Environmental effects of stratospheric ozone depletion, UV radiation and interactions with climate change: UNEP Environmental Effects Assessment Panel, update 2019
Received
23rd March 2020
, Accepted 23rd March 2020
First published on 4th May 2020
Abstract
This assessment, by the United Nations Environment Programme (UNEP) Environmental Effects Assessment Panel (EEAP), one of three Panels informing the Parties to the Montreal Protocol, provides an update, since our previous extensive assessment (Photochem. Photobiol. Sci., 2019, 18, 595–828), of recent findings of current and projected interactive environmental effects of ultraviolet (UV) radiation, stratospheric ozone, and climate change. These effects include those on human health, air quality, terrestrial and aquatic ecosystems, biogeochemical cycles, and materials used in construction and other services. The present update evaluates further evidence of the consequences of human activity on climate change that are altering the exposure of organisms and ecosystems to UV radiation. This in turn reveals the interactive effects of many climate change factors with UV radiation that have implications for the atmosphere, feedbacks, contaminant fate and transport, organismal responses, and many outdoor materials including plastics, wood, and fabrics. The universal ratification of the Montreal Protocol, signed by 197 countries, has led to the regulation and phase-out of chemicals that deplete the stratospheric ozone layer. Although this treaty has had unprecedented success in protecting the ozone layer, and hence all life on Earth from damaging UV radiation, it is also making a substantial contribution to reducing climate warming because many of the chemicals under this treaty are greenhouse gases.
1. Effects on solar ultraviolet radiation due to changes in the stratospheric ozone layer and its interaction with climate
The effectiveness of the Montreal Protocol in preventing large increases in UV-B radiation (280–315 nm) at the Earth's surface has been confirmed by comparing UV measurements with model simulations of the “World Expected” and “World Avoided” scenarios at many clean-air sites. The recently reported non-compliance with the Montreal Protocol implied by increased emissions of the ozone-depleting substance (ODS) CFC-11 has had, at present, no effect on surface UV radiation, but continuing large emissions into the future could lead to environmentally important increases in UV-B radiation. While the total ozone column over Antarctica is recovering, concomitant decreases in UV-B radiation have not yet been reported. Newly published projections of future UV-B radiation generally agree with those in the last EEAP assessment report (see Bais et al.).1 Unusually high monthly mean UV Index (UVI) values reported in the Nordic Countries in 2018 were partly attributed to exceptionally long periods of cloud-free conditions, which may be indicative of climate change. Additional topics discussed include effects of changes in ozone on climate, long-term changes in UV radiation due to variations in ozone and other factors, and advances in satellite monitoring and in modelling of UV radiation.
1.1. The Montreal Protocol has prevented large increases in harmful UV radiation during the last 20 years that would have occurred if emissions of ozone-depleting substances had continued unabated
Without the Montreal Protocol, UV Index (UVI) values at northern and southern latitudes less than 50° would, as of 2018, be 10 to 20% greater in all seasons compared to UVIs observed during the early 1990s. For southern latitudes exceeding 50°, UVI values would have increased by between 25% (year-round at the southern tip of South America) and more than 100% (South Pole in spring and summer). In contrast, with the Montreal Protocol in place, measurements at multiple globally distributed clean-air sites show that the UVI has remained essentially constant over the last ca. 20 years in all seasons.
Seasonal means of the daily maximum UVI measured at the Earth's surface were compared with UVI data calculated using measurements of total ozone column (TOC) and with projections by two Chemistry Climate Models (CCM).2 The ground-based measurements were made at 17 sites (latitude range 73° N–90° S) for up to 30 years by state-of-the-art spectroradiometers. The CCMs assumed either the “World Avoided” scenario, where emissions of ODS would have continued without regulation, or the “World Expected” scenario, where ODS are curbed in compliance with the Montreal Protocol and its amendments.
Measured UVI data from clean-air sites closely match UVI values derived from the measured TOC dataset and follow the World Expected scenario within the limits of the measurement uncertainty. As of 2018, the UVI calculated in the World Avoided simulations is significantly higher than the measured UVI in the Arctic and at mid- and high latitudes in the southern hemisphere, especially in Antarctica. The situation is more complex at mid-latitudes in the northern hemisphere, where the effects of changes in aerosol and clouds mask the effects of ozone.
Without the Montreal Protocol, the UVI at high southern latitudes would have increased over the last 23 years (1995–2018) by ca. 50% per decade in spring, and by 30% per decade in summer (Fig. 1). Increases of up to 20% per decade would have been seen at northern high latitudes. At mid-latitudes, the increases would have been 5–10% per decade.
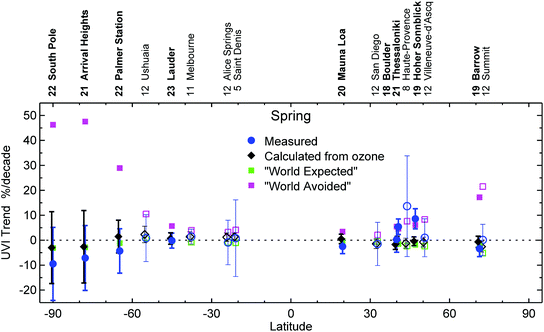 |
| Fig. 1 Decadal spring-time trends in UVI for the 17 sites analysed by McKenzie and colleagues.2 Trends in measured UVI (blue) are compared with trends calculated for clear skies from measurements of total ozone (black), and with trends calculated from total ozone derived by a chemistry-climate model according to the “World Expected” (green) and “World Avoided” (magenta) scenarios. Sites where the time series spans 20 years or more are denoted by bold text and solid symbols. The number of years of data included in the trend analysis at each site is indicated beside the site name. Error bars indicate the 95% confidence interval of the regression model. | |
Calculations of UVIs from the measured TOCs indicate that UVI values increased between 1978 and 1990 by about 5% at northern mid-latitude sites, up to 10% at southern mid-latitude sites and up to 20% at Antarctic sites. These increases are consistent with changes in UVI derived from the CCM. However, they cannot be confirmed with direct observations because measurements of UVI generally did not commence before 1990. There is some evidence that the measured UVI has decreased since 1996 in the southern hemisphere; however, these trends are generally not significant at the 95% confidence level.
1.2. Newly published projections of UV-B radiation generally agree with those in the last EEAP assessment report
The latest projections3 of the UVI for the 21st century agree well with projected changes assessed in the last EEAP Quadrennial Assessment.1 Assuming time-invariant amounts of aerosol, the clear-sky UVI is projected to decrease from 2015 to 2090 by 3% at northern and 6% at southern mid-latitudes. However, in regions that are affected by air pollution, increases will occur if emissions of air pollutants are curtailed in the future.
These new projections3 are based on results from 17 CCMs that were included in the first phase of the Chemistry-Climate Model Initiative (CCMI-1).4,5 Zonal means of noontime UVI data for cloudless skies were calculated for the period 1960–2100 with a radiative transfer model, which was driven by ozone, temperature, and aerosol fields provided by the CCMs. These projections were performed for four future scenarios of greenhouse gases (GHG) described by the Representative Concentration Pathways (RCP)† 2.6, 4.5, 6.0 and 8.5, as defined by the Intergovernmental Panel on Climate Change (IPCC).
When aerosol data provided by the CCM models were used, noontime UVIs in 2100 were projected to increase relative to values calculated for the 1960s for RCPs 2.6, 4.5 and 6.0. These increases depend on latitude and RCP scenario and range between 1% (northern high latitudes for RCP 6.0) and 8% (northern mid-latitudes for RCP 2.6), as shown in Table 1. For the “worst-case scenario”, RCP 8.5, UVIs increase only at tropical latitudes and decrease elsewhere, with the largest decrease of 8% projected at northern high latitudes.
Table 1 Comparison of zonal mean changes in clear-sky UVI calculated by Lamy et al.3 and Bais et al.1
Latitudes |
Lamy et al.3 |
Bais et al.1 |
Change [%] 1960 to 2100a |
Change [%] 2015 to 2090b |
Change [%] 2015 to 2090c |
Transient AODs, RCP = |
Fixed AODs |
Transient AODs |
Fixed AODs |
Fixed AODs |
2.6 |
4.5 |
6.0 |
8.5 |
RCP 6.0 |
RCP 6.0 |
RCP 6.0 |
RCP 6.0 |
Annual meane |
Jan |
Apr |
Jul |
Oct |
All values are rounded. According to Table 5 of Lamy et al.3 Inferred from Fig. 4 and Fig. 6 of Lamy et al.3 Table 1 of Bais et al.1 Latitude range of “High northern (N)” and “High southern (S)” refer to 60–90° in Lamy et al.3 and 60–80° in Bais et al.1 Trends reported by Lamy et al.3 refer to trends averaged over all months; Bais et al.1 provide trends for the months of January, April, July and October. |
High Nd |
6 |
2 |
1 |
−8 |
−5 |
0 |
−6 |
−3 |
−7 |
−5 |
−4 |
30–60° N |
8 |
5 |
5 |
−1 |
−2 |
5 |
−3 |
−4 |
−5 |
−3 |
−2 |
0–30° N |
3 |
3 |
3 |
1 |
3 |
— |
1 |
−1 |
0 |
−1 |
−1 |
0–30° S |
3 |
3 |
3 |
2 |
3 |
0 |
0 |
−1 |
0 |
−1 |
−1 |
30–60° S |
3 |
3 |
2 |
−2 |
0 |
−5 |
−6 |
−5 |
−4 |
−5 |
−6 |
High Sd |
7 |
6 |
4 |
0 |
−2 |
−18 |
−18 |
−8 |
−6 |
−6 |
−23 |
The aerosol optical depths (AOD) used in these projections are based on the median of AODs derived from three CCM models participating in the CCMI-1 initiative. According to these calculations, AODs are projected to decrease by almost 80% between 2000 and 2100 at high- and mid-northern latitudes, resulting in concomitant increases in the UVI of about 2% and 6%, respectively. These changes in UVI due to changes in AOD are of a similar magnitude to those caused by changes in ozone. However, these AOD estimates, as well as the absorption properties of aerosols (quantified by their “single scattering albedo” or SSA), used in these CCM models are highly uncertain because future changes in atmospheric aerosols depend greatly on policy choices such as measures to reduce air pollution.6 Moreover, changes in AOD and absorption properties of aerosols are highly dependent on region, and zonal mean changes in UVI, like those discussed above, are therefore not representative for most regions. To address these concerns, Lamy and colleagues3 also provide UVI projections for temporally invariant or “fixed” AODs based on a current climatology.7 Using this climatology and the RCP 6.0 scenario, noontime UVIs in 2100 are projected to change relative to 1960 by −5% at northern hemisphere (NH) high latitudes, −2% at NH mid latitudes, 3% in the tropical belt, 0% at southern hemisphere (SH) mid-latitudes, and −2% at SH high latitudes (Table 1, column 6).
Table 1 also shows a comparison of changes in zonal mean UVIs between 2015 and 2090 inferred from the study by Lamy and colleagues3 and as published in the last EEAP Quadrennial Assessment.1 The two studies are based on results from the CCMI-1 initiative; however, different subsets of models were used and the method to calculate the UVI from parameters provided by the CCM models differed. Furthermore, Bais and colleagues1 provided projections for different months, while Lamy and colleagues3 only considered annual averages. Despite these differences, changes in UVI calculated by the two studies for fixed AODs are consistent and project a decrease of 2–5% for northern mid-latitudes, a decrease of 4–6% for southern mid-latitudes, and almost no change for the tropics. Both studies also predict large decreases in the UVI over southern high latitudes due to the expected recovery of the stratospheric ozone ‘hole’.
Projections provided in the above two studies were corroborated by a third study that calculated changes in erythemal UV radiation over Eurasia (latitudes 40–80° N, longitudes 10° W–180° E) using a CCM developed by the Russian State Hydrometeorological University (RSHU).8 These calculations consider only changes in ozone (i.e., aerosols are fixed) and predict that erythemal UV levels in the years 2055–2059 will be lower over Eurasia by 4 to 8% relative to the reference period 1979–1983.
Using new model calculations the effect on stratospheric ozone (and by implication UV radiation) of quadrupling concentrations of atmospheric carbon dioxide (CO2) was examined.9 Such an increase would lead to a dynamically driven decrease in concentrations of ozone in the tropical lower stratosphere, an increase in lower stratosphere ozone over the high latitudes, and a chemically driven increase (via stratospheric cooling) throughout the upper stratosphere. In the tropics, a cancellation between the upper- and lower-stratospheric ozone changes means that the total column ozone response is small, with resulting small future changes in tropical UV-B radiation, if all other factors remain the same. A quadrupling of atmospheric CO2 concentrations during the 21st century is currently not expected but could occur in the 22nd century if emissions of CO2 continue unabated, according to the RCP 8.5 scenario.10 The study by Chiodo and colleagues9 suggests that even “worst-case” increases in CO2 will not result in significant increases in tropical UV-B radiation.
1.3. Record-high UV index was measured over Nordic countries in May and July 2018 when low total ozone columns coincided with an exceptionally long period of clear skies and record dry and warm conditions, which may be indicative of climate change
Relative to the long-term mean (2005–2017), monthly mean noon-time UVIs were elevated by 20 to 40% over the Nordic countries in May and July 2018.11 UVIs at Trondheim and Oslo (both Norway) and Sodankylä (Finland) exceeded the historical means by 2.1, 2.3, and 2.5 standard deviations, respectively. These large UVI anomalies cannot be explained by low TOCs alone and were partly caused by exceptionally long periods of clear skies and record dry and warm conditions. For example, at Sodankylä (67° N) the mean temperature in July 2018 was 5.6 °C above the 1981–2010 average and the sunshine duration in 2018 was 405 hours, exceeding the 1981–2010 average of 245 hours by 65%. This exceptional weather pattern was part of a record-setting heat wave that affected large parts of central and Northern Europe.12 It was caused by a stationary high-pressure system, which resulted in the blocking of westerly winds and lasted until September. The frequency of these blocking patterns has increased in recent decades and it has been suggested that this increase is a result of Arctic warming induced by climate change.13 If this is confirmed, long stretches of these weather patterns with elevated UV radiation will likely occur more frequently over the Arctic in the future.
1.4. Additional evidence that the stratospheric ozone layer is recovering over Antarctica has accumulated since our last assessment, but without a clear sign that UV radiation in Antarctica is declining
When the Antarctic ozone ‘hole’ is fully developed, ozone mixing ratios between about 13 and 21 km above sea level are close to zero and no further ozone depletion can occur in this “saturation layer”. Using various datasets (e.g., ozone sonde and satellite measurements of ozone profiles and total columns), it has been shown14 that the frequency of near-complete ozone loss in this layer clearly decreased from 2001 to 2017, revealing the emergence of an important milestone in stratospheric ozone recovery. Additional evidence using multiple metrics shows that the Antarctic ozone ‘hole’ is recovering.15 For example, trends in total ozone for September calculated for the 2001–2017 period are statistically significant at the 95% confidence level, with values ranging between 1.84 ± 1.03 and 2.83 ± 1.48 Dobson Units (DU) per year, depending on the methods used. However, at low- and mid-latitudes, increases in the total ozone column have not been observed with certainty. This is not unexpected because the removal rates of chlorine and bromine from the stratosphere are three to four times slower than the rate at which they were added. Detecting significant increases in total column ozone outside Antarctica will therefore require much more time than the detection of its previous decline.16 Furthermore, increased stratospheric aerosol loading from volcanic eruptions after 2004 impeded the rate of stratospheric ozone recovery.17
Fig. 2 shows the UVI at the South Pole for 1 September through 31 December 2018 (red line) compared with the average and range calculated from measurements of the years 1991–2017. Despite indications that the Antarctic ozone hole is healing,14,15 UVIs in October 2018 were above the long-term mean and approaching historical maxima. UVIs in November and December fluctuated about the historical average. The recovery of the Antarctic ozone ‘hole’ is more difficult to detect with UV radiation data than ozone data because signs of recovery are most pronounced in September,18 when the UVI in Antarctica is very low. In addition, the UVI is also affected by factors other than total ozone (e.g. cloud cover), which interfere with the detection of recovery.
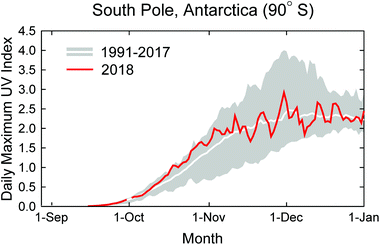 |
| Fig. 2 Daily maximum UV index (UVI) measured at the South Pole in 2018 (red line) compared with the average (white line) and the range (grey shading) of daily maximum observations of the years 1991 to 2017. The UVI was calculated from spectra measured by a SUV-100 spectroradiometer located at the South Pole. Up to 2009, the instrument was part of the NSF UV monitoring network19 and is now a node in the NOAA Antarctic UV Monitoring Network (https://www.esrl.noaa.gov/gmd/grad/antuv/). Consistent data processing methods were applied for all years.20 | |
1.5. Antarctic ozone depletion has wide-ranging effects on climate and weather in both hemispheres
A new study21 confirms that Antarctic ozone depletion has been the primary driver of climate change in the southern hemisphere during summer. However, short-term effects of the ozone ‘hole’ on weather at southern mid-latitudes are less well understood because of the difficulty in separating the influence of ozone variability from effects caused by other factors, such as variations in sea surface temperature. Stratospheric ozone depletion in the Arctic also affects the climate of the northern hemisphere, but the magnitude of the effect is smaller than in the southern hemisphere.
