DOI:
10.1039/D2RA05127C
(Review Article)
RSC Adv., 2022,
12, 31068-31082
Nanotechnology strategies for hepatocellular carcinoma diagnosis and treatment
Received
16th August 2022
, Accepted 20th October 2022
First published on 31st October 2022
Abstract
Hepatocellular carcinoma (HCC) is a common malignancy threatening human health, and existing diagnostic and therapeutic techniques are facing great challenges. In the last decade or so, nanotechnology has been developed and improved for tumor diagnosis and treatment. For example, nano-intravenous injections have been approved for malignant perivascular epithelioid cell tumors. This article provides a comprehensive review of the applications of nanotechnology in HCC in recent years: (I) in radiological imaging, magnetic resonance imaging (MRI), fluorescence imaging (FMI) and multimodality imaging. (II) For diagnostic applications in HCC serum markers. (III) As embolic agents in transarterial chemoembolization (TACE) or directly as therapeutic drugs. (IV) For application in photothermal therapy and photodynamic therapy. (V) As carriers of chemotherapeutic drugs, targeted drugs, and natural plant drugs. (VI) For application in gene and immunotherapy. Compared with the traditional methods for diagnosis and treatment of HCC, nanoparticles have high sensitivity, reduce drug toxicity and have a long duration of action, and can also be combined with photothermal and photodynamic multimodal combination therapy. These summaries provide insights for the further development of nanotechnology applications in HCC.
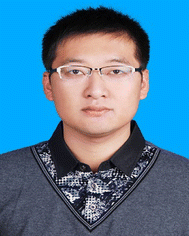 WeiLu Jia | Weilu Jia received his master's degree from GuiZhou medical University of China in 2020. Now he is a MD candidate at Southeast University, supervised by Prof. Yewei Zhang. Currently, his work focuses on drug delivery and Multimodal therapy for tumors. |
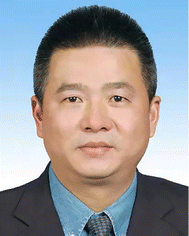 YeWei Zhang | Yewei Zhang earned his PhD degree from Suzhou University of China in 2005. In 2017, he joined the Southeast University. Then he joined The Second Affiliated Hospital of Nanjing Medical University, as a full professor. His current work focused on nanomedicine treatment for liver cancer. |
1 Introduction
Among cancers worldwide, liver cancer ranks seventh in incidence and second in mortality. The incidence rate is increasing year by year.1 Hepatocellular carcinoma (HCC) is the predominant form of liver cancer, accounting for about 90% of cases.2,3 Some projections indicate that by 2025 there will be one million liver cancer patients per year worldwide.4 Prevalence is generally higher in men than in women, and the risk is significantly increased in middle-aged and older (>40) people.5 The most significant risk factor for the development of HCC is cirrhosis, of which hepatitis viruses (including hepatitis B and C) cause about 50% or more. Although, with the emphasis on antiviral therapy, the risk of infection has been reduced compared to before, patients with cirrhosis after viral clearance are still a high-risk group.6–8 In addition, HCC due to alcoholic and non-alcoholic chronic liver disease (e.g. fatty liver, steatohepatitis) is increasing.9–11 The early stage of limited HCC lacks obvious symptoms, while the clinical manifestations of advanced HCC include medium-sized liver and spleen, ascites, jaundice, anorexia, accompanied by weight loss, and upper abdominal discomfort and pain. HCC is highly aggressive and often metastasizes in distant organs such as lung and stomach.12–14 Most cases are found to be advanced, and the prognosis and survival rate are extremely poor.15 Despite our improved understanding of the pathogenesis and molecular level of HCC, the differences in its etiology and genetic mutations have led to poorer treatment outcomes.16–18 Nowadays, the rise of nanotechnology can provide new ideas and methods for the early diagnosis and treatment of HCC.
Nanotechnology, mainly the modification of different nanoparticles (NPs), has been widely used in biomedical research, especially in oncology diagnostics.19 NPs have many irreplaceable advantages; its small diameter makes it easy for the human body to metabolize; its large surface area allows it to load therapeutic drugs, increase the duration of drug action, improve the effect while reducing drug concentration and mitigate drug side effects.20–22 Specific enrichment of NPs in tumor tissues is a prerequisite for nondestructive in vivo tumor diagnosis and targeted therapy. This is achieved by two main mechanisms, passive targeting, which exploits the enhanced penetration and retention (EPR) effect, and active targeting, which involves loading nanomaterials with recognition ligands for tumor marker molecules.23–25 Low toxicity and biocompatible nanocarriers have also been the focus of research. For example, a biodegradable copolymer polylactic acid-ethanolic acid (PLGA), has been approved by FDA (Food and Drug Administration, USA)/EMA (European Medicines Agency).26 It produces the same byproducts (H2O and CO2) as normal human metabolism through ester bond hydrolysis of lactic and glycolic acids.27 In addition, there are ultra-small size NPs with nanoenzyme functions that have anti-inflammatory, antioxidant and anti-tumor effects.28–31
Although NPs-loaded drug therapy can increase its efficacy, the effect of single treatment remains mediocre. Multimodal diagnostics (using light, sound, heat, etc.) greatly improves the diagnosis rate and treatment of tumors.32–35 Herein, this paper reviews the progress of nanotechnology application for HCC in recent years, which opens up new areas for early diagnosis, improved efficacy and prolonged survival of HCC (Fig. 1).
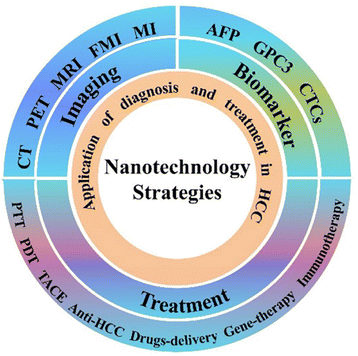 |
| Fig. 1 Nanotechnology strategies for hepatocellular carcinoma. | |
2 Diagnosis
2.1 Imaging
2.1.1 Computed tomography (CT) and positron emission tomography (PET). CT is a common imaging test for hepatocellular carcinoma.36,37 Since the main source of blood supply for hepatocellular carcinoma differs from that of normal liver tissue, with the former being the hepatic artery and the latter being mainly the portal vein, enhancement of the arterial phase and fading of the delayed phase will occur in contrast imaging. It has a sensitivity of about 80% and a specificity of over 90% for the diagnosis of HCC.38,39 Iodine compounds have a high X-ray absorption coefficient and are currently commonly used contrast agents for CT. However, hypertonic iodine contrast agents increase the burden on the kidneys when metabolized, and a small number of patients are allergic to iodine contrast agents.40 It has been shown that the sensitivity and resolving power of CT for HCC are related to the contrast concentration.41In recent years, the advent of nano-CT contrast agent overcomes the shortcomings of conventional contrast agents. Nano-contrast agents are modified to make them persistent and specific in imaging HCC. Kim et al. reported a polyethylene glycol (PEG)-coated gold-based NPs (GNPs) with a diameter of approximately 30 nm that had a long retention time in the blood compared to iodine contrast agents. Moreover, because gold has a higher absorption coefficient than iodine, the CT contrast between normal liver tissue and tumor was improved 2-fold after injection of GNPs in the tail vein of tumor-bearing rats42 (Fig. 2). Based on the above study, Rand et al. used a layer-by-layer coating method of polyelectrolytes to coat anionic polyacrylic acid and cationic polypropylene amine hydrochloride on GNPs layer by layer to increase the affinity of GNPs with cells and reduce their concentration at the time of use.43 Meanwhile, the application of spatial harmonic imaging (SHI) to the images formed by X-ray scattering improved the accuracy of detecting HCC cells down to several millimeters in diameter.43 For now, the cost of gold-based nanoparticles may be higher than that of iodine-based contrast media, especially in developing countries, which is difficult to apply. It is believed that a balance between efficacy and price will be found in the near future.
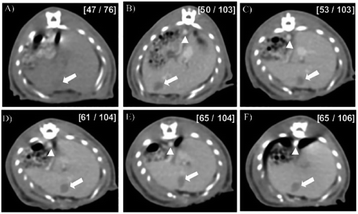 |
| Fig. 2 CT images of rat HCC model after injection of GNPs Images were obtained at (A) 0 h (before injection) and (B) 5 min, (C) 1 h, (D) 2 h, (E) 4 h, and (F) 12 h after injection. Numbers in brackets are the HU values of the hepatoma regions (left) and the surrounding normal liver parenchyma (right). Reproduced with permission from ref. 38. Copyright American Chemical Society, 2007. | |
Micro-CT is a non-invasive imaging tool commonly used in rodents. It helps us to perform preclinical experiments on animal models.44 A team of researchers used micro-CT on liver cancer model mice to compare the effects of two alkaline earth metal nanocontrast agents, Exitron nano 6000/12
000. These two contrast agents target hepatic Kupffer cells and have the advantage of high contrast and long duration (>4 h). In particular, the extended contrast of Exitron nano 12000 on the hepatic vascular system enables semi-automatic segmentation of the liver and tumor, providing a dynamic monitoring method for quantitative estimation of HCC load.45 Encouragingly, Anton et al. developed a completely non-toxic 2-3-5-triiodo-α-tocopherol (α-tocopherol is vitamin E) contrast emulsion to achieve quantification of micro HCC detection and volume and to stay in the liver for 3 months.46 (Fig. 3) In addition, a labeled human serum albumin microspheres (HSAM) was used for imaging and became effective after 2 h and remained stable in the liver after 72 h.47
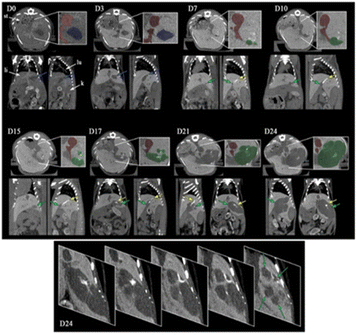 |
| Fig. 3 Micro-CT imaging of tumor-bearing mice after intrahepatic injection of α-tocopherol nanoemulsion and the pictures report maximum intensity projection of transverse section, sagittal and coronal section of the liver, for 0, 3, 7, 10, 15, 17, 21 and 24 days after injection of the cells and iodinated nano-emulsions as contrast agent. At D0, are indicated in the figure the localizations of the stomach (st), liver (li), lung (lu) and kidney (k). Insets show details with colored filters that guide the eye to differentiate the vascularization (in red), cell injection cavity (in blue),and tumor (in green), completed by arrows in the other views. Yellow arrows point out the hyper-contrast zones that appear during the tumor growth. The bottom part, at D24, shows transverse sections of tumor for different depths, emphasizing the different tumor nodules with green arrows. | |
PET uses positron nuclide labeling of body metabolites such as glucose as the imaging agent and reflects metabolic changes through the uptake of the imaging agent by the lesion, thereby detecting microscopic tumors and invasive metastases of tumors and providing a high spatial resolution.48 PET is a second-line option for HCC diagnosis.49,50 Some research teams have modified liposomes (LPs) and radionuclides at the nanoscale to target imaging ligands to diseased tissues or organs using their specific targeting effects, thereby increasing the image signal/contrast of the target tissue at lower concentrations.51 Unfortunately, there are few reports on the direct application of these nanoscale modifications in the diagnosis of HCC. The possible reason is that PET can play a greater advantage in the diagnosis of distant metastasis of tumor. Usually, it is difficult to recommend animal models of tumor metastasis, which may be a direction for future research and development.
