DOI:
10.1039/D1SC02352G
(Edge Article)
Chem. Sci., 2021,
12, 9823-9830
[2 + 2 + 1] Cycloaddition of N-tosylhydrazones, tert-butyl nitrite and alkenes: a general and practical access to isoxazolines†
Received
28th April 2021
, Accepted 19th June 2021
First published on 22nd June 2021
Abstract
N-Tosylhydrazones have proven to be versatile synthons over the past several decades. However, to our knowledge, the construction of isoxazolines based on N-tosylhydrazones has not been examined. Herein, we report the first demonstrations of [2 + 2 + 1] cycloaddition reactions that allow the facile synthesis of isoxazolines, employing N-tosylhydrazones, tert-butyl nitrite (TBN) and alkenes as reactants. This process represents a new type of cycloaddition reaction with a distinct mechanism that does not involve the participation of nitrile oxides. This approach is both general and practical and exhibits a wide substrate scope, nearly universal functional group compatibility, tolerance of moisture and air, the potential for functionalization of complex bioactive molecules and is readily scaled up. Both control experiments and theoretical calculations indicate that this transformation proceeds via the in situ generation of a nitronate from the coupling of N-tosylhydrazone and TBN, followed by cycloaddition with an alkene and subsequent elimination of a tert-butyloxy group to give the desired isoxazoline.
Introduction
The electrophilicity of the carbonyl carbon and the acidity of the α-hydrogen in ketones and aldehydes allow these compounds to play important roles in synthetic chemistry.1 Over the past several decades, N-tosylhydrazones, which are readily obtained from ketones or aldehydes, have rapidly become a new type of versatile synthon for transition metal-catalysed or metal-free reactions.2 Since the pioneering work by Barluenga,3m–3p a wide range of transformations using N-tosylhydrazones has been established, including coupling,3 insertion,4 cyclization,2e,5 olefination6 and alkynylation7 reactions, and these are now standard methods in organic synthesis. Although this unconventional manipulation of carbonyl compounds provides powerful and indispensable protocols for the facile construction of diverse molecular frameworks, this rapidly evolving area is still far from mature and there is ample opportunity for further development. As an example, the use of N-tosylhydrazones in the synthesis of isoxazolines has not been explored to date.
Isoxazolines8 are important structural motifs that are ubiquitous in bioactive molecules, pharmaceuticals and chiral ligands,9 and are also versatile intermediates10 in organic synthesis. Thus, the development of efficient approaches to the construction of these valuable molecules has received increasing attention. State-of-the-art synthetic methods have shown that the formation of isoxazolines proceeds primarily via the [3 + 2] cycloaddition of olefins with nitrile oxides generated in situ. However, the formation of furoxan (a nitrile oxide dimer) is an undesired side reaction that is difficult to avoid during such syntheses. The nitrile oxides that are employed in these procedures are typically derived from aldoximes11 or nitro compounds,12 although the use of various additives (including oxidants, bases, Grignard reagents and dehydrating agents) can cause environmental, cost and safety issues, especially when employed on an industrial scale. Consequently, alternative synthetic methods have been examined, such as the intramolecular cyclization of β,γ-unsaturated oximes,13 the iminoxyl radical promoted formation of isoxazolines14 and others.15 Despite the significant achievements to date, the methods that have been reported often have limited substrate scopes, especially with regard to the range of possible functional groups. Thus, the development of new and versatile techniques for the synthesis of isoxazolines remains challenging.
Recently, our group demonstrated the synthesis of isoxazolines from diazo compounds bearing electron withdrawing groups.15a,15d Unfortunately, these diazo compounds have to be pre-synthesized using a process comprising several time-consuming steps. More importantly, diazo compounds without electron withdrawing substituents are usually unstable and difficult to handle, which limits their synthetic value when constructing complex structures. Consequently, the present work employed N-tosylhydrazones as more stable surrogates for unstable diazo compounds. These compounds were generated in situ from readily obtainable commercial aldehydes and TsNHNH2, thus providing a new platform for the construction of isoxazolines. To illustrate the feasibility of this new approach, we also propose a plausible mechanism herein, as shown in Scheme 1. In this mechanism, the condensation of aldehydes with TsNHNH2 generates N-tosylhydrazones I, which are easily transformed to the diazo compounds II by adding a suitable base. The loss of a diatomic nitrogen molecule from the diazo in the presence of a transition metal catalyst affords the carbene III. Subsequently, III reacts with tert-butyl nitrite (TBN) to generate the nitronate IV, which then undergoes a [3 + 2] cycloaddition with an alkene to afford the cycloadduct V. Finally, the elimination of tBuOH delivers the desired isoxazoline. However, it is very challenging to realize the [2 + 2 + 1] cycloaddition step in this process because the mechanism involves several highly reactive intermediates that tend to undergo undesired side reactions. As an example, the cyclopropanation reaction2e,5h between a carbene and an alkene is a well-known process that can potentially interrupt the formation of the unstable nitronate. Typically, an electron withdrawing group must be attached to the α-carbon to both form and stabilize IV.16 Thus, a lack of suitable electron-withdrawing groups may not permit the successful in situ generation of IV in this transformation. Moreover, this nitronate is prone to decomposition instead of the desired cycloaddition with an alkene to deliver the cycloadduct V.
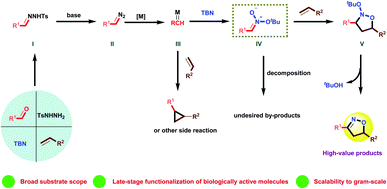 |
| Scheme 1 Reaction design: [2 + 2 + 1] cycloaddition to construct isoxazoline based upon nitronates. | |
Herein, we report the first-ever construction of isoxazolines from N-tosylhydrazones. The most important features of this methodology are the ability to use readily-available aldehydes and alkenes, a wide substrate scope, significant functional group compatibility, the potential for the late-stage modification of bioactive molecules and scalability to the 20 mmol level. This new approach to isoxazolines formation proceeds through a pathway presumably involving unstable nitronates, in sharp contrast to the classical [3 + 2] cycloaddition of nitrile oxides with alkenes.
