DOI:
10.1039/C9FO01844A
(Review Article)
Food Funct., 2020,
11, 32-44
Effects of short-term consumption of strawberry powder on select parameters of vascular health in adolescent males
Received
12th August 2019
, Accepted 9th December 2019
First published on 12th December 2019
Abstract
Cardiovascular disease is a leading cause of death in the United States and much of the developed world, costing billions of dollars in lost work time, lower productivity and high health care expenditures. Research on foods and bioactive food components that have cardioprotective benefits may provide new insights as to how modest changes in one's diet may result in a reduced risk of vascular disease. In intervention trials, the consumption of strawberries, either fresh or freeze-dried, has been reported to improve select markers of cardiovascular health, including improved lipid profiles, microvascular function, and platelet reactivity. Consistent with the above, epidemiological studies suggest beneficial effects of strawberries on vascular function. Preliminary studies on the effects of freeze-dried strawberry powder on vascular health are reviewed in the current paper.
Cardiovascular disease and childhood obesity
A dramatic increase in the prevalence of childhood (ages 2–19) obesity, defined as age and sex adjusted body mass index at or above the 95th percentile, has been noted in the US.1 Similar to adults, obesity in children impacts all major organ systems and increases the risk of morbidity and mortality.2,3 Compared to normal weight children, those who are obese are more likely to endure adverse health effects, including an increased prevalence of cardiovascular risk factors such as insulin resistance, hypertension, hyperlipidemia, and endothelial dysfunction4–11 that persist into adulthood.3,12,13 In response to the above, the American Heart Association has proposed targeting children, adolescents, and young adults in pursuit of their 2020 Impact Goal of reducing deaths attributable to vascular disease and stroke by 20%.14
Cardiovascular disease (CVD) is the number one cause of death in the US.14 By 2030, an estimated 44% of the US population is projected to suffer from CVD, resulting in annual costs in excess of $350 billion.14,15 Risk factors for CVD are divided into lifestyle factors, including smoking, lack of physical activity, poor diet and excess body fat14 and clinical factors, including high cholesterol, high blood pressure, and poor glucose control.14 Atherosclerotic CVD is characterized as a chronic inflammatory disease and disordered lipid metabolism initiated by endothelial dysfunction and promoted by a number of cell types such as platelets.16–18 Vascular homeostasis is maintained in part by the vasodilators nitric oxide (NO), prostacyclin, endothelial derived hyperpolarizing factors (EDHF), and vasoconstrictors such as thromboxane and endothelin.19,20 These mediators also help regulate both smooth muscle cell proliferation, inflammation and platelet activation.21–23 Endothelial dysfunction describes the partial or total loss of balance and regulatory function between vasoconstrictors and vasodilators, growth promoting and inhibiting factors, and pro- and anti-atherogenic factors.21 Atherosclerotic CVD is generally characterized as a disease of adulthood, however, fatty streak lesions that are in part secondary to maternal hypercholesterolemia can be detected in fetuses and young children.21–23
Apart from maternal factors, poor diet, low physical activity and obesity can increase the development of cardiometabolic disease throughout the life span.24–26 In addition, micronutrient deficiencies that are associated with under- and over-nutrition can perturb growth, and promote inflammation and infection that can increase chronic disease risk.27,28 For example, in overweight and obese children, increased inflammation is associated with reduced iron status,29–32 with hypoferremia potentially mediated through the induction of iron regulators such as hepcidin.33 Lower socioeconomic status is also associated with increased childhood obesity, increased infection and lower vascular function in adulthood.28 Therefore, weight control coupled with improvements in dietary patterns at an early age are key to reducing the risk for chronic disease development. Currently, dietary patterns that are high in the intake of plant foods, similar to a Mediterranean dietary pattern, are recommended for the prevention of CVD.34 These dietary patterns are essential nutrient rich, providing vitamins, minerals, fiber, fats and polyphenols that either alone or through their interactive effects can be of benefit towards cardiovascular health in both children and adults. Therefore, understanding how specific foods may be of benefit can provide further insight for future refinements of dietary recommendations. The following review will focus on strawberries as a potential “vascular healthy” food in overweight adolescents.
Methodologies to assess vascular health in children
As an early step in the atherosclerotic process, assessing vascular function in children is useful to identify those who may be at increased risk.7,35,36 These measures are considered physiologically relevant towards future disease development, and are appropriate for use towards dietary health claims.37 Endothelial dysfunction is commonly assessed using the non-invasive ultrasound technique, flow-mediated dilation (FMD).9,38 This technique measures endothelium-dependent dilation of the brachial artery, a large conduit vessel, in response to shear stress induction of NO after reactive hyperemia,38,39 and serves as an indicator of endothelial function.38,39 Nitric oxide-evoked vasodilation plays a critical role in the control of vascular function through the initiation of vascular smooth muscle relaxation by a cascade of steps that leads to reductions in intracellular calcium.40 Peripheral arterial tonometry (PAT) measures digital arterial pulse wave amplitude in the microvasculature.41,42 A reactive hyperemia index (RHI) can be calculated after endothelium-mediated changes in microvessel tone is elicited with hyperemia.42 Given the substantial cross-talk between the endothelium and smooth muscle, measurements of arterial stiffness using either pulse wave velocity (PWV) or the augmentation index (AIx) are also useful assessments of vascular function in children.36,43,44 The above techniques are associated with cardiovascular risk factor burden,36,43,45–47 but are mechanistically distinct. For example, under standard conditions, FMD is predominately NO-dependent,48 while PAT is only partially NO mediated, due to the additional influence of circulating metabolites that are potential EDHFs.49,50
When assessing endothelial function in children, variability in time to peak response, vessel size, and pubertal maturation needs to be considered.7,35 The peak vascular response to reactive hyperemia can occur later in children compared to adults.7,35 For FMD, the brachial artery diameter may need to be measured several times over a 120 seconds reactive hyperemia interval or calculated as the area under the curve in order to account for the later peak response time.7,51 Whether similar calculations are needed for RHI are subject to debate.51 Pubertal and hormonal changes that occur in childhood and adolescence also impact measures of vascular function.52 Pubertal status significantly correlates with RHI and lower RHI values in younger or prepubertal groups may reflect immature vascular function rather than dysfunction.51 The RHI increases during puberty in both sexes as reproductive hormones upregulate NO synthase and activity.52 The phase of the menstrual cycle also needs to be considered.35 Sex differences in measures of vascular function are well-recognized.53–55 Such variability can be partially attributed to the smaller size of the heart and major blood vessels of females compared to males of the same age and race, as well as body height.56,57
Potential benefits of strawberry intake for cardiovascular health
Current dietary guidelines stress the importance of a healthful dietary pattern abundant in fruits, vegetables, whole grains, low-fat or nonfat dairy, seafood, legumes, and nuts, with only modest intakes of red meat, refined grains and added sugars.58 The vascular benefits of plant-based whole foods such as fruits and vegetables can be attributed, in part, to their high content of vitamins, minerals, fiber, and a diversity of bioactive compounds such as polyphenols.59 An increased intake of polyphenols has been associated with decreased risk for CVD,60–62 improved endothelial function and reduced platelet reactivity.60,63–65
Strawberries are rich in polyphenolic compounds, including anthocyanins, flavanols, flavonols, ellagic acid (EA), and ellagitannin (ET),63,66 and can provide a source of dietary nitrate.67,68 Dietary nitrate has been shown to induce positive changes to vascular health69 through its conversion to nitrite and NO, which in turn can induce vasodilation and inhibit platelet aggregation.69 Strawberries also provide an array of vitamins, minerals and fiber, whose health benefits are well-recognized.59 Epidemiological studies suggest that high anthocyanin intakes (16–22 mg day−1) provided from strawberries and blueberries are associated with an eight percent lowered risk of hypertension and reduced CVD mortality.70 Several dietary interventions support the concept that strawberry consumption has favorable effects on vascular outcomes attributed to improved endothelial function and plasma lipid profiles, and inhibition of platelet aggregation, lipid peroxidation, and inflammatory responses (Table 1). Other studies report no apparent effects.72 This may be due to differences in study design and population, or inherent variability in metabolic response. It is also important to note that the outlined trials were mostly conducted in adults, while only limited information is available for children and adolescents.
