DOI:
10.1039/D1RA01880A
(Review Article)
RSC Adv., 2021,
11, 16572-16591
Recent progress of MOF-derived porous carbon materials for microwave absorption
Received
10th March 2021
, Accepted 26th April 2021
First published on 5th May 2021
Abstract
Microwave absorbing materials (MAM) have attracted considerable attention over the years in stealth and information technologies. Metal–organic framework (MOF) with a unique microstructure and electronic state has become an attractive focus as self-sacrificing precursors of microwave absorbers. The MOF-derived porous carbon (PC) materials exhibit a high absorbing performance due to the stable three-dimensional structure and homogeneous distribution of metal particles. MOF-derived PC materials are promising for ideal MAM via tuning of the structure and composition, resulting in appropriate impedance matching and the synergistic effect between magnetic and dielectric loss. In this review, the MOF-derived PC materials and their basic absorption mechanisms (dielectric loss, magnetic loss and impedance matching) are introduced, as well as the characters of various MOF-derived PC materials. In addition, this review provides a comprehensive introduction and tabulates the recent progress based on the classification of the MOF-derived metallic state, such as pure PC (without reduced metals), mono-metal/PC, multi-metal/PC, metal oxides/PC and other derived PC composites. Finally, the challenges faced by MOF-derived PC materials are overviewed, and their further development is mentioned.
1. Introduction
In recent decades, the world has witnessed an eruptible development in stealth, information technology and electronics industry that bring public convenience and high-efficiency.1 However, it is worth noting that the living environment has deteriorated dramatically with the explosive growth of modern electronic technology, which not only induces electrical interference with precision equipment and apparatus but also makes an impact on public health.2 Thus, developing ideal microwave absorbing materials (MAM) with higher attenuating capacity, wider absorption bandwidth and lighter weight is an urgent proposition.3
Metal–organic framework (MOF) materials attract a lot of attention owing to their intriguing structure with a large pore volume, high surface area and uniformity of metals. MOFs have exhibited significant applications in gas storage and separation, sensing, catalysis, proton conduction, drug delivery and other fields.4–10 In addition, the synthesis process of MOF materials is simple and environment friendly, which meets the development requirements of green functional materials in modern society.11 Up to now, more than twenty thousand monomers of MOF are prepared with different metal ions and organic ligands, exhibiting rich and varied micro-morphology such as sphere-like, flake-like, cube-like, octahedron-like, dodecahedron-like and so on,12–20 which have received attention from various fields. And the number of MOF materials reported is refreshed constantly with endless emergence of new monomers.
The metal ions cover almost all kinds of metals like main group metals, transition metals, lanthanide metals and rare-earth metals. As for the organic ligands, the common ligands are polyamines (especially amines produced from imidazole, oxalic acid and benzene), carboxylates, pyridyl, porphyrins, cyano groups, crown ethers and phosphonates.21 Therefore, there are various and complicated classification methods of MOF materials depending on what public focuses on. Different types of MOF can be converted into each other simply by changing the microstructure or one of the elements due to the highly adjustable character.
MOF possesses the advantages of high porosity, low density, large specific surface area, regular channel, adjustable pore structure and topological structure diversity.19 MOF-derived materials, especially the carbon materials, have become a new member of the microwave absorption field. The publications of MOF (almost all of publications searched are about MOF-derived porous carbon (PC) materials) as microwave absorbers are increasing progressively in recent ten years, as shown in Fig. 1. Compared with the MOF materials, MOF-derived PC materials are more promising as microwave absorbers.
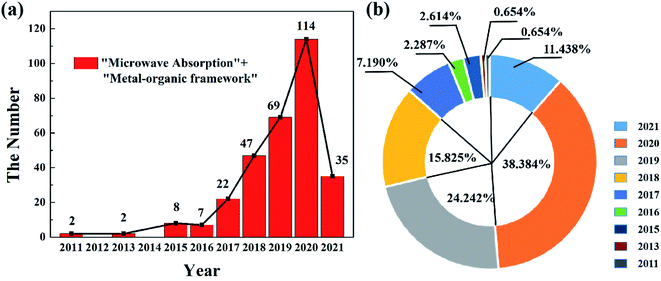 |
| Fig. 1 The number (a) and the proportion (b) of publications on “microwave absorption” and “metal–organic framework” searched by Web of Science. | |
MOF and their composites are used as ideal precursors by controlling pyrolysis and the following postprocessing processes to synthesize MOF-derived PC materials, which have great impedance matching, high porosity and light weight.22,23 The porous structure and the ordered metal arrangement of MOF precursors are greatly inherited into derived PC materials via carbonizing under inert atmosphere (N2 or Ar). Compared with traditional PC materials, MOF-derived PC materials possess unique metal/carbon microstructure with homogeneous metal embedded. Plenty of researches have revealed that the serious aggregation of metals can be effectively avoided because of the metallic ordered arrangement in the MOF-derived metal/carbon construction, so that derived composites often exhibit highly efficient microwave absorbing properties.24 Some of them have confirmed that pure carbon with porous framework can be prepared by calcining or etching the metallic tiny particles. At the same time, with the unique microstructure and composition advantages of MOF, the active ingredients in the derivative materials can be precisely tuned to well overcome the common problems of poor stability and low conductivity for MOF precursors. The tuning progress can provide new reaction sites and expand the applicable reaction range, and also provide great convenience for performance optimization.25,26
MOF-derived PC materials have been widely concerned as microwave absorbers, but there are few literatures focus on the derived PC materials from the view of metallic state.27,28 This review summarizes the MOF-derived PC materials with considerable microwave absorption performance, which are just the tip of the iceberg of big MOF material family. In here, MOF-derived PC materials are divided into pure PC (without metals), metal oxides/PC, mono-metal/PC, multi-metal/PC and other PC composites, which is classified on the different MOF-derived metallic state. The strengths of MOF-derived PC materials are emphasized via comparing with traditional pure PC, and the basic absorption mechanisms are briefly explained. Additionally, this article herein includes the discussion and summarization of major MOF-derived PC materials reported in the recent years. Finally, the MOF-derived PC materials with different classification are tabulated as tables for comparison.
2. MOF-derived PC materials
Carbon-based materials are widely accepted as high MAM due to the strong electromagnetic attenuation ability, low density and chemical stability. Distinct existence forms of carbon-based materials are reported including carbon nanotubes,29 carbon fibers,30 graphene31,32 and carbon spheres.2,33 However, carbon-based materials have relatively high complex permittivity and poor magnetic permeability. These features lead to the unmatched impedance and single attenuation the development as microwave absorbers.34 In the recent years, PC combined with carbon and porous features gain interests, due to the facial synthesis method and high performance.35 The PC materials have been reported and applied in the field of catalysis, supercapacitor and the microwave absorption, due to the high specific areas, tunable pore sizes and unique structures.36 However, pure PC materials commonly exhibit the single loss mechanism, which cannot show the better absorption capacity. One of the common methods is to blend PC materials with the magnetic loss materials, increasing the complex permeability to satisfy the impedance matching.37,38 For instance, Wu et al. synthesized carbon sphere/Fe–Fe3O4 via loading magnetic quantum dots of Fe–Fe3O4 on the mono-dispersed carbon sphere carbonatized from the phenolic resin sphere.39 The as-obtained composites showed excellent electromagnetic absorption properties with the effective absorption bandwidth (EAB) of 5.8 GHz with coating thickness of 1.5 mm. Fan et al. successfully synthesized urchin-like transition metal oxides NiCo2O4 grown on the carbon microsphere, and prepared binary metal oxides ZnO nanoparticles on the surface of C/NiCo2O4 to improve the impedance matching.40 The minimum reflection loss (RL) of composites reached −43.61 dB at 11.61 GHz and the EAB covered 4.32 GHz from 9.74 to 14.06 GHz.
The traditional synthetic methods of carbon/magnetic structure are almost more complicated than methods of MOF-derived PC materials synthesis. Recently, studies have found that high-temperature pyrolysis of MOF precursors in inert atmosphere can generate magnetic metal/carbon composite materials, which maintain the original special structure of MOF materials. Magnetic particles can also be uniformly dispersed on the surface of PC.41 The preparation process of MOF-derived PC materials is facile, and it is easy to disperse reduced metals or metal oxides inside carbon skeleton.42 As a result, PC materials derived from MOF become a new focus for light-weight microwave absorbers and increasingly attract a wide variety of attention.
The unique pore microstructure is retained to MOF-derived PC materials with a large extent and can be flexibly adjusted according to the application performance requirements of the crystal size and pore size.43 The periodic and regular arrangement of metal ion nodes in the carbon framework reduces homogenously dispersion of metal particles.44 These characteristics make it possible for MOF to be used as templates and precursors to prepare the PC composites under suitable calcination conditions.45
The existence of derived carbon/magnetic structure not only prevents the generation of magnetic particles in harsh environments, but also provides stronger interface polarization.46 These results are conducive to the dissipation of microwaves. Various MOF-derived PC materials have been successfully manufactured, such as Fe/C,47,48 Co/C,49,50 Fe3O4/C,51 Co3O4/C,52 CoFe/C,53,54 CoZn/C.55 The MOF-derived PC materials are summarized and tabulated according to different metallic state, so that the composites are easily observed and compared.
3. Principle of MOF-derived PC absorbers
Electromagnetic waves are oscillating particle waves derived from mutually perpendicular electric and magnetic fields in space, so the loss of microwave is mainly induced by the interaction of either one of these fields, or both.56 In the previous literature reported, the mechanisms of absorption could be described as dielectric loss, magnetic loss and their synergistic effect. With unique three-dimensional carbon/magnetic structure, MOF-derived PC materials possess optimized impedance matching and excellent performance as microwave absorbers. The evaluation of the absorbing performance is mainly based on two key characteristics: impedance matching and attenuation capacity.
3.1 Dielectric loss
Dielectric loss is described that the microwave energy is translated into thermal energy loss via the electronic interaction between MOF-derived PC materials and electric field of incident microwave. The polarity changing of the electric field is sinusoidally and orthogonal to the forward propagation direction of incident microwave. The polarization phenomenon is induced by generating of conductive current in an alternating electric field. The dielectric loss occurs when the polarization changes slower than the rate of the oscillation field, and then the energy of incident microwave is converted into thermal loss through repeated polarization relaxation of the absorbing materials.57 In addition, the collision and scattering of electrons can be thought as “friction” during transportation of microwave, and this “friction” induces energy conversion, which manifests as the subsequent generation of heat and reduction of microwave.58 Generally, relative permittivity (εr) is the main parameter for estimating dielectric loss, which is shown in eqn (1): |
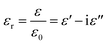 | (1) |
It is widely accepted that carbon absorbers not only possess strong dielectric loss property, but also have the advantage of light weight and great thermal stability for practical application.59 These features are especially enhanced and optimized in the MOF-derived PC materials with high porosity and relative permittivity. The porous structure retained from MOF precursors exhibits large of holes. And carbon skeleton around these pores could attain micro-size even nano-size which are much less than the length of incident microwave, greatly reducing the reflection from the PC surface.57 The MOF-derived PC materials with high conductivity can provide a longer transmission channel for dissipating current to enhance dielectric loss.
3.2 Magnetic loss
Magnetic loss is magnetic interaction between absorption materials and magnetic field of incident microwave. In general, magnetic loss includes magnetic hysteresis loss, eddy current loss and resonance effect. In an alternating magnetic field, the change of the magnetization vector lags behind the changing of the magnetic field and the generation of ring eddy current is perpendicular to the direction of the changing magnetic flux, inducing the loss of microwaves.60 Magnetic metals (Ni, Co, Fe) and metallic compounds (Fe3O4, γ-Fe2O3, ferrite) are the most typical MAM.61 Magnetic materials can effectively induce the entrance of incident waves, avoiding the skin effects at high frequencies and convert the electromagnetic energy into thermal energy and losses.38,41
Reduced magnetic metals and metallic compounds of MOF precursors are evenly distributed in carbon framework as nanoscale quantum dots without agglomeration. This character mainly results in eddy current losses and resonance effects.56 Meanwhile, the nano-size metallic quantum dots have large specific surface area, which cause more interface polarization, multiple scattering and reflections. In addition, the unique quantum size effect of nano-particles can degrade the electron energy level, which causes a new attenuation channel of microwave energy.57 The relative permeability μr of absorbers is used to estimate magnetic loss, as shown in eqn (2).
|
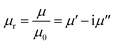 | (2) |
In eqn (1) and (2), the real part (ε′, μ′) of the complex permittivity and complex permeability represent the storage capacity of electromagnetic energy, and the imaginary part (ε′′, μ′′) are often used as key parameters to represent the consumption capacity of electromagnetic energy.
