas tobacco has the lowest value (16
443.3 MJ ha−1).
Table 6 Average, higher and lower values of different types of energy of classified crops
Classifications |
Direct energy |
Indirect energy |
Renewable energy |
Non renewable energy |
Cereals |
Average |
14 169.95 |
19 097.79 |
6172.52 |
27 095.22 |
Higher |
19 816.1 (wheat) |
28 922.1 (corn) |
9056.7 (wheat) |
95 604 (corn) |
Lower |
10 765.2 (rice) |
11 462.4 (barley) |
4353.5 (barley) |
41 655.5 (wheat) |
Pulses/legumes |
Average |
27 042.42 |
37 119.92 |
4041 |
213 713.84 |
Higher |
430 130(Alfalfa) |
882 641 (alfalfa) |
55 020 (alfalfa) |
452 512 (alfalfa) |
Lower |
5385 (chikpea) |
28 410 (chickpea) |
954 (chickpea) |
23 025 (chickpea) |
Oilseed crop |
Average |
8876.24 |
10 316.06 |
1578.56 |
17 519.8 |
Higher |
27 376.5 (soybean) |
515 208.0 (coconut) |
26 107.9 (sunflower) |
497 655.0 (coconut) |
Lower |
9872.8 (sesame) |
16 437.5 (sesame) |
657.5 (sesame) |
6564.7 (sesame) |
Fiber/cash crop |
Average |
16 918.34 |
16 229.08 |
5184.06 |
27 963.36 |
Higher |
33 147.4 (cotton) |
66 128.8 (cotton) |
2698.3 (cotton) |
32 981.3 (cotton) |
Lower |
33 147.4 (cotton) |
66 128.8 (cotton) |
2698.3 (cotton) |
32 981.3 (cotton) |
Nuts |
Average |
9283.6 |
12 443.4 |
3142 |
18 585.11 |
Higher |
29 437.7 (pistachio) |
29 567.1 (walnut) |
2631.9 (walnut) |
9972.7 (walnut) |
Lower |
2862.6 (hazelnut) |
11 255.0 (hazelnut) |
450.2 (hazelnut) |
4922.4 (pistachio) |
![[thin space (1/6-em)]](https://www.rsc.org/images/entities/char_2009.gif) |
Horticultural crops
|
Vegetables |
Average |
38 951.5 |
33 318.84 |
12 341.2 |
59 929.09 |
Higher |
126 167.3 (cucumber) |
92 822.0 (eggplant) |
84 530.3 (cucumber) |
36 525.4 (eggplant) |
Lower |
24 537.0 (lettuce) |
3680.0 (lettuce) |
8000.0 (lettuce) |
58 543 (cucumber) |
Fruits |
Average |
19 874.2 |
21 734.5 |
9881 |
31 727.7 |
Higher |
51 560.1 (banana) |
116 890.8 (grape) |
51 592.0 (banana) |
82 357.3 (grape) |
Lower |
228.6.2 (apricot) |
15 141.4 (strawberry) |
9906.0 (grape) |
2669.7 (strawberry) |
Tobacco |
Average |
12 036.1 |
13 487.1 |
9079.96 |
16 443.3 |
Higher |
12 036.1 (tobacco) |
13 487.1 (tobacco) |
9079.96 (tobacco) |
16 443.3 (tobacco) |
Lower |
12 036.1 (tobacco) |
13 487.1 (tobacco) |
9079.96 (tobacco) |
16 443.3 (tobacco) |
3.13 Mechanization index
For each classification, Table 7 provides the greatest, lowest, and average values of the mechanisation index of agricultural production. The mechanization index was calculated using eqn (5). According to the study, the average mechanisation index level in total agricultural categories was 0.52, with the lowest value of 0.35 in vegetable crops and the greatest value of 0.77 in cereal crops. The research on the mechanisation index showed that as the mechanisation index increases, so does the value of human energy, as machines replace labour. Corn has the greatest mechanisation index (0.90) among cereal crops, whereas rice has the lowest (0.61), which explains why rice is produced in underdeveloped nations. Regarding fruit crop productivity, banana has the greatest mechanisation index value (0.81) while strawberry has the lowest (0.12). The highest and lowest yields in the nut crops are pistachios (0.60) and hazelnuts (0.46). In the case of oil crops, there is a greater mechanization index for rapeseed (0.66) and the lowest for coconuts (031). In leguminous crops, the highest and lowest values of the mechanized index were obtained by alfalfa (0.87) and chickpea (0.47), respectively. Fiber crops scored a 0.52 (cotton) value in the mechanization index.