Results and discussion
In an initial study, we examined the reaction of the diazo compound 1aa with TBN and ethyl acrylate, 2a, catalysed by CuCl2. The desired isoxazoline 3aa was obtained in 10% yield (Scheme 2). Although the yield was very low, the above results demonstrated the feasibility of our design. The efficiency of this isoxazoline formation reaction was enhanced by developing a one-pot, two-step procedure using commercially available aldehydes as starting materials. After exploring a wide range of reaction parameters, we determined that the use of CuCl2 (10 mol%) and N,N,N,N,-tetramethylethylenediamine (TMEDA, 1.5 equiv.) gave 3aa in an 88% yield (Table 1, entry 1). It is important to note that this [2 + 2 + 1] cycloaddition reaction was able to tolerate both air and moisture. In addition, 3aa could still be obtained in a good yield even in the absence of CuCl2 (entry 2). In sharp contrast, a control experiment showed that TMEDA was indispensable to achieving optimal reaction efficiency (entry 3). Similar results were also observed when the reaction was performed using a variety of nitrites or different copper catalysts (entries 10–16). Other bases, including representative inorganic and organic bases, were less effective than TMEDA (entries 17–24), although the atmosphere under which the reaction was performed had little effect on efficiency (entries 25 and 26). When the reaction was performed at 80 °C (entry 27), the yield was nearly identical to that obtained at 65 °C, while very little 3aa was produced at room temperature (entry 28).
 |
| Scheme 2 Initial attempt for the synthesis of isoxazoline. | |
Table 1 Synthesis of isoxazoline: effect of reaction parametersa
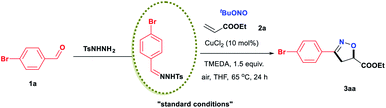
|
Entry |
Variation from the “standard conditions” catalyst |
Yieldb (%) |
Reaction conditions: N-tosylhydrazones (0.65 mmol, generated in situ from 4-bromobenzaldehyde 1a and TsNHNH2), ethyl acrylate 2a (0.50 mmol), CuCl2 (10 mol%), TMEDA (0.75 mmol), and TBN (2.0 mmol) in 4.0 mL THF at 65 °C for 24 h.
Isolated yields of the average of two experiments.
|
1 |
None |
88 |
2 |
No CuCl2 |
61 |
3 |
No TMEDA |
<1 |
4 |
Acetone instead of THF |
84 |
5 |
MeCN instead of THF |
54 |
6 |
EA instead of THF |
31 |
7 |
Toluene instead of THF |
53 |
8 |
CHCl3 instead of THF |
81 |
9 |
DMF instead of THF |
72 |
10 |
i
PrONO instead of tBuONO |
79 |
11 |
n
BuONO instead of tBuONO |
68 |
12 |
i
BuONO instead of tBuONO |
76 |
13 |
CuCl instead of CuCl2 |
77 |
14 |
CuBr instead of CuCl2 |
83 |
15 |
CuI instead of CuCl2 |
56 |
16 |
Cu(OAc)2 instead of CuCl2 |
58 |
17 |
Cs2CO3 instead of TMEDA |
<1 |
18 |
DABCO instead of TMEDA |
68 |
19 |
t
BuOLi instead of TMEDA |
<1 |
20 |
K2CO3 instead of TMEDA |
<1 |
21 |
t
BuOK instead of TMEDA |
<1 |
22 |
NaOH instead of TMEDA |
<1 |
23 |
K3PO4 instead of TMEDA |
<1 |
24 |
Na2CO3 instead of TMEDA |
16 |
25 |
N2 instead of air |
76 |
26 |
O2 instead of air |
84 |
27 |
At 80 °C |
87 |
28 |
At room temperature |
Trace |
With the optimal catalyst and reaction conditions identified, we next assessed the scope of aldehyde that could be used in this process (Table 2). Numerous mono-substituted benzaldehydes containing both electron-donating and electron-withdrawing groups were all found to be viable substrates, delivering the desired products in moderate to excellent yields. The exact structure of 3ab was confirmed by single crystal X-ray diffraction (for details, see the ESI†). Of note, the position of the substituent (para-, meta- or ortho-) had no significant effect on the [2 + 2 + 1] cycloaddition reaction. This method also showed broad compatibility with common functional groups, including alkyl (3ac, 3at), halide (3af, 3ag, 3ar, 3as, 3ax, 3ay, 3ha), ether (3ad, 3au, 3aw, 3ba), amide (3an), cyano (3ak), nitro (3al, 3ap), sulfonyl (3aj) and trifluoromethyl (3ah, 3aq) groups. Remarkably, aldehydes with more reactive functional groups such as hydroxyl (3ao, 3av, 3aw, 3ay), thioether (3ae), amine (3ai) and carboxyl (3am) groups, which are typically challenging to use as substrates in known methods, reacted smoothly with TBN and 2a to provide the corresponding products in satisfactory yields. Naphthyl-substituted aldehydes were also readily transformed into the desired products in high yields (3az, 3da). In addition, polysubstituted aldehydes were accommodated under the optimal conditions, giving the corresponding products in good yields (3ax, 3ay, 3ba, 3ga, 3ha). The example of 3fa is especially noteworthy, because the double bond in the alkyl chain was retained and therefore could be utilized for further modifications. Biologically active heterocycles, including benzo[b]thiophene (3ca), chromone (3ea), antipyrine (3ga) and pyrazole (3ha), could be converted into the corresponding isoxazoline products in good yields. Cyclopropanecarboxaldehyde also delivered the desired product 3ia in moderate yield.
Table 2 Evaluation of aldehydesa
Reaction conditions: N-tosylhydrazones (0.65 mmol, generated in situ from aldehyde 1 and TsNHNH2), ethyl acrylate 2a (0.50 mmol), CuCl2 (10 mol%), TMEDA (0.75 mmol), and TBN (2.0 mmol) in 4.0 mL THF at 65 °C for 24 h under air atmosphere. For details, see the ESI.
|
|
Subsequently, the scope of alkenes was evaluated, as illustrated in Table 3. Not surprisingly, a variety of acrylates reacted smoothly to afford the desired products in good yields. A wide range of synthetically versatile functional groups, including ester (4aa–4ae, 4aj, 4ja), trifluoromethyl (4ae) and alkyne (4aj) moieties, were accommodated under the present conditions. For the reaction of acrylamide, including free amine (4af), primary amine (4ah) and secondary amine (4ag and 4ai), the target products were obtained in good yields. Interestingly, the use of a triene also gave the corresponding isoxazoline 4ja, albeit in a relatively low yield, and pentafluorostyrene delivered the desired product 4as in a reasonable yield under the standard conditions. This [2 + 2 + 1] cycloaddition reaction was also found to be compatible with unactivated aliphatic alkenes (4aw, 4ay, 4ga, 4ka, 4ma–4oa). In addition, styrene derivatives carrying both electron-donating and electron-withdrawing groups afforded the corresponding isoxazoline products (4ak–4ar) in acceptable yields. Under the identical conditions, the reaction with diethyl vinylphosphonate resulted in the desired product 4ea in a good yield. Silylated alkenes, including vinyltrimethylsilane (4ca) and vinylphenyldimethylsilane (4av), were also viable substrates for this transformation. Notably, even enamides (4au), enol esters (4la) and enol ethers (4az) were smoothly transformed into the corresponding products in synthetically useful yields. In addition, both internal alkenes (4ga) and 1,1-disubstitued alkenes (4at, 4ha, 4ma) also underwent smooth cyclization with satisfactory yields, and two spiro products (4at and 4ha) were obtained in good yields. Biologically active heterocycles, including saccharin (4ka) and phthalimide (4na), remained intact during the [2 + 2 + 1] cycloaddition reaction. It is particularly noteworthy that this protocol could generate a wide range of isoxazolines, including those bearing less stable functional groups such as hydroxyl (4ah, 4aw), NHBoc (4ay), free N–H (4af, 4ah), thioether (4da) and acetal (4fa) moieties, which are typically challenging substrates in traditional nitrile oxide cycloadditions. The retention of these versatile functional groups provides the option for further modification of the corresponding isoxazolines products.
