DOI:
10.1039/D4CC00361F
(Communication)
Chem. Commun., 2024,
60, 5423-5426
Fluorescent coumarin-alkynes for labeling of amino acids and peptides via manganese(I)-catalyzed C–H alkenylation†
Received
24th January 2024
, Accepted 19th April 2024
First published on 22nd April 2024
Abstract
The late-stage fluorescent labeling of structurally complex peptides bears immense potential for molecular imaging. Herein, we report on a manganese(I)-catalyzed peptide C–H alkenylation under exceedingly mild conditions with natural fluorophores as coumarin- and chromone-derivatives. The robustness and efficiency of the manganese(I) catalysis regime was reflected by a broad functional group tolerance and low catalyst loading in a resource- and atom-economical fashion.
Cell-imaging, medicinal chemistry and cellular biochemistry are in high demand in selective techniques for tracking bioactive molecules, among others.1 Traditional labeling methods based on isotope probes, photo switchers or electrochemical sensors suffer from low sensitivity in comparison to fluorescent-labeling.1c In recent decades, the demand for small molecule fluorophores to label proteins with minimal disruption to the natural cellular mechanism has gained considerable attention.2 Coumarin and its constitutional isomer chromone are ideal fluorescent labels to study chemical and biochemical activities. Those second metabolites are not giving characteristics of smell and color in plants such as tonka beans or rue, but serve as defense mechanisms against predators in organisms such as bacteria, fungi and even sponges.3 The ability to form noncovalent interactions with enzymes and receptors unlocks a wide range of biochemical applications, including their anti-inflammatory and antithrombotic activities, as exemplified by Warfarin, an oral coagulant and rodenticide.4 Based on their outstanding biocompatibility and low toxicity, coumarins are used as fluorescent sensors to address biological systems or probes for NIR.5 Fluorogenic molecular techniques have been recognized as potent and environmentally sensitive tools in cells.6
Moreover, transition-metal-catalyzed C–H activation has been established as an indispensable toolbox in molecular synthesis over the past few decades.7 In this regard, Lavilla/Albericio,8 Chen,9 Yu10 and Ackermann,6,11 among others,12 have developed fundamental strategies for the late-stage diversification of amino acids and peptides.
While this regime is often associated with toxic and costly palladium- and rhodium-catalysts, accompanied by undesirable metal impurities, cost-efficient, abundant 3d metal catalysis,1e,13 such as resource-friendly and nontoxic manganese catalysis, has recently gained considerable attention (Scheme 1).14
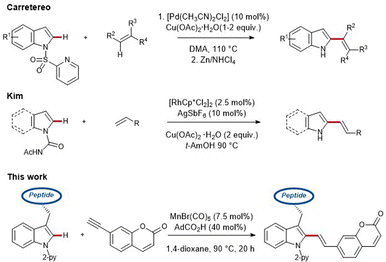 |
| Scheme 1 Selected catalytic alkenylation examples. | |
As part of our research program on sustainable C–H activation, we now report on the first manganese(I)-catalyzed C–H alkenylation with easily accessible coumarin-derivatives for labeling structurally complex peptides.
We initiated our studies by probing various reaction conditions for the envisioned manganese-catalyzed labeling of tryptophan containing peptides with coumarin. The optimal reaction conditions for the C–H alkenylation are 40 mol% of AdCO2H in 1,4-dioxane with 7.5 mol% MnBr(CO)5 (Table 1, entry 1). It was shown that the reaction time has a considerable impact on the reaction outcome (entries 2 and 3). Increasing the reaction temperature to 120 °C did not have an influence on the efficiency (entry 4). However, reducing the temperature to a mild 55 °C, albeit with an increased reaction time, yielded the product in 79% yield (entry 5).
Table 1 Optimization table
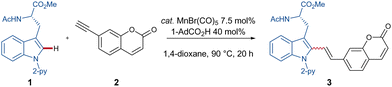
|
Entry |
Deviation from standard conditions |
Yieldab [%] |
General reaction conditions: 1 (0.10 mmol), 2 (0.11 mmol), [Mn] (7.5 mol%), 1-AdCO2H (40 mol%), 1,4-dioxane (1.0 mL), 90 °C, 20 h, under N2-atmosphere. Of isolated products. E/Z ratio = >9/1 determined with 1H-NMR. DCE: 1,2-dichloroethane. |
1b |
— |
92 |
2 |
12 h |
53 |
3 |
16 h |
66 |
4 |
120 °C |
91 |
5 |
55 °C, 71 h |
79 |
6 |
NaOAc instead of AdCO2H |
42 |
7 |
KOAc instead of AdCO2H |
33 |
8 |
5 mol% MnBr(CO)5 |
82 |
9 |
2.5 mol% MnBr(CO)5 |
68 |
10 |
Without MnBr(CO)5 |
nd |
11 |
DCE |
69 |
Altering the additives from AdCO2H to NaOAc (entry 6) or KOAc (entry 7) led to a diminished performance. Importantly, the efficiency of the manganese catalyst was demonstrated by reducing the loading to 5 mol% (entry 8) and 2.5 mol% (entry 9), with product formation in 82% and 68% yield. Control experiments verified the need for the catalyst for the reaction to proceed (entry 10). Additionally, the use of DCE has shown no impact on the E/Z ratio (entry 11).