2.1.2 Magnetic resonance imaging (MRI). MRI is not only non-radioactive, but also more specific than CT in the diagnosis of HCC.1,52 However, the sensitivity and specificity of conventional MRI diagnosis are still unsatisfactory. Gadolinium diethylenetriamine pentaacetic acid (Gd DTPA) is a commonly used contrast agent in clinical practice, but it has a short half-life in the blood and lacks specificity for the target tissue. In this regard, Chen et al. achieved longer retention and better results in HCC imaging by assembling the contrast agent with polylactic acid (PLA)-PEG in a nanocomplex.53In addition to Gd-based contrast agents, Manganese may be a potential metal to replace Gd. Researchers have developed an Mn-based contrast agent encapsulated in apoferritin (Mn-Apo), which exhibits different high and low T1 weighting signal intensities due to its different proportions in hepatocytes and HCC cells. In addition, further studies have shown that Mn-Apo cellular uptake correlates with SCARA5 (an oncogenic molecule) receptor expression, and using SCARA5 as a molecular marker for HCC, other invisible lesions can be detected by Mn-Apo54 (Fig. 4).
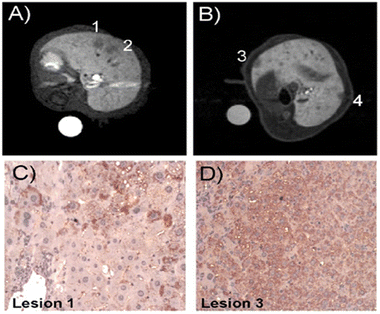 |
| Fig. 4 Histological validation of MRI lesion detection. T1-weighted MR images (liver region), of HBV-Tg transgenic mice 20 min after the injection of Mn-Apo (A) and Gd–BOPTA (B), respectively. Lesions 1 and 2 were easily detectable only after Mn-Apo administration. However, lesions 3 and 4 were detectable only after Gd–BOPTA injection. (C and D) Expression pattern of SCARA5 in HBV-Tg. (C) Immunohistochemical staining of lesion 1 showing low Mn-Apo uptake. SCARA5 was observed only in some HCC cells. (D) Immunohistochemical staining of lesion 3 showing higher Mn-Apo uptake. SCARA5 was present in almost all cancer cells. Reproduced with permission from ref. 50. Copyright John Wiley & Sons, Ltd, 2012. | |
Moreover, manganese conjugated nanodiamond complexes improve the interaction between paramagnetic ions and water molecules, thus improving MRI vertical (T1) and horizontal relaxation (T2).55 3,4-Dihydroxycinnamic acid-functionalized superparamagnetic iron oxide nanoparticles (IONPs) are biocompatible and can produce significant contrast enhancement in T2 weighting.56 Nano bullets (Mn-DTPA-F-MSNs) coupled with mesoporous silica nanoparticle (MSN) were able to detect glutathione (GSH) along with the enhancement of MRI.57
2.1.3 Fluorescence molecular imaging (FMI). More than two-thirds of patients who have undergone hepatectomy for HCC develop tumor recurrence after 5 years, and the possible cause is often explained by complete resection of the tumor lesion.58 The FMI-guided surgery helped the surgeon preserve a greater degree of normal liver tissue and remove the tumor lesion intact.59,60 A commonly used fluorescent probe in clinical practice is indocyanine green (ICG), which aggregates in HCC tissue and emits near-infrared light upon activation.61 Currently, the two main limitations of ICG fluorescence imaging in HCC surgery are the limited depth of tissue penetration (<10 mm) and the false positive rate (45%).62,63 To overcome these shortcomings, the nano-flower particles formed by the combination of SiO2 coated Au and antibody have stronger tissue penetration and luminescence properties.64 A novel europium oxide nanoparticle mediate fluorescent images from emissive drugs and detect even ultra-small tumors below 1 mm.65 (Fig. 5) Cdots developer rapidly penetrate the membrane of HCC cells and maintain its position for 24 h, emitting a bright green fluorescence different from that of normal hepatocytes66 (Fig. 6).
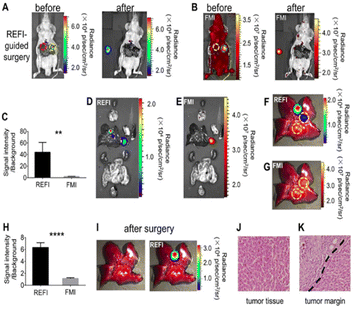 |
| Fig. 5 REF image-guided cancer surgery of the orthotopic HCC tumor-bearing mice. (A) Representative REFI of the mouse after the laparotomy (left). After the REF image-guided cancer surgery, the mouse and the resected tumor were also imaged for REFI (right). (B) Representative fluorescent images of the mouse before and after the tumor resection. (C) Comparison of SNR of REFI and FMI. (D) and (E) REFI and FMI of all the organs and the orthotopic HCC. (F) and (G) Representative REF and FM image of the mouse liver, respectively. (H) Comparison of quantification of SNR of REFI and FMI of the mouse liver. (I) Photograph and REF image of the mouse liver after the tumor second resection, respectively. (J) and (K) H&E of the orthotopic HCC and surgical margin, respectively. Reproduced with permission from ref. 61. Copyright Elsevier Inc, 2017. | |
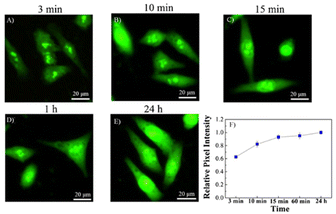 |
| Fig. 6 Fluorescence images of HepG2 cells after incubating with appropriate medium containing Cdots for different time ((A) 3 min, (B) 10 min, (C) 15 min, (D) 1 h, (E) 24 h). (F) Relative pixel intensity of the corresponding fluorescence images (the pixel intensity from HepG2 cells incubated with Cdots for 24 h was defined as 1.0). | |
Recent studies have found that viscosity maintains one of the parameters of microenvironmental homeostasis in organisms, and its abnormal changes can lead to the development of many diseases, such as tumors and diabetes.67 In response to the significantly higher viscosity in hepatocellular carcinoma cells, Li et al. developed a fluorescent probe specific for hepatocytes (HT-V) that not only allows bioimaging but also sensitively detects differences in viscosity for in vivo diagnosis of HCC.68
Furthermore, Surface-Enhanced Raman scattering (SERS) has been reported to be more sensitive and photostable than fluorescence imaging. The ability of Raman imaging of SERS NPs to detect HCC is more accurate and stable than that of ICG.69
2.1.4 Multimodal imaging. Both CT and MRI have limitations in diagnosing swelling, such as penetration depth and specificity.70 Nanotechnology-based multimodal imaging combines the advantages of two or more imaging modalities to compensate for the shortcomings of a single imaging modality, providing a more accurate method for the diagnosis of HCC.71,72 Folic acid (FA) combined with Gd functionalization to form nanodroplets (Gd-NDs-FA) accumulate within the tumor area and exhibit intense ultrasound (US) and MRI signal enhancement.73 A novel nanoprobe formed by HCC-targeting peptide, NIR dye and Gd chelation with enhanced fluorescence and MRI dual-mode imaging for intraoperative detection of lesions smaller than 1 mm.74 Similarly, a rare-earth-doped NPs (dREs@Lips) has the same function.75 Cationic straight-chain starch (CA) and superparamagnetic iron oxide (SPIO) NPs modified to form nanospheres and loaded with small interfering RNA (siRNA) exhibited both good fluorescence and magnetic resonance imaging properties as well as inhibition of HCC growth.76 In addition, a multifunctional PEG-Ta2O5@CuS nanoprobe was designed to enhance gemstone spectral computer tomography (GSCT) and photoacoustic (PA) imaging while allowing ablation of HCC.77Ultrasonography is a routine tool for HCC screening, and unfortunately, the use of NPs in HCC ultrasonography has not been reported compared to other imaging examinations.
2.2 Serum biomarkers
2.2.1 Alpha-fetoprotein (AFP). AFP is one of the most commonly used markers for the diagnosis of HCC. Although some studies have shown that less than 20% of HCC patients have an AFP level of 400 ng mL−1, the combination of AFP testing and imaging greatly improves the accuracy of diagnosis.78 In addition, AFP testing can help in managing the treatment process and predict the prognosis of patients with HCC.79,80 Traditional methods (e.g. enzyme-linked immunosorbent assay, ELISA) for the detection of AFP have disadvantages such as low sensitivity, complex and time-consuming operation, and expensive equipment and consumables.81,82Over the past decade, a number of low cost, efficient and rapid AFP assays based on nano-strategies have been developed.83–85 They usually use electrochemiluminescence (ECL) and electrochemiluminescence immunoassays for the detection and quantification of markers.86,87 Metal nanoparticles, especially gold-based nanoparticles, are usually hybridized with other nanomaterials to make biosensors for detecting AFP. NPs assembled in nano-hydroxyapatite prepared piezoelectric immunosensor for highly sensitive detection by immunoreactivity.88 The combination of Fe3O4–Au and CdS–Au NPs into a sandwich core ECL immunosensor has the advantages of magnetic separation and the stability and biocompatibility of Au, with a detection range of 0.0005 ∼ 5.0 ng mL−1.89 Organic NPs, such as carbon nanotubes, quantum dots, and graphene, have applications in this area as well.83,90 Similarly, other non-metallic nanoparticles (e.g. silica NPS) are also widely used in the fabrication of biosensors.85 Herein, we have selected some representative NPs to summarize (Table 1).
Table 1 Different NPs in detecting AFPa
Category |
Types of nanoprobe |
Detection method |
Ref. |
CV: cyclic voltammetry; HRP: horseradish peroxidase; NiHCF: nickel hexacyanoferrate; LSPR: localized surface plasmon resonance; GNMA: gold nano-m arrays; Pd/APTES-M-CeO2-GS: 3-aminopropyltriethoxysilane supported Pd octahedral nanoparticles; HAT: human alpha-thrombin; Met-Au NCs: methionine-stabilized Au nanoclusters; GNTC: gold nano-truncated cone; GNA: gold nanodot array; PEC: photoelectrochemical; CNT: carbon nanotube SAW: surface acoustic wave; Cu-Flo. NMs: copper-based nanosheet micromaterial; Cys: cysteine; GCE: glassy carbon electrode; ZIS: ZnIn2S4; 3D-AIO: 3D printing all-in-one dual-modal immunoassay. |
Metal-based |
Ru-silica@Au |
CV and ECL |
91 |
Ferrocenemonocarboxylic–HRP@Pt |
Multiple amplification immunoassay |
92 |
Fe3O4–Au/Ab1/AFP/Ab2/CdS–Au |
ECL |
89 |
Au Pt NPs\HRPNPs\NiHCF NPs |
ECL |
84 |
GNMA |
LSPR/iImmunoassay |
93 |
Pd/APTES-M-CeO2-GS |
ECL |
94 |
Multi-HAT-AuNP-Ab2 |
ELISA |
95 |
Met-Au NCs |
ECL |
96 |
GNTC |
LSPR/enzymatic precipitation |
97 |
GNA |
LSPR/enzymatic precipitation |
98 |
MIL-101(Cr) & CdSe QDs |
PEC |
99 |
MoS2/Au NPs |
Love-mode SAW |
100 |
GCE/3D-Cu-Flo.@AuNPs-Cys |
3D-ECL |
101 |
SnO2 Nanotubes |
PEC |
102 |
Organic |
GO-HRP |
Immunoassay |
90 |
MWCNT |
Colorimetric and chemiluminescent assays |
103 |
Multicolor QDs |
Immunoassay |
104 |
Nanocomplexes |
Si NPs/SiC@Ag |
SERS |
105 |
g-C3N4@TiO2 NTs |
PEC |
106 |
GCE/CNTs-gC3N4 (Cu) |
ECL |
107 |
ZIS |
3D-AIO/PEC |
108 |
2.2.2 Glypican-3 (GPC3). GPC3 is a polysaccharide molecule that is highly expressed in HCC.109 It can be released into the serum and is an emerging serum marker for HCC diagnosis.110,111 To improve its accuracy, the researchers developed nano-luciferase with dual functionality to detect trace amounts of GPC3 in serum.112 In addition, attaching specific peptides to gold nanoparticles can be used as a probe to detect GPC3 specificity.113
2.2.3 Circulating tumor cells (CTCs). Metastatic spread of tumors is usually reported from CTCs, which are shed from the primary tumor through the hematologic route and colonize distant tissues.114 A large amount of predictive, disease progression information for HCC can be obtained by detecting CTCs in peripheral blood, providing reliable evidence for clinical diagnosis and individualized treatment evaluation.115 Because CTCs are extremely rare in blood, the specificity and accuracy of epithelial cell adhesion (EpCAM)-coated magnetic bead isolation assays are often inadequate for clinical applications.116 Xia et al. modified Fe3O4 nanobeads with small molecule NIR fluorophore MLP and EpCAM antibody with dual-mode selection and separation, which greatly improved the capture efficiency and detection purity of CTCs117 (Fig. 7). Similarly, a composite nanoparticle with Fe3O4 magnetic cores encapsulated in an anti-EpCAM-modified MIL-100 shell layer can be automatically degraded by MIL in an acidic environment, resulting in high activity of the captured CTCs.118 Reassuringly, a dual-targeted functionalized reduced graphene oxide membrane (DTFGF) detects as low as 5 HCC-CTCs in 1 mL of blood sample.119 It is foreseen that the application of nanotechnology in HCC-CTCs has this great potential.