Son and colleagues,21 using the latest generation of CCMs from the Chemistry Climate Model Initiative (CCMI) experiments, confirm the nature and magnitude of the well-studied relationship between Antarctic ozone depletion and southern hemisphere summer climate change, including the poleward shift of the boundaries of sub-tropical and tropical climatic zones. This shift corresponds to a trend towards a positive index of the Southern Annular Mode (SAM)‡ and associated effects on temperature and precipitation in the mid-latitudes extending into the subtropics.1,22 While this long-term relationship is robust, other studies have explored whether the relationship between ozone and surface climate holds on interannual time scales, and could aid in applications such as seasonal forecasting. One new modelling study,23 also using a subset of CCMs from CCMI, investigated the nature of the relationship between the extent and depth of the ozone ‘hole’ and spring and surface temperatures during the following summer in Australia. The study concludes that the sea surface temperature (SST) could be important for driving variations in both the ozone ‘hole’ and ambient temperatures in Australia. This suggests that there may not be a causal link between the extent of the ozone ‘hole’ and temperatures in Australia on interannual time scales. Yet CCMs and climate models, in general, are complex and subject to biases, which can affect their fidelity at these spatial and temporal scales. Our understanding of the processes that determine short-term effects of the ozone ‘hole’ on weather is still incomplete.23
Using several climate models and observational constraints, a new study concludes that stratospheric ozone depletion is unlikely to have been the primary driver of the observed cooling trends in Southern Ocean SSTs.22 The same study also noted very different responses of SST to ozone change between models, with different directions of change. As above, this highlights the need for deeper investigation into model parameter sensitivities and biases.
After exhibiting an upward trend since 1979, Antarctic sea ice extent declined dramatically during austral spring 2016, reaching a record low in 2017. Several new studies examined the reasons for this reversal in the trend.24–26 None of these studies found that trends or variations in stratospheric ozone contributed to this phenomenon, in accordance with the last EEAP assessment.1 Instead, they attributed these decreases in sea ice extent to unusual atmosphere–ocean internal dynamics (with a possible contribution from forcing by anthropogenic greenhouse gases) driving warm anomalies in the upper Southern Ocean and a weakening of circumpolar winds, associated with positive convection anomalies over the tropical Indian and western Pacific Oceans.
New studies provide evidence that Arctic ozone interannual variability affects the mid-latitude Northern Hemisphere climate. From combined observations, reanalysis of data, and a CCM, a relationship between February–March Arctic ozone and April–May precipitation in Central China has been identified.27 An increase in Arctic stratospheric ozone leads to enhanced westerly winds in the high and low latitudes of the North Pacific, but weakened westerly winds in the mid-latitudes of the North Pacific. This strengthens the warm and humid airstream from the western Pacific Ocean towards the Chinese mainland. These conditions enhance precipitation in central China during positive Arctic ozone anomaly events and reduce precipitation during negative events. Another study, using the same CCM, demonstrates a relationship between Arctic ozone anomalies in March and surface temperature anomalies in central Russia and, to a lesser extent, southern Asia.28 As with the interannual relationships between stratospheric ozone and surface climate in the southern hemisphere, these conclusions need to be further supported by additional modelling studies and observations.
1.6. Several studies confirmed that changes in UV radiation during the last 20 years have generally been small
Trends in UV radiation derived from measurements and reconstructions have recently been published for several sites.29–33 These studies confirm that changes in UV radiation during the last 20 years have been small (e.g. less than ca. 4% per decade) with few exceptions, consistent with the study by McKenzie et al.2 discussed earlier.
Trends in solar spectral irradiance at 307.5 nm were calculated at several stations in Europe, Canada, and Japan over a 25-year period.31 It was found that long-term changes in UV-B radiation at 307.5 nm depend greatly on location and their main drivers are changes in aerosols and total ozone. At high northern latitudes, a part of the observed changes may also be attributed to changes in the surface reflectivity. Over Japan, the spectral irradiance at 307.5 nm has increased significantly by about 3% per decade during the past 25 years, possibly due to the corresponding significant decrease of its absorption by aerosols; the greatest part of this increase took place before the mid-2000s. The only European station where UV-B radiation increased significantly was Thessaloniki, Greece. Here, spectral irradiance at 307.5 nm grew by 3.5% per decade, with an increasing rate of change during the last decade, possibly due to decreasing absorption by aerosols.
Several other studies reported trend estimates of erythemal irradiance (or erythemal doses) at European sites. At Moscow, Russia, no statistically significant trend in erythemal UV radiation was observed over the period from 1999 to 2015.30 At Tõravere, Estonia, no significant trends in UV radiation doses have been found for the period 2004–2016 for either annual or seasonal means.29 The same is true for other factors influencing UV radiation, namely total ozone, aerosol optical depth, and global radiation, which is a proxy for cloud effects.
At Athens, Greece, sunshine duration records were used to reconstruct monthly surface solar radiation between 1900–2012.32 There were very small (0.02%) changes between 1900 and 1953, followed by a negative trend of 2% per decade during the “dimming” period of 1955–1980 and a positive trend of 1.5% per decade during the “brightening” period of 1980–2012. While these trend estimates are unrelated to changes in total ozone and do not directly translate to changes in UV radiation, they qualitatively capture variations in the effect of clouds and aerosols over the period studied, which are also relevant for changes in UV radiation. However, effects of aerosols are larger in the UV region than at visible wavelengths.
Trends of surface UV radiation over the period of 2005–2017 have been calculated for the continental United States using satellite (OMI) measurements and ground-based measurements performed at 31 sites by the United States Department of Agriculture's UV-B Monitoring and Research Program.33 This study concluded that trends in noontime erythemal irradiance estimated from these satellite and ground-based measurements cannot be reconciled. Specifically, trends derived from the satellite dataset were not significant for most of the continental United States, except for a small region in the north eastern part (states of Maine, Vermont, New Hampshire, and Massachusetts). Here, small (less than 10% per decade) positive trends were calculated from OMI data, which were significant at the 95% confidence level. However, data from the two ground-based stations located in this region indicated a significant decrease in erythemal UV over the same period. While trends calculated for several other stations were also significant, the magnitude of these trends is generally within the measurement uncertainty range, so no firm conclusions can be drawn about changes in levels of erythemal UV radiation across the continental United States. It should be noted that satellite measurements of ozone are subject to drifts and should not be used for trend estimates without additional validation. For example, satellite data should first be cross-calibrated against ground-based instruments, as for instance described by Bodeker and colleagues.34
1.7. New capabilities in measuring aerosol absorption properties in the UV-B range will improve predictions of UV-B radiation levels
In polluted areas, aerosols can have a large effect on the amount of UV radiation reaching the Earth's surface. Our ability to estimate future levels of UV radiation hinges on accurate projections of aerosol properties, such as aerosol optical depth (AOD) and single scattering albedo (SSA). In addition, our ability to assess how aerosols have affected measured UV-B irradiance in the past and to attribute observed changes in UV-B irradiance to different factors also depends on knowledge of these aerosol properties. While aerosol properties in the UV-A (315–400 nm) and visible ranges are available at many locations from measurements of NASA's AERONET network, there is a dearth of information in the UV-B spectral range. By combining measurements from several instruments (AERONET, MFRSR, and Pandora), SSAs between 305 and 870 nm have recently been derived at Seoul, South Korea.35 Similar calculations at Thessaloniki used AERONET and Brewer spectroradiometer data.36 Results from both studies are consistent with previous results37 and show that SSA is lower in the UV-B (at wavelengths between 305 and 311 nm) than in the UV-A spectral range (Fig. 3), which is likely caused by the wavelength dependence of absorption by organic aerosols.1 The wavelength-dependence derived by these studies suggests that linear extrapolation of SSA from measurements at long wavelengths to the UV-B range leads to overestimation of SSA, resulting in calculations of UV-B radiation that are too high. The methodology presented by Mok and colleagues35 has the potential to make accurate observations of SSA more readily available on a larger scale in the future, at least in areas where there is significant tropospheric pollution. Measuring SSA in the UV-B spectral range at clean-air sites will likely remain a challenge.
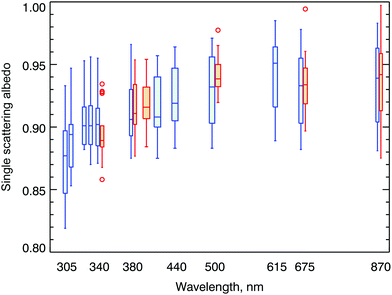 |
| Fig. 3 Spectral single scattering albedo (SSA) derived from AERONET, MFRSR, and Pandora measurements (blue symbols), as well as from SKYNET retrievals (orange symbols). Horizontal lines indicate the median, boxes indicate the interquartile range (IQR), whiskers extend to the minimum and maximum values within 1.5 × IQR, and outliers are shown as circles. Figure from Mok et al.,35 licensed under the Creative Commons Attribution 4.0 License. | |
1.8. New satellites equipped with instruments for deriving surface UV radiation give confidence that UV monitoring established by legacy instruments will continue into the future
Most estimates of the surface UV radiation from space have historically been based on NASA's Total Ozone Mapping Spectrometers (TOMS) and the Ozone Monitoring Instrument (OMI). TOMS measurements are available up to 2006, while OMI measurements on the AURA satellite started in 2004.38 The quality of OMI data has degraded over the years39 and the future of the AURA spacecraft is uncertain beyond 2023. Several satellite instruments (e.g., OMPS40 on the Suomi NPP and NOAA-20 satellites, and TROPOMI on the Sentinel-5 Precursor41) have recently been launched to continue monitoring of ozone and UV radiation from space. Furthermore, TROPOMI instruments are also expected to fly on Sentinel-4 and Sentinel-5 satellites, which are expected to launch in 2021 and 2023, respectively.42 Estimates of surface UV radiation from space measurements will therefore continue into the future.
1.9. A global climatology of atmospheric DNA-damaging fluence rates (or actinic fluxes) has been derived using radiative transfer modelling
This new climatology can help to better quantify the inactivation of microbes suspended in air. The survival rate of microorganisms, including viruses, bacteria, fungal spores, pollen, and seeds, depends on their exposure to solar UV radiation. The radiative quantity relevant to these particles when suspended in air is the fluence rate, which is independent of the direction of the incident radiation. The inactivation rate for many microbes correlates well with the fluence rate weighted by the action spectrum for DNA damage, which is dominated by wavelengths in the UV-B spectral range. Madronich and colleagues43 present an analytical formula to calculate the DNA-damaging UV fluence at altitudes between 0 and 10 km from the irradiance at ground level. This parameterisation from databases of downwelling irradiance at ground-level (available from measurements at the surface or from satellite observations) allows determination of the radiation levels relevant for microorganisms suspended in air. The new climatology of DNA-damaging UV radiation in the atmosphere (Fig. 4) is useful for studying the survival of aerial pathogens that cause diseases in humans, animals and crops, or for understanding the geographic distribution of species over evolutionary time scales.
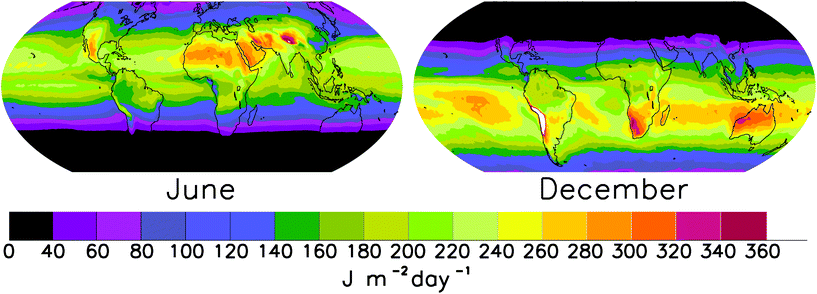 |
| Fig. 4 June and December climatology of DNA-damaging fluence rate. Adapted from Madronich et al.43 by permission of the Royal Society of Chemistry (RSC) on behalf of the European Society for Photobiology, and the European Photochemistry Association. | |
1.10. Recently reported new emissions of the ozone-depleting substance CFC-11 currently have no detectable impact on UV radiation levels at the surface but will become important if these emissions continue
New measurements and atmospheric chemical transport model simulations suggest that the recently reported slow-down in the rate of decrease in atmospheric concentrations of trichlorofluoromethane (CFC-11, a chlorofluorocarbon regulated by the Montreal Protocol)44 can be attributed to emissions from China.45,46 Specifically, Rigby et al.46 provide evidence that emissions from eastern mainland China were 7.0 ± 3.0 (±1 standard deviation) gigagrams (Gg) higher per year in 2014–2017 than in 2008–2012, and that the increase in emissions arises primarily around the north eastern provinces of Shandong and Hebei. This increase accounts for a substantial fraction (at least 40 to 60%) of the global rise in CFC-11 emissions. The increase in CFC-11 emissions is likely the result of new production and use for insulating materials. If these new emissions of CFC-11 continue, the recovery of the stratospheric ozone layer will be delayed, with consequences for UV radiation levels. At present, a temporary increase of 10–13 Gg per year will not have a detectable impact on stratospheric ozone and UV radiation levels, particularly if the emissions are quickly reduced.47 However, sustained emissions over decades, e.g. at 60–80 Gg per year, could have a large impact.
1.11. Solar radiation management schemes designed to counteract global warming would have important consequences for ecosystem services and food security
Effects on climate caused by increasing carbon dioxide (CO2) concentrations cannot be completely circumvented by reducing the solar radiation entering the atmosphere. Simulations with different climate models suggest that reducing the strength of solar radiation to offset the surface warming from a quadrupling of atmospheric CO2 would result in decreased low cloud cover, increased high cloud cover, cooling of the upper troposphere and stratosphere, and decreased water vapour concentration.48 The decrease in low clouds would require additional measures for reducing the amount of solar radiation entering the atmosphere to counteract increases in temperature at the Earth's surface.
Another recent study assessed the effects on UV and visible radiation resulting from a hypothetical solar radiation management scheme using sulfur dioxide (SO2) injected into the stratosphere to counteract the effect of warming resulting from increased GHGs under the RCP 8.5 scenario.49 This intervention would reduce the UVI on average by about 20% at 30° N and 70° N in 2080 relative to 2020. Reductions in photosynthetically active radiation (PAR) would range between 10% at 30° N and 20% at 70° N in summer, with larger changes for other seasons. These large changes in the UVI and PAR would likely have important consequences for ecosystem services and food security; however, such repercussions have not yet been quantified.
2. The effects of exposure to solar ultraviolet radiation on human health
Exposure to the sun has well-established harmful effects, including skin cancer and other skin conditions, eye disorders, and immune suppression. Benefits, beyond vitamin D production, are emerging but not yet well understood. Thus future changes in exposure to UV radiation due to stratospheric ozone depletion and recovery and/or a changing climate will have multifactorial effects on human health. However, behaviour has been and will continue to be the biggest driver of changes in sun exposure and its consequences. Time outdoors and use of sun protective strategies, such as sunscreens, will be influenced by climate change (such as temperature and weather patterns), concerns about the toxicity of sunscreens to humans and aquatic ecosystems, and changes in social norms. These will vary according to both location and culture. It is critical that models to forecast future health impacts of UV radiation take these factors into consideration in order to generate reliable policy-relevant data.
2.1. The incidence of cutaneous malignant melanoma is continuing to increase in some northern European countries, but is falling in other countries that have introduced broad-reaching skin cancer prevention programs
Australia and New Zealand continue to have the highest incidence of melanoma in the world (50.3 and 47.4 per 100
000 population, respectively, in 2015; age standardised using the United States 2000 standard population).50,51 High incidence is also seen in some northern European populations (per 100
000 population), viz. Denmark (33.9), Norway (32.7) and Sweden (29.3).50
From 2012–2015, incidence increased in United States whites, the United Kingdom, Sweden, and Norway, by 1.7% (95% confidence interval (CI) 1.2–2.2), 2.7% (95% CI 2.0–3.5), 4.8% (95% CI 4.0–5.6), and 4.5% per year (95% CI 3.8–5.2), respectively.50 In Denmark, while incidence increased similarly to Sweden and Norway from 1982–2010, it has plateaued since 2011, possibly as a result of the skin cancer prevention campaign introduced in 2007.50 Incidence is declining in New Zealand, in United States whites younger than 45 years,51 and in Australians under 60 years of age.52
A new study, incorporating incidence data from 18 European cancer registries (7 with national coverage) for the time period 1995–2012, reported an annual average percentage change (AAPC) for invasive melanoma of 4.0% for men and 3.0% for women.53 The increase in invasive lesions was driven largely by thin melanomas (≤1 mm) (AAPC 10% men; 8.3% women), but the incidence of thick (>1 mm) melanomas also increased, although more slowly in the most recent time period.
