DOI:
10.1039/D3NA00649B
(Review Article)
Nanoscale Adv., 2024,
6, 32-50
The emerging role of medical foods and therapeutic potential of medical food-derived exosomes
Received
15th August 2023
, Accepted 8th November 2023
First published on 11th November 2023
Abstract
Medical food is consumed for the purpose of improving specific nutritional requirements or disease conditions, such as inflammation, diabetes, and cancer. It involves partial or exclusive feeding for fulfilling unique nutritional requirements of patients and is different from medicine, consisting of basic nutrients, such as polyphenols, vitamins, sugars, proteins, lipids, and other functional ingredients to nourish the patients. Recently, studies on extracellular vesicles (exosomes) with therapeutic and drug carrier potential have been actively conducted. In addition, there have been attempts to utilize exosomes as medical food components. Consequently, the application of exosomes is expanding in different fields with increasing research being conducted on their stability and safety. Herein, we introduced the current trends of medical food and the potential utilization of exosomes in them. Moreover, we proposed Medi-Exo, a exosome-based medical food. Furthermore, we comprehensively elucidate various disease aspects between medical food-derived exosomes (Medi-Exo) and therapeutic natural bionanocomposites. This review highlights the therapeutic challenges regarding Medi-Exo and its potential health benefits.
1. Introduction
In late 2019, the COVID-19 pandemic began to spread globally after the first confirmed human infection.1 This catastrophe prompted increased global health awareness, which increased the production and demand for healthy diet, medicines, and vaccines.2–4 However, the pandemic also caused disruptions in the distribution channels of these products. In addition, the probability of contracting the infectious disease was higher for people with other underlying diseases and once contracted they were more severely affected. Accordingly, people started to show interest in substances that are healthy to eat and in being healthy. Furthermore, they became focused on not contracting COVID-19 as was seen from the increased consumption of nutritional medical supplements and foods.5
Medical food is consumed or administered under the supervision of a physician.6,7 Specifically, it is food with unique nutritional requirements determined by medical evaluation for the purpose of managing a specific diet for a disease or condition.8,9 The first medical food to be released was Lofenalac (Lofenala®) in 1958, which was initially classified as a drug by the FDA and then reclassified as medical food in 1972. Similar to Lofenala, early medical foods were mostly intended to meet special nutritional needs. Recently, the range of medical food has been expanded to include medical foods to manage various diseases, such as inflammation, osteoporosis, and venous insufficiency, and it is subdivided into different categories, such as functional foods, nutraceuticals, and dietary supplements.7 Functional foods are naturally occurring and modified foods with bio-functionalities. Alternatively, nutraceuticals are ingredients or food bio-actives with bio-functionality.10 Medical foods are consumed in the form of food and thus are different from medicines consumed in the form of pills. The medical foods in each of the above-mentioned categories can be classified according to the health status of the body and the purpose of administration. Dietary supplements, such as vitamins, amino acids, probiotics, and natural products, are added in medical food for various purposes.8
Recently, efforts have been made to use nanovesicles, such as liposomes and exosomes, as supplements for medical foods. All cells export extracellular vesicles (exosomes) containing different components and are classified according to their biosynthesis mechanisms.11 Various exosomes inherit the characteristics of their cell origin, given that they include some of its components.11,12 Exosomes are produced in the inner core of cells and contain nucleic acids, proteins, and lipids with a small size of 40 to 100 nm, and thus they can perform various functions and be naturally absorbed by cells.13 Due to the advantages of exosomes, they are used in various fields such as drug delivery, diagnosis, and treatment.13–15 Firstly, exosomes are in the spotlight in the medical community due to their high homologous and low immune responses to avoid their decomposition in animals or patients. Secondly, exosomes can load various cargoes. In addition, the presence of a phospholipid bilayer is beneficial for fusion with the membranes of receptor cells. The structure of exosomes protects them from harsh conditions such as the digestive tract, including the acidic environment of the stomach, and in particular, exosomes derived from food can be absorbed from the intestine. They are transferred to other organs through the bloodstream and can function. Exosomes are also present in actual cooked or processed foods and are common and derived from various sources, ranging from grapes to human cells.16 Exosomes have gained popularity due to their biocompatibility and low immunogenicity as effective delivery systems for therapeutics. Medical-purpose exosomes (Medi-Exo) with therapeutic potential are being applied for the treatment of inflammation, cancer, and cardiovascular disease as natural bionanocomposites.
In this review, we discuss the current trend of medical food components used for the treatment and prevention of different types of diseases. To address the limitations of conventional medical food, medical food-derived exosomes (Medi-Exo) are suggested as medical food (or additives) for the treatment of various diseases and health benefits (Scheme 1). Finally, the integration of food with extracellular vesicle (e.g., exosomes) technology is at the forefront of this field.
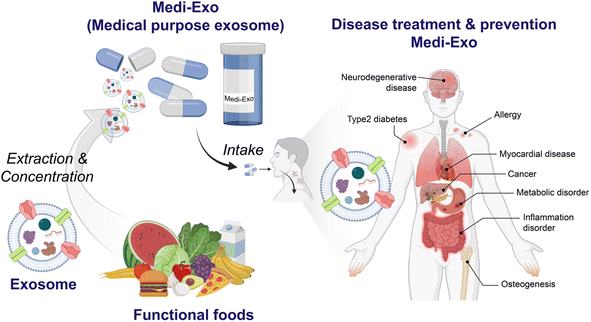 |
| Scheme 1 The overview of Medi-Exo contents and its health benefits. | |
2. Current knowledge of medical food
Medical food is a specialized type of food that has been developed specifically to address the treatment or management of specific diseases or health conditions. It serves various purposes, including the treatment of conditions such as Alzheimer's disease and metabolic diseases, enhancing the quality of life for cancer patients, and even preventing certain conditions such as high risk of pre-eclampsia (Fig. 1). Based on its intended use, medical food can be categorized into three main groups, i.e., supportive medical food, therapeutic medical food, and preventable medical food. Firstly, support medical food aims to improve the quality of life of patients by enhancing their immunity and supports their treatment indirectly. Secondly, the goal of treatment with medical food is to heal patients and help them regain their health. Finally, the prevention medical food can help high-risk people from getting sick (Table 1). Each category serves a distinct purpose in supporting the health needs and addressing specific medical conditions of individuals.
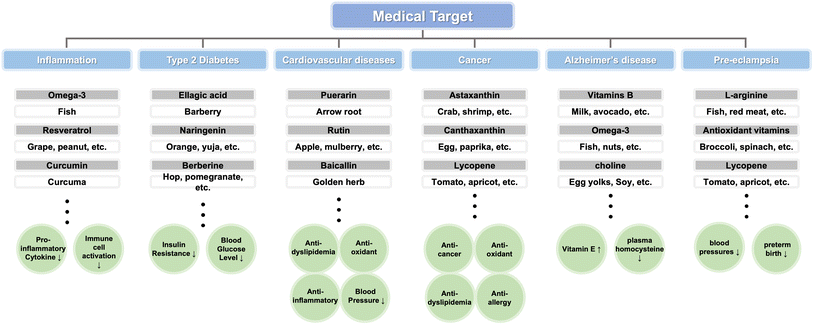 |
| Fig. 1 Medical food and key compounds to treat diseases. The foods used as medical foods and the main ingredients contained in them are illustrated to show which diseases they can be used to treat. | |
Table 1 Classification of medical foods and the treatment of diseases
Disease |
Substances contained in medical food |
Reference |
(i) Support of diseases through medical food
|
Cancer |
High in protein and leucine and enriched with emulsified fish oil (containing EPA and DHA) and a specific oligosaccharide mixture |
17
|
Cancer |
40 g protein, based on casein and whey protein and enriched with 10% free leucine and other specific components |
18
|
Angina secondary to atherosclerotic coronary artery disease (CAD) |
L-Arginine |
19
|
![[thin space (1/6-em)]](https://www.rsc.org/images/entities/char_2009.gif) |
(ii) Treatment of diseases through medical food
|
Aware of the pathogenesis |
PKU (phenylketonuria) |
LNAA (large neutral amino acids) |
20
|
Cow's milk allergy (CMA) |
Hydrolyzed rice formula |
21
|
Unaware of the pathogenesis |
MS(motion sickness) in childhood periodic syndrome |
G. simplicifolia, containing high concentrations of 5-HTP |
22
|
Mild Alzheimer's disease |
Phosphatide precursors, vitamins B (for endogenous choline), vitamins C, vitamins E, selenium, and phospholipids |
23
|
![[thin space (1/6-em)]](https://www.rsc.org/images/entities/char_2009.gif) |
(iii) Prevention of diseases through medical food
|
Population with high risk of pre-eclampsia |
L-Arginine and antioxidant vitamins |
24
|
Anemia in pregnancy |
L-Methylfolate and high-dose vitamin B(12) |
25
|
2.1 Support of diseases through medical food
Chemotherapy is a common treatment for cancer patients, but it has critical drawbacks. It can have effects not only on cancer cell but also normal cells, thus reducing immunity by decreasing the number of white blood cells.26,27 Increasing immunity is essential to overcome the decreased immunity caused by cancer to protect patients from viral infections.28,29 Firstly, due to the increasing immunity by nutrition,30,31 proper nutriment supply can help both reduce the risk of infection and aid in overall treatment.32,33 Secondly, the muscle mass of cancer patients affects their life after chemotherapy.34 Cancer patients maintaining a good weight, muscle index, and muscle density have been shown to have an increased average survival period by approximately three times compared with a decrease in the above-mentioned characteristics in cancer patients (28.4 months and 8.4 months, respectively).35 Particularly, the elderly often become unable to move gradually as they are confined to a bed for a long time. They may experience a decline in mobility as their muscles weaken and their strength decreases. Eventually, it becomes difficult for them to move again, and complications, such as pneumonia and pressure ulcers, are repeated, making it easier for their condition to gradually deteriorate.36 Faber J. et al. examined the effects of a medical food rich in protein, leucine, fish oil, and certain oligosaccharides on 38 cancer patients receiving radiation therapy in a randomized, double-blind trial. The results showed that the group consuming the medical food had significantly higher levels of EPA and DHA integration in their white blood cells and reduced levels of the inflammatory medium PGE2 within a week.17 Nicolaas E. P. Deutz et al. investigated how medical foods manufactured with high leucine and protein content could stimulate muscle protein synthesis in cancer patients, and the results showed that the medical foods increased the FSR muscle protein by 0.024% per hour compared to the beginning.18
Chronic stable angina is a type of angina caused by chronic stenosis due to arteriosclerosis.37 The main objectives of treatment are to control symptoms, prevent myocardial infarction or sudden death, and manage coronary risk factors.38 Proper management of blood pressure and blood sugar, weight, and cholesterol levels is important for managing chronic stable angina.39 Many risk factors in CAD are associated with the reduction of nitrogen monoxide (NO). Hence, increasing the resistance to thrombosis and atherosclerosis by restoring NO activity using medical foods containing L-arginine and additional nutrients was investigated. Maxwell Andrew J. et al. divided their study into two treatment weeks and four recovery weeks.19 Subsequently, they measured different metrics, including treadmill work, walking ECG readings, and quality of life ratings. The treadmill exercise capacity throughout the treatment period increased by 20% compared to the placebo period. After two weeks of treatment, the total walking duration increased by 23%, and the pain-free walking distance increased. Positive outcomes were also seen in the quality of life, and in particular, there was high satisfaction with vitality (general happiness), housework, and work-related performance.
2.2 Treatment of diseases through medical food
2.2.1. Childhood cyclical syndrome.
Esposito Maria et al. studied medical food for the treatment of childhood cyclical syndrome.22 Childhood periodic syndrome is known as the pre-stage of migraines in children and adolescents, which is divided into cyclic vomiting syndrome (CVS), abdominal migraine (AM), and benign paroxysmal vertigo of childhood (BPVC).40 It usually occurs in infancy or adolescence, manifests unexpectedly, recovers spontaneously, and is entirely normal between seizures, although the symptoms recur.41
In the case of childhood periodic syndrome, given that large-scale research is insufficient, it has been treated using medical food based on the mechanism of motion sickness symptoms. In particular, there is already a drug treatment for motion sickness, but it is used in adulthood for acute treatment of motion sickness, which is not suitable for children, and there is no preventive treatment for affected adults to date. The most famous theory for explaining motion sickness is the sensory collision theory, which a mismatch between visual and somatic senses, inducing nausea and vomiting. In addition, exceptionally, motion sickness is often considered a precursor to migraines. In the case of migraine attacks, the concentration of serotonin is lower than the normal level. Magnesium can relieve nerve excitement and muscle stiffness, which can improve motion sickness and migraines. Therefore, an experiment was conducted on 254 children using a seed extract of the 5-HTP-rich Griffonia simplicifolia Bail, a precursor of serotonin, and a compound containing magnesium. Consequently, the prevalence of children in the group who ingested the Griffonia/magnesium complex after 3 months was 36%, which was considerably lower than the prevalence of 73% in the control group.