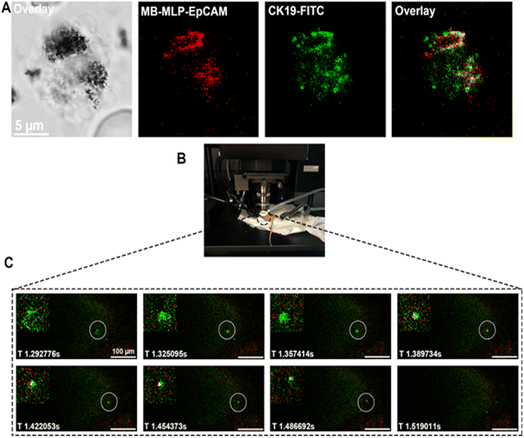 |
| Fig. 7 (A) Fluorescent images of the CTC captured by MB-MLP-EpCAM from clinical blood samples of HCC patients. The red fluorescence represents MB-MLP-EpCAM, and the green fluorescence represents CTC (CK19+) (B) the mouse fixation and imaging diagram. (C) Recognition of MB-MLP-EpCAM for GFP transfected HepG2 cells in vivo at different time points. Reproduced with permission from ref. 113. Copyright American Chemical Society, 2020. | |
3 Treatment
In patients with HCC, different tumor stages and patients' own nutritional status require individualized treatment plans. Clinically, the most commonly used staging system is the Barcelona Clinic Liver Cancer (BCLC) staging system, which guides the selection of different treatment options based not only on tumor growth and invasion, but also on liver function and overall physiological status.120,121 In the early stages of HCC, surgical resection, liver transplantation and ablation can be used. In the intermediate stage, local treatments, such as transarterial chemoembolization (TACE), are mainly used. In the late stage, systemic systemic therapy such as targeted therapy and immunotherapy are used. These treatments improved the course of each stage of the disease and increased the 5 year survival rate.122,123 Unfortunately, HCC is usually diagnosed at an advanced stage and treatment outcomes remain unsatisfactory. The treatment modalities and new drugs are constantly being explored and improved, and the application of nanotechnology has opened up a promising solution in which nanoparticles are designed into targeted, highly efficient regimens that provide personalized, precise treatment modalities.
3.1 Thermal ablation and photothermal therapy (PTT)
Ablation technique is the preferred method to treat early stage small HCC (especially <2 cm, single tumor), which is tumor necrosis by thermal and chemical methods.124 At present, two thermal ablation techniques, radiofrequency ablation (RFA) and microwave ablation (MWA), are more commonly used in clinical practice.125 The researchers focused on the improvement of the thermal ablation probe to achieve better treatment results. Shao et al. compared three nanoparticles CNT, Au and Fe3O4 as therapeutic probes for RFA. These probes have higher heat transfer efficiency and lower maximum temperatures, with a more uniform temperature distribution in the CNT.126 A single-phase calcium phosphate biomineral NPs for contrast imaging under MRI to guide RFA.127 (Fig. 8) In addition, in vivo excitation of nano-hydroxyapatite solutions with high-intensity ultrasound enhances thermal ablation and has a higher biosafety profile.128 Recurrence after ablation therapy is also a rather difficult problem.129 Mannose-derived carbon dots (Man-CDs) can effectively capture tumor-associated antigens and other risk factors after MWA treatment, induce body-specific immune responses, and maintain long-term immune effects to inhibit HCC recurrence.130
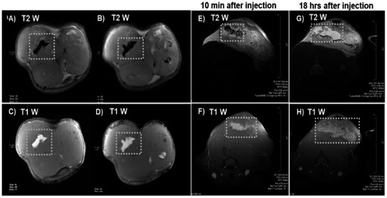 |
| Fig. 8 Dual T1–T2 contrast variation after intra-tumoral injection. (A and B) T2 weighted and (C and D) T1 weighted MR image (coronal section) of subcutaneous tumor after intratumoral nCP: Fe injection. (E) T2 weighted and (F) T1 weighted MRI of subcutaneous tumor (axial sections) 10 min after intratumoral injection of nCP: Fe. (G) T2 weighted and (H) T1 weighted MRI of subcutaneous tumor (axial sections) 18 hours after intratumoral injection of nCP: Fe. Sample injected regions are shown in white dotted boxes. | |
PTT is a recent technique to thermally ablate local tumors by converting light energy into heat through photothermal converters.131 For PTT treatment of HCC, selective uptake of photothermal agents in liver tumor cells, photothermal conversion efficiency and biosafety have been hot topics of research. Metals and metal sulfides NPs are highly efficient photothermal agents. Their biotoxicity is reduced by their encapsulation or modification. Graphene oxide (MGO) has become a hot research topic due to its low biotoxicity, large specific surface area and efficient photothermal conversion efficiency. For example, encapsulation of gold nanorods (AuNRs) inside graphene oxide NPs (NGO) or mesoporous silica shells to form nanocapsules for improved safety and photothermal conversion efficiency.132–134 Similarly, Wen et al. successfully constructed an organic–inorganic hybrid nanomaterial (MGO@CD-CA-HA) by encapsulating β-cyclodextrin-cholic acid-hyaluronic acid polymer (CD-CA-HA) on FeO–MGO. It has high efficiency in photothermal conversion efficiency along with multi-targeting for HCC.135 Copper sulfide wraps hollow spheres with membrane proteins from tumors and macrophages, which can also be loaded with chemotherapeutic agents inside, creating a multimodal treatment for HCC.136 There are also some metal oxides, etc. that have been processed by bionic means and have shown the powerful therapeutic potential of PTT. We have summarized the commonly used photothermal agents and modifications.
3.2 Photodynamic therapy (PDT)
PDT is also a form of ablative treatment for HCC, which has the advantages of less trauma, shorter treatment time, lower side effects and higher repeatability.137 PDT kills tumor cells, destroys tumor blood vessels and stimulates activation of the immune system through reactive oxygen species (ROS), including superoxide anions and free radicals, produced by light-activated photosensitizers (PSs).138,139 Some conventional PSs have many problems such as low ROS generation efficiency and lack of targeting.140 The class of PSs includes organic PSs (e.g. porphyrins), natural products, metal complexes, metal–organic backbones (MOFs), and metal-based nanostructures.141 In addition, some researchers have modified PSs by FA or HA to make tumor-targeting probes, thus further improving their tumor specificity and water solubility.142 The nano-strategy overcome the above disadvantages, and NPs target PSs delivery to HCC cells through EPR effect, which also enhance the effect of PDT.143 Photo-sensitive sulfated zinc phthalocyanine loaded on nanoliposomes significantly enhances the accumulation in HCC cells.144 Similarly, Xu et al. developed a light-triggered nanomicellular beam delivery platform loaded with PSs and paclitaxel (PTX), which not only has the effect of PDT with chemotherapy, but also synergizes with anti-PD-L1 antibody for immunotherapy.145 (Fig. 9) In addition, novel PSs (PS-GNPs) formed by the combination of Pu-18-N-butylimide-NMGA and chloroauric acid in a mouse model of hepatocellular carcinoma showed upregulation of ROS-associated apoptosis proteins and increased DNA fragmentation.146 From polygalactose-co-cinnamaldehyde polyexcipients (PGCA) and the photosensitizer pheophorbide A self-assembled into PGCA@PA NPs, the uptake of NPs by tumor cells was facilitated by the expression of galactose receptors, while the combination of the two enhanced the PDT.147
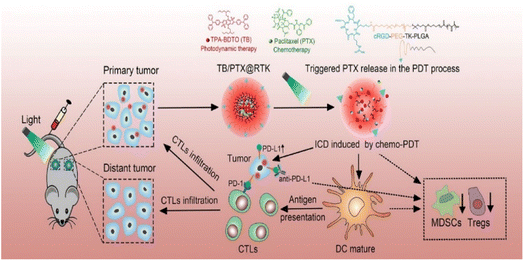 |
| Fig. 9 All-in-one multimodal nanotherapy platform. | |
3.3 TACE
TACE is a minimally invasive approach to treat unresectable HCC. Chemotherapeutic agents and embolic agents are injected into the hepatic artery to reduce the blood supply to HCC and induce necrosis. However, the efficacy of TACE is unsatisfactory, and although drug-eluting beads (DEBs) are used, there are still problems with its low response rate, as well as chemotherapeutic drug escape and tumor drug resistance.148–150 In response, Kong and his colleagues used Arsenic trioxide (ATO) encapsulated in biocompatible PLGA for TACE with strong tumor suppressive effects.151 A novel binary progressive micro- and nanostructured embolic microspheres were developed for application in TACE, which have good swelling properties and both tumor embolization and inhibition of tumor angiogenesis.152 MnO2/vitraphen (BPD) nanocomposites as embolic agents to promote tumor vascular endothelial cell apoptosis and predict treatment efficacy by showing tumor vascular density through multimodal imaging153 (Fig. 10).
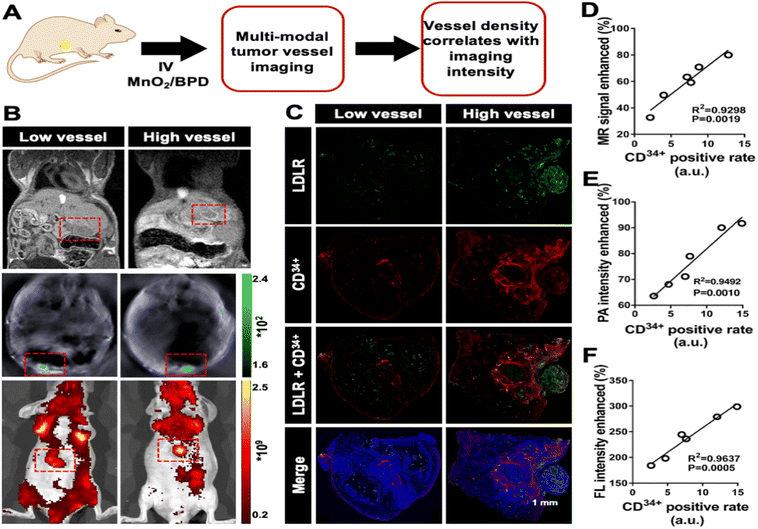 |
| Fig. 10 Vessel density imaging predicts embolization effect. (A) Experimental design. (B) Example of MR, PA, and FL images of hep-G2 tumors presenting low and high vessel intensity. (C) Whole slice imaging of immunofluorescence. (D) Linear regression between vessel density and MR signal enhancement. (E) Linear regression between vessel density and PA intensity enhancement. (F) Linear regression between vessel density and FL intensity enhancement. Reproduced with permission from ref. 146. Copyright American Chemical Society, 2020. | |
Furthermore, nanosized modifications of chemotherapeutic or targeted drugs are also used directly for TACE treatment. Nanosized PTX or sorafenib (SRF) not only improves the chemotherapeutic effect, but also increases the safety without introducing additional carriers.154,155 Wang et al. developed magnetic liquid metal NPs (Fe@EGaIn) loaded with calcium alginate (CA) microspheres with CT/MR dual-mode imaging in addition to embolization and drug coating functions.156 The flexibility and multiple functionalization of this microsphere provides a template for future development strategies.
