DOI:
10.1039/D2GC04347E
(Paper)
Green Chem., 2023,
25, 1884-1897
Combining eutectic solvents and pressurized liquid extraction coupled in-line with solid-phase extraction to recover, purify and stabilize anthocyanins from Brazilian berry waste†
Received
17th November 2022
, Accepted 8th February 2023
First published on 21st February 2023
Abstract
Pressurized techniques are straightforward for high-scale applications and highly controllable, which seems an excellent strategy for recovering unstable natural compounds. In this work, the main advance was the development of a platform based on the pressurized liquid extraction coupled in-line with a solid-phase extraction step (PLE-SPE) combined with the use of eutectic mixtures as solvents to promote an efficient extraction and purification of natural pigments from food wastes. Eutectic mixtures, conventionally known as (deep) eutectic solvents – (D)ES, are combinations of two or more substances with a lower melting point than any of their components. (D)ES are often referred as “green solvents” because they can potentially be more environmentally friendly than other solvents, especially volatile organic solvents (VOSs). Overall, (D)ES have the potential to contribute to the achievement of several of the SDGs (especially 3, 13, and 14) through their positive impacts on health, environment, and sustainable production and consumption practices. Thus, in this work, (D)ES were used as solvents to valorize Brazilian berry waste (Plinia cauliflora). Anthocyanins are the biomass's main compounds of commercial interest, mainly for food and cosmetic applications. However, there are several technological issues regarding color control due to their high sensitivity to light, heat, oxygen, and pH variations. Thus, the data achieved in this work highlighted the high efficiency and low environmental footprint of the PLE-SPE-(D)ES platform developed. The success of the downstream process here developed was proved by the high extraction efficiency and the purity level of the anthocyanins obtained. Besides, thermal stability analysis was evaluated, demonstrating that (D)ES are not only solvents but also stabilizing agents, improving the shelf-life of the extracted colorants.
Introduction
The sustainable development goals (SDGs) were created to define strategies to face and mitigate economic, political, and environmental problems.1 The SDGs 2 (zero hunger) and 12 (responsible consumption and production) are correlated in one target challenge: creating new and efficient strategies to minimize food waste, and to valorize the inevitable waste and the by-products of food production and processing. Nowadays 33% of the food produced worldwide is wasted or misused, showing that the way we produce and consume foods is predatory to the environment and social well-being, reinforcing the need to develop new food and additive sources. Concerning wasted foods, fruits and vegetables contribute approximately 50% of the total amount, generating substantial environmental, social, and health impacts.2 Composing the fruits and vegetables’ wastes are several bioactive compounds with a high commercial interest and industrial demand, namely the colorants. Currently, only 16 natural colorants are commercially used in the food industry.3 Given their higher cost, natural pigments are only applied when synthetic pigments are restricted.4 Volatile organic solvents (VOSs), like methanol, acetone, and ethanol, are the primary choice as solvent media for their production. However, there are some problems associated with this, (i) the organic solvents present a low selectivity with high rates of undesirable compounds being also extracted (mainly macronutrients and ions that usually impair the biological effect of the extract and their color uniformity), and (ii) natural colorants recovered with organic solvents do not present a high thermal/chemical/optical stability.5 Thus, alternative solvents have been used to mitigate such drawbacks. Usually, non-volatile alternative solvents like eutectic solvents, ionic liquids, aqueous solutions of surfactants, and edible oils have been preferred to replace VOSs in the extraction of natural pigments.5 However, although researchers have already demonstrated a compelling performance of these alternative solvents, industrially, they are still not regularly used due to some limitations and restrictions in some fields.6
Eutectic solvents (mostly known as deep eutectic solvents – (D)ES) are mixtures composed of at least one hydrogen-bond acceptor (HBA) and one hydrogen-bond donor (HBD), which establish stronger hydrogen bonds than those present in pure compounds, leading to significant depression of their melting points.7 These eutectic mixtures are considered as designer solvents due to the numerous starting materials that can be used to produce them. Besides, these solvents usually have low toxicity and non-flammability, representing a significant advantage compared to VOSs. Besides, (D)ES have the potential to contribute for the effort of achieving the SDG 3 (good health and well-being), SDG 13 (climate action), and SDG 14 (life below water). Some examples are demonstrating the use of eutectic mixtures investigated as solvents to recover many natural colorants, including carotenoids, chlorophylls, pigmented proteins, and anthocyanins.8–10 Anthocyanins are one of the largest groups of natural colorants from wasted food fruits.11 Despite the high commercial potential of anthocyanins, these are still poorly explored in new formulations due to their high sensitivity to light, heat, oxygen, and pH variations.12,13 Thus, a good advantage could be achieved if a solvent could be designed to extract and stabilize the extracted anthocyanins simultaneously. In this sense, natural acidic eutectic solvents have been preferred for this role since there is no need to make additional pH adjustments. Recently, some authors reported that when eutectic solvents formed with cholinium chloride
:
xylitol are applied to extract anthocyanins from Euterpe edulis fruits, there was an increase in the shelf-life of the colorants compared to those extracted with methanol.14 Other examples have also been investigated, including combining eutectic solvents with high-pressure systems, such as those reported by Benvenutti and co-workers.15 However, some gaps remain, especially regarding the thermal stability and purity of the colored extracts obtained by combining a high-pressure technology with alternative solvents.
Pressurized liquid extraction (PLE) processes have been considered more sustainable alternative technologies to create value from food waste when compared to those conventionally performed.16 PLE is a robust and versatile technique that usually combines high temperatures and pressures, leading to a lower viscosity and surface tension of the solvent. This maximizes the compounds’ solubility and, consequently, the extraction performance of the target compounds.17 Besides, PLE systems inactivate enzymes and microorganisms associated with the compounds’ deterioration,18 preventing the formation of toxic compounds during the extraction processes while improving their antioxidant activity.19 Some authors consider PLE a green and high-performance extraction process since it reduces the extraction time and decreases solvent consumption, maximizing the process performance.20 Despite the high efficiency of PLE, its selectivity is relatively low, and thus, it is often used in combination with the solid-phase extraction (SPE) technique.21 SPE is usually applied offline after the extraction procedure, which is not the most efficient and sustainable form of operation, leading to higher degradation rates,22 when compared with those performed in-line, i.e. PLE-SPE. In this sense, coupling different extraction techniques to improve the selectivity of the final extract (e.g. PLE-SPE) seems to be an excellent alternative to enhance the production and improve the quality of the extract.
In this work, we developed an extraction platform based on PLE-SPE in-line to extract and purify anthocyanins simultaneously. Jabuticaba wastes (Plinia cauliflora), a highly perishable fruit from Brazil, internationally known as Brazilian berry, rich in anthocyanins and other phenolic compounds,23 were selected as the source of colorants. Furthermore, considering there is a high tendency to increase the commercial potential of this fruit, including the international market, the valorization of this biomass becomes very relevant.24 Since 40% of the Jabuticaba biomass is wasted, this opens the doors to new market niches for its valorization. Here, an in-line extraction platform based on the PLE-SPE approach and using eutectic solvents as the solvent media is proposed for the Jabuticaba wastes valorization. In addition, the thermal stability of the pigments was evaluated, and the results were compared with those obtained for the extracts prepared with ethanol and water. In the end, the environmental impact of the PLE-SPE process and the use of conventional solvents and techniques were evaluated.