Typical high permeability absorbers mainly include magnetic metals and metallic compounds, which can be produced via pyrolysis in inert atmosphere. And the absorbers effectively combined with metallic components exhibit improving Debye dipole polarization and multiple interface reflection.62 Diversiform metals and metallic compounds can be combined via simple doping method. As a simple example, Fe–Co alloy is an ideal absorber for constructing a composite of magnetic material and carbon material with high saturation magnetization, Curie temperature and conductivity, which are better than those of PC doped with Co only.14 In addition, FeCo or FeNi alloys generally exhibit greater permeability, Snoek's limit and higher saturation magnetization than ferrites, which avail the modification and tuning of absorbing properties.63
3.3 Synergistic effect of magnetic/dielectric loss
The strong microwave energy loss is common achieved through a multitude of loss mechanism.64 Microwave absorbers composited of dielectric and magnetic loss materials are promising with optimized impedance matching and synergistic effect between dielectric loss and magnetic loss. And the impedance matching characteristic is typically represented by reflectivity R and term Zin, as shown in eqn (3)–(5).65 Nearly all of microwaves can enter into absorbing materials when Zin is close to 1 (nearly zero reflection at interface). The single-component carbon materials are not conducive to impedance matching because of the large dielectric constant, low magnetic permeability and narrow EAB. And single metal materials with overhigh magnetic loss are difficult to meet an ideal microwave absorber. Some of incident waves are reflected with only high eddy current loss, which is not conducive to the absorbing property.66 MOF-derived PC materials are coupled with carbon skeleton and metal particles, and carbon skeleton not only improves the corrosion resistance to some degree, but also effectively isolates metal particles, reducing eddy current losses to maintain excellent absorbing performance.62 |
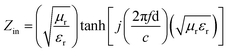 | (3) |
|
 | (4) |
|
 | (5) |
The impedance matching and the absorbing characteristic should be considered comprehensively for an ideal absorber, and the absorbing property is generally indicated by the RL, which is expressed as eqn (6).
|
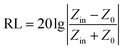 | (6) |
For MOF-derived PC materials, the unique embedded structure of the metals and carbon exhibits abundant interfaces with a large amount of free charge accumulating on them, resulting in the interface polarization. Besides, multiple scattering reflection and interfacial polarization are increased in the porous structure of MOF-derived PC materials, enhancing the absorption performance.38 For instance, Li et al. obtained magnetic Co–C@C composites from ZIF materials. The composites showed the smaller RL of −58.0 dB and wider bandwidth of 5.7 GHz compared with previously reported composites, due to the favorable impedance matching and strong attenuation.67 The size of metal nanoparticles and pores is adjusted with the different pyrolysis temperature, which can tune the impedance matching.68 The introduction of pores could decrease the density of the absorbers and have an effect on the permittivity of carbon materials. Ding et al. studied MIL-53(Fe) derived MCC/rGO composites by gradient temperature calcination and discussed the effect of temperature for absorption performance.69 The framework enhanced the internal scattering, and the graphene provided a larger absorption surface, efficiently enhancing the absorbing performance.
In a word, compared with common materials, the MOF-derived PC materials with nanoscale quantum dots exhibit better absorbing performance. The synergistic effect of absorbers can achieve excellent impedance matching and high absorbing capacity,53 as shown in Fig. 2.
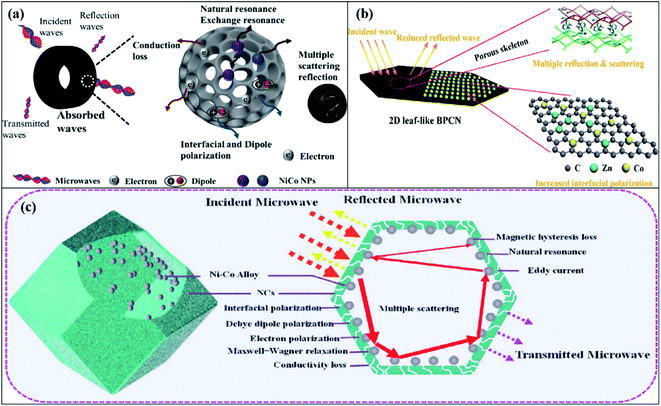 |
| Fig. 2 Schematic diagram of microwave absorption mechanism of MOF-derived metal/C composites, this figure has been adapted from ref. 41, 70 and 71 with permission from Applied Surface Science, Copyright 2020, Composites Communications, Copyright 2020 and Applied Surface Science, Copyright 2020, respectively. | |
4. MOF-derived PC materials for microwave absorption
The MOF-derived PC composites exhibit huge potential application as high-performance microwave absorption materials. And the derived PC mentioned herein from recent literatures are divided into a series of subsets for categorization. Based on the metal state, MOF-derived PC composites can be classified as pure PC, mono-metal/PC, multi-metal/PC, metal oxides/PC and other MOF-derived PC composites. The derived pure PC also be employed not only as absorbers but also as reactive materials.
4.1 Pure PC
As mentioned above, PC exhibits potential application for microwave absorption. MOF-derived pure PC is a high porosity carbon material without metals, which is generally obtained via high temperature pyrolyzation process to remove low-boiling point metals. In addition, it can also be prepared through acid etching to remove reduced active metals. MOF-derived pure PC absorbers are often composited with other loss materials because of their restriction as wide frequency bandwidth microwave absorbers.
Various composite forms of derived pure PC are reported. Some are applied as central core of core–shell or yolk–shell structure with other cooperate materials assembling upon their surface. Besides, some are grown on the matrix to construct the porous and stable carbon shell. Derived pure PC can also be combined with two-dimensional materials to play a disperse and dopant role. Zhao and co-workers synthesized nano-polyhedron PC decorating on the surface of reduced graphene oxide (rGO) by in situ pyrolysis.72 ZIF (zeolitic imidazolate framework) is a typical precursor to obtain pure PC materials, such as ZIF-8 (Zn-MOF). It is demonstrated that the grown of ZIF-8 particles is restrained by GO and the derived PC reduces the re-stacking of rGO nanosheets, which yield optimized performance with minimum RL value of −66.2 dB at the thickness of 2.89 mm at 6.2 GHz (as shown in Fig. 3). Because of the low boiling point of metal Zn, ZIF-8 has played a significant role in acting as admirable templates to obtain pure PC materials.34,73,74 Wu's group also used ZIF-8 as the derived loose PC precursor to prepare high-performance absorbers.75 By the way, the MOF-derived composites are reported that they can be effectively dispersed in one-dimensional and two-dimensional materials such as carbon fiber and graphene, which play a role in avoiding agglomeration.
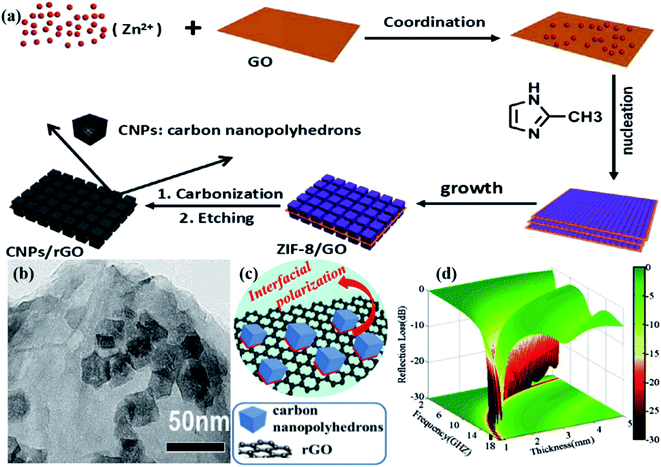 |
| Fig. 3 The fabrication of CNPs/GO derived from ZIF-8/GO (a), TEM images of CNPs/GO (b), interaction (c) and the RL of CNPs/GO (d). This figure has been reproduced from ref. 72 with permission from Journal of Colloid and Interface Science, Copyright 2018. | |
It is feasible to obtain pure PC via using other metal-based MOF-derived composites as precursors and adding acid solution to get rid of metals. For example, Du et al. used Ni-MOF as templates to synthesize the multi-chamber carbon microspheres (MCCMs) via the pyrolysis progress and etching treatment.76 The unique multi-chamber structure possessed better impedance matching characteristic than hollow carbon microspheres. Peng et al. obtained PC though removing Cu from the prepared HKUST-1 (Cu-MOF) and studied the microwave attenuation of the derived PC composites.77
Similar to above absorbers of MOF-derived PC, an increasing number of PC composites have been developed with other magnetic loss and dielectric loss materials to induce microwave absorption, through the increased research of MOF precursors. The minimum RL and EAB with their corresponding loading and thickness of these similar materials are tabulated in Table 1.
Table 1 The microwave absorbing performance of MOF-derived pure PC materials
Absorbers |
Loading (wt%) |
RLmin |
EAB |
Ref. |
Thickness (mm) |
Value (dB) |
Thickness (mm) |
Value (GHz) |
CNPs/GO |
40 |
2.89 |
−66.2 |
1.5 |
3.6 |
72 |
Co@NPC |
25 |
1.45 |
−48 |
1.65 |
5.2 |
73 |
Hollow GO@PC |
10 |
3.7 |
−32.43 |
3.5 |
4.2 |
74 |
N-doped PC |
50 |
4.0 |
−39.7 |
4.0 |
4.3 |
75 |
MCCMs |
30 |
2.0 |
−28.5 |
1.8 |
5.7 |
76 |
NPC@CPI-2 |
50 |
1.9 |
−46.86 |
1.65 |
3.74 |
77 |
C@Co/NC |
25 |
2.2 |
−52.5 |
2.2 |
4.4 |
78 |
Fe/C |
60 |
3.0 |
−40.0 |
1.5 |
6.0 |
79 |
Fe–N/C |
33.3 |
1.7 |
−30.98 |
1.7 |
5.04 |
80 |
4.2 Mono-metal/PC
The derived-PC composites filled with metallic particles possess the increased magnetic interaction with incident microwave and the optimized impedance matching. As a result, the efforts of developing novel microwave absorbers have been devoted to study compounds of carbon materials and magnetic metal nanoparticles in recent years. When MOF-derived PC composites are combined with single component metal and carbon, the composites can be classified as MOF-derived mono-metal/PC materials. Typical magnetic loss absorbing metallic materials includes Fe, Co and Ni, which are also mainly applied in the MOF-derived PC materials.49,81,82
Prussian blue (PB) is one of common Fe-based MOF which consists of Fe4[Fe(CN)6]3 (a mixed-valence iron(III) hexacyanoferrate(II) compound) with a face-centered-cubic (fcc) crystal structure. And it is an eligible precursor candidate for derived Fe/C composites because of abundant contents of carbon and iron species.83–85 Xu et al. used PB as a MOF precursor to fabricate core–shell Fe@graphitic carbon nanoparticles under different pyrolysis temperature (600–700 °C), and the results displayed strong dielectric loss and magnetic loss properties (Fig. 4).83 The PB derived Fe/C and its composites have been noticed as new microwave absorbers.85 In addition, the more efforts are devoted on MIL (named based on the discovery place: Materials of Institut Lavoisier) series for Fe-based PC derivatives. Miao et al. reported the morphology effect on microwave absorbing by annealing the two MOF precursors (MIL-101-Fe and MIL-88B-Fe).18 Both of them had almost identical chemical composition and microstructure, including element content, valence state, pore size and volume, but with different topology. The Fe/C-700@101 and Fe/Fe3C/C-800@101 (the pyrolysis temperature of MIL-101 precursor was 700 °C and 800 °C) were reduced at high temperature from ferric oxide by CO and reductive carbon generated, showing the excellent performance, as shown in Fig. 4. However, in comparison with pure iron, ferric oxides exhibit chemical stability and have wider application for microwave absorption, which are tabulated in the fourth part of this chapter.
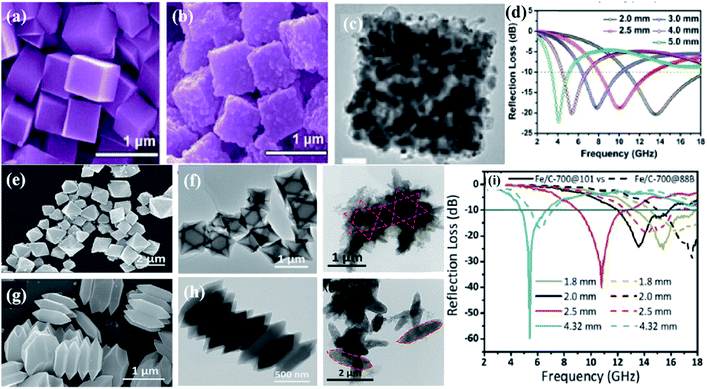 |
| Fig. 4 SEM and TEM images of PB (a), Fe/C-PB (b and c), MIL-101-Fe (e), Fe/Fe3C/C-800@101 (f), MIL-88B-Fe (g), and Fe/Fe3C/C-800@88B (h), respectively. RL of Fe/C-PB (d), Fe/C-700@101 and Fe/C-700@88B (i), respectively. This figure has been reproduced from ref. 18 and 83 with permission from Advanced Materials Interfaces, Copyright 2020 and Journal of Materials Chemistry A, Copyright 2015. | |
The Ni/C composites with the shape of porous spheres are formed by H3BTC (BTC = benzene-1,3,5-tricarboxylate) and Ni2+. The amorphous spheres formed with Ni2+ and H3BTC ligands dissolved and diffused to the surface in the process of crystallization, resulting in rough surface of Ni-based MOF precursors.15,22,86–88 Liu et al. successfully prepared waxberry-like Ni@C core–shell microspheres with hierarchical architecture.15 The composites obtained after pyrolysis at 700 °C showed optimum absorbing performance, including the RL value of −73.2 dB and the EAB of 4.8 GHz with only 1.8 mm applied thickness (Fig. 5). Similarly, the hollow Ni-MOF spheres were synthesized with different surface morphology by tuning the time of hydrothermal reaction, as shown in Fig. 5.88 And it was found that the samples with 8 h reaction time could reach a broad EAB of about 6.8 GHz with only 1.8 mm thickness. Moreover, rod-like,86 flower-like,89 accordion-like90 and other sphere-like Ni/C composites87 derived from Ni-based MOF also exhibited strong microwave absorption and attenuation performance, and these results are listed in Table 2.