Table 3 Evaluation of alkenesa
Reaction conditions: N-tosylhydrazones (0.65 mmol, generated in situ from 4-bromobenzaldehyde 1a and TsNHNH2), alkenes 2 (0.50 mmol), CuCl2 (10 mol%), TMEDA (0.75 mmol), and TBN (2.0 mmol) in 4.0 mL THF at 65 °C for 24 h under air atmosphere.
|
|
The excellent functional group tolerance exhibited by this process prompted us to apply this methodology to bioactive and pharmaceutical molecules (Table 4). The reactions of N-tosylhydrazones with suitable alkenes provided drug candidates such as carbonic anhydrase inhibitors (5),8a anticancer agents (6)8c and antibacterial agents (8)8g in one-pot processes with moderate yields. Furthermore, various complex bioactive molecules (indomethacin 7, naproxen 9, pregnenolone 10, estrone 11, ezetemibe 12) reacted with the N-tosylhydrazone obtained from 4-bromobenzaldehyde, 1a, under the established conditions, and were transformed into the corresponding isoxazolines in moderate to good yields. These examples highlight the wide applicability of this new method, and we therefore envision that this protocol could have potential applications in drug development.
Table 4 Synthetic application for biologically important derivativesa
Reaction conditions: N-tosylhydrazones (0.65 mmol, generated in situ from aldehydes 1 and TsNHNH2), alkenes 2 (0.50 mmol), CuCl2 (10 mol%), TMEDA (0.75 mmol), and TBN (2.0 mmol) in 4.0 mL THF at 65 °C for 24 h under air atmosphere.
|
|
Another advantage of our cycloaddition approach is ready scalability, as shown in Scheme 3. The reaction of 26 mmol 1a, 28 mmol TsNHNH2, 20 mmol 2a and 80 mmol TBN with 10 mol% CuCl2 at 65 °C for 24 h, followed by filtration through a pad of silica gel, afforded 3aa in 85% yield (Scheme 3a). Thus, there was no significant loss in efficiency compared with the reaction on the 0.5 mmol scale. We also conducted further transformations of the isoxazoline as shown in Scheme 3b. Both reductive cleavage (13) and hydrolysis (14) proceeded smoothly to give the corresponding high-value derivatives in good yields.
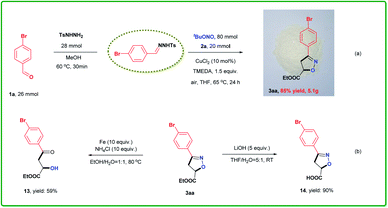 |
| Scheme 3 Scale-up experiment and further transformations. | |
A variety of control experiments were performed to gain preliminary insights into the possible mechanistic pathways (Scheme 4). The addition of butylated hydroxytoluene (BHT) only minimally inhibited the formation of isoxazoline 3aa, indicating that radical intermediates were not involved in this transformation (Scheme 4a). When oxime (15), a precursor of nitrile oxide, was subjected to this cycloaddition reaction, only a small amount of 3aa was obtained (Scheme 4b). In addition, furoxan 16 in Scheme 4c was not detected during the reaction. Because the proposed nitronate intermediate did not incorporate suitable electron-withdrawing groups, and was therefore not sufficiently stable, it could not be isolated. Fortunately, a trace amount of nitronate 17 was detected after careful analysis using liquid chromatography-high resolution mass spectrometry (for details, see the ESI†). Together, these data indicate that nitronates rather than nitrile oxides were involved in the isoxazoline formation reaction.
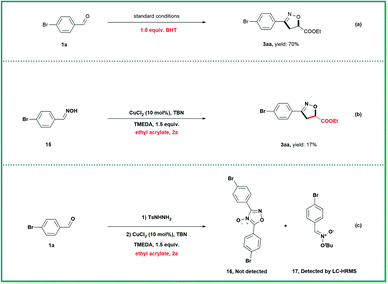 |
| Scheme 4 Probe for the possible mechanism. | |
Computational studies were also performed to gain further insight into the isoxazoline formation mechanism. The results showed that, in the absence of CuCl2 as a catalyst, the nucleophilic substitution reaction between a diazo compound (INT1) generated in situ from the N-tosylhydrazone and TBN produces transition state TS1. Here, the C1⋯N1 distance is shortened to 1.92 Å, while the C1⋯N2 distance is lengthened to 1.39 Å (Fig. 1). Subsequently, a nitronate (INT2) is afforded along with the release of N2. It is noteworthy that, in the presence of the copper catalyst, INT1 might coordinate with the CuCl2 to form complex INT2′. This species can subsequently release N2viaTS1′ to generate intermediate INT3′ exergonically (Fig. 2). Following this, the nucleophilic attack of TBN on INT3′viaTS2′ could afford intermediate INT4′, after which a nitronate (INT2) is generated viaTS3′ along with the regeneration of CuCl2. Similarly, employing CuCl as the catalyst could also promote the formation of nitronate INT2via analogous steps (Fig. S3 in the ESI†). This nitronate could then undergo an intermolecular [3 + 2] cycloaddition with an alkene (2a) to produce a cyclized intermediate. Both regioselective cycloaddition pathways were considered, and the corresponding transition states were determined to be TS2b (Fig. 1) and TS2c (Fig. S2 in the ESI†), respectively. In the case of TS2b, the C1⋯C2 and O1⋯C3 distances are shortened to 2.12 and 2.34 Å, respectively. Thus, the five-membered ring intermediate INT3 is generated in a concerted manner. The computational results also suggested that the energy barrier to the formation of INT3viaTS2b is 1.6 kcal mol−1 lower than that associated with the intermolecular [3 + 2] cycloaddition viaTS2c. Thus, the formation of INT3viaTS2b should occur more readily. Finally, the elimination of tBuOH viaTS3c would be expected to give the desired product. This process could be assisted by a water molecule acting as a shuttle to transfer a proton viaTS3b (Fig. 1). The possible elimination of tBuOH from nitronate INT2 to afford a nitrile oxide intermediate (INT4) was also considered. When a water molecule is present to assist the process, the corresponding TS is TS2a, and the activation energy calculated for this step is 1.7 kcal mol−1 higher than that for the intermolecular [3 + 2] cycloaddition viaTS2b. Thus, the formation of the nitrile oxide intermediate is not suggested in this reaction, although the subsequent intermolecular [3 + 2] cycloaddition with 2aviaTS3a should proceed to give the desired product. At present, we suggest that the product is most likely obtained through intermediate INT3 followed by the elimination of tBuOH.