Table 1 Dietary strawberry intake and cardiovascular surrogate outcomesa
Type |
Quantity |
Study duration |
Subject characteristics (mean age) |
n
|
Response |
WS: whole strawberries; FDSP: freeze-dried strawberry powder; MetS: metabolic syndrome; PP: postprandial; HFM: high-fat meal; g: grams; MDA: malondialdehyde; TBARS: thiobarbituric acid reactive substances; TC: total cholesterol; HDL: high-density lipoprotein; LDL: low-density lipoprotein, OxLDL: oxidized low-density lipoprotein; TG: triglycerides; CRP: C-reactive protein; hs-CRP: high-sensitivity C-reactive protein; 8-OHdG: 8-hydroxy-2′-deoxyguanosine; VCAM-1: vascular adhesion molecule-1; HbA1C: hemoglobin A1C (glycated hemoglobin); SBP: systolic blood pressure; PWV: pulse wave velocity; NOX2: NADPH oxidase 2; IKK: inhibitory κB kinase; IL-6: interleukin 6; IL-1B: interleukin 1 beta; PAI-1: plasminogen activator inhibitor-1. Includes human clinical trials of known physiologically relevant measures related to cardiovascular function. Therefore, excludes studies of antioxidant capacity that are not direct measures of known oxidant products where the relationship to physiology and disease development has been established. Also, excludes studies where either the amount or fresh strawberries or FDSP intake was not clearly defined. |
WS |
454 g per 2000 kcal per day |
4 weeks |
Hyperlipidemic adults (62 years) |
28 |
↓ TBARS, ↑ protein thiols |
No effect on plasma lipids72 |
FDSP |
25 g |
4 weeks |
Females with MetS (51 years) |
16 |
↓ TC, LDL, MDA73 |
FDSP |
50 g |
8 weeks |
Obese adults with MetS (47 years) |
27 |
↓ TC, LDL, small LDL particles, VCAM-1 |
No effect on TG, HDL65 |
FDSP |
10 g |
6 weeks + PP with HFM |
Hyperlipidemic adults (51 years) |
24 |
Acute: ↓ TG, LDL, HDL, OxLDL |
Chronic: ↓ TC, LDL, HDL, TG74 |
FDSP |
10 g |
PP with HFM |
Overweight adults (51 years) |
24 |
↓ IL-6, hsCRP, insulin75 |
FDSP |
10 g |
6 weeks + PP with HFM |
Overweight adults (51 years) |
24 |
↓ PAI-I, IL-1b76 |
FDSP |
320 g |
3 weeks |
Obese adults (30 years) |
20 |
↓ Total cholesterol and small HDL particles |
↑ LDL particle size77 |
FDSP |
50 g |
6 weeks |
Adults with type 2 diabetes mellitus (52 years) |
36 |
↓ CRP, MDA, HbA1C78 |
WS |
500 g |
4 weeks |
Healthy adults (27 years) |
23 |
↓ TC, LDL, TG, MDA, 8-OHdG, isoprostanes79 |
FDSP |
25 g |
12 weeks |
Adults with abdominal adiposity hyperlipidemia (49 years) |
60 |
50 g: ↓ TC, LDL, small LDL particles |
50 g |
25 & 50 g: ↓ MDA, no effect on HDL, TG, CRP or adhesion molecules80 |
FDSP |
50 g |
1 week |
Overweight and obese adolescent males (16 years) |
25 |
↑ Microvascular function related to plasma nitrate/nitrite71 |
FDSP |
40 g |
4 weeks + PP |
Overweight and obese adults (28 years) |
30 |
No effect on arterial stiffness or plasma lipids81 |
FDSP |
25 g |
8 weeks |
Stage 1 hypertensive, postmenopausal females (60 years) |
60 |
Low dose: ↓ SBP, PWV |
50 g |
High dose: ↑ nitrate/nitrite82 |
Effects of a freeze-dried strawberry powder on parameters of vascular health in adolescent males: a randomized trial
Given the dearth of information on strawberries and vascular health in children, we conducted a randomized, controlled, double-blind, crossover trial to assess whether the acute (one hour) or short-term (one week) consumption of a freeze-dried strawberry powder (FDSP) can influence vascular health in adolescents. The children were at increased cardiovascular risk due to their elevated adiposity (>75th percentile for age and sex). We hypothesized that vascular function would increase following the intake of FDSP compared to a control polyphenol-free power. Microvascular function as measured by PAT, platelet reactivity, and plasma nitrate and nitrite (nitrate/nitrite) concentrations were assessed before and after the one-week daily consumption of 50 grams of FDSP or an isocaloric, macronutrient-matched control powder that was devoid of polyphenols (Table 2; Fig. 1). The powders were divided into two 25 g servings. The children were instructed to mix the powder in water, consuming one packet at breakfast and the other at dinner.
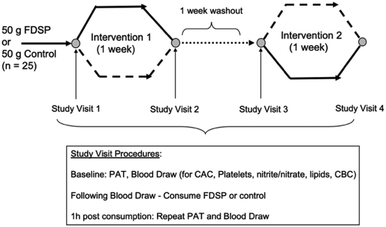 |
| Fig. 1 Study design and visit procedures.71 | |
Table 2 FDSP composition per 50 grams
|
FDSP |
Control powder |
Calories (kcal) |
180 |
180 |
Fructose (g) |
11 |
12 |
Glucose (g) |
10.3 |
9 |
Sucrose (g) |
7.2 |
10 |
Total sugar (g) |
28.4 |
30.5 |
Carbohydrate (g) |
39 |
42 |
Protein (g) |
3.2 |
0 |
Total dietary fiber (g) |
8.1 |
4.3 |
Potassium (mg) |
839 |
350 |
Twenty-five adolescent (aged 14–18 years) males were enrolled into the trial. The mean age was 16 years, and the participants on average were healthy, with normal blood pressures, fasting lipid profiles and fasting blood glucose levels.
The composition of both FDSP and control powder is described in Table 2, with the polyphenolic content outlined in Table 3. Fifty grams of FDSP is equivalent to approximately three cups (450 g) of whole strawberries. The control powder also contained dietary fiber and potassium that may provide some benefit towards vascular health and therefore should not be considered as a true “placebo”. The isoenergetically matched powders were produced and provided by the California Strawberry Commission.
Table 3 FDSP polyphenol content per 50 grams
Polyphenol |
Content (mg) |
Pelargonidin-3-glucoside |
198.5 |
Procyanidin B1 |
15.31 |
(+)Catechin |
12.52 |
Ellagic acid |
6.30 |
Cyanidin-3-glucoside |
5.82 |
Isoquercetin |
3.31 |
Rutin |
1.68 |
Quercetin |
0.73 |
Tiliroside |
0.37 |
Gallic acid |
0.2 |
Sinapic acid |
0.2 |
Kaemferol |
0.18 |
p-Coumaric acid |
0.13 |
2-Hydroxycinnamic acid |
0.1 |
3,4-Dihycrobenzoic acid |
0.08 |
Syringic acid |
0.01 |
Peripheral arterial tonometry was assessed after an overnight fast and one hour after the intake of the assigned powder. The measurement provided the data for the parameters: (1) RHI, (2) Framingham RHI (fRHI), an index associated with cardiovascular risk factors,45 (3) AIx, (4) platelet reactivity assessed by flow cytometry, and (5) total plasma nitrate/nitrite. An initial analysis demonstrated that neither short-term (seven-day) nor acute FDSP intake improved microvascular function (Fig. 2) when assessed as a group. Likewise, no significant differences were observed in blood pressure, plasma lipids, or platelet reactivity.71 Compliance with daily powder intake was difficult to assess as very few children returned their packaging as requested.
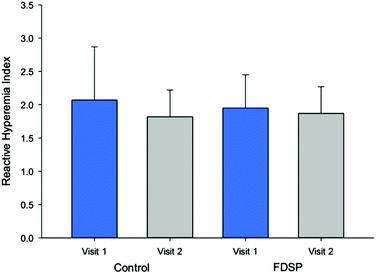 |
| Fig. 2 Reactive hyperemia index with short-term consumption of FDSP vs. control powder. | |
A significant increase in total plasma nitrate/nitrite levels was observed one hour after the intake of FDSP compared to control powder intake.71 However, total fasting plasma nitrate/nitrite was not significantly changed with FDSP compared to control powder after one week of intake. Based on the results of the acute intake, and the observation that strawberries are considered a source of dietary nitrate67 with a half-life of five to eight hours,83 a subset analysis was conducted between those who had an increase in the one week change in total plasma nitrate/nitrite compared to control powder intake (“Responder”), and those who did not (“Non-Responder”; Fig. 3, left panel). Among children who had a significant increase in circulating nitrate/nitrite levels, an increase in both RHI (Fig. 3, right panel) and fRHI was observed, while those showing no detectable increases in plasma nitrate/nitrite had no improvement in vascular function.71
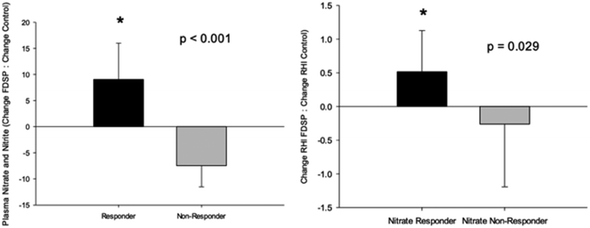 |
| Fig. 3 Individuals who had an increase in fasting plasma nitrate and nitrite levels with one week of FDSP intake relative to control powder intake (left panel) also had an improved vascular function response as measured by the reactive hyperemia index (RHI; right panel). | |
Potential mechanisms
Nitrate/nitrate response
The above results demonstrate an improvement in vascular function after FDSP intake by overweight adolescents that is associated with plasma nitrate levels. Our findings are in agreement with Feresin et al. who reported increased plasma levels of nitrate/nitrite after short-term (four and eight weeks) FDSP intake (50 g day−1).82 Dietary nitrate has gained interest as a potentially bioavailable supply of NO, with green leafy vegetables as a predominate source.83 Nitrate is reduced to nitrite by commensal bacteria in the oral cavity, and further reduced to NO via numerous pathways that involve polyphenols, vitamin C, deoxygenated myoglobin, xanthine oxidoreductase, and deoxygenated haemoglobin (Fig. 4).84 The potential production of NO through oxygen-independent means is of particular importance during tissue ischemia and exercise, which are situations of reduced blood flow and increased tissue oxygen demand.85 Indeed, circulating nitrate/nitrite levels have been positively associated with FMD response,86,87 and the intake of nitrate from beetroot juice has been observed to reduce blood pressure and improve vascular function in healthy adults.83,87,88
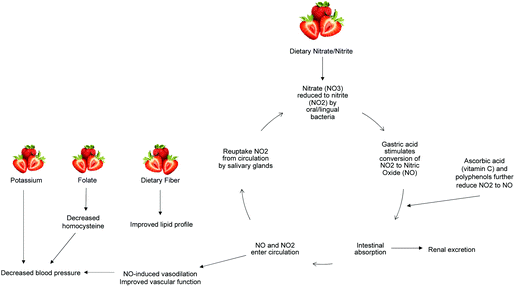 |
| Fig. 4 Potential mechanisms modulating the vascular effects of strawberry consumption. NO3: nitrate; NO2: nitrite; NO: nitric oxide. | |
Rodriguez-Mateos and colleagues demonstrated the potential interactive effects of nitrate-containing foods with polyphenol-rich foods.89 Nitrate intake enhanced the FMD response to cocoa flavanol intake.89 Importantly, the amount of nitrate provided was similar to the potential intake from typical amounts of leafy greens.89 Interestingly, the intake of both flavanols and nitrate together, but not separately, increased the stomach production of NO,89 which is in agreement with other trials.90 As will be discussed in the following sections, strawberries can provide a substantial amount of both vitamin C and polyphenols, both with the potential to reduce nitrate to bioactive NO. The potential interactive effects these components may elicit on the vascular response should be explored further.
