Brimonidine–montmorillonite hybrid formulation for topical drug delivery to the eye†
Received
11th May 2020
, Accepted 21st July 2020
First published on 22nd July 2020
Abstract
Brimonidine (BMD) is often prescribed as an eye drop to reduce the intraocular pressure (IOP) for glaucoma treatment. However, eye drops are limited by rapid clearance from the preocular surface, and hence a low ocular drug bioavailability. Therefore, in this study, we propose montmorillonite (MMT), as a delivery carrier, hybridized with BMD (BMD–MMT) for topical drug delivery to the eye. The BMD–MMT hybrid was prepared by intercalating the BMD molecules in the interlayer space of the MMT lattice via ion-exchange reaction; it was then formulated with polyvinyl alcohol (PVA) to produce a dry tablet (i.e., BMD–MMT@PVA). The BMD–MMT@PVA hybrid drug released BMD in a sustained manner for more than 5 h under in vitro conditions. When the hybrid drug was administered to rabbit eyes in vivo, 43% and 18.5% BMD–MMT still remained on the preocular surface for 10 and 60 min after administration, respectively. Thus, the BMD–MMT@PVA hybrid drug exhibited a prolonged decrease in IOP, that is, for 12 h, which was approximately two times longer than that observed with the commercially available BMD eye drop, Alphagan® P.
1. Introduction
Glaucoma is the second leading cause of blindness in the world, and its prevalence is expected to increase with aging populations.1–3 It is a chronic neuro-degenerative disease, which is often caused by an increase in intraocular pressure (IOP), leading to an irreversible damage to the optic nerve and, consequently, vision loss.4,5 Therefore, the initial treatment of glaucoma focuses mostly on lowering the IOP and thus, it is frequently employed as an adjuvant therapy for patients with glaucoma even with a normal range of IOP.4,6 Brimonidine (BMD), an α2-adrenergic receptor agonist, is often prescribed in eye drops to lower the IOP in patients with glaucoma.5,7–10 However, eye drops rapidly disappear from the preocular surface due to tear clearance and blinking; thus, less than 5% of the instilled drug can be actually absorbed into the eye tissue, resulting in a low drug bioavailability.11
There is an apparent need of carrier materials for topical drug delivery to the eye. Unlike a bolus drug solution, the drug carriers, such as drug-loaded particles, can stay longer on the preocular surface,12,13 and during this period, the drug should be released in a sustained manner to eventually improve ocular drug bioavailability.14–16 For this purpose, here, we propose montmorillonite (MMT), a natural aluminosilicate clay, as a carrier for BMD delivery, as MMT can not only encapsulate cationic drug molecules in the interlayer space to the maximum capacity, but also play a role as a solid lubricant due to its lamellar structure, and eventually reduce the offending feeling to the eye when it is used in an eye drop. MMT has various biomedical applications due to its known biocompatibility.17–19 Among them, the MMT particles are often employed as a drug delivery carrier.20–23 With a superior cation exchange capacity (CEC), various protonated and hydrophilic organic molecules can be intercalated in the interlayer space of MMT, from which the molecules can be released in a sustained manner upon replacement with other cations present in the surrounding aqueous media.24
In this study, the BMD molecules were immobilized in the interlayer space of MMT via an intercalative ion-exchange reaction to produce the BMD-loaded MMT hybrid particles (i.e., the BMD–MMT). The BMD–MMT particles were then formulated into a dry tablet (i.e., BMD–MMT@PVA) with a biocompatible and water-soluble polyvinyl alcohol (PVA) as a medium.25,26 Thus, when administered topically to the eye, the tablet medium of PVA would be dissolved in tear fluids quickly to free BMD–MMT. With the increase in tear viscosity by the dissolved PVA, BMD–MMT would be better retained on the preocular surface. This retention property could be more effective as the formulation did not contain any additional fluid, which often causes expedited tear clearance, as observed with conventional eye drops.15,27 In this study, we carried out powder X-ray diffraction (PXRD), Fourier transform-infrared spectroscopy (FTIR), and dynamic laser scattering (DLS) analyses to confirm successful formation of BMD–MMT. The drug release behavior of BMD–MMT@PVA was assessed under in vitro conditions. In vivo evaluations were also performed with rabbit eyes, where the BMD–MMT@PVA hybrid drug was applied topically and the preocular retention property of BMD–MMT was assessed. The pharmacodynamic profile of this hybrid drug was also examined, and compared with that of Alphagan® P, a commercially available BMD eye drop.