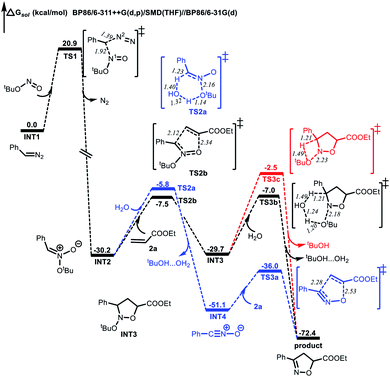 |
| Fig. 1 Energy profiles (in kcal mol−1) for the formation of isoxazolines in the absence of the CuCl2 catalyst. Bond lengths are shown in Å. | |
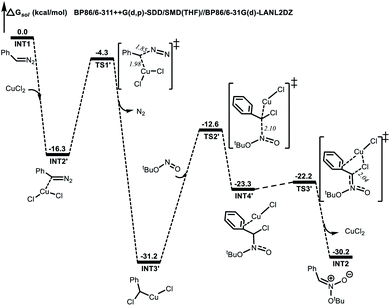 |
| Fig. 2 Energy profile (in kcal mol−1) for the formation of nitronate with the CuCl2 catalyst. Bond lengths are shown in Å. | |
Based on both our experimental results and density functional theory (DFT) calculations, a plausible catalytic cycle was proposed, as shown in Scheme 5. Initially, a condensation reaction between the aldehyde and the TsNHNH2 generates the N-tosylhydrazone A. A then produces the diazo compound B in the presence of TMEDA, which is subsequently captured by TBN to form the nitronate C, aided by CuCl2. Next, the [3 + 2] cycloaddition of C with the alkene gives rise to the nitroso acetal D.15a,15e,17 Finally, the release of a tert-butyloxy group from D delivers the desired isoxazoline.
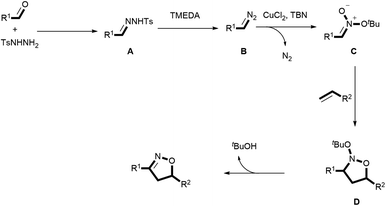 |
| Scheme 5 Proposed catalytic cycle. | |
Conclusions
A novel CuCl2-catalysed [2 + 2 + 1] cycloaddition strategy based on the reaction of N-tosylhydrazones, TBN and alkenes has been developed and characterized. This modular protocol offers a practical and flexible approach to the synthesis of isoxazolines, and a vast array of aldehydes and alkenes have been found to be suitable substrates. Thus, this method represents a highly valuable complement to classical nitrile oxide-based cycloaddition. We believe that this technique may have potential uses in drug discovery because the synthesis is both facile and compatible with a wide range of functional groups. DFT calculations and experimental work provided preliminary mechanistic insights, and the proposed mechanism evidently involves the generation of nitronates and subsequent cycloaddition with alkenes. Further, more detailed investigations of the reaction mechanism and synthetic applications are in progress in our laboratory.
Data availability
All data is in the ESI.† There is no more to deposit.
Author contributions
L. M. performed the experiments and prepared the ESI.† X. C., S. T., G. J., X. L. and J. Y. prepared some substrates and repeated experiments. F. J. and X. B. carried out all computational work. X. B. and X. W. conceived and directed the project and wrote the paper. All the authors discussed the results and commented on the manuscript.
Conflicts of interest
There are no conflicts to declare.
Acknowledgements
This work was funded by the Priority Academic Program Development of Jiangsu Higher Education Institutions (PAPD), NSFC (grant numbers 21971175, 21572148, 21973068). We thank Liwen Bianji (Edanz) (https://www.liwenbianji.cn) for editing the language of a draft of this manuscript.
Notes and references
-
(a)
M. I. Page, Reactions of aldehydes and ketones and their derivatives, in Organic Reaction Mechanisms 1989, ed. A. C. Knipe and W. E. Watts, John Wiley & Sons Ltd, 1991, ch. 1 Search PubMed;
(b)
B. A. Murray, Reactions of Aldehydes and Ketones and their Derivatives, in Organic Reaction Mechanisms 2015, ed. A. C. Knipe, John Wiley & Sons Ltd, 2019, ch 1 Search PubMed.
- For selected reviews on this topic, see:
(a) Y. Xia and J. Wang, J. Am. Chem. Soc., 2020, 142, 10592 CrossRef CAS PubMed;
(b) D. Arunprasath, B. D. Bala and G. Sekar, Adv. Synth. Catal., 2019, 361, 1172 CrossRef CAS;
(c) H. Wang, Y.-H. Deng and Z. Shao, Synthesis, 2018, 50, 2281 CrossRef CAS;
(d) Y. Xia, D. Qiu and J. Wang, Chem. Rev., 2017, 117, 13810 CrossRef CAS PubMed;
(e) Y. Xia and J. Wang, Chem. Soc. Rev., 2017, 46, 2306 RSC;
(f) A. P. Jadhav, D. Ray, V. U. B. Rao and R. P. Singh, Eur. J. Org. Chem., 2016, 2016, 2369 CrossRef CAS;
(g) Z. Shao and H. Zhang, Chem. Soc. Rev., 2012, 41, 560 RSC;
(h) J. Barluenga and C. Valdes, Angew. Chem., Int. Ed., 2011, 50, 7486 CrossRef CAS PubMed.
