DOI:
10.1039/D0QI00785D
(Review Article)
Inorg. Chem. Front., 2020,
7, 3315-3326
External stimuli modulate the magnetic relaxation of lanthanide single-molecule magnets
Received
1st July 2020
, Accepted 23rd July 2020
First published on 27th July 2020
Abstract
Mutilfunctional molecular magnetic materials have promising potential for use as future molecular devices, such as for magneto-luminescence sensing, optical switching, information storage, etc. Lanthanide ions have been considered as excellent candidates to construct molecular switches as they are very sensitive to the subtle changes of structure including the change of molecular geometry, bond distance, even crystal packing, etc., while for transition metal ions, in most cases, only the change of electronic configuration can cause a switch of magnetic properties. This review aims to summarize the effects of stimuli-induced structural transformations on the magnetic relaxation involving light-, solvato-, proton- and redox-switching of Ln-SMM behaviour. Emphasis is placed on the role played by external stimuli in reversibly modulating the magnetic properties realized in the systems with single-crystal-to-single-crystal structural transformation.
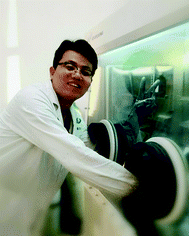 Zhenhua Zhu | Zhenhua Zhu obtained his B.S. degree from Hefei University of Technology in 2015. Now he is a Ph.D. student in the research group of Prof. Tang at CIAC. His current research focuses on the design and synthesis of organometallic molecular magnets and multifunctional molecular materials. |
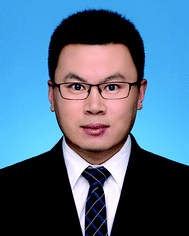 Xiao-Lei Li | Xiao-Lei Li received his B.S. degree in 2010 and MSc in 2013 from Northeast Normal University. He obtained his Ph.D. in 2017 from Nankai University, under the supervision of Prof. Peng Cheng. He joined the group of Prof. Jinkui Tang at CIAC as an assistant researcher in 2018. His research program concerns the self-assembly of interesting magnetic complexes using lanthanide ions and molecular precursors. |
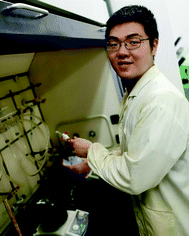 Shuting Liu | Shuting Liu received his B.S. degree from Hebei University of Technology in 2016. He is currently working toward a PhD degree in Inorganic Chemistry under the supervision of Prof. Jinkui Tang at CIAC. His main research interests include the synthesis and surface deposition of molecular magnets based on lanthanide. |
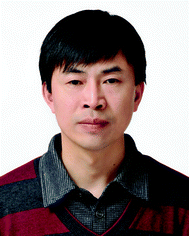 Jinkui Tang | Jinkui Tang received his PhD degree in 2001 from Nankai University under the supervision of Prof. Dai-Zheng Liao and then worked as a postdoctoral fellow at Universität Karlsruhe (TH) in the research group of Prof. Annie K. Powell and Universiteit Leiden with Prof. Jan Reedijk, before initiating his independent career at Changchun Institute of Applied Chemistry, Chinese Academy of Sciences in 2007. Tang group's research interests are centered around the design and characterization of coordination compounds with interesting magnetic properties. |
Introduction
Molecular magnetism, which started in the 1980s, is a multidisciplinary research field.1 Distinguished from the traditional inorganic magnetic materials, molecular magnetic materials exhibit fascinating and valuable cooperative-electronic properties, e.g. combining magnetic properties with conductivity or optical phenomena.2–4 In the past 27 years or so, single molecule magnets (SMMs) and multifunctional magnetic materials have been dominating the field for their practical applications in Quantum Information Technology (QIP) including molecule-based information storage, spintronic devices, quantum computing, etc.5–10 In 2003, Ishikawa et al. reported the first example of lanthanide SMMs (Ln-SMMs),11i.e. double-decker lanthanide complexes [Pc2Ln][NBu4] (Pc = phthalocynanine, Ln = Tb, Dy) containing only one magnetic ion, which motivated chemists to introduce lanthanides into SMMs due to their large magnetic anisotropy and strong spin–orbit coupling,12,13 eventually leading to the discovery of magnetic hysteresis up to 80 K at a sweep rate of 25 Oe s−1 in a near-linear dysprosium metallocene compound [(CpiPr5)Dy(Cp*)][B(C6F5)4] (CpiPr5 = penta-iso-propylcyclopentadienyl, Cp* = pentamethylcyclopentadienyl).14 As the 4f orbitals of lanthanides are well shielded by the outer 5s and 5p orbitals, a smaller crystal field (CF) splitting or weaker metal–ligand covalence is expected for lanthanides compared to d-block metals.15 In most cases, the CF multiplet structure of lanthanides, which generally depends on the molecular geometry or the symmetry of the coordination environment, coordination numbers and the types of donor atoms, defines the magnetic properties (or magnetic relaxation) of the complexes.12 The coordination geometries of lanthanides are determined by ligand steric factors therefore usually forming labile ‘ionic’ complexes that can undergo facile exchange of ligands, which provides more possibilities to modulate the magnetic properties. Taking Dy(III)-SMMs as an example, the perfect molecular geometries are Cn (n > 7), C5h/D5h, S8/D4d and S12/D6d where a pure axial CF was constructed based on the oblate spheroidal shape of the Dy(III) free-ion electron density.16 Although it is difficult to make such ideal coordination environments for Dy(III) ions, building a predominant bond by lowering the coordination number or introducing anionic donor atoms with high electronegativity (e.g. F−, O− or even carbanion) in complexes, which generally refers to the one with the shortest bond length, can also provide strong axial CF.17 For a long time, we have been modeling magnetic relaxation of Dy(III)-SMMs on the general assumption of regarding the energy barrier as a sole criterion on the basis of an ideal condition where the temperature of the lattice is equal to that of the surrounding bath.18 In recent years, consideration of other factors associated with molecular vibrations, such as coupling of anharmonic phonons and spin-phonons that addresses the presence of alternative relaxation processes, has been required.19–21 Therefore, the design criteria for high-temperature Dy(III)-SMMs have been evolving, from the traditional requirements of high axial symmetry of CF based on the electrostatic repulsion interactions between lanthanides and ligand atoms to the new trend of considering the effects of molecular vibrational modes on magnetic relaxation.20,22 In addition, the magnetic relaxation mechanism has also made great progress especially the quenching of the relaxation through the first excited state and the recognition of the vital importance of two-phonon Raman relaxation process.23–25
Apart from Ln-SMMs, in recent years, multifunctional molecular magnetic materials showing peculiar functionalities in addition to and/or interacting with the magnetic properties, such as microporous magnets, conducting magnets, chiral magnets, luminescent magnets etc., have also attracted more and more attention in the molecular magnetism community due to their potential applications in sensors, optical switches, magnetic gas separations, etc.26–32 So far, while numerous reviews or books have been published on Ln-SMMs and multifunctional molecular magnetic materials, however, to the best of our knowledge, the scope of stimuli-responsive Ln-SMMs has been far less highlighted.33 In fact, the SMM behaviour is just a manifestation of the stimuli from alternating current (ac) magnetic field. Compared to the external stimuli modulated transition metal (TM) molecular magnets, the research of stimuli-responsive Ln-SMMs is still in its infancy. For example, the light-induced excited spin state trapping phenomenon or the LIESST effect has been a focus of research over 30 years; especially in the past ten years, the introduction of a photoisomerizable diarylethene-derived ligand into a spin-crossover iron(II) complex led to the construction of magneto-optical molecular devices with reversible photomagnetic conversions.34–39 In 2009, the first example of photochemical control of SMMs was reported in a [Mn4] one-dimensional (1D) chain structure and four years later, the light-actuated SMM combined with a spin-crossover phenomenon was also fabricated.40–42 However, for Ln-SMMs, the efficient photomodulation of magnetic relaxation has not been realized until recently (vide infra).43 Another typical example is that the first combination of high-pressure X-ray crystallography and magnetic measurements on TM-molecular magnets was reported in 2008, while a similar investigation on Ln-SMMs was published in 2020.44,45
Although light-actuated TM-molecular magnets have become a fascinating topic in recent years, most Fe(II) complexes hardly exhibited good SMM properties due to the non-Kramers systems and the quenching of the fist-order spin–orbit coupling (SOC) unless they possess a strict symmetry of CF.46 In contrast, Co(II) complexes usually displayed impressive SMM properties with a record effective energy barrier (Ueff) of 413 cm−1; however, the fact that Co(II) ions can quench the luminescence of organic ligands prevents the design of photomodulated SMMs.47,48 In this connection, some significant advances in stimuli-responsive Ln-SMMs have been made owing to the unique 4f orbitals of lanthanides. In this review, we focus on the manipulations of the magnetic relaxation of Ln-SMMs through external stimuli generally classified as physical stimuli (such as light, temperature, pressure, electric potential) and chemical influences (such as redox, pH-responsive, guest-exchange), shedding light on the utilization of SMMs in molecular devices.