With these optimized reaction conditions in hand, the compatibility of the coumarin-alkyne 2 was probed (Scheme 2). Here, it was shown that the ratio of E/Z was influenced by the properties of the corresponding peptide backbone. The threonine containing dipeptide 7 has shown the highest E/Z ratio of over >9/1. The chromone-labeled compounds 9 and 10 gave E/Z ratios of 9/1 and 8/2, and a linear correlation of alkyne-length to the E/Z ratio was not observed. Isomerization studies were conducted with compounds 3 and 10 to evaluate the influence of UV-light on the E/Z ratio (see Scheme 3, for more information see ESI†). To better understand the catalyst mode of action, DFT calculations were carried out at the PW6B95-D4/def2-TZVP+SMD(1,4-dioxane)//TPSS-D3(BJ)/def2-SVP level of theory11f between C–H activation and the proto-demetalation steps (see ESI† for further information). With this knowledge in hand, we tried to overcome the obstacle by exploiting a coumarin-alkyne 11 with a small linker, in this case a CH2-group. Under the optimized conditions (Table 1), similar good to excellent yields have been isolated (Scheme 4). Different protecting groups of the C- and N-termini as well as a different directing group were tolerated, and the various functional groups of the peptide-backbone like the free indole of the tryptophan did not compromise the yield.
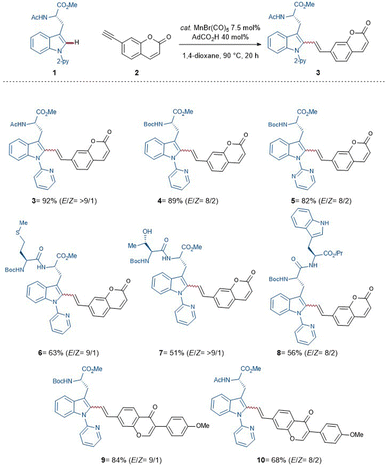 |
| Scheme 2 Alkenylation substrate scope of coumarins and chromones. | |
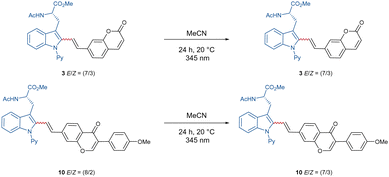 |
| Scheme 3 Isomerization studies. | |
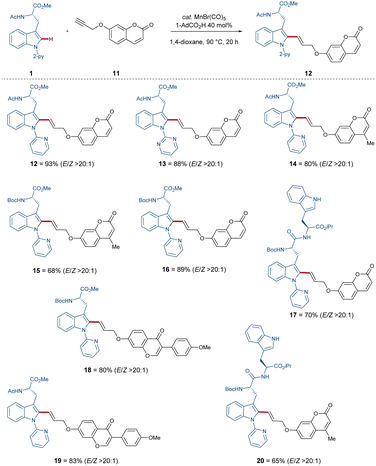 |
| Scheme 4 Expanded substrate scope of coumarins and chromones. | |
Indeed, with this procedure, excellent E/Z ratios for all compounds (Scheme 4) of >20
:
1 were accomplished.
Due to the sensitive nature of the push–pull alkyne motif, previously established protocols have thus far proven ineffective for the removal of the pyridine directing group (for further information, see the ESI†). Furthermore, fluorescent studies showed emission wavelengths of λem. = 426 nm, λem. = 396 nm and λem. = 443 nm for products 3, 10 and 11, respectively, within an excitation wavelength of λexc. = 330 nm (see Fig. 1).
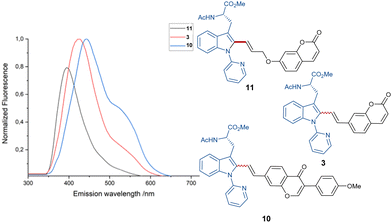 |
| Fig. 1 Fluorescence studies of compounds 3, 10, and 11. | |
Based on the simplicity of the core-structure and the easy tunability, plenty of opportunities regarding dye characteristics in the case of stoke fluorescence become available.