- For recent examples on this topic, see:
(a) Y. Yang, J. Tsien, A. Ben David, J. M. E. Hughes, R. R. Merchant and T. Qin, J. Am. Chem. Soc., 2021, 143, 471 CrossRef CAS PubMed;
(b) Z. Liu, S. Cao, J. Wu, G. Zanoni, P. Sivaguru and X. Bi, ACS Catal., 2020, 10, 12881 CrossRef CAS;
(c) Y. Huang, X. Li, X. Wang, Y. Yu, J. Zheng, W. Wu and H. Jiang, Chem. Sci., 2017, 8, 7047 RSC;
(d) M. Plaza and C. Valdes, J. Am. Chem. Soc., 2016, 138, 12061 CrossRef CAS PubMed;
(e) S. Xu, G. Wu, F. Ye, X. Wang, H. Li, X. Zhao, Y. Zhang and J. Wang, Angew. Chem., Int. Ed., 2015, 54, 4669 CrossRef CAS PubMed;
(f) Z. Liu, H. Tan, T. Fu, Y. Xia, D. Qiu, Y. Zhang and J. Wang, J. Am. Chem. Soc., 2015, 137, 12800 CrossRef CAS PubMed;
(g) A. R. Reddy, C.-Y. Zhou, Z. Guo, J. Wei and C.-M. Che, Angew. Chem., Int. Ed., 2014, 53, 14175 CrossRef CAS PubMed;
(h) T. Yao, K. Hirano, T. Satoh and M. Miura, Angew. Chem., Int. Ed., 2012, 51, 775 CrossRef CAS PubMed;
(i) M. C. Perez-Aguilar and C. Valdes, Angew. Chem., Int. Ed., 2012, 51, 5953 CrossRef CAS PubMed;
(j) H. Li, L. Wang, Y. Zhang and J. Wang, Angew. Chem., Int. Ed., 2012, 51, 2943 CrossRef CAS PubMed;
(k) J. Barluenga, M. Tomas-Gamasa and C. Valdes, Angew. Chem., Int. Ed., 2012, 51, 5950 CrossRef CAS PubMed;
(l) X. Zhao, G. Wu, Y. Zhang and J. Wang, J. Am. Chem. Soc., 2011, 133, 3296 CrossRef CAS PubMed;
(m) J. Barluenga, M. Tomas-Gamasa, F. Aznar and C. Valdes, Angew. Chem., Int. Ed., 2010, 49, 4993 CrossRef CAS PubMed;
(n) J. Barluenga, M. Escribano, F. Aznar and C. Valdes, Angew. Chem., Int. Ed., 2010, 49, 6856 CrossRef CAS PubMed;
(o) J. Barluenga, M. Tomas-Gamasa, F. Aznar and C. Valdes, Nat. Chem., 2009, 1, 494 CrossRef CAS PubMed;
(p) J. Barluenga, P. Moriel, C. Valdes and F. Aznar, Angew. Chem., Int. Ed., 2007, 46, 5587 CrossRef CAS PubMed.
- For representative examples on this topic, see:
(a) Y. Pang, Q. He, Z.-Q. Li, J.-M. Yang, J.-H. Yu, S.-F. Zhu and Q.-L. Zhou, J. Am. Chem. Soc., 2018, 140, 10663 CrossRef CAS PubMed;
(b) R. Lingayya, M. Vellakkaran, K. Nagaiah, P. R. Tadikamalla and J. B. Nanubolu, Chem. Commun., 2017, 53, 1672 RSC;
(c) T. Ishikawa, M. Kimura, T. Kumoi and H. Iida, ACS Catal., 2017, 7, 4986 CrossRef CAS;
(d) C. Zhu, P. Chen, W. Wu, C. Qi, Y. Ren and H. Jiang, Org. Lett., 2016, 18, 4008 CrossRef CAS PubMed;
(e) Q. Teng, Y. Liu, J. Hu, H. Chai, K. Li and H. Zhang, Tetrahedron, 2016, 72, 3890 CrossRef CAS;
(f) R. Lingayya, M. Vellakkaran, K. Nagaiah and J. B. Nanubolu, Adv. Synth. Catal., 2016, 358, 81 CrossRef CAS;
(g) J. Chen, Y. Jiang, J.-T. Yu and J. Cheng, J. Org. Chem., 2016, 81, 271 CrossRef CAS PubMed;
(h) W. Xiong, C. Qi, H. He, L. Ouyang, M. Zhang and H. Jiang, Angew. Chem., Int. Ed., 2015, 54, 3084 CrossRef CAS PubMed;
(i) C. Hepples and G. K. Murphy, Tetrahedron Lett., 2015, 56, 4971 CrossRef CAS;
(j) A. B. Cuenca, J. Cid, D. Garcia-Lopez, J. J. Carbo and E. Fernandez, Org. Biomol. Chem., 2015, 13, 9659 RSC;
(k) Z. Chen, Q. Yan, H. Yi, Z. Liu, A. Lei and Y. Zhang, Chem.–Eur. J., 2014, 20, 13692 CrossRef CAS PubMed;
(l) J. Aziz, G. Frison, M. Gomez, J.-D. Brion, A. Hamze and M. Alami, ACS Catal., 2014, 4, 4498 CrossRef CAS;
(m) A. K. Yadav, V. P. Srivastava and L. D. Yadav, Chem. Commun., 2013, 49, 2154 RSC;
(n) J. Aziz, J.-D. Brion, A. Hamze and M. Alami, Adv. Synth. Catal., 2013, 355, 2417 CrossRef CAS;
(o) A.-H. García-Muñoz, M. Tomás-Gamasa, M. C. Pérez-Aguilar, E. Cuevas-Yañez and C. Valdés, Eur. J. Org. Chem., 2012, 2012, 3925 CrossRef;
(p) A. Hamze, B. Treguier, J. D. Brion and M. Alami, Org. Biomol. Chem., 2011, 9, 6200 RSC;
(q) Q. Ding, B. Cao, J. Yuan, X. Liu and Y. Peng, Org. Biomol. Chem., 2011, 9, 748 RSC.