Table 7 Mechanization index of classified crop production
Classification |
Cereals |
Vegetables |
Fruits |
Nuts |
Oil crops |
Leguminous crops |
Fiber crops |
Tobacco |
Mechanization index |
Average |
0.77 |
0.35 |
0.45 |
0.54 |
0.37 |
0.67 |
0.52 |
0.23 |
Maximum |
0.90 (corn) |
0.55 (lettuce) |
0.80 (banana) |
0.60 (pistachio) |
0.66 (rapeseed) |
0.87 (alfalfa) |
0.52 (cotton) |
0.23 (tobacco) |
Minimum |
0.611 (rice) |
0.03 (watermelon) |
0.12 (strawberry) |
0.46 (hazelnut) |
0.31 (coconut) |
0.47 (chickpea) |
0.52 (cotton) |
0.23 (tobacco) |
Ranking |
|
1 |
7 |
5 |
3 |
6 |
2 |
4 |
8 |
Agricultural operations have traditionally relied on both machinery and labour, but machinery is rapidly displacing manpower, saving time and enhancing the quality of agricultural operations. According to ref. 88, mechanical power has grown from 41.54 percent of total agricultural power to 82.85 percent, and mechanical tractive power (tractor and power tiller) has increased from 8.46 percent to 32.85 percent between 1971 and 2001.
Traditional agriculture usually requires more investment than automated agriculture. Higher land and labour productivity and applying appropriate automation technologies increase yield and lower cultivation costs. The major goal of mechanisation utilisation in a developed nation is to reduce costs or labour energy, but in a developing country, it is to increase productivity.26
4. Conclusions
The following concluding remarks are drawn from this study:
• This study would provide valuable information to the farming community and policymakers on a global scale to carry out/suggest suitable variations in agricultural practices leading to significant energy management in crop production systems.
• The share of fertilizers in energy consumption was the second highest (20%) of the overall energy input. Due to the limited arable land, this was used as an alternative to attain an increased crop yield. This is in response to the increasing population and need to offer appropriate and sufficient dietary nutrition. In addition, farmers had limited knowledge of the appropriate dosage leading to excessive usage of chemical fertilizers.
• The energy input and output varied according to geographical location and type of crops. The energy input and output of major cereal crops varied between 2736.46–35
737.13 MJ ha−1 and 37
906.00–1
00
346 MJ ha−1 in wheat crops, 13
781.00–24
116.40 MJ ha−1 and 127
632.00–1
61
586 MJ ha−1 in paddy/rice and 3283.00–7000.00 MJ ha−1 and 6943–9514 MJ ha−1 in millet crops, respectively. However, energy input was more in the case of horticultural crops such as cucumber (148
837 MJ ha−1), grapes (68
928 MJ ha−1), tomato (47
619 MJ ha−1), chilli (41
552 MJ ha−1), apples (42
819 MJ ha−1), potato (28
613 MJ ha−1) and eggplant (13
911 MJ ha−1), respectively. The highest values of the energy ratio, specific energy and energy productivity were recorded as 29.4 (coconut), 26.73 MJ kg−1 (pistachios) and 6.67 kg MJ−1 (millet), while the lowest values were obtained in tobacco (0.03), sugarcane (0.32 MJ kg−1) and tobacco (0.04 kg MJ−1).
• The mechanization index level varied from the highest value of 0.90 in cereal crops to the lowest value of 0.03 in fruit crops leading to an average value of 0.52.
• Several studies have been undertaken on energy input–output analysis and their linkages, as demonstrated in prior works, focusing on fruit, cereal, and vegetable crops. However, research on energy usage in other crops, such as beverage and spice crops as well as fiber crops, is scarce. There is an urgent need to conduct such an energy-exergy analysis to determine the future actions to enhance the efficiency of agricultural production systems.
• The study of energy usage in crop production may be used to create a reliable computer aided software/programme for computing different energy efficiency measures to optimize energy usage.
Conflicts of interest
There are no conflicts to declare.