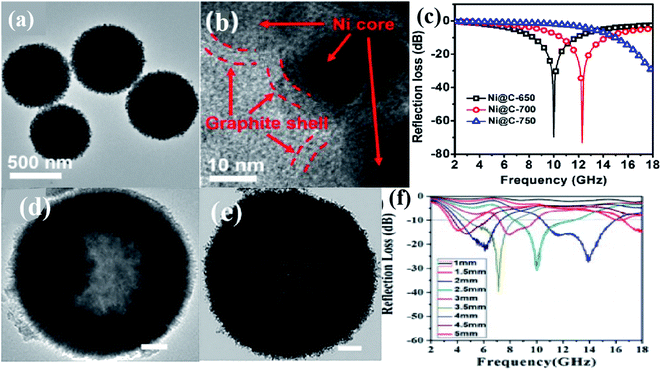 |
| Fig. 5 SEM (a), TEM (b) images and RL (c) of waxberry-like hierarchical Ni/C (annealing at 650 °C), TEM images of Ni-MOF precursors with 8 h (e), Ni/C annealed at 600 °C (d) and RL of Ni/C-8 h (f). This figure has been reproduced from ref. 15 and 88 with permission from Journal of Materials Chemistry C, Copyright 2019 and ACS Applied Nano Materials, Copyright 2019. | |
Table 2 The microwave absorbing performance of mono-metal/PC materials
Absorbers |
Loading (wt%) |
RLmin |
EAB |
Ref. |
Thickness (mm) |
Value (dB) |
Thickness (mm) |
Value (GHz) |
Fe/carbon |
15 |
2.5 |
−29.5 |
2.5 |
4.3 |
48 |
Fe3O4@PB |
60 |
1.55 |
−48.04 |
2.6 |
4.5 |
58 |
Fe/carbon |
40 |
2.0 |
−20.3 |
2.0 |
7.2 |
83 |
Fe/C@Co3O4 |
50 |
1.5 |
−38.1 |
2.5 |
7.65 |
98 |
Fe3O4–C/RGO |
15 |
3.6 |
−60.5 |
1.5 |
5.5 |
98 |
Fe/NPC |
20 |
1.8 |
∼−28 |
1.8 |
5.6 |
99 |
Ni@C |
33 |
3.5 |
−45.8 |
3.5 |
5.1 |
22 |
Ni@C |
40 |
2.7 |
−86.8 |
2.7 |
7.4 |
22 |
Ni/C rod |
40 |
2.6 |
−51.8 |
2.6 |
3.48 |
86 |
Ni@C@ZnO |
25 |
2.5 |
−55.8 |
2.0 |
4.1 |
87 |
Ni/C-8 h |
50 |
— |
— |
1.8 |
6.8 |
88 |
Ni@C |
25 |
1.8 |
−86.9 |
1.8 |
6.6 |
90 |
Ni@C |
30 |
1.85 |
−57 |
1.85 |
6.0 |
100 |
Ni/C nanosheets |
10 |
2.2 |
−71.6 |
2.2 |
4.7 |
101 |
Co/C |
40 |
4.0 |
−35.3 |
2.5 |
5.8 |
17 |
Co/C |
40 |
2.8 |
−41 |
2.0 |
5.6 |
46 |
Rod-like Co/C |
22 |
2.0 |
−47.6 |
2.0 |
5.11 |
92 |
Co/C |
60 |
2.0 |
−26.4 |
2.0 |
6.6 |
93 |
Co@NCNT |
25 |
1.8 |
−53 |
2.0 |
6.2 |
95 |
Co@NPC@TiO2 |
50 |
1.5 |
−31.7 |
1.0–5.0 |
13 |
96 |
Co/C |
25 |
3.0 |
−30.31 |
3.0 |
4.93 |
102 |
Co/N-doped C |
20 |
2.5 |
−65.1 |
3.2 |
9.4 |
103 |
Co/NC@MnO2 |
15 |
3.7 |
−58.9 |
3.7 |
5.56 |
11 |
Co/C@ZnO@GO |
30 |
2.0 |
−45.4 |
2.0 |
5.4 |
97 |
Co@C@RGO |
20 |
2.6 |
−67.5 |
2.0 |
5.4 |
104 |
Co@C@NRGO |
20 |
2.0 |
−73.4 |
2.0 |
5.3 |
105 |
Co–C-MWCNTs |
17.5 |
0.9 |
−25.27 |
1.6 |
2.38 |
106 |
Co/C@V2O3 |
50 |
1.5 |
−40.1 |
1.5 |
4.64 |
107 |
CNT/Co/C |
10 |
2.9 |
−53.3 |
1.6 |
8.02 |
108 |
Mo2C/Co@C |
35 |
1.7 |
−47.98 |
1.6 |
6.0 |
109 |
ZIF materials are designed through the expansion of the zeolite topology with larger pores and higher porosity.91 ZIF-67 and ZIF-8, with the same microporous structure and organic ligands, are formed by bridging the 2-methylimidazolate anions and metal cations (Co2+ and Zn2+), respectively. Due to high porosity, easy preparation and large apertures, ZIF-67 is widely applied as precursor of Co-based highly PC matrix absorbers in the recent years. Lu et al. fabricated porous Co/C composites by the directly decomposing ZIF-67 at different temperatures (500 °C, 600 °C and 800 °C).17 Co/C composites obtained at 500 °C exhibited best absorbing performance with the minimum RL of −35.3 dB, EAB of 5.80 GHz and the absorbing thickness of 2.5 mm. Chen et al. designed and synthesized rod-like Co/C composites derived from cubic Co-based MOF via hydrothermal and calcination processes.92 The prepared composites exhibited optimized absorbing ability with the minimum RL of −47.6 dB with 2.0 mm. In addition, Co-based MOF can also be synthesized with different organic ligands (glucose, 2,5-dihydroxyterephthalic acid and so on) in a more convenient and environment-friendly way.93,94 Co@NC composites with multi-dimensional shapes (sheet-, flower-, cube-, dodecahedron- and octahedron-like) from ZIF-67 precursors were fabricated via regulating the anion/linker ratio and the solvent.95 The observed results provide the way to fabricate high-absorbing MAM with controlled morphology. Other similar Co-based MOF derived PC composite materials are listed at Table 2.
Core–shell structure is a significant research direction for MOF-derived microwave absorbers. For example, Ji et al. developed novel multi-interfaced yolk–shell Co@NPC@TiO2.96 As a typical semiconductor, TiO2 is widely applied as a supplemental material to optimize impedance matching owing to its strong dielectric loss. In addition, the porous core–shell Co/C@ZnO-decorated rGO was synthesized via a facile method, displaying the exceptional microwave absorbency (Fig. 6).97
 |
| Fig. 6 The scheme for the synthesis of Co/NPC@ZnO/rGO (a), SEM images of ZIF-67 (b) and Co/NPC@ZnO/rGO (d), and the RL of Co/NPC@ZnO/rGO (c). This figure has been adapted from ref. 97 with permission from Journal of alloys and compound, Copyright 2020. | |
The MOF-derived PC materials are not only applied as the central core, but also the lightweight carbon shell of the composites. Recently, Liu et al. prepared two different types of ZIF to synthesize nano core–shell carbon cages, made up of N-doped carbon inner shell from ZIF-8 and Co/N-doped carbon outer shell from ZIF-67.78 Derived core–shell composites exhibited hierarchical porous, high specific surface area and thin thickness, which induced excellent microwave attenuating performance. The strong RL reached −52.5 dB with 25 wt% filler loading of composites, and the EAB of 4.4 GHz with the thickness of 2.2 mm. The results provided a new strategy of carbon nanocages fabrication with tunable chemical composition and the impedance matching. Besides the examples discussed above, other mono-metal/PC composites with excellent microwave absorbing performance are tabulated in following Table 2.
4.3 Multi-metal/PC
The MOF-derived multi-metal/PC composites (with two or more metals embedded in the derived carbon matrix) often show the better absorption performance than mono-metal/PC, due to the multi-metal/PC composites artfully integrating the advantages of multi metals and carbon. Effective doping of other metallic components to compose functional multi-metal MOF is served as a prospective approach. The compositions and the structures can be optimized to achieve efficient microwave attenuation like improving Debye dipole polarization and multiple interface loss.110,111 The optimizing absorption properties are due to the synergetic effects of the high porous structure and the multiple components. This expansion method not only provides interesting references for further studying MOF derived carbon-based lightweight MAM, but also broadens the application of MOF materials.
Nowadays, FeCo particles and their composites derived from MOF have been widely reported as microwave absorbers. For example, Ji et al. developed carbon composite with FeCo bimetal nanoparticles embedded via pyrolysis process of ZIF-67.14 The composites showed a strong RL of −21.7 dB with the thin thickness of only 1.2 mm and a broad EAB of 5.8 GHz (covering from 12.2 GHz to 18 GHz). The saturation magnetization gradually increased with the augment of Fe element content. Compared with pure Co/NPC derived from ZIF-67, Fe–Co/NPC exhibited effectively microwave absorption properties, as showed in Fig. 7.
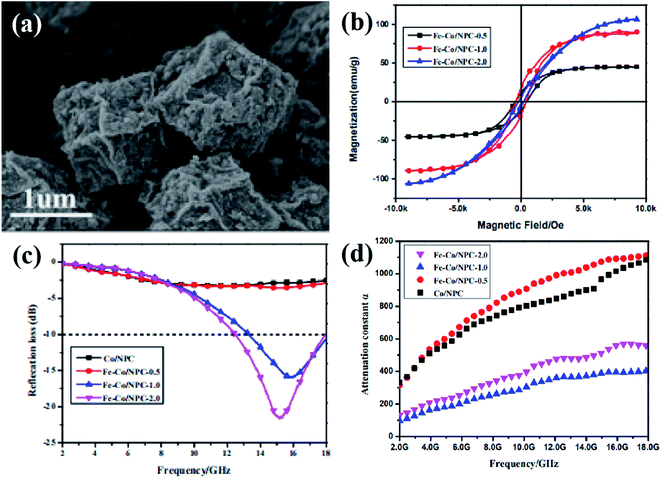 |
| Fig. 7 SEM image of Fe–Co/NPC (a), field-dependent magnetization curve of Fe–Co/NPC (b), the RL (c) and attenuation constant (d) of the Co/NPC, Fe–Co/NPC. This figure has been adapted from ref. 14 with permission from Nanoscale, Copyright 2015. | |
Besides, hollow sphere-like FeCoNi@C absorbers were obtained via high-temperature carbonization, which used trimetallic FeCoNi-MOF as the precursor, and more excellent absorption properties are obtained compared with the carbonized mono-metal MOF or bi-metal MOF.112 Doping with metallic elements can also be achieved through opposite direction, which illustrates the replaceability of similar valence transition metals. Dielectric loss material manganese dioxide (MnO2) with flower nanostructures was assembled on the derived CoFe@C nanocube via a hydrothermal reaction, giving rise to the improvement of the impedance matching.113 The Co element was added into the PB nanocube and reduced to Co magnetic metallic nanoparticles at the high annealing temperature. The CoFe@C@MnO2 achieved a minimum RL of −64 dB and a maximum EAB of 9.2 GHz from 8.8 GHz to 18 GHz with a thickness of 1.3 mm and 1.6 mm, respectively. Yang et al. designed NiFe@C nanocubes derived from NiFe PB on the GO nanosheets, having a minimum RL of −51 dB at a thin thickness of 2.8 mm.114
Sometimes the doping elements can not only enrich the composition, but also result in a novel structure. Lu and his group designed the MOF derived nanocomposites NiCo/GC/NPC with hierarchical double yolk–shell structure, which were synthesized by regulating Co elements doped into Ni-based MOF.71 The emergence of yolk–shell could be ascribed to the heterogeneous decomposition induced by Co and Ni component, which not only decreases the density of composites, but also facilitates the multiple interfacial polarization (Fig. 8). Similarly, the additional metal Ni was introduced into the derived porous Co/C composites to obtain hollow structure, which yield the RL of −61.02 dB and the EAB of 5.2 GHz.115 Similarly, Che et al. successfully synthesized porous Ni1−xCox@C composites derived from MOF with different Ni, Co millimole content.116 The generated carbon/alloy particles acted as catalysis to encourage the carbon sp2 arrangement through the thermal decomposition, forming special micro architecture. Other MOF-derived multi-metal/PC composites mentioned above are tabulated in Table 3.