- For representative examples on this topic, see:
(a) P. R. Clark, G. D. Williams, J. F. Hayes and N. C. O. Tomkinson, Angew. Chem., Int. Ed., 2020, 59, 6740 CrossRef CAS PubMed;
(b) K. Luo, S. Mao, K. He, X. Yu, J. Pan, J. Lin, Z. Shao and Y. Jin, ACS Catal., 2020, 10, 3733 CrossRef CAS;
(c) M. Zhou, M. Lankelma, J. I. van der Vlugt and B. de Bruin, Angew. Chem.,
Int. Ed., 2020, 59, 11073 CrossRef CAS PubMed;
(d) Y. Yu, P. Chakraborty, J. Song, L. Zhu, C. Li and X. Huang, Nat. Commun., 2020, 11, 461 CrossRef CAS PubMed;
(e) Y. Ning, X. Zhang, Y. Gai, Y. Dong, P. Sivaguru, Y. Wang, B. R. P. Reddy, G. Zanoni and X. Bi, Angew. Chem., Int. Ed., 2020, 59, 6473 CrossRef CAS PubMed;
(f) Y. Wang, X. Wen, X. Cui and X. P. Zhang, J. Am. Chem. Soc., 2018, 140, 4792 CrossRef CAS PubMed;
(g) X. Kou, Q. Shao, C. Ye, G. Yang and W. Zhang, J. Am. Chem. Soc., 2018, 140, 7587 CrossRef CAS PubMed;
(h) Y. Wang, X. Wen, X. Cui, L. Wojtas and X. P. Zhang, J. Am. Chem. Soc., 2017, 139, 1049 CrossRef CAS PubMed;
(i) S. Mai and Q. Song, Angew. Chem., Int. Ed., 2017, 56, 7952 CrossRef CAS PubMed;
(j) A. J. Xia, T. R. Kang, L. He, L. M. Chen, W. T. Li, J. L. Yang and Q. Z. Liu, Angew. Chem., Int. Ed., 2016, 55, 1441 CrossRef CAS PubMed;
(k) A. R. Reddy, F. Hao, K. Wu, C. Y. Zhou and C. M. Che, Angew. Chem., Int. Ed., 2016, 55, 1810 CrossRef CAS PubMed;
(l) B. G. Das, A. Chirila, M. Tromp, J. N. Reek and B. Bruin, J. Am. Chem. Soc., 2016, 138, 8968 CrossRef CAS PubMed;
(m) R. Barroso, A. Jimenez, M. Carmen Perez-Aguilar, M.-P. Cabal and C. Valdes, Chem. Commun., 2016, 52, 3677 RSC;
(n) N. Su, J. A. Theorell, D. J. Wink and T. G. Driver, Angew. Chem., Int. Ed., 2015, 54, 12942 CrossRef CAS PubMed;
(o) Z. Liu, H. Tan, L. Wang, T. Fu, Y. Xia, Y. Zhang and J. Wang, Angew. Chem., Int. Ed., 2015, 54, 3056 CrossRef CAS PubMed;
(p) N. D. Paul, S. Mandal, M. Otte, X. Cui, X. P. Zhang and B. de Bruin, J. Am. Chem. Soc., 2014, 136, 1090 CrossRef CAS PubMed;
(q) X. Q. Hu, J. R. Chen, Q. Wei, F. L. Liu, Q. H. Deng, A. M. Beauchemin and W. J. Xiao, Angew. Chem., Int. Ed., 2014, 53, 12163 CrossRef CAS PubMed;
(r) Z.-J. Cai, X.-M. Lu, Y. Zi, C. Yang, L.-J. Shen, J. Li, S.-Y. Wang and S.-J. Ji, Org. Lett., 2014, 16, 5108 CrossRef CAS PubMed;
(s) I. Thome, C. Besson, T. Kleine and C. Bolm, Angew. Chem., Int. Ed., 2013, 52, 7509 CrossRef CAS PubMed;
(t) M. C. Perez-Aguilar and C. Valdes, Angew. Chem., Int. Ed., 2013, 52, 7219 CrossRef CAS PubMed;
(u) Z. Chen, Q. Yan, Z. Liu, Y. Xu and Y. Zhang, Angew. Chem., Int. Ed., 2013, 52, 13324 CrossRef CAS PubMed;
(v) S. S. van Berkel, S. Brauch, L. Gabriel, M. Henze, S. Stark, D. Vasilev, L. A. Wessjohann, M. Abbas and B. Westermann, Angew. Chem., Int. Ed., 2012, 51, 5343 CrossRef CAS PubMed;
(w) V. K. Aggarwal, E. Alonso, G. Hynd, K. M. Lydon, M. J. Palmer, M. Porcelloni and J. R. Studley, Angew. Chem., Int. Ed., 2001, 40, 1430 CrossRef CAS;
(x) V. K. Aggarwal, E. Alonso, G. Fang, M. Ferrara, G. Hynd and M. Porcelloni, Angew. Chem., Int. Ed., 2001, 40, 1433 CrossRef CAS.
- For representative examples on this topic, see:
(a) J. L. Han, Y. Qin, C. W. Ju and D. Zhao, Angew. Chem., Int. Ed., 2020, 59, 6555 CrossRef CAS PubMed;
(b) Q. Zhou, S. Li, Y. Zhang and J. Wang, Angew. Chem., Int. Ed., 2017, 56, 16013 CrossRef CAS PubMed;
(c) J. Feng, B. Li, Y. He and Z. Gu, Angew. Chem., Int. Ed., 2016, 55, 2186 CrossRef CAS PubMed;
(d) F. Hu, Y. Xia, F. Ye, Z. Liu, C. Ma, Y. Zhang and J. Wang, Angew. Chem., Int. Ed., 2014, 53, 1364 CrossRef CAS PubMed;
(e) X. Zeng, G. Cheng, J. Shen and X. Cui, Org. Lett., 2013, 15, 3022 CrossRef CAS PubMed;
(f) Q. Xiao, B. Wang, L. Tian, Y. Yang, J. Ma, Y. Zhang, S. Chen and J. Wang, Angew. Chem., Int. Ed., 2013, 52, 9305 CrossRef CAS PubMed;
(g) Q. Sha, Y. Ling, W. Wang and Y. Wei, Adv. Synth. Catal., 2013, 355, 2145 CrossRef CAS;
(h) Q. Xiao, Y. Xia, H. Li, Y. Zhang and J. Wang, Angew. Chem., Int. Ed., 2011, 50, 1114 CrossRef CAS PubMed;
(i) X. Zhao, J. Jing, K. Lu, Y. Zhang and J. Wang, Chem. Commun., 2010, 46, 1724 RSC;
(j) Q. Xiao, J. Ma, Y. Yang, Y. Zhang and J. Wang, Org. Lett., 2009, 11, 4732 CrossRef CAS PubMed.
- For representative examples on this topic, see:
(a) K. Ishitobi, K. Muto and J. Yamaguchi, ACS Catal., 2019, 9, 11685 CrossRef CAS;
(b) W.-D. Chu, F. Guo, L. Yu, J. Hong, Q. Liu, F. Mo, Y. Zhang and J. Wang, Chin. J. Chem., 2018, 36, 217 CrossRef CAS;
(c) Z. Zhang, Q. Zhou, W. Yu, T. Li, G. Wu, Y. Zhang and J. Wang, Org. Lett., 2015, 17, 2474 CrossRef CAS PubMed;
(d) F. Ye, X. Ma, Q. Xiao, H. Li, Y. Zhang and J. Wang, J. Am. Chem. Soc., 2012, 134, 5742 CrossRef CAS PubMed;
(e) L. Zhou, F. Ye, J. Ma, Y. Zhang and J. Wang, Angew. Chem., Int. Ed., 2011, 50, 3510 CrossRef CAS PubMed;
(f) L. Zhou, F. Ye, Y. Zhang and J. Wang, J. Am. Chem. Soc., 2010, 132, 13590 CrossRef CAS PubMed.