2. Experimental section
2.1 Materials
Montmorillonite (Kunipia-F; cation exchange capacity (CEC) = 115 milliequivalents/100 g) was purchased from Kunimine Industries (Tokyo, Japan) and used without further purification. The chemical formula of MMT is Na0.7K0.02Ca0.04(Si7.78Al0.22) (Al3.20Mg0.64Fe0.16)O20(OH)4. Polyvinyl alcohol (average molecular weight = 31.50 kDa, 87–89% hydrolyzed) was supplied by Sigma (MO, USA). Brimonidine tartrate (BMD, purity > 99.8%) was purchased from Nanjing Yuance Industry & Trade (Nanjing, China). Phosphate-buffered saline (PBS, pH 7.4) was obtained from Seoul National University Hospital Biomedical Research Institute (Seoul, Korea). Proparacaine eye drops (Alcaine®; 0.5% ophthalmic solution) were obtained from Alcon-Couvreur (Puurs, Belgium). Alphagan® P was gifted by Samil Allergan (Seoul, Korea).
2.2 Preparation of BMD–MMT and BMD–MMT@PVA
The BMD–MMT particles were prepared via an intercalative ion-exchange reaction (Fig. 1).28–31 Briefly, 1 g of MMT was dispersed in 100 mL of deionized water for 3 h at room temperature to obtain a 1.0 wt% MMT suspension. To this suspension, 442 mg of BMD was dissolved to be equivalent to the CEC of MMT, which was stirred for 4 h at room temperature to facilitate the ion exchange reaction. The solid product of BMD–MMT was then filtered and washed twice with deionized water to remove tartrate anions and residual BMD molecules remained in the reaction solution. The actual loading amount of BMD in the BMD–MMT hybrid was obtained by subtracting the amount of residual BMD after intercalation reaction from that added to the initial solution (442 mg). The concentration of BMD was measured by high-performance liquid chromatography (HPLC, Agilent 1260 series; Agilent Technologies, CA, USA) using Poroshell (120 EC-C18, 4.6 × 100 mm, 2.7 μm-pore; Agilent Technologies, CA, USA) as previously reported.12,15
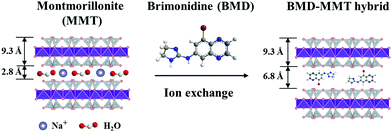 |
| Fig. 1 Schematic illustration of the BMD molecules intercalated in the MMT layer via ion-exchange reaction. | |
To prepare the dry tablet formulation, that is, the BMD–MMT@PVA hybrid drug, a known amount of the BMD–MMT hybrid, equivalent to 52.5 μg BMD, was suspended homogeneously in 40 μL of 2% w/v PVA solution. The particle suspension was poured into a mold of oval shape (6.5 mm × 3.5 mm × 2.5 mm; l × w × h) and immediately frozen and lyophilized (FreeZone 18; Labconco, MO, USA) for 1 day. We also prepared a PVA tablet with BMD only (i.e., BMD@PVA). For this, 52.5 μg of BMD was completely dissolved in 40 μL of 2% w/v PVA solution, which was then poured into the same mold and freeze-dried as described above.
2.3 Characterization
Powder X-ray diffraction (PXRD) analysis was performed using the Rigaku D/MAX RINT 2200-Ultima+ diffractometer (Tokyo, Japan), equipped with a Ni-filtered Cu Kα radiation source (λ = 1.5418 Å). The PXRD patterns were recorded at a tube voltage of 40 kV and current of 30 mA. FTIR spectroscopy was performed using the Jasco FT/IR-6100 spectrometer (Tokyo, Japan) following the standard KBr-disk method in the transmission mode (spectroscopic range: 4000–400 cm−1, resolution: 1 cm−1, 40 scans per spectrum). The particle size and surface charge of pristine MMT and the BMD–MMT hybrid were measured at 0.1 wt% in deionized water using the Coulter counter (Multisizer 4; Beckman Coulter, CA, USA) and zetasizer (Zetasizer Nano ZS; Malvern Instruments, Malvern, UK), respectively.
2.4
In vitro drug release study
In vitro drug release study was carried out using the paddle stirring method with the DST-810 dissolution tester (Labfine, Seoul, Korea),32 where the impeller speed and bath temperature were set at 50 rpm and 37 °C, respectively. For this, the BMD@PVA or BMD–MMT@PVA hybrid drug was immersed in the release medium of PBS pH 7.2 and the aliquot was collected at scheduled intervals for 24 h. The sampled aliquot was filtered using a 0.45 μm-pore polypropylene membrane filter (Pall Corporation, NY, USA) and analyzed by HPLC as described above. The experiments were performed in triplicate for each tablet type.