References
- R. Nandan, S. P. Poonia, S. S. Singh, C. P. Nath, V. Kumar, R. K. Malik, A. McDonald and K. K. Hazra, Potential of conservation agriculture modules for energy conservation and sustainability of rice-based production systems of Indo-Gangetic Plain region, Environ. Sci. Pollut. Res., 2021, 28(1), 246–261 CrossRef CAS.
- S. Elsoragaby, A. Yahya, M. R. Mahadi, N. M. Nawi and M. Mairghany, Energy utilization in major crop cultivation, Energy, 2019 Apr 15, 173, 1285–1303 Search PubMed.
- R. Kargwal, V. K. Yadvika, M. K. Garg, M. K. Vinod and M. Mathur, Energy Consumption Pattern of Value Added Products of Pearl Millet, Energy, 2019, 35(1), 1–5 Search PubMed.
- S. C. Deshmukh and V. A. Patil, Energy conservation and audit, International Journal of Scientific and Research Publications, 2013, 3(8), 1–5 Search PubMed.
- S. Khan, M. A. Khan and N. Latif, Energy requirements and economic analysis of wheat, rice and barley production in Australia, Soil Environ., 2010, 29(1), 61–68 CAS.
- T. YILDIZ, An input-output energy analysis of wheat production in Çarşamba district of Samsun province, Gaziosmanpaşa Üniversitesi Ziraat Fakültesi Dergisi., 2016, 33(3), 10–20 Search PubMed.
- A. K. Islam, M. A. Rahman, R. I. Saker, M. Ahiduzzaman and M. A. Baqui, Energy audit for rice production under power tiller and bullock farming systems in Bangladesh, J. Biol. Sci., 2001, 1, 873–876 CrossRef.
- J. R. Quilty, J. McKinley, V. O. Pede, R. J. Buresh, T. Q. Correa Jr and J. M. Sandro, Energy efficiency of rice production in farmers' fields and intensively cropped research fields in the Philippines, Field Crops Research, 2014 Nov 1, 168, 8–18 Search PubMed.
- S. Yuan and S. Peng, Input-output energy analysis of rice production in different crop management practices in central China, Energy, 2017, 141, 1124–1132 CrossRef.
- V. Khambalkar, J. Pohare, S. Katkhede, D. Bunde and S. Dahatonde, Energy and economic evaluation of farm operations in crop production, J. Agric. Sci., 2010, 2(4), 191 Search PubMed.
-
K. R. Sudha, Ecomomicsanalysis of Energy Utilization in Rainfed Area Sugarcane Production in Srikakulam District of Andhra Pradesh, M.Sc. thesis, 2006 Search PubMed.
-
FAO and Agricultural Organization of United Nation, Office of the Secretary-General's Envoy on Youth Annual Report 2020, 2020, pp. 8–9, https://express.adobe.com/page/06PsUc57OP1hI, accessed on 15th March 2022 Search PubMed.
- A. Koocheki, R. Ghorbani, F. Mondani, Y. Alizade and R. Moradi, Pulses production systems in term of energy use efficiency and economical analysis in Iran, International Journal of Energy Economics and Policy, 2011, 1(4), 95–106 Search PubMed.
-
WorldAtlas, Oilseeds and Products Annual Report (Global Agricultural Information Report), 2020, https://www.statista.com/statistics/267271/worldwide-oilseed-production-since-2008/ Search PubMed.
-
Statista, Cotton Production by Country Worldwide in 2017/2018 (In 1
000 Metric Tons), The Statistics Portal, 2019 Search PubMed.
- B. Ozkan, R. F. Ceylan and H. Kizilay, Comparison of energy inputs in glasshouse double crop (fall and summer crops) tomato production, Renewable Energy, 2011, 36(5), 1639–1644 CrossRef.
- R. S. Yadav and N. K. Khandelwal, Effect of various energy inputs on energy requirement for wheat production in agro-climatic region (Kamore plateau and Satpura Hill), MP India, Int. J. Eng. Res. Ind. Appl., 2013, 3(3), 531–536 Search PubMed.
- M. Karimi, A. RajabiPour, A. Tabatabaeefar and A. Borghei, Energy analysis of sugarcane production in plant farms a case study in Debel Khazai Agro-industry in Iran, Am.-Eurasian J. Agric. Environ. Sci., 2008, 4(2), 165–171 Search PubMed.