Materials and methods
Sample and materials
Jabuticaba wastes (mainly peels, seeds, and remaining pulp) were kindly donated by Sabarabuçu Produtos da Jabuticaba (Sabará, Minas Gerais). Cholinium chloride – ChCl, and betaine – Bet, were purchased from Sigma-Aldrich and used as HBAs. The HBDs tested were the acetic acid – AA, citric acid – CA, lactic acid – LA, malic acid – MA, 1,4-butanediol – But, ethylene glycol – Ety, and xylitol – Xyl. The chemical structure, molecular formula, molecular weight, and source of the starting materials used to produce the eutectic mixtures are depicted in Table 1. Ethanol absolute was purchased from Synth (Diadema, Brazil). The ultra–pure water was provided by a Purelab Flex 3 purifying system (ElgaVeolia, High Wycombe, United Kingdom). The chromatographic standard cyanidin-3-O-glucoside (Cy-Glu) was purchased from Sigma-Aldrich (>99%). Three hydrophobic adsorbent materials were evaluated to perform the SPE; namely, Sepra C18-E (Phenomenex, USA – particle size: 50 μm, pore size: 85 Å, reversed phase, small molecule selectivity from aqueous samples), Strata-X polymeric C18 (Phenomenex, USA – particle size: 33 μm, pore size: 85 Å, reversed phase, tightly retain analytes by several different sorbent-analyte interactions, removing the unwanted contaminants including phospholipids, proteins, and particulates), and Oasis HBL C18 (Waters, USA – particle size 30 μm, pore size: 80 Å, universal polymeric reversed-phase sorbent that was developed for the extraction of a wide range of acidic, basic, and neutral compounds from various matrices).
Table 1 Chemical structure, molecular formula, molecular weight, purity, and source of the starting materials used to produce the eutectic mixtures
Chemical name |
Chemical structure |
Molecular formula |
Molar mass |
Purity (%) |
Source |
Cholinium chloride (HBA) |
|
C5H14NOCl |
139.62 |
96.4 |
Sigma-Aldrich |
Betaine (HBA) |
|
C5H11NO2 |
117.15 |
98 |
Sigma-Aldrich |
Acetic acid (HBD) |
|
C2H4O2 |
60.05 |
≥99 |
J. T. Baker |
Lactic acid (HBD) |
|
C3H6O3 |
90.08 |
85 |
Dinâmica |
Citric acid (HBD) |
|
C6H8O7 |
192.12 |
≥99 |
Dinâmica |
Malic acid (HBD) |
|
C4H6O5 |
134.09 |
98 |
Dinâmica |
1,4-Butanediol (HBD) |
|
C4H10O2 |
90.12 |
99 |
Neon |
Ethylene glycol (HBD) |
|
C2H6O2 |
62.07 |
99 |
Neon |
Xylitol (HBD) |
|
C5H10O5 |
152.1 |
98 |
Sigma-Aldrich |
Eutectic solvents preparation
Briefly, the eutectic solvents formed by two-component mixtures (HBA and HBD) were accurately weighed into a closed glass flask.7 For the screening assay, all eutectic solvents were formulated using a molar ratio of 1
:
2 and 15% water (considering each starting material's initial water content). Then, the mixtures were stirred in an oil bath at 60 ± 2 °C at 500 rpm until a homogenous and transparent liquid was obtained. The solvents were stored for less than 24 h until use.
Screening of eutectic solvents – COSMO-RS model
COSMO-RS calculations were performed in a two-step procedure. First, using the software Turbomole (TmoleX19 Version 4.5), the geometry of each molecule (HBA, HBD, and anthocyanin models) was optimized using the COSMO-BP-TZVP. Then, using the optimum designed molecules, the polarization charges (σ) and the surface composition functions, p(σ), were calculated via COSMOtherm® software. For this, the COSMOtherm® (Version 21.0) package with the parameterization BP_TZVP_21.ctd was used.25 The COSMO-BP-TZVP model includes a base set of def-TZVP, DFT with the theory functional level B-P83, and the COSMO solvation model. Then, COSMO-RS was used to predict the activity coefficient at infinite dilution of anthocyanin models (cyanidin-3-O-glucoside and cyanidin-3-O-rutinoside) in 1652 combination of HBAs and HBDs at 308.15 K. The activity coefficient at infinite dilution (γ∞) was used as a parameter to select the best HBA/HBD combinations to be experimentally tested. Additionally, the σ-potentials and σ-profiles were obtained. The list of tested HBAs and HBDs is presented in Table S1 from ESI.†
Experimental screening of solvents
The eutectic mixtures tested were produced based on the activity coefficient results. After the COSMO-RS screening, 14 eutectic solvents were selected to be tested experimentally. Besides, aqueous solutions (250 mM) of the starting materials used to prepare the eutectic mixtures were tested. For this, an ultrasound-assisted extraction (UAE), using an ultrasonic bath (P60H, Elmasonic, Singen, Germany, 2.75 L, 37 kHz, 135 W) was applied (operational conditions fixed at solid–liquid ratio -SLR- 0.1 gbiomass mL−1 for 120 min). After extraction, centrifugation was performed in an Eppendorf 16R centrifuge (4 °C, 12
000 rpm for 10 min) to separate the extract from the residual biomass. Finally, the supernatant, rich in anthocyanins, was separated, characterized, and quantified by high-pressure liquid chromatography coupled with a photodiode array detector (HPLC-PDA).
Pressurized-liquid extraction coupled in line with solid-phase extraction (PLE-SPE)
The experiments were performed using a PLE-SPE system17 composed of a high-pressure binary pump (Waters, 1525, Milford, MA, USA); an oven for the SPE column (Waters, 1525, Milford, MA, USA); oven for extraction cell (Memmert GmbH, UF55, Buechenbach, Germany); adsorbent column (50 × 4.6 mm, Waters, 1525, Milford, MA, USA); valves for selection, depressurization, automatic pressurization, and fractions collection (Waters, Two-Position Six-Port Selector Valve, Milford, MA, USA); and a stainless steel extraction cell (111.5 × 20 mm, Citua, Campinas, SP, Brazil). A computer automatically controls the system through Waters Empower™ 3 software. The schematic representation of the prototype is depicted in Fig. 1.
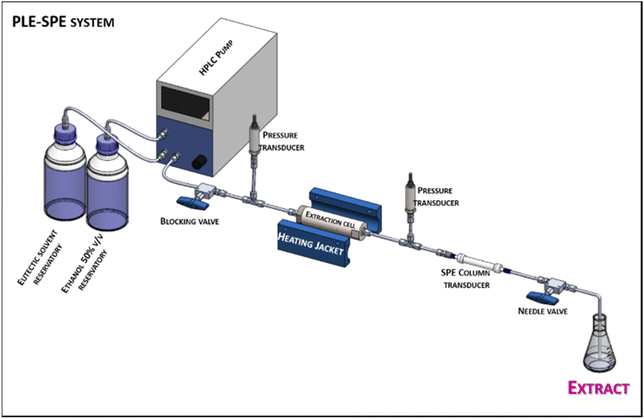 |
| Fig. 1 Simplified diagram of the PLE-SPE system used (see the complete diagram of the equipment used in Viganó et al. (2021).17 | |
The anthocyanins were recovered from Jabuticaba waste by combining eutectic mixtures with an in-line PLE-SPE procedure. First, the stainless-steel extraction cell was filled as follows: 0.3 g of glass wool, 16 g of glass beads (2 mm), and 0.75 g of Jabuticaba waste. The glass wool was used to filter the extract and remove solid particles from the biomass (debris) while the glass beads were used to reduce the dead volume of the extraction cell. The cell was connected to the PLE-SPE system, and the solvent inlet tube was connected to the extraction cell. In-line, an SPE column was filled with 3 g of the adsorbent material and connected to the system. First, to begin the extraction, the valves V1–V4 were set to position 1, and the adsorbent material was activated with 30 mL of ethanol absolute at a flow rate of 3 mL min−1. After that, the same procedure was performed using water to condition the adsorbent material (30 mL, 3 mL min−1). Then, the oven of the extraction cell was heated to the experimental temperature (using a pre-heating time of 60 min to guarantee that the interior of the extraction cell was at the desired temperature). The valve V4 was then set to position 2, and the extraction cell was filled with the selected eutectic solvent until the pressure reached 1500 psi. Before initiating the flow of the eutectic solvent, the static time (tsta) was optimized, i.e. the time the biomass was in contact with the solvent but without a flow of solvent (0–25.1 min). After proper tsta optimization, the flow of eutectic solvent was started, and the first experiments were performed using a dynamic flow of 1.5 mL min−1 (15 min = 30 mL of extract). After completing the extraction with the eutectic solvent, valve V4 was switched to position 1, the solvent flow was interrupted, and initiated the elution of the retained pigments in the adsorbent material. Ethanol 50% (flow of 2 mL min−1) with the temperature of the SPE column fixed at 40 °C was used to finish the elution step and complete the PLE-SPE extraction procedure.