2.5
In vivo evaluation
For in vivo evaluations, we used male New Zealand White rabbits without ocular abnormalities (3.0–4.0 kg; Cheonan Yonam College, Chungcheongnam-do, Korea). The experimental protocol was approved by the Institutional Animal Care and Use Committee (IACUC No. 13-0101) at the Biomedical Research Institute of the Seoul National University Hospital. The rabbits were placed in a separate standard cage in a well-controlled environment (temperature, 21 ± 1 °C; humidity, 55 ± 1%; and 12/12 h light/dark cycle) and provided food and water ad libitum.
We first assessed the preocular retention property of the BMD–MMT hybrid particles, following our previous protocol with slight modifications.15,33 Briefly, the BMD–MMT@PVA hybrid drug or a suspension of the BMD–MMT hybrid, each containing 52.5 μg BMD, was administered to the lower cul-de-sac of the left eye, whereas the right eye was left untreated (Fig. S1 in the ESI†). Then, the rabbits were returned to the cage until sample collection. At scheduled times of 10, 30, 60, and 120 min after administration, the rabbits were placed in a restraining bag and a 35 μL drop of 0.5% proparacaine eye drops was administered topically to anesthetize the eye surface. The particles were then collected by wiping the entire preocular surface with a surgical sponge (PVA Spears; Network Medical Products, Ripon, UK). The surgical sponge was then fully immersed in an acidic solution mixed with hydrofluoric acid/nitric acid/water (2
:
3
:
4, v/v/v) and subjected to microwave digestion; the sample was subjected to inductively coupled plasma mass (ICP-MS) spectroscopy (Agilent 7800, Agilent Technologies) to quantify the amount of Si. We determined the amount of the BMD–MMT particles remaining on the preocular surface; the values are presented as a percentage based on the amount of the initially administered BMD–MMT. To examine the ophthalmic drug efficacy, we assessed the profile of IOP after the administration of the BMD-loaded formulations.15,34 Briefly, BMD–MMT@PVA or BMD–MMT suspension, each containing 52.5 μg BMD, was administered as described above. At scheduled times of 1, 2, 4, 6, 8 and 12 h after administration, the rabbits were placed in a restraining bag and a 35 μL drop of 0.5% proparacaine eye drops was administered topically. Then, a tonometer (Tono-Pen AVIA; Reichert, NY, USA) was applied to the eye for IOP measurements. The change in the IOP at each scheduled time is presented as a percentage based on the initial value of IOP measured before administration:15,35
For all in vivo experiments, at least three eyes were tested at each sampling time and for each formulation type.
2.6 Statistical analysis
The mean percentage of particle amount remaining on the preocular surface and change in the IOP were analyzed using the Mann–Whitney U-test; differences with P < 0.05 were considered statically significant (SPSS version 22; IBM, USA).
3. Results and discussion
3.1 Particle characterization
To examine the successful fabrication of the BMD–MMT hybrid, we first assessed the PXRD patterns of the intact BMD, pristine MMT, and BMD–MMT hybrid particles. As shown in Fig. 2, due to ordered spacing between the planes in two-dimensional (2-D) lattice, the pristine MMT exhibited an apparent characteristic peak of (001) at around 7.3°,36,37 which was considerably shifted to the lower 2 theta angle of 5.5° for BMD–MMT. Therefore, according to the Bragg's law,38 the basal spacing increased from 12.1 to 16.1 Å upon the formation of the BMD–MMT intercalate, suggesting the successful immobilization of the BMD molecules in the interlayer space of MMT. The peaks of intact crystalline BMD were not observed after its hybridization with MMT, indicating that the BMD drug was completely dissolved and intercalated in the MMT interlayer space under the present synthetic condition, as previously reported with other hybrid systems.28–31 By subtracting the monolayer thickness of MMT (9.3 Å) from the basal spacing of pristine MMT (12.1 Å), the gallery height was determined to be 2.8 Å, due to the presence of solvated cations (Na+) with H2O molecules in the interlayer space of MMT.39,40 However, for the BMD–MMT hybrid, the basal spacing was further expanded to 16.1 Å with the gallery height of 6.8 Å (16.1 − 9.3 Å = 6.8 Å), implying that the BMD molecules were horizontally oriented with a monolayer structure within the MMT lattices (Fig. 1).