Strawberry polyphenols
The FDSP products for the forementioned trial were particularly rich in the anthocyanin pelargonidin-3-glucoside (P3G), with a single daily intake providing approximately 200 mg, which is 13 to 15-fold higher than that of the flavan-3-ols procyanidin B1 and catechin, respectively. The FDSP intake also provided 6 mg of the anthocyanin cyanidin-3-glucoside. In addition to flavonoids, the FDSP provided phenolic and hydroxycinnamic acids (Table 3).
Anthocyanins are glycosylated flavonoids that, depending on the pH, provide red, purple, and blue pigmentation to berries, grapes and flowers.91 Following dietary intake, anthocyanins are present in the circulation as conjugated aglycones and aglycone glycosides or degraded to phenolic acids and aldehydes.92,93 Within an hour of FDSP intake, P3G is predominately converted to its glucuronide form (pelargonidin-O-glucuronide), with considerably lower circulating amounts of P3G and pelargonidin-3-rutinoside, along with several phenolic acids and aldehydes, including hippuric acid, 3,4-dihydroxybenzaldehyde, 4-hydroxybenzaldehyde, p-coumaric, and 3-hydroxybenzoic acid.93–95
Numerous investigators have utilized in vitro systems to investigate the role of strawberry polyphenols on a number of parameters. However, it should be noted that positive effects have been predominately observed with the anthocyanidin pelargonidin and not the specific anthocyanins that are found within strawberry or its’ physiologically relevant metabolites. Amini et al. observed that in vitro administration of 0.08 μmol L−1 of P3G extract to stimulated human whole blood significantly increased the concentration of interleukin-10, an anti-inflammatory cytokine.96 Pelargonidin, but not P3G, increased clotting time (prothrombin time) and reduced the ratio of plasminogen activator inhibitor (PAI-1): tissue plasminogen activator (t-PA) in human umbilical vein endothelial cells.97 In isolated rat aorta, pelargonidin inhibited thromboxane induced vasoconstriction in an endothelium-independent fashion,98 while cyanidin-3-glycoside enhanced endothelial nitric oxide synthase expression in bovine artery endothelial cells in a dose-and time-dependent manner, with the highest response observed at 0.1 μmol L−1.99 While the above in vitro work is promising, data from dietary interventions that specifically examine the association between circulating strawberry polyphenol or phenolic metabolites and physiological effects have yet to be established. However, in a diabetic mouse model, the addition of serum providing strawberry metabolites attenuated endothelial dysfunction and markers of inflammation.100 In addition, other anthocyanin-rich foods such as blueberries, have demonstrated improvements in vascular function that are associated with the presence of a number of circulating phenolic and aromatic acids.101
Fifty grams of the FDSP used in the above trial provided 6.3 micrograms of EA (Table 3). Ellagitannins are another potential source of bioactive polyphenols102 that are hydrolyzed in the gastrointestinal tract to EA, and further metabolized by the gut microbiota to urolithins.103 In animal models, the addition of polyphenolic-rich strawberry extracts to the diet beneficially affects colonic enzymes and improves gut dysbiosis, while increasing short chain fatty acid production.104–106 The influence of strawberry polyphenols on microbial derived metabolites are of considerable interest as they can influence cellular signalling and physiological response both locally in the gastrointestinal tract and systemically after absorption. Short chain fatty acids have received considerable interest as potential blood pressure regulators.107 Urolithins have been observed to reduce oxidative damage108,109 and inflammation.102,108 Finally, dietary nitrate can be reduced by the gut microbiota to bioactive NO and as we have demonstrated above, can be associated with improved vascular response.71
Other cardioprotective nutrients
Beyond polyphenols and nitrate, strawberries contain additional vasculoprotective nutrients, such as folate, vitamin C, potassium, and dietary fiber (Table 4). When folate levels are low, the conversion of homocysteine to methionine is decreased and homocysteine levels rise.110 Elevated serum homocysteine has been associated with increased vascular disease risk.111,112
Table 4 Vitamins, minerals and fiber provided in 39 g of FDSP (2018 formulation provided by the California Strawberry Commission)
|
Content |
% RDA |
Folic acid (mcg) |
108 |
27 |
Pantothenic acid (mcg) |
0.35 |
7 |
Thiamin (mg) |
0.02 |
5 |
Niacin (mg) |
1.83 |
11 |
Vitamin C (mg) |
154 |
171 |
Potassium (mg) |
659 |
19 |
Dietary fiber (g) |
5.1 |
20 |
Low levels of vitamin C are associated with vascular disease.113 Thirty-nine grams of FDSP provides 171% of the Recommended Dietary Allowance for vitamin C. Plasma levels of vitamin C are significantly increased two to four hours after the intake of 300 g of fresh strawberries.114 Vitamin C scavenges free radicals, and protects lipoproteins from oxidative damage.115 In addition, vitamin C has been shown to improve both arterial stiffness and endothelial function.116,117
Potassium influences vascular disease risk through its critical role in blood pressure regulation.118,119 Dietary fiber has the ability to bind cholesterol and increase its’ excretion, lowering circulating cholesterol levels.120,121 This allows for less lipoprotein to be susceptible to oxidation and atherogenesis.122
Research considerations when evaluating the potential health effects of strawberries
A number of variables exist that could contribute to differential findings with products such as FDSP. Examples of these follow.
Metabotype
Recent studies have demonstrated a relationship between urolithin metabotype (UM) and cardiovascular risk factors.123,124 Three UMs have been identified: Metabotype A (urolithin-A producing), Metabotype B (urolithin-A and/or -B producing), and Metabotype 0 (not urolithin-producing).125 Studies suggest that UM-A is cardioprotective, while UM-B may be associated with gut dysbiosis and disease.123,125 Correlations between UM and cardiometabolic risk factors in individuals have been reported in overweight or obese adults;89 information about adolescents and children is currently lacking. UM-A has been correlated with levels of apolipoprotein A and high-density lipoprotein-cholesterol (HDL), while UM-B has been associated with apolipoprotein B, total cholesterol, very low density lipoprotein (VLDL)-cholesterol, low density lipoprotein (LDL)-cholesterol, and oxidized LDL-cholesterol.89 These patterns may be significant in that apolipoprotein A and HDL are associated with decreased cardiovascular risk, while apolipoprotein B and its’ lipoproteins (LDL and VLDL) are associated with increased risk.126 Similar results of enhanced endothelial function have been reported following acute (three-day) consumption of ET and production of their microbial metabolites.124 Taken together, these results suggest that individuals who produce UM-B are at increased risk of CVD, whereas production of UM-A may confer vasculoprotective effects.89,124 Ideally, metabotypes should be considered in future studies that assess the potential benefits of ET-rich foods and cardiovascular health.
Food matrix
An important influence of the food matrix on the potential health benefits of strawberries is suggested by studies demonstrating a change in anthocyanin pharmacokinetics with the addition of food.95 While FDSP allows for a consistent and convenient supplementation across studies, it is important to note that the amount of P3G provided in 50 g of FDSP is about 12 times higher than the amount found in whole strawberries.127 Food processing, preservation, and storage conditions affect the stability and bioavailability of the active compounds in strawberries. For example, the freeze-drying process may denature anthocyanin content and reduce bioactivity.128 Juicing and preserving strawberry may increase the level of EA relative to fresh strawberry via hydrolysis.129 The conversion of ET to EA can be reduced by using low processing temperatures. Prolonged storage at low temperature can also enhance hydrolysis, although at a slower rate.129 Additional data are needed on the potential interactive effects between strawberry bioactives and other nutrients within a diet. The above highlights the need for more information on the influence of a complex diet on the potential health effects of strawberries. Such interactions can be positive as well as negative. The identification of positive interactions is particularly important as it may provide insight for the development of new vascular-health foods. Conversely, the identification of negative interactions may be of great value in the design of new food processing and handling techniques that can amplify the health effects of strawberries.