2.2.2. Neurodegenerative disease.
The exact mechanism and cause of Alzheimer's disease are still not clearly understood. Currently, it is known that a little protein called beta-amyloid is overproduced and deposited in the brain, harming brain cells.42–45 In addition, the tau protein, which is crucial for maintaining the skeleton of brain cells, is damaged by oxidation, inflammation, and hyperphosphorylation, all of which may potentially cause damage to brain cells and have an impact on development.46,47 A typical brain pathology finding, the neuroblast (or senile), is associated with the deposition of beta-amyloid proteins, and the bundle of nerve fibers is associated with tau protein hyperphosphorylation. Here, the loss of hippocampus and cortical synapses was considered to be the most closely associated, and thus researchers experimented with medical food containing nutrients that support the regeneration of synapses.23 The nucleotide uridine, omega-3 polyunsaturated fatty acids, and choline are examples of foods that are known to promote synapse development. As a result of the 12 weeks core study, improvements in language imaging work were found in 24% of patients in the control group and 40% in the active group. In addition, the significant intake of docosahexaenoic (DHA) and EPA was observed in the entire active group, and the plasma levels of vitamin E were significantly increased. Moreover, the response was calculated by combining the scores of ADAS-cog scale (cognition), ADCS-ADL scale (function), and CIBIC-plus (behavior), and the active group showed a higher response of 18.2% in comparison to the control group.
2.2.3 Inflammatory disorder and allergy.
Inflammation is one of the biological responses to harmful stimuli and is a phenomenon that occurs when immune cells, blood vessels, and inflammatory mediators are involved.48,49 Inflammation appears with the purpose of cell damage inhibition, scar tissue removal, and tissue regeneration, but also causes tissue damage and disease, such as rheumatoid arthritis, dermatitis, asthma, and inflammatory bowel disease.48,50–54 Therefore, although an inflammatory response may be necessary for the body, there are cases in which it is necessary to control or stop it. Curcumin, which is present in turmeric, suppresses the production of TNF-a, NF-kB, and COX-2, which are representative inflammatory mediators and reported to be effective in the treatment of inflammatory diseases.55–57 Resveratrol is a phytoalexin found in berries such as raspberries and grapes.58,59 Resveratrol inhibits the proliferation of spleen cells induced by IL-2 or allo-antigens and suppresses inflammatory cytokines of immune cells, thereby preventing inflammatory reactions effectively.59 Dietary polyunsaturated fatty acids (PUFAs), which are abundant in fish, have been studied to regulate inflammation by suppressing the expression of COX-2, VEGFR2, and NF-kB, although the inhibitory mediators vary slightly depending on the type of PUFA.60,61 Many food components that control inflammation have the potential to not only control inflammation, but also improve the diseases associated with it.62
Cow's milk allergy (CMA) is defined as an adverse effect on one or more milk proteins mediated by IgE or non-IgE mechanisms.21 The prevalence rate for this common symptom in kids is about 4.9% of children aged <3 years. IgE-mediated reactions manifest as gastrointestinal, respiratory, and skin symptoms within 1 to 2 hours of ingestion, whereas non-IgE-mediated reactions include food protein-induced enteropathy (FPE) and food protein-induced allergic enteritis. It causes a variety of symptoms of food protein-induced allergic proctocolitis (FPIAP). Hypoallergenic milk powder is a diet for children who usually have this at one year of age and do not breastfeed.63 Also, extensively hydrolyzed formula (eHF), which is generally proven to be hypoallergenic, is recommended, but hydrolyzed rice-based formulas (HRF) can be used as an alternative for infants who reject eHF or have severe CMA.64 Anania Caterina et al.64 reported the assessment of three factors, as follows: (i) the analysis of growth comes first. The growth discrepancies between infants with moderate or severe CMA and healthy children were not substantially different, and the growth trends of newborns fed HRF, soybean milk powder, and eHF were equivalent. Thus, they evaluated whether HRF could be a nutritionally appropriate alternative for infants. (ii) The second is the symbolism of the taste of different formulations, which was evaluated by both adults and children, and the findings revealed that the preference for HRF was superior to eHF. (iii) The third is arsenic. Given that rice absorbs arsenic at a high rate, which is a non-threshold human carcinogen, the European Commission limits the amount of arsenic in newborn food to 0.10 mg kg−1. In conclusion, the use of cheap and highly symbolic HRFs was evaluated to be useful in the treatment of CMA in 1 month-old infants.
2.2.4 Cardiovascular disease.
Cardiovascular diseases include diseases such as coronary heart disease, heart failure, and hypertensive heart disease.65 The causes of these diseases are diverse, including hypertension, dyslipidemia, oxidative stress, and inflammation.66 Therefore, some anti-inflammatory, anti-cancer activity compounds can be utilized as anti-cardiovascular compounds.
Puerarin, an isoflavonoid present in kudzu, improves the NO and cGMP levels.67,68 In addition, it enhances the level of phosphorylated eNOS protein and decreases the level of caveolin-1 and angiotensin II type 1-receptor. Through this process, puerarin has been reported to have an effect of lowering blood pressure, and may also contribute to the prevention of myocardial infarction.67,69 Similar to puerarin, rutin, which is abundant in foods such as apples, figs, and asparagus, can lower blood pressure through eNOS signaling.70,71 Additionally, it can inhibit coronary heart disease by regulating extracellular signal-regulated kinase 1/2 and Akt signaling. It has been reported that baicalin, which is abundant in Baikal skullcap, lowers blood pressure by acting on intracellular Ca2+ regulation.72,73 Given that the number one cause of death worldwide is cardiovascular disease, efforts are being made to uncover the mechanisms of identified components or new functional components.74
2.2.5 Metabolic disorders.
Diabetes is a metabolic disorder caused by high blood sugar levels,75 which is divided into type 1 and type 2.76 Type 1 has problems with the insulin produced by the pancreas and can be managed with insulin injection. In type 2, insulin is secreted normally, but due to insulin resistance, cells cannot accept insulin and the blood sugar rises. Type 2 is thought to have a problem with the insulin receptor, although its exact mechanism is not known. Therefore, studies are being conducted on compounds that can control insulin resistance.76,77
Ellagic acid is a component abundantly contained in pomegranate and Castanea crenata inner shell.78 Although its exact mechanism has not been identified, it has been reported that it has activity to lower the blood glucose level and increase the insulin concentration.79,80 Naringenin, a flavanone found in oranges, citrons, and grapefruit, has carbohydrate metabolism promoter, anti-inflammatory, and anti-oxidant effects.81,82 Furthermore, it has been confirmed that it can alleviate diabetic nephropathy caused by type 2 diabetes. Berberine, one of the components of barberry, has also been found to have a hypoglycemic effect.83,84 It is expected to help alleviate diabetic nephropathy by effectively reducing total cholesterol and low-density lipoprotein, but some complain of gastrointestinal adverse effects. Therefore, it needs to be used with caution. Ingredients for diabetes, such as berberine, often have adverse effects. Thus, to overcome this, efforts to find new anti-diabetic sources are on-going.85
PKU is a condition in which phenylalanine hydroxylase activity is deficient or severely reduced, resulting in high plasma phenylalanine levels of 1000 mmol L−1.20 If left untreated, nerve cells in the cerebrum that are sensitive to phenylalanine levels can be damaged, resulting in catastrophic disorders such as microcephaly, epilepsy, severe cognitive impairment, behavioral issues, and even paralysis. Concolino et al. investigated a medical food called PheLNAA, which contains a lot of LNAA.20 Seven of the 16 subjects successfully improved blood Phe control to more than the first final goal setting (30% reduction) four weeks after taking PheLNAA. These seven patients were found to have a 32% to 90% reduction in blood pH levels from the baseline throughout the study. Therefore, it was demonstrated that increased LNAA concentrations in PheLNAA medical foods significantly inhibit Phe transport from these patients to the gastrointestinal tract and BBB. Consequently, a novel approach for enhancing the diet and improving the adaptation of patients to the diet, which become restricted as they age, was offered.
Wang et al. combined the facts that the polymorphism of MTHFR reduces the L-methylfolic acid, serum methylcobalamin, and serum homocysteine levels and causes hypertension and DR, and methylation of homocysteine and B12 in their study.86 They examined the effects of Ocufolin, a dietary supplement comprised of L-methylfolate and vitamin D, and eye-folate, a multivitamin with similar ingredients, on the progression of retinopathy in seven patients. Using fundus photography, all participants exhibited intraretinal hemorrhage, microaneurysms (MA), and reduced exudate.
2.2.6 Cancer.
Aerobic metabolism produces reactive oxygen species, including superoxide and hydrogen peroxide, which oxidize biological components such as DNA, proteins, and lipids, causing cancer.87 Oxidation is not the only cause of cancer, but because it is considered the most important cause, various studies have been conducted on antioxidant compounds that show anti-cancer effects.88–90
Astaxanthin is an alpha-hydroxyl-keto-carotenoid that is contained in various types of crustaceans such as shrimp and crab.91 Astaxanthin is both a scavenger for free radicals and an oxidation protector.92,93 Although the exact anti-cancer mechanism is still unknown, modification of gap junction communication is expected to affect anti-cancer activity.94 Canthaxanthin, which is contained in foods such as eggs and paprika, also shows anti-cancer activity as a free radical scavenger.95 Additionally, canthaxanthin has also been reported to inhibit the proliferation of human colon cancer cells and the development of skin tumors.96,97 The chemopreventive activity of canthaxanthin has also been shown to be a result of gap junction communication modification.98 Lycopene, which is typically contained in tomatoes, is a free radical scavenger together with astaxanthin and canthaxanthin.99 Lycopene is known to inhibit the growth of human cancer cells. In particular, it has been reported to interfere with cell cycle progression and growth factor receptor signaling in prostate cancer.100 In addition to the above-mentioned components, antioxidant compounds such as lutein and fucoxanthin have been confirmed to have anti-cancer activity.101,102
2.3 Prevention of diseases through medical food
Pre-eclampsia (PE) is high blood pressure accompanied by excessive protein in the urine and is a problem that occurs only during pregnancy.103 Pre-eclampsia can cause the placenta to peel off and deliver early, which increases the likelihood that newborns will have issues.104 Also, the hands, fingers, neck, and feet of women may also swell, and if their pre-eclampsia is severe and untreated, seizures (eclampsia) or organ damage may occur. One of the major causes of morbidity and mortality in mothers and newborns is pre-eclampsia and eclampsia.105 According to Vadillo-Ortega Felipe et al., hypertension disorder in mothers is caused by the promotion of maternal vasoconstriction due to a defective placenta and the activation or destruction of endothelial cells.24 Therefore, it was decided that L-arginine administration will be beneficial for mothers who are at high risk for liver disease. L-Arginine is an endothelial-derived vasodilator and is used as a substrate for nitrogen oxide synthesis enzymes that show synthetic defects in liver disease. Women who had pre-eclampsia in the past or who had a family history of the condition received a bar containing L-arginine and antioxidant vitamins. The rate of pre-eclampsia or eclampsia declined to 13% in patients taking a combination of L-arginine and vitamins, and the premature delivery rate was only 11%. Additionally, it was discovered that at the start of the study there was no difference in the plasma concentration of L-arginine between the treatment groups. However, two neonatal deaths occurred in the placebo group and three in the vitamin group at birth, but not in the group that took both L-arginine and vitamins. This is related to extremely premature babies. Specifically, the intake of L-arginine and vitamin-added medical foods significantly reduced the risk of maternal pre-eclampsia, eclampsia, and premature birth.
Premature birth, natural miscarriage, and childbirth with underweight babies are all affected by anemia during pregnancy.106 Furthermore, pregnant women with anemia are more likely to have a premature birth and mild labor contractions, resulting in prolonged delivery durations and an increased risk of excessive bleeding. In the study by Bentley Susan et al., the effect of medical foods that added L-methylfolate and vitamin B12 to the nutrition of existing prenatal vitamins (PNVs) as a control group was tested with PNVs.25 The hemoglobin changed by −10.9 in the case of prenatal vitamins and merely by −4.0 in the case of medical food from the start of pregnancy until delivery. Additionally, prenatal vitamins had a 74.1% incidence of anemia and medicinal foods had a 39.7% incidence, both of which were significant outcomes.
3. Therapeutic aspects of natural bionanocomposites: exosomes
Exosomes are a type of extracellular vesicle (EV) that are released by cells into the extracellular space. They are small, spherical vesicles with an average diameter of less than 200 nm, and they possess a phospholipid bilayer structure. Exosomes can be found in various body fluids, including blood, urine, saliva, and milk. They play a crucial role in cell-to-cell communication and the transport of bioactive molecules, as well as molecules that are overexpressed in response to the specific condition or environment of cells. Exosomes contain a variety of bioactive molecules, including proteins, lipids, and nucleic acids such as RNA and DNA. Recently, milk-derived exosomes were discovered, which have been recognized as versatile bioactive materials. Subsequently, their potential application has been extended in various fields, including drug delivery, disease treatment, and cell communication.
Functional ingredients are low in concentration and are used by preparing extracts of raw food for their functions. However, commonly, it is not easy to isolate only the desired component from food, and thus food extracts contain various components in addition to the target component.107,108 Consequently, adverse effects may be induced by unidentified ingredients. In this case, is relatively easy to identify the components of exosomes, and in addition to the target functional components, additional benefits from the proteins, lipids, and nucleic acids of exosomes can be obtained with higher efficiency.109 Therefore, utilizing exosomes as a functional component of medical food can be a good strategy for obtaining health benefits (Table 2).