In Australia,52 Germany,53 and other European countries,54,55 the incidence of melanoma in situ (also called stage 0 melanoma) is also increasing. The incidence is highest in Australia; it is difficult, however, to know how much of the variation between countries or over time is attributable to real differences in incidence vs. differences in skin cancer awareness and/or screening and treatment practices.
2.2. Mortality due to melanoma is continuing to rise, albeit more slowly than increases in incidence
Mortality is highest in Australia and New Zealand (age-standardised mortality rate per 100
000: 6.3 and 7.5, respectively);56 the highest mortality in Europe is in Norway (ca. 6 per 100
000) and Sweden (ca. 4 per 100
000).56
Data from 18 European cancer registries for the period 1992–2012 suggest that mortality rates are continuing to increase in Northern Europe, particularly Iceland (average annual percentage change (AAPC) 5.4%), Ireland (AAPC 3.4%), and Norway (AAPC 1.6%).53 Newly reported data from Germany for the period 1990–2012 show increases in melanoma mortality at an average annual rate of 4.8% for men and 3.9% for women.57 In Australia and New Zealand, the decade to 2012 saw average annual increases in melanoma mortality rates of ca. 1.5% per annum.56 Data for the Australian state of Queensland suggest that the increases are restricted to older age groups (over 60 years).52 In the United States, mortality rates climbed slowly (AAPC 0.2%).56
2.3. Despite the high incidence of melanoma in some countries, the global burden of disease is low
Worldwide in 2018, melanoma affected ca. 300
000 people (1.6% of all new cancer cases) and was responsible for ca. 60
000 deaths (0.6% of all cancer deaths).58 In terms of disability-adjusted life years (DALYs), in 2016 melanoma contributed only 0.06% of the global burden of disease, primarily affecting white-skinned populations in Australasia, North America and Europe, and elderly and male population groups.59 The relatively low global burden in DALYs is due to the lower weighting for diseases, such as skin cancer, that particularly cause death and/or disability in older age groups and the relatively low proportion of the global population that is affected by skin cancer.
2.4. Exposure to ultraviolet radiation is the primary cause of melanomas, but the population attributable fraction varies according to geographic location, and associations with sun exposure are complex
The population attributable fraction (PAF; the proportion of melanomas attributable to exposure to UV radiation) globally was previously reported to be 75%, but it was much higher in Oceania (96%).60 Recent studies estimated PAFs for Canada (62%)61 and France (PAF 83%).62 These lower PAFs are as expected and reflect the lower intensity of UV radiation in these areas.
Among measures of exposure to UV radiation, severe sunburns in early life appear to have the strongest association with melanoma risk;63 many studies show no association with self-reported cumulative exposure. This lack of association may be due to measurement issues, with sunburn easier to recall and quantify than lifetime sun exposure. In support of this argument, there are strong associations with keratinocyte cancer (KC) and premalignant lesions that are caused by cumulative exposure.64 It is also likely that the patterns (intermittent vs. chronic) and timing of sun exposure that give rise to melanoma may differ according to the type/location of the melanoma.65–67
2.5. Keratinocyte cancers continue to be a major burden for healthcare services in light-skinned populations
The lifetime risk of basal cell carcinoma (BCC) is estimated to be ca. 20–30% in United States whites,68 and keratinocyte cancer (KC) incidence rates are predicted to increase at least until 2040 due to an aging population with historically high exposure to UV radiation. The burden is particularly high in Australia and New Zealand. New data from South Australia show a 59% increase in age-standardised incidence rates of KC between 2000 and 2015,69 and in 2008 the Auckland region of New Zealand had the highest incidence of KC in the world (1907 per 100
000 inhabitants; standardised to the 2001 Australian standard population).70
Two recent cancer registry studies reported BCC and squamous cell carcinoma (SCC) incidence trends in other populations. In mid-Brazil (source population ca. 600
000 inhabitants, with 32% skin type I–III, 56% skin type IV/V, 10% skin type VI, and 2% classified as Asian or Indigenous) the Aracaju Cancer Registry reported a stable incidence rates during the past 10 years, with age-standardised incidence rates of 130 per 100
000 people per year for BCC and 14 per 100
000 people per year for SCC.71 The nationwide South Korea Central Cancer registry (estimated completeness: 98%) reported age-standardised incidence rates (2011–2014) of 2 per 100
000 people per year for BCC and 1 per 100
000 people per year for SCC.72
It is critical to recognise that an individual often has multiple KCs, but most estimates of burden consider only ‘incident people’, rather than ‘incident lesions’. National cancer registration data from the United Kingdom showed that an additional 51% of KCs were included by counting the first KC per person per year, rather than including only the first KC in a person's lifetime.73
Premalignant lesions also contribute to the burden of KC and the incidence is increasing. Exposure to UV radiation causes the development of actinic keratosis (AK). Only a small proportion of AKs progress to SCC, but their diagnosis and treatment places a considerable burden on health systems.74 In South Korea, the incidence of AK increased from 18 to 54 per 100
000 people per year between 2006 and 2015.75 It has been estimated previously that 24% of Dutch people aged 50 years and older had at least one AK,76 and recent studies among primary care practices in Switzerland and dermatology outpatients in Spain showed comparable prevalence estimates of 25% and 29%, respectively.77,78
The large numbers of KC result in a considerable drain on healthcare resources. For example, in 2013 a total of USD 2.5 billion was spent on skin cancer-related diagnoses in United States Medicare patients (source population of 60 million beneficiaries of 65 years and older).79 Half (49%) of this spending was on BCC ($715 million) and SCC ($525 million). Per 1000 beneficiaries, 1051 AKs were treated per year, at a cost of $554 million. In Canada the increase in cost of KC management between 2004 and 2008 (12% per year) outstripped the increase in incidence during the same period (3% per year), suggesting a change in treatment practices.80
2.6. People who have received a solid organ transplant are at higher risk of keratinocyte cancer than the general population, but with newer immunosuppressive regimens the risk has decreased
A population-based study from Ontario, Canada, including 10
198 people who received a transplant between 1994 and 2012, found a 6.6-fold increased risk of KC.81 The risk was lower in those who had a transplant between 2007 and 2012 than for those whose transplant occurred in 1994–2000. Despite this falling risk, there has been a marked increase in the number of people undergoing transplantation,82 so the burden of KC in this susceptible population remains high.
2.7. As global ambient temperatures increase, the incidence of keratinocyte cancer is predicted to increase
A new study extending the work of van der Leun and colleagues83 has projected the percentage increases in skin cancer incidence that will occur as a result of warmer ambient temperatures in the future.84 The estimates were based on previously proposed mathematical relationships between: (a) the change in effective carcinogenicity of solar UV radiation dose and the change in incidence of SCC and BCC;85 and (b) the change in effective carcinogenicity of solar UV radiation in relation to the change in ambient temperature.83 Changes in ambient temperature were modelled under a range of Representative Concentration Pathways (RCPs) as developed by the Intergovernmental Panel on Climate Change (see also section 1.2). The results show up to a 21% increase in incidence of SCC and 10% increase in BCC by 2100 under the most extreme scenario (RCP 8.5). The validity of the findings depends on the validity of the underlying mathematical relationships.
2.8. Photodermatoses occur in both light- and dark-skinned populations, and have considerable negative impacts on health and well-being
Photodermatoses are non-malignant conditions of the skin caused by exposure to solar UV-B, UV-A and visible radiation. Population-based studies examining the prevalence of photodermatoses are scarce, so the true burden of disease overall and within population subgroups is difficult to capture. However, a comprehensive review of data generated mostly from single-centre clinics indicates that while certain conditions are commonly seen, the distribution of specific photodermatoses differs between light- and dark-skinned populations.86
Photodermatoses have effects on sufferers both directly through their symptoms, and indirectly via sun avoidance and treatment measures. A recent systematic review found that ca. one-third of adults and children with photodermatoses experience markedly reduced quality of life, and that anxiety and depression is twice that of the normal population.87
2.9. Exposure of the skin to UV radiation may reduce the effectiveness of some vaccinations and increase the risk of folate deficiency, with implications for public health programs
A recent study found that higher natural sun exposure measured around the time of vaccination reduced the cellular, but not the antibody, response to vaccination.88 Folate, a member of the B vitamin group, is an essential cofactor for enzymes involved in DNA synthesis. UV radiation degrades folate in vitro and in human skin. Findings from a study in Spain confirm the effect of UV radiation in field conditions; folate deficiency was ca. 40% more common in summer compared to winter, and serum folate levels varied inversely with total solar UV radiation.89 Changes in exposure to UV radiation due to either ozone recovery or climate change may thus influence vaccination effectiveness and folate deficiency.
2.10. Exposure of the eyes to UV radiation causes pterygium, the prevalence of which is very high in older people
A meta-analysis that included data from over 400
000 people from 24 countries found that the prevalence of pterygium was 12%, ranging from 1.6% in young adults (20–29 years) to 19.5% in people 80 years and older. Several factors influencing solar exposure of the eyes were associated with the risk of pterygium, including: spending more vs. less than 5 hours outdoors per day (odds ratio (OR) 1.24; 95% CI 1.11–1.36); outdoor vs. indoor occupations (OR 1.46; 95% CI 1.36–1.55); and wearing sunglasses (OR 0.47; 95% CI 0.19–0.57).90
In Ecuador, a study using Public Health Ministry data that captured the presence of pterygium in hospitalised patients found a clear correlation between modelled surface UV radiation and the prevalence of pterygium; the prevalence in the highest of the five UV radiation zones was five times higher than that in the lowest UV radiation zone.91
2.11. New evidence highlights the high prevalence of cataract in China, India, and Kenya
Current evidence suggests that exposure to UV-B radiation is associated with cortical cataract and that it may also have an effect on the development of nuclear and posterior subcapsular cataracts. A systematic review of cataract in China found that the prevalence of age-related cataract (the most common type, typically with a mixed cortical, nuclear and posterior subcapsular location) ranged from less than 5% in people aged 45–49 up to ca. 75% of those aged 85–89 years. Between 1990 and 2015 the prevalence of age-related cataract remained stable, although the number of people with cataract has doubled.92
A population-based study conducted in India between 2009 and 2011 found that the prevalence of cataract in people aged 60 years and older was ca. 40%, with no difference between rural and urban areas. In Kenya, the prevalence of visually significant (visual acuity less than 6/12) cataract in people aged 50 years and older in 2007/2008 was 18%.93 Over a 6-year follow-up period, 25% of people free of cataract at the baseline had developed a visually significant cataract, ranging from 13% in those aged 50–59 to 62% in those ≥80 years.
2.12. There is little evidence that sunlight exposure causes age-related macular degeneration
Age-related macular degeneration (AMD) results from damage to the macular region of the retina. It is a leading cause of blindness worldwide, accounting for ca. half of all cases of central blindness in older people in Western countries. A link with sunlight exposure has been posited and investigated in multiple studies, but a recent meta-analysis including 14 studies suggests that there is no significant association.94
2.13. Exposure of the skin to UV-B radiation upregulates innate immunity in the skin, providing protection from infections, but suppresses adaptive immunity both within the skin and systemically, with both beneficial and harmful effects
Within hours of exposure to UV-B radiation, pathogen-killing white blood cells (neutrophils and macrophages) infiltrate the skin; recent work shows that this effect is modulated by the skin microbiome.95 In in vitro studies, UV irradiation of neutrophils triggers the release of neutrophil extracellular traps, or “NETs”, that capture and kill bacteria.96 This immediate, non-specific cell-based attack on pathogens forms the innate immune system. In addition, the macrophages and neutrophils release a chemical (cytokine) called interleukin-10; this downregulates the adaptive immune system that is locally important in controlling skin cancer genesis. Exposure to UV-B radiation also activates regulatory T cells97,98 and induces changes in circulating B cells,99 providing a potential mechanism for the protective effect of sun exposure on the onset of autoimmune diseases such as multiple sclerosis100 and inflammatory bowel disease.101 A new study found that 20 out of 22 people who spent a week holidaying in an environment with high ambient UV radiation experienced complete suppression of contact hypersensitivity, highlighting the strong effect of UV radiation on the immune system.102
The effect of UV radiation on immunity is modified by the frequency and dose of exposure, as well as the wavelength. Even low doses of UV-B radiation, especially when delivered concomitantly with immune modulators, such as alcohol, can suppress the immune system.103 However, a recent study showed that repeated daily exposure of mice to very low doses of UV-B radiation (ca. 0.1 MED) actually enhanced systemic adaptive immune responses.104 This non-linear dose response to UV-B irradiation is also seen with UV-A, where moderate, but not high or low doses, are potently immune suppressive.105–107 Thus, the effect of exposure to UV radiation on immunity is likely to be dictated by not just the type of UV irradiation (UV-A or UV-B) but the dose (very low, moderate or high) and frequency of exposure.
2.14. Generation of vitamin D is the most well-recognised benefit of exposure to UV radiation, but the role of vitamin D in health, other than for bone, remains controversial
The most notable new data regarding vitamin D and health come from a randomised controlled trial of vitamin D and omega-3 fatty acid supplementation among 25
871 adults in the United States.108 Vitamin D supplementation did not alter the risk of either cancer or cardiovascular disease. These findings are supported by two meta-analyses,109 although one found some evidence that vitamin D supplementation reduces cancer mortality.110 In contrast, a Mendelian randomisation study found no association between genetically determined 25 hydroxy vitamin D (25(OH)D) concentration and cancer mortality.111 Several new Mendelian randomisation studies have also found no association between genetically determined 25(OH)D concentration and melanoma, low birthweight,112 low bone mineral density,113 depression,114,115 type 2 diabetes,116 fatigue,117 colorectal cancer,15 and inflammatory bowel disease,118 suggesting that links seen in observational studies may not be causal.
2.15. There is marked variability in the prevalence of vitamin D deficiency around the world
Based on bone health indicators, many authorities regard sufficient vitamin D status as 25(OH)D > 50 nmol L−1, insufficient status as 30–49 nmol L−1, and deficient status as <30 or <25 nmol L−1.119 Comparing the prevalence of vitamin D deficiency between countries and over time continues to be challenging due to the use of inaccurate and imprecise laboratory assays, and bias resulting from selecting participants that do not represent the resident population. However, there are now reliable data for some countries. A recent review has summarised the vitamin D status in Europe and the Middle East.120 The prevalence of vitamin D deficiency in European populations varies. It is particularly low in most Nordic countries; with the exception of Iceland, the prevalence of 25(OH)D < 30 nmol L−1 is less than 1%, and up to 20% of people have 25(OH)D < 50 nmol L−1. This is largely ascribed to the use of cod-liver oil, vitamin D supplements, and food fortification (in Finland). Conversely, the prevalence in the United Kingdom is high; over half of those included in a population-based survey in 2008–2012 had 25(OH)D < 50 nmol L−1, and ca. 15% of adults had <30 nmol L−1.
There are few population-based studies from the Middle East but, in general, the prevalence of vitamin D deficiency is higher in the Middle East than in Europe and is particularly high in women due to the clothing style.120
In the United States National Health and Nutrition Survey the prevalence of 25(OH)D < 30 nmol L−1 between 2011 and 2014 in adults and children was 5%.121 In adults it ranged from 7.6% in those aged 20–39 years to 2.9% in people 60 years or older. It was much higher in non-Hispanic blacks compared with whites (17.5% vs. 2.1%). The prevalence of 25(OH)D of 30–49 nmol L−1 was 18.3%, but with marked variability according to age, race, and use of vitamin D supplements. There was no evidence of a change in the prevalence of 25(OH)D < 30 nmol L−1 from 2003/2004, but a modest decline in the prevalence of 25(OH)D of 30–49 nmol L−1 occurred (21% to 18%).
2.16. Brief, daily noon-time sun exposures in March–September in the United Kingdom maintain year-round vitamin D status in white Caucasians but not in South Asians with skin type V
A modelling study estimated that across the United Kingdom (latitude 50–60°N), white Caucasians require ca. 9 minutes of noon sunlight exposure daily from March–September to maintain 25(OH)D ≥ 25 nmol L−1 year round.122 The model assumed 35% body surface exposure from June to August (e.g., wearing shorts and a short-sleeved shirt) and exposure of face and hands in other months. While a similar modelling study suggested that ca. 25 minutes at these times may be sufficient in people with skin type V,123 an earlier in vivo study found that sun exposure was unlikely to maintain adequate vitamin D and that supplementation may be required.124 UV radiation during the 21st century is projected to remain within a few percent of current levels. This implies that adequate vitamin D status will be achievable at least for white Caucasians throughout this century.