Optimization of the PLE-SPE approach by surface responsive methodology
After selecting the most efficient eutectic solvent, the extraction process variables were optimized (by central composite rotatable design – CCRD 23 plus axial points). In this step, PLE-SPE was used to maximize the extraction yield of anthocyanins and, simultaneously, to purify the extracted pigments (separate them from the non-desirable extracted compounds). For this, the operational variables (V1), namely temperature (T – °C), (V2) static time (tsta – min), and (V3) water content in the eutectic solution (wt% – %) were selected as independent variables. 17 experiments (8 linear points, 6 axial points, and 3 central points) were performed, varying the levels as described in Table S2 (from ESI†). Here the dynamic extraction time and solvent flow were fixed at 15 min and 1.5 mL min−1, respectively. The responsive variable used to guide the optimization experiments was the final concentration of anthocyanins extracted (mganthocyanins gbiomass−1). After selecting the best operational conditions, a validation assay was conducted in triplicate, with the respective relative deviation calculated based on the experimental vs. predicted results (expressed in %). Also, the kinetic curve of anthocyanins recovery was measured at the optimum conditions, recording fractions at each 5 min (5–100 min). Finally, the optimum dynamic extraction time was considered at the beginning of the diffusional phase, representing 100% of the extraction efficiency (%relative).
Optimization of the solid-phase extraction (SPE)
Three adsorbent materials were tested to evaluate which would promote the highest purification rate of anthocyanins, i.e., eliminate the undesirable compounds from the anthocyanins-rich extract. Since a considerable amount of colorants was retained in the SPE column, after the PLE, a desorption step was performed (to guarantee that no colorant was retained in the adsorbent material of the SPE). Then, a flow of ethanol 50% (v/v) was used to remove the remaining anthocyanins from the SPE column. The temperature of the SPE column was fixed at 40 °C. Finally, after recovering the anthocyanins-rich extract, the extracts were analyzed by HPLC-PDA for quantification.
Quantification and identification of anthocyanins (HPLC-PDA)
The HPLC system has a column oven and a photodiode array detector (Waters, Milford, USA). The software for control and data collection was Empower™ 3 (Waters, Milford, MA, USA). The chromatographic runs were performed using a fused-core C18 column (Kinetex, 100 × 4.6 mm i.d.; 2.6 μm; Phenomenex, Torrance, CA, USA). An isocratic chromatographic method using (A) acidified water (0.25 mol L−1 citric acid), and (B) ethanol absolute (HPLC grade) at a flow rate of 1.5 mL min−1, at a proportion 85%(A)
:
15%(B) was performed (run of 2 min, column temperature at 50 °C), with injection volume fixed at 5 μL. The chromatographic eluate was analyzed at 520 nm. The quantification of the total anthocyanins content was performed using a calibration curve (r2 > 0.99, 1.6–195 ppm) of an external standard (cyanidin-3-O-glucoside, Sigma-Aldrich, ≥98%). Each assay's total concentration of anthocyanins was expressed in mganthocyanins gbiomass−1.
The identification of the anthocyanins peaks was made by tandem mass spectrometry (MS/MS), performed in a Thermo Finnigan (San Jose, Ca, USA) LCQ mass spectrometer equipped with an electrospray ionization source (ESI) and an ion-trap analyzer (IT), and Xcalibur software for data processing. Briefly, a direct infusion was made in positive ion mode, with capillary temperature fixed at 300 °C; 85 arbitrary units of nitrogen, and gas-assisted with 5 arbitrary units. Additionally, a comparison with the literature data concerning the UV-vis spectra of the representative ions and the MS2 and MS3 fragments was performed to confirm the characterization, which was confirmed by the identification performed in Biazotto et al. (2019).23
Purity calculation
The purity (%) of anthocyanins was estimated based on the anthocyanin concentration, according to eqn (1), where c represents the anthocyanin content (mganthocyanins mL−1), V is the total volume (mL) obtained, mextract is the mass (mg) of the extract after evaporation of the solvent, and meutectic mixture (mg) is the mass (mg) of the eutectic mixture used in the extraction step. |
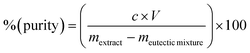 | (1) |
Extraction performance of other solvents and techniques
The PLE-SPE extraction performance was compared with those obtained by other extraction techniques. In this work, the conventional techniques tested were static maceration (24 h, RSL 0.1), ultrasound-assisted extraction (UAEprobe—240 W, dissipated power 1.99 W h mL−1, 5 min, 10 mL, continuous application; and UAEbath—150 W, dissipated power 0.30 W h mL−1, 120 min, bath volume 1000 mL, bath dimensions (cm): 27 × 12×7), ultra-dispersion (4000 W, dissipated power 18.75 W h mL−1, 5 min, 10 mL, 1500 rpm), and magnetic stirring (185 W, dissipated power 222 W h mL−1, 720 min, 10 mL, 500 rpm). In this set of experiments, the SLR fixed was 0.1 gbiomass mLsolvent−1, and all the extractions were performed protected from light. The solvents used in each conventional approach were ethanol (50, 100% v/v) and water. Three replicates were performed for each assay, and the results were expressed as the mean ± standard deviation of the mean (mganthocyanins gbiomass−1).
Thermal stability of the obtained pigments
Three different temperatures were tested to evaluate the stability of the pigments when heated, 55, 75, and 95 °C. Besides, to compare the stability of the pigments obtained by the PLE-SPE approach using eutectic mixtures as solvents with those conventionally extracted, the pigments obtained using ethanol and water were also evaluated. Thus, after extraction using the validated condition from the CCRD assay (optimum condition), the extracts obtained using water and ethanol were filtered in nylon syringe filters (0.22 μm – Nova Analítica, São Paulo, Brazil), conditioned in vial flasks (1.5 mL), and heated to the specific assay temperature (Memmert GmbH, UF55, Buechenbach, Germany). After collecting the samples at different times, the vials were immediately cooled in an ice bath to stop any possible reaction occurring caused by heating. Then, by chromatographic analysis (HPLC-PDA), the concentration of colorants was calculated (mganthocyanins mL−1), which was used to calculate the constant degradation rate (Kd), estimated by a semi-natural logarithmic plot of the anthocyanin concentration vs. time. Then, using the Kd values and the kinetic degradation curves from the three different temperature assays, other thermal kinetic parameters, namely, half-life time (t1/2), and thermal activation energy (Ea), were calculated.
Environmental analysis – ecoscale
The environmental analysis was performed using the Ecoscale database.26 Penalty points were attributed considering (i) safety, (ii) solvent, (iii) price/availability, (iv) technical setup, (v) temperature used in the extraction process, and (vi) workup and purification steps. Then, all these aspects were normalized by the relative yield of extraction (%relative) of anthocyanins from Jabuticaba wastes. The results were depicted in an Ecoscale score (1 to 100), where 1 and 100 represent the lowest and the most eco-friendly process, respectively.26 A comparison between the conventional (maceration and magnetic stirring), emergent (ultradispersion and ultrasound-assisted extraction), and PLE-SPE extraction techniques using different solvents (water, ethanol 50% v/v, and ethanol 10% v/v) was performed.
Statistical analysis
After selecting the best operational conditions by CCRD, an analysis of variance (ANOVA) followed by the Bonferroni post-hoc test was applied to compare the extraction yield between different molar ratios and techniques and to select the best extraction time. A linear Pearson correlation (rPearson) was used to investigate a possible correlation between the Ecoscale score and the extraction efficiency (%relative). The significance level selected for all analyses was 95% (p < 0.05, n = 3). All analyses were performed using the JAMOVI software (1.6.6).