Photo-switching of Ln-SMM behaviour
Light as an extremely attractive tool in the field of molecular materials due to its easy availability, environmental friendliness, sustainability, etc. has garnered substantial attention. Photomagnets refer to a very special class of multifunctional molecular magnetic materials where the magnetization can be controlled by photons.50 Until now, the most widely used strategy for designing and synthesizing photo-switchable Ln-SMMs is to introduce a photochromic ligand on account of its light-driven reversible transformation between two isomeric forms. The most formidable challenge to this approach is that the light-driven structural changes hardly alter the first coordination environment of lanthanide ions, which is closely related to the magnetic anisotropy and slow relaxation of magnetization of Ln-SMMs, leading to only a few examples being known. However, these structural changes often lead to modulation of electronic properties, polarity, conjugation, molecular geometry, physical dimensions, intermolecular interactions, etc.,51 which provide other ways to externally manipulate the SMM behaviour.
Since 2011, the Yamashita group has used a diarylethene photochromic ligand (DTE) to explore the photo-control of magnetic interactions and slow relaxation of magnetization in Dy(III)/Ho(III)-complexes. Although there are some differences in the sub-level of the ground state and quantum-tunneling relaxation between the open form and the closed form of DTE, the unclear photo-control mechanism and the unsatisfactory SMM properties attributed to the lack of single-crystal-to-single-crystal (SC–SC) structural transformation and the reasonable CF environment prevent further investigations.52–54
Recently, the Long group reported a photochromic chain of Dy(III)-SMM based on a highly magnetic anisotropic compound, [Dy(Tppy)(pyridine)2]PF6 (Tppy = tris(3-(2-pyridyl)pyrazolyl)hydroborate), using a bispyridyl dithienylethene photochromic ligand as the photoactive unit (Fig. 1a).49,55 Compared to previous efforts, the main breakthroughs in this contribution are (1) the magnetic unit has a significantly improved SMM property due to the presence of a strong axial magnetic anisotropy provided by a relatively short Dy–F bond; (2) the SC–SC isomerization was achieved upon visible irradiation, which is the essential prerequisite for ab initio calculations, providing possibilities to explore the mechanism of photo-modulated SMM behaviour (Fig. 1b). Photocrystallography investigations showed that continuous irradiation of a single crystal of compound 1c (closed form) of 1 h with green light resulted in obvious variations of the unit cell parameters with a small increase of 1.3% and 2.75% in b and c lattice parameters, respectively, and a decrease of 1.5% in the a lattice parameter (Fig. 1c). However, only partial conversions of 1o (open form) toward 1c with 365 nm light were observed, suggesting a more preferred packing rearrangement in 1o. In order to investigate the influences of photoisomerization on the magnetic properties, static (dc) and dynamic (ac) magnetic measurements were performed for compounds 1c and 1o, and the results revealed that the peak in out-of-phase susceptibility of 1c was observed as high as 44 K under a zero dc field giving the Ueff of 157 cm−1, which displayed almost identical magnetic behaviour to 1o due to the intact first coordination environment. The most striking difference between 1c and 1o was the quantum-tunneling relaxation (τtunnel), 0.0337 s for 1c while 0.0015 s for 1o. The significantly decreased τtunnel value of 1o was also reflected by the narrower hysteresis hoop, for which a slightly stronger nearest-neighbor dipolar coupling in 1o than 1c induced by the subtle changes in crystal packing should be responsible, evident by ab initio calculations.
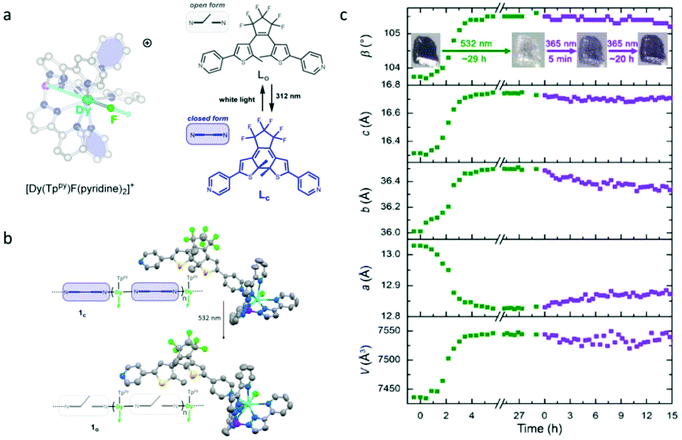 |
| Fig. 1 (a) Molecular structure of [Dy(Tppy) (pyridine)2]PF6 in the cationic form and the open and closed form of the photoactive bispyridyl dithienylethene unit used in building 1c; (b) single crystal structures of 1c and 1o; (c) evolution of the unit cell parameters of 1c upon continuous irradiation with 532 nm light (green dots) followed by 365 nm light (purple dots). Reproduced with permission from ref. 49. Copyright 2020 American Chemical Society.49 | |
Although the photo-modulated magnetic hysteresis was observed in the above compounds, the On/Off switching of SMM behaviour has not been realized. In 2020, the Wang group reported a series of chain compounds, [Ln3(HEDP3−)3(HEDP2−)3]·2TPT3+·HEDP·10H2O (QDU-1(Ln)) (Ln = Dy, Gd, Y; HEDP = hydroxyethylidene diphosphonate, TPT = 2,4,6-tri(4-pyridyl)-1,3,5-triazine), prepared via solvothermal reactions, among which, the radical-actuated On/Off SMM behaviour via room temperature (RT) light irradiation was achieved for the first time.43 Note that the TPT ligand is in the form of pyridine salt with three positive charges. As shown in Fig. 2a, the oxygen atoms from HEDP serve as electron donors, while the nitrogen atoms in TPT units play the role of electron acceptors showing obvious H-bonding interactions between them. When illuminated by UV light in air, the crystal, QDU-1Dy, quickly turned blue, named QDU-1a(Dy), from colorless. QDU-1a(Dy) displayed a very stable state when stored below −20 °C and can return to the initial colorless state (QDU-1b(Dy)) by heating to 100 °C for 0.5 h (Fig. 2b). UV-vis, fluorescence, and ESR spectra confirmed the presence of photoinduced electron transfer (PET) from HEDP-Dy(III) chains to TPT ligands, during which the stable photogenerated anionic O˙ and cationic TPT˙ radicals were observed upon irradiation of QDU-1(Dy). More importantly, only electron transfer process took place before and after irradiation, namely the main structure was maintained without photoinduced photolysis or isomerization, proved by the unchanged XRD, IR spectra and elemental analysis. The single crystal structures of QDU-1(Dy) and QDU-1a(Dy) confirmed this PET process with an obvious volume reduction of the unit cell and a closer N⋯O distance between the HEDP-Ln chain and TPT ligand.