2.17. New studies suggest beneficial effects of exposure to UV radiation, but it is difficult to ascertain if these are mediated through vitamin D or other pathways
Beneficial effects of higher levels of sun exposure have been suggested for: non-Hodgkin lymphoma (20% decrease in risk for high vs. low exposure in a meta-analysis, with null association with 25(OH)D level or vitamin D supplementation);125 reduced risk of kidney cancer (15% reduction in odds in the high vs. unexposed/low exposed groups; involvement of vitamin D not tested);126 lower blood pressure and reduced risk of cardiovascular disease (systematic review without meta-analysis; protective effect persisted after adjustment for 25(OH)D level where those data were available);127 reduced risk of onset128 or active disease post-diagnosis129 for multiple sclerosis, the latter persisting following adjustment for 25(OH)D level; and around 40% reduced odds of developing oesophageal or gastric cancer (for the highest tertile of annual ambient UV-B radiation dose compared to the lowest) in a data linkage study from the United Kingdom (vitamin D status parameters not considered).130
2.18. Multi-component programs for skin cancer prevention improve sun protection behaviours, and there is reduced prevalence of sunburn in some countries
A comprehensive review confirmed that sustained multi-component interventions can increase the adoption of sun protection measures. These interventions included education, policy, formal recognition of prevention efforts, provision of equipment, and reminders in contexts where sun exposure is likely.131 Evidence for the use of the UV Index to promote sun protection was equivocal, but there is limited research on the UV index in isolation from other intervention components.
Rates of sunburn in the United States have not decreased, but there is evidence of a decline in some other countries. Analysis of data from the National Health and Nutrition Surveys in the United States shows no change between 2005 and 2015 in the proportion of respondents reporting at least one sunburn in the previous 12 months (34% in both years).132 In Australia, a different measure is used so the prevalence is not directly comparable, but between 2003/2004 and 2016/2017 the proportion of people who reported being sunburnt on the previous weekend (in summer) decreased from 14% to 11%.133 This decrease was accompanied by an increase in the proportion of people who reported using two or more sun protection behaviours (from 41% to 45%). A sun safety campaign was launched in Denmark in 2007; from then until 2015 there was a 1% decrease per annum in the proportion of people reporting a sunburn in the previous 12 months.134
2.19. Sunscreen use can reduce erythema while allowing vitamin D production
A study conducted among holiday makers spending one week in a sunny environment found that the provision of one of two SPF ca. 15 sunscreens, along with instructions about optimal use, resulted in less erythema than in people assigned to discretionary sunscreen use. The effect was the same for the two sunscreens, one of which had greater absorption in the UV-A range.135 In the discretionary sunscreen use group the 25(OH)D concentration increased by 28 nmol L−1 between the beginning and end of the holiday; in the sunscreen group using the high UV-A protection sunscreen group the increase was 19 nmol L−1, whereas in the low UV-A protection group it was 13 nmol L−1. These results suggest that a clinically relevant increase in 25(OH)D can be obtained even when sunscreen is applied optimally.136
A systematic review found that experimental studies using artificially generated UV radiation show a marked reduction of vitamin D production when sunscreen is applied prior to exposure.137 However, these studies have limitations, particularly related to the UV radiation spectrum used. Most observational studies show either no association between sunscreen use and 25(OH)D concentration, or that 25(OH)D concentrations are higher in sunscreen users. In two randomised controlled trials of sunscreen use, with sunscreen of SPF ca. 17, there was no difference in 25(OH)D concentration between daily sunscreen users and control groups. It is not known if the use of high SPF sunscreens in environments with moderate ambient UV radiation can lower 25(OH)D concentration and increase the risk of vitamin D deficiency.
2.20. Sunscreen applied under maximal use conditions can result in plasma concentrations of sunscreen ingredients that exceed the United States Food and Drug Administration threshold for potentially waiving some non-clinical toxicology studies
In a phase one study, 24 volunteers were randomised to the application of one of four sunscreens, applied four times per day to 75% of the body surface area for four days.138 There was evidence of sunscreen absorption into the bloodstream at levels that suggest further safety testing is warranted. These observations are consistent with two other studies.139,140 These results do not indicate that people should refrain from sunscreen use, but that studies are needed to determine if these findings are clinically significant. A randomised controlled trial of daily sunscreen use for 4.5 years, with 21 years of follow-up, found no evidence that frequent application of SPF 15+ sunscreen altered overall or cause-specific mortality.141
2.21. Sunscreen should be applied daily to exposed skin on days when the UV index is forecast to reach at least 3, but protection may also be required when the UV index is less than 3
At a Sunscreen Summit held in Australia in 2018, the evidence for and against daily sunscreen use was considered by scientists, clinicians, and regulatory and public health bodies.142 The consensus was that the benefits of routine sunscreen use to reduce exposure to UV radiation during incidental activities outweigh the potential harms, and that sunscreen should be applied routinely on all days when the UV Index is forecast to reach at least 3.
Some health agencies currently recommend that no sun protection is required when the UV Index is less than 3. A new study demonstrates that this advice should be modified, particularly for mid-latitude conditions. For days when the peak UV Index is below this threshold, the daily dose of sunburning UV radiation available can exceed 15 standard erythemal doses (SEDs) and frequently exceeds the 2 SED threshold that causes erythema to lightly pigmented skin. This may have important health consequences, as populations at mid-latitudes include a significant proportion with fair skin that is susceptible to damage.143,144 These conclusions have recently been corroborated by a study based on data measured at nine stations in Germany.145,146
3. Plant and terrestrial ecosystem response to changes in UV radiation and climate
Future changes in surface solar UV radiation will depend on several factors, including the extent and rate of stratospheric ozone recovery, the degree to which air pollution is controlled, and the interacting effects of climate change on stratospheric ozone, clouds, and land cover. These combined changes in solar UV radiation and climate will have direct effects on terrestrial plants and ecosystems and also modify how organisms respond to these changes. These alterations in UV radiation and climate are concurrent with changing patterns of land use and shifts in the distributions of native and non-native plants along elevational and latitudinal gradients, with potential threats to biodiversity and food security.
3.1. Extreme losses in crop productivity would have occurred without the Montreal Protocol
An assessment based on results from field studies conducted at high-latitude locations indicates that plant productivity declines by about 3% for every 10% increase in biologically weighted UV-B radiation.147 If this linear relationship were to hold at all levels of stratospheric ozone depletion, these findings suggest that losses in crop productivity in the absence of the Montreal Protocol (i.e. “World Avoided” scenarios1,2) would have been severe (>90%).148 However, considerable uncertainty exists in these extrapolations, as this relationship may not necessarily hold for all plants, including agricultural species, at all latitudes, and it is unknown how productivity would be affected by the interactive effects of very high levels of UV-B radiation under different climate change scenarios.
3.2. Changes in climate and land use are disrupting tropical ecosystems and increasing the vulnerability of these systems to interacting effects of UV radiation and climate
UV radiation and its effects need to be placed in the context of current and predicted rapid climate change events, as rising temperatures and other changes in climate shape the environment of plants and animals.149
To date, climate change research has largely concentrated on environmental effects in mid- and high-latitude regions and far less in tropical areas.150 Tropical mountain ecosystems provide valuable services in terms of food, carbon sinks, and community livelihoods.151 These systems are also susceptible to decreases in the abundance and biodiversity of species as climate change and other environmental factors cause shifts in habit distribution.152–155 For example, a tropical pasture grass in the Ecuadorian Andes is being outcompeted by an invasive fern that appears to be better adapted to higher UV radiation and temperature extremes along elevational gradients.156
3.3. Small increases in UV-B radiation due to projected decreases in stratospheric ozone in the tropics may reduce crop production and the quality of some foods
Global stratospheric ozone is projected to recover to pre-1980 levels in the near future, assuming full compliance with the Montreal Protocol, although some models project small but variable decreases in stratospheric ozone over the tropics.157 If future emissions and aerosol loading are reduced, this would increase the UV radiation received by terrestrial plants and ecosystems in many regions, although only the high RCP 8.5 scenario would result in an increased UV radiation in the tropics (see section 1.2).
The consequences of relatively modest increases in UV-B radiation on tropical crops or wild plants are currently uncertain. However, available evidence suggests that while current levels of UV-B radiation can alter the morphology (e.g., smaller leaves, reduced shoot height) and chemistry (increased flavonoid levels) of native tropical plants, biomass production is rarely decreased in these species (e.g.ref. 158). In comparison, several field experiments conducted using filters to reduce solar UV-B radiation have shown that certain varieties of introduced temperate-zone crops (e.g. wheat, soybean)159–161 can exhibit decreases in photosynthesis and yield when exposed to ambient UV-B radiation in the tropics. These findings suggest that some crop species now grown in the tropics may be less adapted to small increases in UV-B radiation than the native species.
3.4. Climate change and UV radiation can potentially interact to affect biodiversity by altering competition among plants and their interactions with pests
Many plant and animal species are migrating or shifting their distribution ranges to higher elevations and latitudes in response to ongoing changes in climate.162 These changes in distribution expose organisms to higher or lower solar UV radiation. The shifts in distribution range are often most pronounced for non-native, invasive species.163–165 It is unclear at present to what extent native and non-native species respond to changes in UV-B radiation,166–170 although changes in exposure to UV-B radiation resulting from shifts in distribution patterns have the potential to reduce biodiversity in several ways.171 For example, because UV-protective compounds (e.g. flavonoids) and herbivore defences generally vary along elevational and latitudinal gradients,168,172–178 changes in exposure to UV radiation resulting from the climate change-driven migration of species may alter herbivory and plant defences against pests along these gradients, either positively or negatively. Exposure to UV-B radiation can also increase the allocation of plant resources to chemical defences that could otherwise be used for growth and competitive advantage.179,180
Research conducted along elevational and latitudinal gradients can provide valuable, albeit indirect, information about the effects of interactions between UV radiation and climate on plant competition and survival, degree of acclimation and adaptation, and consequent impacts on species diversity and ecosystem functioning (e.g.ref. 181–183). Assessments of these plant and ecosystem responses are useful for guiding crop selections and managing agricultural practices in areas affected by increasing temperature and enhanced UV radiation at regional and latitudinal scales (e.g.ref. 184–186).
3.5. Reduced snowfall is altering the seasonal timing of exposure to UV-B radiation in plants and this may increase their susceptibility to damage from extreme weather conditions
Climate change is causing less snowfall at high latitudes and high elevations.187–190 The resultant smaller snowpack leaves certain plants more vulnerable to unfavourable conditions during winter (i.e., increased exposure to UV and total solar radiation, greater temperature fluctuations and desiccating winds)191 (Fig. 5). This has led to plant mortality in some areas of the high Arctic tundra192,193 and in Antarctic moss-beds.194 However, plants tend to screen UV more effectively when they are exposed to low temperatures, potentially increasing their capacity to avoid damage from high solar irradiances. This effect has been shown in experimental studies195,196 and by monitoring shrubs above and below the snowpack.197,198 Once snow has melted and during the onset of growth, UV-B radiation received in spring can help limit plant damage and the reductions in photosynthesis that would otherwise result from fluctuating weather conditions at this time of year. The extended growing season due to climate change, bringing earlier spring and later autumn, is accompanied by reduced exposure to UV-B radiation during these seasonal transitions. As a consequence, some plants will be more susceptible to damage from extreme weather events at the onset and cessation of growth.
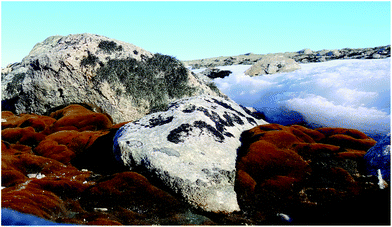 |
| Fig. 5 Moss turf and lichen encrusted rocks near Casey Station in East Antarctica. Antarctic mosses emerge from under the snow in early summer and can be exposed to high solar radiation, including UV-B radiation. When plants first emerge they are bright green and those in protected areas, such as under melt water or in small depressions, will remain green; however, plants on exposed ridges quickly accumulate sunscreen pigments to protect themselves, evident here in the red-brown colour of the exposed moss. Photo by Sharon Robinson on 18 December 2012. | |
3.6. Increases in atmospheric carbon dioxide can stimulate photosynthesis and plant growth in some species, but this beneficial effect depends on levels of UV-B radiation and other factors
Depending on the photosynthetic pathway (i.e., C3vs. C4 photosynthesis), elevated concentrations of CO2 can increase the yields of some crops, although generally only under optimal conditions for plant growth (e.g. adequate water and nutrient supply).199 Thus, the stimulating effects of rising CO2 are often reduced when plants are exposed to UV-B radiation and grown under conditions of low water availability and/or nutrient supply.200–202 However, exposure to UV-B radiation can increase plant tolerance to drought and low nutrient availability.203,204 These complex interactions between UV radiation, CO2 and other climate change factors make it challenging to model and assess how crop productivity would be affected by the changes in UV radiation that would occur under different scenarios of climate change and stratospheric ozone, including the projected “World Avoided” scenario without the Montreal Protocol.
3.7. UV-B radiation stimulates plant defences and can improve crop health and nutritional value for consumers
Solar UV-B radiation can increase plant resistance to a variety of pests and pathogens due to altered plant chemistry, especially by inducing secondary metabolites such as polyphenolics (e.g. flavonoids), which also serve as antioxidants and UV-screening compounds.205–207 Applications of this knowledge can be used to enhance desirable crop response to UV-B radiation.208 This includes defence responses against pests and disease, and accumulation of the antioxidant compounds in food crops.209–211 Similarly, this knowledge can be applied to manipulate light conditions in greenhouses and controlled environments to improve the yield, health and nutritional value of horticultural crops.212,213 These findings represent an important side effect of the Montreal Protocol with regard to societal benefits and the fundamental research that is being conducted to better understand how plants respond when exposed to UV radiation.
4. Effects of stratospheric ozone depletion, UV radiation and interactions with climate change on aquatic ecosystems
Recent research on the effects of UV-B radiation, as well as UV radiation in general, on the aquatic environment evaluates how climate change and other anthropogenic effects interact with exposure to UV radiation in both freshwater and marine ecosystems. Whereas stratospheric ozone depletion increases exposure to UV radiation, other processes change transparency to UV radiation, often decreasing exposure in the aquatic environment. This has consequences for species distributions and services provided by aquatic ecosystems, such as freshwater, fisheries, and recreation. Aquatic ecosystems also play a major role in mediating the effects of other human actions related to ozone depletion and UV radiation, including fluorinated refrigerants (see section 6.8), sunscreen use and micro/nanoplastics.
4.1. Climate change and other effects of human activity are becoming more important than variations in stratospheric ozone in controlling the exposure of aquatic ecosystems to UV radiation
Implementation of the Montreal Protocol has limited the increase in UV-B radiation incident on the aquatic environment which would have otherwise occurred. However, climate change and other human activities since implementation have altered the water transparency, and therefore exposure to UV radiation, across a range of marine and freshwater habitats. Most recent reports indicate that the UV transparency of aquatic ecosystems has decreased. These include long-term declines in the depths of penetration of UV-B radiation in a region of the Chesapeake Bay, United States, probably related to human disturbance around the shoreline;214 and the “browning” of Norwegian alpine lakes as forest cover expands to higher elevations in their warming watersheds and organic matter from the trees enters runoff into the lakes.215 Declines in transparency are also occurring in the open ocean, where deepening of the vertical circulation of plankton, attributed to changes in weather patterns and ocean currents, decreases the average exposure to UV radiation of plankton in near-surface waters.216 These trends are evident in observations from long-term monitoring stations in the middle of the Atlantic and Pacific oceans. Conversely, the loss of Arctic sea ice217 is dramatically increasing exposure to UV radiation in areas of the Arctic Ocean previously shielded from direct solar radiation. The constituents involved in these changes (chiefly ice cover, dissolved organic matter and suspended particulates) affect transparency across the spectrum, including both PAR and UV radiation, but have the strongest effect on UV-B radiation.218 These contrasting trends in transparency, and hence exposure to UV-B radiation, will likely continue as the effects of climate change intensify.