- For representative examples on this topic, see:
(a) M. D'Ascenzio, D. Secci, S. Carradori, S. Zara, P. Guglielmi, R. Cirilli, M. Pierini, G. Poli, T. Tuccinardi, A. Angeli and C. T. Supuran, J. Med. Chem., 2020, 63, 2470 CrossRef PubMed;
(b) L. Sun, H. Xu, W. Su, F. Xue, S. An, C. Lu and R. Wu, Plant Physiol. Biochem., 2018, 129, 101 CrossRef CAS PubMed;
(c) G. S. Lingaraju, K. S. Balaji, S. Jayarama, S. M. Anil, K. R. Kiran and M. P. Sadashiva, Bioorg. Med. Chem. Lett., 2018, 28, 3606 CrossRef CAS PubMed;
(d) M. Zayane, A. Rahmouni, M. Daami-Remadi, M. Ben Mansour, A. Romdhane and H. Ben Jannet, J. Enzyme Inhib. Med. Chem., 2016, 31, 1566 CrossRef CAS PubMed;
(e) N. Puttaswamy, G. S. Pavan Kumar, M. Al-Ghorbani, V. Vigneshwaran, B. T. Prabhakar and S. A. Khanum, Eur. J. Med. Chem., 2016, 114, 153 CrossRef CAS PubMed;
(f) S. Castellano, D. Kuck, M. Viviano, J. Yoo, F. Lopez-Vallejo, P. Conti, L. Tamborini, A. Pinto, J. L. Medina-Franco and G. Sbardella, J. Med. Chem., 2011, 54, 7663 CrossRef CAS PubMed;
(g) M. R. Barbachyn, G. J. Cleek, L. A. Dolak, S. A. Garmon, J. Morris, E. P. Seest, R. C. Thomas, D. S. Toops, W. Watt, D. G. Wishka, C. W. Ford, G. E. Zurenko, J. C. Hamel, R. D. Schaadt, D. Stapert, B. H. Yagi, W. J. Adams, J. M. Friis, J. G. Slatter, J. P. Sams, N. L. Oien, M. J. Zaya, L. C. Wienkers and M. A. Wynalda, J. Med. Chem., 2003, 46, 284 CrossRef CAS PubMed.
- For representative examples on this topic, see:
(a) K. Takenaka, X. Lin, S. Takizawa and H. Sasai, Chirality, 2015, 27, 532 CrossRef CAS PubMed;
(b) K. Takenaka, M. Akita, Y. Tanigaki, S. Takizawa and H. Sasai, Org. Lett., 2011, 13, 3506 CrossRef CAS PubMed;
(c) M. A. Arai, T. Arai and H. Sasai, Org. Lett., 1999, 1, 1795 CrossRef CAS.
- For representative examples on this topic, see:
(a) D. Jiang and Y. Chen, J. Org. Chem., 2008, 73, 9181 CrossRef CAS PubMed;
(b) J. P. Scott, S. F. Oliver, K. M. Brands, S. E. Brewer, A. J. Davies, A. D. Gibb, D. Hands, S. P. Keen, F. J. Sheen, R. A. Reamer, R. D. Wilson and U. H. Dolling, J. Org. Chem., 2006, 71, 3086 CrossRef CAS PubMed;
(c) A. A. Fuller, B. Chen, A. R. Minter and A. K. Mapp, J. Am. Chem. Soc., 2005, 127, 5376 CrossRef CAS PubMed;
(d) A. R. Minter, A. A. Fuller and A. K. Mapp, J. Am. Chem. Soc., 2003, 125, 6846 CrossRef CAS PubMed;
(e) A. P. Kozikowski, Acc. Chem. Res., 2002, 17, 410 CrossRef;
(f) E. Marotta, L. M. Micheloni, N. Scardovi and P. Righi, Org. Lett., 2001, 3, 727 CrossRef CAS PubMed;
(g) J. W. Bode and E. M. Carreira, Org. Lett., 2001, 3, 1587 CrossRef CAS PubMed.
- For selected examples on this topic, see:
(a) L. De Angelis, A. M. Crawford, Y. L. Su, D. Wherritt, H. Arman and M. P. Doyle, Org. Lett., 2021, 23, 925 CrossRef CAS PubMed;
(b) G. Zhao, L. Liang, C. H. E. Wen and R. Tong, Org. Lett., 2019, 21, 315 CrossRef CAS PubMed;
(c) M. Xiong, X. Liang, Z. Gao, A. Lei and Y. Pan, Org. Lett., 2019, 21, 9300 CrossRef CAS PubMed;
(d) P. Dai, X. Tan, Q. Luo, X. Yu, S. Zhang, F. Liu and W. H. Zhang, Org. Lett., 2019, 21, 5096 CrossRef CAS PubMed;
(e) X. Shang, K. Liu, Z. Zhang, X. Xu, P. Li and W. Li, Org. Biomol. Chem., 2018, 16, 895 RSC;
(f) A. N. Baumann, F. Reiners, T. Juli and D. Didier, Org. Lett., 2018, 20, 6736 CrossRef CAS PubMed;
(g) S. L. Bartlett, Y. Sohtome, D. Hashizume, P. S. White, M. Sawamura, J. S. Johnson and M. Sodeoka, J. Am. Chem. Soc., 2017, 139, 8661 CrossRef CAS PubMed;
(h) T. D. Svejstrup, W. Zawodny, J. J. Douglas, D. Bidgeli, N. S. Sheikh and D. Leonori, Chem. Commun., 2016, 52, 12302 RSC;
(i) C. Kesornpun, T. Aree, C. Mahidol, S. Ruchirawat and P. Kittakoop, Angew. Chem., Int. Ed., 2016, 55, 3997 CrossRef CAS PubMed;
(j) A. Yoshimura, C. Zhu, K. R. Middleton, A. D. Todora, B. J. Kastern, A. V. Maskaev and V. V. Zhdankin, Chem. Commun., 2013, 49, 4800 RSC;
(k) A. Yoshimura, K. R. Middleton, A. D. Todora, B. J. Kastern, S. R. Koski, A. V. Maskaev and V. V. Zhdankin, Org. Lett., 2013, 15, 4010 CrossRef CAS PubMed;
(l) S. Minakata, S. Okumura, T. Nagamachi and Y. Takeda, Org. Lett., 2011, 13, 2966 CrossRef CAS PubMed;
(m) T. Jen, B. A. Mendelsohn and M. A. Ciufolini, J. Org. Chem., 2011, 76, 728 CrossRef CAS PubMed;
(n) B. A. Mendelsohn, S. Lee, S. Kim, F. Teyssier, V. S. Aulakh and M. A. Ciufolini, Org. Lett., 2009, 11, 1539 CrossRef CAS PubMed;
(o) M. P. Sibi, K. Itoh and C. P. Jasperse, J. Am. Chem. Soc., 2004, 126, 5366 CrossRef CAS PubMed;
(p) M. G. Kociolek and C. Hongfa, Tetrahedron Lett., 2003, 44, 1811 CrossRef CAS;
(q) H. J. Gi, Y. Xiang, R. F. Schinazi and K. Zhao, J. Org. Chem., 1997, 62, 88 CrossRef CAS PubMed;
(r) M. Iwasaki, Y. Kazao, T. Ishida and Y. Nishihara, Org. Lett., 2020, 22, 7343 CrossRef CAS PubMed.