Appropriate controls for strawberry investigative trials
An inherent difficulty with most nutrition studies is the identification of appropriate controls or placebos. This is particularly difficult when examining the potential health effects of whole foods that contain a number of components that either on their own or through their interaction with each other are bioactive. With respect to strawberries, an attempt has been made to develop dietary powders that can be used in dietary intervention trials that are matched with respect to several nutrients excluding polyphenols and are thought to drive many of the health effects reported with strawberry intake. It is important to stress that the “control” powders used in such studies will likely underestimate the positive health effects that these foods can provide. Thus, the positive health effects observed with powders used in strawberry research are underestimates of the positive health effects of strawberry intake. Complimenting this caveat with respect to control food or diet, it is important to note that placebos can elicit biological effects, despite containing no pharmaceutical compounds or known bioactive components.130 Placebo treatments in randomized controlled clinical trials have demonstrated significant improvement in symptoms.131 These effects have been seen both with discrete and disclosed provision of placebos. Interestingly, positive effects seen with the provision of an “open-label placebo”, where participants are knowingly prescribed placebo treatment, still have been shown to evoke some level of response.130,132–134 The mechanisms behind these phenomena are not well understood, but are thought to include neurobiological and psychological mechanisms, classical conditioning, and simple hope for change.130,135
Summary
The literature to date strongly supports the concept that the regular consumption of strawberries can be associated with improvements in cardiovascular health. Dietary interventions that examine the influence of strawberry intake on measures of vascular function are currently limited, especially for longer periods of intake. Moreover, it is reasonable to further examine the health effects of strawberries in additional at-risk populations that include children and adults. Trial designs that capture the relationship between circulating strawberry-derived metabolites and physiologic response are desired. This would include studies that assess the effects of strawberry intake on vascular health in a variety of populations, as hormonal status, sex, age, genetic polymorphisms and microbial metabolism can affect polyphenol metabolism and ultimately cardiometabolic response.136 Current recommendations stress a dietary pattern that is high in plant foods. Therefore, a better understanding of the synergy between the diverse constituents of strawberries within the diet and their relationship to vascular health is desired, including a better appreciation of individual metabotypes in response to strawberry intake. The above information will better enable practical recommendations about the amount and frequency of strawberry intake to consume on a regular basis as part of a healthy vascular dietary pattern over the lifespan.
Conflicts of interest
There are no conflicts to declare.
Acknowledgements
Funding for this work was provided, in part, from grants from the California Strawberry Commission to CLK, RMH and RRH, the National Needs Fellowship Training Grants, “Childhood Obesity” (Grant No. 2018-38420-27959 and 2010-03341) to CLK and MZ, Agriculture and Food Research Initiative Competitive Grants (Grant # 2012-01370 and 2017-06986) to RRH, and a Royal Thai Government Scholarship to PC.
References
- C. M. Hales, C. D. Fryar, M. D. Carroll, D. S. Freedman and C. L. Ogden, Trends in Obesity and Severe Obesity Prevalence in US Youth and Adults by Sex and Age, 2007–2008 to 2015–2016, J. Am. Med. Assoc., 2018, 319, 1723–1725 CrossRef PubMed.
- M. Raj, Obesity and cardiovascular risk in children and adolescents, Indian J. Endocrinol. Metab., 2012, 16, 13–19 CrossRef PubMed.
- A. C. Skinner, E. M. Perrin, L. A. Moss and J. A. Skelton, Cardiometabolic Risks and Severity of Obesity in Children and Young Adults, N. Engl. J. Med., 2015, 373, 1307–1317 CrossRef PubMed.
- S. R. Daniels, M. S. Jacobson, B. W. McCrindle, R. H. Eckel and B. M. Sanner, American Heart Association Childhood Obesity Research Summit: executive summary, Circulation, 2009, 119, 2114–2123 CrossRef PubMed.
- S. S. Sun, R. Liang, T. T. Huang, S. R. Daniels, S. Arslanian, K. Liu, G. D. Grave and R. M. Siervogel, Childhood obesity predicts adult metabolic syndrome: the Fels Longitudinal Study, J. Pediatr., 2008, 152, 191–200 CrossRef PubMed.
- B. K. Kit, E. Kuklina, M. D. Carroll, Y. Ostchega, D. S. Freedman and C. L. Ogden, Prevalence of and trends in dyslipidemia and blood pressure among US children and adolescents, 1999–2012, JAMA Pediatr., 2015, 169, 272–279 CrossRef PubMed.
- M. J. Järvisalo, T. Rönnemaa, I. Volanen, T. Kaitosaari, K. Kallio, J. J. Hartiala, K. Irjala, J. S. Viikari, O. Simell and O. T. Raitakari, Brachial artery dilatation responses in healthy children and adolescents, Am. J. Physiol.: Heart Circ. Physiol., 2002, 282, H87–H92 CrossRef PubMed.
- P. Clarkson, D. S. Celermajer, A. J. Powe, A. E. Donald, R. Henry and J. E. Deanfield, Endothelium-Dependent Dilatation Is Impaired in Young Healthy Subjects With a Family History of Premature Coronary Disease, Circulation, 1997, 96, 3378–3383 CrossRef.
- D. S. Celermajer, K. E. Sorensen, V. M. Gooch, D. J. Spiegelhalter, O. I. Miller, I. D. Sullivan, J. K. Lloyd and J. E. Deanfield, Non-invasive detection of endothelial dysfunction in children and adults at risk of atherosclerosis, Lancet, 1992, 340, 1111–1115 CrossRef.
- P. Tounian, Y. Aggoun, B. Dubern, V. Varille, B. Guy-Grand, D. Sidi, J. P. Girardet and D. Bonnet, Presence of increased stiffness of the common carotid artery and endothelial dysfunction in severely obese children: a prospective study, Lancet, 2001, 358, 1400–1404 CrossRef.
- S. D. de Ferranti, K. Gauvreau, D. S. Ludwig, E. J. Neufeld, J. W. Newburger and N. Rifai, Prevalence of the metabolic syndrome in American adolescents: findings from the Third National Health and Nutrition Examination Survey, Circulation, 2004, 110, 2494–2497 CrossRef.
- A. Umer, G. A. Kelley, L. E. Cottrell, P. Giacobbi, K. E. Innes and C. L. Lilly, Childhood obesity and adult cardiovascular disease risk factors: a systematic review with meta-analysis, BMC Public Health, 2017, 17, 683 CrossRef PubMed.
- M. Juonala, C. G. Magnussen, G. S. Berenson, A. Venn, T. L. Burns, M. A. Sabin, S. R. Srinivasan, S. R. Daniels, P. H. Davis, W. Chen, C. Sun, M. Cheung, J. S. Viikari, T. Dwyer and O. T. Raitakari, Childhood adiposity, adult adiposity, and cardiovascular risk factors, N. Engl. J. Med., 2011, 365, 1876–1885 CrossRef.
- E. J. Benjamin, P. Muntner, A. Alonso, M. S. Bittencourt, C. W. Callaway, A. P. Carson, A. M. Chamberlain, A. R. Chang, S. Cheng, S. R. Das, F. N. Delling, L. Djousse, M. S. V. Elkind, J. F. Ferguson, M. Fornage, L. C. Jordan, S. S. Khan, B. M. Kissela, K. L. Knutson, T. W. Kwan, D. T. Lackland, T. T. Lewis, J. H. Lichtman, C. T. Longenecker, M. S. Loop, P. L. Lutsey, S. S. Martin, K. Matsushita, A. E. Moran, M. E. Mussolino, M. O'Flaherty, A. Pandey, A. M. Perak, W. D. Rosamond, G. A. Roth, U. K. A. Sampson, G. M. Satou, E. B. Schroeder, S. H. Shah, N. L. Spartano, A. Stokes, D. L. Tirschwell, C. W. Tsao, M. P. Turakhia, L. B. VanWagner, J. T. Wilkins, S. S. Wong, S. S. Virani and American Heart Association Council on Epidemiology and Prevention Statistics Committee and Stroke Statistics Subcommittee, Heart Disease and Stroke Statistics-2019 Update: A Report From the American Heart Association, Circulation, 2019, 139, e56–e528 CrossRef.
- D. Mozaffarian, E. J. Benjamin, A. S. Go, D. K. Arnett, M. J. Blaha, M. Cushman, S. R. Das, S. de Ferranti, J. P. Després, H. J. Fullerton, V. J. Howard, M. D. Huffman, C. R. Isasi, M. C. Jiménez, S. E. Judd, B. M. Kissela, J. H. Lichtman, L. D. Lisabeth, S. Liu, R. H. Mackey, D. J. Magid, D. K. McGuire, E. R. Mohler, C. S. Moy, P. Muntner, M. E. Mussolino, K. Nasir, R. W. Neumar, G. Nichol, L. Palaniappan, D. K. Pandey, M. J. Reeves, C. J. Rodriguez, W. Rosamond, P. D. Sorlie, J. Stein, A. Towfighi, T. N. Turan, S. S. Virani, D. Woo, R. W. Yeh, M. B. Turner, W. G. Members and American Heart Association Statistics Committee; Stroke Statistics Subcommittee, Executive Summary: Heart Disease and Stroke Statistics–2016 Update: A Report From the American Heart Association, Circulation, 2016, 133, 447–454 CrossRef.