3.4 Direct anti-HCC effect of NPs
From the current literature, some NPs act directly on tumor cells to induce apoptosis. For example, direct treatment of HCC with selenium NPs may act by regulating DNA methylation, where the molecular mechanisms include: (a) reduced the ratio of 8-OHdG/2-dG production to avoid DNA damage caused by oxidative stress, (b) upregulated the expression of two oncogenes, Akr1b10 and ING3, and (c) significantly reduced the expression of Foxp1 gene.157 AgNPs promotes caspase-3 (signaling pathway is p53, AKT and MAPKs) to participate in cell apoptosis through ROS generation.158 Moreover, AgNPs also reduce the protective effect of liver related biochemical indexes, such as aspartate transaminase (AST) and gamma glutamyltranspeptidase (GGT).159 Nano β-TCP not only induced apoptosis through ROS, but also blocked the G0/G1 cell cycle of HCC cells and interfered with the expression of cyclin.160 Niu et al., through bioinformatics analysis, found that nano-sio2 can up-regulate tumor cell-related death gene ZBP-1, which has a killing effect on HCC cells.161 Other research teams have also found that NPs such as AgO2, CeO2 and CuO2 have HCC-killing effects, but their molecular mechanisms are not yet clear.162
3.5 NPs for delivery of drugs
3.5.1 NPs for delivery of chemotherapeutic drugs. Different chemotherapeutic drugs were modified with nanotechnology to form functionalized NPs with stronger targeting and efficacy. Doxorubicin (DOX) encapsulated in biological metal organic frameworks (MOFs) is biologically low in toxicity and can be precisely administered.163 In addition, nigella sativa oil and DOX formed nanoemulsifiers with lower tumor-inhibiting concentrations, mitigating DOX toxicities and providing a reference for further oral administration.164 A polymeric micelle capable of rapid mucus penetration and villi absorption allows effective delivery of PTX into HCC by oral administration.165 A novel sulfhydryl polymer-based nanocomposite loaded with 5-fluorouracil (5-FU) reduces serum levels of liver enzymes in addition to targeting of HCC.166 Moreover, chemotherapeutic drugs such as cisplatin (CDDP) and ceramide have been nano-engineered.167 Herein, our team summarize the commonly used chemotherapeutic drug nanoparticles in recent years (Table 2).
Table 2 Different NPs for delivery of chemotherapeutic drugsa
Chemotherapy drugs |
Types of NPs |
Ref. |
ZFH-DGR: Zn2+-coordinated Fmoc His-Asp-Gly-Arg peptide; CAP: capsaicin; GA: glycyrrhetinic acid; HA: hyaluronic acid; AL-azo: alginate-azo; CH: chitosan; TLS11a: HCC-specific aptamer; TATp: TAT peptide; CAAP5: cystamine dihydrochloride (CA)-capped pillar[5]arene; G: galactose derivative; OPDEA: poly[2-(N-oxide-N,N-diethylamino)ethyl methacrylate]; PCL: poly(ε-caprolactone); NEMs: nanoerythrocyte membranes; OA: oleanolic acid; LCC: lipid-coated calcium carbonate; PEI: polyethylenimine; Bcl-2, Pl3K, Akt/PKB, and Stat-3 signaling pathways to inhibit HCC progression.188 In addition, silver nanocolloid delivery of betulinic acid (BA) and polymeric micelles delivery of ursolic acid (UA) both increased the bioavailability of natural drugs.189,190 |
DOX |
ZFH-DGR/DOX |
163 |
CAP/GA-sHA-DOX |
168 |
DOX/AL-azo/CH |
169 |
TLS11a-LB@TATp-MSN/DOX |
170 |
CAAP5G |
171 |
DOX@Fe3O4-ZIF-8 |
172 |
PTX |
OPDEA-PCL |
165 |
Poly(allylamine)-based amphiphile |
173 |
5-FU |
Thiolated polymer-based nanocomposite |
166 |
NEM-5-FULPs |
174 |
CDDP |
Algal-mediated CuO |
175 |
CDDP/OA-LCC |
176 |
ATO |
ZrO2 |
177 |
Ceramide |
NGO-PEG-PEI |
167 |
3.5.2 NPs for delivery of molecularly targeted drugs. Chemotherapeutic drugs are less effective and have more pronounced toxic side effects in HCC, especially in mid-to late-stage progressive HCC.178 Molecularly targeted drug therapy, which is the first and second line of treatment, is commonly used in clinical practice today. First-line drugs such as sorafenib (SRF) and lenvatinib (LEN) are multiple receptor tyrosine kinase inhibitors (TKIs) that can improve overall survival.179However, their effects are limited by their side effects and low bioavailability.180 Peptide nanogels with stimulatory response were developed for LEN delivery with good tumor suppression effect and few side effects.181 LPs carrying SRF and ganoderic acid are used as anti-HCC agents. They significantly inhibit tumor growth and inflammatory factors, as well as reduce liver enzyme levels.182 Mn-doped silica NPs loaded with SRF double deplete intracellular glutathione (GSH) and thus disrupt redox homeostasis in HCC cells.183 In addition, PEG galactose-coupled nanosolid lipid-loaded SRF has better pharmacokinetics and hepatic targeting.184
3.5.3 NPs for delivery of natural plant medicine. Natural plant drugs are used for anti-HCC treatment because of their low toxicity, but most of them have poor water solubility that limits their wide application.185 Curcumin (CU) is an anti-inflammatory and anti-cancer natural polyphenolic plant component with enhanced solubility and anti-cancer effects, which was modified into nano vesicles by Abbas et al.186 Similarly, CU mitochondrial delivery system based on polyamide dendrimer molecules increase mitochondrial ROS levels and induce apoptosis at lower doses.187 GA LPs interfere with various signaling proteins involved in HCC pathogenesis, altering.
3.6 NPs for delivery of gene therapy
Gene therapy is a promising treatment for HCC, introducing exogenous genes to correct or improve tumor symptoms. It needs to be efficient, safe and specific.191 RNAi is a promising gene silencing technology in HCC. The selective interaction between NPs and cellular molecules is a promising pathway for RNAi.192 Extracellular vesicle (TDEV) membranes isolated from hepatocellular carcinoma cells were mixed into lipid nanovacuoles (LEVs) as small interfering RNA (siRNA) vectors, which have homing (into parental HCC cells) targeting ability and exponential transfection efficiency to enhance their anti-tumor ability by gene silencing at tumor loci.193 The PEG- and GA-modified NGO adducts transporting siRNA have an effective VEGFa gene silencing effect.194 Similarly, Wang et al. developed a novel biodegradable cationic polymer based on disulfide linkages loaded with small interfering VEGF RNA (siVEGF) with anti-HCC angiogenic effects.195 Exosomes exogenously modified as microRNA (miRNA) carriers regulate the expression of their downstream target genes to inhibit HCC cell proliferation and migration.196 Furthermore, there are AuNPs, functionalized SeNPs, dipeptide NPs, etc. transporting different miRNAs to inhibit the corresponding downstream targets for HCC inhibition.197–199
3.7 NPs for immunotherapy
The use of immunotherapy in HCC has been a research point in recent years, where several immune checkpoint inhibitors such as Atezolizumab, Durvalumab and Nivolumab have been approved for use by the FDA, which activates the autoimmune system and allows immune cells to regain their killing power against tumor cells.200–202 Qiang et al. formed chimeras by CNT/PEI and Durvalumab, which inhibited the expression of trigger receptor-2 on myeloid cells (Trem2), prolonged Durvalumab release, increased the proportion of T cells and CD8+ T cells, and promoted apoptosis in HCC cells compared to Durvalumab alone.203 A novel nano-biomechanical probe was developed with which researchers tested the dissociation kinetics of three clinically approved PD-1 blocking monoclonal antibodies (Nivolumab, Pembrolizumab and Camrelizumab) and found a strong correlation between the three monoclonal antibodies and objective remission rates for HCC treatment, which could guide the screening, optimization and selection of therapeutic antibodies.204
Although the molecular mechanisms underlying chronic liver disease associated with HCC are unclear, factors associated with it, including a tumor-associated macrophage (TAM) and tumor microenvironment (TME), which are major factors as well as potential therapeutic targets.205 A transferrin cell membrane nanovesicle encapsulated with IR820-dihydroartemisinin can activate oxidative stress to promote PD-L1-mediated immune checkpoint blockage (ICB), reshape the tumor immune microenvironment, and achieve dual T cell (CD4+ T and CD8+ T) activation, and IR820 molecules can also generate ROS under ultrasound activation to achieve multiple, precise anti-HCC effects.206 In addition, a bicellular core–shell nanoparticle (TCN), TAM-based immunotherapy in conjunction with SRF, enhances m2-type TAM polarization for better synergistic anti-tumor effects.207
Moreover, an encapsulated ngc gel vaccine with tumor-specific neoantigens is used for the treatment and prevention of HCC. It activates an increase in 41BB+ CD8+ T cells, decreases the proportion of Foxp3+ CD25+ T cells, improves the intrahepatic immune microenvironment, and enhances the antitumor immune effect.208
4 Summary and outlook
HCC is a serious threat to human health as a malignant tumor of the digestive tract. Early diagnosis and effective treatment have been the focus of research. The advent of nanotechnology has brought promising modalities for the diagnosis and treatment of HCC. This paper summarizes the application of nanotechnology in these two aspects in recent years to provide further directions for future development. NPs have been extensively studied for HCC imaging applications. NPs-based contrast imaging agents can be used at low concentrations to enhance the contrast and resolution in CT/PET and MRI imaging. In FMI imaging, especially intraoperative imaging, the results of the study showed more accurate and stable results than ICG, which is currently commonly used in clinical practice. In addition, NPs have shown significant results in multimodal imaging of HCC. NPs can sensitively detect AFP, GPC3 and CTCs at low limits in serological marker tests, which can be extremely helpful in the early diagnosis of HCC. In the treatment of HCC, NPs can not only kill HCC cells directly, but also act as chemotherapeutic agents, targeted drugs, gene therapy and immune carriers for targeted delivery to HCC cells. In addition, the improvement of embolic agents in TACE by nanotechnology can improve their efficacy. Not only that, NPs also treat HCC by PTT and PDT.
Although several NPs have been approved by the FDA for clinical use, the safety of NPs has always been an inevitable issue during their application in humans. The magnitude of their toxicity during retention in the body, the process of metabolism, and the rate of excretion need to be further studied and demonstrated. In most of the references, the effectiveness or activity of these materials is usually only studied, while the toxicological properties have not been fully studied and investigated, and only short-term animal experiments have proved to be relatively safe. While the toxicity of organic composite NPs is generally considered negligible, the long-term toxicity of these nanomaterials has never really been considered. In addition, based on the existing literature, nanomaterials are stored at room temperature for one week to one month, during which there will be no precipitation, dissolution or precipitation, etc., so the formed NPs are considered to be relatively stable. In the literature, the efficacy of NPs after a period of time is not compromised. This is one of the reasons NPs have not entered clinical trials.
In conclusion, nanotechnology has a promising future in the diagnosis and treatment of HCC with great potential for improvement. For the early diagnosis of HCC, a combination of various methods (including imaging and serology, etc.) is needed to improve the diagnostic accuracy and lay the foundation for early treatment. In terms of treatment, multiple treatment modalities can be combined to reduce toxicity to normal hepatocytes, reduce drug resistance, and improve survival. Recently, the development of efficient diagnosis and treatment of integrated NPs is the direction of our efforts. The study of molecular mechanisms of NPs in HCC treatment may be the next step of effort.
Author contributions
Conceptualization, W. J. and Y. Z.; writing – original draft preparation, W. J. and Y. H.; writing – review and editing, W. J., Y. H. and X. M.; suggestions on revision, W. X.; funding acquisition, Y. Z. All authors have read and agreed to the published version of the manuscript. All authors have contributed substantially to the work reported.