Results and discussion
Solvent screening via COSMO-RS
COSMO-RS was used in this work to predict the best solvents for the extraction of anthocyanins. The criterion used was the activity coefficient at infinite dilution (γ∞). According to Hildebrand's solubility parameters theory,27 the γ∞ is a vital parameter to describe the dissolution capability of a solute in a solvent. A small value of ln
γ∞ indicates that the two substances are mutually soluble. The γ∞ COSMO-RS data for 1652 possible eutectic mixtures (resulting from the combination of 29 HBAs and 59 HBDs, at a molar ratio 1
:
2, respectively) were estimated. These results are shown in Fig. 2, where the color scale represents the ln
γ∞ for cyanidin-3-O-rutinoside (left) or cyanidin-3-O-glucoside (right) in the eutectic mixtures at 308.15 K. The dark blue squares represent mixtures with a value of ln
γ∞ ≤ 5, while the yellow squares represent mixtures with a value of ln
γ∞ > 5. The lower the ln
γ∞ value, the greater the solvent capacity.28 The mixtures with the highest solvating capacity are in the dark blue regions. COSMO-RS data reveal that cyanidin-3-O-rutinoside and cyanidin-3-O-glucoside solubility are affected by HBA and HBD choice. Eutectic solvents composed of betaine (2 – Table S1†), cholinium chloride (19 – Table S1†), and sodium acetate (20 – Table S1†) led to low values of ln
γ∞ for cyanidin-3-O-rutinoside and cyanidin-3-O-glucoside for several HBDs, suggesting that the solubility is dominated by the HBA hydrophobicity. For example, for betaine (2 – Table S1†) the ln
γ∞ decreases when combined with HBDs composed of urea (1 – Table S1†), alcohols (3–15 – Table S1†), and carboxylic acids (33–41 – Table S1†), while for sugars (21–31 – Table S1†), it is possible to observe a yellowish region, meaning higher ln
γ∞ values. In general, it is possible to state that the results obtained for cyanidin-3-O-rutinoside and cyanidin-3-O-glucoside are qualitatively identical, so the best and worst HBA
:
HBD combination is the same. Betaine (2 – Table S1†), cholinium chloride (19 – Table S1†), and sodium acetate (20 – Table S1†) combined with alcohols and carboxylic acids formed the most promising eutectic solvents to be used in the extraction of anthocyanins and were selected for the experimental screening.
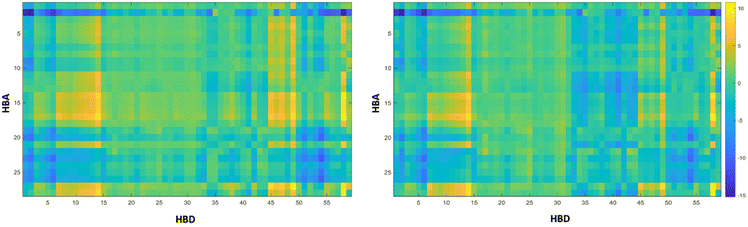 |
| Fig. 2 The activity coefficients at infinite dilution (ln γ∞) of cyanidin-3-O-glucoside (left) and cyanidin-3-O-rutinoside (right) in eutectic mixtures (1 : 2) at 308.15 K. Table S1 from ESI† contains the label of each corresponding HBA and HBD analysed. | |
Experimental screening of eutectic solvents
Considering the results obtained from the COSMO-RS, 14 eutectic mixtures were selected to be tested as solvents on the recovery of anthocyanins from Jabuticaba wastes (at a molar ratio 1
:
2 and with 15 wt% water). In addition, aqueous solutions (250 mM) of the individual starting materials were also tested. It is possible to note in Fig. 3 that, except for the solvent ChCl
:
CA, all the other eutectic mixtures promoted higher yields of extraction than those obtained with water or EtOH 100% v/v, here both representing the conventional solvents. Also, it is possible to note that EtOH 50% v/v promoted an extraction yield of almost 6.5 mganthocyanins gbiomass−1, a value comparable with those obtained for the eutectic mixtures (around 6–10 mganthocyanins gbiomass−1). Meanwhile, the solvent ChCl
:
LA (1
:
2, 15 wt%) allowed recovering 13.07 mganthocyanins gbiomass−1, twice the yield obtained using EtOH 50% v/v as solvent. The σ-profile and σ-potential – μ(σ) – (Fig. S1 – ESI†) can be used to support the understanding of the affinity of this (D)ES and anthocyanins. The σ-profile provides information about the polarity distribution of molecules, and its normalized distribution function describes the μ(σ). The anthocyanin model molecules present a similar σ-profile and σ-potential, with most of the peaks located in the non-polar region due to the contribution of the carbon chain of the molecule, in addition to peaks in the H donor region (contribution of the H atoms) and peaks in the H acceptor region (the hydroxyl groups). ChCl
:
LA and Bet
:
LA present peaks in the three regions, having a similar profile to the anthocyanin model molecules, which indicates a higher affinity between anthocyanin and the solvent. The ethanol presents a distinct profile, with a lower intensity peak in the non-polar region, related to its small carbon chain, indicative of a lower affinity with anthocyanins.28
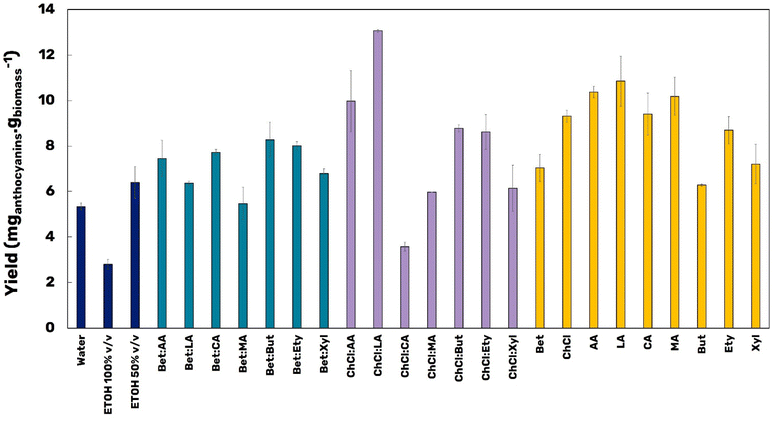 |
| Fig. 3 Screening of aqueous solutions of different eutectic mixtures used to recover anthocyanins from Jabuticaba wastes. Water and ethanol (50% and 100% v/v) were used as controls. The results are expressed as the mean of yield (in mganthocyanins gbiomass−1). The experiments were performed in triplicate (n = 3). Bet: Betaine, ChCl: cholinium chloride, AA: acetic acid, LA, lactic acid, MA, malic acid, CA: citric acid, But: 1,4-butanediol. Ety: ethylene glycol, Xyl: xylitol. | |
On the other hand, the aqueous solutions of the starting materials alone, despite having a large extraction yield, also around 6–10 mganthocyanins gbiomass−1, do not reach an equivalent yield compared to the best eutectic mixture (ChCl
:
LA), highlighting the synergism of this solvent by improving the solvation potential and forming hydrogen bonds with the anthocyanins,29 since ChCl and LA alone do not promote an equivalent yield. The eutectic mixture formed by ChCl
:
LA is a safe choice considering the future applications of the anthocyanin-rich extract, being allowed for application in both the food and pharmaceutical sectors. For example, LA is already used as food preservative and acidulant agent in several food formulations, especially in feed, candies and juices.30 The same happens with ChCl, that is largely used as vitamin precursor additive in feeds, or even as stabilizing agent in nanoemulsions,31 which highlights the potential dual function of the eutectic solvent proposed as solvent and stabilizing agent.
After selecting the best solvent to recover high yields of anthocyanins from Jabuticaba wastes, the molar ratio of the HBA and HBD was studied separately (Fig. 4). For this, eutectic solvents with different molar ratios (3
:
1, 2
:
1, 1
:
1, 1
:
3, 1
:
4, 1
:
5), besides the already tested 1
:
2 were prepared and used as solvent. Since the molar ratio 1
:
2 promoted the best yield (ANOVA p < 0.05), it was further used in the optimization assays.