4.2. UV-B radiation excludes some invasive organisms from clear lakes, while a decrease in radiation, due to increases in dissolved organic matter, promotes their invasion into browning lakes
Coloured dissolved organic matter (DOM) is increasing in areas affected by increased runoff, which is often associated with climate change.219,220 This “browning” of inland waters reduces UV-transparency, facilitating invasion by UV-sensitive species. This has already been demonstrated for fish in cold, clearwater lakes, where high DOM can create a spawning refuge that facilitates the invasion of UV-sensitive warm-water species.221,222 Regulations that maintain high levels of UV transparency in lakes may help manage fish invasions.223 Two new studies show how UV-B radiation excludes important invertebrate predators and invasive species from clear lakes, while increases in DOM facilitate their establishment in browning lakes. Experiments with the predatory larvae of the aquatic insect Chaoborus, which is only found in brown lakes (i.e. those with >3 mg L−1 total organic carbon), showed a much higher rate of egg deposition in water with high vs. low concentrations of UV-absorbing DOM.215 This suggests that the range expansion of this important predator of freshwater zooplankton is regulated by variations in the transparency of the waters to UV radiation.215 Another important species that responds to local variations in UV transparency is the jellyfish Craspedacusta sowerbii, which is invading many freshwater systems world-wide. Its behaviour and survival rate is sensitive to UV radiation in field incubations.224 This species does not contain photoprotective compounds known to occur in other zooplankton and survives better in habitats with low UV transparency.224 These studies show that UV transparency changes the species composition of zooplankton in lakes, which can affect the value of the zooplankton community as a food resource supporting fisheries.
4.3. Micro- and nanoplastics arising from the breakdown of plastics by solar UV radiation may have negative consequences for aquatic ecosystem services, as well as for food and water security
The degradation by solar UV radiation of plastics into micro- and nanoplastics can increase substrates for pathogens and other microorganisms, sequester contaminants, and increase ingestion by aquatic organisms. However, these potentially negative effects are as yet poorly quantified. Plastics are ubiquitous pollutants in all ecosystems worldwide and include not only large fragments, but also microplastics (defined as those <5 mm in diameter) and nanoplastics (those <100 nm in diameter).225,226 There has been tremendous growth in both the published scientific research and public interest in the potential effects of microplastics on aquatic ecosystems, which has led to the recent passage of regulations in some jurisdictions.227 Plastic fragmentation following exposure to UV radiation stimulates microbial colonisation and further plastic degradation228,229 (see also section 7.7). Microplastics can, in turn, be ingested by many types of aquatic organisms, ranging from zooplankton and other invertebrates to fish.230
While there is a dearth of evidence, suggestions have been put forward for how the ingestion of microplastics can negatively affect organisms, including direct chemical toxicity and physical obstruction, which can be external or in the digestive tract, as well as serving as a substrate for concentrating and increasing exposure to contaminants and pathogens.230,231 For example, exposure to UV radiation and consequent fragmentation increases the particle surface area, which may be responsible for the increasing microplastic adsorption of contaminants such as metals.232 A major unresolved question is whether these microplastics cleanse the water column of such contaminants, leaving them bound to long-lasting plastics in the sediments, or whether they act as a conduit for these contaminants to the aquatic organisms that ingest them.
Two recent reviews discuss the evidence for the negative effects of microplastics on the feeding, survival, growth, and reproduction of aquatic organisms, as well as their accumulation in seafood sold in public markets for human consumption.227,230 A meta-analysis of 43 experimental laboratory and field studies was carried out on the effects of microplastics on fish and aquatic invertebrates in freshwater and marine systems.230 This study found negative effects of microplastics in many cases, with the greatest impact being on zooplankton survival, growth, and reproduction. This has important implications for both water quality in inland waters, and aquatic food webs in marine and inland waters, since zooplankton comprise a critical link in food webs, as well as serving as a vital food for young and in some cases even adult fish. Overall, neutral or no effects were more common than negative effects, but only four response characteristics were studied, and other unexamined effects of microplastics could pose substantial threats to aquatic life.
A second review examined 320 peer-reviewed studies of the effects of microplastics in freshwater and marine systems, including sediments.227 While negative effects of microplastics were common, there were many conceptual and methodological limitations of previous microplastic studies, which limit the ability to draw overarching conclusions on the real nature or magnitude of the threat. For example, although polyethylene fibres and fragments are the most common microplastics in the environment, many studies have used spherical polystyrene beads, often much smaller than those found in nature, to examine the effects of microplastics.227 More recently, however, heat-degraded particles, similar to UV degradation products, have been shown to alter the behaviour and morphology of the microcrustacean Daphnia magna.233
Despite the prevalence of microplastics and widespread evidence of negative effects on some aquatic organisms, a recent ecological risk assessment indicates that currently available data are not adequate to conclude that their presence in ecosystems represents a substantial risk.231 In some cases, studies have shown no effects of microplastics. For example, in one species of larval fish, polyethylene microplastics seem to have minimal effects on feeding rates or short-term (30 d) growth rates.234 Better data and consistent endpoints and methods for testing the effects of exposure are needed before conclusions on the potential risks and impacts of microplastic particles can be fully summarised.231 Conclusions on the effects of microplastics and the potential role of UV radiation must account for the diversity of microplastics,226 the potential for artefacts in experimental studies,235 and the need to recognise complex interactions with other contaminants.236,237
4.4. Sunscreens that protect humans from excess exposure to UV radiation can be harmful to aquatic organisms
Based on in vitro studies showing harmful effects of organic sunscreens on aquatic organisms, particularly corals, and the detection of such sunscreens in coral reef habitats, the United States state of Hawaii has banned the sale and distribution of organic sunscreens, effective January 2021.238 Alternative methods for photoprotection include clothing, which can provide protection without the use of sunscreen. However, some types of outdoor clothing now have dyes or sunscreens incorporated into the fabric, which is rated using the Ultraviolet Protection Factor (UPF). The release of chemicals into seawater from these fabrics needs to be evaluated to ensure that they are not an additional source of contamination to aquatic ecosystems.
Organic sunscreens, such as oxybenzone and avobenzone, and mineral-based inorganic sunscreens, such as titanium dioxide (TiO2) and zinc oxide (ZnO), have been detected in surface waters, sediments, and organisms.239 ZnO is toxic to the symbiotic algae within corals,240 and uncoated ZnO can induce coral bleaching, whereas modified forms of TiO2 are less damaging to corals.241
All experiments testing the toxicity of sunscreens have thus far been conducted in laboratory settings in which the maximum concentrations used often exceed environmentally relevant levels, so caution must be used in the interpretation of these results. It is important that further studies be undertaken to determine the utility of mineral-based UV filters in photoprotection as alternatives to organic filters.
5. Interactions of biogeochemical cycles with changing UV radiation and climate
The most relevant advances with respect to the impacts of changes in solar UV radiation and its interaction with climate change and biogeochemical cycling are: (1) modeling and quantification of how exposure to UV radiation transforms contaminants; (2) changes in the amount of organic carbon susceptible to photochemical reactions due to the melting of permafrost in the Arctic; and (3) impacts of land use and climate change affecting the UV-mediated degradation of carbon in terrestrial ecosystems. Additionally, we discuss fire in high-latitude systems, particularly the production of trace gases from wildfires and interactions of pyrogenic carbon with UV radiation in the global carbon cycle.
5.1. Advances in modelling increase our ability to assess the effect of changes in UV radiation on the photodegradation of contaminants
Contaminants are chemicals or biological substances that are intentionally or accidentally released into the environment. Pesticides, pharmaceuticals, household and industrial products, petrochemicals, nanomaterials, and microplastics are examples of chemical contaminants. Biological contaminants include pathogens that are released by human activities, such as treating wastewater, and harmful algal blooms; the latter of which are a natural component of ecosystems, but are becoming more abundant because of human activities.
Significant advances have been achieved in mathematical model simulations of photoreactions and transport of contaminants in aquatic environments.242–244
Several modeling studies of carbon-based nanomaterials (e.g., multiwalled carbon nanotubes (MWCNT) and graphene oxide (GO)) using the Water Quality Assessment Simulation Program (WASP) have been published since our last assessment.242,245,246 WASP is a dynamic, spatially resolved surface water model. It has been updated (Fig. 6) to account for nano-specific processes and can simulate the direct phototransformation of nanomaterials and other contaminants as a function of wavelength over the solar spectrum.242,246 Potentially, WASP can use projected changes in UV-B radiation to predict changes in contaminant phototransformation. New improved biological weighting functions and information on natural photosensitisers have been used to refine models of the photoinactivation of coliphages (a type of virus) that are potential indicators of pathogenic viral activity in recreational water.243,247–249 Improvements were made to the spectral irradiance model (Simple Model of the Atmospheric Radiative Transfer of Sunshine, SMARTS),250 which has been widely used to calculate photoinactivation rate constants of biological contaminants.243
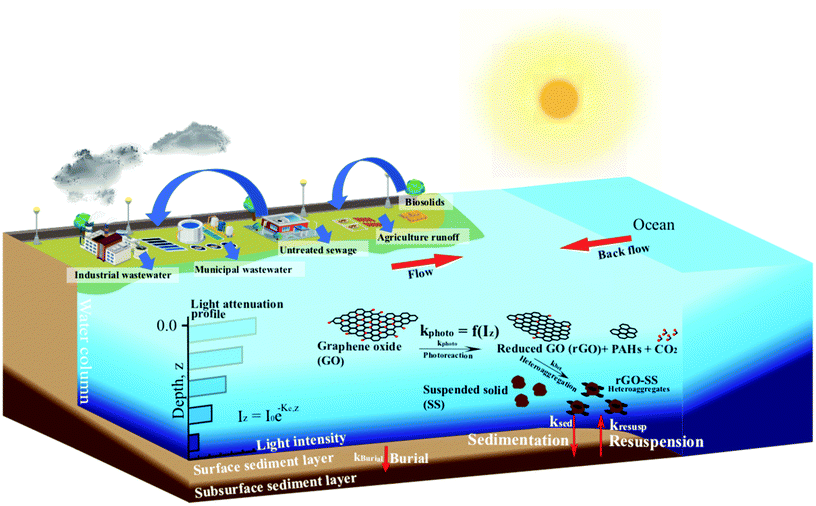 |
| Fig. 6 The updated Water Quality Analysis Simulation Program 8 (WASP8) predicts the photoreactions of contaminants (here using graphene oxide as an example) as a function of the penetration of UV radiation with depth of the water column. The model can also simulate interactions of UV radiation with contaminants such as pesticides, metals, pathogens, and industrial and household organic compounds. Figure designed by Wen-Che Hou. | |
5.2. Projected recovery of the stratospheric ozone layer and consequent decrease in UV-B radiation could accelerate or retard the photodegradation of contaminants
Reduced levels of UV radiation can increase the biodegradability of plastics251 by micro-organisms, and decrease the release of toxic additives/byproducts associated with microplastics252–254 and nanomaterials.255–257 Reduced UV radiation might also mitigate the effects of TiO2 and ZnO nanoparticles; once released into the aquatic environment, these common UV-blocking agents in sunscreen products generate damaging reactive oxygen species (ROS) under UV radiation.258 Conversely, decreased UV radiation could attenuate the self-cleansing ability of the environment by slowing the direct and/or indirect photoreactions of certain pesticides and biological contaminants.243,247,252,259 Another negative effect could be the decreased photochemical conversion of highly toxic silver ions to much less toxic silver nanoparticles.260 These complex, competing effects of decreased UV radiation on contaminant photoreactions must be taken into consideration by models that predict future concentrations of contaminants in the environment.242,245,246
5.3. UV radiation induces carbon dioxide production from organic carbon released by thawing permafrost, thus contributing to a significant positive feedback on global warming
The permafrost carbon pool in the Arctic is now thought to comprise organic carbon in the top 3 m of surface soil, carbon in deposits deeper than 3 m (including those within the yedoma region, an area of deep sediment deposits that cover unglaciated parts of Siberia and Alaska), and carbon within permafrost that formed on land during glacial periods but which is now found on shallow submarine shelves.261,262 Multiple lines of evidence suggest that permafrost warming is accelerating more rapidly than originally projected,263,264 so carbon stored in this pool is now being exposed to sunlight, including UV radiation. This previously sequestered organic carbon becomes available for photochemical and microbial degradation, releasing CO2 and methane to the atmosphere.261 The total release of greenhouse gases in this region due to global warming has been referred to as the permafrost carbon feedback (PCF)261,262,264 (Fig. 7). Generally, deeper layers of organic matter are exposed over decades, or even centuries, and some models are beginning to track these slow changes. As the deeper permafrost warms and thaws, the average age of the released carbon is increasing,261,265 exposing older carbon that is photoreactive but biologically persistent. Permafrost thawing can also operate in an abrupt way, collapsing suddenly and destabilising several metres of soil within days or weeks,264 dramatically accelerating the exposure of previously shielded carbon to solar UV radiation. A part of this newly exposed carbon may be susceptible to photodegradation on land, accelerating carbon release to the atmosphere.266 As carbon moves from the terrestrial land surface to Arctic lakes and river ecosystems, photodegradation may account for a substantial fraction of the degradation of this semi-labile organic carbon,267 although its importance will be determined in part by water mixing and the amount of suspended sediment.268 There is an urgent need to incorporate the role of UV radiation in the degradation of exposed carbon from thawing permafrost, and to consider how changes in the exposure to solar radiation of sequestered carbon affect the acceleration of carbon release from both terrestrial and aquatic ecosystems in the Arctic.
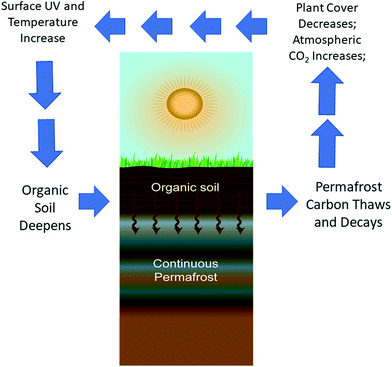 |
| Fig. 7 Feedbacks from UV irradiation and melting of permafrost. Illustration by Richard Zepp. | |
5.4. Land-use and climate change alter vegetative cover and affect the extent of photodegradation as a control of plant litter decomposition in terrestrial ecosystems
Substantial differences in vegetative cover occur naturally in terrestrial ecosystems due to differences in abiotic factors such as soil and climate. Additionally, human activities, such as land use change, intentional planting of exotic species, drought or fire, function as major determinants of vegetative cover and the amount of UV and total solar radiation reaching the soil surface. These changes in plant cover affect plant biomass but also carbon and nutrient cycling and may be key in determining the role of sunlight, and UV radiation, as a control of carbon release.
Photodegradation, the breakdown of plant litter driven by exposure to UV and short wavelength visible radiation, continues to be shown as a key component of decomposition in low rainfall ecosystems, such as deserts and grasslands. Various abiotic and biotic factors interact to influence the balance of photodegradation vs. microbial (bacteria and fungi) litter decomposition, including the degree of sunlight exposure, moisture, relative humidity and temperature, as well as the initial litter chemistry determined by plant species identity.269–272 Very recent evidence has demonstrated that the exposure of plant litter to sunlight is of much greater import than the direct effect of temperature on decomposition (i.e. thermal degradation).273
Photodegradation can also alter litter chemistry and microbial access to carbohydrates in the litter, which can facilitate microbial decomposition (i.e. photo-facilitation),274–276 although this is not always observed.277 This facilitative effect of sunlight on microbial respiration may be one of the main avenues by which photodegradation increases litter decay,274 and implies that photodegradation may be important in terrestrial habitats beyond deserts and grasslands. The broader role of solar radiation exposure in litter decay is supported by recent experimental studies, indicating that accelerated decomposition from sunlight can also occur in wetter, forested ecosystems.278,279 Taken together, further understanding of the mechanistic controls of sunlight on carbon release suggests that land use changes that increase the exposure of plant litter to solar radiation will accelerate carbon losses from these ecosystems due to the effects of photodegradation and photo-facilitation. These alterations in the carbon cycle should be considered when modeling the effects of land use and climate change in terrestrial ecosystems.
5.5. Fires contribute to greenhouse gas release and the production of UV-sensitive burnt biomass
Forest fires are becoming more severe and frequent, and this trend is predicted to increase due to climate change, particularly in boreal forests where extreme warming is expected.280–286 Forest fires produce a variety of trace gases and aerosols, including the greenhouse gases CO2, methane, and nitrous oxide. Emission factors for these species have recently been compiled by Andreae.281 Fires provide an important pathway for opening up the soil surfaces to UV radiation. Moreover, due to the incomplete combustion of wood and other biomass, fire converts a substantial fraction of the vegetation carbon into burnt biomass, also named charcoal, black carbon, or pyrogenic carbon (PyC).283,287 Approximately 256 Tg (uncertainty range: 196–340 Tg) of biomass carbon was converted annually into PyC between 1997 and 2016.287 Burnt biomass is more resistant to environmental degradation than unburnt biomass and can act as a long-term sink for CO2. Photodegradation provides the main pathway for the decomposition of burnt biomass into CO2.287,288 Rainfall washes a fraction of PyC into streams, rivers, lakes, coastal inland waters and the ocean. Upon exposure to solar UV radiation, PyC undergoes photochemical conversion to CO2 at a global rate estimated to be between 20 and 490 Tg C per year in the ocean.288
6. Interaction of changes in stratospheric ozone with air quality in a changing climate
The composition of the lower atmosphere (the quality of the air we breathe) has a significant impact on human and environmental health. This composition is altered by variations in the concentration of stratospheric ozone and by climate change. The effects on human health that are most studied are those associated with increases in ground-level ozone and aerosols. Since our last assessment,289 there has been ongoing research on the impact of poor air quality on health, in particular focusing on aerosols (particulate matter with an aerodynamic diameter smaller than 2.5 μm, PM2.5) and ozone, and the findings corroborate our previous conclusions.289 For urban environments, improving air quality has been viewed as a matter of controlling local emissions, but it is becoming more evident that the problem will need to be managed, for many locations, on a regional scale.290,291
Air quality is also dependent upon changes in several atmospheric parameters on a global scale, including changes in climate and stratospheric ozone. Importantly, these changes are not independent, as climate change can alter the emission of several chemicals (such as methane, halogens and nitrogen-containing compounds from natural sources) that will alter concentrations of stratospheric ozone. Recent developments in our understanding of the coupling between changes in stratospheric ozone, climate change, and composition of the ground-level atmosphere are highlighted below.