Table 2 Exosomal components as biomarkers
Exosomal components |
Disease |
Associated molecules |
Reference |
Protein |
Prostate cancer |
CD9 |
126
|
Breast cancer |
CD81 |
127
|
Melanoma |
TRIP2, VLA-4, HSP70, HSP90 |
128
|
Pancreatic cancer |
Glypican-1 |
129
|
Colorectal cancer |
CD147, CD9 |
130
|
Chronic hepatitis C |
CD81 |
131
|
Alzheimer's disease |
Exosomal amyloid peptides, Thr-181 |
132
|
Parkinson's disease |
DJ-1, α-synuclein |
133
|
Nucleic acid |
Type 2 diabetes |
miRNA-1249-3p |
134
|
Myocardial infarction |
miRNA-125b-5p |
135
|
Acute myocardial infarction |
miRNA-93-5p |
136
|
Oral squamous cell carcinoma |
miR-155 |
137
|
Liver cancer |
miR-26a |
138
|
Colorectal cancer |
miR-146a-5p, miR-155-5p |
139
|
Pancreatic ductal adenocarcinoma |
miR-196a, miR-124, miR-1226-3p |
140
|
Breast cancer |
BCL-2 siRNA |
141
|
Bladder cancer |
PLK-1 siRNA |
142
|
Exosomes are produced by endocytosis.110,111 Exosomes are colloidal nanoparticles and their surface charge is reflected by their zeta potential, which is considered as one of the characteristics of exosomes.112,113 Exosomes secreted by animal cells have a spherical structure formed of a lipid bilayer.114 Similar to most plant-derived vesicles, nano-vesicles have a simple lipid bilayer like eukaryotic cell membranes and are structurally spherical.115 The composition of exosomes is not random and their function and composition depend on the cell of their origin.116,117 Similar to normal cell membranes, the membrane components of exosomes are composed of lipids, carbohydrates and proteins.118–120 The unique characteristics of exosomes have attracted significant attention in clinical applications as both diagnostic biomarkers and therapeutic cargo carriers. One of their appealing attributes is their reduced immunogenicity, which is a result of their biocompatibility and bilayer lipid structures.121 These structures effectively shield the encapsulated cargo from degradation, making exosomes an attractive option as a therapeutic vector. Moreover, exosomes offer the advantage of accommodating various cargoes, and their ability to fuse with the receptors in the call membrane makes them highly efficient for the delivery of cargo. However, although exosomes are considered promising vectors, their components have gained greater popularity in the medical community due their potential in treating inflammation, cancer, and cardiovascular diseases.122 In addition, due to their ability to represent metabolic stages of cell/organ origin and their important role in major pathological processes, exosomes are considered new and promising biomarkers for a wide range of diseases (Fig. 2). Mesenchymal stem cell-derived exosomes (MSC-exosomes) are one of the exosomes that show potential for the regeneration of tissue damage. Mesenchymal stem cells have been reported to alleviate type 2 diabetes in animals and clinical trials, and it was confirmed that MSC-exosomes are also effective in treating type 2 diabetes.123 Treatment with MSC-exosomes in a type 2 diabetic rat model reduced blood sugar levels and restored phosphorylation of insulin receptor substrate 1 and protein kinase B.124 In addition, it has been confirmed that they alleviate diabetes and restore insulin secretion through muscle cell glucose transporter expression, liver glycogen storage increase, and B-cell death suppression.125
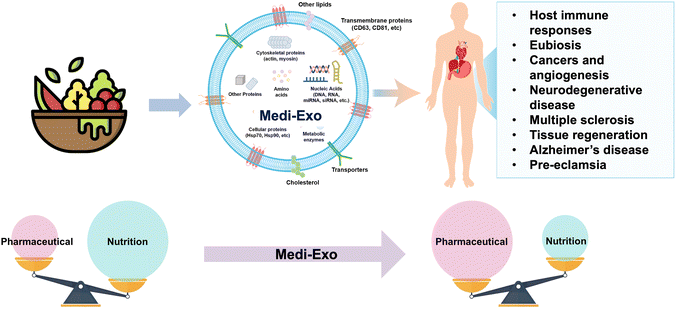 |
| Fig. 2 A schematic representation of biogenesis and uptake of exosomes. | |
3.1 Exosomal proteins
Various proteins exist on the surface of exosomes, and proteins derived from the parent cells play a major role in intracellular communication.143 Proteins with various roles such as targeting, adhesion, and T-cell stimulation are present in the exosome membrane.144 In particular, tetraspanins are abundant, which are involved in cell penetration, invasion, and fusion (Fig. 3A). Heat shock proteins (HSP70 and HSP90) are involved in antigen binding and antigen presentation in stress response.145 Exosomes contain various proteins such as membrane transport proteins, heat shock proteins, and enzymes to play functional roles, and highly abundant proteins can be used to distinguish exosomes as biomarkers.144,146 For example, CD9 and CD81 were found to be positive as exosomal protein markers in prostate cancer126 and breast cancer cell lines,127 respectively. Additionally, TRIP2, VLA-4, HSP70, and HSP90 were identified as potential diagnostic biomarkers for melanoma128 and CD147 and CD9 for colorectal cancer.130 It has also been reported that glypican-1, a surface protein of exosomes, is exclusively present in the serum of pancreatic cancer.129 Exosomes can serve as biomarkers in various diseases besides cancer. In the case of hepatitis C patients, an increase in exosomal CD81 has been observed.131 Furthermore, exosomal amyloid peptides and phospho-tau (Thr-181) have been implicated in Alzheimer's disease,132 while DJ-1 and α-synuclein are potentially associated with the diagnosis of Parkinson's disease.133 In the case of exosomes derived from animal cells, it is known that the proteins contained in the cells of their origin are also contained in the exosomes in relative abundance.147 However, it has been reported that plant-derived exosomes contain relatively simpler proteins than animal cell-derived exosomes.148
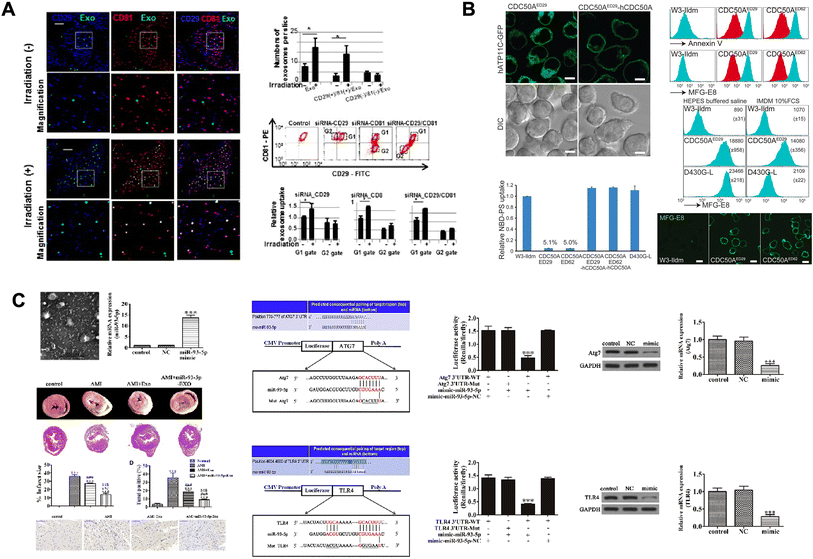 |
| Fig. 3 Exosome components: a diverse array of therapeutic agents. (A) Cellular uptake of exosomes through the CD29/CD81 complex formation. This figure has been adapted from Ref. Hazawa et al. with permission from Elsevier, copyright 2014.203 (B) Caspase-mediated cleavage of phospholipid flippase for apoptotic phosphatidylserine exposure for engulfment of diseases. This figure has been adapted from Ref. Segawa et al. with permission from Science, copyright 2014.151 (C) miR-93-5p-containing exosomes treatment attenuates acute myocardial infarction-induced myocardial damage. This figure has been adapted from Ref. Liu et al. with permission from CellPress, copyright 2018.161 | |
3.2 Exosomal lipids
Lipids in exosome membranes are essential components of their lipid bilayer structure.119 It contains lipids such as cholesterol, sphingolipids, and phosphatidylserines, and most of the lipids of exosomes are found in their outer membrane due to their formation process.149,150 In general, sphingomyelin is predominantly found in the outer leaflet of exosomes, while phosphatidylserine is found in their inner leaflet. Alternatively, it appears that phosphatidylethanolamine is randomly distributed in their bilayer (Fig. 3B).151 The lipid composition varies depending on the type of cell of origin, but interestingly, it differs greatly between exosomes and their parent cells.152 However, a similar lipid composition is shown between exosomes from a single cell line. The higher the content of sphingomyelin, desaturated lipids, and cholesterol in the exosome membrane than in the parent cell, the greater the stiffness and resistance to degradation of the exosome, and the more effective the delivery of substances such as proteins and nucleic acids.143,153
3.3 Exosomal nucleic acids
Exosomes may include RNAs and DNAs such as mRNAs, miRNAs, and lncRNAs that were present in their cell of origin.154 Exosomes contain abundant miRNAs and genomic DNA, and contain up to 500 different miRNAs. Exosomes carry their nucleic acids into the target cells, which are then transcribed and translated to affect the cells.155 The nucleic acids can be stably detected in the circulatory system and protected from RNase degradation by the stability of exosomes. Consequently, exosomal miRNA is a potential candidate as an ideal biomarker in clinical diagnostics. Exosomes have demonstrated remarkable sensitivity and specificity, particularly in diverse cancer types. The nucleic acids encapsulated within exosomes can be categorized into several classes, including messenger RNA (mRNA), microRNA (miRNA), small interfering RNA (siRNA), circular RNA (circRNA), and long non-coding RNA (lncRNA). The type and amount of nucleic acids in exosomes, especially lncRNAs and circRNAs, are greatly affected by the health status of their origin. Utilizing these characteristics, RNAs in exosomes can be used as disease diagnostic markers.156 miRNAs contained in exosomes have many effects on cells based on cell-to-cell communication such as angiogenesis, hematopoiesis, and tumorigenesis.157
It has been confirmed that natural killer (NK)-derived exosomes derived from lean mice attenuate insulin resistance and inflammatory response in type 2 diabetes.158 miRNA-1249-3p accumulates in NK-derived exosomes. miRNA-1249-3p targets SKOR1, which regulates the formation of SMAD6/MYD88/SMURF1.
The hypoxic bone marrow mesenchymal stem cell (BM-MSC) exosomes extracted by inducing hypoxia in BM-MSCs were found to increase the therapeutic effect by concentrating miRNAs, showing therapeutic effects in the myocardium.159 A particularly high concentration of miRNA-125b-5p was accumulated in the exosomes of hypoxic BM-MSCs.159,160
Adipose-derived stromal cells (ADSCs) contain miRNA-93-5p, and the cardioprotective effect of this exosome was confirmed in vitro and in vivo (Fig. 3C).161In vitro, miRNA-93-5p effectively inhibited hypoxia-induced autophagy and inflammatory cytokine expression by targeting Atg7 and TLR4.
When exosomes loaded with miR-26a were tested on class B type 1-expressing liver cancer cells, it was found that the upregulation of miR-26a led to a decrease in cell migration and proliferation rates.138 This was attributed to the reduction in the levels of key proteins involved in G1/S transition, which halted the cells in the G1 phase and inhibited the progression of the cell cycle.
Many estrogen receptor-positive (ER+) breast cancer patients exhibit overexpression of the anti-apoptotic protein BCL-2.141 This protein, being an estrogen-responsive gene, promotes disease progression and enhances the survival of tumor cells, allowing cancer cells to evade programmed cell death. NK cell-derived exosomes were used as a carrier for BCL-2 targeted siRNAs, and it was found that exosomes loaded with siBLC-2 activated mitochondria-dependent apoptosis in malignant breast cancer cell lines.
Upon loading PLK-1 siRNA into exosomes and co-culturing them with an invasive bladder cancer cell line, it was discovered that the gene expression of PLK-1 was inhibited, resulting in the induction of apoptosis.142
4. Treatment and prevention of diseases through food-derived exosomes
Pathogenesis is defined as the occurrence and development of disease. The prevention, management, and treatment of numerous diseases depend on an understanding of their etiology and progression.162 This is owing to the fact that the mechanical properties of the tissue or cellular environment play a role in the progression or development of many diseases (Fig. 2).163
Food-derived exosomes (FDE) are exposed to the gastrointestinal environment after food consumption (Table 3). However, the lipid bilayer surrounding FDE prevents their degradation under the conditions of the digestive tract, including the acidic environment of the stomach. Therefore, substances loaded within FDE are not broken down in the gastrointestinal tract. They are safely absorbed through the intestines, affecting the whole body.164 FDE absorbed through the intestinal walls reach peripheral organs via the bloodstream.165 This stability and mechanism have been evaluated for their potential use in the treatment and prevention of various diseases. Exosomes not only serve as carriers but also contain substances that contribute to therapy.166 For example, exosomes derived from blueberries carry miRNAs such as miR-156e, which have shown effectiveness against cardiovascular inflammation.167 Additionally, the stability of exosomes is utilized for the delivery of methotrexate.168 FDE originate not only from fruits but also from various sources, including milk and broccoli.