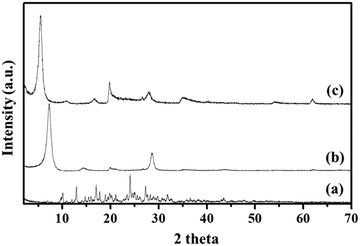 |
| Fig. 2 Powder X-ray diffraction patterns of (a) BMD, (b) pristine MMT, and (c) BMD–MMT. | |
Fig. 3 depicts the FTIR spectra of intact BMD, pristine MMT, and BMD–MMT. The intact BMD showed a characteristic peak at 1593 cm−1 corresponding to –NH bending vibration (Fig. 3a). An intense band due to the stretching vibration of the (C
N) and (C
C) groups was observed at 1651 cm−1 and the stretching band ascribed to the (C
O) group appeared at 1732 cm−1. All spectral features measured with BMD were consistent with those reported in a previous study.41 The pristine MMT showed a characteristic broad band near 3400 cm−1 due to the –OH stretching vibrations of interlayered water molecules (Fig. 3b). The bands at 3630 and 1041 cm−1 were owing to the –OH groups originating from the Al–OH and Si–O bonds in clay, respectively. The characteristic band at 915 cm−1 is due to Al–Al–OH stretching in the octahedral layer and that at 538 cm−1 was due to the Al–O–Si stretching mode.42,43 Upon intercalation of BMD into MMT, that is, with BMD–MMT, the characteristic –NH bending vibration at 1593 cm−1 from intact BMD disappeared because of the formation of the protonated ammonium ion in the MMT interlayer space (Fig. 3c). The particle size of pristine MMT and BMD–MMT was not very different (1.33 ± 0.32 and 1.43 ± 0.39 μm, respectively). The zeta potential of pristine MMT (−30.8 ± 0.32 mV) was increased upon intercalation of positively charged BMD into MMT to form the BMD–MMT hybrid (−11.3 ± 0.06 mV) due to the charge compensation effect. The loading amount of BMD in MMT was 24.5 ± 0.24 μg mg−1, measured from the BMD–MMT hybrid.
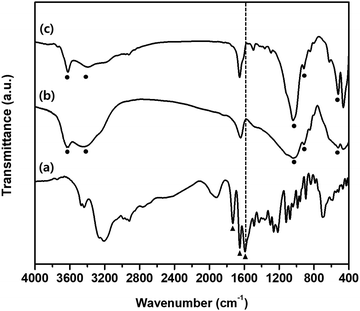 |
| Fig. 3 Fourier transform-infrared spectra of (a) BMD, (b) pristine MMT, and (c) BMD–MMT. | |
3.2
In vitro drug release profiles
We pursued to enhance the ocular bioavailability of BMD after its topical administration to the eye. For this purpose, the BMD–MMT hybrid should release BMD in a sustained manner and stay longer on the preocular surface. For the former, the BMD molecules were encapsulated in the 2-D delivery carrier MMT to form the BMD–MMT hybrid, from which the bound BMD drug molecules could be released slowly in the tear fluid. For the latter, the BMD–MMT particles were embedded in a dry tablet of water-soluble PVA to produce the BMD–MMT@PVA hybrid drug. The tablet of the BMD–MMT@PVA possessed an oval shape, which was small enough to be administered into the lower cul-de-sac of rabbit eyes herein (6.5 mm × 3.5 mm × 2.5 mm, l × w × h). As prepared by freeze-drying, the tablet medium could be highly porous to allow for rapid dissolution in tear fluids. Thus, the dissolved tablet medium PVA increases tear viscosity to hamper tear clearance mechanism. Moreover, the drug carrier MMT with a solid lubricant property tends to enhance mucosal adhesion, thereby improve preocular retention of BMD–MMT without any possible discomfort on the external eye surface.
We tested the drug release profile of the final formulation, BMD–MMT@PVA, under in vitro conditions, by comparing with BMD@PVA, that is, the PVA tablet with BMD only, without MMT. As shown in Fig. 4, BMD@PVA released almost all drug immediately. This could be ascribed to the rapid dissolution of the PVA tablet medium, which was facilitated by its porous structure created by freeze-drying in this study. However, the drug was released in a sustained manner for up to 5 h with the BMD–MMT@PVA hybrid drug. Even after the rapid dissolution of the tablet medium, the freed BMD–MMT hybrid particles still played a major role, where the BMD molecules could be diffused slowly from the 2-D lattice of MMT.17,44
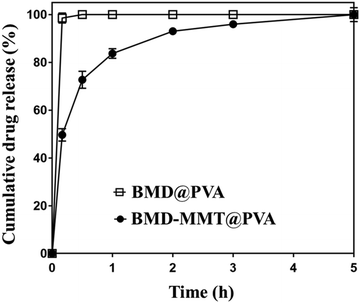 |
| Fig. 4
In vitro drug release profiles of BMD@PVA and BMD–MMT@PVA. | |
3.3
In vivo evaluation
In order to evaluate the effect of tablet formulation on preocular retention of the BMD–MMT particles, the BMD–MMT@PVA hybrid drug or BMD–MMT suspension was applied topically to rabbit eyes and the amount of particles remaining on the preocular surface was compared with respect to time. At all-time points of particle collection, the present BMD–MMT@PVA hybrid drug showed significantly higher preocular retention (P < 0.05) than the BMD–MMT suspension (Fig. 5). Even in a suspension, BMD–MMT exhibited improved retention, owing to the mucoadhesive property of MMT,18,45,46 compared with conventional eye drops,12,47 which were reported to completely disappear from the eye surface in 3–7 min after administration.11,48 However, the improvement was more prominent when BMD–MMT was formulated as a tablet in PVA medium. Due to the increase in tear viscosity by PVA dissolution, the BMD-MMT particles were not rapidly cleared from the preocular surface. Thus, more than three-fold increase in particle retention was observed at all time points compared with the suspension. Even at 120 min after administration, more than 10.2% particles were observed to remain on the preocular surface.