6.1. Ozone at ground level is predicted to substantially increase by 2100, as a result of increased transport of ozone from the stratosphere to the troposphere
Models are now capturing the effects of the coupling of atmospheric circulation and chemistry, particularly the link between the stratosphere (> ca. 12 km altitude) and the troposphere (≤
12 km altitude). Changes in stratospheric ozone, stratospheric circulation, and alterations in temperature affect the transport of ozone between the stratosphere and the troposphere. An increase in ozone in the upper troposphere is then distributed throughout the troposphere. Of particular relevance here is that this can lead to changes in the concentration of ground-level (the atmosphere to which terrestrial organisms are exposed) ozone in both hemispheres.292,293
Modelled predictions of the concentration of ozone in the lower troposphere and at ground level in 2100 continue to show substantial increases (≥
20%) compared to the present. These increases will be caused by a warmer climate increasing the rate of mixing between the lower stratosphere and the upper troposphere, and by the recovery of stratospheric ozone due to reductions in ozone-depleting substances (ODS).294 The relative importance of the two effects depends on the hemisphere and season, with the decrease in ODS being more important in the summer in the southern hemisphere. Overall, the predicted impact of warming caused by greenhouse gases is substantially larger than the changes due to ODS, although the two processes are not independent.
6.2. Aerosols with complex physical and chemical properties can affect the formation and loss of ground-level ozone in polluted regions
In polluted regions, aerosols can affect UV photolysis rates, atmospheric dynamics, cloud optical thickness, and heterogeneous reactions, which are all important factors in the formation and loss of ground-level ozone.
For eastern China, a global atmospheric chemical model previously found that aerosols often reduce the photolysis rates of ozone [J(O3)] and nitrogen dioxide [J(NO2)] by 10–30%, but the calculated effect on surface O3 was relatively small (2–4% reduction). More recent studies find increases in ground-level O3 that are associated with PM2.5 reductions295–300via changes in UV radiation, as well as via heterogeneous (gas-particle) chemical reactions.301,302 Thus, the health benefits from reducing aerosols are partly offset by the increase in ground-level O3.
The reduction in UV photolysis during severe haze-pollution events can also lower the urban concentrations of OH radicals,303 slowing the photochemical removal and thus allowing more local accumulation of many pollutants other than ozone and PM2.5. Ozone, PM2.5 and OH interact in complex ways304 that can be modified by solar UV radiation, and characterisation and quantification of these links are ongoing.
6.3. Concentrations of ozone at the Earth's surface will be altered by the changing climate
A study of recent periods of high concentrations of ambient ozone in China (2016–2017) highlights that, in addition to the impact of anthropogenic emissions, significant changes in ozone at ground level can be expected from a multitude of effects associated with climate change. These include changes in emissions of volatile organic compounds (VOCs) from growing plants, changes in lightning and, as already mentioned above, in the exchange of air from the stratosphere.88,305
6.4. The stable concentration of hydroxyl radicals over the last 35 years is not likely to continue in the future
Hydroxyl radicals remove many harmful chemicals from the atmosphere.289 Hydroxyl radical concentrations have remained stable over the last 35 years due to the near-offsetting of changes in their production (by UV radiation and nitrogen oxides) and destruction (by carbon monoxide, methane, and other volatile organic compounds). Such stability is largely fortuitous and is not likely to continue in the future, as these now-offsetting factors are expected to vary by different rates in the coming decades. Changes in the global concentration of OH would have a significant impact on air quality. The concentration of OH is highly dependent on the amount of UV-B radiation, and hence on the amount of stratospheric ozone,289 as well as on other factors, as discussed below.
Methane is a key factor in determining OH concentration, because its reaction with OH is a major sink for OH, as well as the main mechanism of removal of CH4 from the atmosphere.289 Rising concentrations of CH4 due to increases in natural and anthropogenic emissions should therefore decrease the concentration of OH in the atmosphere; however, this appears not to have been the case in the recent past. Calculations for the period 1980–2015 indicate that this decrease was almost totally offset by other changes in the chemistry of the atmosphere, including the concentration of water vapour, nitrogen oxides (NOx), and the total amount of ozone in the atmosphere.306 This result is consistent with earlier conclusions based on measurements of the concentration of methylchloroform, a synthetic ODS that is primarily removed by OH.307 Whether a continuing increase in emissions of CH4 will result in a significant decrease in the global mean concentration of OH (by up to ca. 10%, according to ref. 308) is an open question.
Measuring the average global concentration of OH is difficult, and new methods are needed. Methane is one of the key candidates considered for estimating OH, as its reaction with OH is the primary route for the removal of CH4 from the atmosphere, and there is an expanding range of measurements of atmospheric CH4 both at ground level and from satellites. As a result, models of atmospheric CH4 are more able to assign observed changes to variations in regional emissions and the concentration of OH.309,310 However, there are still significant uncertainties in this estimation of OH that could possibly be addressed via measuring the isotopic composition (12CH4; 13CH4) of methane.311 Overall, there are ongoing significant advances in our ability to measure changes in the state of the reactive chemistry of the global atmosphere.
6.5. Regional trends in concentrations of OH are highly variable
Regional trends in the concentration of OH are not readily measured because OH has an atmospheric lifetime shorter than a second. However, some calculations have been carried out, such as over eastern China, where OH increased substantially between 1990 and 2000.312 As this trend is not observed in the global estimates of the concentration of OH, such changes have not occurred everywhere, but may well be important for regional air quality.
6.6. Concentrations of methane in the atmosphere have increased substantially in the last decade, with implications for climate change, stratospheric ozone chemistry, and regional air quality including ground-level ozone
Causes of the increase in methane include increased rates of anthropogenic and natural climate-sensitive emissions, while its removal is determined by the OH radical and is thus sensitive to UV radiation. In the last decade, the global average concentration of methane in the atmosphere has increased at a rate of 5–10 ppb per year (see Fig. 8).308,313–315 CH4 is also a greenhouse gas (GHG) with a radiative forcing of climate of ca. 25% of that due to CO2.316 Like other GHGs, CH4 alters concentrations of stratospheric ozone.1 Outside the polar regions, the radiative cooling of the middle and upper stratosphere due to increasing concentrations of GHGs is expected to result in an increase in stratospheric ozone.1 At polar latitudes, however, radiative cooling increases the prevalence of polar stratospheric ice clouds (ice PSC), a prerequisite for ozone depletion to occur in polar spring.1 Record low vortex temperatures were observed in the Arctic winters of 2010–2011 and 2015–2016, followed by significant loss of stratospheric ozone in the Arctic spring317–320 (by up to 38% and 27%, respectively).319 Furthermore, the oxidation of CH4 by OH is an important in situ source of stratospheric water vapour.321 It is likely that water vapour in the polar stratosphere raises the temperature at which PSC particles form. These particles enable the rapid conversion of reservoirs of chlorine into chlorine radicals, which deplete ozone in the polar spring.317
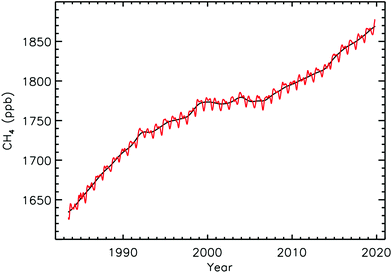 |
| Fig. 8 Global monthly mean methane (CH4) concentration (reported as a mole fraction) measured by NOAA (https://www.esrl.noaa.gov/gmd/ccgg/trends_ch4/). Data from 2019 are preliminary. Figure prepared by Ed Dlugokencky (NOAA). | |
Emissions of CH4 from natural sources (ca. 40% of total CH4 emissions) are affected by climate change. For example, CH4 emissions from wetlands (the most important natural source at present) are predicted to increase by 33–60% by 2100, based on estimated increases in CO2.322 Thawing of permafrost soils may become an increasingly important source of CH4, particularly in the Arctic because of “Arctic amplification” (i.e., the prediction that the Arctic troposphere will continue to warm faster than elsewhere in response to increasing GHG concentrations) and since permafrost soils in this region store vast amounts of carbon.323,324 Emissions of CH4 from beneath warming lakes and retreating ice sheets are also now recognised as an additional source.323,325
When permafrost soils thaw, they release natural organic matter (NOM) into the surrounding environment. This release increases the susceptibility of NOM to microbial decomposition and production of CH4. Note that the release of NOM via permafrost thaw primarily results in CO2 production, a process that is induced by UV radiation (see section 5.3).326 The microbial production of CH4 increases with rising temperature and spring rainfall, particularly where the loss of ice-rich permafrost converts forest and tundra into wetlands.327 The release of CH4 from permafrost soils is moderated by methanotrophs, microorganisms that oxidise 20–60% of CH4 before its emission to the atmosphere.328 According to model calculations,323 which also included abrupt thaws beneath thermocast lakes, CH4, not CO2, has been the dominant driver (ca. 70%) of the radiative effect due to the release of GHG via thawing of circumpolar permafrost this century.
The main competitor of CH4 for OH is CO,329,330 because CO is the strongest sink of OH in the troposphere, making up 39% of the global annual mean of total OH sinks.329 Since the rate of photoproduction of CO depends, in part, on the concentration of natural organic matter (NOM) that is photoreactive,331 effects of climate change, such as forest fires, thawing of permafrost soils, “browning of lakes”,218 and enhanced runoff of NOM into rivers and coastal areas are expected to enhance the photochemical production and emission of CO from terrestrial and aquatic ecosystems.326
6.7. The role of naturally produced halogen-containing compounds in altering air quality in polar regions is larger than previously recognised
The emission of these natural ozone-depleting compounds (e.g., those containing bromine) will depend on climate change, and will therefore link climate change, stratospheric ozone depletion, and air quality. Reactive bromine species (i.e. the bromine atom (Br) and bromine monoxide (BrO)) oxidise tropospheric pollutants including ozone and gaseous elemental mercury, a contaminant of global concern.332 The presence of reactive bromine is considered to be the main cause of near-complete ozone depletion events in the polar boundary layer near ice and of mercury deposition during polar spring.333–335 Reactive bromine species are formed by UV-induced processes, through: (i) photolysis of brominated very-short-lived substances (BrVSLS); and (ii) photolysis of Br2 on ice- and snow-covered surfaces, where Br2 is formed via oxidation of Br− by OH on frozen saline surfaces such as sea-ice.333,335,336 BrVSLS, particularly bromoform (CHBr3, lifetime of 17 days) and dibromomethane (CH2Br2, lifetime of 150 days), can reach the lower stratosphere, where their photoproducts participate in the destruction of stratospheric ozone. One of the main routes for the transport of BrVSLS into the stratosphere is the circulation of the Indian summer monsoon,337 which is affected by climate change.338
BrVSLS are produced by abiotic and biotic processes. The abiotic process consists of the oxidation of Br− deposited on ice and snow surfaces by ozone, followed by the reaction of hypobromous acid (HOBr) with DOM.339 This process occurs in sea ice in the Antarctic winter339 and may be an important source of BrVSLS. The biogeochemical formation of BrVSLS in marine environments involves a suite of reactions,340 which are, in part, dependent on UV radiation (see Fig. 9). The yield of BrVSLS via bromoperoxidase-mediated halogenation of DOM depends on the chemical composition of the DOM compounds, where terrestrially-derived (allochthonous) DOM contains precursors of CHBr3, such as humic and fulvic acids.341 Coastal zones were found to be important sources of BrVSLS337,342 and may become even more important due to enhanced fluxes of allochthonous DOM to rivers and coastal areas as a consequence of more frequent storm events in a changing climate. Hence, climate change influences not only the transport of BrVSLS from the troposphere into the lower stratosphere, but also their biogeochemical production in marine environments.
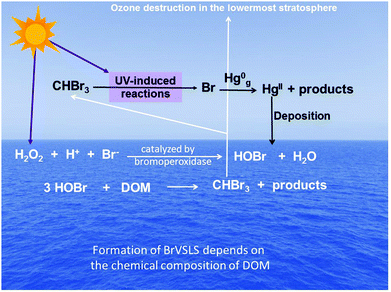 |
| Fig. 9 The formation of BrVSLS in marine environments results from the reduction of hydrogen peroxide (H2O2) by bromide ion (Br−), yielding hydrobromous acid (HOBr), which subsequently reacts with dissolved organic matter (DOM) to form CHBr3.340 The former reaction is catalysed by the enzyme bromoperoxidase, which is produced by micro- and macroalgae340,343 (figure modified from ref. 326). | |
6.8. Limited new measurements of trifluoroacetic acid in the environment reconfirm that it is not a threat to human health or an environmental concern at current or projected future concentrations
As noted previously,344 there are many sources of trifluoroacetic acid (TFA) in the environment, including the degradation of HCFCs, HFCs and HFOs. A review of short- and ultrashort-chain poly- and perfluorinated substances345 noted a paucity of information on the sources of TFA other than fluorinated refrigerants.
TFA can be formed from the breakdown of fluorinated polymers used in industry and in the home. A study on thermal breakdown (thermolysis) of the fluoropolymers poly(vinylidene fluoride-co-hexafluoropropylene) (PVDF-HFP), poly(vinylidene fluoride-co-chlorotrifluoroethylene) (PVDF-CTFE) and poly(tetrafluoroethylene) (PTFE) showed that thermolysis occurred at temperatures ranging from 400 °C to 650 °C.346 Yields of TFA ranged from 0.3 to 1.2%, depending on the polymer. Using a model, the authors estimated that 1.6 to 14% of the TFA detected in rainwater in Beijing in 2014 came from this thermolysis source. The applicability of these data to other locations is unknown. TFA continues to be detected in environmental media. Samples of precipitation collected in 2016 in 28 cities in mainland China were found to contain TFA in higher concentrations than other longer chain perfluorinated compounds. Maximum concentrations ranged from 8.8–1.8 μg L−1 with an average of 0.15 μg L−1, with higher concentrations in coastal cities.347 The sources of TFA were not reported but the values are consistent with previous reports.344
Future projections assuming 100% conversion of all automobile air conditioners to TFA-precursors (HFO-1234yf) predict TFA concentrations in precipitation in the range of 0.48–0.64 μg L−1 across the United States, Europe, and China.348 Wastewater treatment plants may be a source of TFA to downstream aquatic ecosystems, and concentrations in the Neckar River in Germany were over 100 μg L−1 downstream of industrial TFA production sources.349 Common techniques used in wastewater treatment such as ozonation and activated carbon filtration are ineffective at removing TFA, while ion exchange and reverse osmosis are partially effective349 but are difficult and costly to implement on a large scale.
Two new studies of TFA in plants and animals have been reported in the recent literature, neither of which reports negative outcomes. In a study on wheat (Triticum aestivum), TFA was shown to be absorbed via the roots.350 Using a hydroponic testing system, young wheat seedlings were exposed to environmentally unrealistic high concentrations of TFA dissolved in Hoagland's nutrient solution (1 mg L−1). It is not clear if the solution buffered the acidity of the TFA, but after 80 h of exposure, the mean concentration of TFA in the roots was 175 mg per kg dry weight, and in the shoots the concentration was 100 mg kg−1. Uptake was dependent on the concentration of TFA in the nutrient solution and was an active process requiring energy. Effects on the plants were not reported and uptake from soil was not investigated. Whether this is a relevant route for the environmental exposure of plants to TFA is uncertain. The other study tested the hypothesis that pretreatment with TFA sodium salt in cattle would provide protection from exposure to the toxic natural product monofluoroacetic acid (MFA).351 In this study, calves were fed 0.1 mg TFA per kg body weight for 28 days and then challenged with 2.0 g per kg body weight of leaves of Palicourea marcgravii, which contained 0.15% MFA. Calves receiving TFA for 28 days showed no signs of toxicity or abnormal blood parameters. There were only seven test animals and one replicate at one dose of TFA, but it appeared to be non-toxic under these conditions. TFA is of low to moderate toxicity when tested in laboratory animals (as summarised in ref. 352).