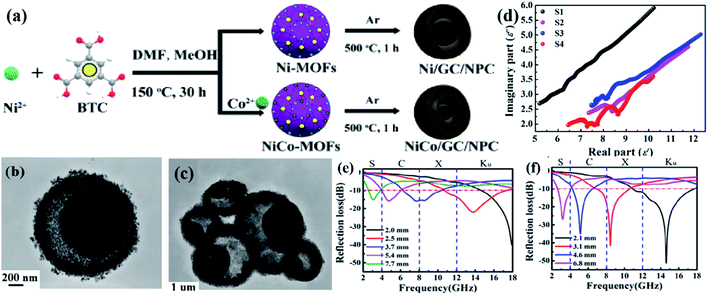 |
| Fig. 8 The schematic formation process of the NiCo/GC/NPC composites (a), TEM images of S1 (b), S4 (c), the Cole–Cole semicircles of S1–S4 (d), the RL with various thicknesses for S1 (e), S4 (f) (S1 represents Ni/GC/NPC, and the content of Co increases from S1 to S4). This figure has been reproduced from ref. 71 with permission from Applied Surface Science, Copyright 2020. | |
Table 3 The microwave absorbing performance of multi-metal/PC materials
Absorbers |
Loading (wt%) |
RLmin |
EAB |
Ref. |
Thickness (mm) |
Value (dB) |
Thickness (mm) |
Value (GHz) |
Fe–Co/NPC |
50 |
1.2 |
−21.7 |
1.2 |
5.8 |
14 |
CoFe@C@MnO2 |
50 |
1.3 |
−64 |
1.6 |
9.2 |
113 |
CoFe@C |
10 |
2.8 |
−61.8 |
2.8 |
9.2 |
117 |
Solid Fe/Co/C |
33 |
2.0 |
−54.6 |
2.5 |
8.8 |
118 |
FeCoNi@C |
38 |
2.1 |
−64.75 |
2.47 |
8.08 |
112 |
NiFe/C@GO |
40 |
3.0 |
−65.5 |
3.0 |
4.5 |
114 |
CoZn/C/graphene |
6 |
1.5 |
−47.31 |
2.2 |
4.01 |
2 |
CoZn/C |
40 |
2.5 |
−45.2 |
2.5 |
5.7 |
41 |
CoZn/NPC |
30 |
2.0 |
−49.0 |
2.0 |
5.3 |
119 |
CoNi/C-650 |
30 |
1.8 |
−74.7 |
1.8 |
15.1 |
24 |
Air@NC/Ni–Co |
25 |
2.2 |
−36.5 |
2.3 |
6.55 |
70 |
NiCo/GC/NPC |
30 |
2.1 |
−52.2 |
2.1 |
7.2 |
71 |
CoNi/C |
10 |
2.0 |
−61.02 |
2.0 |
5.2 |
115 |
Ni0.8Co0.2@C |
25 |
3.5 |
−39.3 |
2.0 |
4.8 |
116 |
G/CoNi@NCNTA |
10 |
2.0 |
−44.23 |
1.6 |
4.63 |
120 |
CoNi@NG-NCPs |
35 |
3.0 |
−45.73 |
2.5 |
4.32 |
121 |
MoW-NC |
30 |
2.8 |
−55.6 |
2.8 |
8.8 |
122 |
CoMo@NC |
30 |
2.5 |
−44.8 |
2.5 |
6.56 |
123 |
4.4 Metal oxides/PC
If the obtained derived PC is formed by metal oxides and reduced carbon, it can be categorized as MOF-derived metal oxides/PC composites. The saturation magnetization and the compatible dielectric loss of metal oxides are lower than those of magnetic metal nanoparticles (e.g. Fe, Co, Ni and related alloys).83 However, they have the great chemical stability, characteristic magnetic property and facile manipulation. Some metal oxides like Fe3O4, have been often served as magnetic promoters for the enhancement of microwave absorbing, and other non-magnetic metallic oxide (ZnO, Co3O4) applied to optimize impedance matching. In addition, it is reported that MOF-derived metal or metal oxide particles carbon composites are mainly based on the reduction potential of metal ions at the pyrolysis process. The metal oxides nanoparticles tend to form when the reduction potential of metal ions is lower than −0.27 V. On the contrary, the metal nanoparticles tend to form at a reduction potential higher than −0.27 V.124
Fe3O4 is one of the widely accepted magnetic metal oxide microwave absorbers because of its low cost, chemical stability and high absorption performances. And it exhibits optimized impedance matching after being composed with other materials. Xiang et al. obtained magnetic Fe3O4@NPC composites through two thermolysis processes with increasing saturation magnetization.51 The sample exhibited excellent absorbing properties, including a strong RL of −65.5 dB as well as a broad EAB of 4.5 GHz with a matching thickness of 3.0 mm. Similarly, Shu et al. compounded MOF-derived Fe3O4@C and rGO by solvothermal and pyrolysis.98 The obtained Fe3O4–C/RGO displayed the minimum RL of −60.5 dB with a thickness of 3.6 mm and the EAB of 5.5 GHz with an ultrathin thickness of merely 1.5 mm. Moreover, derived metal oxides can translate into stronger dielectric loss absorbers as the raw materials. Currently, metal sulfides such as MoS2, CdS and CuS develop fast owing to their remarkable RL value. Based on this, Man et al. reported a yolk–shell FeS2@C nanocomposite for microwave absorption.16 The MIL nano-spindles were firstly synthesized and then converted to Fe2O3@C after annealing in N2 atmosphere. Finally, the FeS2@C was obtained via a sulfidation process. The as-fabricated composites showed excellent absorbing properties having the RL of −45 dB with the matching thickness of 1.45 mm, and the broad bandwidth of 15.4 GHz, as shown in Fig. 9.
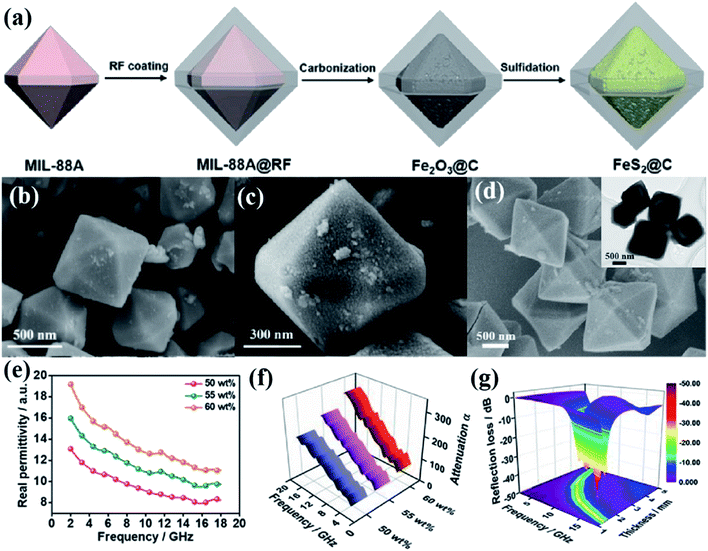 |
| Fig. 9 The schematic formation process of FeS2/C composites (a), SEM images of Fe-MOF (b), Fe3O4/C (c), SEM and TEM images of FeS2/C (d), the real permittivity (e), attenuation constant (f) and the RL of FeS2/C composites (g). This figure has been reproduced from ref. 16 with permission from Nano Letters, Copyright 2020. | |
It is accepted that nonmagnetic metal oxides can be used to tune impedance matching. For example, Liang et al. designed a hetero-structured ZnO/NPC by using Zn-based MOF ZIF-8 as precursor materials, and the minimum RL achieved −25 dB with merely 1.55 mm of absorber thickness.125 Similarly, Ji et al. compounded the rGO and ZnO/NPC composites through the simple hydrothermal method.126 Thereinto, the ZnO/NPC were obtained via high-temperature carbonization at 700 °C under N2 flow. The minimum RL of ZnO/NPC/RGO could reach −50.5 dB with a thin thickness of 2.4 mm. Ji and his group synthesized Co3O4 nanoparticles embedded in the carbon matrix, and the Co3O4 nanoparticles grow on the carbon paper, which exhibited the excellent absorbing properties. The samples showed RL value of −41.38 dB at 7.32 GHz with the absorbing thickness of 2.3 mm.52 Besides, Bai et al. fabricated stratiform Co3O4/N-doped carbon using a Co-based MOF as the precursor.127 The optimal RL of the samples reached −42.63 dB with the thickness of only 1.5 mm. Besides the above metal oxides/PC composites, some similar composites and other certain elements derived materials like ZrO2/C,128 TiO2/C129 and CoO/Co/C130 are listed in Table 4.
Table 4 The microwave absorbing performance of metal oxides/PC materials
Absorbers |
Loading (wt%) |
RLmin |
EAB |
Ref. |
Thickness (mm) |
Value (dB) |
Thickness (mm) |
Value (GHz) |
FeS2@C |
55 |
1.45 |
−45 |
1.2–5.0 |
15.4 |
16 |
Fe3O4@NPC |
40 |
3.0 |
−65.5 |
3.0 |
9.8 |
51 |
Fe3O4–C/RGO |
15 |
3.6 |
−60.5 |
1.5 |
5.5 |
98 |
Fe3O4/RGO |
5 |
2.2 |
−67.1 |
1.8 |
5.24 |
131 |
γ-Fe2O3/CrGO |
50 |
3.25 |
−43.13 |
3.6 |
11.68 |
132 |
Fe3O4@NC@rGO |
25 |
2.0 |
−72.6 |
2.0 |
5.5 |
133 |
ZnO/NPC |
40 |
1.55 |
−25 |
— |
— |
125 |
ZnO/NPC/RGO |
40 |
2.4 |
−50.5 |
2.6 |
7.4 |
126 |
NC-Co3O4/CP |
40 |
1.6 |
−41.27 |
— |
— |
52 |
Co3O4/N–C |
40 |
1.5 |
−42.63 |
1.5 |
4.14 |
127 |
ZrO2/C |
50 |
1.5 |
−58.7 |
— |
— |
128 |
TiO2/C |
60 |
1.6 |
−49.6 |
1.6 |
4.6 |
129 |
CoO/Co/C |
25 |
3.3 |
−66.7 |
5.1 |
1.8 |
130 |
Co3O4@C@α-Fe2O3 |
20 |
3.7 |
−52.2 |
2.5 |
6.6 |
134 |
CoO/Co@C |
70 |
1.5 |
−38.46 |
2.0 |
4.8 |
135 |
SnO2/Co3Sn2@C |
30 |
2.0 |
−46.8 |
2.5 |
4.8 |
136 |
4.5 Other MOF-derived PC
In addition to those mentioned above, there are some PC composites with other composition, such as rare-earth metal, bimetal oxides and so on. Up to now, the rare-earth metal organic frameworks (RE-MOFs) for microwave absorption are barely reported. However, RE-MOFs are one of the attractive members of MOFs family in the recent years due to the unique 4f electron layer of RE metal ions. And the highly connected structures of RE-MOFs would induce ultra-high surface areas, resulting in excellent absorbing performance.137 Zhu et al. synthesized four RE-MOFs by hydrothermal reactions for microwave absorption with synergetic and complementary of permittivity and permeability.138
Metal and metal oxides encapsulated in MOF-derived PC are generally received more attention. And multiple component composites are significant candidates with high property as microwave absorbers. Xu et al. synthesized flower-like heterogeneous Co/MnO@C, deriving from Co/Mn bimetal oxides MOF derivatives.139 When the molar ratio of Co and MnO reached 2
:
1, composites exhibited an optimized RL of −55.3 dB with an absorber thickness of 2.4 mm and the EAB of 4.6 GHz from 7.4 GHz to 12.0 GHz. Moreover, necklace-like CNFs@Co/CoO composites derived from ZIF-67 penetrated throughout the carbon nanofibers were synthesized by simple carbonization process (Fig. 10).140 Attributed to the unique conductive network and the synergistic effect of dielectric loss, magnetic loss and impedance matching, the incident microwaves would be sufficiently absorbed after the multiple reflections, scattering and attenuation. The more similar composites are tabulated in Table 5.