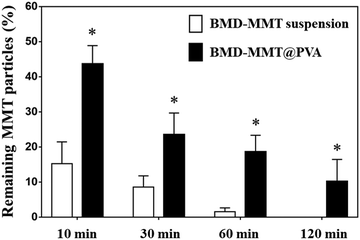 |
| Fig. 5
In vivo preocular retention profiles of the BMD–MMT particles. * BMD–MMT@PVA was significantly different from the BMD–MMT suspension (P < 0.05). | |
We also sought to determine the ocular drug efficacy with the BMD formulations, and thus, the IOP-lowering effect was examined as pharmacodynamic parameters (Table 1). For this purpose, Alphagan® P was employed as the control. As shown in Fig. 6, Alphagan® P lowered the IOP for 6 h (Tend) with the maximum decrease in the IOP (Imax) of 25.38%, which was consistent with the results in previous studies.15,49 For the BMD–MMT suspension, there was an increase in Tend to 10 h, possibly because even in a suspension, the particles could be retained on the eye surface to some extent as expected (Fig. 5) and that they would release the drug in a sustained manner (Fig. 4). Importantly, the highest improvement in drug efficacy was observed with the BMD–MMT@PVA hybrid drug. Due to the synergistic effect of improved preocular retention and sustained drug release properties, the Imax was elevated to 31.3% and the Tend was further extended to 12 h, which was two-times longer than that of Alphagan® P with the same dose of BMD. The area under the curve (AUC), a parameter that represents drug bioavailability, of the BMD–MMT@PVA hybrid drug was approximately two-times higher than that of Alphagan®. For all formulations, there was no shift in the Tmax, which was observed at 2 h after administration.
Table 1
In vivo pharmacodynamic parameters of IOP change after the administration of the BMD-loaded formulations
Formulation |
I
max (%) |
T
end (h) |
T
max (h) |
AUC |
T
max, time required to reach the maximum IOP decrease percentage. Imax, maximum IOP decrease percentage. Tend, IOP-lowering period. AUC, area under the IOP decrease percentage versus time curve. |
Alphagan® P |
25.38 ± 1.35 |
6 |
2 |
82.9 |
BMD–MMT suspension |
25.82 ± 0.96 |
10 |
2 |
122.8 |
BMD–MMT@PVA |
31.28 ± 1.92 |
12 |
2 |
164.9 |
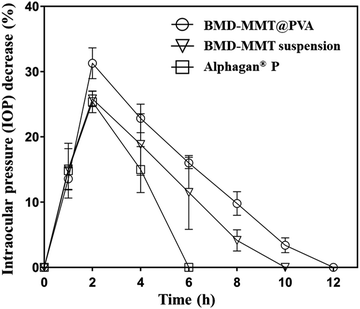 |
| Fig. 6
In vivo profiles of IOP change after the administration of Alphagan® P, BMD–MMT suspension, and BMD–MMT@PVA. | |
3.4 Perspectives of BMD–MMT in ocular drug delivery
In this study, we proposed MMT, with a 2-D structure, as a carrier for the topical delivery of BMD to the eye. The MMT herein possess a large cation exchange capacity and thus, BMD, a positively-charged drug, can be successfully intercalated and arranged at a molecular level in MMT via an ion exchange reaction (Fig. 2).12,50 Thus, the hybridized BMD–MMT could release the drug in a sustained manner (Fig. 4) due to the ionic bonding between the guest drug and host carrier. In addition, a water-soluble polymer PVA was composited with BMD–MMT to produce a dry tablet (BMD–MMT@PVA). In order not to cause any discomfort to the eye, PVA was made to be highly porous by freeze-drying. Thus, the tablet medium could dissolve in tear almost immediately after administration, where only the BMD–MMT particles were freed and remained on the preocular surface, owing to the mucoadhesive property of carrier MMT.18,45,46 The particle size of MMT, known as a solid lubricant, and its drug hybrid, BMD–MMT, was considerably <5 μm, and thus, they were not expected to cause any eye irritation and unpleasant feeling.5,51