Considering the conclusions of previous EEAP Assessments and published reviews, there are no new data to suggest that TFA formed from the breakdown of HFCs, HCFCs and HFOs presents a risk to human health or the environment. The environmental concentrations found in recent studies are well below the concentrations known to be toxic in plants, algae, zooplankton and fish.344 However, TFA is known to be extremely persistent and it has been suggested that chemicals should be restricted if they are very persistent.353 While this suggestion is appropriate for chemicals that have adverse effects on the environment and/or the health of organisms at realistic exposures, it is not justified for TFA because of its lack of biological or chemical activity. TFA, like many other biologically inactive elements and/or minerals, has no effects at exposures much greater than those found or expected in the environment.
6.9. Atmospheric aerosol particles are estimated to cause several million premature deaths and over 100 million disability-adjusted life years globally each year
Changes in UV radiation and ground level ozone can alter atmospheric aerosols, as can changes in both anthropogenic and natural emissions. High concentrations of aerosols (including black carbon (BC) and PM2.5) are found during haze pollution events in urban areas and as a result of wildfires. It is recognised that aerosols pose a hazard to human health.354 Increased exposure to PM2.5 has been shown to cause cancer, including kidney and bladder cancer, and to exacerbate respiratory distress (asthma and chronic obstructive pulmonary disease).289 Changes in aerosol compositions may affect their impact on human health,355 although our understanding of the chemical composition of aerosols is quite limited due to the very large number of chemical species that may be present.
A significant fraction of the aerosols in the atmosphere are the product of UV photochemistry (via OH radical-initiated chemistry) and their generation also depends on the amount of ozone and VOCs present. In many regions of the world, VOCs in the atmosphere are predominantly from biogenic sources (i.e. from plants), leading to the release of compounds like isoprene and terpenes to the atmosphere. However, the composition and mass of the resulting aerosol depends on the mixture of compounds present. In unpolluted air, isoprene may reduce concentrations of OH, leading to less oxidation of terpenes in the same air mass. This, in turn, leads to less production of aerosol.356 However, when such biogenic mixtures are exposed to common air pollutants, e.g. Amazon forest air mixing with nitrogen oxides from the Manaus urban plume, the concentrations of OH and production of aerosol are increased substantially.357
Aside from the direct emission of particles to the atmosphere, complex chemical reactions of pollutants such as SO2, NOx, NH3, and VOCs in the atmosphere play important roles in forming the secondary components of aerosols. The secondary compositions of aerosols, SIA (secondary inorganic aerosol) and SOA (secondary organic aerosol), and ozone, are chemically coupled, with NOx and VOCs as their common precursors. The conversion of gas phase molecules to compounds that form part of an aerosol (gas-to-particle conversion) plays important roles for the formation of SIA and SOA, and generates ozone. NOx and VOCs gas-phase chemistry is driven by the OH radical, the levels of which mainly depend on the mixture of organics, the VOCs/NOx relative concentrations,358 and solar UV radiation. Ozone promotes the nucleation and growth of secondary aerosol particles by enhancing atmospheric oxidation.359 Hydroxyl radicals, H2O2, RCHO and other oxidants are generated and lead to the formation of secondary aerosols; thus SIA (SO42−, NO3−) and SOA are formed through the reaction of SO2, NOx and VOCs by gas and liquid phase chemistry and heterogeneous chemistry driven by atmospheric oxidants. The latest research in China showed that the mass concentration and proportion of NO3− exceeded SO42−, so that NO3− became the main secondary inorganic component of PM2.5 in the Beijing–Tianjin–Hebei region. The rapid increase in the atmospheric NO3− concentration from NOx emissions and oxidation has become one of the key factors for the explosive growth of PM2.5 in this region,360 with major implications for human health.
7. Interactive effects of solar UV radiation and climate change on material damage
Solar UV radiation promotes the degradation of plastic,361,362 wood,363–366 and other materials routinely exposed outdoors including paints and coatings. Wood in outdoor applications undergoes surface discolouration and cracking from exposure to solar UV radiation.363–366 Cracking due to the partial degradation of wood polymers promotes subsequent biodegradation of the wood.367
Several technologies, including the use of additives and coatings, are available to protect materials from the effects of solar UV radiation. Emerging technologies suggest that nanoscale particles and bio-derived substances are potentially effective against weathering degradation. Their use in textile fibres, for instance, results in increased sun protection factor (SPF) values. However, nanofiller particles used in fabrics and materials are released to the environment during use, especially when they are weathered by solar UV radiation, and may detrimentally impact aquatic ecosystems.
This section deals with the assessment of recent developments in wood, plastic and fibre technologies pertaining to UV degradation and stabilisation. Environmental implications of the use of emerging nanomaterial-based technologies for UV stabilisation are also discussed.
7.1. Continuing studies on the weathering of polymer nanocomposites confirm the potential for the incidental release of nanoparticles as a result of exposure to solar UV radiation
Using nanoparticles (NPs) in polymer composites improves their mechanical properties and solar UV resistance,368 but there is growing concern about the potential of these composites to release micro- and nanoparticles, especially during use and disposal. However, a risk assessment addressing the large-volume use of nanocomposites outdoors is yet to be carried out. Recent work suggests that thinner products such as films, laminates and coatings are more likely to release nanoparticles upon weathering, compared to thicker laminates.369 During accelerated weathering, polypropylene (PP) and its composite with multiwall carbon nanotubes (MWCNT) release fragments of the composite <1 mm in size, as well as free carbon nanotubes (CNTs).369 The same was confirmed with Epoxy/MWCNT composites subjected to accelerated weathering in a six-lab round-robin test, where the release of CNTs was observed when weathered samples were agitated or treated with ultrasound in water.370
Nanoscale particles of metal oxides are particularly effective UV stabilisers in wood371 and can either be generated chemically on the wood surface372 or used as nanofillers in clear wood coatings.373–375 However, the potential release of NPs when working with treated wood or due to surface abrasion poses a potential risk.376 The weathering of wood outdoors may increase the likelihood of this as it degrades the surface. For instance, NPs of copper oxide (30–50 nm) are now used as a wood preservative in place of soluble copper compounds. These NPs are released at a rate of 0.882 ng cm−2 from swiping even unweathered wood surfaces protected with a coating carrying 9.5 μg cm−2 of copper NPs.377 Water-based coatings of nano-cerium dioxide (CeO2) on walnut wood similarly released NPs by dermal contact during weathering, with a maximum of 66 ± 12 mg m−2 of the NPs during a 6 month period.378 The rate of release in this case, however, decreased as weathering progressed.
Ultra-thin nano-scale oxide films for screening UV radiation are deposited on plastic films used in specialty packaging and glazing applications. For instance, titanium dioxide (TiO2) films are vacuum deposited on poly(methyl methacrylate) (PMMA), blocking up to 99% of solar UV radiation, while allowing the transmission of more than 90% of visible light.379,380 More economical and nearly as effective are composite plastic films that incorporate nanofillers,381 including those that employ in situ generation of NPs in the films. The in situ generation of ZnO in poly(vinyl alcohol) films yielded a composite with excellent UV-shielding capability; UV wavelengths less than 350 nm were completely absorbed with no effect on the visible transparency of the film.382 Also, CeO2 NPs (25–90 nm in size) generated in cellophane films using Ce(NO3)3 as a precursor383 showed transmission spectra that excluded UV radiation and allowed ca. 80% transmission of visible wavelengths. The technology is economically scalable.
7.2. Inorganic nanoparticles used in cotton and other textile fibres dramatically enhance their UV-screening effectiveness
Inorganic NPs, such as those of titanium dioxide (TiO2) and zinc oxide (ZnO), can enhance the UV-screening efficiency of textile fibres. However, they may be released into the environment during use and laundering. Nanoparticles of TiO2384,385 and ZnO386–388 are particularly effective and have been successfully incorporated into cotton fibres. In some instances, such NP treatment increased the ultraviolet protection factor (UPF) of cotton by an order of magnitude. Specific UPF values depend on the thickness of the yarn and structure of the fabric, loading of NPs, and NP characteristics.389 Results from different studies cannot, therefore, be easily compared. The UPF values of wool390 and polyester (PET) fabric383 treated with TiO2 and with ZnO391 show large increases.
NPs that absorb UV radiation are also used in sunscreens. Several sun protection formulations have been banned this year based on their potential to release NPs during use with consequent projected damage to aquatic ecosystems. While the use of nanoparticles imparts improved properties to sunscreen products, their ultimate environmental fate is less well understood. More work on the environmental risk assessment associated with the presence of nanoparticles released into ecosystems is needed prior to the large-scale use of nanocomposites.
7.3. Heat treatment of wood improves the water repellency and durability, but often at the expense of the UV-resistance
Environmental considerations have made chemical-free, heat-treated wood material increasingly important. Thermal treatment makes wood material less hygroscopic, less susceptible to swelling or shrinking, and more resistant to fungi.392 Treatment also makes the wood appealingly darker393,394 and reduces biodegradation, although often at the expense of UV screening. Heat-treated wood is generally discoloured readily upon exposure to solar UV radiation375,395,396 and hence requires effective surface coatings for routine outdoor use. However, it is difficult to select coatings that adhere well to the hydrophobic surface of the material. Several coatings that can be effectively used on heat-treated wood to reduce UV-induced discolouration have been identified. Good resistance to discolouration with up to 2000 h of laboratory exposure under UV-340 lamps has been shown for Scots pine treated at 212 °C and surface-impregnated with TiO2,375 and for Ashwood heat-treated at 192–212 °C and clear-coated with a water-based polyurethane.397
7.4. Some biodegradable polymers are potentially environmentally friendly alternatives to conventional plastics
Poly(lactic acid) (PLA), the fastest growing biodegradable plastic, used primarily in consumer packaging, was evaluated for use in building products. Coatings of PLA or poly(hydroxybutyrate) (PHB) evaluated as a sealant/coating on limestone structures were as stable as the conventional acrylic sealants typically used in this application, based on accelerated exposure to UV radiation and humidity for 104 days.398 In other studies, these plastics were also evaluated as the matrix in wood–plastic composites (WPC); it is the resistance of the polymer matrix to solar UV radiation that determines the durability of WPCs in outdoor use.399,400
The loss in strength of PLA–wood fibre composites upon exposure to accelerated weathering over 1200 h was acceptable, and in fact was marginally superior to that of unfilled PLA plastic. Empirical models showed that the rate of loss of strength of these composites decreased with the duration of exposure.401 Outdoor weathering data on PLA and poly(3-hydroxy butyrate-co-3-hydroxy valerate) (PHBV) plastics and their WPCs (with up to 50% wood) show the plastic matrices to be minimally affected by solar UV radiation and moisture over 12 months of exposure at a sub-tropical exposure site.402 However, the WPCs of these biodegradable plastics, and those based on polyethylene used as a control, fared poorly because the filler absorbed water, leading to mould attack. Using hydrophobic glass fibre (GF) as filler (30 wt%) was able to avoid this problem in both PLA/GF.403
7.5. Sustainability considerations and green building initiatives favour the replacement of conventional UV-stabilisers in materials with ‘greener’ alternatives
There is increased interest in replacing conventional additives and stabilisers with bio-derived compounds to increase the environmental sustainability of industrial wood products. Nanoparticles of lignin derived from wood404 are used as a surface treatment or in clear coats used on wood surfaces to control UV-induced discolouration. Exposing coatings with lignin NPs derived from waste wood on beech wood panels shows good control of UV-induced discolouration in treated samples. Unusually, however, discolouration decreased with exposure time.405 Flavonoids derived from wood extracts are also effective UV stabilisers.406 For instance, the two most abundant flavones in Acacia confusa sp. (the extract of which is already known as a UV stabiliser for wood) were demonstrated to have marked capacity to scavenge radicals and quench singlet oxygen.407
7.6. Biodegradable nanoparticles and bio-derived chemicals used in the textile industry can be feasible, sustainable alternatives to synthetic processing chemicals
It is possible to use biodegradable nanoparticles derived from cellulose nanocrystals and chitosans in preparing nanocomposite textile fibres for enhanced UV protection.408 Synthetic dyes, mordants/capping agents typically used in dying/coating fabric can be replaced with those derived from biomaterials to enhance the sustainability of the product. These include date seeds,386 eucalyptus leaves,409 marigold flowers,410 krill oil,411 and peanut skins.412 While initial data are promising, the economic feasibility and availability of the raw materials have not yet been assessed.
7.7. The fragmentation rate of microplastics under weathering from exposure to UV radiation is determined not only by their chemical class but also by their levels of pre-oxidation
Plastic litter is converted into microplastics (MPs) by fragmentation as a result of UV-initiated natural weathering in the marine environment.413,414 Their fate, especially further fragmentation into micro- and nanoplastics, is believed to be primarily determined by the chemical class of the polymer.415 However, polyethylene samples pre-oxidised to different extents by exposure to UV radiation for up to 30 days in the laboratory responded differently upon subsequent field exposure in the ocean. The extent of pre-degradation influenced the rates of further oxidation, fragmentation and biofouling.416 Fragmentation was minimal in samples that were not pre-oxidised. In a separate study, agitating common packaging plastics with marine sand or aggregate generated MPs.417,418 This implies that some of the secondary MPs in oceans may be produced without the assistance of solar UV radiation.
The presence of MPs in every ecosystem is well documented,225 but their impact on ecosystems or biota is still not well understood (see also section 4.3). Possible impacts of MPs in waterways include concentrating toxic organic contaminants and making them potentially bioavailable to aquatic animals via ingestion. MPs irreversibly absorbing organic contaminants can also sink and settle on the ocean floor. The full ecological impact of the presence of microplastic contamination in the bottom sediment requires further study.
Author contributions
All authors of this article have contributed equally.
Conflicts of interest
There are no conflicts of interest.
Acknowledgements
The views expressed in this article are those of the authors and do not necessarily represent the views or policies of the U.S. Environmental Protection Agency.
Generous contributions by UNEP/Ozone Secretariat for the convened author meeting, and support for the following authors are acknowledged: Pieter Aucamp, Amy Austin, Carlos Ballaré, Anastazia Banaszak, Hong Li, and Krishna Pandey.
Support by the U.S. Global Change Research Program is gratefully acknowledged for: Anthony Andrady, Paul Barnes (also supported by the J.H. Endowment for Environmental Biology), Germar Bernhard, Sasha Madronich, Kevin Rose (also supported by NSF DEB grant 1754265), and Craig Williamson (also supported by NSF DEB-1754276).
The following funding and support is also gratefully acknowledged by: Marcel Jansen (Science Foundation Ireland (16-IA-4418)), Donat-P. Häder (Bundesministerium für Umwelt Naturschutz und Reaktorsicherheit), Samuel Hylander (the Swedish Environmental Protection Agency and Linnaeus University), Patrick Neale (Smithsonian Institution), Rachel Neale (NHMRC Research Fellowship), Lesley Rhodes (NIHR Manchester Biomedical Research Centre), Robyn Lucas (NHMRC Senior Research Fellowship), Sharon Robinson (Australian Research Council and the University of Wollongong's Global Challenges Program), Matthew Robson (partially supported by the University of Helsinki, Faculty of Biological & Environmental Sciences, and by the Academy of Finland (#324555)), Stephen Wilson (University of Wollongong), Seyhan Yazar (Australian National Health and Medical Research Council CJ Martin Fellowship), and Richard Zepp (US Environmental Protection Agency).
Anastazia Banaszak thanks M. en C. Laura Celis for help with literature searches.
References
- A. F. Bais, G. Bernhard, R. L. McKenzie, P. J. Aucamp, P. J. Young, M. Ilyas, P. Jöckel and M. Deushi, Ozone–climate interactions and effects on solar ultraviolet radiation, Photochem. Photobiol. Sci., 2019, 18, 602–640 RSC.
- R. McKenzie, G. Bernhard, B. Liley, P. Disterhoft, S. Rhodes, A. Bais, O. Morgenstern, P. Newman, L. Oman, C. Brogniez and S. Simic, Success of Montreal Protocol demonstrated by comparing high-quality UV measurements with “World Avoided” calculations from two chemistry-climate models, Sci. Rep., 2019, 9, 12332 CrossRef PubMed.