Table 3 Derived exosomes and their targeted diseases
Food source |
Therapeutic components of Medi-Exo |
Extraction methods |
Target mechanism or disease |
Reference |
Honey |
miR-4057 |
Ultracentrifugation and filtration |
Acute liver injury |
185
|
Grapefruit |
Exosome + luciferase siRNA |
Purified on sucrose after centrifugation |
Colon cancer |
186
|
Exosome component + miR17 |
Purified on sucrose after centrifugation |
Brain tumor |
187
|
Exosome + methotrexate |
Purified on sucrose after centrifugation |
Inflammatory gut disease |
168
|
Grape |
Lipids |
Purified on a sucrose step gradient after centrifugation |
Dextran sulfate sodium-induced colitis |
188
|
Exosomes |
Purified on a sucrose step gradient after centrifugation |
Intestinal stem cell |
189
|
Blueberry |
miR-156e, miR-162, miR-319d |
Sequential centrifugation, ultra-filtered and centrifugation |
Cardiovascular inflammation |
167
|
Lemon |
Citrus nanovesicles |
Centrifuged and transferred to a 30% sucrose/D2O cushion and ultracentrifugation |
Cancer cell proliferation |
181
|
Broccoli |
Lipids |
Sequential centrifugation, sucrose purification and ultracentrifugation |
Mouse colitis |
171
|
Ginger |
Exosome + cancer-specific ligands |
Membrane filtration, differential ultracentrifugation, and equilibrium density gradient ultracentrifugation |
Nasopharyngeal cancer |
190
|
Exosome with doxorubicin (Dox) |
Centrifugation, sucrose purification and ultracentrifugation |
Colon cancer |
191
|
siRNA-CD98 |
Centrifugation, sucrose purification and ultracentrifugation |
Ulcerative colitis |
192
|
Ath-miR167a |
Centrifugation, sucrose purification and ultracentrifugation |
DSS-induced colitis |
193
|
mi-RNA125 |
Centrifugation and ultracentrifugation |
Chronic colitis and colitis-associated cancer(CAC) |
194
|
Shogaol |
Centrifugation, ultracentrifugation, sucrose step gradient and ultracentrifugation |
Alcohol-induced liver damage |
169
|
DMT1 siRNA |
Centrifugation, mixing with FA, dry and add equal volume Hepes buffer |
Hereditary hemochromatosis |
195
|
Milk |
Curcumin |
Use miRCURY™ Exosome isolation kit (Exiqon) |
Adenocarcinoma |
196
|
Exosome + anthocyanins |
Differential ultracentrifugation |
Lung tumor |
197
|
Exosome + Dox, EPT1, Ce6 |
Differential centrifugation |
Oral squamous cell carcinoma |
198
|
Has-miR-148a-3p |
Sequential centrifugation and ultracentrifugation |
Lung tumor |
199
|
Lactoferrin and α-lactalbumin |
Differential ultracentrifugation |
Breast cancer |
172
|
GRP94 |
Ultracentrifugation and extraction |
Necrotizing enterocolitis |
200
|
miR-148a |
Centrifugation, filtration and ultracentrifugation |
Osteoarthritis |
201
|
Moringa |
miRNA-160h, miRNA-166, and miRNA-159c |
Filtration and ultracentrifugation |
Tumorigenesis |
202
|
4.1 Food-derived exosomes
Food-derived exosomes (FDE) have a variety of potential functions for the treatment and prevention of diseases. FDE contain biomaterials such as microRNAs, proteins, and lipids. They regulates gene expression, cellular processes, and signaling pathways in target cells. FDE also play a role in cell-to-cell communication, potentially influencing physiological processes and immune responses, and they can used as therapeutic agents. Some types of FDE contain biomaterial molecules with anti-inflammatory properties, which means they can reduce inflammation-associated disease. Thus, it is crucial to highlight that FED have functions in the treatment and prevention of diseases, and here we provide a detailed explanation of some various diseases.
Raimondo S. et al. found that the juice of Citrus limon L. contains nanoparticles that can be considered exosome-like nanovesicles, and isolating these nanovesicles and co-culturing them with various cells resulted in a decrease in the growth of tumor cell lines (A549, SW480, and LAMA84). Furthermore, they confirmed an increased expression of the pro-apoptotic molecules, Bad and Bax, indicating the promotion of tumor cell apoptosis.181
Ahn G. et al. extracted physiologically active exosomes (Mi-Exo) from bovine milk. They confirmed their cytoprotective effect through antioxidant activity and anti-inflammatory effects, and also discovered that they contain TGF-β3, which reduces scar formation. The authors described their potential as a therapeutic material to minimize scars and keloids, such as skin tissue damage.170
Nanoparticles derived from broccoli target DCs, inducing tolerogenic DCs and preventing mouse colitis. Deng Z. et al. treated a mouse colitis model with broccoli-derived nanoparticles for 10 days. Although the mice treated only with DSS exhibited weight loss, those treated with the broccoli-derived nanoparticles did not show any weight loss. Moreover, it was confirmed that broccoli-derived nanoparticles can be efficiently absorbed by dendritic cells (DCs), suggesting a novel therapeutic strategy for regulating gut immune homeostasis through dietary means and the treatment and prevention of diseases.171
When Badawy A. A. et al. confirmed the inhibitory effect of camel milk-derived exosomes on the MCF7 human breast cancer cell line and found that they inhibited cell migration and exhibited dose-dependent anti-proliferative activity. Furthermore, they observed reduced inflammation, angiogenesis, and metastasis in tumor tissues, as well as the inhibition of oxidative stress. In conclusion, it was confirmed that camel milk exosomes partially induced cell apoptosis in cancer cells.172
4.2 Host immune responses
Honey-derived exosomes have been shown to suppress inflammation by inhibiting the formation of the nucleotide-binding domain and leucine-rich repeat related (NLR) family, pyrin domain containing 3 (NLRP3) inflammasome (Fig. 4A).173 Honey-derived exosomes did not affect the inflammasome protein levels, but prevented oligomerized NLRP3 from recruiting apoptotic speck protein (ASC). It was confirmed from the immunofluorescence staining results that the visualized spots were significantly reduced with the honey-derived exosome treatment. In addition, miRNA-4057 in the honey-derived exosomes strongly inhibited the self-cleavage of Casp1 after NLRP3 inflammasome activation, thereby inhibiting the inflammatory response. However, miRNA-4057 from the honey-derived exosomes did not reduce the expression levels of IL-6 and TNF-a, which are inflammatory cytokines. However, even if the inflammatory cytokine level was not reduced, it was proven that the honey-derived exosomes inhibited the inflammatory response through the formation of inflammasomes and inhibition of Casp1 self-cleavage.
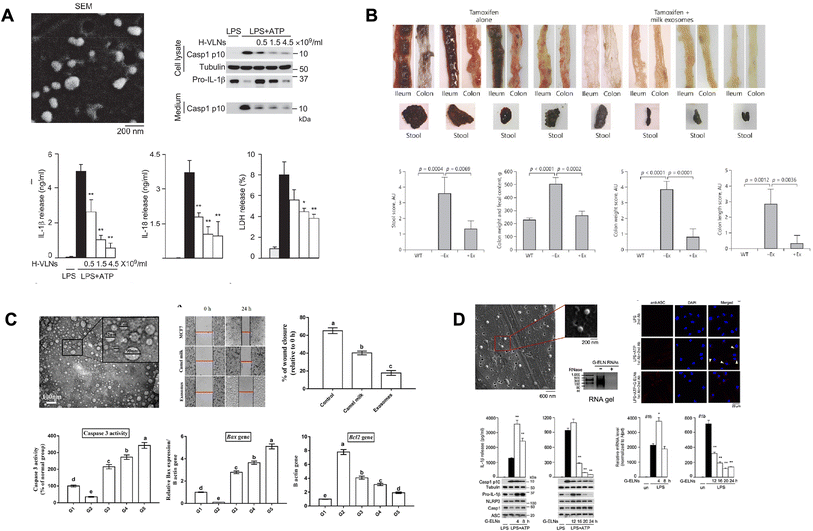 |
| Fig. 4 The treatment and prevention of diseases through food-derived exosomes. (A) The anti-inflammatory effect of vesicle-like nanoparticles in honey. This figure has been reproduced from Ref. Chen et al. with permission from Wiley, copyright 2021.173 (B) Milk exosomes prevent intestinal inflammation in a mouse model for ulcerative colitis. This figure has been reproduced from Ref. Stremmel et al. with permission from Karger, copyright 2020.176 (C) The tumor therapeutic effect of camel milk and its exosomes on MCF7 cells in vitro and in vivo for cancer cells. This figure has been adapted from Ref. Badawy et al. with permission from Sage, copyright 2021.179 (D) Exosome-like nanoparticles in ginger can inhibit the NLRP3 inflammasome activity. This figure has been adapted from Ref. Chen et al. with permission from ACS, copyright 2019.182 | |
Ginger-derived exosomes contain the bioactive constituents of ginger, 6-gingerol and 6-shogaol, in addition to normal exosome components. Ginger-derived exosomes decreased pro-inflammatory cytokines such as TNF-a, IL-6, and IL-1b and increased anti-inflammatory cytokines IL-10 and IL-22.87 It was proven that ginger-derived exosomes can prevent and improve inflammatory diseases by targeting the inflamed intestinal mucosa and reducing damage factors and promoting healing factors.
4.3 Eubiosis
The composition of the microbial community in the intestines is influenced by factors such as the genetic makeup, environment, and diet of the host. The microbial community produces signaling molecules and metabolites that impact various important functions in the intestines, including colonization, movement, digestion, permeability, secretion, energy extraction, mucosal immunity, and barrier effects. Moreover, certain components of the microbial community or groups of microorganisms can enter other organs such as the brain, liver, and pancreas through the circulatory system, influencing their functions. Essentially, the interactions between the immune system of the host and the microbial community are crucial for maintaining intestinal balance. However, if the equilibrium between the host and the microbial community is disrupted, it can lead to diseases. When the microbial communities are in balance, this is referred to as eubiosis, whereas an imbalance is called dysbiosis, which can contribute to both infectious and non-infectious diseases.174 It is widely recognized that the intestinal microbial community regulates the expansion of specific subsets of lymphocytes. T helper 17 (TH17) cells, a specific type of CD4+ T helper cells, play a significant role in the development of autoimmune diseases by producing inflammatory cytokines such as IL17A, IL17F, and IL-22. These TH17 cells accumulate in the intestine, indicating that the gut itself induces the maturation of TH17 cells. Another subset of CD4+ T helper cells known as forkhead box P3 (FOXP3)+ regulatory T cells (Tregs) is also abundant in the intestines and contributes to maintaining intestinal balance. The development of intestinal Treg cells has been shown to rely, at least partially, on the presence of microbial communities, as observed by a significant reduction in the intestinal lamina propria of germ-free mice.175 Research has discovered that exosomes derived from milk have an impact on ulcerative colitis. To investigate this, a study used mice with a specific genetic modification in their intestines (kindlin 2 intestine-specific knockout mice) induced by tamoxifen treatment. These mice were administered either a control solution of PBS or a suspension of exosomes in PBS for four days. The results demonstrated that the addition of exosomes led to the significant reversal of the colitis state, resembling the condition of normal mice. Additionally, their colon appeared clean without the presence of bloody stool (Fig. 4B).176 Several studies have shown that imbalances in the gut microbiota can induce inflammatory responses and contribute to the progression of rheumatoid arthritis (RA) in both RA patients and animal models. In the case of new-onset rheumatoid arthritis (NORA) patients, Prevotella copri was found in their gut, making these individuals more susceptible to RA. When dominant P. copri from RA patients was inoculated into mice that were raised in a germ-free environment, it induced arthritis through a mechanism involving Th17 cells. Furthermore, a decrease in the expression of claudin-1 and occludin, which are important proteins for maintaining gut barrier function, was observed before the onset of RA. In the lamina propria, which is located beneath the epithelial cells of the gut, there was an increase in CD3+ T cells, dendritic cells, and B cells in NORA patients. The lamina propria contains T cells, dendritic cells, B cells, and stromal cells, and Paneth cells promote the differentiation of both Th17 and Treg cells. The components found in milk can enhance gut barrier function and microbial diversity, thereby limiting the inflammatory process and protecting the gut.164 Extracellular vesicles (EVs) derived from human breast milk have been shown to have a protective effect on intestinal epithelial cells, reducing cell death caused by oxidative stress by activating the MAPK pathway.177
4.4 Cancers and angiogenesis
Moringa-derived exosomes lowered the viability of leukemia cells and increased apoptosis by decreasing B-cell lymphoma 2 protein expression and reducing the mitochondrial membrane potential.178 Cytotoxicity was shown in BCL2 positive cells, and these results were expected to be caused by miRNAs in moringa-derived exosomes, and thus the miRNA expression profile was confirmed. The moringa-derived exosomes contained miRNA-160h, miRNA-166, and miRNA-159c, which are expected to inhibit the transcription of the BCL2 protein involved in tumorigenesis. In a similar study, miRNAs did not affect the cell viability in PBMCs, highlighting the anti-tumor activity of moringa-derived miRNAs.