- V. P. Chaudhary, B. Gangwar and D. K. Pandey, Auditing of energy use and output of different cropping systems in India, Agricultural Engineering International: CIGR Journal, 2006, 1–13 Search PubMed.
- N. Samavatean, S. Rafiee, H. Mobli and A. Mohammadi, An analysis of energy use and relation between energy inputs and yield, costs and income of garlic production in Iran, Renewable Energy, 2011, 36(6), 1808–1813 CrossRef.
- C. R. Bojaca and E. Schrevens, Energy assessment of peri-urban horticulture and its uncertainty: case study for Bogota, Colombia, Energy, 2010, 35(5), 2109–2118 CrossRef.
- K. P. Singh, V. Prakash, K. Srinivas and A. K. Srivastva, Effect of tillage management on energy-use efficiency and economics of soybean (Glycine max) based cropping systems under the rainfed conditions in North-West Himalayan Region, Soil Tillage Res., 2008, 100(1–2), 78–82 CrossRef.
- H. Sahabi, H. Feizi and S. Amirmoradi, Which crop production system is more efficient in energy use: wheat or barley?, Environment, Development and Sustainability, 2013, 15(3), 711–721 CrossRef.
- H. Kazemi, B. Kamkar, S. Lakzaei, M. Badsar and M. Shahbyki, Energy flow analysis for rice production in different geographical regions of Iran, Energy, 2015, 84, 390–396 CrossRef.
- S. H. Mousavi Avval, S. Rafiee, A. Jafari and A. Mohammadi, Improving energy productivity of sunflower production using data envelopment analysis (DEA) approach, J. Sci. Food Agric., 2011, 91(10), 1885–1892 CrossRef.
- R. Fadavi, A. Keyhani and S. S. Mohtasebi, Estimation of a mechanization index in apple orchard in Iran, J. Agric. Sci., 2010, 2(4), 180–185 Search PubMed.
- O. Gökdoğan and B. Sevim, Determination of energy balance of wheat production in Turkey: A case study of Eskil district, Tekirdağ Ziraat Fakültesi Dergisi., 2016, 13(4), 36–43 Search PubMed.
- G. Kusek, H. H. Ozturk and S. Akdemir, An assessment of energy use of different cultivation methods for sustainable rapeseed production, J. Cleaner Prod., 2016, 112, 2772–2783 CrossRef.
- P. Singh, G. Singh and G. P. Sodhi, Applying DEA optimization approach for energy auditing in wheat cultivation under rice-wheat and cotton-wheat cropping systems in north-western India, Energy, 2019, 181, 18–28 CrossRef.
-
F. Baptista, L. L. Silva, C. de Visser, J. Gołaszewski, A. Meyer-Aurich, D. Briassoulis, H. Mikkola, D. Murcho, C. Marques, J. R. Marques da Silva and M. Rosado, Energy Efficiency in Agriculture. Showcase and Alternatives for Wheat Production in Portugal, 2014, pp. 45–59 Search PubMed.
-
A. K. Islam, M. M. Hossain, R. I. Sarker, M. A. Saleque, M. A. Rahman, M. E. Haque and R. W. Bell, Energy Utilization in Unpuddled Transplanting of Wet Season Rice, 2011, pp. 1–5 Search PubMed.
- A. Muazu, A. Yahya, W. I. Ishak and S. Khairunniza-Bejo, Energy audit for sustainable wetland paddy cultivation in Malaysia, Energy, 2015, 87, 182–191 CrossRef.
- P. Pradhan, R. K. Naik, M. Sahu and C. Thakur, A study on the energy use pattern and cost of production under transplanted paddy production system in Chhattisgarh, India, Int. J. Eng. Res. Technol., 2015, 4(7), 1014–1018 Search PubMed.
- I. Mani and S. K. Patel, Energy consumption pattern in production of paddy crop in haryana state in India, AMA, Agricultural Mechanization in Asia, Africa and Latin America, 2012, 43(2), 39–42 Search PubMed.
- V. P. Chaudhary, K. K. Singh, G. Pratibha, R. Bhattacharyya, M. Shamim, I. Srinivas and A. Patel, Energy conservation and greenhouse gas mitigation under different production systems in rice cultivation, Energy, 2017, 130, 307–317 CrossRef CAS.
- R. Chilur and S. Yadachi, Energy audit of maize production system of selected villages of North Karnataka, India, Int. J. Curr. Microbiol. Appl. Sci., 2017, 6(8), 3564–3571 CrossRef.