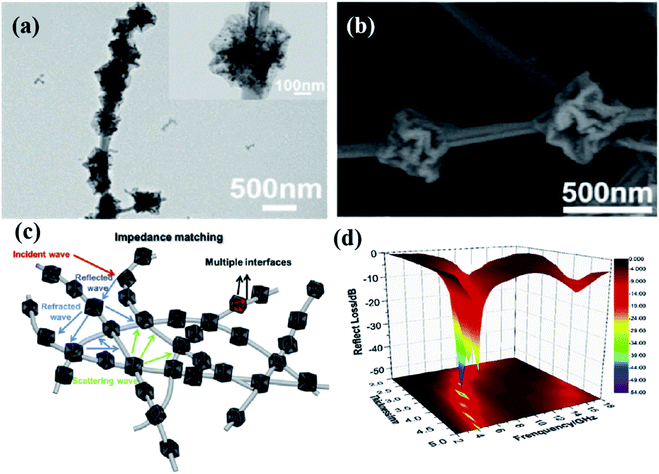 |
| Fig. 10 TEM (a) and SEM (b) images, schematic diagram of microwave absorption mechanisms (c) and the RL (d) of CNFs@Co/CoO composites. This figure has been reproduced from ref. 140 with permission from Journal of Alloys and Compound, Copyright 2018. | |
Table 5 The microwave absorbing performance of other MOF-derived PC materials
Absorbers |
Loading (wt%) |
RLmin |
EAB |
Ref. |
Thickness (mm) |
Value (dB) |
Thickness (mm) |
Value (GHz) |
[Er2(MH)6]n |
20 |
5 |
−22.78 |
5 |
2.24 |
138 |
[Yb2(MH)6]n |
20 |
4.5 |
−19.99 |
4.5 |
2.12 |
138 |
Co/MnO@C |
25 |
2.4 |
−55.3 |
2.4 |
4.6 |
139 |
CNFs@Co/CoO |
20 |
3.54 |
−53.1 |
2.0–5.0 |
13.52 |
140 |
C/CoNiO2 |
40 |
2.5 |
−53 |
— |
— |
141 |
C@NC/CoS2 |
30 |
2.8 |
−59.6 |
2.8 |
7.2 |
142 |
NiSe/C |
50 |
3.7 |
−59.70 |
2.1 |
4.67 |
143 |
Co/ZnO/C |
30 |
3.0 |
−52.6 |
2.5 |
5.8 |
144 |
Fe/Fe2O3 |
30 |
2.2 |
−70.2 |
2.2 |
5.2 |
145 |
Fe2N@NC |
50 |
1.55 |
−59.3 |
1.9 |
4.32 |
146 |
NiO/Ni/C@Air@NiO/Ni/C |
50 |
1.7 |
−34.5 |
1.7 |
∼5.5 |
147 |
ZnO/C@Co/C |
50 |
1.9 |
−28.8 |
1.9 |
4.2 |
148 |
Bimetal oxides are explored to act as microwave absorbers. Bi et al. found the unique polaron excitations-enhanced absorbing properties via synthesizing PC with CoNiO2 through ZIF-67. This properties effectively improved electron polarization, multiple internal reflections and high RL.141 In addition, metal sulfides and selenides can be homogeneously dispersed in the derived PC under the appropriate reaction conditions. For example, the CoS2 was obtained after the carbonization and sulphidation process of Co-MOF precursors.142 Mao et al. successfully synthesized rod-like NiSe/C composites via the in situ selenization of Ni-MOFs with excellent microwave absorption properties.143 The results and the performance of other MOF-derived PC materials are displayed in the Table 5.
5. Summary and conclusions
In the past few decades, MOF-derived PC composites have been increasingly reported for microwave absorption. And the diverse and unique chemical structures (light-weight, specific surface area, chemical stability and high porosity), tunable properties (electronic conductivity, impedance matching) and facile preparation methods make derived PC composites be sought-after. The varied microstructures and compositions of derived PC are ascribed to the special nature of MOF, and the porosity can be adjusted via controlling the carbonization temperatures. These indicate that MOF-derived PC composites are admirable candidates of microwave absorbers.
The synergistic effect between the dielectric loss and the magnetic loss contributes to the efficient and strong attenuation capability, which can be tuned by well designing the constitution of derived PC materials and other microwave absorbers and adjusting the proportion of effective constituent. Up to now, the MOF-derived PC composited magnetic metals or metal oxides and nano-PC are growing fast, which can overcome the problem of nano-scale particles agglomeration and protect the metal particles from external corrosion. The present of derived carbon skeleton with rich pores establishes the conductive path for electron migrating and hopping, and enhances the interfacial polarization and multiple reflections.
However, there are still some challenges for MOF-derived PC materials to face. For example, the toxic solvents and harsh fabrication condition are generally required in the fabrication process of MOF materials. It is highly desirable to design an environment friendly and facile fabrication strategy to construct MOF materials. The compositions of current absorbers of MOF-derived carbon materials are mainly large density metal like Fe, Co and Ni, which have been studied comprehensively in the previous research. However, the chemical composition is much dependent on the corresponding MOF precursors to be tuned, besides, the heavy metal composites often lead to the high filler loading. Thus, it is significant to find novel composite, diverse and lightweight MOF-based PC composites to achieve preferable microwave absorption. In addition, the research progress is almost theoretical stage. The practical applications as microwave absorbers are rarely reported due to the challenge of mass production. Based on this, MOF-derived PC materials are expected to be one of admirable candidates with efficient microwave absorption performance and facile preparation process. With the widespread attentions, MOF-derived PC materials would increasingly promote the advancement of microwave absorption field.
Author contributions
Ma Mingliang: conceptualization, resources, writing-reviewing and editing Bi Yuxin: conceptualization, investigation, writing-original draft preparation, writing-review and editing Tong Zhouyu: writing-reviewing and investigation Liu Yanyan: editing Lyu Ping: supervision Wang Rongzhen: investigation Ma Yong: writing-review and editing Wu Guanglei: editing Liao Zijian: investigation Chen Yan: investigation.
Conflicts of interest
There are no conflicts to declare.
Acknowledgements
This work is financially supported by the National Natural Science Foundation of China (Grant No. 51503116).
References
- X. X. Sun, M. L. Yang, S. Yang, S. S. Wang, W. L. Yin, R. C. Che and Y. B. Li, Ultrabroad band microwave absorption of carbonized waxberry with hierarchical structure, Small, 2019, 15, 1902974 CrossRef CAS PubMed.
- X. Zhang, J. Xu, X. Y. Liu, S. Zhang, H. R. Yuan, C. L. Zhu, X. T. Zhang and Y. J. Chen, Metal organic framework-derived three-dimensional graphene-supported nitrogen-doped carbon nanotube spheres for electromagnetic wave absorption with ultralow filler mass loading, Carbon, 2019, 155, 233–242 CrossRef CAS.
- J. Yan, Y. Huang, X. P. Han, X. G. Gao and P. B. Liu, Metal organic framework (ZIF-67)-derived hollow CoS2/N-doped carbon nanotube composites for extraordinary electromagnetic wave absorption, Composites, Part B, 2019, 163, 67–76 CrossRef CAS.
- X. R. Li, Z. Y. Le, X. L. Chen, Z. Q. Li, W. C. Wang, X. Y. Liu, A. Wu, P. C. Xu and D. Q. Zhang, Graphene oxide enhanced amine-functionalized titanium metal organic framework for visible-light-driven photocatalytic oxidation of gaseous pollutants, Appl. Catal., B, 2018, 236, 501–508 CrossRef CAS.
- C. S. Shan, X. Feng, J. X. Yang, X. Yang, H. Y. Guan, M. Argueta, X. L. Wu, D. S. Liu, D. J. Austin, P. Nie and Y. F. Yue, Hierarchical porous carbon pellicles: electrospinning synthesis and applications as anodes for sodium-ion batteries with an outstanding performance, Carbon, 2020, 157, 308–315 CrossRef CAS.
- X. Wang, Z. Zhu, L. Chai, J. Ding, L. Zhong, A. Dong, T. T. Li, Y. Hu, J. Qian and S. Huang, Generally transform 3-dimensional In-based metal–organic frameworks into 2-dimensional Co,N-doped carbon nanosheets for Zn–air battery, J. Power Sources, 2019, 440, 227158 CrossRef CAS.
- L. Zhang, Z. Su, F. Jiang, L. Yang, J. Qian, Y. Zhou, W. Li and M. Hong, Highly graphitized nitrogen-doped porous carbon nanopolyhedra derived from ZIF-8 nanocrystals as efficient electrocatalysts for oxygen reduction reactions, Nanoscale, 2014, 6, 6590–6602 RSC.
- Q. Huang, Y. Guo, X. Wang, L. Chai, J. Ding, L. Zhong, T. T. Li, Y. Hu, J. Qian and S. Huang, In-MOF-derived ultrathin heteroatom-doped carbon nanosheets for improving oxygen reduction, Nanoscale, 2020, 12, 10019–10025 RSC.
- Y. Xu, L. Xu, Q. Li, K. Su, Y. Hu, T. Miao and J. Qian, Metal–Organic Framework-Impregnated Calixarene-Based Cluster-Derived Hierarchically Porous Bimetallic Phosphide Nanocomposites for Efficient Water Splitting, Energy Technol., 2020, 8, 2000059 CrossRef CAS.
- L. Chai, Z. Hu, X. Wang, Y. Xu, L. Zhang, T. T. Li, Y. Hu, J. Qian and S. Huang, Stringing Bimetallic Metal–Organic Framework-Derived Cobalt Phosphide Composite for High-Efficiency Overall Water Splitting, Adv. Sci., 2020, 7, 1903195 CrossRef CAS PubMed.
- R. Wang, M. He, Y. Zhou, S. Nie, Y. Wang, W. Liu, Q. He, W. Wu, X. Bu and X. Yang, Metal–organic frameworks self-templated cubic hollow Co/N/C@MnO2 composites for electromagnetic wave absorption, Carbon, 2020, 156, 378–388 CrossRef CAS.
- J. C. Shu, X. Y. Yang, X. R. Zhang, X. Y. Huang, M. S. Cao, L. Li, H. J. Yang and W. Q. Cao, Tailoring MOF-based materials to tune electromagnetic property for great microwave absorbers and devices, Carbon, 2020, 162, 157–171 CrossRef CAS.
- X. Sun, J. Z. He, Z. M. Song, J. J. Pan, Y. P. He, J. P. He, R. H. Yu and X. F. Liu, Sodium phosphinate-assisted synthesis of P-doped FeCo microcubes and their electromagnetic scattering characteristics, J. Alloys Compd., 2020, 820, 153280 CrossRef CAS.
- X. M. Zhang, G. B. Ji, W. Liu, B. Quan, X. H. Liang, C. M. Shang, Y. Cheng and Y. W. Du, Thermal conversion of Fe3O4@metal organic framework: a new method for efficient Fe–Co/nanoporous carbon microwave absorbing material, Nanoscale, 2015, 7, 12932–12942 RSC.
- D. W. Liu, Y. C. Du, P. Xu, N. Liu, Y. H. Wang, H. H. Zhao, L. R. Cui and X. J. Han, Waxberry-like hierarchical Ni@C microspheres with high performance microwave absorption, J. Mater. Chem. C, 2019, 7, 5037–5046 RSC.
- Z. Man, P. Li, D. Zhou, Y. Wang, X. Liang, R. Zang, P. Li, Y. Zuo, Y. M. Lam and G. Wang, Two birds with one stone: FeS2@C yolk–shell composite for high-performance sodium-ion energy storage and electromagnetic wave absorption, Nano Lett., 2020, 20, 3769–3777 CrossRef CAS PubMed.
- Y. Y. Lu, Y. T. Wang, H. L. Li, Y. Lin, Z. Y. Jiang, Z. X. Xie, Q. Kuang and L. S. Zheng, MOF-Derived porous Co/C nanocomposites with excellent electromagnetic wave absorption properties, ACS Appl. Mater. Interfaces, 2015, 7, 13604–13611 CrossRef CAS PubMed.
- P. Miao, R. Zhou, K. J. Chen, J. Liang, Q. F. Ban and J. Kong, Tunable electromagnetic wave absorption of supramolecular isomer-derived nanocomposites with different morphology, Adv. Mater. Interfaces, 2020, 7, 1901820 CrossRef CAS.
- M. Ramezanzadeh, B. Ramezanzadeh, M. Mahdavian and G. Bahlakeh, Development of metal–organic framework (MOF) decorated graphene oxide nanoplatforms for anti-corrosion epoxy coatings, Carbon, 2020, 161, 231–251 CrossRef CAS.
- Y. Mu, D. Wang, X. D. Meng, J. Pan, S. D. Han and Z. Z. Xue, Construction of Iodoargentates with Diverse Architectures: Template Syntheses, Structures, and Photocatalytic Properties, Cryst. Growth Des., 2020, 20, 1130–1138 CrossRef CAS.
- M. Safaei, M. M. Foroughi, N. Ebrahimpoor, S. Jahani, A. Omidi and M. Khatami, A review on metal–organic frameworks: synthesis and applications, TrAC, Trends Anal. Chem., 2019, 118, 401–425 CrossRef CAS.
- J. Yan, Y. Huang, Y. H. Yan, L. Ding and P. B. Liu, High-performance electromagnetic wave absorbers based on two kinds of nickel-based MOF-derived Ni@C microspheres, ACS Appl. Mater. Interfaces, 2019, 11, 40781–40792 CrossRef CAS PubMed.
- R. L. Wang, M. He, Y. M. Zhou, S. X. Nie, Y. J. Wang, W. Q. Liu, Q. He, W. T. Wu, X. H. Bu and X. M. Yang, Metal–organic frameworks self-templated cubic hollow Co/N/C@MnO2 composites for electromagnetic wave absorption, Carbon, 2020, 156, 378–388 CrossRef CAS.
- C. Liu, J. Qiao, X. Zhang, D. M. Xu, N. N. Wu, L. F. Lv, W. Liu and J. R. Liu, Bimetallic MOF-derived porous CoNi/C nanocomposites with ultra-wide band microwave absorption properties, New J. Chem., 2019, 43, 16546–16554 RSC.
- W. Liu, S. J. Tan, Z. H. Yang and G. B. Ji, Enhanced low-frequency electromagnetic properties of MOF-derived cobalt through interface design, ACS Appl. Mater. Interfaces, 2018, 10, 31610–31622 CrossRef CAS PubMed.