Conflicts of interest
There are no conflicts to declare.
Acknowledgements
This work was funded by the National Natural Science Foundation of China, grant number 81872255, 62041101, and the Key Medical Talents Foundation of Jiangsu Province, grant number 2016KJQWZDRC-03.
References
- A. Villanueva, N. Engl. J. Med., 2019, 380, 1450–1462 CrossRef CAS PubMed.
- J. Petrick, A. Florio, A. Znaor, D. Ruggieri, M. Laversanne, C. Alvarez, J. Ferlay, P. Valery, F. Bray and K. McGlynn, Int. J. Cancer, 2020, 147, 317–330 CrossRef CAS PubMed.
- T. Akinyemiju, S. Abera, M. Ahmed, N. Alam, M. Alemayohu, C. Allen, R. Al-Raddadi, N. Alvis-Guzman, Y. Amoako, A. Artaman, T. Ayele, A. Barac, I. Bensenor, A. Berhane, Z. Bhutta, J. Castillo-Rivas, A. Chitheer, J. Choi, B. Cowie, L. Dandona, R. Dandona, S. Dey, D. Dicker, H. Phuc, D. Ekwueme, M. Zaki, F. Fischer, T. Fürst, J. Hancock, S. Hay, P. Hotez, S. Jee, A. Kasaeian, Y. Khader, Y. Khang, A. Kumar, M. Kutz, H. Larson, A. Lopez, R. Lunevicius, R. Malekzadeh, C. McAlinden, T. Meier, W. Mendoza, A. Mokdad, M. Moradi-Lakeh, G. Nagel, Q. Nguyen, G. Nguyen, F. Ogbo, G. Patton, D. Pereira, F. Pourmalek, M. Qorbani, A. Radfar, G. Roshandel, J. Salomon, J. Sanabria, B. Sartorius, M. Satpathy, M. Sawhney, S. Sepanlou, K. Shackelford, H. Shore, J. Sun, D. Mengistu, R. Topór-Mądry, B. Tran, K. N. Ukwaja, V. Vlassov, S. Vollset, T. Vos, T. Wakayo, E. Weiderpass, A. Werdecker, N. Yonemoto, M. Younis, C. Yu, Z. Zaidi, L. Zhu, C. Murray, M. Naghavi and C. Fitzmaurice, JAMA Oncol., 2017, 3, 1683–1691 CrossRef PubMed.
- F. Bray, J. Ferlay, I. Soerjomataram, R. Siegel, L. Torre and A. Jemal, CA Cancer J. Clin., 2018, 68, 394–424 CrossRef PubMed.
- S. Mittal, J. Kramer, R. Omino, M. Chayanupatkul, P. Richardson, H. El-Serag and F. Kanwal, Clin. Gastroenterol. Hepatol.Clin. Gastroenterol. Hepatol., 2018, 16, 252–259 CrossRef PubMed.
- F. Kanwal, J. Kramer, S. Asch, M. Chayanupatkul, Y. Cao and H. El-Serag, Gastroenterology, 2017, 153, 996–1005 CrossRef CAS PubMed.
- K. McGlynn, J. Petrick and H. El-Serag, Hepatology, 2021, 4–13, DOI:10.1002/hep.31288.
- H. El-Serag and F. Kanwal, Hepatology, 2014, 60, 1767–1775 CrossRef PubMed.
- C. Estes, H. Razavi, R. Loomba, Z. Younossi and A. Sanyal, Hepatology, 2018, 67, 123–133 CrossRef CAS PubMed.
- N. Ganne-Carrié and P. Nahon, J. Hepatol., 2019, 70, 284–293 CrossRef PubMed.
- F. Kanwal, J. Kramer, S. Mapakshi, Y. Natarajan, M. Chayanupatkul, P. Richardson, L. Li, R. Desiderio, A. Thrift, S. Asch, J. Chu and H. El-Serag, Gastroenterology, 2018, 155, 1828–1837 CrossRef PubMed.
- S. Katyal, J. Oliver, M. Peterson, J. Ferris, B. Carr and R. Baron, Radiology, 2000, 216, 698–703 CrossRef CAS PubMed.
- M. Natsuizaka, T. Omura, T. Akaike, Y. Kuwata, K. Yamazaki, T. Sato, Y. Karino, J. Toyota, T. Suga and M. Asaka, J. Gastroenterol. Hepatol., 2005, 20, 1781–1787 CrossRef PubMed.
- M. Le Grazie, M. Biagini, M. Tarocchi, S. Polvani and A. Galli, World J. Hepatol, 2017, 9, 907–920 CrossRef PubMed.
- A. Benson, M. D'Angelica, D. Abbott, D. Anaya, R. Anders, C. Are, M. Bachini, M. Borad, D. Brown, A. Burgoyne, P. Chahal, D. Chang, J. Cloyd, A. Covey, E. Glazer, L. Goyal, W. Hawkins, R. Iyer, R. Jacob, R. Kelley, R. Kim, M. Levine, M. Palta, J. Park, S. Raman, S. Reddy, V. Sahai, T. Schefter, G. Singh, S. Stein, J. Vauthey, A. Venook, A. Yopp, N. McMillian, C. Hochstetler and S. Darlow, J. Natl. Compr. Cancer Netw., 2021, 19, 541–565 Search PubMed.
- K. Schulze, S. Imbeaud, E. Letouzé, L. Alexandrov, J. Calderaro, S. Rebouissou, G. Couchy, C. Meiller, J. Shinde, F. Soysouvanh, A. Calatayud, R. Pinyol, L. Pelletier, C. Balabaud, A. Laurent, J. Blanc, V. Mazzaferro, F. Calvo, A. Villanueva, J. Nault, P. Bioulac-Sage, M. Stratton, J. Llovet and J. Zucman-Rossi, Nat. Genet., 2015, 47, 505–511 CrossRef CAS PubMed.
- D. Galun, D. Mijac, A. Filipovic, A. Bogdanovic, M. Zivanovic and D. Masulovic, J. Pers. Med., 2022, 12 Search PubMed.
- J. Zucman-Rossi, A. Villanueva, J. Nault and J. Llovet, Gastroenterology, 2015, 149, 1226–1239 CrossRef CAS PubMed.
- S. Zhang, Q. Li, N. Yang, Y. Shi and X. Dong, Adv. Funct. Mater., 2019, 29, 190685.1–190685.9 Search PubMed.
- S. Zhang, C. Cao, X. Lv, H. Dai, Z. Zhong, C. Liang, W. Wang, W. Huang, X. Song and X. Dong, Chem. Sci., 2020, 11, 1926–1934 RSC.
- D. Chen, Q. Tang, J. Zou, X. Yang, W. Huang, Q. Zhang, J. Shao and X. Dong, Adv. Healthcare Mater., 2018, 7, e1701272 CrossRef PubMed.
- S. Mohamed, S. Veeranarayanan, T. Maekawa and D. Sakthi Kumar, Adv. Drug Deliv. Rev., 2019, 138, 18–40 CrossRef PubMed.
- F. Danhier, J. Controlled Release, 2016, 244, 108–121 CrossRef CAS PubMed.
- Y. Cai, Q. Tang, X. Wu, W. Si, Q. Zhang, W. Huang and X. Dong, ACS Appl. Mater. Interfaces, 2016, 8, 10737–10742 CrossRef CAS PubMed.
- R. Petros and J. DeSimone, Nat. Rev. Drug Discov., 2010, 9, 615–627 CrossRef CAS PubMed.
- H. Makadia and S. Siegel, Polymers, 2011, 3, 1377–1397 CrossRef CAS PubMed.
- S. Rezvantalab, N. Drude, M. Moraveji, N. Güvener, E. Koons, Y. Shi, T. Lammers and F. Kiessling, Front. Pharmacol., 2018, 9, 1260 CrossRef CAS PubMed.
- J. Kim, G. Hong, L. Mazaleuskaya, J. Hsu, D. Rosario-Berrios, T. Grosser, P. Cho-Park and D. Cormode, ACS Appl. Mater. Interfaces, 2021, 13, 60852–60864 CrossRef CAS PubMed.
- S. Ning, Y. Zheng, K. Qiao, G. Li, Q. Bai and S. Xu, J. Nanobiotechnol., 2021, 19, 344 CrossRef PubMed.
- A. Alhalmi, S. Beg, K. Kohli, M. Waris and T. Singh, Curr. Drug Targets, 2021, 22, 779–792 CrossRef CAS PubMed.
- F. Graur, A. Puia, E. I. Mois, S. Moldovan, A. Pusta, C. Cristea, S. Cavalu, C. Puia and N. Al Hajjar, Materials, 2022, 15, 3893 CrossRef CAS PubMed.
- Q. Tang, W. Si, C. Huang, K. Ding, W. Huang, P. Chen, Q. Zhang and X. Dong, J. Mater. Chem. B, 2017, 5, 1566–1573 RSC.
- W. Xiao, P. Wang, C. Ou, X. Huang, Y. Tang, M. Wu, W. Si, J. Shao, W. Huang and X. Dong, Biomaterials, 2018, 183, 1–9 CrossRef CAS PubMed.
- Y. Wang, X. Huang, Y. Tang, J. Zou, P. Wang, Y. Zhang, W. Si, W. Huang and X. Dong, Chem. Sci., 2018, 9, 8103–8109 RSC.
- G. Liu, S. Zhang, Y. Shi, X. Huang, Y. Tang, P. Chen, W. Si, W. Huang and X. Dong, Adv. Funct. Mater., 2018, 28, 1804317.1–1804317.11 Search PubMed.
- C. Chang, H. Chen, Y. Chang, M. Yang, C. Lo, W. Ko, Y. Lee, K. Liu and R. Chang, Comput. Methods Programs Biomed., 2017, 145, 45–51 CrossRef PubMed.
- European Association for Study of Liver and European Organisation for Research and Treatment of Cancer, European Association for Study of Liver; European Organisation for Research and Treatment of Cancer. EASL-EORTC clinical practice guidelines: management of hepatocellular carcinoma, Eur. J. Cancer, 2012, 48(5), 599–641 CrossRef PubMed ; Erratum in: Eur. J. Cancer, 2012, 48(8), 1255–1256, PMID: 22424278.
- O. Matsui, S. Kobayashi, J. Sanada, W. Kouda, Y. Ryu, K. Kozaka, A. Kitao, K. Nakamura and T. Gabata, Abdom. Imaging, 2011, 36, 264–272 CrossRef PubMed.
- L. Roberts, C. Sirlin, F. Zaiem, J. Almasri, L. Prokop, J. Heimbach, M. Murad and K. Mohammed, Hepatology, 2018, 67, 401–421 CrossRef PubMed.
- L. Xiang, N. Anton, G. Zuber and T. Vandamme, Adv. Drug Deliv. Rev., 2014, 76, 116–133 CrossRef PubMed.
- S. T. Schindera, L. F. Hareter, S. Raible, J. C. Torrente and Z. Szucs-Farkas, Invest. Radiol., 2012, 47, 197–201 CrossRef PubMed.
- K. Dongkyu, P. Sangjin, J. H. Lee and Y. Y. Jeong, J. Am. Chem. Soc., 2007, 129, 12585 Search PubMed.
- D. Rand, V. Ortiz, Y. Liu, Z. Derdak, J. Wands, M. Tatíček and C. Rose-Petruck, Nano Lett., 2011, 11, 2678–2683 CrossRef CAS PubMed.
- S. Prajapati and C. Keller, J. Vis. Exp., 2011, 27, 2377 Search PubMed.
- J. Rothe, I. Rudolph, N. Rohwer, D. Kupitz, B. Gregor-Mamoudou, T. Derlin, C. Furth, H. Amthauer, W. Brenner, R. Buchert, T. Cramer and I. Apostolova, Acad. Radiol., 2015, 22, 169–178 CrossRef PubMed.