-
M. Caudill and M. Stipanuk, Cholesterol and Lipoproteins: Synthesis, Transport, and Metabolism, Elsevier, 3rd edn, 2013, pp. 395–415 Search PubMed.
- G. R. Geovanini and P. Libby, Atherosclerosis and inflammation: overview and updates, Clin. Sci., 2018, 132, 1243–1252 CrossRef CAS.
- G. Davì and C. Patrono, Platelet activation and atherothrombosis, N. Engl. J. Med., 2007, 357, 2482–2494 CrossRef PubMed.
- M. P. Libby, M. P. Ridker and M. A. Maseri, Inflammation and Atherosclerosis, Circulation, 2002, 105, 1135–1143 CrossRef.
- H. Shimokawa, Primary Endothelial Dysfunction: Atherosclerosis, J. Mol. Cell Cardiol., 1999, 31, 23–37 CrossRef CAS PubMed.
- A. A. Quyyumi, Endothelial function in health and disease: new insights into the genesis of cardiovascular disease, Am. J. Med., 1998, 105, 32S–39S CrossRef CAS.
- F. de Nigris, F. Cacciatore, F. P. Mancini, D. F. Vitale, G. Mansueto, F. P. D'Armiento, C. Schiano, A. Soricelli and C. Napoli, Epigenetic Hallmarks of Fetal Early Atherosclerotic Lesions in Humans, JAMA Cardiol., 2018, 3(12), 1184–1191 CrossRef.
- C. Napoli, C. K. Glass, J. L. Witztum, R. Deutsch, F. P. D'Armiento and W. Palinski, Influence of maternal hypercholesterolaemia during pregnancy on progression of early atherosclerotic lesions in childhood: Fate of Early Lesions in Children (FELIC) study, Lancet, 1999, 354, 1234–1241 CrossRef CAS.
- M. Juonala, J. S. Viikari and O. T. Raitakari, Main findings from the prospective Cardiovascular Risk in Young Finns Study, Curr. Opin. Lipidol., 2013, 24, 57–64 CrossRef CAS.
- J. Steinberger, S. R. Daniels, N. Hagberg, C. R. Isasi, A. S. Kelly, D. Lloyd-Jones, R. R. Pate, C. Pratt, C. M. Shay, J. A. Towbin, E. Urbina, L. V. Van Horn and J. P. Zachariah, American Heart Association Atherosclerosis, Hypertension, and Obesity in the Young Committee of the Council on Cardiovascular Disease in the Young, Council on Cardiovascular and Stroke Nursing, Council on Epidemiology and Prevention, Council on Functional Genomics and Translational Biology and Stroke Council, Cardiovascular Health Promotion in Children: Challenges and Opportunities for 2020 and Beyond: A Scientific Statement From the American Heart Association, Circulation, 2016, 134, e236–e255 CrossRef.
- J. A. Morrison, L. A. Friedman, P. Wang and C. J. Glueck, Metabolic syndrome in childhood predicts adult metabolic syndrome and type 2 diabetes mellitus 25 to 30 years later, J. Pediatr., 2008, 152, 201–206 CrossRef CAS PubMed.
- R. L. Guerrant, M. D. DeBoer, S. R. Moore, R. J. Scharf and A. A. Lima, The impoverished gut–a triple burden of diarrhoea, stunting and chronic disease, Nat. Rev. Gastroenterol. Hepatol., 2013, 10, 220–229 CrossRef PubMed.
- R. S. Liu, D. P. Burgner, M. A. Sabin, C. G. Magnussen, M. Cheung, N. Hutri-Kähönen, M. Kähönen, T. Lehtimäki, E. Jokinen, T. Laitinen, L. Taittonen, T. Dwyer, J. S. Viikari, M. Kivimäki, O. T. Raitakari and M. Juonala, Childhood Infections, Socioeconomic Status, and Adult Cardiometabolic Risk, Pediatrics, 2016, 137(6), e20160236 CrossRef.
- L. B. Yanoff, C. M. Menzie, B. Denkinger, N. G. Sebring, T. McHugh, A. T. Remaley and J. A. Yanovski, Inflammation and iron deficiency in the hypoferremia of obesity, Int. J. Obes., 2007, 31, 1412–1419 CrossRef CAS.
- O. Pinhas-Hamiel, R. S. Newfield, I. Koren, A. Agmon, P. Lilos and M. Phillip, Greater prevalence of iron deficiency in overweight and obese children and adolescents, Int. J. Obes., 2003, 27, 416 CrossRef CAS.
- I. Aeberli, R. F. Hurrell and M. B. Zimmermann, Overweight children have higher circulating hepcidin concentrations and lower iron status but have dietary iron intakes and bioavailability comparable with normal weight children, Int. J. Obes., 2009, 33, 1111–1117 CrossRef CAS PubMed.
- M. C. Dao and S. N. Meydani, Iron biology, immunology, aging, and obesity: four fields connected by the small peptide hormone hepcidin, Adv. Nutr., 2013, 4, 602–617 CrossRef CAS.
- V. Sangkhae and E. Nemeth, Regulation of the Iron Homeostatic Hormone Hepcidin, Adv. Nutr., 2017, 8, 126–136 CrossRef CAS.
- D. Mozaffarian, Dietary and Policy Priorities for Cardiovascular Disease, Diabetes, and Obesity: A Comprehensive Review, Circulation, 2016, 133, 187–225 CrossRef CAS.
- L. Bruyndonckx, T. Radtke, P. Eser, C. J. Vrints, J. Ramet, M. Wilhelm and V. M. Conraads, Methodological considerations and practical recommendations for the application of peripheral arterial tonometry in children and adolescents, Int. J. Cardiol., 2013, 168, 3183–3190 CrossRef.
- A. T. Cote, A. A. Phillips, K. C. Harris, G. G. Sandor, C. Panagiotopoulos and A. M. Devlin, Obesity and arterial stiffness in children: systematic review and meta-analysis, Arterioscler., Thromb., Vasc. Biol., 2015, 35, 1038–1044 CrossRef CAS.
- D. Turck, J. L. Bresson, B. Burlingame, T. Dean, S. Fairweather-Tait, M. Heinonen, K. I. Hirsch-Ernst, I. Mangelsdorf, H. J. McArdle, A. Naska, M. Neuhäuser-Berthold, G. Nowicka, K. Pentieva, Y. Sanz, A. Sjödin, M. Stern, D. Tomé, H. Van Loveren, M. Vinceti, P. Willatts, A. Martin, J. J. Strain, L. Heng, S. Valtueña Martínez and A. Siani, Guidance for the scientific requirements for health claims related to antioxidants, oxidative damage and cardiovascular health, EFSA J., 2018, 16(1), 5136 Search PubMed.
- M. C. Corretti, T. J. Anderson, E. J. Benjamin, D. Celermajer, F. Charbonneau, M. A. Creager, J. Deanfield, H. Drexler, M. Gerhard-Herman, D. Herrington, P. Vallance, J. Vita and R. Vogel, Guidelines for the ultrasound assessment of endothelial-dependent flow-mediated vasodilation of the brachial artery: A report of the International Brachial Artery Reactivity Task Force: A report of the International Brachial Artery Reactivity Task Force, J. Am. Coll. Cardiol., 2002, 39, 257–265 CrossRef.
- M. E. Widlansky, N. Gokce, J. F. Keaney and J. A. Vita, The clinical implications of endothelial dysfunction, J. Am. Coll. Cardiol., 2003, 42, 1149–1160 CrossRef CAS.
- S. Fernández Vallinas, N. López Carreras, M. Miguel and A. Aleixandre, Nitric oxide mediates the antihypertensive and vascular relaxing effects of a soluble cocoa fiber product in spontaneously hypertensive rats, Nitric Oxide, 2013, 29, 1–3 CrossRef.
- L. Bruyndonckx, V. Y. Hoymans, A. H. Van Craenenbroeck, D. K. Vissers, C. J. Vrints, J. Ramet and V. M. Conraads, Assessment of endothelial dysfunction in childhood obesity and clinical use, Oxid. Med. Cell. Longevity, 2013, 2013, 174782 Search PubMed.
- A. L. Axtell, F. A. Gomari and J. P. Cooke, Assessing endothelial vasodilator function with the Endo-PAT 2000, J. Visualized Exp., 2010,(44), e2167 Search PubMed.
- A. Correia-Costa, L. Correia-Costa, A. Caldas Afonso, F. Schaefer, A. Guerra, C. Moura, C. Mota, H. Barros, J. C. Areias and A. Azevedo, Determinants of carotid-femoral pulse wave velocity in prepubertal children, Int. J. Cardiol., 2016, 218, 37–42 CrossRef.
- L. D. Hudson, A. Rapala, T. Khan, B. Williams and R. M. Viner, Evidence for contemporary arterial stiffening in obese children and adolescents using pulse wave velocity: A systematic review and meta-analysis, Atherosclerosis, 2015, 241, 376–386 CrossRef CAS.
- N. M. Hamburg, J. Palmisano, M. G. Larson, L. M. Sullivan, B. T. Lehman, R. S. Vasan, D. Levy, G. F. Mitchell, J. A. Vita and E. J. Benjamin, Relation of brachial and digital measures of vascular function in the community: the Framingham heart study, Hypertension, 2011, 57, 390–396 CrossRef CAS.
- M. Freemark, Endothelial dysfunction and cardiovascular disease in childhood obesity, J. Pediatr., 2019, 95, 503–505 CrossRef.