In summary, we have developed an efficient, site selective manganese(I) catalyzed C–H alkenylation, which enabled labeling of tryptophan-containing-peptides with coumarin and chromone probes, thus expanding the toolbox of fluorescent probes to image living cells in real-time.
A. K., and L. A. conceived the project and wrote the manuscript. A. K. performed the synthetic experiments. T. O. performed the DFT calculations.
We gratefully acknowledge the support from the DFG Gottfried Wilhelm Leibniz award (L. A.), DZHK (PI at German Centre for Cardiovascular Research), Werner Siemens-Stiftung (WSS), and the European Union's Horizon 2020 research and innovation programme (Marie Skłodowska-Curie Grant Agreement No. 860762) to T. O.
Conflicts of interest
There are no conflicts to declare.
Notes and references
-
(a) M. Shadmehr, G. J. Davis, B. T. Mehari, S. M. Jensen and J. C. Jewett, ChemBioChem, 2018, 19, 2550–2552 CrossRef CAS PubMed;
(b) M. Muttenthaler, G. F. King, D. J. Adams and P. F. Alewood, Nat. Rev. Drug Discovery, 2021, 20, 309–325 CrossRef CAS PubMed;
(c)
A. Pereira, S. Martins and A. T. Caldeira, Phytochemicals in Human Health, IntechOpen, Rijeka, 2019, ch. 8 Search PubMed;
(d) Z. Guo, S. Park, J. Yoon and I. Shin, Chem. Soc. Rev., 2014, 43, 16–29 RSC;
(e) S. Sharma, S. Han, M. Kim, N. K. Mishra, J. Park, Y. Shin, J. Ha, J. H. Kwak, Y. H. Jung and I. S. Kim, Org. Biomol. Chem., 2014, 12, 1703–1706 RSC;
(f) Y. Zhao, Y. Shen, J. He, J. Li, S. Zhou, Y. Wang, C. Li and H. Liu, Chem. – Eur. J., 2023, 29, e202301878 CrossRef CAS PubMed;
(g) H. J. Bell and L. R. Malins, Org. Biomol. Chem., 2022, 20, 6250–6256 RSC;
(h) Y. Li, Y. Ma, Y. Xia, T. Zhang, S. Sun, J. Gao, H. Yao and H. Wang, Nat. Commun., 2023, 14, 2944 CrossRef CAS PubMed.
- C. Uttamapinant, K. A. White, H. Baruah, S. Thompson, M. Fernández-Suárez, S. Puthenveetil and A. Y. Ting, Proc. Natl. Acad. Sci. U. S. A., 2010, 107, 10914–10919 CrossRef CAS PubMed.
-
(a) X.-Y. Sun, T. Liu, J. Sun and X.-J. Wang, RSC Adv., 2020, 10, 10826–10847 RSC;
(b) R. Ananthakrishnan, P. Chandra, B. Kumar and K. B. Rameshkumar, Int. J. Food Prop., 2018, 21, 50–57 CrossRef CAS.
-
(a) J. D. Hadjipavlou-Litina, E. K. Litinas and C. Kontogiorgis, Anti-Inflammatory Anti-Allergy Agents Med. Chem., 2007, 6, 293–306 CrossRef;
(b) R. K. Wadhera, C. E. Russell and G. Piazza, Circulation, 2014, 130, e191–e193 CrossRef PubMed.
-
(a) S. J. Sharma and N. Sekar, Dyes Pigm., 2022, 202, 110306 CrossRef CAS;
(b) J. Li, L. Tu, Q. Ouyang, S.-G. Yang, X. Liu, Q. Zhai, Y. Sun, J. Yoon and H. Teng, J. Mater. Chem. B, 2022, 10, 1427–1433 RSC.
- N. Kaplaneris, F. Kaltenhäuser, G. Sirvinskaite, S. Fan, T. De Oliveira, L. C. Conradi and L. Ackermann, Sci. Adv., 2021, 9, eabe6202 CrossRef PubMed.