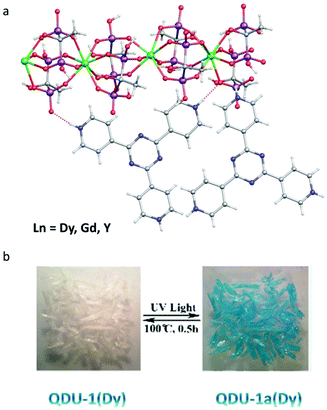 |
| Fig. 2 (a) Crystal structures of QDU-1(Ln); (b) reversible photochromic behaviour between QDU-1(Dy) and QDU-1a(Dy). Reproduced with permission from ref. 43. Copyright 2020 American Chemical Society.43 | |
Static and dynamic magnetic measurements were carried out to explore the difference in magnetic properties before and after irradiation. Expectedly, QDU-1a(Dy) showed a χT value of 43.67 cm3 mol−1 K at 300 K, slightly larger (3%) than the value of QDU-1(Dy) indicating the presence of photogenerated radicals. As shown in Fig. 3a, QDU-1(Dy) exhibited a gradual decrease of the χT value suggesting weak interactions; however, a steady increase of the χT value for QDU-1a(Dy) was observed, reaching up to 52.67 cm3 mol−1 K at 2 K, demonstrating the presence of strong ferromagnetic interactions between Dy(III) ions and anionic O˙ radicals, which were also confirmed by the isostructural Y(III) and Gd(III) complexes. Once heated, QDU-1b(Dy) showed a similar trend of χT values toQDU-1(Dy), that is to say, the manipulation of On/Off magnetic interactions was realized. In addition, a more exciting result is that the out-of-phase susceptibility of QDU-1a(Dy) exhibited strong temperature dependency with clear χ′′ peaks under a zero-dc field corresponding to the typical SMM behaviour, which can be attributed to the combination of large anisotropy of Dy(III) ions and strong ferromagnetic coupling between photogenerated anionic O˙ radicals and Dy(III) ions induced by the PET process, while only slow relaxation of magnetization was observed in QDU-1(Dy) (Fig. 3b).
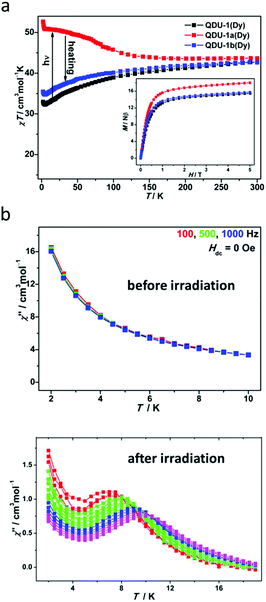 |
| Fig. 3 (a) Temperature dependence of χmT values for QDU-1(Dy), QDU-1a(Dy) and QDU-1b(Dy); (b) plots of χ′′(T) in the absence of a dc field at various ac frequencies for QDU-1(Dy) (up) and QDU-1a(Dy) (bottom). Reproduced with permission from ref. 43. Copyright 2020 American Chemical Society.43 | |
In addition, photoactive ligands including 1,2-bis(4-pyridyl)ethene (bpe) and anthracene (or its derivatives) were also used to construct photo-responsive Ln-SMMs. Although the SC–SC transformation was achieved in bde-based Ln-SMMs (Ln = Dy, Er) by the photodimerized [2 + 2] cycloaddition reaction of two bde ligands upon UV irradiation, this physical process was not reversible.56–58 In 2018, the Zheng group reported a mononuclear Dy(III)-compound, Dy(depma)(NO3)3(hmpa)2 (hmpa = hexamethylphosphoramide, depma = 9-diethylphosphono-methylanthracene), exhibiting a reversible SC–SC transformation in anthracene-based lanthanide complexes.59 When the yellow crystal of this compound was irradiated at 365 nm for 0.5 h, a colorless crystal of Dy2(depma2)(NO3)6(hmpa)4 without loss of crystallinity was obtained involving the [4 + 4] cycloaddition reaction of the neighboring anthracene moieties and meanwhile accompanied by a profound change in magneto-optic properties, i.e. from a single-ion magnet with yellow-green emission to a single-molecule magnet with blue-white emission. A completely reversed transformation occurred if this dinuclear compound was heated at 100 °C in air, opening a new route for designing photo-switchable smart molecular materials.
Solvato-switching of Ln-MOF-SMM behaviour
Compared to photomodulation, thermal treatment may be a more effective physical stimulus for SMM systems, during which, the desolvation or solvation process induced phase transformation usually took place resulting in the changes of the coordination environment of lanthanides therefor affecting the magnetic properties. Herein, we only discuss Ln-MOF-SMMs due to the generally higher structural stability of MOFs than low-dimensional coordination compounds providing greater possibilities to achieve reversible SC–SC structural transformation. In 2013, the Shi group investigated the magnetic behaviour of a very classic MOF system with relatively high thermal stability, Dy(BTC)(H2O)·DMF and Dy(BTC) (BTC = 1,3,5-benzenetricarboxylate), which was first reported by Qiu and co-workers in 2006.60,61 As shown in Fig. 4a, when Dy(BTC)(H2O)·DMF was heated under vacuum at 240 °C for 12 h, the terminal coordinated water molecules and DMF were removed producing Dy(BTC), accompanied by the change of coordination geometry of Dy(III) ions from seven-coordinate pentagonal-bipyramidal to six-coordinate distorted trigonal prism. If Dy(BTC) was immersed in the mixture of DMF and H2O, it returned to Dy(BTC)(H2O)·DMF as confirmed by single crystal X-ray diffraction, XRD, IR and elemental analysis. Ac susceptibility measurements showed that Dy(BTC) is a field-induced SMM while no χ′′ signals were observed for Dy(BTC)(H2O)·DMF under a 1000 Oe dc field (Fig. 4b), so the reversible switching of SMM behaviour by the desolvation and solvation process in a Ln-MOF system was achieved for the first time.
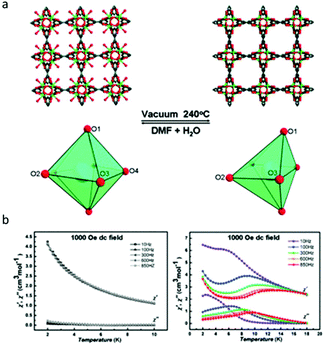 |
| Fig. 4 (a) The structural or coordination geometry changes of the framework and Dy(III) ions; (b) temperature dependence of χ′ and χ′′ for Dy(BTC)(H2O)·DMF (left) and Dy(BTC) (right). Reproduced with permission from ref. 61. Copyright 2013 Royal Society of Chemistry.61 | |
In the same year, the Maji group reported a Dy(III)–K(I) oxalate framework, {KDy(ox)2(H2O)4}n (ox = oxalate dianion), where each Dy(III) ion is octacoordinated possessing a distorted square antiprismatic coordination geometry.62 Static magnetic measurements suggested the presence of ferromagnetic interactions operating between the ox-connected Dy(III) centers in the system. When it was heated at 130 °C for 6 h under vacuum, the H2O molecules bonded to K(I) ions were removed producing a dehydrated framework, {KDy(ox)2}n, in which the coordination environment of Dy(III) ions was unchanged. However, the antiferromagnetic interaction was observed in {KDy(ox)2}n confirmed by the magnetically diluted sample {KDy0.25Y0.75(ox)2}n and if it was exposed to H2O vapor for three days, the rehydrated framework was obtained showing the ferromagnetic interactions again. The result indicated that in this Ln-MOF system, the reversible ferromagnetic to antiferromagnetic phase transition can be completed by the dehydration and rehydration process. However, the SMM properties have hardly changed when the magnetic interactions were switched to antiferromagnetic from ferromagnetic attributed to the almost unchanged D4d coordination geometry of Dy(III) ions (Ueff: 417 K for {KDy(C2O4)2(H2O)4}n; 418 K for {KDy(C2O4)2}n).