- M. M. Xu, Q. Chen, L. H. Xie and J. R. Li, Exchange reactions in metal–organic frameworks: new advances, Coord. Chem. Rev., 2020, 421, 213421 CrossRef CAS.
- M. H. Yap, K. L. Fow and G. Z. Chen, Synthesis and applications of MOF-derived porous nanostructures, Green Energy Environ., 2017, 2, 218–245 CrossRef.
- X. Wang, A. R. Dong, Y. Hu, J. J. Qian and S. M. Huang, A review of recent work on using metal–organic frameworks to grow carbon nanotubes, Chem. Commun., 2020, 56, 10809–10823 RSC.
- X. F. Yu, Y. Zhan, L. Wang, L. S. Xing, W. B. You, J. W. Liu, G. Y. Chen, G. Z. Ding, J. J. Ding, X. H. Liu, M. Wang and R. C. Che, Improved microwave absorption performance of a multi-dimensional Fe2O3/CNTCM@CN assembly achieved by enhanced dielectric relaxation, J. Mater. Chem. C, 2020, 8, 5715–5726 RSC.
- X. F. Xu, S. H. Shi, G. P. Wan, C. C. Hao, Z. Y. He and G. Z. Wang, Uniformly coating MnOx nanoflakes onto carbon nanofibers as lightweight and wideband microwave absorbers with frequency-selective absorption, Mater. Des., 2019, 183, 108167 CrossRef CAS.
- P. Liu, Y. Zhang, J. Yan, Y. Huang, L. Xia and Z. Guang, Synthesis of lightweight N-doped graphene foams with open reticular structure for high-efficiency electromagnetic wave absorption, Chem. Eng. J., 2019, 368, 285–298 CrossRef CAS.
- X. H. Liang, B. Quan, B. W. Sun, Z. M. Man, X. Xu and G. B. Ji, Extended effective frequency of three-dimensional graphene with sustainable energy attenuation, ACS Sustainable Chem. Eng., 2019, 7, 10477–10483 CrossRef CAS.
- Y. Cheng, Z. Y. Li, Y. Li, S. S. Dai, G. B. Ji, H. Q. Zhao, J. M. Cao and Y. W. Du, Rationally regulating complex dielectric parameters of mesoporous carbon hollow spheres to carry out efficient microwave absorption, Carbon, 2018, 127, 643–652 CrossRef CAS.
- J. Ma, X. Zhang, W. Liu and G. Ji, Direct synthesis of MOF-derived nanoporous CuO/carbon composites for high impedance matching and advanced microwave absorption, J. Mater. Chem. C, 2016, 4, 11419–11426 RSC.
- J. Wang, Y. L. Wang, H. B. Hu, Q. P. Yang and J. J. Cai, From metal–organic frameworks to porous carbon materials: recent progress and prospects from energy and environmental perspectives, Nanoscale, 2020, 12, 4238–4268 RSC.
- J. B. Cheng, H. G. Shi, M. Cao, T. Wang, H. B. Zhao and Y. Z. Wang, Porous carbon materials for microwave absorption, Mater. Adv., 2020, 1, 2631–2645 RSC.
- K. Zhang, F. Wu, A. Xie, M. X. Sun and W. Dong, In situ stringing of metal organic frameworks by SiC nanowires for high-performance electromagnetic radiation elimination, ACS Appl. Mater. Interfaces, 2017, 9, 33041–33048 CrossRef CAS PubMed.
- P. Liu, S. Gao, C. Chen, F. Zhou, Z. Meng, Y. Huang and Y. Wang, Vacancies-engineered and heteroatoms-regulated N-doped porous carbon aerogel for ultrahigh microwave absorption, Carbon, 2020, 169, 276–287 CrossRef CAS.
- G. L. Wu, Y. H. Cheng, Z. H. Yang, Z. R. Jia, H. J. Wu, L. J. Yang, H. L. Li, P. Z. Guo and H. L. Lv, Design of carbon sphere/magnetic quantum dots with tunable phase compositions and boost dielectric loss behavior, Chem. Eng. J., 2018, 333, 519–528 CrossRef CAS.
- J. Fan, W. Xing, Y. Huang, J. Dai, Q. Liu, F. Hu and G. Xu, Facile fabrication hierarchical urchin-like C/NiCo2O4/ZnO composites as excellent microwave absorbers, J. Alloys Compd., 2020, 821, 153491 CrossRef CAS.
- J. J. Pan, W. Xia, X. Sun, T. Wang, J. J. Li, L. Sheng and J. P. He, Improvement of interfacial polarization and impedance matching for two-dimensional leaf-like bimetallic (Co, Zn) doped porous carbon nanocomposites with broadband microwave absorption, Appl. Surf. Sci., 2020, 512, 144894 CrossRef CAS.
- C. Liu, X. Huang, J. Wang, H. Song, Y. Yang, Y. Liu, J. Li, L. Wang and C. Yu, Hollow mesoporous carbon nanocubes: rigid-interface-induced outward contraction of metal–organic frameworks, Adv. Funct. Mater., 2017, 28(6), 1705253 CrossRef.
- S. U. Rehman, M. Z. Sun, M. S. Xu, J. Liu, R. Ahmed, M. A. Aslam, R. A. Ahmad and H. Bi, Carbonized zeolitic imidazolate framework-67/polypyrrole: a magnetic-dielectric interface for enhanced microwave absorption properties, J. Colloid Interface Sci., 2020, 574, 87–96 CrossRef PubMed.
- W. Liu, S. Tan, Z. Yang and G. Ji, Hollow graphite spheres embedded in porous amorphous carbon matrices as lightweight and low-frequency microwave absorbing material through modulating dielectric loss, Carbon, 2018, 138, 143–153 CrossRef CAS.
- X. L. Wang, H. Xiao, A. Li, Z. Li, S. J. Liu, Q. H. Zhang, Y. Gong, L. R. Zheng, Y. Q. Zhu, C. Chen, D. S. Wang, Q. Peng, L. Gu, X. Han, J. Li and Y. Li, Constructing NiCo/Fe3O4 heteroparticles within MOF-74 for efficient oxygen evolution reactions, J. Am. Chem. Soc., 2018, 140, 15336–15341 CrossRef CAS PubMed.
- C. L. Peng, Y. N. Zhang and B. S. Zhang, MOF-derived jujube pit shaped C/Co composites with hierarchical structure for electromagnetic absorption, J. Alloys Compd., 2020, 826, 154203 CrossRef CAS.
- N. N. Wu, D. M. Xu, Z. Wang, F. L. Wang, J. R. Liu, W. Liu, Q. Shao, H. Liu, Q. Gao and Z. H. Guo, Achieving superior electromagnetic wave absorbers through the novel metal–organic frameworks derived magnetic porous carbon nanorods, Carbon, 2019, 145, 433–444 CrossRef CAS.
- Q. T. Liu, X. F. Liu, H. B. Feng, H. C. Shui and R. H. Yu, Metal organic framework-derived Fe/carbon porous composite with low Fe content for lightweight and highly efficient electromagnetic wave absorber, Chem. Eng. J., 2017, 314, 320–327 CrossRef CAS.
- B. Y. Zhu, P. Miao, J. Kong, X. L. Zhang, G. Y. Wang and K. J. Chen, Co/C composite derived from a newly constructed metal–organic framework for effective microwave absorption, Cryst. Growth Des., 2019, 19, 1518–1524 CrossRef CAS.
- X. Xiao, W. zhu, Z. Tan, W. Tian, Y. Guo, H. Wang, J. Fu and X. Jian, Ultra-small Co/CNTs nanohybrid from metal organic framework with highly efficient microwave absorption, Composites, Part B, 2018, 152, 316–323 CrossRef CAS.
- Z. Xiang, Y. M. Song, J. Xiong, Z. B. Pan, X. Wang, L. Liu, R. Liu, H. W. Yang and W. Lu, Enhanced electromagnetic wave absorption of nanoporous Fe3O4@carbon composites derived from metal–organic frameworks, Carbon, 2019, 142, 20–31 CrossRef CAS.
- B. Quan, X. H. Liang, X. Zhang, G. Y. Xu, G. B. Ji and Y. W. Du, Functionalized carbon nanofibers enabling stable and flexible absorbers with effective microwave response at low thickness, ACS Appl. Mater. Interfaces, 2018, 10, 41535–41543 CrossRef CAS PubMed.
- Y. Q. Wang, H. G. Wang, J. H. Ye, L. Y. Shi and X. Feng, Magnetic CoFe alloy@C nanocomposites derived from ZnCo–MOF for electromagnetic wave absorption, Chem. Eng. J., 2020, 383, 123096 CrossRef CAS.
- S. S. Wang, Y. C. Xu, R. R. Fu, H. H. Zhu, Q. Z. Jiao, T. Y. Feng, C. H. Feng, D. X. Shi, H. S. Li and Y. Zhao, Rational construction of hierarchically porous Fe–Co/N-doped carbon/rGO composites for broadband microwave absorption, Nano-Micro Lett., 2019, 11, 76 CrossRef CAS PubMed.
- W. Feng, Y. M. Wang, J. C. Chen, B. Q. Li, L. X. Guo, J. H. Ouyang, D. C. Jia and Y. Zhou, Metal organic framework-derived CoZn alloy/N-doped porous carbon nanocomposites: tunable surface area and electromagnetic wave absorption properties, J. Mater. Chem. C, 2018, 6, 10–18 RSC.
- M. Green and X. B. Chen, Recent progress of nanomaterials for microwave absorption, J. Mater. Chem., 2019, 5, 503–541 Search PubMed.
- S. Xie, Z. Ji, L. Zhu, J. Zhang, Y. Cao, J. Chen, R. Liu and J. Wang, Recent progress in electromagnetic wave absorption building materials, J. Build. Eng., 2020, 27, 100963 CrossRef.
- W. Liu, J. Liu, Z. Yang and G. Ji, Extended working frequency of ferrites by synergistic attenuation through a controllable carbothermal route based on prussian blue shell, ACS Appl. Mater. Interfaces, 2018, 10, 28887–28897 CrossRef CAS PubMed.
- M. L. Ma, W. T. Li, Z. Y. Tong, Y. Ma, Y. X. Bi, Z. J. Liao, J. Zhou, G. L. Wu, M. X. Li, J. W. Yue, X. Y. Song and X. Y. Zhang, NiCo2O4 nanosheets decorated on one-dimensional ZnFe2O4@SiO2@C nanochains with high-performance microwave absorption, J. Colloid Interface Sci., 2020, 578, 58–68 CrossRef CAS PubMed.
- Z. N. Li, X. J. Han, Y. Ma, D. W. Liu, Y. H. Wang, P. Xu, C. L. Li and Y. Du, MOFs-derived hollow Co/C microspheres with enhanced microwave absorption performance, ACS Sustainable Chem. Eng., 2018, 6, 8904–8913 CrossRef CAS.
- Z. L. Jia, D. Lan, K. Lin, M. Qin, K. Kou, G. Wu and H. Wu, Progress in low-frequency microwave absorbing materials, J. Mater. Sci.: Mater. Electron., 2018, 29, 17122–17136 CrossRef CAS.
- P. B. Liu, S. Gao, Y. Wang, Y. Huang, Y. Wang and J. H. Luo, Core–shell CoNi@graphitic carbon decorated on B,N-codoped hollow carbon polyhedrons toward lightweight and high-efficiency microwave attenuation, ACS Appl. Mater. Interfaces, 2019, 11, 25624–25635 CrossRef CAS PubMed.
- X. A. Li, D. X. Du, C. S. Wang, H. Y. Wang and Z. P. Xu, In situ synthesis of hierarchical rose-like porous Fe@C with enhanced electromagnetic wave absorption, J. Mater. Chem. C, 2018, 6, 558–567 RSC.
- Y. Jiao, J. Li, A. Xie, F. Wu, K. Zhang, W. Dong and X. Zhu, Confined polymerization strategy to construct polypyrrole/zeolitic imidazolate frameworks (PPy/ZIFs) nanocomposites for tunable electrical conductivity and excellent electromagnetic absorption, Compos. Sci. Technol., 2019, 174, 232–240 CrossRef CAS.
- M. Ma, W. Li, Z. Tong, Y. Yang, Y. Ma, Z. Cui, R. Wang, P. Lyu and W. Huang, 1D flower-like Fe3O4@SiO2@MnO2 nanochains inducing RGO self-assembly into aerogels for high-efficient microwave absorption, Mater. Des., 2020, 188, 108462 CrossRef CAS.
- K. Zhang, A. M. Xie, M. X. Sun, W. C. Jiang, F. Wu and W. Dong, Electromagnetic dissipation on the surface of metal organic framework (MOF)/reduced graphene oxide (RGO) hybrids, Mater. Chem. Phys., 2017, 199, 340–347 CrossRef CAS.
- S. Li, L. F. Lin, L. X. Yao, H. F. Zheng, Q. Luo, W. J. Xu, C. Y. Zhang, Q. S. Xie, L. S. Wang and D. L. Peng, MOFs-derived Co–C@C hollow composites with high-performance electromagnetic wave absorption, J. Alloys Compd., 2021, 856, 158183 CrossRef CAS.