- Z. B. Barut, C. Ertekin and H. A. Karaagac, Tillage effects on energy use for corn silage in Mediterranean Coastal of Turkey, Energy, 2011, 36(9), 5466–5475 CrossRef.
- M. S. Abubakar and D. Ahmad, Pattern of energy consumption in millet production for selected farms in Jigawa, Nigeria, Aust. J. Basic Appl. Sci., 2010, 4(4), 665–672 Search PubMed.
- M. S. Sidhpuria, P. S. Sangwan, B. S. Jhorar, S. B. Mittal, S. K. Sharma and A. Kumar, Resource Conservation Practices in Rainfed Pearl Millet-Energy Input-Output Analysis, Indian J. Dryland Agric. Res. & Dev., 2014, 29(2), 83–86 Search PubMed.
-
M. S. Abubakar, Energy use pattern in millet production in semi-arid zone of Nigeria, in Crop Production Technologies, IntechOpen, 2012, pp. 263–276 Search PubMed.
- R. Kargwal, M. K. Yadvika, V. K. Singh and Y. K. Yadav, Energy auditing of pearl millet production system in dry land region of Haryana Agrcultural University in Hisar, India, Int. J. Agric. Biol. Eng., 2019, 12(2), 191–195 Search PubMed.
- M. Yousefi and A. M. Damghani, Evaluation of energy flow and indicators of chickpea under rainfed condition in Iran, International Journal of Farming and Allied Sciences, 2012, 1(2), 57–61 Search PubMed.
- D. S. Karale, V. P. Khambalkar, S. M. Bhende, S. Amale and P. Wankhede, Energy economic of small farming crop production operations, World J. Agric. Sci., 2008, 4(4), 476–482 Search PubMed.
- Z. Ramedani, S. Rafiee and M. D. Heidari, An investigation on energy consumption and sensitivity analysis of soybean production farms, Energy, 2011, 36(11), 6340–6344 CrossRef.
- Y. Mohammad and M. Ali, Economical analysis and energy use efficiency in alfalfa production systems in Iran, Sci. Res. Essays, 2011, 6(11), 2332–2336 Search PubMed.
- S. Firouzi and H. Aminpanah, Energy use efficiency for groundnut (Arachis hypogaea L.) production in a semi-mechanized cultivation system, Ann. Biol. Res., 2012, 3(8), 3994–3997 Search PubMed.
- M. S. Davoodi and E. Houshyar, Energy consumption of canola and sunflower production in Iran, American-Eurasian Journal of Agricultural and Environmental Science., 2009, 6(4), 381–384 Search PubMed.
- M. G. Akpinar, B. Ozkan, C. Sayin and C. Fert, An input-output energy analysis on main and double cropping sesame production, J. Food, Agric. Environ., 2009, 7(3/4), 464–467 Search PubMed.
- A. Nabavi-Pelesaraei, R. Abdi and S. Rafiee, Applying artificial neural networks and multi-objective genetic algorithm to modeling and optimization of energy inputs and greenhouse gas emissions for peanut production, Int. J. Biosci., 2014, 4(7), 170–183 CAS.
- M. F. Baran, Energy efficiency analysis of cotton production in Turkey: A case study for Adiyaman province, Am.-Eurasian J. Agric. Environ. Sci., 2016, 16(2), 229–233 CAS.
- S. H. Pishgar-Komleh, P. Sefeedpari and M. Ghahderijani, Exploring energy consumption and CO2 emission of cotton production in Iran, J. Renewable Sustainable Energy, 2012, 4(3), 033115 CrossRef.
- A. I. Bamgboye and B. S. Kosemani, Energy input in the production of cassava, Adv. Energy Environ. Res., 2015, 5(1), 42–48 Search PubMed.
- A. Chamsing, V. M. Salokhe and G. Singh, Energy consumption analysis for selected crops in different regions of Thailand, Agricultural Engineering International: CIGR Journal, 2006, 1–18 Search PubMed.
- R. SINGH, O. P. Gupta and S. K. Patel, Energy use pattern and scenario change in sugarcane (ratoon) cultivation for Bhabar region of Uttarakhand, India, Journal of AgriSearch, 2015, 2(2), 119–125 Search PubMed.