- N. Anton, A. Parlog, G. B. About, M. F. Attia, M. Wattenhofer-Donzé, H. Jacobs, I. Goncalves, E. Robinet, T. Sorg and T. F. Vandamme, Sci. Rep., 2017, 7, 13935 CrossRef PubMed.
- H. Ni, C. Yu, S. Chen, L. Chen, C. Lin, W. Lee, C. Chuang, C. Ho, C. Chang and T. Lee, Appl. Radiat. Isot., 2015, 99, 117–121 CrossRef CAS PubMed.
- B. Ariff, C. Lloyd, S. Khan, M. Shariff, A. Thillainayagam, D. Bansi, S. Khan, S. Taylor-Robinson and A. Lim, World J. Gastroenterol., 2009, 15, 1289–1300 CrossRef PubMed.
- B. Howard and T. Wong, Radiol. Clin. North Am., 2021, 59, 737–753 CrossRef PubMed.
- S. Kwee, M. Tiirikainen, M. Sato, J. Acoba, R. Wei, W. Jia, L. Le Marchand and L. Wong, Cancer Res., 2019, 79, 1696–1704 CrossRef CAS PubMed.
- M. Silindir, A. Özer and S. Erdoğan, Drug. Deliv., 2012, 19, 68–80 CrossRef CAS PubMed.
- A. Karaosmanoglu, M. Onur, M. Ozmen, D. Akata and M. Karcaaltincaba, Semin. Ultrasound CT MRI, 2016, 37, 533–548 CrossRef PubMed.
- Z. Chen, D. Yu, S. Wang, N. Zhang, C. Ma and Z. Lu, Nanoscale Res. Lett., 2009, 4, 618–626 CrossRef CAS PubMed.
- S. Geninatti Crich, J. Cutrin, S. Lanzardo, L. Conti, F. Kálmán, I. Szabó, N. Lago, A. Iolascon and S. Aime, Contrast Media Mol. Imaging, 2012, 7, 281–288 CrossRef CAS PubMed.
- W. Hou, T. B. Toh, L. N. Abdullah, T. Yvonne, K. J. Lee, I. Guenther and K. H. Chow, Nanomed.: Nanotechnol. Biol. Med., 2017, 13, 783–793 CrossRef CAS PubMed.
- R. Hachani, M. A. Birchall, M. W. Lowdell, G. Kasparis, L. D. Tung, B. B. Manshian, S. J. Soenen, W. Gsell, U. Himmelreich and C. A. Gharagouzloo, Sci. Rep., 2017, 7, 7850 CrossRef PubMed.
- R. Khatik, Z. Wang, F. Li, D. Zhi, S. Kiran, P. Dwivedi, R. Xu, G. Liang, B. Qiu and Q. Yang, Nanomed.: Nanotechnol. Biol. Med., 2019, 15, 264–273 CrossRef CAS PubMed.
- D. Aufhauser, E. Sadot, D. Murken, K. Eddinger, M. Hoteit, P. Abt, D. Goldberg, R. DeMatteo and M. Levine, Ann. Surg., 2018, 267, 922–928 CrossRef PubMed.
- A. Antaris, H. Chen, K. Cheng, Y. Sun, G. Hong, C. Qu, S. Diao, Z. Deng, X. Hu, B. Zhang, X. Zhang, O. Yaghi, Z. Alamparambil, X. Hong, Z. Cheng and H. Dai, Nat. Mater., 2016, 15, 235–242 CrossRef CAS PubMed.
- Q. Nguyen, E. Olson, T. Aguilera, T. Jiang, M. Scadeng, L. Ellies and R. Tsien, Proc. Natl. Acad. Sci. U. S. A., 2010, 107, 4317–4322 CrossRef CAS PubMed.
- K. Mori, H. Ohishi, M. Yamamoto, T. Yamamoto and Y. Nakao, Innov. Neurosurg., 2013, 1, 109–113 Search PubMed.
- T. Tanaka, M. Takatsuki, M. Hidaka, T. Hara, I. Muraoka, A. Soyama, T. Adachi, T. Kuroki and S. Eguchi, J Hepatobiliary Pancreat. Sci., 2014, 21, 199–204 CrossRef PubMed.
- K. Gotoh, T. Yamada, O. Ishikawa, H. Takahashi, H. Eguchi, M. Yano, H. Ohigashi, Y. Tomita, Y. Miyamoto and S. Imaoka, J. Surg. Oncol., 2009, 100, 75–79 CrossRef PubMed.
- Y. Dai, L. Zhu, Y. Zhang, S. Wang, K. Chen, T. Jiang, X. Xu and X. Geng, Hepatobiliary Pancreatic Dis. Int., 2019, 18, 266–272 CrossRef PubMed.
- Z. Hu, C. Chi, M. Liu, H. Guo, Z. Zhang, C. Zeng, J. Ye, J. Wang, J. Tian, W. Yang and W. Xu, Nanomed.: Nanotechnol. Biol. Med., 2017, 13, 1323–1331 CrossRef CAS PubMed.
- Q. Duan, M. Che, S. Hu, H. Zhao, Y. Li, X. Ma, W. Zhang, Y. Zhang and S. Sang, Anal. Bioanal. Chem., 2019, 411, 967–972 CrossRef CAS PubMed.
- S. Mukherjee and F. Maxfield, Annu. Rev. Cell Dev. Biol., 2004, 20, 839–866 CrossRef CAS PubMed.
- Z. Li, J. Cheng, P. Huang, W. Song, L. Nong, L. Huang and W. Lin, Anal. Chem., 2022, 94, 3386–3393 CrossRef CAS PubMed.
- C. Andreou, V. Neuschmelting, D. Tschaharganeh, C. Huang, A. Oseledchyk, P. Iacono, H. Karabeber, R. Colen, L. Mannelli, S. Lowe and M. Kircher, ACS Nano, 2016, 10, 5015–5026 CrossRef CAS PubMed.
- C. Sciallero, L. Balbi, G. Paradossi and A. Trucco, R. Soc. Open Sci., 2016, 3, 160063 CrossRef PubMed.
- P. Yang, F. Wang, X. Luo, Y. Zhang, J. Guo, W. Shi and C. Wang, ACS Appl. Mater. Interfaces, 2014, 6, 12581–12587 CrossRef CAS PubMed.
- H. Guo, Z. Jiang, S. Song, T. Dai, X. Wang, K. Sun, G. Zhou and H. Dou, J. Colloid Interface Sci., 2016, 482, 95–104 CrossRef CAS PubMed.
- F. Maghsoudinia, M. Tavakoli, R. Samani, S. Hejazi, T. Sobhani, F. Mehradnia and M. Mehrgardi, Talanta, 2021, 228, 122245 CrossRef CAS PubMed.
- Y. Jin, K. Wang and J. Tian, Bioconjugate Chem., 2018, 29, 1475–1484 CrossRef CAS PubMed.
- Y. Ren, S. He, L. Huttad, M. Chua, S. So, Q. Guo and Z. Cheng, Nanoscale, 2020, 12, 11510–11517 RSC.
- H. Zhang, L. Deng, H. Liu, S. Mai, Z. Cheng, G. Shi, H. Zeng and Z. Wu, Mater. Today Bio, 2022, 13, 100220 CrossRef CAS PubMed.
- P. Lei, H. Chen, C. Feng, X. Yuan, Z. Xiong, Y. Liu and W. Liao, ACS Nano, 2022, 16, 897–909 CrossRef CAS PubMed.
- M. Serper, T. Taddei, R. Mehta, K. D'Addeo, F. Dai, A. Aytaman, M. Baytarian, R. Fox, K. Hunt, D. Goldberg, A. Valderrama and D. Kaplan, Gastroenterology, 2017, 152, 1954–1964 CrossRef PubMed.
- S. Berhane, H. Toyoda, T. Tada, T. Kumada, C. Kagebayashi, S. Satomura, N. Schweitzer, A. Vogel, M. Manns, J. Benckert, T. Berg, M. Ebker, J. Best, A. Dechêne, G. Gerken, J. Schlaak, A. Weinmann, M. Wörns, P. Galle, W. Yeo, F. Mo, S. Chan, H. Reeves, T. Cox and P. Johnson, Clin. Gastroenterol. Hepatol. Clin. Gastroenterol. Hepatol., 2016, 14, 875–886 CrossRef CAS PubMed.
- M. Reig, A. Forner, J. Rimola, J. Ferrer-Fàbrega, M. Burrel, Á. Garcia-Criado, R. Kelley, P. Galle, V. Mazzaferro, R. Salem, B. Sangro, A. Singal, A. Vogel, J. Fuster, C. Ayuso and J. Bruix, J. Hepatol., 2022, 76, 681–693 CrossRef PubMed.
- Z. Luo, L. Zhang, R. Zeng, L. Su and D. Tang, Anal. Chem., 2018, 90, 9568–9575 CrossRef CAS PubMed.
- D. Sun, H. Li, M. Li, C. Li, L. Qian and B. Yang, Biosens. Bioelectron., 2019, 132, 68–75 CrossRef CAS PubMed.
- X. Ran, R. Yuan, Y. Chai, C. Hong and X. Qian, Colloids Surf., B, 2010, 79, 421–426 CrossRef CAS PubMed.
- Q. Zhu, R. Yuan, Y. Chai, J. Han, Y. Li and N. Liao, Analyst, 2013, 138, 620–626 RSC.
- J. Rashidiani, M. Kamali, H. Sedighian, M. Akbariqomi, M. Mansouri and H. Kooshki, Biosens. Bioelectron., 2018, 102, 226–233 CrossRef PubMed.
- Y. Cao, R. Yuan, Y. Chai, L. Mao, H. Niu, H. Liu and Y. Zhuo, Biosens. Bioelectron., 2012, 31, 305–309 CrossRef CAS PubMed.
- G. Jie, J. Zhang, D. Wang, C. Cheng, H. Chen and J. Zhu, Anal. Chem., 2008, 80, 4033–4039 CrossRef CAS PubMed.
- Y. Ding, J. Liu, H. Wang, G. Shen and R. Yu, Biomaterials, 2007, 28, 2147–2154 CrossRef CAS PubMed.
- H. Zhou, N. Gan, T. Li, Y. Cao, S. Zeng, L. Zheng and Z. Guo, Anal. Chim. Acta, 2012, 746, 107–113 CrossRef CAS PubMed.
- H. Xu, D. Wang, S. He, J. Li, B. Feng, P. Ma, P. Xu, S. Gao, S. Zhang, Q. Liu, J. Lu, S. Song and C. Fan, Biosens. Bioelectron., 2013, 50, 251–255 CrossRef CAS PubMed.
- S. Yuan, R. Yuan, Y. Chai, L. Mao, X. Yang, Y. Yuan and H. Niu, Talanta, 2010, 82, 1468–1471 CrossRef CAS PubMed.
- H. Su, R. Yuan, Y. Chai, L. Mao and Y. Zhuo, Biosens. Bioelectron., 2011, 26, 4601–4604 CrossRef CAS PubMed.
- W. Li, X. Jiang, J. Xue, Z. Zhou and J. Zhou, Biosens. Bioelectron., 2015, 68, 468–474 CrossRef CAS PubMed.
- Y. Wei, Y. Li, N. Li, Y. Zhang, T. Yan, H. Ma and Q. Wei, Biosens. Bioelectron., 2016, 79, 482–487 CrossRef CAS PubMed.
- Y. Wu, W. Guo, W. Peng, Q. Zhao, J. Piao, B. Zhang, X. Wu, H. Wang, X. Gong and J. Chang, ACS Appl. Mater. Interfaces, 2017, 9, 9369–9377 CrossRef CAS PubMed.
- L. Yu, Q. Zhang, Q. Kang, B. Zhang, D. Shen and G. Zou, Anal. Chem., 2020, 92, 7581–7587 CrossRef CAS PubMed.
- N. Jo and Y. Shin, Sci. Rep., 2020, 10, 1024 CrossRef CAS PubMed.