- E. E. McClendon, S. K. Musani, T. E. Samdarshi, S. Khaire, D. Stokes, N. M. Hamburg, K. Sheffy, G. F. Mitchell, H. R. Taylor, E. J. Benjamin and E. R. Fox, The relation of digital vascular function to cardiovascular risk factors in African-Americans using digital tonometry: the Jackson Heart Study, J. Am. Soc. Hypertens., 2017, 11(6), 325–333 CrossRef.
- D. H. Thijssen, M. A. Black, K. E. Pyke, J. Padilla, G. Atkinson, R. A. Harris, B. Parker, M. E. Widlansky, M. E. Tschakovsky and D. J. Green, Assessment of flow-mediated dilation in humans: a methodological and physiological guideline, Am. J. Physiol.: Heart Circ. Physiol., 2011, 300, H2–12 CrossRef CAS.
- R. R. Holt, S. J. Yim, G. C. Shearer, R. M. Hackman, D. Djurica, J. W. Newman, A. W. Shindel and C. L. Keen, Effects of short-term
walnut consumption on human microvascular function and its relationship to plasma epoxide content, J. Nutr. Biochem., 2015, 26, 1458–1466 CrossRef CAS.
- A. Nohria, M. Gerhard-Herman, M. A. Creager, S. Hurley, D. Mitra and P. Ganz, Role of nitric oxide in the regulation of digital pulse volume amplitude in humans, J. Appl. Physiol., 2006, 101, 545–548 CrossRef CAS.
- Y. Chen, F. Dangardt, W. Osika, K. Berggren, E. Gronowitz and P. Friberg, Age- and sex-related differences in vascular function and vascular response to mental stress. Longitudinal and cross-sectional studies in a cohort of healthy children and adolescents, Atherosclerosis, 2012, 220, 269–274 CrossRef CAS.
- T. Radtke, K. Khattab, P. Eser, S. Kriemler, H. Saner and M. Wilhelm, Puberty and microvascular function in healthy children and adolescents, J. Pediatr., 2012, 161, 887–891 CrossRef.
- M. R. Williams, R. A. Westerman, B. A. Kingwell, J. Paige, P. A. Blombery, K. Sudhir and P. A. Komesaroff, Variations in endothelial function and arterial compliance during the menstrual cycle, J. Clin. Endocrinol. Metab., 2001, 86, 5389–5395 CrossRef CAS.
- J. Y. Kim, J. B. Park, D. S. Kim, K. S. Kim, J. W. Jeong, J. C. Park, B. H. Oh, N. Chung and K. investigators, Gender Difference in Arterial Stiffness in a Multicenter Cross-Sectional Study: The Korean Arterial Aging Study (KAAS), Pulse, 2014, 2, 11–17 CrossRef.
- M. Hashimoto, M. Akishita, M. Eto, M. Ishikawa, K. Kozaki, K. Toba, Y. Sagara, Y. Taketani, H. Orimo and Y. Ouchi, Modulation of endothelium-dependent flow-mediated dilatation of the brachial artery by sex and menstrual cycle, Circulation, 1995, 92, 3431–3435 CrossRef CAS.
- C. S. Hayward and R. P. Kelly, Gender-related differences in the central arterial pressure waveform, J. Am. Coll. Cardiol., 1997, 30, 1863–1871 CrossRef CAS.
- R. S. Vasan, M. G. Larson, D. Levy, J. C. Evans and E. J. Benjamin, Distribution and categorization of echocardiographic measurements in relation to reference limits: the Framingham Heart Study: formulation of a height- and sex-specific classification and its prospective validation, Circulation, 1997, 96, 1863–1873 CrossRef CAS.
- S. McGuire, Scientific Report of the 2015 Dietary Guidelines Advisory Committee. Washington, DC: US Departments of Agriculture and Health and Human Services, 2015, Adv. Nutr., 2016, 7, 202–204 CrossRef PubMed.
- J. L. Slavin and B. Lloyd, Health benefits of fruits and vegetables, Adv. Nutr., 2012, 3, 506–516 CrossRef CAS PubMed.
- J. A. Vita, Polyphenols and cardiovascular disease: effects on endothelial and platelet function, Am. J. Clin. Nutr., 2005, 81, 292S–297S CrossRef CAS PubMed.
- P. Tuso, S. R. Stoll and W. W. Li, A plant-based diet, atherogenesis, and coronary artery disease prevention, Perm. J., 2015, 19, 62–67 CrossRef PubMed.
- A. Mente, L. de Koning, H. S. Shannon and S. S. Anand, A systematic review of the evidence supporting a causal link between dietary factors and coronary heart disease, Arch. Intern. Med., 2009, 169, 659–669 CrossRef CAS.
- E. Ginter and V. Simko, Plant polyphenols in prevention of heart disease, Bratisl. Lek. Listy, 2012, 113, 476–480 CAS.
- M. F. Chong, R. Macdonald and J. A. Lovegrove, Fruit polyphenols and CVD risk: a review of human intervention studies, Br. J. Nutr., 2010, 104(Suppl 3), S28–S39 CrossRef CAS.
- A. Basu, D. X. Fu, M. Wilkinson, B. Simmons, M. Wu, N. M. Betts, M. Du and T. and J. Lyons, Strawberries decrease atherosclerotic markers in subjects with metabolic syndrome, Nutr. Res., 2010, 30, 462–469 CrossRef CAS.
- F. Giampieri, S. Tulipani, J. M. Alvarez-Suarez, J. L. Quiles, B. Mezzetti and M. Battino, The strawberry: composition, nutritional quality, and impact on human health, Nutrition, 2012, 28, 9–19 CrossRef CAS.
- M. Nabrzyski and R. Gajewska, The content of nitrates and nitrites in fruits, vegetables and other foodstuffs, Rocz. Panstw. Zakl. Hig., 1994, 45, 167–180 CAS.
- J. Susin, V. Kmecl and A. Gregorcic, A survey of nitrate and nitrite content of fruit and vegetables grown in Slovenia during 1996–2002, Food Addit. Contam., 2006, 23, 385–390 CrossRef CAS PubMed.
- A. Machha and A. N. Schechter, Dietary nitrite and nitrate: a review of potential mechanisms of cardiovascular benefits, Eur. J. Nutr., 2011, 50, 293–303 CrossRef CAS PubMed.
- A. Basu, A. Nguyen, N. M. Betts and T. J. Lyons, Strawberry as a functional food: an evidence-based review, Crit. Rev. Food Sci. Nutr., 2014, 54, 790–806 CrossRef CAS.
- D. Djurica, R. R. Holt, J. Ren, A. W. Shindel, R. M. Hackman and C. L. Keen, Effects of a dietary strawberry powder on parameters of vascular health in adolescent males, Br. J. Nutr., 2016, 116, 639–647 CrossRef CAS PubMed.
- D. J. Jenkins, T. H. Nguyen, C. W. Kendall, D. A. Faulkner, B. Bashyam, I. J. Kim, C. Ireland, D. Patel, E. Vidgen, A. R. Josse, H. D. Sesso, B. Burton-Freeman, R. G. Josse, L. A. Leiter and W. Singer, The effect of strawberries in a cholesterol-lowering dietary portfolio, Metabolism, 2008, 57, 1636–1644 CrossRef CAS PubMed.
- A. Basu, M. Wilkinson, K. Penugonda, B. Simmons, N. M. Betts and T. J. Lyons, Freeze-dried strawberry powder improves lipid profile and lipid peroxidation in women with metabolic syndrome: baseline and post intervention effects, Nutr. J., 2009, 8, 43 CrossRef PubMed.
- B. Burton-Freeman, A. Linares, D. Hyson and T. Kappagoda, Strawberry modulates LDL oxidation and postprandial lipemia in response to high-fat meal in overweight hyperlipidemic men and women, J. Am. Coll. Nutr., 2010, 29, 46–54 CrossRef CAS PubMed.
- I. Edirisinghe, K. Banaszewski, J. Cappozzo, K. Sandhya, C. L. Ellis, R. Tadapaneni, C. T. Kappagoda and B. M. Burton-Freeman, Strawberry anthocyanin and its association with postprandial inflammation and insulin, Br. J. Nutr., 2011, 106, 913–922 CrossRef CAS.
- C. L. Ellis, I. Edirisinghe, T. Kappagoda and B. Burton-Freeman, Attenuation of meal-induced inflammatory and thrombotic responses in overweight men and women after 6-week daily strawberry (Fragaria) intake. A randomized placebo-controlled trial, J. Atheroscler. Thromb., 2011, 18, 318–327 CrossRef CAS.
- S. J. Zunino, M. A. Parelman, T. L. Freytag, C. B. Stephensen, D. S. Kelley, B. E. Mackey, L. R. Woodhouse and E. L. Bonnel, Effects of dietary strawberry powder on blood lipids and inflammatory markers in obese human subjects, Br. J. Nutr., 2012, 108, 900–909 CrossRef CAS.
- S. Moazen, R. Amani, A. Homayouni Rad, H. Shahbazian, K. Ahmadi and M. Taha Jalali, Effects of freeze-dried strawberry supplementation on metabolic biomarkers of atherosclerosis in subjects with type 2 diabetes: a randomized double-blind controlled trial, Ann. Nutr. Metab., 2013, 63, 256–264 CrossRef CAS PubMed.