The BMD–MMT particles could stay longer on the preocular surface even when delivered in suspensions (Fig. 5) compared with the conventional eye drops that would completely disappear within 3 min.5,52 This could be explained by the mucoadhesive property of MMT,18,45,46,53 in which the hydroxyl groups of MMT could form a strong hydrogen bonding with the mucous layer in the eye surface. This effect appeared to be more prominent when BMD–MMT was delivered in a dry tablet form, where the PVA dissolved from the tablet increased the tear viscosity to decrease tear clearance, thereby provided more time for the hybrid particles to react with the mucous layer.27,33 Thus, the BMD–MMT@PVA hybrid drug exhibited a 3- and 12-fold more BMD–MMT particles on the preocular surface at 10 and 60 min after administration, respectively, compared with those delivered in a suspension (Fig. 5). Therefore, the BMD–MMT@PVA hybrid drug would better retain the BMD–MMT particles on the preocular surface for a longer period, and during this period, they would release the drug in a sustained manner. This combined effect resulted in higher BMD absorption into the eye, and eventually enhanced the efficacy of BMD as shown by the extended Tend and AUC of the IOP-lowering curve (Fig. 6 and Table 1). The BMD–MMT particles of micron size would not be absorbed into the ocular tissues but they would eventually disappear from the preocular surface by blinking or tear clearance through nasolacrimal drainage (Fig. 5).54,55 Probably because of this and along with the known biocompatibility of the MMT,17–19 the animal eyes herein did not exhibit any apparent complications when visually observed after administration of the BMD–MMT.
4. Conclusions
In this study, we developed BMD–MMT particles in a porous PVA tablet as a potential formulation for the topical delivery of BMD, a glaucoma drug, to the eye. The BMD molecules can be encapsulated in a 2-D lattice of MMT via an intercalative ion-exchange reaction and released from the hybridized particles in a sustained manner. When formulated as a rapidly dissolving tablet of PVA, BMD–MMT particles can stay longer on the preocular surface. Overall, our findings showed that the present BMD–MMT@PVA hybrid drug significantly improved the BMD ocular bioavailability, which was more than two times higher than that of Alphagan® P, a commercially available BMD eye drop. Therefore, we conclude that this ternary hybrid drug made of BMD, MMT, and water-soluble PVA can be a promising formulation to enhance the bioavailability of topically delivered ocular drugs.
Conflicts of interest
There are no conflicts of interest.
Acknowledgements
This work was supported by Basic Science Research Program through the National Research Foundation of Korea (NRF) funded by the Ministry of Science, ICT & Future planning (2017R1A2B3004830). This work was also supported under the framework of international cooperation program managed by NRF (No. 2017K2A9A2A10013104) and by the National Research Foundation of Korea (NRF) grant funded by the Korea government (MSIT) (NRF-2019R1C1C1006300 and NRF-2019R1A4A1028700).
References
- I. P. Kaur, H. Singh and S. Kakkar, Crit. Rev. Ther. Drug, 2011, 28, 165–202 CrossRef PubMed.
- C. B. Toris and H. A. Quigley, Exp. Eye Res., 2011, 93, 238 CrossRef CAS.
- H. A. Quigley and A. T. Broman, Br. J. Ophthalmol., 2006, 90, 262–267 CrossRef CAS PubMed.
- D. A. Lee and E. J. Higginbotham, Am. J. Health-Syst. Pharm., 2005, 62, 691–699 CrossRef PubMed.
- D. Ghate and H. F. Edelhauser, Expert Opin. Drug Delivery, 2006, 3, 275–287 CrossRef CAS PubMed.
- H. A. Quigley, Lancet, 2011, 377, 1367–1377 CrossRef.
- L. B. Cantor and J. Burke, Expert Opin. Investig. Drugs, 1997, 6, 1063–1083 CrossRef CAS PubMed.
- R. J. Derick, Ophthalmology, 1997, 104, 346 CrossRef.
- C. Le Bourlais, L. Acar, H. Zia, P. A. Sado, T. Needham and R. Leverge, Prog. Retinal Eye Res., 1998, 17, 33–58 CrossRef CAS.
- E. M. del Amo and A. Urtti, Drug Discovery Today, 2008, 13, 135–143 CrossRef CAS PubMed.