-
(a) B. Zhao, T. Rogge, L. Ackermann and Z. Shi, Chem. Soc. Rev., 2021, 50, 8903–8953 RSC;
(b) L. Guillemard, N. Kaplaneris, L. Ackermann and M. J. Johansson, Nat. Rev. Chem., 2021, 5, 522–545 CrossRef CAS PubMed;
(c) T. Rogge, N. Kaplaneris, N. Chatani, J. Kim, S. Chang, B. Punji, L. L. Schafer, D. G. Musaev, J. Wencel-Delord, C. A. Roberts, R. Sarpong, Z. E. Wilson, M. A. Brimble, M. J. Johansson and L. Ackermann, Nat. Rev. Methods Primers, 2021, 1, 43 CrossRef CAS;
(d) P. Gandeepan, L. H. Finger, T. H. Meyer and L. Ackermann, Chem. Soc. Rev., 2020, 49, 4254–4272 RSC;
(e) P. Gandeepan, T. Müller, D. Zell, G. Cera, S. Warratz and L. Ackermann, Chem. Rev., 2019, 119, 2192–2452 CrossRef CAS PubMed;
(f) Y. Wei, P. Hu, M. Zhang and W. Su, Chem. Rev., 2017, 117, 8864–8907 CrossRef CAS PubMed;
(g) M. M. Lorion, K. Maindan, A. R. Kapdi and L. Ackermann, Chem. Soc. Rev., 2017, 46, 7399–7420 RSC;
(h) J. A. Leitch and C. G. Frost, Chem. Soc. Rev., 2017, 46, 7145–7153 RSC;
(i) J. He, M. Wasa, K. S. L. Chan, Q. Shao and J. Q. Yu, Chem. Rev., 2017, 117, 8754–8786 CrossRef CAS PubMed;
(j) B. Ye and N. Cramer, Acc. Chem. Res., 2015, 48, 1308–1318 CrossRef CAS PubMed;
(k) J. Wencel-Delord and F. Glorius, Nat. Chem., 2013, 5, 369–375 CrossRef CAS PubMed.
-
(a) R. Subiros-Funosas, V. C. L. Ho, N. D. Barth, L. Mendive-Tapia, M. Pappalardo, X. Barril, R. Ma, C.-B. Zhang, B.-Z. Qian, M. Sintes, O. Ghashghaei, R. Lavilla and M. Vendrell, Chem. Sci., 2020, 11, 1368–1374 RSC;
(b) R. Subiros-Funosas, L. Mendive-Tapia, J. Sot, J. D. Pound, N. Barth, Y. Varela, F. M. Goñi, M. Paterson, C. D. Gregory, F. Albericio, I. Dransfield, R. Lavilla and M. Vendrell, Chem. Commun., 2017, 53, 945–948 RSC;
(c) L. Mendive-Tapia, A. Albornoz-Grados, A. Bertran, F. Albericio and R. Lavilla, Chem. Commun., 2017, 53, 2740–2743 RSC;
(d) S. Preciado, L. Mendive-Tapia, F. Albericio and R. Lavilla, J. Org. Chem., 2013, 78, 8129–8135 CrossRef CAS PubMed;
(e) J. Ruiz-Rodríguez, F. Albericio and R. Lavilla, Chem. – Eur. J., 2010, 16, 1124–1127 CrossRef PubMed.
-
(a) X. Chen, F. Ye, X. Luo, X. Liu, J. Zhao, S. Wang, Q. Zhou, G. Chen and P. Wang, J. Am. Chem. Soc., 2019, 141, 18230–18237 CrossRef CAS PubMed;
(b) X. Zhang, G. Lu, M. Sun, M. Mahankali, Y. Ma, M. Zhang, W. Hua, Y. Hu, Q. Wang, J. Chen, G. He, X. Qi, W. Shen, P. Liu and G. Chen, Nat. Chem., 2018, 10, 540–548 CrossRef CAS PubMed;
(c) G. He, B. Wang, W. A. Nack and G. Chen, Acc. Chem. Res., 2016, 49, 635–645 CrossRef CAS PubMed;
(d) S.-Y. Zhang, Q. Li, G. He, W. A. Nack and G. Chen, J. Am. Chem. Soc., 2013, 135, 12135–12141 CrossRef CAS PubMed.
-
(a) T. Liu, J. X. Qiao, M. A. Poss and J. Q. Yu, Angew. Chem., Int. Ed., 2017, 56, 10924–10927 CrossRef CAS PubMed;
(b) Q.-F. Wu, P.-X. Shen, J. He, X.-B. Wang, F. Zhang, Q. Shao, R.-Y. Zhu, C. Mapelli, J. X. Qiao, M. A. Poss and J.-Q. Yu, Science, 2017, 355, 499–503 CrossRef CAS PubMed;
(c) J. He, S. Li, Y. Deng, H. Fu, B. N. Laforteza, J. E. Spangler, A. Homs and J. Q. Yu, Science, 2014, 343, 1216–1220 CrossRef CAS PubMed;
(d) W. Gong, G. Zhang, T. Liu, R. Giri and J.-Q. Yu, J. Am. Chem. Soc., 2014, 136, 16940–16946 CrossRef CAS PubMed.