The above two examples inspired us that the change of the first coordination environment of Dy(III) ions can induce the switching of SMM behaviour more clearly. In 2019, the Ohkoshi group reported a bimetal cyanido-bridged Dy–Co MOF system, {[Dy(III)(H2O)2][Co(III)(CN)6]}·2.2H2O, where each Dy(III) ion is eight-coordinate (N6O2) with square antiprism geometry (D4d) (Fig. 5).63 The single crystal of its dehydrated compound, {Dy(III)[Co(III)(CN)6]}, was obtained by heating {[Dy(III)(H2O)2][Co(III)(CN)6]}·2.2H2O to ca. 470 K under the nitrogen flow in which the coordination environment of Dy(III) ions was changed to trigonal prism geometry (D3h). In addition, during this phase transformation, water of crystallization that occupied the interstitial space in the hydrated compound was also removed completely; meanwhile the space group changed to P63/mmc of a hexagonal crystal system from Cmcm of an orthorhombic crystal system. When keeping a crystal of {Dy(III)[Co(III)(CN)6]} for several days under a humid atmosphere, a rehydrated compound with excellent crystallinity was obtained showing the isostructural characteristics to the hydrated compound. For magnetic properties, no obvious χ′′(v) signals were observed in the hydrated compound under a zero dc field or even after the application of the external dc magnetic field. In contrast, the dehydrated compound exhibited the typical SMM behaviour with clear maximum values of χ′′(v) under a zero or 2000 Oe dc field giving the corresponding Ueff of 52 K and 58 K, respectively. The result proved that dehydration driven phase transformation between {[Dy(III)(H2O)2][Co(III)(CN)6]}·2.2H2O and {Dy(III)[Co(III)(CN)6]} leads to the reversible ON/OFF switching of SMM behaviour.
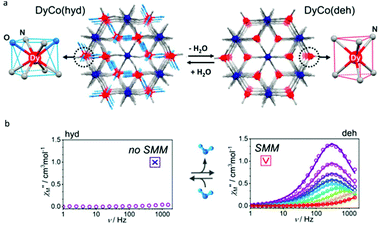 |
| Fig. 5 (a) Crystal structures and (b) plots of χ′′(v) in the absence of a dc field for {[Dy(III)(H2O)2][Co(III)(CN)6]}·2.2H2O (left) and {Dy(III)[Co(III)(CN)6]} (right). Reproduced with permission from ref. 63. Copyright 2019 American Chemical Society.63 | |
Proton-switching of Ln-SMM behaviour
In this section, we introduce two typical examples of proton switching of Ln-SMM behaviour. The first one is a tetraphenylporphyrin (TPP)-based Tb(III) double-decker complex reported by Ishikawa and collaborators in 2012 (Fig. 6).64 Single crystal structure analysis showed that the Tb(III) ion is hepta-coordinate with seven Tb–N bond lengths in the range of 2.430(7)–2.627(7) Å in the protonated complex, [Tb(III)H(TPP)2], where a proton is located on a nitrogen atom of the TPP pyrrole ring leading to a significantly longer distance between the Tb(III) ion and N atom of 2.840(6) Å. When DBU (a base as an organic proton acceptor) is added to the solution of this protonated complex, deprotonation occurs and the complex, [Tb(III)(TPP)2(H-DBU)], was obtained in which the Tb(III) ion possesses a square antiprism coordination geometry with eight identical Tb–N bond lengths of 2.513(4) Å. On the other hand, if the acetic acid is added to the solution of [Tb(III)(TPP)2(H-DBU)], the protonation occurs, giving the protonated complex again. The protonation/deprotonation process is reversible as corroborated by UV-vis and IR spectra as well as cyclic voltammetry (CV) investigations. Ac magnetic susceptibility measurements were performed to study the effects of the protonation/deprotonation process on the magnetic relaxation. The results indicated that the protonated complex is not a SMM under a zero dc field without obvious χ′′(v) signals at a frequency of 1000 Hz and temperature of 2 K; however, the deprotonated complex exhibited a typical SMM behaviour under a zero dc field with the Ueff of 269 cm−1 due to the high anisotropy of CF experienced by Tb(III) ions.
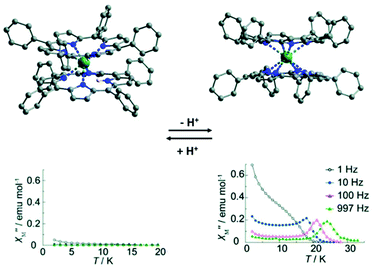 |
| Fig. 6 Crystal structures (up) and plots of χ′′(T) in the absence of a dc field (bottom) for the protonated (left) and the deprotonated (right) compounds. Reproduced with permission from ref. 64. Copyright 2012 Royal Society of Chemistry.64 | |
The other example of proton-controlled Ln-SMM behaviour is a polyoxometalate (POM)-based lanthanide complex containing a dinuclear dysprosium core reported by the Mizuno group in 2013.65 POM 1 ([{Dy(H2O)2(CH3COCH3)}2(γ-SiW10O36)2]10−) synthesized by the reaction of TBA4H4[γ-SiW10O36] (TBA = tetra-n-butylammonium) with Dy(acac)3 (acac = acetylacetonato) contains two isolated Dy(III) ions and each one is seven-coordinate with four Dy–O bonds from the [γ-SiW10O36]8− unit and the remaining three one from two inner water molecules and one outer acetone molecule possessing a distorted monocapped trigonal prism geometry, as shown in Fig. 7. When TBAOH (2 equiv.) was added to the dichloromethane solution containing POM 1 and molecular sieve 3A and this system was kept at 313 K for 1 day, colorless crystals of POM 2 ([Dy2(μ2-OH)2(γ-SiW10O36)2]12−) were obtained due to the successive dehydration condensation between two Dy(III) ions. Single crystal X-ray diffraction showed that these two Dy(III) ions in POM 2 are bridged by two μ2-OH ligands and each one is six-coordinate with four Dy–O bonds from the [γ-SiW10O36]8− unit and two from the μ2-OH ligand resulting in the change of coordination geometry from a distorted monocapped trigonal prism to distorted trigonal prism. More importantly, this chemical process is reversible benefitting from the excellent stability of the POM ligand, that is to say, if two equiv. HNO3 and a small amount of water are added to a solution mixture of acetone and acetonitrile of POM 2, the bridging ligand μ2-OH will be eliminated to form POM 1. For magnetic properties, only weak slow relaxation of magnetization was observed in POM 1 under a zero dc field due to the fast quantum-tunneling relaxation process while the typical SMM behaviour with clear frequency-dependent relaxation peaks was observed in POM 2 giving a Ueff of 65.7 K.
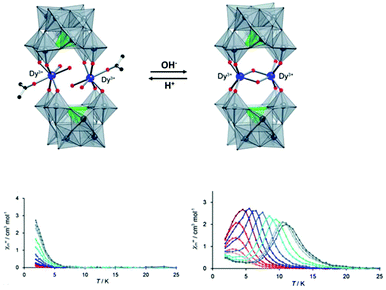 |
| Fig. 7 (Up) Reversible SC–SC transformation between POM 1 and POM 2; (bottom) plots of χ′′(T) in the absence of a dc field for POM 1 (left) and POM 2 (right). Reproduced with permission from ref. 65. Copyright 2013 Royal Society of Chemistry.65 | |
Redox-switching of Ln-SMM behaviour
In the field of molecular magnetism, the most known redox-active ligand is Pc derivatives due to the revolutionary discovery of the Pc-based Ln-SMMs in 2003. Four years later, Ishikawa and collaborators synthesized an anionic complex, [{Pc(OEt)8}2Tb]−(nBu4N)+ and a cationic complex, [{Pc(OEt)8}2Tb]+(SbCl6)−, based on the electrically neutral complex, [{Pc(OEt)8}2Tb]0 through a redox reaction.66 Note that the modification of Pc with an octaethoxy group ensures a moderate solubility. Although every compound has D4d symmetry, compared to the anionic complex, a longitudinal contraction of the coordination space of Tb(III) ions in the cationic complex was observed, leading to a significant increase of Ueff (8%) accompanied by an extension of the χ′′ peak from 40 K to 52 K at a frequency of 1000 Hz. In 2010, the Veciana group used magnetic circular dichroism (MCD) spectroscopy to study their SMM behaviour and the results showed that a greater coercive field for [{Pc(OEt)8}2Tb]+ than [{Pc(OEt)8}2Tb]− was observed, which was consistent with the results of ac magnetic susceptibility measurements.67
In addition to Pc derivatives, other redox-active ligands such as tetraoxolene and tetrathiafulvalene were also used in the design of electro-active Ln-SMMs. However, as far as we are aware, most oxidized or reduced species based on these two ligands were not isolated in the solid state preventing possible simultaneous investigation of SMM behaviour and redox activity.68–71 In 2018, J. van Slageren and collaborators succeeded in obtaining an anionic radical-bridged dinuclear Dy(III) complex [(HBpz3)2Dy(μ-CA˙)Dy(HBpz3)2]−[CoCp2]+ (1Dy) (HBpz = hydrotris(pyrazol-1-yl)borate, CA3˙− = chloroanilate) in the solid state with enough purity by chemical reduction of the neutral compound [(HBpz3)2Dy(μ-CA)Dy(HBpz3)2]·2CH2Cl2 (2Dy) using cobaltocene as the reducing agent.72 Although the low quality of crystal limited its crystal structure, its chemical formation was confirmed by ESI-MS (positive and negative mode), IR spectra and elemental analysis. Static magnetic susceptibility measurements showed that an obvious increase of χT value was observed for 1Dy when the temperature was cooled to 50 K indicating a strong magnetic interactions between Dy(III) ions and π radicals, whereas 2Dy did not display similar behaviour due to the long Dy⋯Dy distance of 8.64(9) Å causing relatively weak magnetic interactions. Dynamic magnetic susceptibility measurements were also performed to study their magnetic relaxation and the results suggested that only 2Dy displayed SMM behaviour with clear χ′′ peaks under a zero dc field.