- J. M. Alvarez-Suarez, F. Giampieri, S. Tulipani, T. Casoli, G. Di Stefano, A. M. González-Paramás, C. Santos-Buelga, F. Busco, J. L. Quiles, M. D. Cordero, S. Bompadre, B. Mezzetti and M. Battino, One-month strawberry-rich anthocyanin supplementation ameliorates cardiovascular risk, oxidative stress markers and platelet activation in humans, J. Nutr. Biochem., 2014, 25, 289–294 CrossRef CAS PubMed.
- A. Basu, N. M. Betts, A. Nguyen, E. D. Newman, D. Fu and T. J. Lyons, Freeze-dried strawberries lower serum cholesterol and lipid peroxidation in adults with abdominal adiposity and elevated serum lipids, J. Nutr., 2014, 144, 830–837 CrossRef CAS.
- C. K. Richter, A. C. Skulas-Ray, T. L. Gaugler, J. D. Lambert, D. N. Proctor and P. M. Kris-Etherton, Incorporating freeze-dried strawberry powder into a high-fat meal does not alter postprandial vascular function or blood markers of cardiovascular disease risk: a randomized controlled trial, Am. J. Clin. Nutr., 2017, 105, 313–322 CrossRef CAS PubMed.
- R. G. Feresin, S. A. Johnson, S. Pourafshar, J. C. Campbell, S. J. Jaime, N. Navaei, M. L. Elam, N. S. Akhavan, S. Alvarez-Alvarado, G. Tenenbaum, K. Brummel-Smith, G. Salazar, A. Figueroa and B. H. Arjmandi, Impact of daily strawberry consumption on blood pressure and arterial stiffness in pre- and stage 1-hypertensive postmenopausal women: a randomized controlled trial, Food Funct., 2017, 8, 4139–4149 RSC.
- S. Lidder and A. J. Webb, Vascular effects of dietary nitrate (as found in green leafy vegetables and beetroot) via the nitrate-nitrite-nitric oxide pathway, Br. J. Clin. Pharmacol., 2013, 75, 677–696 CrossRef CAS PubMed.
- O. L. Jon, W. Eddie and T. G. Mark, The nitrate–nitrite–nitric oxide pathway in physiology and therapeutics, Nat. Rev. Drug Discovery, 2008, 7, 156 CrossRef PubMed.
- C. Kenyatta, S. P. Kristine, H. C. Jack, P. P. Rakesh, D. R. Christopher, M. Sabrina, K. Y. Benjamin, A. W. Myron, Z. Gloria, X. Xiuli, T. H. Kris, S. Howard, B. K.-S. Daniel, N. S. Alan, O. C. Richard and T. G. Mark, Nitrite reduction to nitric oxide by deoxyhemoglobin vasodilates the human circulation, Nat. Med., 2003, 9, 1498 CrossRef.
- D. P. Casey, D. T. Beck and R. W. Braith, Systemic plasma levels of nitrite/nitrate (NOx) reflect brachial flow-mediated dilation responses in young men and women, Clin. Exp. Pharmacol. Physiol., 2007, 34, 1291–1293 CrossRef CAS.
- D. A. Hobbs, T. W. George and J. A. Lovegrove, The effects of dietary nitrate on blood pressure and endothelial function: a review of human intervention studies, Nutr. Res. Rev., 2013, 26, 210–222 CrossRef CAS.
- D. A. Hobbs, M. G. Goulding, A. Nguyen, T. Malaver, C. F. Walker, T. W. George, L. Methven and J. A. Lovegrove, Acute ingestion of beetroot bread increases endothelium-independent vasodilation and lowers diastolic blood pressure in healthy men: a randomized controlled trial, J. Nutr., 2013, 143, 1399–1405 CrossRef CAS.
- A. Rodriguez-Mateos, M. Hezel, H. Aydin, M. Kelm, J. O. Lundberg, E. Weitzberg, J. P. Spencer and C. Heiss, Interactions between cocoa flavanols and inorganic nitrate: additive effects on endothelial function at achievable dietary amounts, Free Radicals Biol. Med., 2015, 80, 121–128 CrossRef CAS.
- B. S. Rocha, B. Gago, R. M. Barbosa and J. Laranjinha, Dietary polyphenols generate nitric oxide from nitrite in the stomach and induce smooth muscle relaxation, Toxicology, 2009, 265, 41–48 CrossRef CAS.
- H. Khoo, A. Azlan, S. Tang and S. Lim, Anthocyanidins and anthocyanins: colored pigments as food, pharmaceutical ingredients, and the potential health benefits, Food Nutr. Res., 2017, 61(1), 1361779 CrossRef PubMed.
- I. Krga and D. Milenkovic, Anthocyanins: From Sources and Bioavailability to Cardiovascular-Health Benefits and Molecular Mechanisms of Action, J. Agric. Food Chem., 2019, 67, 1771–1783 CrossRef CAS PubMed.
- A. K. Sandhu, M. G. Miller, N. Thangthaeng, T. M. Scott, B. Shukitt-Hale, I. Edirisinghe and B. Burton-Freeman, Metabolic fate of strawberry polyphenols after chronic intake in healthy older adults, Food Funct., 2018, 9, 96–106 RSC.
- C. Carkeet, B. A. Clevidence and J. A. Novotny, Anthocyanin excretion by humans increases linearly with increasing strawberry dose, J. Nutr., 2008, 138, 897–902 CrossRef CAS.
- A. K. Sandhu, Y. Huang, D. Xiao, E. Park, I. Edirisinghe and B. Burton-Freeman, Pharmacokinetic Characterization and Bioavailability of Strawberry Anthocyanins Relative to Meal Intake, J. Agric. Food Chem., 2016, 64, 4891–4899 CrossRef CAS.
- A. M. Amini, K. Muzs, J. P. Spencer and P. Yaqoob, Pelargonidin-3-O-glucoside and its metabolites have modest anti-inflammatory effects in human whole blood cultures, Nutr. Res., 2017, 46, 88–95 CrossRef CAS.
- S.-K. Ku, E.-K. Yoon, W. Lee, S. Kwon, T. Lee and J.-S. Bae, Antithrombotic and antiplatelet activities of pelargonidin in vivo and in vitro, Arch. Pharmacal Res., 2016, 39, 398–408 CrossRef CAS PubMed.
- Y. S. Min, H. J. Yoon, H. D. Je, J. H. Lee, S. S. Yoo, H. S. Shim, H. Y. Lee, H. O. La and U. D. Sohn, Endothelium independent effect of pelargonidin on vasoconstriction in rat aorta, Biomol. Ther., 2018, 26, 374–379 CrossRef CAS PubMed.
- J.-W. Xu, K. Ikeda and Y. Yamori, Upregulation of Endothelial Nitric Oxide Synthase by Cyanidin-3-Glucoside, a Typical Anthocyanin Pigment, Hypertension, 2004, 44, 217–222 CrossRef CAS PubMed.
- C. Petersen, D. Bharat, B. R. Cutler, S. Gholami, C. Denetso, J. E. Mueller, J. M. Cho, J. S. Kim, J. D. Symons and P. V. Anandh Babu, Circulating metabolites of strawberry mediate reductions in vascular inflammation and endothelial dysfunction in db/db mice, Int. J. Cardiol., 2018, 263, 111–117 CrossRef.
- A. Rodriguez-Mateos, C. Rendeiro, T. Bergillos-Meca, S. Tabatabaee, T. W. George, C. Heiss and J. P. Spencer, Intake and time dependence of blueberry flavonoid-induced improvements in vascular function: a randomized, controlled, double-blind, crossover intervention study with mechanistic insights into biological activity, Am. J. Clin. Nutr., 2013, 98, 1179–1191 CrossRef CAS.
- J. C. Espín, M. Larrosa, M. T. García-Conesa and F. Tomás-Barberán, Biological significance of urolithins, the gut microbial ellagic acid-derived metabolites: the evidence so far, Evidence-Based Complementary Altern. Med., 2013, 2013, 270418 Search PubMed.
- D. Bialonska, S. G. Kasimsetty, S. I. Khan and D. Ferreira, Urolithins, intestinal microbial metabolites of Pomegranate ellagitannins, exhibit potent antioxidant activity in a cell-based assay, J. Agric. Food Chem., 2009, 57, 10181–10186 CrossRef CAS PubMed.
- B. Fotschki, J. Juśkiewicz, A. Jurgoński, K. Kołodziejczyk, J. Milala, M. Kosmala and Z. Zduńczyk, Anthocyanins in Strawberry Polyphenolic Extract Enhance the Beneficial Effects of Diets with Fructooligosaccharides in the Rat Cecal Environment, PLoS One, 2016, 11, e0149081 CrossRef PubMed.
- Y. Han, M. Song, M. Gu, D. Ren, X. Zhu, X. Cao, F. Li, W. Wang, X. Cai, B. Yuan, T. Goulette, G. Zhang and H. Xiao, Dietary Intake of Whole Strawberry Inhibited Colonic Inflammation in Dextran-Sulfate-Sodium-Treated Mice via Restoring Immune Homeostasis and Alleviating Gut Microbiota Dysbiosis, J. Agric. Food Chem., 2019, 67, 9168–9177 CrossRef CAS PubMed.