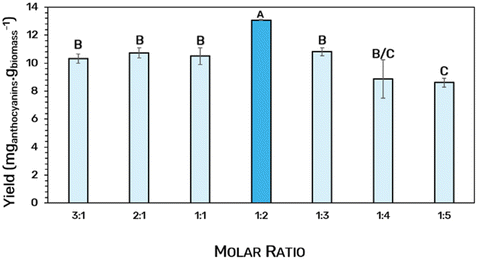 |
| Fig. 4 Extraction yield of anthocyanins from Jabuticaba wastes (mganthocyanins gbiomass−1) by applying different molar ratios of ChCl : LA. | |
Process optimization
After selecting the best solvent able to recover the anthocyanins from the Jabuticaba wastes (ChCl
:
LA, molar ratio 1
:
2), the optimization assays by PLE-SPE were initiated. Table S2 (ESI†) reports the real and encoded levels studied. In the set of experiments performed, an average of 14.68 mganthocyanins gbiomass−1 was achieved. Besides, all the independent variables were significant in the design of the predictive model (R2 = 0.99). The assay 10 (performed using a T = 90.2 °C, tsta = 12.5 min, and wt% = 30%) led to the best anthocyanin yield, 20.22 mganthocyanins gbiomass−1. On the other hand, assay 1 (T = 50 °C, tsta = 5 min, and wt% = 25%) promoted the lowest observed yield 3.83 mganthocyanins gbiomass−1. The results obtained in the set of experiments revealed that the quadratic level of temperature (V12), and the cross interaction between tsta and wt% (V2 × V3), do not influence the results in the predictive model. For this reason, they were excluded (see Pareto's chart in Fig. S2 – ESI†). Thus, eqn (2) was designed as a predictive model at a 95% significance level. The model defined is highly predictive (variation coefficient of 11.44% and Ftabulated = 71.58) and accurate (see observed vs. expected values in Fig S3 – ESI†). |
y(anthocynin yield) = −62.95 − 0.19(V1) + 0.99(V2)−0.03(V2)2 + 3.95(V3) − 0.07(V3)2 + 0.02(V1 × V3) | (2) |
The contour plots shown in Fig. 5 represent the optimum condition to recover anthocyanins from Jabuticaba wastes. As it is possible to note in Fig. 5A and B, the highest temperature evaluated (90.2 °C) significantly improved the anthocyanin extraction yield. In Fig. 5C, a remarkable zone of optimization is found between 32–36% wt%, and in 10–20 min of tsta. Thus, considering the tested levels of the CCRD assay, the optimum extraction conditions could be considered as follows: T = 90.2 °C, tsta = 12.5 min, and wt% = 35%. Thus, applying these optimum values in eqn (2), it is possible to predict an extraction yield of 24.1 mganthocyanins gbiomass−1. For validation, new extractions were made using the considered optimum operational conditions (n = 3), which promoted an extraction yield of 25.7 ± 0.7 mganthocyanins gbiomass−1, representing a relative deviation of around 6%.
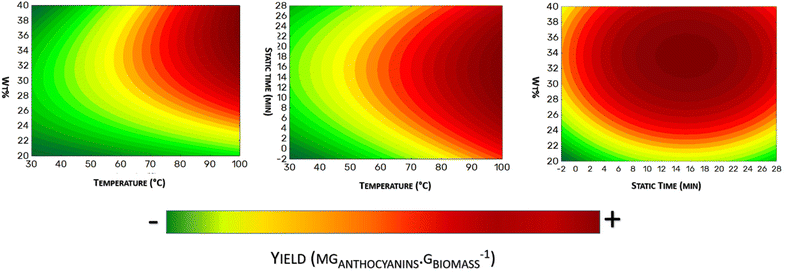 |
| Fig. 5 Contour plots obtained from the CCRD (23 plus axial points) predictive model for anthocyanins’ extraction yield from Jabuticaba wastes. The independent variables studied in this model were: temperature (T, °C), static time (tsta, min), and water content in the eutectic mixture (wt%). The combination between the independent variables was performed 2 by 2 as follows, from the left to the right: tstavs. T; wt% vs. T; wt% vs. tsta. | |
Kinetic study (dynamic extraction time – min)
A kinetic assay was conducted after adopting the optimum operational conditions stipulated in the CCRD (T = 90.2 °C, tsta = 12.5 min, and wt% = 35%) to define the best operational dynamic time able to maximize the extraction yield of anthocyanins. Considering that, in a dynamic extraction approach as PLE, a new solvent is injected into the extraction cell over time, an “exhaustive” extraction process can be developed aiming for the maximum recovery of the colorants from the biomass, although requiring more solvent and energy inputs. To infer the sustainability of using the aqueous solution of ChCl
:
LA against the conventional solvents, a kinetic study was developed, with the results depicted in Fig. 6. Considering the three extraction phases described for PLE, namely: (i) constant extraction rate (CER), falling extraction rate (FER), and diffusion-controlled rate (DC); we assumed the ideal extraction dynamic time to recover the highest yield of colorants from the biomass as the starting of the DC phase (which represents 100%relative of the extraction efficiency). From the results of Fig. 6, the eutectic mixture (ChCl
:
LA) originated the highest extraction efficiency (100%relative) when compared with the conventional solvents (water 35.8%relative, EtOH 50% v/v with 42.3%relative, and EtOH 100% v/v with 24.8%relative). These data reinforce the role of the eutectic solvent composed of ChCl and LA on the extraction of anthocyanins from Jabuticaba wastes. It is also possible to note that the kinetic behavior of the four extraction systems is similar, although the time dependency to reach the DC phase. More than 90%relative of the total amount of anthocyanins extracted are recovered after 40 min of extraction with ChCl
:
LA. At the same time, only 32.8%relative, 18.9%relative, and 38.97%relative are recovered when water, EtOH 100% v/v, and EtOH 50% v/v, respectively, are used. The possible degradation of the colorants may justify this due to the high temperature applied (90.2 °C),32,33 which allowed changing the color of the extract to brown. This indeed happened when water and ethanol mixtures were used as solvents. Moreover, it seems that conventional solvents do not lead to an efficient release of anthocyanins.
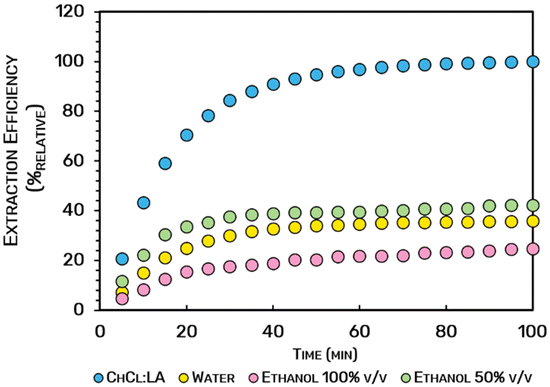 |
| Fig. 6 Kinetic performance of anthocyanins recovered from Jabuticaba wastes using a PLE approach optimized by CCRD (operational conditions: T = 90.2 °C, tsta = 12.5 min, and wt% = 35%). ChCl : LA = eutectic solvent formed with ChCl : LA (molar ratio 1 : 2). | |
Selection of adsorbents to perform the PLE-SPE (in-line system)
For the development of the in-line PLE-SPE, three commercial C18 adsorbent materials were tested, namely the Sepra C18-E, Strata-X C18, and Oasis HBL C18. These adsorbent materials were selected considering their high ability to fractionate hydrophobic compounds, as specified by the fabricant datasheet. As depicted in Fig. 7, the order of efficiency of the different absorbent materials is Strata-X C18 > Sepra C18-E > Oasis HBL C18. Indeed, Strata-X C18 is the adsorbent material with the highest extraction efficiency = 100%relative, thus allowing to obtain the colorant with the highest purity = 96.1%. The lower affinity may explain these results between the anthocyanins and the absorbent material, and the higher affinity of the contaminants present in the extract rich in colorants for this resin. Contrarily to what happens when water is used during the extraction phase, when ChCl
:
LA is used, the colorants are poorly retained in the absorbent material, allowing the anthocyanins to be released from the SPE column.