- M. F. Baran and O. Gokdogan, Determination of energy balance of sugar beet production in Turkey: a case study of Kırklareli Province, Energy Effic., 2016, 9(2), 487–494 CrossRef.
- M. F. Baran, O. Gökdoğan and H. İ. Oğuz, Determining the energy usage efficiency of walnut (Juglans Regia L.) cultivation in Turkey, Erwerbs-Obstbau, 2017, 59(1), 77–82 CrossRef.
- A. Nabavi-Pelesaraei, A. Sadeghzadeh, M. H. Payman and H. G. Mobtaker, An analysis of energy use, CO2 emissions and relation between energy inputs and yield of hazelnut production in Guilan province of Iran, Int. J. Adv. Biol. Biomed. Res., 2013, 1(12), 1601–1613 CAS.
- R. K. Afshar, A. Alipour, M. Hashemi, M. A. Jovini and D. Pimentel, Energy inputs-yield relationship and sensitivity analysis of pistachio (Pistacia vera L.) production in Markazi Region of Iran, Spanish Journal of Agricultural Research, 2013, 11(3), 661–669 CrossRef.
- S. R. Hamedani, A. Keyhani and R. Alimardani, Energy use patterns and econometric models of grape production in Hamadan province of Iran, Energy, 2011, 36(11), 6345–6351 CrossRef.
- S. R. Hamedani, Z. Shabani and S. Rafiee, Energy inputs and crop yield relationship in potato production in Hamadan province of Iran, Energy, 2011, 36(5), 2367–2371 CrossRef.
- B. Ozkan, H. Akcaoz and F. Karadeniz, Energy requirement and economic analysis of citrus production in Turkey, Energy Convers. Manage., 2004, 45(11–12), 1821–1830 CrossRef.
- V. Demircan, K. Ekinci, H. M. Keener, D. Akbolat and C. Ekinci, Energy and economic analysis of sweet cherry production in Turkey: A case study from Isparta province, Energy Convers. Manage., 2006, 47(13–14), 1761–1769 CrossRef.
- A. K. An, Analysis of energy use for banana production: a case study from Turkey, Afr. J. Agric. Res., 2011, 6(25), 5618–5624 Search PubMed.
- A. Mohammadi and M. Omid, Economical analysis and relation between energy inputs and yield of greenhouse cucumber production in Iran, Appl. Energy, 2010, 87(1), 191–196 CrossRef CAS.
- A. Nabavi-Pelesaraei, A. Sadeghzadeh, M. H. Payman and H. G. Mobtaker, Energy flow modeling, economic and sensitivity analysis of eggplant production in Guilan province of Iran, Int. J. Agric. Crop Sci., 2013, 5(24), 3006–3015 Search PubMed.
- N. Kuswardhani, P. Soni and G. P. Shivakoti, Comparative energy input–output and financial analyses of greenhouse and open field vegetables production in West Java, Indonesia, Energy, 2013, 53, 83–92 CrossRef.
- V. P. Chaudhary, B. Gangwar, D. K. Pandey and K. S. Gangwar, Energy auditing of diversified rice–wheat cropping systems in Indo-gangetic plains, Energy, 2009, 34(9), 1091–1096 CrossRef.
- C. M. Parihar, S. L. Jat, A. K. Singh, K. Majumdar, M. L. Jat, Y. S. Saharawat, S. Pradhan and B. R. Kuri, Bio-energy, water-use efficiency and economics of maize-wheat-mungbean system under precision-conservation agriculture in semi-arid agro-ecosystem, Energy, 2017, 119, 245–256 CrossRef.
- A. A. Saad, T. K. Das, D. S. Rana, A. R. Sharma, R. Bhattacharyya and K. Lal, Energy auditing of a maize–wheat–greengram cropping system under conventional and conservation agriculture in irrigated north-western Indo-Gangetic Plains, Energy, 2016, 116, 293–305 CrossRef.
- D. Pimentel, Energy inputs in food crop production in developing and developed nations, Energies, 2009, 2(1), 1–24 CrossRef.
- S. Khosruzzaman, M. A. Asgar, K. R. Rahman and S. Akbar, Energy intensity and productivity in relation to agriculture-Bangladesh perspective, J. Bangladesh Acad. Sci., 2010, 34(1), 59–70 CrossRef.