-
(a) T. Oyama, L. Mendive-Tapia, V. Cowell, A. Kopp, M. Vendrell and L. Ackermann, Chem. Sci., 2023, 14, 5728–5733 RSC;
(b) M. Stangier, A. M. Messinis, J. C. A. Oliveira, H. Yu and L. Ackermann, Nat. Commun., 2021, 12, 4736 CrossRef CAS PubMed;
(c) R. N. Lima, J. A. C. Delgado, D. I. Bernardi, R. G. S. Berlinck, N. Kaplaneris, L. Ackermann and M. W. Paixão, Chem. Commun., 2021, 57, 5758–5761 RSC;
(d) W. Wang, J. Wu, R. Kuniyil, A. Kopp, R. N. Lima and L. Ackermann, Chem, 2020, 6, 3428–3439 CrossRef CAS;
(e) A. Schischko, N. Kaplaneris, T. Rogge, G. Sirvinskaite, J. Son and L. Ackermann, Nat. Commun., 2019, 10, 3553 CrossRef PubMed;
(f) N. Kaplaneris, T. Rogge, R. Yin, H. Wang, G. Sirvinskaite and L. Ackermann, Angew. Chem., Int. Ed., 2019, 58, 3476–3480 CrossRef CAS PubMed;
(g) M. M. Lorion, N. Kaplaneris, J. Son, R. Kuniyil and L. Ackermann, Angew. Chem., Int. Ed., 2019, 58, 1684–1688 CrossRef CAS PubMed;
(h) W. Wang, P. Subramanian, O. Martinazzoli, J. Wu and L. Ackermann, Chem. – Eur. J., 2019, 25, 10585–10589 CrossRef CAS PubMed;
(i) M. Bauer, W. Wang, M. M. Lorion, C. Dong and L. Ackermann, Angew. Chem., Int. Ed., 2018, 57, 203–207 CrossRef CAS PubMed;
(j) C. S. Wang, P. H. Dixneuf and J. F. Soule, Chem. Rev., 2018, 118, 7532–7585 CrossRef CAS PubMed;
(k) A. Schischko, H. Ren, N. Kaplaneris and L. Ackermann, Angew. Chem., Int. Ed., 2017, 56, 1576–1580 CrossRef CAS PubMed.
-
(a) M. San Segundo, I. Guerrero and A. Correa, Org. Lett., 2017, 19, 5288–5291 CrossRef CAS PubMed;
(b) A. J. Reay, L. A. Hammarback, J. T. W. Bray, T. Sheridan, D. Turnbull, A. C. Whitwood and I. J. S. Fairlamb, ACS Catal., 2017, 7, 5174–5179 CrossRef CAS PubMed;
(c) G. L. Tolnai, J. P. Brand and J. Waser, Beilstein J. Org. Chem., 2016, 12, 745–749 CrossRef CAS PubMed;
(d) T. J. Osberger, D. C. Rogness, J. T. Kohrt, A. F. Stepan and M. C. White, Nature, 2016, 537, 214–219 CrossRef CAS PubMed;
(e) B. Mondal, B. Roy and U. Kazmaier, J. Org. Chem., 2016, 81, 11646–11655 CrossRef CAS PubMed;
(f) L. D. Tran and O. Daugulis, Angew. Chem., Int. Ed., 2012, 51, 5188–5191 CrossRef CAS PubMed;
(g) H. Dong, C. Limberakis, S. Liras, D. Price and K. James, Chem. Commun., 2012, 48, 11644–11646 RSC.
- A. García-Rubia, R. Gómez Arrayás and J. C. Carretero, Angew. Chem., Int. Ed., 2009, 48, 6511–6515 CrossRef PubMed.
-
(a) N. Kaplaneris and L. Ackermann, Beilstein J. Org. Chem., 2022, 18, 86–88 CrossRef CAS PubMed;
(b) Y. Hu and C. Wang, ChemCatChem, 2019, 11, 1167–1174 CrossRef CAS;
(c) W. Liu and L. Ackermann, ACS Catal., 2016, 6, 3743–3752 CrossRef CAS;
(d) B. Zhou, H. Chen and C. Wang, J. Am. Chem. Soc., 2013, 135, 1264–1267 CrossRef CAS PubMed.
|
This journal is © The Royal Society of Chemistry 2024 |