Another method of constructing redox-switching of Ln-SMMs is to employ the redox activity of 3d or 4d transition metals.73–75 Herein, a typical example reported by the Nippe group in 2017 was introduced.76 As shown in Fig. 8a, the FeCp2 ligand was used to associate with lanthanide ions aiming to modulate the dynamic magnetic properties by its redox properties. The trinuclear compounds [1]− and [2]− were synthesized by the reaction between two equivalents of [K2(OEt2)]fc[NSi(t-Bu)Me2]2 and one equivalent of LnI3. Although the complete crystal structures of these two compounds were not obtained due to the presence of severe disorder, the important structural feature of equivalent distances between the lanthanide ion and the two Fe2+ ions can be determined. When half an equivalent of iodine was added, the oxidation reaction occurred to yield the corresponding neutral compounds, 1 and 2, where the mixed valence of Fe3+/Fe2+ was observed. Single crystal X-ray diffraction showed that these two neutral compounds are isostructural featuring an inequivalent Ln⋯Fe distance and the lanthanide ion was surrounded by a lower symmetry coordination environment. Ac magnetic susceptibility measurements confirmed the SMM behaviour of [1]− under a zero dc field with a Ueff of 39.3 K; however, this behaviour was switched off in 1 owing to the appreciable loss of symmetry and the change in CF upon oxidation. When a 1000 Oe dc field was applied, both [1]− and 1 exhibited a field-induced SMM behaviour but a faster magnetic relaxation was observed in 1. For both Er(III) compounds, [2]− and 2, no reasonable χ′′ signals were observed under a zero dc field at an ac frequency of 1000 Hz; however, a typical field-induced SMM behaviour was observed for [2]− in the presence of a 500 Oe dc field while 2 exhibited no evidence of slow relaxation at any investigated temperature or field (Fig. 8b).
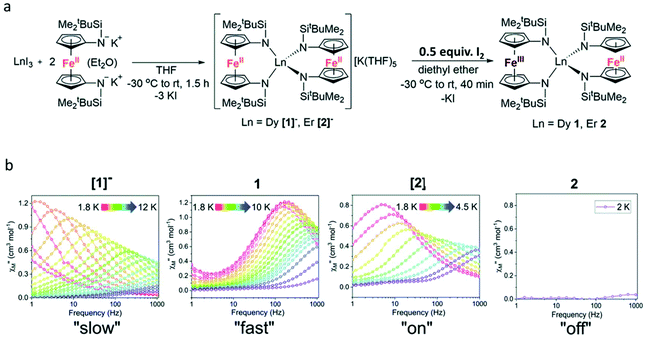 |
| Fig. 8 (a) Synthesis of [1]−, 1, [2]−, and 2; (b) plots of χ′′(v) for [1]− and 1 under a 1000 Oe dc field and [2]− and 2 under a 500 Oe dc field. Reproduced with permission from ref. 76. Copyright 2017 Royal Society of Chemistry.76 | |
Conclusions and perspectives
In recent years, organometallic chemistry have helped researchers obtain many eye-catching results in the field of molecular magnetism, some of which represented the state-of-the-art achievements; however, it also hampered the addition of other physical properties to build multi-responsive molecular magnetic materials as most such Ln-SMMs were air-sensitive. In this review, we mainly discussed four external stimuli switching of SMM behaviour, i.e. light irradiation, thermal treatment, protonation/deprotonation and oxidation/reduction. No matter what kind of modulation, any slight change of the first coordination environment of lanthanide ions usually has remarkable impact on the magnetic relaxation making the manipulation of On/Off switching of Ln-SMM behaviour possible. On the other hand, for some structural changes which occurred far from the centre metal, such as the photoisomerization of DTE, they can also alter the magnetic properties through the change of intramolecular magnetic interactions, diplolar interactions, crystal packing, etc. as lanthanides are very sensitive to the subtle changes of structure. Most examples involved here have achieved reversible SC–SC transformation, which is very vital for determining the changes in the molecular structure and the further investigations of magneto-structural correlations by ab initio calculations. In fact, apart from these examples, other dynamic systems on the subject of this review have also been reported such as solvato-switching of SMM behaviour in low-dimensional lanthanide compounds,77 the effects of guest-exchange on the magnetic relaxation,78 multistimuli-responsive Ln-SMMs,79etc. Recently, the ultrafast transient absorption spectra were used to unveil the mechanism of the effects of ultrafast photoexcitation on the magnetic relaxation in a very simple Mn3 SMM, providing new possibilities for optical control of the magnetization in SMMs on femtosecond timescales.80
From the perspective of the practical applications of Ln-SMMs, designing and building external stimuli modulated high-temperature Ln-SMMs with highly efficient reversible transformation and enough chemical stability in solid and solution will become the focus of future research.
Conflicts of interest
There are no conflicts to declare.
Acknowledgements
We thank the National Natural Science Foundation of China (Grants 21525103 and 21871247) and Key Research Program of Frontier Sciences, CAS (Grant ZDBS-LY-SLH023) for financial support. J. T. gratefully acknowledges support of the Royal Society-Newton Advanced Fellowship (NA160075).
Notes and references
- E. Coronado, Molecular magnetism: from chemical design to spin control in molecules, materials and devices, Nat. Rev. Mater., 2020, 5, 87–104 CrossRef.
- E. Coronado, J. R. Galán-Mascarós, C. J. Gómez-García and V. Laukhin, Coexistence of ferromagnetism and metallic conductivity in a molecule-based layered compound, Nature, 2000, 408, 447–449 CrossRef CAS PubMed.
- C. Train, R. Gheorghe, V. Krstic, L.-M. Chamoreau, N. S. Ovanesyan, G. L. J. A. Rikken, M. Gruselle and M. Verdaguer, Strong magneto-chiral dichroism in enantiopure chiral ferromagnets, Nat. Mater., 2008, 7, 729–734 CrossRef CAS PubMed.
-
M. Perfetti, F. Pointillart, O. Cador, L. Sorace and L. Ouahab, Molecular Magnetic Materials, Wiley-VCH Verlag GmbH & Co. KGaA, 2017, pp. 345–368 Search PubMed.
- L. Bogani and W. Wernsdorfer, Molecular spintronics using single-molecule magnets, Nat. Mater., 2008, 7, 179 CrossRef CAS PubMed.
- D. Aguilà, L. A. Barrios, V. Velasco, O. Roubeau, A. Repollés, P. J. Alonso, J. Sesé, S. J. Teat, F. Luis and G. Aromí, Heterodimetallic [LnLn’] Lanthanide Complexes: Toward a Chemical Design of Two-Qubit Molecular Spin Quantum Gates, J. Am. Chem. Soc., 2014, 136, 14215–14222 CrossRef PubMed.