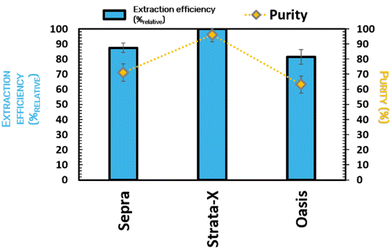 |
| Fig. 7 Extraction efficiency (%relative) and purity rate (%) of anthocyanins from Jabuticaba wastes with different absorbent materials (Sepra C18-E, Strata-X C18, and Oasis HBL C18) in the SPE step. | |
Comparison with other extraction techniques and solvents in terms of concentration of anthocyanins and purity
After selecting Strata-X C18 as the best absorbent material, other solvents and extraction techniques were evaluated, these including ultradispersion and ultrasound-assisted extraction, the latest using two cavitation methods: UAEprobe and UAEbath. It is notorious in Fig. 8 that the PLE-SPE approach using ChCl
:
LA as solvent was the most efficient and provided the highest purity level compared to the other extraction approaches (expressed in 100%relative and 96.1% of purity). The PLE-SPE using ethanol (50% and 100% v/v) and water as solvents do not display equally good results (efficiencies around 24.8–42.3%relative and purities around 75%–87.5%), even when compared with the results obtained for UAE and ultradispersion (with efficiency around 86.2%relative). In general, it is possible to note that non-conventional solvents perform better in emergent techniques than conventional ones in terms of extraction yield and the purity of the final product. The same trend was already reported in literature, for the extraction of isoflavones with cholinium chloride
:
citric acid (molar ratio of 1
:
1),34 or carotenoids with aqueous solutions of ionic liquids.35 This behavior may be justified by the higher affinity of the alternative solvents for anthocyanins. Anthocyanins are naturally positively charged molecules able to establish relevant intermolecular interactions with the eutectic mixtures composed of cholinium chloride. Some authors have also postulated that eutectic solvents could form supramolecular aggregates with the solutes,36 consequently increasing the product purity and stability.
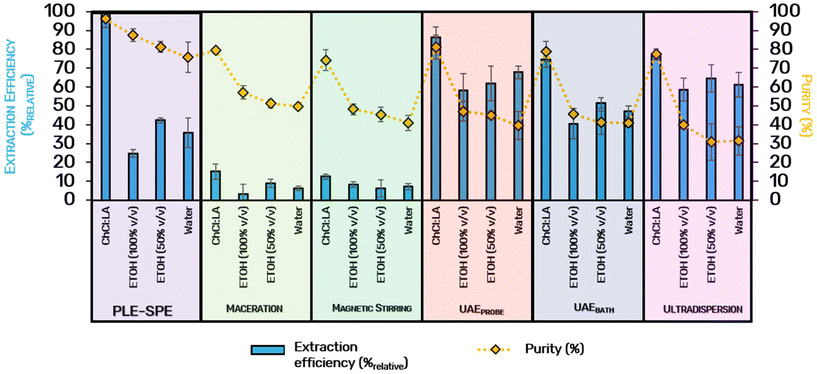 |
| Fig. 8 Comparison of the recovery (%relative) and purity (%) of anthocyanins obtained with different extraction methods (PLE-SPE, maceration, magnetic stirring, UAE, and ultradispersion) using different solvents (ChCl : LA, ethanol 50% v/v and 100% v/v, and water). | |
Thermal stability of the anthocyanins
Several conditions can lead to colorants’ degradation during their recovery from the biomass, namely the (i) increase in temperature,33 (ii) exposure to light and oxygen, and (iii) prolonged extraction times.32 Thus, solutions to overcome this drawback are required, especially if an industrial application is envisaged. An example of the most recurrent stabilization strategies is the use of stabilizing agents (SiO2, starches, sugars, gums)37 or the application of microencapsulation techniques.38 Besides, during the PLE process, the biomass does not come into contact with oxygen or light; the high-pressure system inactivates both enzymes and microorganisms associated with quality deterioration/compound degradation, thus extending the shelf life of the final extract.20 Another strategy is the use of alternative solvents, specifically modulated to extract a specific group of molecules, in this case, anthocyanins and simultaneously decreasing the possibility of co-extraction of non-desired compounds, eventually improving the target compounds’ stability.5 For example, when sulfites are removed from red wines, the product color is improved, restoring the original color of the anthocyanins.39 In addition, if some minerals (like Ca2+, and other divalent ions) are co-extracted or added as food preservatives, the anthocyanins bleaching occurs, impairing the standard color of the product, consequently decreasing their commercial value.40 Thus, to increase the purity level of the target compounds, some of us have already demonstrated that alternative solvents could increase the shelf-life of some natural colorants.8 Hence, in this work, the stabilizing effect of the eutectic solvent used was investigated, and the results were compared with the data obtained for conventional solvents (Table 2). Table 2 shows that the results obtained for the thermal stability of anthocyanins extracted from Jabuticaba wastes using alternative solvents agree with our past findings. As shown in Fig. S4 of the ESI,† first-order degradation kinetics is observed regardless of the exposure temperature (55 °C, 75 °C, or 95 °C).
Table 2 Influence of the extraction solvent (water, ethanol 50% v/v, ethanol 100%, and ChCl
:
LA) on the anthocyanins’ thermal stability (55 °C, 75 °C, and 95 °C)
Extraction solvent |
55 °C |
75 °C |
95 °C |
E
a (KJ mol−1) |
K
d (min−1) |
t
(1/2) (h) |
K
d (min−1) |
t
(1/2) (h) |
K
d (min−1) |
t
(1/2) (h) |
K
d = thermal degradation constant (min−1); t(1/2) = half-life time (hours); Ea = thermal activation energy. Extract obtained under the optimum operational conditions. |
Water (pH 3) |
0.0016 |
7.22 |
0.0031 |
3.73 |
0.0142 |
0.81 |
54.27 |
Ethanol 50% v/v (pH 3) |
0.0026 |
4.44 |
0.0059 |
1.95 |
0.0305 |
0.37 |
61.30 |
Ethanol 100% (pH 3) |
0.0024 |
4.81 |
0.0097 |
1.19 |
0.0251 |
0.46 |
59.01 |
ChCl : LA (pH 3)a |
0.0005 |
23.10 |
0.0028 |
4.13 |
0.0098 |
1.18 |
74.75 |
It is possible to note that the minimum energy required to start the thermal anthocyanins’ degradation is higher when ChCl
:
LA is used as the solvent (Ea = 74.75 kJ mol−1), confirming the advantageous effect of the eutectic mixture to stabilize the anthocyanins’ color. These data reflect the higher half-life time (t(1/2), h) of the colorants extracted with ChCl
:
LA for the temperatures evaluated. Despite the previous examples reported in the literature for using eutectic solvents to improve the shelf life of natural compounds,29 the mechanisms are still not yet fully understood. The most plausible justification remains on the possible formation of supramolecular aggregates between the eutectic solvent and the positively charged anthocyanins, acting as a physical shield and protecting the colorants from their interaction with the oxidative stressors.14
Environmental analysis – ecoscale
The Ecoscale tool was applied to evaluate the environmental footprint of the PLE-SPE process when compared with the conventional approaches. Two main outputs are considered to perform this analysis: the (i) solvent and the (ii) amount of product obtained (in this case, expressed as the relative yield of anthocyanins – %relative). All the extracts obtained with ethanol (50% v/v or 100% v/v) had 10 points discounted in the safety parameter (Table 3). Although ethanol is considered an eco-friendly solvent, used in several industries, including the food and pharmaceutical sectors, it is also a volatile organic solvent. That's why there are intrinsic risks of flammability and vapor generated, which impair the safety of the manufacturing process and labor quality. However, for being conventionally used, there is no penalty points regarding the parameter “price/availability”, contrasting with the processes performed using ChCl
:
LA as solvent, which had 5 penalty points (Table 3). Indeed, ChCl
:
LA is a safe solvent but not conventionally used on a large scale, which impairs its industrial application. To have the chance of applying an alternative process, one of two situations need to occur (i) the use of the conventional solvents must be strongly discouraged by the legal entities or (ii) the lack of any process with or without the use of conventional solvents. Considering the technical setup and heat generation, the PLE-SPE developed here suffered 9 penalty points (6 regarding the technical setup plus 3 considering the temperature) since a more complex apparatus is required to perform the extraction (high-pressure pumps, ovens, and pipes). Besides, 3 penalty points were attributed to the purification strategy due to the need to spend more energy, money, and raw materials.