- N. Jo, K. Lee and Y. Shin, Biosens. Bioelectron., 2016, 81, 324–333 CrossRef CAS PubMed.
- X. Zhong, M. Zhang, L. Guo, Y. Xie, R. Luo, W. Chen, F. Cheng and L. Wang, Biosens. Bioelectron., 2021, 189, 113389 CrossRef CAS PubMed.
- X. Wang, J. Ji, P. Yang, X. Li, Y. Pang and P. Lu, Talanta, 2022, 243, 123328 CrossRef CAS PubMed.
- J. Guo, S. Li, J. Wang and J. Wang, Biosens. Bioelectron., 2022, 198, 113820 CrossRef CAS PubMed.
- H. Yu, X. Tan, S. Sun, L. Zhang, C. Gao and S. Ge, Biosens. Bioelectron., 2021, 185, 113250 CrossRef CAS PubMed.
- B. Zhao, J. Yan, D. Wang, Z. Ge, S. He, D. He, S. Song and C. Fan, Small, 2013, 9, 2595–2601 CrossRef CAS PubMed.
- C. Wang, F. Hou and Y. Ma, Biosens. Bioelectron., 2015, 68, 156–162 CrossRef CAS PubMed.
- L. Zhou, J. Zhou, Z. Feng, F. Wang, S. Xie and S. Bu, Analyst, 2016, 141, 2534–2541 RSC.
- Q. Wu, F. Zhang, H. Li, Z. Li, Q. Kang and D. Shen, Analyst, 2018, 143, 5030–5037 RSC.
- L. Chen, X. Wang, Q. Zhang, Z. Li, Q. Kang and D. Shen, Analyst, 2020, 145, 2389–2397 RSC.
- X. Li, X. Pan, J. Lu, Y. Zhou and J. Gong, Biosens. Bioelectron., 2020, 158, 112158 CrossRef CAS PubMed.
- Y. Wu, H. Liu and H. Ding, J. Hepatocell. Carcinoma, 2016, 3, 63–67 CrossRef PubMed.
- W. Mu, D. Jiang, S. Mu, S. Liang, Y. Liu and N. Zhang, ACS Appl. Mater. Interfaces, 2019, 11, 23591–23604 CrossRef CAS PubMed.
- S. Zhao, M. Long, X. Zhang, S. Lei, W. Dou, J. Hu, X. Du and L. Liu, Ann. Transl. Med., 2020, 8, 536 CrossRef CAS PubMed.
- S. Yu, Z. Li, J. Li, S. Zhao, S. Wu, H. Liu, X. Bi, D. Li, J. Dong, S. Duan and B. Hammock, Sens. Actuators, B, 2021, 336 Search PubMed.
- Y. Huang, H. Li, T. Gao, X. Liu and G. Li, Analyst, 2014, 139, 3744–3747 RSC.
- T. Tayoun, V. Faugeroux, M. Oulhen, A. Aberlenc, P. Pawlikowska and F. Farace, Cells, 2019, 8, 1145 CrossRef CAS PubMed.
- L. Qi, B. Xiang, F. Wu, J. Ye, J. Zhong, Y. Wang, Y. Chen, Z. Chen, L. Ma, J. Chen, W. Gong, Z. Han, Y. Lu, J. Shang and L. Li, Cancer Res., 2018, 78, 4731–4744 CrossRef CAS PubMed.
- R. Kelley, M. Magbanua, T. Butler, E. Collisson, J. Hwang, N. Sidiropoulos, K. Evason, R. McWhirter, B. Hameed, E. Wayne, F. Yao, A. Venook and J. Park, BMC Cancer, 2015, 15, 206 CrossRef PubMed.
- W. Xia, H. Li, Y. Li, M. Li, J. Fan, W. Sun, N. Li, R. Li, K. Shao and X. Peng, Nano Lett., 2021, 21, 634–641 CrossRef CAS PubMed.
- W. Xie, T. Yin, Y. Chen, D. Zhu, M. Zan, B. Chen, L. Ji, L. Chen, S. Guo, H. Huang, X. Zhao, Y. Wang, Y. Wu and W. Liu, Nanoscale, 2019, 11, 8293–8303 RSC.
- C. Wu, P. Li, N. Fan, J. Han, W. Zhang, W. Zhang and B. Tang, ACS Appl. Mater. Interfaces, 2019, 11, 44999–45006 CrossRef CAS PubMed.
- J. Llovet, A. Villanueva, J. Marrero, M. Schwartz, T. Meyer, P. Galle, R. Lencioni, T. Greten, M. Kudo, S. Mandrekar, A. Zhu, R. Finn and L. Roberts, Hepatology, 2021, 158–191, DOI:10.1002/hep.31327.
- J. Llovet, C. Brú and J. Bruix, Semin. Liver Dis., 1999, 19, 329–338 CrossRef CAS PubMed.
- G. D'Amico, A. Morabito, M. D'Amico, L. Pasta, G. Malizia, P. Rebora and M. Valsecchi, J. Hepatol., 2018, 68, 563–576 CrossRef PubMed.
- J. Llovet, J. Bustamante, A. Castells, R. Vilana, M. C. Ayuso, M. Sala, C. Brú, J. Rodés and J. Bruix, Hepatology, 1999, 29, 62–67 CrossRef CAS PubMed.
- O. Miltiadous, D. Sia, Y. Hoshida, M. Fiel, A. Harrington, S. Thung, P. Tan, H. Dong, K. Revill, C. Chang, S. Roayaie, T. Byrne, V. Mazzaferro, J. Rakela, S. Florman, M. Schwartz and J. Llovet, J. Hepatol., 2015, 63, 1368–1377 CrossRef PubMed.
- J. Llovet, T. De Baere, L. Kulik, P. Haber, T. Greten, T. Meyer and R. Lencioni, Nat. Rev. Gastroenterol. Hepatol., 2021, 18, 293–313 CrossRef CAS PubMed.
- Y. Shao, B. Arjun, H. Leo and K. Chua, J. Therm. Biol., 2017, 66, 101–113 CrossRef CAS PubMed.
- A. Ashokan, V. Somasundaram, G. Gowd, I. Anna, G. Malarvizhi, B. Sridharan, R. Jobanputra, R. Peethambaran, A. Unni, S. Nair and M. Koyakutty, Sci. Rep., 2017, 7, 14481 CrossRef PubMed.
- L. Liu, Z. Xiao, Y. Xiao, Z. Wang, F. Li, M. Li and X. Peng, Oncol. Lett., 2014, 7, 1485–1492 CrossRef CAS PubMed.
- H. Hu, G. Chen, W. Yuan, J. Wang and B. Zhai, Int. J. Hyperthermia, 2018, 34, 1351–1358 CrossRef PubMed.
- Q. Zhou, N. Gong, D. Zhang, J. Li, X. Han, J. Dou, J. Huang, K. Zhu, P. Liang, X. Liang and J. Yu, ACS Nano, 2021, 15, 2920–2932 CrossRef CAS PubMed.
- J. Li and K. Pu, Acc. Chem. Res., 2020, 53, 752–762 CrossRef CAS PubMed.
- X. Chen, Q. Zhang, J. Li, M. Yang, N. Zhao and F. Xu, ACS Nano, 2018, 12, 5646–5656 CrossRef CAS PubMed.
- L. Mocan, C. Matea, F. Tabaran, O. Mosteanu, T. Pop, C. Puia, L. Agoston-Coldea, G. Zaharie, T. Mocan, A. Buzoianu and C. Iancu, Biomaterials, 2017, 119, 33–42 CrossRef CAS PubMed.
- C. Xu, D. Yang, L. Mei, Q. Li, H. Zhu and T. Wang, ACS Appl. Mater. Interfaces, 2013, 5, 12911–12920 CrossRef CAS PubMed.
- C. Wen, R. Cheng, T. Gong, Y. Huang, D. Li, X. Zhao, B. Yu, D. Su, Z. Song and W. Liang, Colloids Surf., B, 2021, 199, 111510 CrossRef CAS PubMed.
- B. Ji, H. Cai, Y. Yang, F. Peng, M. Song, K. Sun, F. Yan and Y. Liu, Acta Biomater., 2020, 111, 363–372 CrossRef CAS PubMed.
- A. Oniszczuk, K. Wojtunik-Kulesza, T. Oniszczuk and K. Kasprzak, Biomed. Pharmacother., 2016, 83, 912–929 CrossRef CAS PubMed.
- D. Hu, L. Chen, Y. Qu, J. Peng, B. Chu, K. Shi, Y. Hao, L. Zhong, M. Wang and Z. Qian, Theranostics, 2018, 8, 1558–1574 CrossRef CAS PubMed.
- Y. Han, Y. An, G. Jia, X. Wang, C. He, Y. Ding and Q. Tang, Nanoscale, 2018, 10, 6511–6523 RSC.
- J. Kaneko, T. Kokudo, Y. Inagaki and K. Hasegawa, Transl. Gastroenterol. Hepatol., 2018, 3, 78 CrossRef PubMed.
- M. Lan, S. Zhao, W. Liu, C. Lee, W. Zhang and P. Wang, Adv. Healthcare Mater., 2019, 8, e1900132 CrossRef PubMed.
- J. Kim, C. Tung and Y. Choi, Chem. Commun., 2014, 50, 10600–10603 RSC.
- H. Kang, S. Rho, W. Stiles, S. Hu, Y. Baek, D. Hwang, S. Kashiwagi, M. Kim and H. Choi, Adv. Healthcare Mater., 2020, 9, e1901223 CrossRef PubMed.
- D. Abdel Fadeel, G. Al-Toukhy, A. Elsharif, S. Al-Jameel, H. Mohamed and T. Youssef, Photodiagnosis Photodyn Ther., 2018, 23, 25–31 CrossRef CAS PubMed.
- J. Xu, Q. Zheng, X. Cheng, S. Hu, C. Zhang, X. Zhou, P. Sun, W. Wang, Z. Su, T. Zou, Z. Song, Y. Xia, X. Yi and Y. Gao, J. Nanobiotechnol., 2021, 19, 355 CrossRef CAS PubMed.
- J. Kwon, I. Song, M. Kim, B. Lee, J. Kim, I. Yoon, Y. Shim, N. Kim, J. Han and J. Youm, Integr. Med. Res., 2013, 2, 106–111 CrossRef PubMed.
- Z. Feng, J. Guo, X. Liu, H. Song, C. Zhang, P. Huang, A. Dong, D. Kong and W. Wang, Biomaterials, 2020, 255, 120210 CrossRef CAS PubMed.
- A. Facciorusso, World J. Gastroenterol., 2018, 24, 161–169 CrossRef CAS PubMed.
- F. Piscaglia and S. Ogasawara, Liver Cancer, 2018, 7, 104–119 CrossRef CAS PubMed.
- S. Daher, M. Massarwa, A. Benson and T. Khoury, J. Clin. Transl. Hepatol., 2018, 6, 69–78 Search PubMed.
- D. Kong, T. Jiang, J. Liu, X. Jiang, B. Liu, C. Lou, B. Zhao, S. Carroll and G. Feng, Drug. Deliv., 2020, 27, 1729–1740 CrossRef CAS PubMed.
- L. Wei-Ze, H. Wen-Xia, Z. Ning, H. Shu-Miao, L. Fei, F. Li-Na, Z. Zhan-Rui, Z. Xi-Feng and Y. Li-Bin, Eur. J. Pharm. Sci., 2020, 153, 105496 CrossRef PubMed.
- Y. Wang, W. Shang, H. Zhong, T. Luo, M. Niu, K. Xu and J. Tian, ACS Nano, 2020, 14, 14907–14918 CrossRef PubMed.
- A. Dev, A. Sood, S. Choudhury and S. Karmakar, Mater. Sci. Eng., C, 2020, 107, 110315 CrossRef CAS PubMed.
- D. Su, Bioengineered, 2021, 12, 11124–11135 CrossRef CAS PubMed.
- D. Wang, Q. Wu, R. Guo, C. Lu, M. Niu and W. Rao, Nanoscale, 2021, 13, 8817–8836 RSC.