- K. S. Pedersen, A.-M. Ariciu, S. McAdams, H. Weihe, J. Bendix, F. Tuna and S. Piligkos, Toward Molecular 4f Single-Ion Magnet Qubits, J. Am. Chem. Soc., 2016, 138, 5801–5804 CrossRef CAS PubMed.
- R. Hussain, G. Allodi, A. Chiesa, E. Garlatti, D. Mitcov, A. Konstantatos, K. S. Pedersen, R. De Renzi, S. Piligkos and S. Carretta, Coherent Manipulation of a Molecular Ln-Based Nuclear Qudit Coupled to an Electron Qubit, J. Am. Chem. Soc., 2018, 140, 9814–9818 CrossRef CAS PubMed.
- Z. Hu, B.-W. Dong, Z. Liu, J.-J. Liu, J. Su, C. Yu, J. Xiong, D.-E. Shi, Y. Wang, B.-W. Wang, A. Ardavan, Z. Shi, S.-D. Jiang and S. Gao, Endohedral Metallofullerene as Molecular High Spin Qubit: Diverse Rabi Cycles in Gd2@C79N, J. Am. Chem. Soc., 2018, 140, 1123–1130 CrossRef CAS PubMed.
- M. Atzori and R. Sessoli, The Second Quantum Revolution: Role and Challenges of Molecular Chemistry, J. Am. Chem. Soc., 2019, 141, 11339–11352 CrossRef CAS PubMed.
- N. Ishikawa, M. Sugita, T. Ishikawa, S.-Y. Koshihara and Y. Kaizu, Lanthanide Double-Decker Complexes Functioning as Magnets at the Single-Molecular Level, J. Am. Chem. Soc., 2003, 125, 8694–8695 CrossRef CAS PubMed.
- F.-S. Guo, A. K. Bar and R. A. Layfield, Main Group Chemistry at the Interface with Molecular Magnetism, Chem. Rev., 2019, 119, 8479–8505 CrossRef CAS PubMed.
- Z. Zhu, M. Guo, X.-L. Li and J. Tang, Molecular magnetism of lanthanide: Advances and perspectives, Coord. Chem. Rev., 2019, 378, 350–364 CrossRef CAS.
- F.-S. Guo, B. M. Day, Y.-C. Chen, M.-L. Tong, A. Mansikkamäki and R. A. Layfield, Magnetic hysteresis up to 80 kelvin in a dysprosium metallocene single-molecule magnet, Science, 2018, 362, 1400–1403 CrossRef CAS PubMed.
-
Z. Zhu and J. Tang, in Organometallic Magnets, ed. V. Chandrasekhar and F. Pointillart, Springer International Publishing, Cham, 2019, pp. 191–226 Search PubMed.
- J.-L. Liu, Y.-C. Chen and M.-L. Tong, Symmetry strategies for high performance lanthanide-based single-molecule magnets, Chem. Soc. Rev., 2018, 47, 2431–2453 RSC.
- L. Ungur and L. F. Chibotaru, Strategies toward High-Temperature Lanthanide-Based Single-Molecule Magnets, Inorg. Chem., 2016, 55, 10043–10056 CrossRef CAS PubMed.
- K. S. Pedersen, J. Dreiser, H. Weihe, R. Sibille, H. V. Johannesen, M. A. Sørensen, B. E. Nielsen, M. Sigrist, H. Mutka, S. Rols, J. Bendix and S. Piligkos, Design of Single-Molecule Magnets: Insufficiency of the Anisotropy Barrier as the Sole Criterion, Inorg. Chem., 2015, 54, 7600–7606 CrossRef CAS PubMed.
- A. Lunghi, F. Totti, R. Sessoli and S. Sanvito, The role of anharmonic phonons in under-barrier spin relaxation of single molecule magnets, Nat. Commun., 2017, 8, 14620 CrossRef PubMed.
- L. Escalera-Moreno, J. J. Baldovi, A. Gaita-Arino and E. Coronado, Spin states, vibrations and spin relaxation in molecular nanomagnets and spin qubits: a critical perspective, Chem. Sci., 2018, 9, 3265–3275 RSC.
- C. A. P. Goodwin, F. Ortu, D. Reta, N. F. Chilton and D. P. Mills, Molecular magnetic hysteresis at 60 kelvin in dysprosocenium, Nature, 2017, 548, 439–442 CrossRef CAS PubMed.
- J. D. Rinehart and J. R. Long, Exploiting single-ion anisotropy in the design of f-element single-molecule magnets, Chem. Sci., 2011, 2, 2078–2085 RSC.
- Y.-N. Guo, L. Ungur, G. E. Granroth, A. K. Powell, C. Wu, S. E. Nagler, J. Tang, L. F. Chibotaru and D. Cui, An NCN-pincer ligand dysprosium single-ion magnet showing magnetic relaxation via the second excited state, Sci. Rep., 2014, 4, 5471 CrossRef CAS PubMed.
- R. J. Blagg, L. Ungur, F. Tuna, J. Speak, P. Comar, D. Collison, W. Wernsdorfer, E. J. L. McInnes, L. F. Chibotaru and R. E. P. Winpenny, Magnetic relaxation pathways in lanthanide single-molecule magnets, Nat. Chem., 2013, 5, 673–678 CrossRef CAS PubMed.
- M. J. Giansiracusa, A. K. Kostopoulos, D. Collison, R. E. P. Winpenny and N. F. Chilton, Correlating blocking temperatures with relaxation mechanisms in monometallic single-molecule magnets with high energy barriers (Ueff > 600 K), Chem. Commun., 2019, 55, 7025–7028 RSC.
- A. E. Thorarinsdottir and T. D. Harris, Metal–Organic Framework Magnets, Chem. Rev., 2020 DOI:10.1021/acs.chemrev.9b00666.
- D. Maspoch, D. Ruiz-Molina, K. Wurst, N. Domingo, M. Cavallini, F. Biscarini, J. Tejada, C. Rovira and J. Veciana, A nanoporous molecular magnet with reversible solvent-induced mechanical and magnetic properties, Nat. Mater., 2003, 2, 190–195 CrossRef CAS PubMed.
- O. Sato, T. Iyoda, A. Fujishima and K. Hashimoto, Photoinduced Magnetization of a Cobalt-Iron Cyanide, Science, 1996, 272, 704–705 CrossRef CAS PubMed.
- J. Wang, J. J. Zakrzewski, M. Heczko, M. Zychowicz, K. Nakagawa, K. Nakabayashi, B. Sieklucka, S. Chorazy and S.-I. Ohkoshi, Proton Conductive Luminescent Thermometer Based on Near-Infrared Emissive {YbCo2} Molecular Nanomagnets, J. Am. Chem. Soc., 2020, 142, 3970–3979 CrossRef CAS PubMed.
- F. Houard, Q. Evrard, G. Calvez, Y. Suffren, C. Daiguebonne, O. Guillou, F. Gendron, B. Le Guennic, T. Guizouarn, V. Dorcet, M. Mannini and K. Bernot, Chiral Supramolecular Nanotubes of Single-Chain Magnets, Angew. Chem., 2020, 59, 780–784 CrossRef CAS PubMed.
- E. Coronado, M. C. Giménez-López, G. Levchenko, F. M. Romero, V. García-Baonza, A. Milner and M. Paz-Pasternak, Pressure-Tuning of Magnetism and Linkage Isomerism in Iron(II) Hexacyanochromate, J. Am. Chem. Soc., 2005, 127, 4580–4581 CrossRef CAS PubMed.
- J. Long, Y. Guari, R. A. S. Ferreira, L. D. Carlos and J. Larionova, Recent advances in luminescent lanthanide based Single-Molecule Magnets, Coord. Chem. Rev., 2018, 363, 57–70 CrossRef CAS.
- O. Cador, B. Le Guennic and F. Pointillart, Electro-activity and magnetic switching in lanthanide-based single-molecule magnets, Inorg. Chem. Front., 2019, 6, 3398–3417 RSC.
- S. Decurtins, P. Gütlich, C. P. Köhler, H. Spiering and A. Hauser, Light-induced excited spin state trapping in a transition-metal complex: The hexa-1-propyltetrazole-iron(II) tetrafluoroborate spin-crossover system, Chem. Phys. Lett., 1984, 105, 1–4 CrossRef CAS.