- For representative examples on this topic, see:
(a) S. S. Prasad and S. Baskaran, J. Org. Chem., 2018, 83, 1558 CrossRef CAS PubMed;
(b) G. W. Wang, M. X. Cheng, R. S. Ma and S. D. Yang, Chem. Commun., 2015, 51, 6308 RSC;
(c) C. Li, H. Deng, C. Li, X. Jia and J. Li, Org. Lett., 2015, 17, 5718 CrossRef CAS PubMed;
(d) N. Maugein, A. Wagner and C. Mioskowski, Tetrahedron Lett., 1997, 38, 1547 CrossRef;
(e) Y. Basel and A. Hassner, Synthesis, 1997, 1997, 309 CrossRef;
(f) R. A. Kunetsky, A. D. Dilman, S. L. Ioffe, M. I. Struchkova, Y. A. Strelenko and V. A. Tartakovsky, Org. Lett., 2003, 5, 4907 CrossRef CAS PubMed.
- For representative examples on this topic, see:
(a) E. F. Lopes, F. Penteado, S. Thurow, M. Pinz, A. S. Reis, E. A. Wilhelm, C. Luchese, T. Barcellos, B. Dalberto, D. Alves, M. S. da Silva and E. J. Lenardao, J. Org. Chem., 2019, 84, 12452 CrossRef CAS PubMed;
(b) X. Li, X. Wang, Z. Wang, X. Yan and X. Xu, ACS Sustainable Chem. Eng., 2019, 7, 1875 CrossRef CAS;
(c) J. Zhao, M. Jiang and J.-T. Liu, Adv. Synth. Catal., 2017, 359, 1626 CrossRef CAS;
(d) I. Triandafillidi and C. G. Kokotos, Org. Lett., 2017, 19, 106 CrossRef CAS PubMed;
(e) W. Kong, Q. Guo, Z. Xu, G. Wang, X. Jiang and R. Wang, Org. Lett., 2015, 17, 3686 CrossRef CAS PubMed;
(f) C. B. Tripathi and S. Mukherjee, Angew. Chem., Int. Ed., 2013, 52, 8450 CrossRef CAS PubMed;
(g) D. Jiang, J. Peng and Y. Chen, Org. Lett., 2008, 10, 1695 CrossRef CAS PubMed.
- For representative examples on this topic, see:
(a) A. A. Jimoh, S. Hosseyni, X. Ye, L. Wojtas, Y. Hu and X. Shi, Chem. Commun., 2019, 55, 8150 RSC;
(b) X. T. Li, Q. S. Gu, X. Y. Dong, X. Meng and X. Y. Liu, Angew. Chem., Int. Ed., 2018, 57, 7668 CrossRef CAS PubMed;
(c) W. J. Han, Y. R. Wang, J. W. Zhang, F. Chen, B. Zhou and B. Han, Org. Lett., 2018, 20, 2960 CrossRef CAS PubMed;
(d) X.-X. Peng, D. Wei, W.-J. Han, F. Chen, W. Yu and B. Han, ACS Catal., 2017, 7, 7830 CrossRef CAS;
(e) F. Chen, F. F. Zhu, M. Zhang, R. H. Liu, W. Yu and B. Han, Org. Lett., 2017, 19, 3255 CrossRef CAS PubMed;
(f) R.-H. Liu, D. Wei, B. Han and W. Yu, ACS Catal., 2016, 6, 6525 CrossRef CAS;
(g) L. Zhu, G. Wang, Q. Guo, Z. Xu, D. Zhang and R. Wang, Org. Lett., 2014, 16, 5390 CrossRef CAS PubMed;
(h) B. Han, X. L. Yang, R. Fang, W. Yu, C. Wang, X. Y. Duan and S. Liu, Angew. Chem., Int. Ed., 2012, 51, 8816 CrossRef CAS PubMed.
- For representative examples on this topic, see:
(a) L. Ma, L. Kou, F. Jin, X. Cheng, S. Tao, G. Jiang, X. Bao and X. Wan, Chem. Sci., 2021, 12, 774 RSC;
(b) C. Y. Wang, F. Teng, Y. Li and J. H. Li, Org. Lett., 2020, 22, 4250 CrossRef CAS PubMed;
(c) M. Gao, Y. Gan and B. Xu, Org. Lett., 2019, 21, 7435 CrossRef CAS PubMed;
(d) R. Chen, Y. Zhao, S. Fang, W. Long, H. Sun and X. Wan, Org. Lett., 2017, 19, 5896 CrossRef CAS PubMed;
(e) M. Jiang, L. Feng, J. Feng and P. Jiao, Org. Lett., 2017, 19, 2210 CrossRef CAS PubMed;
(f) M. Gao, Y. Li, Y. Gan and B. Xu, Angew. Chem., Int. Ed., 2015, 54, 8795 CrossRef CAS PubMed;
(g) T. Kudoh, T. Ishikawa, Y. Shimizu and S. Saito, Org. Lett., 2003, 5, 3875 CrossRef CAS PubMed.
-
H. Feuer, Nitrile Oxides, Nitrones and Nitronates in Organic Synthesis, Wiley, Hoboken, 2008 Search PubMed.
-
(a) S. E. Denmark and E. A. Martinborough, J. Am. Chem. Soc., 1999, 121, 3046 CrossRef CAS;
(b) S. E. Denmark and A. Thorarensen, J. Am. Chem. Soc., 1997, 119, 125 CrossRef CAS;
(c) S. E. Denmark and A. Thorarensen, Chem. Rev., 1996, 96, 137 CrossRef CAS PubMed.
Footnote |
† Electronic supplementary information (ESI) available: Experimental procedures, spectroscopic data, NMR spectra. CCDC 2083541. For ESI and crystallographic data in CIF or other electronic format see DOI: 10.1039/d1sc02352g |
|
This journal is © The Royal Society of Chemistry 2021 |
Click here to see how this site uses Cookies. View our privacy policy here.