Although it is not known which component is responsible for the effect, camel milk exosomes have been confirmed to have various anticancer effects against breast cancer in vitro and in vivo (Fig. 4C).179,180 Their apoptosis-inducing ability for MCF7 breast cancer cells was confirmed by changes in the expression level of various apoptosis marker mRNAs. Bax was up-regulated by a minimum of 2.79 ± 0.12-fold to a maximum of 5.11 ± 0.22-fold and Bcl2 was up-regulated by a maximum of 4.09 ± 0.22-fold and a minimum of 1.92 ± 0.11-fold in mammary tumor tissue from mice treated with camel milk and camel milk exosomes. In rats treated with camel milk and camel milk exosomes, the mRNA levels of the lipid peroxidation biomarkers malondialdehyde (MDA) and iNOS were significantly reduced, and the oxidative stress markers superoxide dismutase, glutathione peroxidase and catalase increased, resulting in increased resistance to oxidation. Additionally, there was a decrease in inflammatory cytokine mRNA and an increase in immune cells. The effects were higher in camel milk exosomes than in camel milk, and the potential of camel milk exosomes as an anti-cancer treatment was proven by combining several pieces of evidence. To test the effects of exosomes on tumor cell growth, A549, SW480 (human lung and colorectal adenocarcinoma cell lines), and LAM84 (chronic myeloid leukemia cell line) were treated with Citrus limon L. juice-derived nanovesicles.181 It was observed that the treated groups formed fewer colonies compared to the untreated control group. Furthermore, to evaluate the mechanism of tumor growth inhibition, the expression of various molecules was tested, revealing an increase in the expression of the pro-apoptotic genes Bad and Bax and a decrease in the expression of the anti-apoptotic genes survivin and Bcl-xl. Additionally, activation through the TNF-related apoptosis-inducing ligand (TRAIL)/DR5 pathway, consisting of the TRAIL signal and the receptor Dr5, which controls the apoptotic process, was confirmed.
LAM cells were xenografted into NOD/SCID mice, and lemon-derived nanovesicles were locally (intratumoral) or intraperitoneally (IP) administered three times a week. The results, as shown in the figure below, demonstrated that the mice treated with the lemon-derived nanovesicles formed smaller tumors compared to the control group. Therefore, this suggests that citrus limon nanovesicles reduce tumor growth in vivo through the induction of TRAIL-mediated cell apoptosis and inhibition of cytokine secretion associated with angiogenesis.
4.5 Neurodegenerative disease
Recently, neuroinflammation has been recognized as a significant factor in neurodegenerative diseases such as Alzheimer's and Parkinson's disease. Inflammasomes are a complex of proteins that is formed when the body encounters danger signals from pathogenic infections or cellular stress. One specific type of inflammasome, known as NLRP3 (nucleotide-binding domain and leucine-rich repeat-containing family, pyrin domain containing 3), recruits Casp-1 to assemble a multi-protein complex (Fig. 4D).182 Activation of the NLRP3 inflammasome by Casp-1 leads to the production of IL-1β and IL-18 and triggers a form of cell death called pyroptosis.
In a recent experiment using bone marrow-derived macrophages from C57BL/6J mice, various essential oil nanoemulsions (ELNs) derived from ginseng, aloe vera, grapefruit, garlic, turmeric, dandelion, lavender, cactus, and ginger were cultured together. Most of these ELNs demonstrated weak inhibitory or stimulatory effects on the release of IL-1β and the levels of Casp1 p10 upon activation of the NLRP3 inflammasome. However, the G-ELN (ginseng essential oil nanoemulsion) showed a strong inhibitory effect on the release of IL-1β and IL-18, as well as the levels of Casp1 p10 during NLRP3 inflammasome activation. Importantly, G-ELN did not significantly affect the levels of the cytokines IL-6 and TNFα induced by LPS, suggesting that G-ELN has minimal impact on the general inflammatory response of macrophages. Furthermore, it was observed that lipids, among the components of G-ELN, play a role in its anti-inflammasome activity.
4.6 Multiple sclerosis
Multiple sclerosis (MS) is a condition characterized by the immune system's attack on myelin, a protective covering of nerve fibers. This attack disrupts the communication between the brain and the body, potentially leading to difficulties in independent walking when nerve fibers suffer permanent damage or deterioration. Presently, although there is no cure for MS, treatment focuses on accelerating recovery from attacks, modifying the progression of the disease, and managing symptoms. Autoimmune reactions of TCD4 cells primarily drive the development of MS, and the presence of myelin oligodendrocyte glycoprotein (MOG) on the outer layer of the myelin sheath facilitates the access of brain inflammation-inducing proteins such as myelin basic protein (MBP) and proteolipid protein (PLP). Strategies to induce antigen-specific tolerance in MS treatment involve modifying the structure, dosage, or administration route of the antigen. Butyrophilin (BTN) is a protein that shares over 50% amino acid similarity with the relevant domain of MOG. It has been identified in milk-derived exosomes and the milk fat globule membrane (MFGM), which are vesicular structures found in milk.183
4.7 Tissue regeneration
Wound healing refers to the process of replacing damaged tissue with newly generated tissue. The successful transition from inflammation to tissue regeneration, involving distinct phases of inflammation, proliferation, and remodeling, is crucial for the restoration of the extracellular matrix (ECM) and blood vessels. Macrophages play a versatile role in regulating each stage of wound healing. Fibroblasts and endothelial cells are also vital in the remodeling of the ECM and the formation of new blood vessels, which are essential for wound closure. Epigenetic factors, such as transcription factors and microRNAs (miRNAs), are known to be involved in the transition from inflammation to proliferation.
Milk-derived extracellular vesicles (EVs) have been shown to possess the ability to enhance the proliferation of fibroblasts by approximately 40–50% and endothelial cells by 20–30%. The extent of fibroblast proliferation was dependent on the concentration of milk EVs. In tube formation experiments using endothelial cells, milk EVs promoted an increase in both the branch point number and total tube length by approximately 2–30%. Moreover, milk EVs stimulated the proliferation and migration of fibroblasts (NIH-3T3 cells) and facilitated capillary formation in endothelial cells (SVEC4-10). Conversely, EVs derived from serum did not exhibit similar effects, possibly due to the presence of various pro-inflammatory factors in the serum. Consequently, milk EVs were identified as promoters of wound healing. Colostrum EVs and mature milk EVs exhibited higher expression levels of wound healing-related proteins compared to serum EVs. Specifically, colostrum EVs displayed increased expression of IL-4, which supports anti-inflammatory responses, and ECM accumulation in comparison to other types of EVs. Treatment with milk EVs resulted in a reduction in the expression of Smad7, a key inhibitor of the TGF-β signaling pathway involved in epithelialization, a crucial signaling pathway in wound healing. Additionally, miRNA21, which is known to promote wound healing through the Smad7-Smad2/3-elastin pathway, was upregulated in colostrum EVs.
In a mouse model of excisional wound healing, colostrum EVs labeled with Flamma 675 N-hydroxysuccinimide (NHS) were observed to remain in the skin for over 3 days before gradually decreasing and found to be distributed in the organs of mice following subcutaneous injection of EVs. The wound closure rate was significantly accelerated in the group treated with colostrum EVs compared to the control group (saline treatment). Notably, the mice receiving additional treatment with EVs starting on the 4th day after injury (Colos EVs-D4 group) exhibited significantly improved wound closure rates compared to the colostrum EV group.184
5 Conclusion
Medical food for specific nutritional requirements or the improvement of medical conditions has recently gained significant attention. The consumption of medical food has shown therapeutic effects in various diseases, ranging from Alzheimer's disease to metabolic disorders, and is available for sale as a formulated product.
Exosomes may also play a prominent role as medical materials as stable vectors and diagnostic biomarkers. Exosomes have a stable structure with a lipid bilayer and their ability to contain various proteins and nucleic acids make them suitable as a therapeutic agent. Furthermore, exosomes can remain stable in strong acidic environments because they consist of lipids. Accordingly, exosomes can act as carriers that can be absorbed by human tissue for the delivery of cargo to the target site. Also, they have been widely discussed for their potential use as diverse biomarkers, particularly in various types of cancer. They have been shown to have therapeutic effects in various diseases, ranging from metabolic to neurodegenerative disorders. Also, an advantage of exosomes is the availability of various natural and food-derived exosomes in our daily lives.
Exosomes in medical food (Medi-Exo) reveal health benefits and demonstrate potential to improve human diseases and provide new therapeutic approaches as natural bionanocomposites. They originate from natural food or sources and are composed of a lipid bilayer membrane enclosing a variety of biomolecules, which allows them to interact with target cells efficiently. Thus, with further studies on exosomes, they can be become a powerful component in medical food as “Medi-Exo”.
Although many opportunities lie ahead, the Medi-Exo technique as nascent technology faces limitations and challenges. Typically, to facilitate the application of exosomes in various disease treatments, it is essential to have confidence in their safety or toxicity for humans. Addressing this limitation will require efforts to understand the clear identification and mechanism of disease treatment using exosomes. Understanding the biodistribution of exosomes and their clearance mechanisms is crucial for their therapeutic application. Exosome therapy is a novel technology that is still undergoing clinical research and requires further investigation. It needs extensive clinical trials to establish its efficacy and safety in humans. The identification of exosomes often requires highly efficient extraction methods from food or natural materials, which can provide novel biomarkers. However, these food or natural materials suffer from poorly defined compositions, which may be problematic for identifying the detail information of exosomes. Ongoing efforts to find novel biomarkers of exosomes as natural materials may hold the key to addressing this problem. Despite these limitations, Medi-Exo for diseases such as inflammation, cancer, and metabolic disorders is expected to yield beneficial results through various applications in biology and medicine mechanisms.
Abbreviations
EPA | Eicosapentaenoic acid |
DHA | Docosahexaenoic acid |
PGE2 | Prostaglandin E2 |
FSR | Fractional synthesis rate |
CAD | Coronary artery disease |
ECG | Electrocardiogram |
TNF-a | Tumor necrosis factor a |
NF-kB | Nuclear factor kappa-light-chain-enhancer of activated B cells |
COX-2 | Cyclooxygenase-2 |
VEGFR2 | Vascular endothelial growth factor receptor 2 |
IgE | Immunoglobulin E |
CMA | Cow's milk allergy |
eNOS protein | Endothelial NOS protein |
BBB | Blood–brain barrier |
MTHFR | Methylenetetrahydrofolate reductase |
DR | Diabetic retinopathy |
CD9 | Cluster of differentiation 9 |
CD63 | Cluster of differentiation 63 |
CD81 | Cluster of differentiation 81 |
HSP70 | Heat shock protein 70 |
HSP90 | Heat shock protein 90 |
CD147 | Cluster of differentiation 147 |
Thr-181 | Phospho-tau |
DJ-1 | Parkinson's disease protein 7 |
Atg7 | Autophagy-related 7 |
TLR4 | Toll-like receptor 4 |
BCL-2 | B-cell lymphoma 2 |
siBLC-2 | Bcl2 siRNA |
PLK-1 | Polo-like kinase 1 |
NLR | Nucleotide-binding domain leucine-rich repeat containing |
NLRP3 | Nucleotide-binding domain and leucine-rich repeat protein-3 |
ASC | Recruiting apoptotic speck protein |
Casp1 | Cysteine–aspartic acid protease 1 (caspase-1) |
PBMCs | Peripheral blood mononuclear cells |
Bax | Apoptosis regulator |
Bad | BCL2 associated agonist of cell death |
Bcl-xl | B-cell lymphoma-extra large |
NOD/SCID | Nonobese diabetic/severe combined immune deficiency |
MOG | Myelin oligodendrocyte glycoprotein |
MBP | Myelin basic protein |
PLP | Proteolipid protein |
BTN | Butyrophilin |
ECM | Extracellular matrix |
Smad7 | SMAD family member 7, decapentaplegic homolog 7 |
Author contributions
J.-Y. H. and S. H. L. wrote the manuscript with input from all authors. Both W.-R. S and J.-Y. A. contributed to the final version of the manuscript. Y.-H. K. supervised the project. J.-Y. A. devised the project, the main conceptual ideas and proof outline.
Conflicts of interest
The authors declare that the research was conducted in the absence of any commercial or financial relationships that could be construed as a potential conflict of interest.
Acknowledgements
This work was supported by a Basic Science Research Program through the National Research Foundation of Korea (NRF) funded by the Ministry of Education (2020R1A6A1A06046235). This work was also partly supported by the Commercialization Promotion Agency for R&D Outcomes (COMPA) grant funded by the Korean government (MSIT) (No. 1711173792). This research was supported by a grant of the Korea Health Technology R&D Project through the Korea Health Industry Development Institute (KHIDI), funded by the Ministry of Health & Welfare, Republic of Korea (grant number : HI22C1941).
References
- M. Ciotti, M. Ciccozzi, A. Terrinoni, W.-C. Jiang, C.-B. Wang and S. Bernardini, Crit. Rev. Clin. Lab. Sci., 2020, 57, 365–388 CrossRef CAS.