- M. Nihei, Y. Suzuki, N. Kimura, Y. Kera and H. Oshio, Bidirectional Photomagnetic Conversions in a Spin-Crossover Complex with a Diarylethene Moiety, Chem. – Eur. J., 2013, 19, 6946–6949 CrossRef CAS PubMed.
- M. Milek, F. W. Heinemann and M. M. Khusniyarov, Spin Crossover Meets Diarylethenes: Efficient Photoswitching of Magnetic Properties in Solution at Room Temperature, Inorg. Chem., 2013, 52, 11585–11592 CrossRef CAS PubMed.
- B. Rösner, M. Milek, A. Witt, B. Gobaut, P. Torelli, R. H. Fink and M. M. Khusniyarov, Reversible Photoswitching of a Spin-Crossover Molecular Complex in the Solid State at Room Temperature, Angew. Chem., Int. Ed., 2015, 54, 12976–12980 CrossRef PubMed.
- M. Estrader, J. Salinas Uber, L. A. Barrios, J. Garcia, P. Lloyd-Williams, O. Roubeau, S. J. Teat and G. Aromí, A Magneto-optical Molecular Device: Interplay of Spin Crossover, Luminescence, Photomagnetism, and Photochromism, Angew. Chem., Int. Ed., 2017, 56, 15622–15627 CrossRef CAS PubMed.
- Z.-Y. Li, J.-W. Dai, M. Damjanović, T. Shiga, J.-H. Wang, J. Zhao, H. Oshio, M. Yamashita and X.-H. Bu, Structure Switching and Modulation of the Magnetic Properties in Diarylethene-Bridged Metallosupramolecular Compounds by Controlled Coordination-Driven Self-Assembly, Angew. Chem., Int. Ed., 2019, 58, 4339–4344 CrossRef CAS PubMed.
- M. Morimoto, H. Miyasaka, M. Yamashita and M. Irie, Coordination Assemblies of [Mn4] Single-Molecule Magnets Linked by Photochromic Ligands: Photochemical Control of the Magnetic Properties, J. Am. Chem. Soc., 2009, 131, 9823–9835 CrossRef CAS PubMed.
- X. Feng, C. Mathonière, I.-R. Jeon, M. Rouzières, A. Ozarowski, M. L. Aubrey, M. I. Gonzalez, R. Clérac and J. R. Long, Tristability in a Light-Actuated Single-Molecule Magnet, J. Am. Chem. Soc., 2013, 135, 15880–15884 CrossRef CAS PubMed.
- T. Liu, H. Zheng, S. Kang, Y. Shiota, S. Hayami, M. Mito, O. Sato, K. Yoshizawa, S. Kanegawa and C. Duan, A light-induced spin crossover actuated single-chain magnet, Nat. Commun., 2013, 4, 2826 CrossRef.
- Y.-J. Ma, J.-X. Hu, S.-D. Han, J. Pan, J.-H. Li and G.-M. Wang, Manipulating On/Off Single-Molecule Magnet Behavior in a Dy(III)-Based Photochromic Complex, J. Am. Chem. Soc., 2020, 142, 2682–2689 CrossRef CAS PubMed.
- A. Prescimone, C. J. Milios, S. Moggach, J. E. Warren, A. R. Lennie, J. Sanchez-Benitez, K. Kamenev, R. Bircher, M. Murrie, S. Parsons and E. K. Brechin, [Mn6] under Pressure: A Combined Crystallographic and Magnetic Study, Angew. Chem., Int. Ed., 2008, 47, 2828–2831 CrossRef CAS PubMed.
- M. S. Norre, C. Gao, S. Dey, S. K. Gupta, A. Borah, R. Murugavel, G. Rajaraman and J. Overgaard, High-Pressure Crystallographic and Magnetic Studies of Pseudo-D5h Symmetric Dy(III) and Ho(III) Single-Molecule Magnets, Inorg. Chem., 2020, 59, 717–729 CrossRef CAS PubMed.
- J. M. Zadrozny, M. Atanasov, A. M. Bryan, C.-Y. Lin, B. D. Rekken, P. P. Power, F. Neese and J. R. Long, Slow magnetization dynamics in a series of two-coordinate iron(II) complexes, Chem. Sci., 2013, 4, 125–138 RSC.
- X.-N. Yao, J.-Z. Du, Y.-Q. Zhang, X.-B. Leng, M.-W. Yang, S.-D. Jiang, Z.-X. Wang, Z.-W. Ouyang, L. Deng, B.-W. Wang and S. Gao, Two-Coordinate Co(II) Imido Complexes as Outstanding Single-Molecule Magnets, J. Am. Chem. Soc., 2017, 139, 373–380 CrossRef CAS PubMed.
- P. C. Bunting, M. Atanasov, E. Damgaard-Møller, M. Perfetti, I. Crassee, M. Orlita, J. Overgaard, J. van Slageren, F. Neese and J. R. Long, A linear cobalt(II) complex with maximal orbital angular momentum from a non-Aufbau ground state, Science, 2018, 362, eaat7319 CrossRef CAS PubMed.
- M. Hojorat, H. Al Sabea, L. Norel, K. Bernot, T. Roisnel, F. Gendron, B. L. Guennic, E. Trzop, E. Collet, J. R. Long and S. Rigaut, Hysteresis Photomodulation via Single-Crystal-to-Single-Crystal Isomerization of a Photochromic Chain of Dysprosium Single-Molecule Magnets, J. Am. Chem. Soc., 2020, 142, 931–936 CrossRef CAS PubMed.
- M. Magott, M. Reczyński, B. Gaweł, B. Sieklucka and D. Pinkowicz, A Photomagnetic Sponge: High-Temperature Light-Induced Ferrimagnet Controlled by Water Sorption, J. Am. Chem. Soc., 2018, 140, 15876–15882 CrossRef CAS PubMed.
- H. K. Bisoyi and Q. Li, Light-Driven Liquid Crystalline Materials: From Photo-Induced Phase Transitions and Property Modulations to Applications, Chem. Rev., 2016, 116, 15089–15166 CrossRef CAS PubMed.
- T. Shiga, H. Miyasaka, M. Yamashita, M. Morimoto and M. Irie, Copper(II)-terbium(III) Single-Molecule Magnets linked by photochromic ligands, Dalton Trans., 2011, 40, 2275–2282 RSC.
- G. Cosquer, M. Morimoto, M. Irie, A. Fetoh, B. K. Breedlove and M. Yamashita, Photo-control of the magnetic properties of Dy(III) and Ho(III) homometal coordination polymers bridged by a diarylethene ligand, Dalton Trans., 2015, 44, 5996–6002 RSC.
- D. Pinkowicz, M. Ren, L.-M. Zheng, S. Sato, M. Hasegawa, M. Morimoto, M. Irie, B. K. Breedlove, G. Cosquer, K. Katoh and M. Yamashita, Control of the Single-Molecule Magnet Behavior of Lanthanide-Diarylethene Photochromic Assemblies by Irradiation with Light, Chem. – Eur. J., 2014, 20, 12502–12513 CrossRef CAS PubMed.
- L. Norel, L. E. Darago, B. Le Guennic, K. Chakarawet, M. I. Gonzalez, J. H. Olshansky, S. Rigaut and J. R. Long, A Terminal Fluoride Ligand Generates Axial Magnetic Anisotropy in Dysprosium Complexes, Angew. Chem., Int. Ed., 2018, 57, 1933–1938 CrossRef CAS PubMed.
- L.-F. Wang, J.-Z. Qiu, J.-L. Liu, Y.-C. Chen, J.-H. Jia, J. Jover, E. Ruiz and M.-L. Tong, Modulation of single-molecule magnet behaviour via photochemical [2+2] cycloaddition, Chem. Commun., 2015, 51, 15358–15361 RSC.
- L.-F. Wang, J.-Z. Qiu, Y.-C. Chen, J.-L. Liu, Q.-W. Li, J.-H. Jia and M.-L. Tong, [2+2] Photochemical modulation of the Dy(iii) single-molecule magnet: opposite influence on the energy barrier and relaxation time, Inorg. Chem. Front., 2017, 4, 1311–1318 RSC.