- K. Basavalingaiah, Y. M. Ramesha, V. Paramesh, G. A. Rajanna, S. L. Jat, S. Dhar Misra, A. Kumar Gaddi, H. C. Girisha, G. S. Yogesh, S. Raveesha and T. K. Roopa, Energy budgeting, data envelopment analysis and greenhouse gas emission from rice production system: A case study from puddled transplanted rice and direct-seeded rice system of Karnataka, India, Sustainability, 2020, 12(16), 6439 CrossRef CAS.
- O. Bazaluk, V. Havrysh, M. Fedorchuk and V. Nitsenko, Energy Assessment of Sorghum Cultivation in Southern Ukraine, Agriculture, 2021, 11(8), 695 CrossRef CAS.
- S. P. Komleh, A. Keyhani, S. H. Rafiee and P. Sefeedpary, Energy use and economic analysis of corn silage production under three cultivated area levels in Tehran province of Iran, Energy, 2011, 36(5), 3335–3341 CrossRef.
- H. Singh, D. Mishra and N. M. Nahar, Energy use pattern in production agriculture of a typical village in arid zone, India–part I, Energy Convers. Manage., 2002, 43(16), 2275–2286 CrossRef.
- P. L. Rani, M. Yakadri, N. Mahesh and P. S. Bhatt, Energy usage and economic analysis of cotton under various weed management practices, Indian J. Weed Sci., 2016, 48(1), 99–101 CrossRef.
- M. Sami, M. J. Shiekhdavoodi and A. Asakereh, Energy use in apple production in the Esfahan province of Iran, African Crop Science Journal, 2011, 19(2), 125–130 Search PubMed.
- S. Akdemir, H. Akcaoz and H. Kizilay, An analysis of energy use and input costs for apple production in Turkey, J. Food, Agric. Environ., 2012, 10(2), 473–479 Search PubMed.
- K. Esengun, O. Gündüz and G. Erdal, Input–output energy analysis in dry apricot production of Turkey, Energy Convers. Manage., 2007, 48(2), 592–598 CrossRef.
- A. Mohammadi, S. Rafiee, S. S. Mohtasebi, S. H. Avval and H. Rafiee, Energy efficiency improvement and input cost saving in kiwifruit production using Data Envelopment Analysis approach, Renewable Energy, 2011, 36(9), 2573–2579 CrossRef.
- N. Banaeian, M. Omid and H. Ahmadi, Energy and economic analysis of greenhouse strawberry production in Tehran province of Iran, Energy Convers. Manage., 2011, 52(2), 1020–1025 CrossRef.
- P. Salami, H. Ahmadi and A. Keyhani, Estimating the energy indices and profitability of strawberry production in Kamyaran zone of Iran, Energy, 2010, 1(1), 32–35 Search PubMed.
- M. C. Michos, A. P. Mamolos, G. C. Menexes, C. A. Tsatsarelis, V. M. Tsirakoglou and K. L. Kalburtji, Energy inputs, outputs and greenhouse gas emissions in organic, integrated and conventional peach orchards, Ecol. Indic., 2012, 13(1), 22–28 CrossRef CAS.
- S. M. Tabatabaie, S. Rafiee, A. Keyhani and M. D. Heidari, Energy use pattern and sensitivity analysis of energy inputs and input costs for pear production in Iran, Renewable Energy, 2013, 51, 7–12 CrossRef.
- A. Nabavi-Pelesaraei, R. Abdi and S. Rafiee, Neural network modeling of energy use and greenhouse gas emissions of watermelon production systems, J. Saudi Soc. Agric. Sci., 2016, 15(1), 38–47 Search PubMed.
- S. H. Pishgar-Komleh, M. Ghahderijani and P. Sefeedpari, Energy consumption and CO2 emissions analysis of potato production based on different farm size levels in Iran, J. Cleaner Prod., 2012, 33, 183–191 CrossRef.
- K. Allali, B. Dhehibi, S. N. Kassam and A. Aw-Hassan, Energy consumption in onion and potato production within the province of El Hajeb (Morocco): towards energy use efficiency in commercialized vegetable production, J. Agric. Sci., 2017, 9(1), 118–127 Search PubMed.
- G. Singh, Estimation of a mechanisation index and its impact on production and economic factors—A case study in India, Biosyst. Eng., 2006, 93(1), 99–106 CrossRef.
|
This journal is © The Royal Society of Chemistry 2022 |