- Q. Wu, J. Wang, H. Jin, T. Yan, G. Yi, X. Su, W. Dai and X. Wang, MOF-derived rambutan-like nanoporous carbon/nanotubes/Co composites with efficient microwave absorption property, Mater. Lett., 2019, 244, 138–141 CrossRef CAS.
- L. Ding, Y. Huang, Z. P. Xu, J. Yan, X. D. Liu, T. H. Li and P. B. Liu, MIL-53(Fe) derived MCC/rGO nanoparticles with excellent broadband microwave absorption properties, Compos. Commun., 2020, 21, 100362 CrossRef.
- H. F. Qiu, X. Y. Zhu, P. Chen, J. L. Liu and X. L. Zhu, Self-etching template method to synthesize hollow dodecahedral carbon capsules embedded with Ni–Co alloy for high-performance electromagnetic microwave absorption, Compos. Commun., 2020, 20, 100354 CrossRef.
- J. Xiong, Z. Xiang, B. W. Deng, M. C. Wu, L. Z. Yu, Z. C. Liu, E. B. Cui, F. Pan, R. Liu and W. Lu, Engineering compositions and hierarchical yolk–shell structures of NiCo/GC/NPC nanocomposites with excellent electromagnetic wave absorption properties, Appl. Surf. Sci., 2020, 513, 145778 CrossRef CAS.
- H. H. Zhao, X. J. Han, Z. N. Li, D. W. Liu, Y. H. Wang, Y. Wang, W. Zhou and Y. C. Du, Reduced graphene oxide decorated with carbon nanopolyhedrons as an efficient and lightweight microwave absorber, J. Colloid Interface Sci., 2018, 528, 174–183 CrossRef CAS PubMed.
- H. Zhao, Y. Cheng, Y. Zhang, Z. Zhang, L. Zhou and B. Zhang, Core–shell hybrid nanowires with Co nanoparticles wrapped in N-doped porous carbon for lightweight microwave absorption, Dalton Trans., 2019, 48, 15263–15271 RSC.
- H. L. Xu, X. W. Yin, M. Zhu, M. H. Li, H. Zhang, H. J. Wei, L. T. Zhang and L. F. Cheng, Constructing hollow graphene nano-spheres confined in porous amorphous carbon particles for achieving full X band microwave absorption, Carbon, 2019, 142, 346–353 CrossRef CAS.
- Q. L. Wu, H. H. Jin, W. Chen, S. Q. Huo, X. Chen, X. G. Su, H. Wang and J. Wang, Graphitized nitrogen-doped porous carbon composites derived from ZIF-8 as efficient microwave absorption materials, Mater. Res. Express, 2018, 5, 065602 CrossRef.
- D. Liu, Y. Du, F. Wang, Y. Wang, L. Cui, H. Zhao and X. Han, MOFs-derived multi-chamber carbon microspheres with enhanced microwave absorption, Carbon, 2020, 157, 478–485 CrossRef CAS.
- H. L. Peng, X. Zhang, H. L. Yang, Z. Q. Xiong, C. B. Liu and Y. Xie, Fabrication of core–shell nanoporous carbon@chiral polyschiff base iron(II) composites for high-performance electromagnetic wave attenuationthe low-frequency, J. Alloys Compd., 2021, 850, 156816 CrossRef CAS.
- P. B. Liu, S. Gao, Y. Wang, Y. Huang, W. J. He, W. H. Huang and J. H. Luo, Carbon nanocages with N-doped carbon inner shell and Co/N-doped carbon outer shell as electromagnetic wave absorption materials, Chem. Eng. J., 2020, 381, 122653 CrossRef CAS.
- L. Heng, Z. Zhang, S. Wang, X. Chen, X. Jia, Z. Tang and Y. Zou, Microwave absorption enhancement of Fe/C core–shell hybrid derived from a metal–organic framework, Nano, 2019, 14, 1950002 CrossRef CAS.
- J. Yu, J. Yu, T. Ying, X. Liu, X. Zhang and D. Han, Zeolitic imidazolate framework derived Fe–N/C for efficient microwave absorbers, J. Alloys Compd., 2020, 838, 155629 CrossRef CAS.
- X. Li, E. Cui, Z. Xiang, L. Yu, J. Xiong, F. Pan and W. Lu, Fe@NPC@CF nanocomposites derived from Fe–MOFs/biomass cotton for lightweight and high-performance electromagnetic wave absorption applications, J. Alloys Compd., 2020, 819, 152952 CrossRef CAS.
- Y. Wang, W. Zhang, X. Wu, C. Luo, Q. Wang, J. Li and L. Hu, Conducting polymer coated metal–organic framework nanoparticles: facile synthesis and enhanced electromagnetic absorption properties, Synth. Met., 2017, 228, 18–24 CrossRef CAS.
- Q. Rong, Y. C. Du, H. T. Zhao, Y. Wang, C. H. Tian, Z. G. Li, X. J. Han and P. Xu, Metal organic framework-derived Fe/C nanocubes toward efficient microwave absorption, J. Mater. Chem. A, 2015, 3, 13426–13434 RSC.
- Z. X. Zhao, S. W. Xu, Z. J. Du, C. Jiang and X. Z. Huang, Metal–organic framework-based PB@MoS2 core–shell microcubes with high efficiency and broad bandwidth for microwave absorption performance, ACS Sustainable Chem. Eng., 2019, 7, 7183–7192 CrossRef CAS.
- Y. J. Sun, N. Wang, H. Y. Yu and X. Z. Jiang, Metal–organic framework-based Fe/C@Co3O4 core–shell nanocomposites with outstanding microwave absorption properties in low frequencies, J. Mater. Sci., 2020, 55, 7304–7320 CrossRef CAS.
- W. Liu, Q. W. Shao, G. B. Ji, X. H. Liang, Y. Cheng, B. Quan and Y. W. Du, Metal–organic-frameworks derived porous carbon-wrapped Ni composites with optimized impedance matching as excellent lightweight electromagnetic wave absorber, Chem. Eng. J., 2017, 313, 734–744 CrossRef CAS.
- L. Wang, X. F. Yu, X. Li, J. Zhang, M. Wang and R. C. Che, MOF-derived yolk–shell Ni@C@ZnO Schottky contact structure for enhanced microwave absorption, Chem. Eng. J., 2020, 383, 123099 CrossRef CAS.
- Z. Yang, Y. Zhang, M. Li, L. Yang, J. Liu, Y. Hou and Y. Yang, Surface architecture of Ni-Based metal organic framework hollow spheres for adjustable microwave absorption, ACS Appl. Nano Mater., 2019, 2, 7888–7897 CrossRef CAS.
- Z. L. Zhang, Y. Y. Lv, X. Q. Chen, Z. Wu, Y. Y. He, L. Zhang and Y. H. Zou, Porous flower-like Ni/C composites derived from MOFs toward high-performance electromagnetic wave absorption, J. Magn. Magn. Mater., 2019, 487, 165334 CrossRef CAS.
- Z. Xiang, C. Huang, Y. M. Song, B. W. Deng, X. Zhang, X. J. Zhu, D. Batalu, O. Tutunaru and W. Lu, Rational construction of hierarchical accordion-like Ni@porous carbon nanocomposites derived from metal–organic frameworks with enhanced microwave absorption, Carbon, 2020, 167, 364–377 CrossRef CAS.
- B. Quan, X. H. Lang, G. B. Ji, J. N. Ma, P. Y. Ouyang, H. Gong, G. Y. Xu and Y. W. Du, Strong electromagnetic wave response derived from the construction of dielectric/magnetic media heterostructure and multiple interfaces, ACS Appl. Mater. Interfaces, 2017, 9, 9964–9974 CrossRef CAS PubMed.
- J. Li, P. Miao, K. J. Chen, J. W. Cao, J. Liang, Y. S. Tang and J. Kong, Highly effective electromagnetic wave absorbing prismatic Co/C nanocomposites derived from cubic metal–organic framework, Composites, Part B, 2020, 182, 107613 CrossRef CAS.
- B. H. Han, W. L. Chu, X. J. Han, P. Xu, D. W. Liu, L. R. Cui, Y. H. Wang, H. H. Zhao and Y. C. Du, Dual functions of glucose induced composition-controllable Co/C microspheres as high-performance microwave absorbing materials, Carbon, 2020, 168, 404–414 CrossRef CAS.
- K. F. Wang, Y. J. Chen, R. Tian, H. Li, Y. Zhou, H. N. Duan and H. Z. Liu, Porous Co–C core–shell nanocomposites derived from Co–MOF-74 with enhanced electromagnetic wave absorption performance, ACS Appl. Mater. Interfaces, 2018, 10, 11333–11342 CrossRef CAS PubMed.
- M. Q. Huang, L. Wang, K. Pei, W. B. You, X. F. Yu, Z. C. Wu and R. C. Che, Multidimension-controllable synthesis of MOF-derived Co@N-doped carbon composite with magnetic-dielectric synergy toward strong microwave absorption, Small, 2020, 16, 2000158 CrossRef CAS PubMed.
- X. M. Zhang, G. B. Ji, W. Liu, X. X. Zhang, Q. W. Gao, Y. C. Lia and Y. W. Du, A novel Co/TiO2 nanocomposite derived from a metal–organic framework: synthesis and efficient microwave absorption, J. Mater. Chem. C, 2016, 4, 1860–1870 RSC.
- S. Kang, W. Zhang, Z. M. Hu, J. R. Yu, Y. Wang and J. Zhu, Porous core–shell zeolitic imidazolate framework-derived Co/NPC@ZnO-decorated reduced graphene oxide for lightweight and broadband electromagnetic wave absorber, J. Alloys Compd., 2020, 818, 152932 CrossRef CAS.
- R. W. Shu, Y. Wu, W. J. Li, J. B. Zhang, Y. Liu, J. J. Shi and M. D. Zheng, Fabrication of ferroferric oxide-carbon/reduced graphene oxide nanocomposites derived from Fe-based metal–organic frameworks for microwave absorption, Compos. Sci. Technol., 2020, 196, 108240 CrossRef CAS.
- L. Heng, Z. Zhang, X. Chen, S. Wang, Z. Wu, Z. Xie, Z. Tang and Y. Zou, Fe/nanoporous carbon hybrid derived from metal–organic framework for highly effective microwave absorption, Appl. Organomet. Chem., 2019, 33, e4991 CrossRef.
- Z. L. Zhang, Q. H. Zhu, X. Q. Chen, Z. Wu, Y. Y. He, Y. Y. Lv, L. Zhang and Y. H. Zou, Ni@C composites derived from Ni-based metal organic frameworks with a lightweight, ultrathin, broadband and highly efficient microwave absorbing properties, Appl. Phys. Express, 2019, 12, 011001 CrossRef.
- B. Wen, H. B. Yang, Y. Lin, L. Wang, L. Ma and Y. Qiu, In situ anchoring carbon nanotubes on the Ni/C nanosheets with controllable thickness for boosting the electromagnetic waves absorption, Composites, Part A, 2020, 138, 106044 CrossRef CAS.
- H. C. Wang, L. Xiang, W. Wei, J. An, J. He, C. H. Gong and Y. L. Hou, Efficient and lightweight electromagnetic wave absorber derived from metal organic framework-encapsulated cobalt nanoparticles, ACS Appl. Mater. Interfaces, 2017, 9, 42102–42110 CrossRef CAS PubMed.
- P. B. Liu, S. Gao, Y. Wang, F. T. Zhou, Y. Huang and J. H. Luo, Metal–organic polymer coordination materials derived Co/N-doped porous carbon composites for frequency-selective microwave absorption, Composites, Part B, 2020, 202, 108406 CrossRef CAS.
- Y. Wang, X. Di, X. Gao and X. Wu, Design of MOF-derived hierarchical Co@C@RGO composite with controllable heterogeneous interfaces as a high-efficiency microwave absorbent, Nanotechnology, 2020, 31, 395710 CrossRef PubMed.
- Y. Wang, X. C. Di, X. Gao, X. M. Wu and P. Wang, Rational construction of Co@C polyhedrons covalently-grafted on magnetic graphene as a superior microwave absorber, J. Alloys Compd., 2020, 843, 156031 CrossRef CAS.
- H. Y. Fan, Z. J. Yao, J. T. Zhou, P. S. Yi, B. Wei, L. Lei and Y. M. Mao, Enhanced microwave absorption of epoxy composite by constructing 3D Co–C-MWCNTs derived from metal organic frameworks, J. Mater. Sci., 2021, 56, 1426–1442 CrossRef.
- C. H. Zhou, C. Wu, D. Liu and M. Yan, Metal–organic framework derived hierarchical Co/C@V2O3 hollow spheres as a thin, lightweight, and high-efficiency electromagnetic wave absorber, Chem.–Eur. J., 2019, 25, 2234–2241 CrossRef CAS PubMed.
- M. L. Yang, Y. Yuan, Y. Li, X. X. Sun, S. S. Wang, L. Liang, Y. H. Ning, J. J. Li, W. L. Yin, R. C. Che and Y. B. Li, Dramatically enhanced electromagnetic wave absorption of hierarchical CNT/Co/C fiber derived from cotton and metal–organic-framework, Carbon, 2020, 161, 517–527 CrossRef CAS.