- B. Fotschki, J. Juśkiewicz, K. Kołodziejczyk, A. Jurgoński, M. Kosmala, J. Milala, K. Ognik and Z. Zduńczyk, Protective Effects of Ellagitannin-Rich Strawberry Extracts on Biochemical and Metabolic Disturbances in Rats Fed a Diet High in Fructose, Nutrients, 2018, 10, 445 CrossRef PubMed.
- J. L. Pluznick, Microbial Short-Chain Fatty Acids and Blood Pressure Regulation, Curr. Hypertens. Rep., 2017, 19, 25 CrossRef PubMed.
- H. Ishimoto, M. Shibata, Y. Myojin, H. Ito, Y. Sugimoto, A. Tai and T. Hatano, In vivo anti-inflammatory and antioxidant properties of ellagitannin metabolite urolithin A, Bioorg. Med. Chem. Lett., 2011, 21, 5901–5904 CrossRef CAS PubMed.
- M. Larrosa, M. T. García-Conesa, J. C. Espín and F. A. Tomás-Barberán, Ellagitannins, ellagic acid and vascular health, Mol. Aspects Med., 2010, 31, 513–539 CrossRef CAS PubMed.
- R. Clarke, Lowering blood homocysteine with folic acid-based supplements: meta-analysis of randomised trials, Indian Heart J., 2000, 52, S59–S64 CAS.
- D. S. Wald, M. Law and J. K. Morris, Homocysteine and cardiovascular disease: evidence on causality from a meta-analysis, Br. Med. J., 2002, 325, 1202 CrossRef PubMed.
- N. J. Wald, H. C. Watt, M. R. Law, D. G. Weir, J. McPartlin and J. M. Scott, Homocysteine and ischemic heart disease: results of a prospective study with implications regarding prevention, Arch. Intern. Med., 1998, 158, 862–867 CrossRef CAS PubMed.
- C. M. Loria, M. J. Klag, L. E. Caulfield and P. K. Whelton, Vitamin C status and mortality in US adults, Am. J. Clin. Nutr., 2000, 72, 139–145 CrossRef CAS PubMed.
- E. Azzini, P. Vitaglione, F. Intorre, A. Napolitano, A. Durazzo, M. S. Foddai, A. Fumagalli, G. Catasta, L. Rossi, E. Venneria, A. Raguzzini, L. Palomba, V. Fogliano and G. Maiani, Bioavailability of strawberry antioxidants in human subjects, Br. J. Nutr., 2010, 104, 1165–1173 CrossRef CAS PubMed.
- B. Frei, Ascorbic acid protects lipids in human plasma and low-density lipoprotein against oxidative damage, Am. J. Clin. Nutr., 1991, 54, 1113S–1118S CrossRef CAS PubMed.
- M. M. Engler, M. B. Engler, M. Malloy, E. Chiu, M. C. Schloetter, S. M. Paul, M. Stuehlinger, K. Lin, J. P. Cooke, J. D. Morrow, P. Ridker, N. Rifai, E. Miller, J. Witztum and M. Mietus, Antioxidant vitamins C and E improve endothelial function in children with hyperlipidemia - Endothelial assessment of risk from lipids in youth (EARLY) trial, Circulation, 2003, 108, 1059–1063 CrossRef CAS PubMed.
- Y. Plantinga, L. Ghiadoni, A. Magagna, C. Giannarelli, F. Franzoni, S. Taddei and A. Salvetti, Supplementation With Vitamins C and E Improves Arterial Stiffness and Endothelial Function in Essential Hypertensive Patients, Am. J. Hypertens., 2007, 20, 392–397 CrossRef CAS PubMed.
- N. J. Aburto, S. Hanson, H. Gutierrez, L. Hooper, P. Elliott and F. P. Cappuccio, Effect of increased potassium intake on cardiovascular risk factors and disease: systematic review and meta-analyses, Br. Med. J., 2013, 346, f1378 CrossRef PubMed.
- D. A. Sica, A. D. Struthers, W. C. Cushman, M. Wood, J. S. Banas and M. Epstein, Importance of potassium in cardiovascular disease, J. Clin. Hypertens., 2002, 4, 198–206 CrossRef CAS PubMed.
- J. L. Slavin, M. C. Martini, D. R. Jacobs and L. Marquart, Plausible mechanisms for the protectiveness of whole grains, Am. J. Clin. Nutr., 1999, 70, 459S–463S CrossRef CAS PubMed.
- J. Lunn and J. L. Buttriss, Carbohydrates and dietary fibre, Nutr. Bull., 2007, 32, 21–64 CrossRef.
- D. E. Threapleton, D. C. Greenwood, C. E. Evans, C. L. Cleghorn, C. Nykjaer, C. Woodhead, J. E. Cade, C. P. Gale and V. J. Burley, Dietary fibre intake and risk of cardiovascular disease: systematic review and meta-analysis, Br. Med. J., 2013, 347, f6879 CrossRef PubMed.
- M. V. Selma, A. González-Sarrías, J. Salas-Salvadó, C. Andrés-Lacueva, C. Alasalvar, A. Örem, F. A. Tomás-Barberán and J. C. Espín, The gut microbiota metabolism of pomegranate or walnut ellagitannins yields two urolithin-metabotypes that correlate with cardiometabolic risk biomarkers: Comparison
between normoweight, overweight-obesity and metabolic syndrome, Clin. Nutr., 2018, 37, 897–905 CrossRef CAS PubMed.
- G. Istas, R. P. Feliciano, T. Weber, R. Garcia-Villalba, F. Tomas-Barberan, C. Heiss and A. Rodriguez-Mateos, Plasma urolithin metabolites correlate with improvements in endothelial function after red raspberry consumption: A double-blind randomized controlled trial, Arch. Biochem. Biophys., 2018, 651, 43–51 CrossRef CAS PubMed.
- F. A. Tomás-Barberán, R. García-Villalba, A. González-Sarrías, M. V. Selma and J. C. Espín, Ellagic acid metabolism by human gut microbiota: consistent observation of three urolithin phenotypes in intervention trials, independent of food source, age, and health status, J. Agric. Food Chem., 2014, 62, 6535–6538 CrossRef PubMed.
- B. J. Arsenault, S. M. Boekholdt and J. J. Kastelein, Lipid parameters for measuring risk of cardiovascular disease, Nat. Rev. Cardiol., 2011, 8, 197–206 CrossRef CAS PubMed.
- G. Corona, F. Tang, D. Vauzour, A. Rodriguez-Mateos and J. P. E. Spencer, Assessment of the anthocyanidin content of common fruits and development of a test diet rich in a range of anthocyanins, J. Berry Res., 2011, 1, 209–216 CAS.
- H. Çoklar and M. Akbulut, Effect of sun, oven and freeze-drying on anthocyanins, phenolic compounds and antioxidant activity of black grape (Ek_ikara) (Vitis vinifera L.), S. Afr. J. Enol. Vitic., 2017, 38, 264–272 Search PubMed.
- E. Bakkalbaşi, Ö. Menteş and N. Artik, Food Ellagitannins–Occurrence, Effects of Processing and Storage, Crit. Rev. Food Sci. Nutr., 2008, 49, 283–298 CrossRef.
- T. J. Kaptchuk, Open-Label Placebo: Reflections on a Research Agenda, Perspect. Biol. Med., 2018, 61, 311–334 CrossRef PubMed.
- T. J. Kaptchuk, J. M. Kelley, L. A. Conboy, R. B. Davis, C. E. Kerr, E. E. Jacobson, I. Kirsch, R. N. Schyner, B. H. Nam, L. T. Nguyen, M. Park, A. L. Rivers, C. McManus, E. Kokkotou, D. A. Drossman, P. Goldman and A. J. Lembo, Components of placebo effect: randomised controlled trial in patients with irritable bowel syndrome, Br. Med. J., 2008, 336, 999–1003 CrossRef PubMed.
- L. C. Park and L. Covi, Nonblind placebo trial: an exploration of neurotic patients’ responses to placebo when its inert content is disclosed, Arch. Gen. Psychiatry, 1965, 12, 36–45 CAS.
- T. J. Kaptchuk, E. Friedlander, J. M. Kelley, M. N. Sanchez, E. Kokkotou, J. P. Singer, M. Kowalczykowski, F. G. Miller, I. Kirsch and A. J. Lembo, Placebos without deception: a randomized controlled trial in irritable bowel syndrome, PLoS One, 2010, 5, e15591 CrossRef CAS PubMed.
- J. E. G. Charlesworth, G. Petkovic, J. M. Kelley, M. Hunter, I. Onakpoya, N. Roberts, F. G. Miller and J. Howick, Effects of placebos without deception compared with no treatment: A systematic review and meta-analysis, J. Evidence-Based Complementary Altern. Med., 2017, 10, 97–107 Search PubMed.
- T. J. Kaptchuk and F. G. Miller, Placebo Effects in Medicine, N. Engl. J. Med., 2015, 373, 8–9 CrossRef CAS.
- D. Milenkovic, C. Morand, A. Cassidy, A. Konic-Ristic, F. Tomás-Barberán, J. M. Ordovas, P. Kroon, R. De Caterina and A. Rodriguez-Mateos, Interindividual Variability in Biomarkers of Cardiometabolic Health after Consumption of Major Plant-Food Bioactive Compounds and the Determinants Involved, Adv. Nutr., 2017, 8, 558–570 Search PubMed.
Footnote |
† Co-first authors. |
|
This journal is © The Royal Society of Chemistry 2020 |