Table 3 Penalty points regarding the environmental analysis by the Ecoscale for each extraction technique and solvent used in the extraction and purification of anthocyanins from Jabuticaba wastes
Technique |
Solvent |
Yield (%relative) |
Price/availability |
Safety |
Technical setup |
Temperature/time |
Workup and purification |
Ecoscale |
PLE-SPE |
ChCl : LA |
100.0 |
−5 |
0 |
−6 |
−3 |
−2 |
89.0 |
EtOH 100% v/v |
24.8 |
0 |
−10 |
−6 |
−3 |
−2 |
41.4 |
EtOH 50% v/v |
42.3 |
0 |
−10 |
−6 |
−3 |
−2 |
50.1 |
Water |
35.8 |
0 |
0 |
−6 |
−3 |
−2 |
56.9 |
Maceration |
ChCl : LA |
15.2 |
−5 |
0 |
0 |
−1 |
0 |
56.6 |
EtOH 100% v/v |
3.10 |
0 |
−10 |
0 |
−1 |
0 |
40.5 |
EtOH 50% v/v |
8.9 |
0 |
−10 |
0 |
−1 |
0 |
43.4 |
Water |
6.2 |
0 |
0 |
0 |
−1 |
0 |
52.1 |
Magnetic Stirring |
ChCl : LA |
12.5 |
−5 |
0 |
−1 |
−1 |
0 |
49.2 |
EtOH 100% v/v |
8.2 |
0 |
−10 |
−1 |
−1 |
0 |
42.1 |
EtOH 50% v/v |
6.3 |
0 |
−10 |
−1 |
−1 |
0 |
41.1 |
Water |
7.2 |
0 |
0 |
−1 |
−1 |
0 |
51.6 |
UAE probe |
ChCl : LA |
86.2 |
−5 |
0 |
−3 |
−2 |
0 |
83.1 |
EtOH 100% v/v |
58.2 |
0 |
−10 |
−3 |
−2 |
0 |
64.1 |
EtOH 50% v/v |
61.9 |
0 |
−10 |
−3 |
−2 |
0 |
65.9 |
Water |
67.8 |
0 |
0 |
−3 |
−2 |
0 |
78.9 |
UAE bath |
ChCl : LA |
74.6 |
−5 |
0 |
−3 |
−3 |
0 |
76.3 |
EtOH 100% v/v |
40.6 |
0 |
−10 |
−3 |
−3 |
0 |
54.3 |
EtOH 50% v/v |
51.6 |
0 |
−10 |
−3 |
−3 |
0 |
59.8 |
Water |
47.2 |
0 |
0 |
−3 |
−3 |
0 |
77.9 |
Ultradispersion |
ChCl : LA |
76.5 |
−5 |
0 |
−1 |
0 |
0 |
82.3 |
EtOH 100% v/v |
58.6 |
0 |
−10 |
−1 |
0 |
0 |
68.3 |
EtOH 50% v/v |
64.6 |
0 |
−10 |
−1 |
0 |
0 |
71.3 |
Water |
61.3 |
0 |
0 |
−1 |
0 |
0 |
79.7 |
On the other hand, the UAE-based extractions (UAEprobe and UAEbath) had 3 penalty points regarding the technical setup attributed due to the intense use of energy to promote the cavitation force and heat dissipation. Besides, since the extraction process based on UAEbath is performed for more than 60 min, a more intense energy demands occurs, leading to the attribution of more 3 penalty points. Thus, considering only the technical approaches, the conventional extraction techniques (maceration and magnetic stirring) displayed the best score (−1 and 0, respectively). However, since conventional techniques do not promote a high extraction yield (<20%relative), the ratio between effort and product does not lead to a high Ecoscale score. In general, the extractions performed by ultradispersion reached a high Ecoscale score (between 71.3–82.3), which can be explained by the accessible operation mode (which is fast and not energy demanding) while generating a high yield of anthocyanins.
In the end, a linear regression was performed to investigate the relationship between the extraction yield (%relative) and the Ecoscale score (Fig. 9). Three categories were assessed considering the Ecoscale value (high: ≥75, medium: between 55–75, and low: <55). It is possible to note that there is a positive correlation between the variables (r2 = 0.8356, rpearson = 0.9141), which highlights that the Ecoscale score depends mainly on the yield. Besides, considering the different solvents used to perform each extraction technique, it is possible to point out that when ChCl
:
LA is used as the solvent, there is a tendency to obtain a higher Ecoscale (note that the highest Ecoscale for each extraction method is reached with ChCl
:
LA). Besides, the PLE-SPE only reached a high Ecoscale score when the eutectic mixture was used, suggesting that the conventional solvents are not an ideal strategy to be implemented in the PLE-SPE systems to recover anthocyanins from Jabuticaba wastes (once water and ethanol promoted low yield and purity levels). Another critical piece of information evidenced in Fig. 9 is that the conventional techniques, regardless of the used solvent, do not display satisfactory Ecoscale scores (see yellow and green dots). On the other hand, ultradispersion seems to be a good option, regardless of the solvent used.
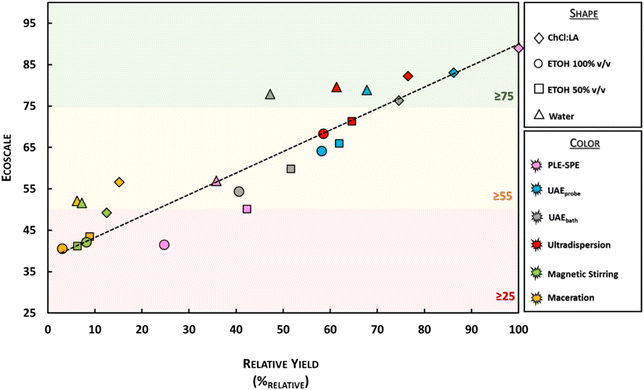 |
| Fig. 9 Linear regression concerning the Ecoscale vs. relative yield (%relative) (rPearson = 0.9141). | |
Conclusions
A PLE-SPE extraction process for the anthocyanins from Jabuticaba wastes was developed using the eutectic solvent composed of cholinium chloride
:
lactic acid, 1
:
2, wt% = 35%. The process variables were optimized, the optimal operational conditions were selected, namely T = 90.2 °C, and tsta = 12.5 min. Using a commercial adsorbent material, a solid-phase extraction system was coupled with the solid–liquid extraction system at high pressure. This approach displayed outstanding results regarding the simultaneous extraction and purification of anthocyanins from Jabuticaba wastes. To our knowledge, this is the first work that used eutectic mixtures in coupled automatized extraction systems, which opens perspectives to facilitate possible online analysis, which is an exciting strategy for analytical chemistry but also applications requiring high-scale downstream processes. Besides, ChCl
:
LA showed the best extraction efficiency, with the extracts’ thermal kinetic parameters suggesting the eutectic mixture's dual function as the solvent and stabilizing agent. An environmental analysis was carried out using the Ecoscale methodology. The best Ecoscale score was obtained for the process using ChCl
:
LA as extractant media in the PLE-SPE system compared with other conventional and emergent extraction techniques, highlighting the need and advantages of developing modern and alternative pathways to recover biological compounds in an eco-friendlier way.
Conflicts of interest
There are no conflicts to declare.
Acknowledgements
This work was supported by “Fundação de Amparo à Pesquisa do Estado de São Paulo – FAPESP” through the projects (2018/14582-5 and 2019/13496-0) and fellowships (L. M. de S. M.: 2020/08421-9, 2021/11022-1; L. C. S.: 2020/03623-2, 2021/11023-8; R. S. P.: 2020/04067-6 and J. V.: 2020/15774-5). This work was supported by Conselho Nacional de Desenvolvimento Científico e Tecnológico (CNPq, Brazil) (productivity grant 302610/2021-9) and Coordenação de Aperfeiçoamento de Pessoal de Nível Superior (CAPES, Brazil) [grant number 88887.310558/2018–00 and Finance Code 001]. This work was developed within the scope of the project CICECO-Aveiro Institute of Materials, UIDB/50011/2020, UIDP/50011/2020 & LA/P/0006/2020, financed by national funds through the FCT/MEC (PIDDAC).