- H. Ahmed, W. Khalil and A. Hamza, Toxicol. Mech. Methods, 2014, 24, 593–602 CrossRef CAS PubMed.
- B. Zhu, Y. Li, Z. Lin, M. Zhao, T. Xu, C. Wang and N. Deng, Nanoscale Res. Lett., 2016, 11, 198 CrossRef PubMed.
- K. Kitchin, J. Richards, B. Robinette, K. Wallace, N. Coates, B. Castellon, E. Grulke, J. Kou and R. Varma, J. Nanosci. Nanotechnol., 2020, 20, 5833–5858 CrossRef CAS PubMed.
- L. Liu, H. Dai, Y. Wu, B. Li, J. Yi, C. Xu and X. Wu, Int. J. Nanomed., 2019, 14, 3491–3502 CrossRef CAS PubMed.
- Y. Niu, E. Tang and Q. Zhang, Toxicol. Res., 2019, 8, 1042–1049 CrossRef CAS PubMed.
- M. Younas, M. Rizwan, M. Zubair, A. Inam and S. Ali, Ecotoxicol. Environ. Saf., 2021, 223, 112575 CrossRef CAS PubMed.
- B. Mugaka, S. Zhang, R. Li, Y. Ma, B. Wang, J. Hong, Y. Hu, Y. Ding and X. Xia, ACS Appl. Mater. Interfaces, 2021, 13, 11195–11204 CrossRef CAS PubMed.
- A. Usmani, A. Mishra, M. Arshad and A. Jafri, Artif. Cells, Nanomed., Biotechnol., 2019, 47, 933–944 CrossRef CAS PubMed.
- W. Fan, Q. Wei, J. Xiang, Y. Tang, Q. Zhou, Y. Geng, Y. Liu, R. Sun, L. Xu, G. Wang, Y. Piao, S. Shao, Z. Zhou, J. Tang, T. Xie, Z. Li and Y. Shen, Adv. Mater., 2022, 34, e2109189 CrossRef PubMed.
- S. Bhat, D. Mukherjee, P. Sukharamwala, R. Dehuri, A. Murali and B. Teja, Drug Deliv. Transl. Res., 2021, 11, 2252–2269 CrossRef CAS PubMed.
- S. Wang, Y. Ma, X. Chen, Y. Zhao and X. Mou, Front. Pharmacol., 2019, 10, 69 CrossRef PubMed.
- Z. Li, F. Wang, Y. Li, X. Wang, Q. Lu, D. Wang, C. Qi, C. Li, Z. Li, B. Lian, G. Tian, Z. Gao, B. Zhang and J. Wu, Biomaterials, 2021, 276, 121003 CrossRef CAS PubMed.
- Q. Chen, X. Li, Y. Xie, W. Hu, Z. Cheng, H. Zhong and H. Zhu, Int. J. Biol. Macromol., 2021, 187, 214–222 CrossRef CAS PubMed.
- Z. Ding, D. Wang, W. Shi, X. Yang, S. Duan, F. Mo, X. Hou, A. Liu and X. Lu, Int. J. Nanomed., 2020, 15, 8383–8400 CrossRef CAS PubMed.
- Y. Lu, C. Hou, J. Ren, K. Yang, Y. Chang, Y. Pei, H. Dong and Z. Pei, Int. J. Nanomed., 2019, 14, 3525–3532 CrossRef CAS PubMed.
- C. Cheng, C. Li, X. Zhu, W. Han, J. Li and Y. Lv, J. Biomater. Appl., 2019, 33, 1373–1381 CrossRef CAS PubMed.
- W. Al-Shakarchi, A. Alsuraifi, A. Curtis and C. Hoskins, Pharmaceutics, 2018, 10 Search PubMed.
- S. AlQahtani, G. Harisa, M. Badran, K. AlGhamdi, A. Kumar, M. Salem-Bekhit, S. Ahmad and F. Alanazi, Artif. Cells, Nanomed., Biotechnol., 2019, 47, 989–996 CrossRef CAS PubMed.
- N. Aboeita, S. Fahmy, M. El-Sayed, H. Azzazy and T. Shoeib, Pharmaceutics, 2022, 14 Search PubMed.
- M. Khan, P. Zhao, A. Khan, F. Raza, S. Raza, M. Sarfraz, Y. Chen, M. Li, T. Yang, X. Ma and G. Xiang, Int. J. Nanomed., 2019, 14, 3753–3771 CrossRef CAS PubMed.
- Q. Wu, X. Chen, P. Wang, Q. Wu, X. Qi, X. Han, L. Chen, X. Meng and K. Xu, ACS Appl. Mater. Interfaces, 2020, 12, 8016–8029 CrossRef CAS PubMed.
- Y. Sun, W. Ma, Y. Yang, M. He, A. Li, L. Bai, B. Yu and Z. Yu, Asian J. Pharm. Sci., 2019, 14, 581–594 CrossRef PubMed.
- J. Llovet, R. Montal, D. Sia and R. Finn, Nat. Rev. Clin. Oncol., 2018, 15, 599–616 CrossRef PubMed.
- J. Kaur, S. Tuler and C. Dasanu, J. Oncol. Pharm. Pract., 2022, 28, 475–478 CrossRef PubMed.
- L. Ding, P. Zhang, X. Huang, K. Yang, X. Liu and Z. Yu, Front. Pharmacol., 2021, 12, 809125 CrossRef CAS PubMed.
- B. Wang, L. Sun, M. Wen, Y. Tan, W. Almalki, H. Katouah, I. Kazmi, O. Afzal, A. Altamimi, F. Al-Abbasi, M. Alrobaian, K. Alharbi, S. Alenezi, A. Alghaith, S. Beg and M. Rahman, Saudi Pharm. J., 2021, 29, 843–856 CrossRef CAS PubMed.
- H. Tang, C. Li, Y. Zhang, H. Zheng, Y. Cheng, J. Zhu, X. Chen, Z. Zhu, J. Piao and F. Li, Theranostics, 2020, 10, 9865–9887 CrossRef CAS PubMed.
- L. Tunki, H. Kulhari, L. Vadithe, M. Kuncha, S. Bhargava, D. Pooja and R. Sistla, Eur. J. Pharm. Sci., 2019, 137, 104978 CrossRef CAS PubMed.
- D. Ling, H. Xia, W. Park, M. Hackett, C. Song, K. Na, K. Hui and T. Hyeon, ACS Nano, 2014, 8, 8027–8039 CrossRef CAS PubMed.
- H. Abbas, Y. El-Feky, M. Al-Sawahli, N. El-Deeb, H. El-Nassan and M. Zewail, Drug. Deliv., 2022, 29, 714–727 CrossRef CAS PubMed.
- S. Kianamiri, A. Dinari, M. Sadeghizadeh, M. Rezaei, B. Daraei, N. Bahsoun and A. Nomani, Mol. Pharmaceutics, 2020, 17, 4483–4498 CrossRef CAS PubMed.
- M. Rahman, S. Al-Ghamdi, K. Alharbi, S. Beg, K. Sharma, F. Anwar, F. Al-Abbasi and V. Kumar, Drug. Deliv., 2019, 26, 782–793 CrossRef CAS PubMed.
- M. Zhou, Y. Yi, L. Liu, Y. Lin, J. Li, J. Ruan and Z. Zhong, J. Cancer, 2019, 10, 5820–5831 CrossRef CAS PubMed.
- I. Pinzaru, C. Sarau, D. Coricovac, I. Marcovici, C. Utescu, S. Tofan, R. Popovici, H. Manea, I. Pavel, C. Soica and C. Dehelean, Nanomaterials, 2021, 11, 152 CrossRef CAS PubMed.
- T. Li, G. Kang, T. Wang and H. Huang, Oncol. Lett., 2018, 16, 687–702 Search PubMed.
- S. Senapati, T. Sarkar, P. Das and P. Maiti, Bioconjugate Chem., 2019, 30, 2544–2554 CrossRef CAS PubMed.
- X. Zhou, Y. Miao, Y. Wang, S. He, L. Guo, J. Mao, M. Chen, Y. Yang, X. Zhang and Y. Gan, J. Extracell. Vesicles, 2022, 11, e12198 CAS.
- Y. Qu, F. Sun, F. He, C. Yu, J. Lv, Q. Zhang, D. Liang, C. Yu, J. Wang, X. Zhang, A. Xu and J. Wu, Eur. J. Pharm. Sci., 2019, 139, 105036 CrossRef CAS PubMed.
- G. Wang, X. Gao, G. Gu, Z. Shao, M. Li, P. Wang, J. Yang, X. Cai and Y. Li, Int. J. Nanomed., 2017, 12, 3591–3603 CrossRef CAS PubMed.
- S. Mahati, X. Fu, X. Ma, H. Zhang and L. Xiao, Front. Mol. Biosci., 2021, 8, 738219 CrossRef CAS PubMed.
- A. Varshney, J. Panda, A. Singh, N. Yadav, C. Bihari, S. Biswas, S. Sarin and V. Chauhan, Hepatology, 2018, 67, 1392–1407 CrossRef CAS PubMed.
- Y. Mo, L. He, Z. Lai, Z. Wan, Q. Chen, S. Pan, L. Li, D. Li, J. Huang, F. Xue and S. Che, Artif. Cells, Nanomed., Biotechnol., 2019, 47, 2830–2837 CrossRef CAS PubMed.
- D. Singh and M. Singh, Pharmaceutics, 2021, 13 Search PubMed.
- R. Finn, S. Qin, M. Ikeda, P. Galle, M. Ducreux, T. Kim, M. Kudo, V. Breder, P. Merle, A. Kaseb, D. Li, W. Verret, D. Xu, S. Hernandez, J. Liu, C. Huang, S. Mulla, Y. Wang, H. Lim, A. Zhu and A. Cheng, N. Engl. J. Med., 2020, 382, 1894–1905 CrossRef CAS PubMed.
- A. El-Khoueiry, B. Sangro, T. Yau, T. Crocenzi, M. Kudo, C. Hsu, T. Kim, S. Choo, J. Trojan, T. Welling, T. Meyer, Y. Kang, W. Yeo, A. Chopra, J. Anderson, C. Dela Cruz, L. Lang, J. Neely, H. Tang, H. Dastani and I. Melero, Lancet, 2017, 389, 2492–2502 CrossRef CAS.
- R. Vaddepally, P. Kharel, R. Pandey, R. Garje and A. Chandra, Cancers, 2020, 12 Search PubMed.
- N. Qiang, L. Wei, Y. Tao, W. Jin, Y. Bin and Z. DingHua, Biomed. Mater., 2022, 17, 025015 CrossRef PubMed.
- C. An, W. Hu, J. Gao, B. Ju, P. Obeidy, Y. Zhao, X. Tu, W. Fang, L. Ju and W. Chen, Nano Lett., 2020, 20, 5133–5140 CrossRef CAS PubMed.
- O. Helm, J. Held-Feindt, E. Grage-Griebenow, N. Reiling, H. Ungefroren, I. Vogel, U. Krüger, T. Becker, M. Ebsen, C. Röcken, D. Kabelitz, H. Schäfer and S. Sebens, Int. J. Cancer, 2014, 135, 843–861 CrossRef CAS PubMed.
- S. Bai, Z. Lu, Y. Jiang, X. Shi, D. Xu, Y. Shi, G. Lin, C. Liu, Y. Zhang and G. Liu, ACS Nano, 2022, 16, 997–1012 CrossRef CAS PubMed.
- T. Wang, J. Zhang, T. Hou, X. Yin and N. Zhang, Nanoscale, 2019, 11, 13934–13946 RSC.
- Q. Zhao, Y. Wang, B. Zhao, H. Chen, Z. Cai, Y. Zheng, Y. Zeng, D. Zhang and X. Liu, Nano Lett., 2022, 22, 2048–2058 CrossRef PubMed.
Footnote |
† These authors contributed equally to this work. |
|
This journal is © The Royal Society of Chemistry 2022 |