- R. Forman, S. Shah, P. Jeurissen, M. Jit and E. Mossialos, Health policy, 2021, 125, 553–567 CrossRef PubMed.
- J. Sultana, S. Crisafulli, F. Gabbay, E. Lynn, S. Shakir and G. Trifirò, Front. Pharmacol., 2020, 11, 588654 CrossRef CAS.
- C. Rodríguez-Pérez, E. Molina-Montes, V. Verardo, R. Artacho, B. García-Villanova, E. J. Guerra-Hernández and M. D. Ruíz-López, Nutrients, 2020, 12, 1730 CrossRef.
-
Fi Global Insights 2021 Trend Guide, 2021 Search PubMed.
- B. P. Ciampa, E. Reyes Ramos, M. Borum and D. B. Doman, J. Gastroenterol. Hepatol., 2017, 13, 104–115 Search PubMed.
- J. L. Holmes, A. Biella, T. Morck, J. Rostorfer and B. Schneeman, Curr. Dev. Nutr., 2021, 5, nzaa172 CrossRef CAS PubMed.
- R. Casale, Z. Symeonidou, S. Ferfeli, F. Micheli, P. Scarsella and A. Paladini, Pain Ther., 2021, 10, 225–242 CrossRef.
- N. Ramalingum and M. F. Mahomoodally, Adv. Pharmacol. Sci., 2014, 2014, 354264 Search PubMed.
- C. M. Hasler, J. Nutr., 2002, 132, 3772–3781 CrossRef CAS.
- Y. Zhang, Y. Liu, H. Liu and W. H. Tang, Cell Biosci., 2019, 9, 19 CrossRef.
- Y. Zhang, J. Bi, J. Huang, Y. Tang, S. Du and P. Li, Int. J. Nanomed., 2020, 15, 6917–6934 CrossRef CAS PubMed.
- C. Yang, M. Zhang and D. Merlin, J. Mater. Chem. B, 2018, 6, 1312–1321 RSC.
- T. D. Brown, K. A. Whitehead and S. Mitragotri, Nat. Rev. Mater., 2020, 5, 127–148 CrossRef.
- B. Zhou, K. Xu, X. Zheng, T. Chen, J. Wang, Y. Song, Y. Shao and S. Zheng, Signal Transduction Targeted Ther., 2020, 5, 144 CrossRef CAS.
- P. Akuma, O. D. Okagu and C. C. Udenigwe, Front. Sustain. Food Syst., 2019, 3, 23 CrossRef.
- J. Faber, M. Berkhout, U. Fiedler, M. Avlar, B. Witteman, A. Vos, M. Henke, J. Garssen, A. Van Helvoort and M. Otten, Clin. Nutr., 2013, 32, 338–345 CrossRef CAS.
- N. E. P. Deutz, A. Safar, S. Schutzler, R. Memelink, A. Ferrando, H. Spencer, A. van Helvoort and R. R. Wolfe, Clin. Nutr., 2011, 30, 759–768 CrossRef CAS PubMed.
- A. J. Maxwell, M. P. Zapien, G. L. Pearce, G. MacCallum and P. H. Stone, J. Am. Coll. Cardiol., 2002, 39, 37–45 CrossRef CAS PubMed.
- D. Concolino, I. Mascaro, M. Moricca, G. Bonapace, K. Matalon, J. Trapasso, G. Radhakrishnan, C. Ferrara, R. Matalon and P. Strisciuglio, Eur. J. Clin. Nutr., 2017, 71, 51–55 CrossRef CAS.
- J. Walsh, R. Meyer, N. Shah, J. Quekett and A. T. Fox, Br. J. Gen. Pract., 2016, 66, e609–e611 CrossRef PubMed.
- M. Esposito, F. Precenzano, M. Sorrentino, D. Avolio and M. Carotenuto, J. Med. Food, 2015, 18, 916–920 CrossRef PubMed.
- P. Scheltens, P. J. Kamphuis, F. R. Verhey, M. G. O. Rikkert, R. J. Wurtman, D. Wilkinson, J. W. Twisk and A. Kurz, Alzheimer's Dementia, 2010, 6, 1–10 CrossRef CAS PubMed.
- F. Vadillo-Ortega, O. Perichart-Perera, S. Espino, M. A. Avila-Vergara, I. Ibarra, R. Ahued, M. Godines, S. Parry, G. Macones and J. F. Strauss, Bmj, 2011, 342, d2901 CrossRef PubMed.
- S. Bentley, A. Hermes, D. Phillips, Y. A. Daoud and S. Hanna, Clin. Ther., 2011, 33, 204–210 CrossRef CAS PubMed.
- L. Zitvogel, L. Apetoh, F. Ghiringhelli and G. Kroemer, Nat. Rev. Immunol., 2008, 8, 59–73 CrossRef CAS PubMed.
- S. Senapati, A. K. Mahanta, S. Kumar and P. Maiti, Signal Transduction Targeted Ther., 2018, 3, 7 CrossRef.
- T. Kawai and S. Akira, Nat. Immunol., 2006, 7, 131–137 CrossRef CAS.
-
V. T. DeVita, T. S. Lawrence and S. A. Rosenberg, DeVita, Hellman, and Rosenberg's Cancer: Principles & Practice of Oncology, Lippincott Williams & Wilkins, 2008 Search PubMed.
- R. K. Chandra and S. Kumari, J. Nutr., 1994, 124, 1433S–1435S CrossRef CAS PubMed.
-
M. Gershwin, Nutrition and Immunity, Elsevier, 2012 Search PubMed.
- M. R. Mainous and E. A. Deitch, Surg. Clin. North Am., 1994, 74, 659–676 CrossRef CAS PubMed.
- J. C. Frost and A. J. Baldwin, Clin. Med., 2021, 21, e272 CrossRef.
- F. Pin, M. E. Couch and A. Bonetto, Curr. Opin. Support Palliat., 2018, 12, 420 CrossRef.
- L. Martin, L. Birdsell, N. MacDonald, T. Reiman, M. T. Clandinin, L. J. McCargar, R. Murphy, S. Ghosh, M. B. Sawyer and V. E. Baracos, J. Clin. Oncol., 2013, 31, 1539–1547 CrossRef PubMed.
- A. Kamiya, T. Hayashi, R. Sakon, K. Ishizu, T. Wada, S. Otsuki, Y. Yamagata, H. Katai and T. Yoshikawa, BMC Surg., 2022, 22, 220 CrossRef PubMed.
- R. Kones, Vasc. Health Risk Manage., 2010, 6, 635 CrossRef PubMed.
- D. R. Zanger, A. J. Solomon and B. J. Gersh, Am. Fam. Physician, 2000, 61, 129–138 CAS.
- E. M. Ohman, N. Engl. J. Med., 2016, 374, 1167–1176 CrossRef CAS.
- A. A. Gelfand, Curr. Opin. Neurol., 2013, 26, 262–268 CrossRef PubMed.
- D. Spiri, V. E. Rinaldi and L. Titomanlio, Ital. J. Pediatr., 2014, 40, 1–11 CrossRef PubMed.
- X. Sun, W.-D. Chen and Y.-D. Wang, Front. Pharmacol., 2015, 6, 221 Search PubMed.
- D. J. Selkoe, Annu. Rev. Neurosci., 1994, 17, 489–517 CrossRef CAS PubMed.
- S. M. Khan, D. S. Cassarino, N. N. Abramova, P. M. Keeney, M. K. Borland, P. A. Trimmer, C. T. Krebs, J. C. Bennett, J. K. Parks and R. H. Swerdlow, Ann. Neurol., 2000, 48, 148–155 CrossRef CAS.
- S. Sadigh-Eteghad, B. Sabermarouf, A. Majdi, M. Talebi, M. Farhoudi and J. Mahmoudi, Med. Princ. Pract., 2015, 24, 1–10 CrossRef.
- R. Medeiros, D. Baglietto-Vargas and F. M. LaFerla, CNS Neurosci. Ther., 2011, 17, 514–524 CrossRef CAS PubMed.
- C. Bancher, C. Brunner, H. Lassmann, H. Budka, K. Jellinger, G. Wiche, F. Seitelberger, I. Grundke-Iqbal, K. Iqbal and H. Wisniewski, Brain Res., 1989, 477, 90–99 CrossRef CAS.
- L. Chen, H. Deng, H. Cui, J. Fang, Z. Zuo, J. Deng, Y. Li, X. Wang and L. Zhao, Oncotarget, 2018, 9, 7204–7218 CrossRef.
- J. M. Bennett, G. Reeves, G. E. Billman and J. P. Sturmberg, Front. Med., 2018, 5, 316 CrossRef.
- A. J. Guimond, K. Shiba, E. S. Kim and L. D. Kubzansky, Psychoneuroendocrinology, 2022, 141, 105746 CrossRef CAS PubMed.
- E. H. Choy and G. S. Panayi, N. Engl. J. Med., 2001, 344, 907–916 CrossRef CAS.
- D. Y. Leung, M. Boguniewicz, M. D. Howell, I. Nomura and Q. A. Hamid, J. Clin. Invest., 2004, 113, 651–657 CrossRef CAS PubMed.
- A. Kay, J. Allergy Clin. Immunol.J. Clin. Invest., 1991, 87, 893–910 CrossRef CAS PubMed.
- D. C. Baumgart and S. R. Carding, Lancet, 2007, 369, 1627–1640 CrossRef CAS.
- N. J. Olsen, Minerva Med., 2011, 102, 33–40 CAS.
- Y. Peng, M. Ao, B. Dong, Y. Jiang, L. Yu, Z. Chen, C. Hu and R. Xu, Drug Des., Dev. Ther., 2021, 15, 4503–4525 CrossRef CAS.
- I. Rinkunaite, E. Simoliunas, M. Alksne, D. Dapkute and V. Bukelskiene, BMC Complementary Med. Ther., 2021, 21, 39 CrossRef CAS PubMed.
- H. I. Rocha-Gonzalez, M. Ambriz-Tututi and V. Granados-Soto, CNS Neurosci. Ther., 2008, 14, 234–247 CrossRef CAS PubMed.
- T. Meng, D. Xiao, A. Muhammed, J. Deng, L. Chen and J. He, Molecules, 2021, 26(1), 229 CrossRef CAS PubMed.
- C. N. Serhan, S. Yacoubian and R. Yang, Annu. Rev. Pathol.: Mech. Dis., 2008, 3, 279–312 CrossRef CAS.
- M. Piotrowska, A. Binienda and J. Fichna, Mol. Cell. Endocrinol., 2021, 538, 111448 CrossRef CAS PubMed.
- B. Sears and C. Ricordi, J. Obes., 2011, 2011, 431985 Search PubMed.
- L. Panahipour, A. A. Tabatabaei and R. Gruber, J. Dairy Sci., 2020, 103, 6771–6781 CrossRef CAS PubMed.
- C. Anania, I. Martinelli, G. Brindisi, D. De Canditiis, G. De Castro, A. M. Zicari and F. Olivero, J. Clin. Med., 2022, 11, 4823 CrossRef CAS.
- G. D. Flora and M. K. Nayak, Curr. Pharm. Des., 2019, 25, 4063–4084 CrossRef CAS.
- R. Hajar, Heart Failure Rev., 2017, 18, 109–114 Search PubMed.
- Y. X. Zhou, H. Zhang and C. Peng, Front. Pharmacol, 2021, 12, 771793 CrossRef CAS PubMed.
- W. Shi, R. Yuan, X. Chen, Q. Xin, Y. Wang, X. Shang, W. Cong and K. Chen, Am. J. Chin. Med., 2019, 47, 19–38 CrossRef CAS PubMed.
- H. Wenjun, W. Jing, L. Tao, M. Ling, Y. Yan, Z. Xiaorong and Z. Rui, Med. Sci. Monit., 2015, 21, 1700–1706 CrossRef.
- A. Carrizzo, M. Ambrosio, A. Damato, M. Madonna, M. Storto, L. Capocci, P. Campiglia, E. Sommella, V. Trimarco, F. Rozza, R. Izzo, A. A. Puca and C. Vecchione, Mol. Nutr. Food Res., 2016, 60, 2304–2311 CrossRef CAS PubMed.
- L. Lv, Y. Yao, G. Zhao and G. Zhu, Exp. Ther. Med., 2018, 15, 506–512 CAS.
- L. Ding, C. Jia, Y. Zhang, W. Wang, W. Zhu, Y. Chen and T. Zhang, Biomed. Pharmacother., 2019, 111, 325–330 CrossRef CAS.
- L. Xin, J. Gao, H. Lin, Y. Qu, C. Shang, Y. Wang, Y. Lu and X. Cui, Front. Pharmacol, 2020, 11, 583200 CrossRef CAS PubMed.
- H. C. Kim, Glob. Adv. Health Med., 2021, 3, 134–141 CrossRef PubMed.
- A. E. Kitabchi, G. E. Umpierrez, J. M. Miles and J. N. Fisher, Diabetes Care, 2009, 32, 1335–1343 CrossRef CAS.
- I. Tuma, Vnitr. Lek., 2007, 53, 486–488 CAS.