References
- C. Reynolds, L. Goucher, T. Quested, S. Bromley, S. Gillick, V. K. Wells, D. Evans, L. Koh, A. Carlsson Kanyama, C. Katzeff, Å. Svenfelt and P. Jackson, Food Policy, 2019, 83, 7–27 CrossRef.
- V. De Laurentiis, S. Corrado and S. Sala, Waste Manage., 2018, 77, 238–251 CrossRef PubMed.
- E. Janiszewska-Turak, A. Pisarska and J. B. Królczyk, Nauka Przyr. Technol., 2016, 10, 51 Search PubMed.
- L. Ngamwonglumlert, S. Devahastin and N. Chiewchan, Crit. Rev. Food Sci. Nutr., 2017, 57, 3243–3259 CrossRef CAS PubMed.
- L. M. de Souza Mesquita, M. Martins, L. P. Pisani, S. P. M. Ventura and V. V. de Rosso, Compr. Rev. Food Sci. Food Saf., 2021, 20, 787–818 CrossRef PubMed.
- F. Chemat, M. Abert Vian, H. K. Ravi, B. Khadhraoui, S. Hilali, S. Perino and A.-S. Fabiano Tixier, Molecules, 2019, 24, 3007 CrossRef CAS PubMed.
- A. P. Abbott, D. Boothby, G. Capper, D. L. Davies and R. K. Rasheed, J. Am. Chem. Soc., 2004, 126, 9142–9147 CrossRef CAS PubMed.
- L. M. de Souza Mesquita, S. P. M. Ventura, A. R. C. Braga, L. P. Pisani, A. C. R. V. Dias and V. V. De Rosso, Green Chem., 2019, 21, 2380–2391 RSC.
- M. Martins, L. M. D. S. Mesquita, B. M. C. Vaz, A. C. R. V. Dias, M. A. Torres-Acosta, B. Quéguineur, J. A. P. Coutinho and S. P. M. Ventura, ACS Sustainable Chem. Eng., 2021, 9, 6599–6612 CrossRef CAS.
- F. A. Vicente, I. S. Cardoso, M. Martins, C. V. M. Gonçalves, A. C. R. V. Dias, P. Domingues, J. A. P. Coutinho and S. P. M. Ventura, Green Chem., 2019, 2019, 3816–3826 RSC.
- Ø. M. Andersen and M. Jordheim, Anthocyanins Health Dis., 2013, 1, 13–89 CrossRef PubMed.
- I. Benucci, C. Lombardelli, C. Mazzocchi and M. Esti, Compr. Rev. Food Sci. Food Saf., 2022, 21, 2715–2737 CrossRef PubMed.
- I. Luzardo-Ocampo, A. K. Ramírez-Jiménez, J. Yañez, L. Mojica and D. A. Luna-Vital, Foods, 2021, 10, 634 CrossRef CAS PubMed.
- N. Vannuchi, A. R. C. Braga and V. V. De Rosso, Processes, 2022, 10, 615 CrossRef CAS.
- L. Benvenutti, A. A. F. Zielinski and S. R. S. Ferreira, Food Chem.: X, 2022, 13, 100236 CAS.
- J. Li, M. Pettinato, R. Campardelli, I. De Marco and P. Perego, Appl. Sci., 2022, 12, 3642 CrossRef CAS.
- J. Viganó, V. L. Sanches, L. M. de Souza Mesquita, M. C. de Souza, L. C. da Silva, J. O. Chaves, T. Forster-Carneiro and M. A. Rostagno, Anal. Chim. Acta, 2021, 1178, 338845 CrossRef PubMed.
- F. J. Barba, M. J. Esteve and A. Frígola, Compr. Rev. Food Sci. Food Saf., 2012, 11, 307–322 CrossRef CAS.
- H.-W. Huang, M.-C. Cheng, B.-Y. Chen and C.-Y. Wang, J. Food Sci. Technol., 2019, 56, 4016–4024 CrossRef CAS PubMed.
- F. Chemat, M. Abert Vian, A.-S. Fabiano-Tixier, M. Nutrizio, A. Režek Jambrak, P. E. S. Munekata, J. M. Lorenzo, F. J. Barba, A. Binello and G. Cravotto, Green Chem., 2020, 22, 2325–2353 RSC.
- L. C. da Silva, M. C. Souza, B. R. Sumere, L. G. S. Silva, D. T. da Cunha, G. F. Barbero, R. M. N. Bezerra and M. A. Rostagno, Food Chem., 2020, 318, 126450 CrossRef CAS PubMed.
- S. Kueppers and M. Haider, Anal. Bioanal. Chem., 2003, 376, 313–315 CrossRef CAS PubMed.
- K. R. Biazotto, L. M. de Souza Mesquita, B. V. Neves, A. R. C. Braga, M. M. P. Tangerina, W. Vilegas, A. Z. Mercadante and V. V. De Rosso, J. Agric. Food Chem., 2019, 67, 1860–1876 CrossRef CAS PubMed.
- L. Benvenutti, A. A. F. Zielinski and S. R. S. Ferreira, Trends Food Sci. Technol., 2021, 112, 118–136 CrossRef CAS.
- F. Eckert and A. Klamt, AIChE J., 2002, 48, 369–385 CrossRef CAS.
- K. Van Aken, L. Strekowski and L. Patiny, Beilstein J. Org. Chem., 2006, 2(3), 1–7 Search PubMed.
- Y. Marcus, Chem. Soc. Rev., 1993, 22, 409 RSC.
- E. Durand, J. Lecomte, B. Baréa, E. Dubreucq, R. Lortie and P. Villeneuve, Green Chem., 2013, 15, 2275 RSC.
- Y. Dai, E. Rozema, R. Verpoorte and Y. H. Choi, J. Chromatogr. A, 2016, 1434, 50–56 CrossRef CAS PubMed.
- M. Janssen, A. H. Geeraerd, A. Cappuyns, L. Garcia-Gonzalez, G. Schockaert, N. Van Houteghem, K. M. Vereecken, J. Debevere, F. Devlieghere and J. F. Van Impe, Appl. Environ. Microbiol., 2007, 73, 1601–1611 CrossRef CAS PubMed.
- J. Ojala, M. Visanko, O. Laitinen, M. Österberg, J. Sirviö and H. Liimatainen, Molecules, 2018, 23, 2765 CrossRef PubMed.
- A. Patras, N. P. Brunton, C. O'Donnell and B. K. Tiwari, Trends Food Sci. Technol., 2010, 21, 3–11 CrossRef CAS.
- S. Oancea, Antioxidants, 2021, 10, 1337 CrossRef CAS PubMed.
- S. Bajkacz and J. Adamek, Talanta, 2017, 168, 329–335 CrossRef CAS PubMed.
- L. M. de Souza Mesquita, M. Martins, É. Maricato, C. Nunes, P. S. G. N. Quinteiro, A. C. R. V. Dias, J. A. P. Coutinho, L. P. Pisani, V. V. de Rosso and S. P. M. Ventura, ACS Sustainable Chem. Eng., 2020, 8, 4085–4095 CrossRef CAS.
- T. El Achkar, S. Fourmentin and H. Greige-Gerges, J. Mol. Liq., 2019, 288, 111028 CrossRef CAS.
- L. M. de Souza Mesquita, F. H. B. Sosa, L. S. Contieri, P. R. Marques, J. Viganó, J. A. P. Coutinho, A. C. R. V. Dias, S. P. M. Ventura and M. A. Rostagno, Food Chem., 2023, 406, 135093 CrossRef CAS PubMed.
- S. Mohammadalinejhad and M. A. Kurek, Appl. Sci., 2021, 11, 3936 CrossRef CAS.
- F. He, N.-N. Liang, L. Mu, Q.-H. Pan, J. Wang, M. J. Reeves and C.-Q. Duan, Molecules, 2012, 17, 1483–1519 CrossRef CAS PubMed.
- B. Enaru, G. Dreţcanu, T. D. Pop, A. Stǎnilǎ and Z. Diaconeasa, Antioxidants, 2021, 10, 1967 CrossRef CAS PubMed.
|
This journal is © The Royal Society of Chemistry 2023 |
Click here to see how this site uses Cookies. View our privacy policy here.