- J. Li, M. Kong, L. Yin, J. Zhang, F. Yu, Z.-W. Ouyang, Z. Wang, Y.-Q. Zhang and Y. Song, Photochemically Tuned Magnetic Properties in an Erbium(III)-Based Easy-Plane Single-Molecule Magnet, Inorg. Chem., 2019, 58, 14440–14448 CrossRef CAS PubMed.
- X.-D. Huang, Y. Xu, K. Fan, S.-S. Bao, M. Kurmoo and L.-M. Zheng, Reversible SC-SC Transformation involving [4+4] Cycloaddition of Anthracene: A Single-Ion to Single-Molecule Magnet and Yellow-Green to Blue-White Emission, Angew. Chem., Int. Ed., 2018, 57, 8577–8581 CrossRef CAS PubMed.
- X. Guo, G. Zhu, Z. Li, F. Sun, Z. Yang and S. Qiu, A lanthanide metal–organic framework with high thermal stability and available Lewis-acid metal sites, Chem. Commun., 2006, 3172–3174 RSC.
- Q. Zhou, F. Yang, B. Xin, G. Zeng, X. Zhou, K. Liu, D. Ma, G. Li, Z. Shi and S. Feng, Reversible switching of slow magnetic relaxation in a classic lanthanide metal–organic framework system, Chem. Commun., 2013, 49, 8244–8246 RSC.
- S. Mohapatra, B. Rajeswaran, A. Chakraborty, A. Sundaresan and T. K. Maji, Bimodal Magneto-Luminescent Dysprosium (DyIII)-Potassium (KI)-Oxalate Framework: Magnetic Switchability with High Anisotropic Barrier and Solvent Sensing, Chem. Mater., 2013, 25, 1673–1679 CrossRef CAS.
- Y. Xin, J. Wang, M. Zychowicz, J. J. Zakrzewski, K. Nakabayashi, B. Sieklucka, S. Chorazy and S. I. Ohkoshi, Dehydration-Hydration Switching of Single-Molecule Magnet Behavior and Visible Photoluminescence in a Cyanido-Bridged Dy(III)Co(III) Framework, J. Am. Chem. Soc., 2019, 141, 18211–18220 CrossRef CAS PubMed.
- D. Tanaka, T. Inose, H. Tanaka, S. Lee, N. Ishikawa and T. Ogawa, Proton-induced switching of the single molecule magnetic properties of a porphyrin based TbIII double-decker complex, Chem. Commun., 2012, 48, 7796–7798 RSC.
- K. Suzuki, R. Sato and N. Mizuno, Reversible switching of single-molecule magnet behaviors by transformation of dinuclear dysprosium cores in polyoxometalates, Chem. Sci., 2013, 4, 596–600 RSC.
- S. Takamatsu, T. Ishikawa, S.-Y. Koshihara and N. Ishikawa, Significant Increase of the Barrier Energy for Magnetization Reversal of a Single-4f-Ionic Single-Molecule Magnet by a Longitudinal Contraction of the Coordination Space, Inorg. Chem., 2007, 46, 7250–7252 CrossRef CAS PubMed.
- M. Gonidec, E. S. Davies, J. McMaster, D. B. Amabilino and J. Veciana, Probing the Magnetic Properties of Three Interconvertible Redox States of a Single-Molecule Magnet with Magnetic Circular Dichroism Spectroscopy, J. Am. Chem. Soc., 2010, 132, 1756–1757 CrossRef CAS PubMed.
- F. Gao, L. Cui, W. Liu, L. Hu, Y.-W. Zhong, Y.-Z. Li and J.-L. Zuo, Seven-Coordinate Lanthanide Sandwich-Type Complexes with a Tetrathiafulvalene-Fused Schiff Base Ligand, Inorg. Chem., 2013, 52, 11164–11172 CrossRef CAS PubMed.
- M. A. Dunstan, E. Rousset, M.-E. Boulon, R. W. Gable, L. Sorace and C. Boskovic, Slow magnetisation relaxation in tetraoxolene-bridged rare earth complexes, Dalton Trans., 2017, 46, 13756–13767 RSC.
- F. Pointillart, Y. Le Gal, S. Golhen, O. Cador and L. Ouahab, Single-Molecule Magnet Behaviour in a Tetrathiafulvalene-Based Electroactive Antiferromagnetically Coupled Dinuclear Dysprosium(III) Complex, Chem. – Eur. J., 2011, 17, 10397–10404 CrossRef CAS PubMed.
- F. Pointillart, B. L. Guennic, S. Golhen, O. Cador, O. Maury and L. Ouahab, A redox-active luminescent ytterbium based single molecule magnet, Chem. Commun., 2013, 49, 615–617 RSC.
- P. Zhang, M. Perfetti, M. Kern, P. P. Hallmen, L. Ungur, S. Lenz, M. R. Ringenberg, W. Frey, H. Stoll, G. Rauhut and J. van Slageren, Exchange coupling and single molecule magnetism in redox-active tetraoxolene-bridged dilanthanide complexes, Chem. Sci., 2018, 9, 1221–1230 RSC.
- L. Norel, M. Feng, K. Bernot, T. Roisnel, T. Guizouarn, K. Costuas and S. Rigaut, Redox Modulation of Magnetic Slow Relaxation in a 4f-Based Single-Molecule Magnet with a 4d Carbon-Rich Ligand, Inorg. Chem., 2014, 53, 2361–2363 CrossRef CAS PubMed.
- F. Meng, Y.-M. Hervault, Q. Shao, B. Hu, L. Norel, S. Rigaut and X. Chen, Orthogonally modulated molecular transport junctions for resettable electronic logic gates, Nat. Commun., 2014, 5, 3023 CrossRef PubMed.
- E. Di Piazza, L. Norel, K. Costuas, A. Bourdolle, O. Maury and S. Rigaut, d–f Heterobimetallic Association between Ytterbium and Ruthenium Carbon-Rich Complexes: Redox Commutation of Near-IR Luminescence, J. Am. Chem. Soc., 2011, 133, 6174–6176 CrossRef CAS PubMed.
- C. M. Dickie, A. L. Laughlin, J. D. Wofford, N. S. Bhuvanesh and M. Nippe, Transition metal redox switches for reversible “on/off” and “slow/fast” single-molecule magnet behaviour in dysprosium and erbium bis-diamidoferrocene complexes, Chem. Sci., 2017, 8, 8039–8049 RSC.
- W.-Y. Zhang, Y.-Q. Zhang, S.-D. Jiang, W.-B. Sun, H.-F. Li, B.-W. Wang, P. Chen, P.-F. Yan and S. Gao, Dramatic impact of the lattice solvent on the dynamic magnetic relaxation of dinuclear dysprosium single-molecule magnets, Inorg. Chem. Front., 2018, 5, 1575–1586 RSC.
- X. Zhang, V. Vieru, X. Feng, J.-L. Liu, Z. Zhang, B. Na, W. Shi, B.-W. Wang, A. K. Powell, L. F. Chibotaru, S. Gao, P. Cheng and J. R. Long, Influence of Guest Exchange on the Magnetization Dynamics of Dilanthanide Single-Molecule-Magnet Nodes within a Metal–Organic Framework, Angew. Chem., Int. Ed., 2015, 54, 9861–9865 CrossRef CAS PubMed.
- H. Tian, J.-B. Su, S.-S. Bao, M. Kurmoo, X.-D. Huang, Y.-Q. Zhang and L.-M. Zheng, Reversible ON–OFF switching of single-molecule-magnetism associated with single-crystal-to-single-crystal structural transformation of a decanuclear dysprosium phosphonate, Chem. Sci., 2018, 9, 6424–6433 RSC.
- F. Liedy, J. Eng, R. McNab, R. Inglis, T. J. Penfold, E. K. Brechin and J. O. Johansson, Vibrational coherences in manganese single-molecule magnets after ultrafast photoexcitation, Nat. Chem., 2020, 12, 452–458 CrossRef CAS PubMed.
|
This journal is © the Partner Organisations 2020 |