- K. Jarvinen, T. Jarvinen and A. Urtti, Adv. Drug Delivery Rev., 1995, 16, 3–19 CrossRef.
- S. N. Kim, C. G. Park, B. K. Huh, S. H. Lee, C. H. Min, Y. Y. Lee, Y. K. Kim, K. H. Park and Y. B. Choy, Acta Biomater., 2018, 79, 344–353 CrossRef CAS PubMed.
- M. M. Silva, R. Calado, J. Marto, A. Bettencourt, A. J. Almeida and L. M. D. Goncalves, Mar. Drugs, 2017, 15, 370 CrossRef PubMed.
- S. N. Kim, S. A. Ko, C. G. Park, S. H. Lee, B. K. Huh, Y. H. Park, Y. K. Kim, A. Ha, K. H. Park and Y. B. Choy, Mol. Pharm., 2018, 15, 3143–3152 CrossRef CAS PubMed.
- C. G. Park, Y. K. Kim, M. J. Kim, M. Park, M. H. Kim, S. H. Lee, S. Y. Choi, W. S. Lee, Y. J. Chung, Y. E. Jung, K. H. Park and Y. Bin Choy, J. Controlled Release, 2015, 220, 180–188 CrossRef CAS PubMed.
- W. Chaiyasan, S. P. Srinivas and W. Tiyaboonchai, Mol. Vision, 2015, 21, 1224–1234 CAS.
- J. H. Yang, J. H. Lee, H. J. Ryu, A. A. Elzatahry, Z. A. Alothman and J. H. Choy, Appl. Clay Sci., 2016, 130, 20–32 CrossRef CAS.
- M. H. Kim, G. Choi, A. Elzatahry, A. Vinu, Y. B. Choy and J. H. Choy, Clays Clay Miner., 2016, 64, 115–130 CrossRef CAS PubMed.
- J. I. Dawson and R. O. Oreffo, Adv. Mater., 2013, 25, 4069–4086 CrossRef CAS PubMed.
- Y. J. Oh, G. Choi, Y. B. Choy, J. W. Park, J. H. Park, H. J. Lee, Y. J. Yoon, H. C. Chang and J. H. Choy, Chemistry, 2013, 19, 4869–4875 CrossRef CAS PubMed.
- M. Baek, J. H. Choy and S. J. Choi, Int. J. Pharm., 2012, 425, 29–34 CrossRef CAS PubMed.
- L. A. de Sousa Rodrigues, A. Figueiras, F. Veiga, R. M. de Freitas, L. C. C. Nunes, E. C. da Silva Filho and C. M. da Silva Leite, Colloids Surf., B, 2013, 103, 642–651 CrossRef PubMed.
- S. G. Intasa-ard and M. Ogawa, Enzymes, 2018, 44, 117–136 Search PubMed.
- H. P. He, Y. H. Ma, J. X. Zhu, P. Yuan and Y. H. Qing, Appl. Clay Sci., 2010, 48, 67–72 CrossRef CAS.
- I. Pal Kaur and M. Kanwar, Drug Dev. Ind. Pharm., 2002, 28, 473–493 CrossRef PubMed.
-
J. Murube, A. Paterson and E. Murube, in Lacrimal gland, tear film, and dry eye syndromes 2, Springer, 1998, pp. 693–704 Search PubMed.
- H. Zhu and A. Chauhan, Curr. Eye Res., 2005, 30, 841–854 CrossRef PubMed.
- J. K. Park, Y. Bin Choy, J. M. Oh, J. Y. Kim, S. J. Hwang and J. H. Choy, Int. J. Pharm., 2008, 359, 198–204 CrossRef CAS PubMed.
- H. Jung, H. M. Kim, Y. B. Choy, S. J. Hwang and J. H. Choy, Appl. Clay Sci., 2008, 40, 99–107 CrossRef CAS.
- H. Jung, H. M. Kim, Y. Bin Choy, S. J. Hwang and J. H. Choy, Int. J. Pharm., 2008, 349, 283–290 CrossRef CAS PubMed.
- J. H. Yang, Y. S. Han, M. Park, T. Park, S. J. Hwang and J. H. Choy, Chem. Mater., 2007, 19, 2679–2685 CrossRef CAS.
-
U. Pharmacopeia, The Standard. USA: US Pharmacopeia 1995, 2002.
- C. G. Park, M. J. Kim, M. Park, S. Y. Choi, S. H. Lee, J. E. Lee, G. S. Shin, K. H. Park and Y. B. Choy, Acta Biomater., 2014, 10, 77–86 CrossRef CAS PubMed.