- S. S. Dai, B. Quan, B. S. Zhang, X. H. Liang and G. B. Ji, Constructing multi-interface Mo2C/Co@C nano-rods for a microwave response based on a double attenuation mechanism, Dalton Trans., 2018, 47, 14767–14773 RSC.
- K. Y. Li, H. Sun, X. Zhang, S. Zhang, H. W. Dong, C. L. Zhu and Y. J. Chen, Micro-nanospheres assembled with helically coiled nitrogen-doped carbon nanotubes: fabrication and microwave absorption properties, Mater. Des., 2020, 186, 108290 CrossRef CAS.
- J. Xiong, Z. Xiang, J. Zhao, L. Z. Yu, E. B. Cui, B. W. Deng, Z. C. Liu, R. Liu and W. Lu, Layered NiCo alloy nanoparticles/nanoporous carbon composites derived from bimetallic MOFs with enhanced electromagnetic wave absorption performance, Carbon, 2019, 154, 391–401 CrossRef CAS.
- J. Ouyang, Z. L. He, Y. Zhang, H. M. Yang and Q. H. Zhao, Trimetallic FeCoNi@C nanocomposite hollow spheres derived from metal–organic frameworks with superior electromagnetic wave absorption ability, ACS Appl. Mater. Interfaces, 2019, 11, 39304–39314 CrossRef CAS PubMed.
- Y. Zhang, Z. Yang, M. Li, L. Yang, J. Liu, Y. Ha and R. Wu, Heterostructured CoFe@C@MnO2 nanocubes for efficient microwave absorption, Chem. Eng. J., 2020, 382, 123039 CrossRef CAS.
- Z. Yang, H. Lv and R. Wu, Rational construction of graphene oxide with MOF-derived porous NiFe@C nanocubes for high-performance microwave attenuation, Nano Res., 2016, 9, 3671–3682 CrossRef CAS.
- Y. L. Wang, S. H. Yang, H. Y. Wang, G. S. Wang, X. B. Sun and P. G. Yin, Hollow porous CoNi/C composite nanomaterials derived from MOFs for efficient and lightweight electromagnetic wave absorber, Carbon, 2020, 167, 485–494 CrossRef CAS.
- L. Wang, M. Q. Huang, X. F. Yu, W. B. You, J. Zhang, X. H. Liu, M. Wang and R. C. Che, MOF-derived Ni1−xCox@carbon with tunable nano-microstructure as lightweight and highly efficient electromagnetic wave absorber, Nano-Micro Lett., 2020, 12, 150 CrossRef CAS PubMed.
- L. Wang, B. Wen, H. B. Yang, Y. Qiu and N. R. He, Hierarchical nest-like structure of Co/Fe MOF derived CoFe@C composite as wide-bandwidth microwave absorber, Composites, Part A, 2020, 135, 105958 CrossRef CAS.
- P. Miao, J. X. Chen, Y. S. Tang, K. J. Chen and J. Kong, Highly efficient and broad electromagnetic wave absorbers tunedviatopology-controllable metal–organic frameworks, Sci. China Mater., 2020, 63, 2050–2061 CrossRef CAS.
- W. Feng, Y. Wang, J. Chen, B. Li, L. Guo, J. Ouyang, D. Jia and Y. Zhou, Metal organic framework-derived CoZn alloy/N-doped porous carbon nanocomposites: tunable surface area and electromagnetic wave absorption properties, J. Mater. Chem. C, 2018, 6, 10–18 RSC.
- X. Zhang, X. Zhang, D. Wang, H. Yuan, S. Zhang, C. Zhu, X. Zhang and Y. Chen, Three dimensional graphene-supported nitrogen-doped carbon nanotube architectures for attenuation of electromagnetic energy, J. Mater. Chem. C, 2019, 7, 11868–11878 RSC.
- X. Zhang, F. Yan, S. Zhang, H. R. Yuan, C. L. Zhu, X. T. Zhang and Y. J. Chen, Hollow N-doped carbon polyhedron containing CoNi alloy nanoparticles embedded within few-layer N-doped graphene as high-performance electromagnetic wave absorbing material, ACS Appl. Mater. Interfaces, 2018, 10, 24920–24929 CrossRef CAS PubMed.
- P. B. Liu, S. Gao, W. H. Huang, J. Ren, D. Y. Yu and W. J. He, Hybrid zeolite imidazolate framework derived N-implanted carbon polyhedrons with tunable heterogeneous interfaces for strong wideband microwave attenuation, Carbon, 2020, 159, 83–93 CrossRef CAS.
- W. Huang, X. Zhang, Y. Zhao, J. Zhang and P. Liu, Hollow N-doped carbon polyhedra embedded Co and Mo2C nanoparticles for high-efficiency and wideband microwave absorption, Carbon, 2020, 167, 19–30 CrossRef CAS.
- R. Das, P. Pachfule, R. Banerjee and P. Poddar, Metal and metal oxide nanoparticle synthesis from metal organic frameworks (MOFs): finding the border of metal and metal oxides, Nanoscale, 2012, 4, 591–599 RSC.
- X. Liang, X. Xu, Z. Man, B. Quan, B. Sun, J. Chen, W. Gu and G. Ji, Zinc oxide/nanoporous carbon hybrid materials derived from metal–organic frameworks with different dielectric and absorption performances, Inorg. Chem. Front., 2019, 6, 2521–2527 RSC.
- X. Liang, B. Quan, G. Ji, W. Liu, H. Zhao, S. Dai, J. Lv and Y. Du, Tunable dielectric performance derived from the metal–organic framework/reduced graphene oxide hybrid with broadband absorption, ACS Sustainable Chem. Eng., 2017, 5, 10570–10579 CrossRef CAS.
- Y. W. Bai, G. M. Shi, J. Gao and F. N. Shi, MOF decomposed for the preparation of Co3O4/N-doped carbon with excellent microwave absorption, J. Solid State Chem., 2020, 288, 121401 CrossRef CAS.
- X. Zhang, J. Qiao, C. Liu, F. L. Wang, Y. Y. Jiang, P. Cui, Q. Wang, Z. Wang, L. L. Wu and J. R. Liu, A MOF-derived ZrO2/C nanocomposite for efficient electromagnetic wave absorption, Inorg. Chem. Front., 2020, 7, 385–393 RSC.
- J. N. Ma, W. Liu, X. H. Liang, B. Quan, Y. Cheng, G. B. Ji and W. Meng, Nanoporous TiO2/C composites synthesized from directly pyrolysis of a Ti-based MOFs MIL-125(Ti) for efficient microwave absorption, J. Alloys Compd., 2017, 728, 138–144 CrossRef CAS.
- R. W. Shu, W. J. Li, Y. Wu, J. B. Zhang, G. Y. Zhang and M. D. Zheng, Fabrication of nitrogen-doped cobalt oxide/cobalt/carbon nanocomposites derived from heterobimetallic zeolitic imidazolate frameworks with superior microwave absorption properties, Composites, Part B, 2019, 178, 107518 CrossRef CAS.
- Y. S. Liu, B. Quan, X. H. Liang, B. Huang, S. L. Huang, X. D. Li, G. B. Ji, Z. Jin and G. C. Yang, Energetic metal–organic frameworks deflagration enabled ultrafast low-temperature synthesis of ultra-light magnetic nanoparticles decorated high-lossy materials, Carbon, 2020, 165, 286–295 CrossRef CAS.
- L. Ding, Y. Huang, Z. P. Xu, J. Yan, X. D. Liu, T. H. Li and P. B. Liu, MIL-53(Fe) derived MCC/rGO nanoparticles with excellent broadband microwave absorption properties, Compos. Commun., 2020, 21, 100362 CrossRef.
- X. Zhen, X. Juan, B. W. Deng, E. Cui, L. Y. Yu, Q. W. Zeng, K. Pei, R. C. Che and W. Lu, Rational design of 2D hierarchically laminated Fe3O4@nanoporous carbon@rGO nanocomposites with strong magnetic coupling for excellent electromagnetic absorption applications, J. Mater. Chem. C, 2020, 8, 2123–2134 Search PubMed.
- Z. G. Gao, J. Q. Zhang, S. J. Zhang, D. Lan, Z. H. Zhao and K. C. Kou, Strategies for electromagnetic wave absorbers derived from zeolite imidazole framework (ZIF-67) with ferrocene containing polymers, Polymer, 2020, 202, 122679 CrossRef CAS.
- X. K. Wang, P. P. Zhou, G. H. Qiu, X. Y. Zhang, L. X. Wang, Q. T. Zhang, M. Wang and Z. H. Liu, Excellent electromagnetic wave absorption properties of porous core–shell CoO/Co@C nanocomposites derived from a needle-shaped Co(OH)2@ZIF-67 template, J. Alloys Compd., 2020, 842, 155807 CrossRef CAS.
- S. Y. Wang, S. S. Peng, S. T. Zhong and W. Jiang, Construction of SnO2/Co3Sn2@C and SnO2/Co3Sn2@Air@C hierarchical heterostructures for efficient electromagnetic wave absorption, J. Mater. Chem. C, 2018, 6, 9465–9474 RSC.
- F. Saraci, V. Quezada-Novoa, P. R. Donnarumma and A. J. Howarth, Rare-earth metal–organic frameworks: from structure to applications, Chem. Soc. Rev., 2020, 49, 7949–7977 RSC.
- L. W. Zhu, N. Liu, X. H. Jiang, L. M. Yu and X. Li, Four novel 3D RE-MOFs based on maleic hydrazide: syntheses, structural diversity, efficient electromagnetic wave absorption and antibacterial activity properties, Inorg. Chim. Acta, 2020, 501, 119291 CrossRef CAS.
- D. M. Xu, N. N. Wu, K. Le, F. L. Wang, Z. Wang, L. L. Wu, W. Liu, A. C. Ouyang and J. R. Liu, Bimetal oxide-derived flower-like heterogeneous Co/MnO@C composites with synergistic magnetic-dielectric attenuation for electromagnetic wave absorption, J. Mater. Chem. C, 2020, 8, 2451–2459 RSC.
- H. Chen, R. Hong, Q. C. Liu, S. K. Li, F. Z. Huang, Y. Lu, L. Wang, K. Z. Li and H. Zhang, CNFs@carbonaceous Co/CoO composite derived from CNFs penetrated through ZIF-67 for high-efficient electromagnetic wave absorption material, J. Alloys Compd., 2018, 752, 115–122 CrossRef CAS.
- S. U. Rehman, J. M. Wang, Q. H. Luo, M. Z. Sun, L. Jiang, Q. Han, J. C. Liu and H. Bi, Starfish-like C/CoNiO2 heterostructure derived from ZIF-67 with tunable microwave absorption properties, Chem. Eng. J., 2019, 373, 122–130 CrossRef.
- P. B. Liu, C. Y. Zhu, S. Gao, C. Guan, Y. Huang and W. J. He, N-doped porous carbon nanoplates embedded with CoS2 vertically anchored on carbon cloths for flexible and ultrahigh microwave absorption, Carbon, 2020, 163, 348–359 CrossRef CAS.
- Q. N. Mao, J. Liu, Q. Cao, D. X. Yang, J. S. Zhong, G. Y. Sun, J. H. Zheng, L. F. Kuang, B. Y. Guo, Q. W. Liu, X. Z. Hao, Z. G. Ji, T. Yang and X. G. Liu, Porous carbon nanorods decorated with graphitic carbon bubbles encapsulated NiSe nanoparticles as an efficient microwave absorber, Ceram. Int., 2020, 46, 13752–13761 CrossRef CAS.
- Q. Liao, M. He, Y. Zhou, S. Nie, Y. Wang, S. Hu, H. Yang, H. Li and Y. Tong, Highly cuboid-shaped heterobimetallic metal–organic frameworks derived from porous Co/ZnO/C microrods with improved electromagnetic wave absorption capabilities, ACS Appl. Mater. Interfaces, 2018, 10, 29136–29144 CrossRef CAS PubMed.
- T. G. Zhu, Y. Sun, Y. J. Wang, H. N. Xing, X. Y. Li, P. Hu, Y. Zong and X. L. Zheng, A MOF-driven porous iron with high dielectric loss and excellent microwave absorption properties, J. Mater. Sci.: Mater. Electron., 2020, 31, 6843–6854 CrossRef CAS.
- X. Q. Cui, X. H. Liang, J. B. Chen, W. H. Gu, G. B. Ji and Y. W. Du, Customized unique core–shell Fe2N@N-doped carbon with tunable void space for microwave response, Carbon, 2020, 156, 49–57 CrossRef CAS.
- X. Liang, B. Quan, Y. Sun, G. Ji, Y. Zhang, J. Ma, D. Li, B. Zhang and Y. Du, Multiple interfaces structure derived from metal–organic frameworks for excellent electromagnetic wave absorption, Part. Part. Syst. Charact., 2017, 34, 1700006 CrossRef.
- X. Liang, B. Quan, G. Ji, W. Liu, Y. Cheng, B. Zhang and Y. Du, Novel nanoporous carbon derived from metal–organic frameworks with tunable electromagnetic wave absorption capabilities, Inorg. Chem. Front., 2016, 3, 1516–1526 RSC.
|
This journal is © The Royal Society of Chemistry 2021 |
Click here to see how this site uses Cookies. View our privacy policy here.