- S. Padhi, A. K. Nayak and A. Behera, Biomed. Pharmacother., 2020, 131, 110708 CrossRef CAS PubMed.
- J. Long, Y. Guo, J. Yang, S. M. Henning, R. P. Lee, A. Rasmussen, L. Zhang, Q. Y. Lu, D. Heber and Z. Li, Food Funct., 2019, 10, 6582–6588 RSC.
- N. Fatima, R. M. Hafizur, A. Hameed, S. Ahmed, M. Nisar and N. Kabir, Eur. J. Nutr., 2017, 56, 591–601 CrossRef.
- A. Ahad, A. A. Ganai, M. Mujeeb and W. A. Siddiqui, Chem.-Biol. Interact., 2014, 219, 64–75 CrossRef CAS.
- M. Oza and Y. Kulkarni, Int. J. Pharma Sci. Res., 2016, 7, 14–24 CAS.
- S. J. Tsai, C. S. Huang, M. C. Mong, W. Y. Kam, H. Y. Huang and M. C. Yin, J. Agric. Food Chem., 2012, 60, 514–521 CrossRef CAS.
- J. Yin, H. Xing and J. Ye, Metabolism, 2008, 57, 712–717 CrossRef CAS PubMed.
- J. H. Zhang, J. F. Zhang, J. Song, Y. Bai, L. Deng, C. P. Feng, X. Y. Xu, H. X. Guo, Y. Wang, X. Gao, Y. Gu, C. Jin, J. F. Zheng, Z. Zhen and H. Su, Am. J. Chin. Med., 2021, 49, 1399–1415 CrossRef CAS.
- P. Tiwari, J. Diabetes Res., 2015, 2015, 340838 Search PubMed.
- J. Wang, C. Brown, C. Shi, J. Townsend, G. R. Gameiro, P. Wang and H. Jiang, Eye Vis., 2019, 6, 1–11 CrossRef CAS PubMed.
- M. Aghajanpour, M. R. Nazer, Z. Obeidavi, M. Akbari, P. Ezati and N. M. Kor, Am. J. Cancer Res., 2017, 7, 740–769 CAS.
- J. D. Hayes, A. T. Dinkova-Kostova and K. D. Tew, Cancer Cell, 2020, 38, 167–197 CrossRef CAS PubMed.
- B. Perillo, M. Di Donato, A. Pezone, E. Di Zazzo, P. Giovannelli, G. Galasso, G. Castoria and A. Migliaccio, Exp. Mol. Med., 2020, 52, 192–203 CrossRef CAS.
- T. Khaldi, N. Chekchaki, Z. Rouibah, K. Chouala, H. Cheniti, M. Boumendjel, F. Taibi, M. Messarah and A. Boumendjel, J. Toxicol. Environ. Health Sci., 2022, 14, 291–300 CrossRef.
- J. Hu, W. Lu, M. Lv, Y. Wang, R. Ding and L. Wang, Rev. Bras. Farmacogn., 2019, 29, 24–29 CrossRef CAS.
- B. P. Chew, J. S. Park, M. W. Wong and T. S. Wong, Anticancer Res., 1999, 19, 1849–1853 CAS.
- H. Kurihara, H. Koda, S. Asami, Y. Kiso and T. Tanaka, Life Sci., 2002, 70, 2509–2520 CrossRef CAS.
- H. Jyonouchi, S. Sun, K. Iijima and M. D. Gross, Nutr. Cancer, 2000, 36, 59–65 CrossRef CAS PubMed.
- T. Tanaka, M. Shnimizu and H. Moriwaki, Molecules, 2012, 17, 3202–3242 CrossRef CAS PubMed.
- P. Palozza, N. Maggiano, G. Calviello, P. Lanza, E. Piccioni, F. O. Ranelletti and G. M. Bartoli, Carcinogenesis, 1998, 19, 373–376 CrossRef CAS PubMed.
- M. M. Mathews-Roth and N. I. Krinsky, Photochem. Photobiol., 1985, 42, 35–38 CrossRef CAS PubMed.
- L. X. Zhang, R. V. Cooney and J. S. Bertram, Cancer Res., 1992, 52, 5707–5712 CAS.
- X. Zhang, Q. Wang, B. Neil and X. Chen, China Med. J., 2010, 123, 2231–2236 CAS.
- H. S. Kim, P. Bowen, L. Chen, C. Duncan, L. Ghosh, R. Sharifi and K. Christov, Nutr. Cancer, 2003, 47, 40–47 CrossRef CAS.
- A. Ronco, E. De Stefani, P. Boffetta, H. Deneo-Pellegrini, M. Mendilaharsu and F. Leborgne, Nutr. Cancer, 1999, 35, 111–119 CrossRef CAS.
- A. Iannone, C. Rota, S. Bergamini, A. Tomasi and L. M. Canfield, J. Biochem. Mol. Toxicol., 1998, 12, 299–304 CrossRef CAS.
-
P. August and B. M. Sibai, Clinical features and, diagnosis, accessed December 2022, https://www.uptodate.com/contents/preeclampsia-clinical-features-and-diagnosis Search PubMed.
- J. M. Roberts and C. Escudero, Pregnancy Hypertension, 2012, 2, 72–83 CrossRef PubMed.
- A. Nakimuli, S. Nakubulwa, O. Kakaire, M. O. Osinde, S. N. Mbalinda, N. Kakande, R. C. Nabirye and D. K. Kaye, BMC Pregnancy Childbirth, 2016, 16, 1–8 CrossRef.
- O. Api, C. Breyman, M. Çetiner, C. Demir and T. Ecder, Turk. J. Obstet. Gynecol., 2015, 12, 173–181 CrossRef.
- K. Kumar, A. N. Yadav, V. Kumar, P. Vyas and H. S. Dhaliwal, Bioresour. Bioprocess., 2017, 4, 18 CrossRef.
- A. Lama-Munoz and M. D. M. Contreras, Foods, 2022, 11(22), 3671 CrossRef CAS PubMed.
- Y. Zhang, Y. Liu, H. Liu and W. H. Tang, Cell Biosci., 2019, 9, 1–18 CrossRef.
- S. Gurung, D. Perocheau, L. Touramanidou and J. Baruteau, Cell Commun. Signaling, 2021, 19, 1–19 CrossRef PubMed.
- M. Alfieri, A. Leone and A. Ambrosone, Pharmaceutics, 2021, 13, 498 CrossRef CAS.
- G. Midekessa, K. Godakumara, J. Ord, J. Viil, F. Lättekivi, K. Dissanayake, S. Kopanchuk, A. Rinken, A. Andronowska and S. Bhattacharjee, ACS Omega, 2020, 5, 16701–16710 CrossRef CAS.
- E. Beit-Yannai, S. Tabak and W. D. Stamer, J. Cell. Mol. Med., 2018, 22, 2001–2006 CrossRef PubMed.
- Y. Zhang, J. Bi, J. Huang, Y. Tang, S. Du and P. Li, Int. J. Nanomed., 2020, 15, 6917 CrossRef CAS.
- T. Karamanidou and A. Tsouknidas, Int. J. Mol. Sci., 2021, 23, 191 CrossRef PubMed.
- H. Wei, Q. Chen, L. Lin, C. Sha, T. Li, Y. Liu, X. Yin, Y. Xu, L. Chen and W. Gao, Int. J. Biol. Sci., 2021, 17, 163 CrossRef CAS PubMed.
- S. Gurunathan, M.-H. Kang and J.-H. Kim, Int. J. Nanomed., 2021, 16, 1281 CrossRef PubMed.
- T. Skotland, K. Sandvig and A. Llorente, Prog. Lipid Res., 2017, 66, 30–41 CrossRef CAS PubMed.
- T. Skotland, N. P. Hessvik, K. Sandvig and A. Llorente, J. Lipid Res., 2019, 60, 9–18 CrossRef CAS PubMed.
- W. Zhang, X. Jiang, J. Bao, Y. Wang, H. Liu and L. Tang, Front. Immunol., 2018, 9, 90 CrossRef PubMed.
- M. Lu and Y. Huang, Biomaterials, 2020, 242, 119925 CrossRef CAS PubMed.
- Q. Shen, Z. Huang, J. Yao and Y. Jin, J. Adv. Res., 2022, 37, 221–233 CrossRef CAS PubMed.
- Y. Sun, H. Shi, S. Yin, C. Ji, X. Zhang, B. Zhang, P. Wu, Y. Shi, F. Mao, Y. Yan, W. Xu and H. Qian, ACS Nano, 2018, 12, 7613–7628 CrossRef CAS PubMed.
- J. Xiong, H. Hu, R. Guo, H. Wang and H. Jiang, Front. Endocrinol., 2021, 12, 646233 CrossRef PubMed.
- J. Y. Kim, W.-K. Rhim, Y.-I. Yoo, D.-S. Kim, K.-W. Ko, Y. Heo, C. G. Park and D. K. Han, J. Tissue Eng., 2021, 12, 20417314211008626 Search PubMed.
- F. H. Jansen, J. Krijgsveld, A. van Rijswijk, G.-J. van den Bemd, M. S. van den Berg, W. M. van Weerden, R. Willemsen, L. J. Dekker, T. M. Luider and G. Jenster, Mol. Cell. Proteomics, 2009, 8, 1192–1205 CrossRef CAS PubMed.
- V. Luga, L. Zhang, A. M. Viloria-Petit, A. A. Ogunjimi, M. R. Inanlou, E. Chiu, M. Buchanan, A. N. Hosein, M. Basik and J. L. Wrana, Cell, 2012, 151, 1542–1556 CrossRef CAS.
- H. Peinado, M. Alečković, S. Lavotshkin, I. Matei, B. Costa-Silva, G. Moreno-Bueno, M. Hergueta-Redondo, C. Williams, G. García-Santos and C. M. Ghajar, Nat. Med., 2012, 18, 883–891 CrossRef CAS PubMed.
- S. A. Melo, L. B. Luecke, C. Kahlert, A. F. Fernandez, S. T. Gammon, J. Kaye, V. S. LeBleu, E. A. Mittendorf, J. Weitz and N. Rahbari, Nature, 2015, 523, 177–182 CrossRef CAS.
- Y. Yoshioka, N. Kosaka, Y. Konishi, H. Ohta, H. Okamoto, H. Sonoda, R. Nonaka, H. Yamamoto, H. Ishii and M. Mori, Nat. Commun., 2014, 5, 3591 CrossRef PubMed.
- M.-W. Welker, D. Reichert, S. Susser, C. Sarrazin, Y. Martinez, E. Herrmann, S. Zeuzem, A. Piiper and B. Kronenberger, PLoS One, 2012, 7, e30796 CrossRef CAS PubMed.
- S. Saman, W. Kim, M. Raya, Y. Visnick, S. Miro, S. Saman, B. Jackson, A. C. McKee, V. E. Alvarez and N. C. Lee, J. Biol. Chem., 2012, 287, 3842–3849 CrossRef CAS PubMed.
- Z.-H. Zhao, Z.-T. Chen, R.-L. Zhou, X. Zhang, Q.-Y. Ye and Y.-Z. Wang, Front. Aging Neurosci., 2019, 10, 438 CrossRef PubMed.
- Y. Wang, M. Li, L. Chen, H. Bian, X. Chen, H. Zheng, P. Yang, Q. Chen and H. Xu, Signal Transduction Targeted Ther., 2021, 6, 409 CrossRef CAS.
- L.-P. Zhu, T. Tian, J.-Y. Wang, J.-N. He, T. Chen, M. Pan, L. Xu, H.-x. Zhang, X.-T. Qiu and C.-C. Li, Theranostics, 2018, 8, 6163 CrossRef CAS PubMed.
- J. Liu, M. Jiang, S. Deng, J. Lu, H. Huang, Y. Zhang, P. Gong, X. Shen, H. Ruan and M. Jin, Mol. Ther.–Nucleic Acids, 2018, 11, 103–115 CrossRef CAS.
- P. Kirave, P. Gondaliya, B. Kulkarni, R. Rawal, R. Garg, A. Jain and K. Kalia, Oncotarget, 2020, 11, 1157 CrossRef PubMed.
- G. Liang, S. Kan, Y. Zhu, S. Feng, W. Feng and S. Gao, Int. J. Nanomed., 2018, 13, 585 CrossRef CAS PubMed.
- D. Wang, X. Wang, Y. Song, M. Si, Y. Sun, X. Liu, S. Cui, X. Qu and X. Yu, Cell Death Dis., 2022, 13, 380 CrossRef CAS PubMed.
- C. Wang, J. Wang, W. Cui, Y. Liu, H. Zhou, Y. Wang, X. Chen, X. Chen and Z. Wang, OncoTargets Ther., 2021, 14, 1441 CrossRef PubMed.
- K. Kaban, C. Hinterleitner, Y. Zhou, E. Salva, A. G. Kantarci, H. R. Salih and M. Märklin, Cancers, 2021, 13, 2397 CrossRef CAS PubMed.
- K. A. Greco, C. A. Franzen, K. E. Foreman, R. C. Flanigan, P. C. Kuo and G. N. Gupta, Urology, 2016, 91, 241 CrossRef PubMed.