- T. R. Walters, Surv. Ophthalmol., 1996, 41, S19–S26 CrossRef PubMed.
- C. G. Park, Y. K. Kim, S. N. Kim, S. H. Lee, B. R. Huh, M. A. Park, H. Won, K. H. Park and Y. B. Choy, Int. J. Pharm., 2017, 522, 66–73 CrossRef CAS PubMed.
- J. Kabilaphat, N. Khaorapapong, K. Saito and M. Ogawa, Appl. Clay Sci., 2015, 115, 248–253 CrossRef CAS.
- M. D. Alba Carranza, A. Cota Reguero, F. J. Osuna Barroso, E. Pavón González, A. C. Perdigón Aller and F. Raffin, Sci. Rep., 2019, 9, 10265 CrossRef PubMed.
- E. Padilla-Ortega, M. Darder, P. Aranda, R. F. Gouveia, R. Leyva-Ramos and E. Ruiz-Hitzky, Appl. Clay Sci., 2016, 130, 40–49 CrossRef CAS.
- J. H. Lee, G. Choi, Y. J. Oh, J. W. Park, Y. Bin Choy, M. C. Park, Y. J. Yoon, H. J. Lee, H. C. Chang and J. H. Choy, Int. J. Nanomed., 2012, 7, 1635–1649 CAS.
- J. Kabilaphat, N. Khaorapapong, A. Ontam and M. Ogawa, Eur. J. Inorg. Chem., 2015, 1631–1637 CrossRef CAS.
- M. H. Aburahma and A. A. Mahmoud, AAPS PharmSciTech, 2011, 12, 1335–1347 CrossRef CAS PubMed.
- P. Komadel, J. Bujdak, J. Madejova, V. Sucha and F. Elsass, Clay Miner., 1996, 31, 333–345 CrossRef CAS.
- A. Nasser, M. Gal, Z. Gerstl, U. Mingelgrin and S. Yariv, J. Therm. Anal., 1997, 50, 257–268 CrossRef CAS.
- N. Meng, N.-L. Zhou, S.-Q. Zhang and J. Shen, Int. J. Pharm., 2009, 382, 45–49 CrossRef CAS PubMed.
- P. Garcia-Guzman, L. Medina-Torres, F. Calderas, M. J. Bernad-Bernad, J. Gracia-Mora, X. Marcos, J. Correa-Basurto, D. M. Nunez-Ramirez and O. Manero, Appl. Clay Sci., 2019, 180, 105202 CrossRef CAS.
- I. Salcedo, C. Aguzzi, G. Sandri, M. C. Bonferoni, M. Mori, P. Cerezo, R. Sánchez, C. Viseras and C. Caramella, Appl. Clay Sci., 2012, 55, 131–137 CrossRef CAS.
- T. Meng, V. Kulkarni, R. Simmers, V. Brar and Q. G. Xu, Drug Discovery Today, 2019, 24, 1524–1538 CrossRef CAS PubMed.
- A. Urtti, Adv. Drug Delivery Rev., 2006, 58, 1131–1135 CrossRef CAS PubMed.
- C. G. Park, K. Choi, Y. K. Kim, K. H. Park, S. Kim and Y. B. Choy, J. Ind. Eng. Chem., 2016, 39, 194–202 CrossRef CAS.
-
D. W. Ball, J. W. Hill and R. J. Scott, The basics of general, organic, and biological chemistry, Open Textbook Library, 2011 Search PubMed.
- W. S. Yun, S. Aryal, Y. J. Ahn, Y. J. Seo and J. Key, Biomed. Eng. Lett., 2020, 1–15 Search PubMed.
- A. Zimmer and J. Kreuter, Adv. Drug Delivery Rev., 1995, 16, 61–73 CrossRef CAS.
- M. Sarmah, N. Banik, A. Hussain, A. Ramteke, H. K. Sharma and T. K. Maji, J. Mater. Sci., 2015, 50, 7303–7313 CrossRef CAS.
- Y. Sultana, R. Jain, M. Aqil and A. Ali, Curr. Drug Delivery, 2006, 3, 207 CrossRef CAS PubMed.
- H. Gupta, M. Aqil, R. K. Khar, A. Ali, A. Bhatnagar and G. Mittal, Nanomedicine, 2010, 6, 324–333 CrossRef CAS PubMed.
Footnotes |
† Electronic supplementary information (ESI) available: Fig. S1: Representative image of the rabbit eye. See DOI: 10.1039/d0tb01213k |
‡ Chun Gwon Park, Goeun Choi, and Myung Hun Kim contributed equally to this work as first authors. |
|
This journal is © The Royal Society of Chemistry 2020 |