- S. Gurung, D. Perocheau, L. Touramanidou and J. Baruteau, Cell Commun. Signaling, 2021, 19, 47 CrossRef CAS PubMed.
- Q. Hu, H. Su, J. Li, C. Lyon, W. Tang, M. Wan and T. Y. Hu, Precis. Clin. Med., 2020, 3, 54–66 CrossRef PubMed.
- M. F. Tsan and B. Gao, J. Leukocyte Biol., 2009, 85, 905–910 CrossRef CAS PubMed.
- S. Samanta, S. Rajasingh, N. Drosos, Z. Zhou, B. Dawn and J. Rajasingh, Acta Pharmacol. Sin., 2018, 39, 501–513 CrossRef CAS PubMed.
- R. Mears, R. A. Craven, S. Hanrahan, N. Totty, C. Upton, S. L. Young, P. Patel, P. J. Selby and R. E. Banks, Proteomics, 2004, 4, 4019–4031 CrossRef CAS PubMed.
- S. Di Gioia, M. N. Hossain and M. Conese, Open Med., 2020, 15, 1096–1122 CrossRef CAS PubMed.
- J. Donoso-Quezada, S. Ayala-Mar and J. González-Valdez, Traffic, 2021, 22, 204–220 CrossRef CAS PubMed.
- S. Xie, Q. Zhang and L. Jiang, Membranes, 2022, 12, 498 CrossRef CAS PubMed.
- K. Segawa, S. Kurata, Y. Yanagihashi, T. R. Brummelkamp, F. Matsuda and S. Nagata, Science, 2014, 344, 1164–1168 CrossRef CAS PubMed.
-
S. E. Sedykh, E. E. Burkova, L. V. Purvinsh, D. A. Klemeshova, E. I. Ryabchikova and G. A. Nevinsky, Extracellular Vesicles and Their Importance in Human Health, 2020, pp. 1–28 Search PubMed.
- J. Donoso-Quezada, S. Ayala-Mar and J. Gonzalez-Valdez, Traffic, 2021, 22, 204–220 CrossRef CAS PubMed.
- F. Chen, N. Wang, H.-Y. Tan, W. Guo, C. Zhang and Y. Feng, Cancer Biol. Ther., 2019, 20, 583–592 CrossRef CAS PubMed.
- S. Mathivanan, H. Ji and R. J. Simpson, J. Proteomics, 2010, 73, 1907–1920 CrossRef CAS PubMed.
- J. Wang, B.-L. Yue, Y.-Z. Huang, X.-Y. Lan, W.-J. Liu and H. Chen, Int. J. Mol. Sci., 2022, 23, 2461 CrossRef CAS PubMed.
- M. Ahmadi and J. Rezaie, J. Transl. Med., 2020, 18, 1–17 CrossRef PubMed.
- Y. Wang, M. Li, L. Chen, H. Bian, X. Chen, H. Zheng, P. Yang, Q. Chen and H. Xu, Signal Transduction Targeted Ther., 2021, 6, 409 CrossRef CAS.
- L. P. Zhu, T. Tian, J. Y. Wang, J. N. He, T. Chen, M. Pan, L. Xu, H. X. Zhang, X. T. Qiu, C. C. Li, K. K. Wang, H. Shen, G. G. Zhang and Y. P. Bai, Theranostics, 2018, 8, 6163–6177 CrossRef CAS.
- X. F. Zhang, T. Wang, Z. X. Wang, K. P. Huang, Y. W. Zhang, G. L. Wang, H. J. Zhang, Z. H. Chen, C. Y. Wang, J. X. Zhang and H. Wang, Mol. Ther.–Nucleic Acids, 2021, 26, 347–359 CrossRef CAS PubMed.
- J. Liu, M. Jiang, S. Deng, J. Lu, H. Huang, Y. Zhang, P. Gong, X. Shen, H. Ruan, M. Jin and H. Wang, Mol. Ther.–Nucleic Acids, 2018, 11, 103–115 CrossRef CAS.
-
S. Baron, Medical microbiology, University of Texas Medical Branch at Galveston, Galveston (TX), 4th edn, 1996 Search PubMed.
- S. Mostowy and A. R. Shenoy, Nat. Rev. Immunol., 2015, 15, 559–573 CrossRef CAS PubMed.
- K. K. Ayyar and A. C. Moss, Front. Pharmacol, 2021, 12, 1295 Search PubMed.
- J. Munir, M. Lee and S. Ryu, Adv. Nutr., 2020, 11, 687–696 CrossRef PubMed.
- R. Anusha and S. Priya, Mol. Nutr. Food Res., 2022, 66, 2200142 CrossRef CAS PubMed.
- M. De Robertis, A. Sarra, V. D’oria, F. Mura, F. Bordi, P. Postorino and D. Fratantonio, Biomolecules, 2020, 10, 742 CrossRef CAS PubMed.
- B. Wang, X. Zhuang, Z.-B. Deng, H. Jiang, J. Mu, Q. Wang, X. Xiang, H. Guo, L. Zhang and G. Dryden, Mol. Ther., 2014, 22, 522–534 CrossRef CAS.
- X. Zhuang, Z.-B. Deng, J. Mu, L. Zhang, J. Yan, D. Miller, W. Feng, C. J. McClain and H.-G. Zhang, J. Extracell. Vesicles, 2015, 4, 28713 CrossRef PubMed.
- G. Ahn, Y.-H. Kim and J.-Y. Ahn, Nanoscale Adv., 2021, 3, 528–537 RSC.
- Z. Deng, Y. Rong, Y. Teng, J. Mu, X. Zhuang, M. Tseng, A. Samykutty, L. Zhang, J. Yan and D. Miller, Mol. Ther., 2017, 25, 1641–1654 CrossRef CAS PubMed.
- A. A. Badawy, M. A. El-Magd and S. A. AlSadrah, Integr. Cancer Ther., 2018, 17, 1235–1246 CrossRef CAS PubMed.
- X. Chen, B. Liu, X. Li, T. T. An, Y. Zhou, G. Li, J. Wu-Smart, S. Alvarez, M. J. Naldrett, J. Eudy, G. Kubik, R. A. Wilson, S. D. Kachman, J. Cui and J. Yu, J. Extracell. Vesicles, 2021, 10, e12069 CrossRef CAS PubMed.
- V. Iebba, V. Totino, A. Gagliardi, F. Santangelo, F. Cacciotti, M. Trancassini, C. Mancini, C. Cicerone, E. Corazziari and F. Pantanella, New Microbiol., 2016, 39, 1–12 CAS.
- H. E. Al-Rashidi, Saudi J. Biol. Sci., 2022, 29, 1628–1643 CrossRef CAS PubMed.
- W. Stremmel, R. Weiskirchen and B. C. Melnik, Inflamm. Bowel Dis., 2020, 5, 117–123 Search PubMed.
- J. Aarts, A. Boleij, B. C. Pieters, A. L. Feitsma, R. J. van Neerven, J. P. Ten Klooster, L. M'Rabet, O. J. Arntz, M. I. Koenders and F. A. van de Loo, Front. Immunol., 2021, 12, 703277 CrossRef CAS PubMed.
- M. Potesta, V. Roglia, M. Fanelli, E. Pietrobono, A. Gismondi, S. Vumbaca, R. G. Nguedia Tsangueu, A. Canini, V. Colizzi, S. Grelli, A. Minutolo and C. Montesano, Cell Death Discovery, 2020, 6, 43 CrossRef CAS.
- A. A. Badawy, M. A. El-Magd and S. A. AlSadrah, Integr. Cancer Ther., 2018, 17, 1235–1246 CrossRef CAS PubMed.
- A. A. Badawy, R. Q. A. Othman and M. A. El-Magd, Mol. Cell. Toxicol., 2021 DOI:10.1007/s13273-021-00163-4.
- S. Raimondo, F. Naselli, S. Fontana, F. Monteleone, A. L. Dico, L. Saieva, G. Zito, A. Flugy, M. Manno and M. A. Di Bella, Oncotarget, 2015, 6, 19514 CrossRef PubMed.
- X. Chen, Y. Zhou and J. Yu, Mol. Pharmaceutics, 2019, 16, 2690–2699 CrossRef CAS PubMed.
- A. Mokarizadeh, K. Hassanzadeh, M. Abdi, H. Soraya, M. R. Faryabi, E. Mohammadi and A. Ahmadi, Med. Hypotheses, 2015, 85, 141–144 CrossRef CAS PubMed.
- H. Kim, D. E. Kim, G. Han, N. R. Lim, E. H. Kim, Y. Jang, H. Cho, H. Jang, K. H. Kim and S. H. Kim, Adv. Healthcare Mater., 2022, 11, 2102027 CrossRef CAS PubMed.
- X. Chen, B. Liu, X. Li, T. T. An, Y. Zhou, G. Li, J. Wu-Smart, S. Alvarez, M. J. Naldrett and J. Eudy, J. Extracell. Vesicles, 2021, 10, e12069 CrossRef CAS PubMed.
- Q. Wang, X. Zhuang, J. Mu, Z.-B. Deng, H. Jiang, L. Zhang, X. Xiang, B. Wang, J. Yan and D. Miller, Nat. Commun., 2013, 4, 1867 CrossRef PubMed.
- X. Zhuang, Y. Teng, A. Samykutty, J. Mu, Z. Deng, L. Zhang, P. Cao, Y. Rong, J. Yan and D. Miller, Mol. Ther., 2016, 24, 96–105 CrossRef CAS PubMed.
- S. Ju, J. Mu, T. Dokland, X. Zhuang, Q. Wang, H. Jiang, X. Xiang, Z.-B. Deng, B. Wang and L. Zhang, Mol. Ther., 2013, 21, 1345–1357 CrossRef CAS PubMed.
- M. R. Ghiasi, E. Rahimi, Z. Amirkhani and R. Salehi, Adv. Biomed. Res., 2018, 7, 125 CrossRef PubMed.
- Z. Li, H. Wang, H. Yin, C. Bennett, H.-g. Zhang and P. Guo, Sci. Rep., 2018, 8, 14644 CrossRef PubMed.
- M. Zhang, B. Xiao, H. Wang, M. K. Han, Z. Zhang, E. Viennois, C. Xu and D. Merlin, Mol. Ther., 2016, 24, 1783–1796 CrossRef CAS PubMed.
- M. Zhang, X. Wang, M. K. Han, J. F. Collins and D. Merlin, Nanomedicine, 2017, 12, 1927–1943 CrossRef CAS PubMed.
- Y. Teng, Y. Ren, M. Sayed, X. Hu, C. Lei, A. Kumar, E. Hutchins, J. Mu, Z. Deng and C. Luo, Cell Host Microbe, 2018, 24, 637–652 CrossRef CAS PubMed.
- M. Zhang, E. Viennois, M. Prasad, Y. Zhang, L. Wang, Z. Zhang, M. K. Han, B. Xiao, C. Xu and S. Srinivasan, Biomaterials, 2016, 101, 321–340 CrossRef CAS.
- X. Wang, M. Zhang, S. R. Flores, R. R. Woloshun, C. Yang, L. Yin, P. Xiang, X. Xu, M. D. Garrick and S. Vidyasagar, Mol. Ther., 2019, 27, 493–506 CrossRef CAS PubMed.
- M. Vashisht, P. Rani, S. K. Onteru and D. Singh, Appl. Biochem. Biotechnol., 2017, 183, 993–1007 CrossRef CAS PubMed.
- R. Munagala, F. Aqil, J. Jeyabalan, A. K. Agrawal, A. M. Mudd, A. H. Kyakulaga, I. P. Singh, M. V. Vadhanam and R. C. Gupta, Cancer Lett., 2017, 393, 94–102 CrossRef CAS PubMed.
- Q. Zhang, Q. Xiao, H. Yin, C. Xia, Y. Pu, Z. He, Q. Hu, J. Wang and Y. Wang, RSC Adv., 2020, 10, 28314–28323 RSC.
- R. Munagala, F. Aqil, J. Jeyabalan and R. C. Gupta, Cancer Lett., 2016, 371, 48–61 CrossRef CAS PubMed.
- B. Li, A. Hock, R. Y. Wu, A. Minich, S. R. Botts, C. Lee, L. Antounians, H. Miyake, Y. Koike and Y. Chen, PLoS One, 2019, 14, e0211431 CrossRef CAS.
- B. C. Pieters, O. J. Arntz, J. Aarts, A. L. Feitsma, R. J. van Neerven, P. M. van der Kraan, M. C. Oliveira and F. A. van de Loo, Mol. Nutr. Food Res., 2022, 66, 2100764 CrossRef CAS.
- M. Potestà, V. Roglia, M. Fanelli, E. Pietrobono, A. Gismondi, S. Vumbaca, R. G. Nguedia Tsangueu, A. Canini, V. Colizzi and S. Grelli, Cell Death Discovery, 2020, 6, 43 CrossRef PubMed.
- M. Hazawa, K. Tomiyama, A. Saotome-Nakamura, C. Obara, T. Yasuda, T. Gotoh, I. Tanaka, H. Yakumaru, H. Ishihara and K. Tajima, Biochem. Biophys. Res. Commun., 2014, 446, 1165–1171 CrossRef CAS PubMed.
|
This journal is © The Royal Society of Chemistry 2024 |