- K. Lamy, T. Portafaix, B. Josse, C. Brogniez, S. Godin-Beekmann, H. Bencherif, L. Revell, H. Akiyoshi, S. Bekki, M. I. Hegglin, P. Jöckel, O. Kirner, B. Liley, V. Marecal, O. Morgenstern, A. Stenke, G. Zeng, N. L. Abraham, A. T. Archibald, N. Butchart, M. P. Chipperfield, G. Di Genova, M. Deushi, S. S. Dhomse, R.-M. Hu, D. Kinnison, M. Kotkamp, R. McKenzie, M. Michou, F. M. Connor, L. D. Oman, G. Pitari, D. A. Plummer, J. A. Pyle, E. Rozanov, D. Saint-Martin, K. Sudo, T. Y. Tanaka, D. Visioni and K. Yoshida, Clear-sky ultraviolet radiation modelling using output from the Chemistry Climate Model Initiative, Atmos. Chem. Phys., 2019, 19, 10087–10110 CrossRef CAS.
- O. Morgenstern, M. I. Hegglin, E. Rozanov, F. M. O'Connor, N. L. Abraham, H. Akiyoshi, A. T. Archibald, S. Bekki, N. Butchart, M. P. Chipperfield, M. Deushi, S. S. Dhomse, R. R. Garcia, S. C. Hardiman, L. W. Horowitz, P. Jöckel, B. Josse, D. Kinnison, M. Lin, E. Mancini, M. E. Manyin, M. Marchand, V. Marécal, M. Michou, L. D. Oman, G. Pitari, D. A. Plummer, L. E. Revell, D. Saint-Martin, R. Schofield, A. Stenke, K. Stone, K. Sudo, T. Y. Tanaka, S. Tilmes, Y. Yamashita, K. Yoshida and G. Zeng, Review of the global models used within phase 1 of the Chemistry–Climate Model Initiative (CCMI), Geosci. Model Dev., 2017, 10, 639–671 CrossRef.
- V. Eyring, J.-F. Lamarque, P. Hess, F. Arfeuille, K. Bowman, M. P. Chipperfiel, B. Duncan, A. Fiore, A. Gettelman and M. A. Giorgetta, Overview of IGAC/SPARC Chemistry-Climate Model Initiative (CCMI) community simulations in support of upcoming ozone and climate assessments, SPARC Newsletter, 2013, 40, 48–66 Search PubMed.
- D. P. van Vuuren, J. Edmonds, M. Kainuma, K. Riahi, A. Thomson, K. Hibbard, G. C. Hurtt, T. Kram, V. Krey, J.-F. Lamarque, T. Masui, M. Meinshausen, N. Nakicenovic, S. J. Smith and S. K. Rose, The representative concentration pathways: an overview, Clim. Change, 2011, 109, 5–31 CrossRef.
- S. Kinne, D. O'Donnel, P. Stier, S. Kloster, K. Zhang, H. Schmidt, S. Rast, M. Giorgetta, T. F. Eck and B. Stevens, MAC-v1: A new global aerosol climatology for climate studies, J. Adv. Model. Earth Syst., 2013, 5, 704–740 CrossRef.
- A. S. Pastukhova, N. E. Chubarova, Y. Y. Zhdanova, V. Y. Galin and S. P. Smyshlyaev, Numerical simulation of variations in ozone content, erythemal ultraviolet radiation, and ultraviolet resources over Northern Eurasia in the 21st century, Izv. Atmos. Ocean. Phys., 2019, 55, 242–250 CrossRef.
- G. Chiodo, L. M. Polvani, D. R. Marsh, A. Stenke, W. Ball, E. Rozanov, S. Muthers and K. Tsigaridis, The response of the ozone layer to quadrupled CO2 concentrations, J. Clim., 2018, 31, 3893–3907 CrossRef.
-
IPCC, in Climate change 2014: synthesis report. Contribution of Working Groups I, II and III to the fifth assessment report of the Intergovernmental Panel on Climate Change, ed. R. K. Pachauri and L. A. Meyer, IPCC, Geneva, Switzerland, 2014, p. 151 Search PubMed.
- G. H. Bernhard, V. E. Fioletov, J.-U. Grooß, I. Ialongo, B. Johnsen, K. Lakkala, G. L. Manney and R. Müller, Ozone and UV radiation [in “State of the Climate in 2018”], Bull. Am. Meteorol. Soc., 2019, 100, S165–S168 Search PubMed.
- B. Rösner, I. Benedict, C. Van Heerwaarden, A. Weerts, W. Hazeleger, P. Bissolli and K. Trachte, The long heat wave and drought in Europe in 2018 [in “State of the Climate in 2018”], Bull. Am. Meteorol. Soc., 2019, 100, S222–S223 Search PubMed.
- M. E. Mann, S. Rahmstorf, K. Kornhuber, B. A. Steinman, S. K. Miller and D. Coumou, Influence of anthropogenic climate change on planetary wave resonance and extreme weather events, Sci. Rep., 2017, 7, 45242 CrossRef CAS PubMed.
- J. Kuttippurath, P. Kumar, P. J. Nair and P. C. Pandey, Emergence of ozone recovery evidenced by reduction in the occurrence of Antarctic ozone loss saturation, npj Clim. Atmos. Sci., 2018, 1, 42 CrossRef.
- A. Pazmiño, S. Godin-Beekmann, A. Hauchecorne, C. Claud, S. Khaykin, F. Goutail, E. Wolfram, J. Salvador and E. Quel, Multiple symptoms of total ozone recovery inside the Antarctic vortex during austral spring, Atmos. Chem. Phys., 2018, 18, 7557–7572 CrossRef.
- W. Steinbrecht, M. I. Hegglin, N. Harris and M. Weber, Is global ozone recovering?, C. R. Geosci., 2018, 350, 368–375 CrossRef.
- C. Wilka, K. Shah, K. Stone, S. Solomon, D. Kinnison, M. Mills, A. Schmidt and R. R. Neely, On the role of heterogeneous chemistry in ozone depletion and recovery, Geophys. Res. Lett., 2018, 45, 7835–7842 CrossRef CAS.
- S. Solomon, D. J. Ivy, D. Kinnison, M. J. Mills, R. R. Neely and A. Schmidt, Emergence of healing in the Antarctic ozone layer, Science, 2016, 353, 269–274 CrossRef CAS PubMed.
-
C. R. Booth, T. B. Lucas, J. H. Morrow, S. Weiler and P. Penhale, The United States national science foundation's polar network for monitoring ultraviolet radiation, in AGU Antarctic Research Series, ed. C. S. Weiler and P. A. Penhale, AGU, Washington, 1994, pp. 17–37 Search PubMed.
- G. Bernhard, C. R. Booth and J. C. Ehramjian, Version 2 data of the National Science Foundation's Ultraviolet Radiation Monitoring Network: South Pole, J. Geophys. Res.: Atmos., 2004, 109, D21207 CrossRef.
- S.-W. Son, B.-R. Han, C. I. Garfinkel, S.-Y. Kim, R. Park, N. L. Abraham, H. Akiyoshi, A. T. Archibald, N. Butchart, M. P. Chipperfield, M. Dameris, M. Deushi, S. S. Dhomse, S. C. Hardiman, P. Jöckel, D. Kinnison, M. Michou, O. Morgenstern, F. M. O'Connor, L. D. Oman, D. A. Plummer, A. Pozzer, L. E. Revell, E. Rozanov, A. Stenke, K. Stone, S. Tilmes, Y. Yamashita and G. Zeng, Tropospheric jet response to Antarctic ozone depletion: An update with Chemistry-Climate Model Initiative (CCMI) models, Environ. Res. Lett., 2018, 13, 054024 CrossRef.
- W. J. M. Seviour, F. Codron, E. W. Doddridge, D. Ferreira, A. Gnanadesikan, M. Kelley, Y. Kostov, J. Marshall, L. M. Polvani, J. L. Thomas and D. W. Waugh, The southern ocean sea surface temperature response to ozone depletion: a multimodel comparison, J. Clim., 2019, 32, 5107–5121 CrossRef.
- Z. E. Gillett, J. M. Arblaster, A. J. Dittus, M. Deushi, P. Jöckel, D. E. Kinnison, O. Morgenstern, D. A. Plummer, L. E. Revell, E. Rozanov, R. Schofield, A. Stenke, K. A. Stone and S. Tilmes, Evaluating the relationship between interannual variations in the Antarctic ozone hole and southern hemisphere surface climate in chemistry–climate models, J. Clim., 2019, 32, 3131–3151 CrossRef.
- G. A. Meehl, J. M. Arblaster, C. T. Y. Chung, M. M. Holland, A. DuVivier, L. Thompson, D. Yang and C. M. Bitz, Sustained ocean changes contributed to sudden Antarctic sea ice retreat in late 2016, Nat. Commun., 2019, 10, 14 CrossRef CAS PubMed.
- G. Wang, H. H. Hendon, J. M. Arblaster, E. P. Lim, S. Abhik and P. van Rensch, Compounding tropical and stratospheric forcing of the record low Antarctic sea-ice in 2016, Nat. Commun., 2019, 10, 13 CrossRef CAS PubMed.
- Z. Wang, J. Turner, Y. Wu and C. Liu, Rapid Decline of Total Antarctic Sea Ice Extent during 2014–16 Controlled by Wind-Driven Sea Ice Drift, J. Clim., 2019, 32, 5381–5395 CrossRef.
- F. Xie, X. Ma, J. Li, J. Huang, W. Tian, J. Zhang, Y. Hu, C. Sun, X. Zhou, J. Feng and Y. Yang, An advanced impact of Arctic stratospheric ozone changes on spring precipitation in China, Clim. Dyn., 2018, 51, 4029–4041 CrossRef.
- K. A. Stone, S. Solomon, D. E. Kinnison, C. F. Baggett and E. A. Barnes, Prediction of northern hemisphere regional surface temperatures using stratospheric ozone information, J. Geophys. Res.: Atmos., 2019, 124, 5922–5933 Search PubMed.
- M. Aun, K. Eerme, I. Ansko and M. Aun, Daily, seasonal, and annual characteristics of UV radiation and its influencing factors in Tõravere, Estonia, 2004–2016, Theor. Appl. Climatol., 2019, 138, 887–897 CrossRef.
- N. E. Chubarova, A. S. Pastukhova, V. Y. Galin and S. P. Smyshlyaev, Long-Term variability of UV irradiance in the Moscow region according to measurement and modeling data, Izv. Atmos. Ocean. Phys., 2018, 54, 139–146 CrossRef.
- I. Fountoulakis, C. S. Zerefos, A. F. Bais, J. Kapsomenakis, M.-E. Koukouli, N. Ohkawara, V. Fioletov, H. De Backer, K. Lakkala, T. Karppinen and A. R. Webb, Twenty-five years of spectral UV-B measurements over Canada, Europe and Japan: Trends and effects from changes in ozone, aerosols, clouds, and surface reflectivity, C. R. Geosci., 2018, 350, 393–402 CrossRef.
- S. Kazadzis, D. Founda, B. E. Psiloglou, H. Kambezidis, N. Mihalopoulos, A. Sanchez-Lorenzo, C. Meleti, P. I. Raptis, F. Pierros and P. Nabat, Long-term series and trends in surface solar radiation in Athens, Greece, Atmos. Chem. Phys., 2018, 18, 2395 CrossRef CAS.
- H. Zhang, J. Wang, L. Castro García, J. Zeng, C. Dennhardt, Y. Liu and N. A. Krotkov, Surface erythemal UV irradiance in the continental United States derived from ground-based and OMI observations: quality assessment, trend analysis and sampling issues, Atmos. Chem. Phys., 2019, 19, 2165–2181 CrossRef CAS.
- G. E. Bodeker, J. C. Scott, K. Kreher and R. L. McKenzie, Global ozone trends in potential vorticity coordinates using TOMS and GOME intercompared against the Dobson network: 1978–1998, J. Geophys. Res., 2001, 106, 23029–23042 CrossRef CAS.
- J. Mok, N. A. Krotkov, O. Torres, H. Jethva, Z. Li, J. Kim, J.-H. Koo, S. Go, H. Irie, G. Labow, T. F. Eck, B. N. Holben, J. Herman, R. P. Loughman, E. Spinei, S. S. Lee, P. Khatri and M. Campanelli, Comparisons of spectral aerosol single scattering albedo in Seoul, South Korea, Atmos. Meas. Tech., 2018, 11, 2295–2311 CrossRef CAS.
- I. Fountoulakis, A. Natsis, N. Siomos, T. Drosoglou and A. F. Bais, Deriving aerosol absorption properties from solar ultraviolet radiation spectral measurements at Thessaloniki, Greece, Remote Sens., 2019, 11, 2179 CrossRef.
- J. Mok, N. A. Krotkov, A. Arola, O. Torres, H. Jethva, M. Andrade, G. Labow, T. F. Eck, Z. Li, R. R. Dickerson, G. L. Stenchikov, S. Osipov and X. Ren, Impacts of brown carbon from biomass burning on surface UV and ozone photochemistry in the Amazon Basin, Sci. Rep., 2016, 6, 36940 CrossRef CAS PubMed.
- P. F. Levelt, J. Joiner, J. Tamminen, J. P. Veefkind, P. K. Bhartia, D. C. Stein Zweers, B. N. Duncan, D. G. Streets, H. Eskes, A. R. van der, C. McLinden, V. Fioletov, S. Carn, J. de Laat, M. DeLand, S. Marchenko, R. McPeters, J. Ziemke, D. Fu, X. Liu, K. Pickering, A. Apituley, G. González Abad, A. Arola, F. Boersma, C. Chan Miller, K. Chance, M. de Graaf, J. Hakkarainen, S. Hassinen, I. Ialongo, Q. Kleipool, N. Krotkov, C. Li, L. Lamsal, P. Newman, C. Nowlan, R. Suleiman, L. G. Tilstra, O. Torres, H. Wang and K. Wargan, The Ozone Monitoring Instrument: overview of 14 years in space, Atmos. Chem. Phys., 2018, 18, 5699–5745 CrossRef CAS.
- O. Torres, P. K. Bhartia, H. Jethva and C. Ahn, Impact of the ozone monitoring instrument row anomaly on the long-term record of aerosol products, Atmos. Meas. Tech., 2018, 11, 2701–2715 CrossRef CAS.
- L. Flynn, C. Long, X. Wu, R. Evans, C. T. Beck, I. Petropavlovskikh, G. McConville, W. Yu, Z. Zhang and J. Niu, Performance of the ozone mapping and profiler suite (OMPS) products, J. Geophys. Res.: Atmos., 2014, 119, 6181–6195 Search PubMed.
- A. V. Lindfors, J. Kujanpää, N. Kalakoski, A. Heikkilä, K. Lakkala, T. Mielonen, M. Sneep, N. A. Krotkov, A. Arola and J. Tamminen, The TROPOMI surface UV algorithm, Atmos. Meas. Tech., 2018, 11, 997–1008 CrossRef CAS.
- U. Cortesi, S. Ceccherini, S. Del Bianco, M. Gai, C. Tirelli, N. Zoppetti, F. Barbara, M. Bonazountas, A. Argyridis, A. Bós, E. Loenen, A. Arola, J. Kujanpää, A. Lipponen, W. Wandji Nyamsi, A. R. van der, J. van Peet, O. Tuinder, V. Farruggia, A. Masini, E. Simeone, R. Dragani, A. Keppens, J.-C. Lambert, M. van Roozendael, C. Lerot, H. Yu and K. Verberne, Advanced ultraviolet radiation and ozone retrieval for applications (AURORA): A project overview, Atmosphere, 2018, 9, 454 CrossRef.
- S. Madronich, L. O. Björn and R. L. McKenzie, Solar UV radiation and microbial life in the atmosphere, Photochem. Photobiol. Sci., 2018, 17, 1918–1931 RSC.
- S. A. Montzka, G. S. Dutton, P. Yu, E. Ray, R. W. Portmann, J. S. Daniel, L. Kuijpers, B. D. Hall, D. Mondeel, C. Siso, J. D. Nance, M. Rigby, A. J. Manning, L. Hu, F. Moore, B. R. Miller and J. W. Elkins, An unexpected and persistent increase in global emissions of ozone-depleting CFC-11, Nature, 2018, 557, 413–417 CrossRef CAS PubMed.
- Y. Lin, D. Gong, S. Lv, Y. Ding, G. Wu, H. Wang, Y. Li, Y. Wang, L. Zhou and B. Wang, Observations of high levels of ozone-depleting CFC-11 at a remote mountain-top site in Southern China, Environ. Sci. Technol. Lett., 2019, 6, 114–118 CrossRef CAS.
- M. Rigby, S. Park, T. Saito, L. M. Western, A. L. Redington, X. Fang, S. Henne, A. J. Manning, R. G. Prinn, G. S. Dutton, P. J. Fraser, A. L. Ganesan, B. D. Hall, C. M. Harth, J. Kim, K. R. Kim, P. B. Krummel, T. Lee, S. Li, Q. Liang, M. F. Lunt, S. A. Montzka, J. Muhle, S. O'Doherty, M. K. Park, S. Reimann, P. K. Salameh, P. Simmonds, R. L. Tunnicliffe, R. F. Weiss, Y. Yokouchi and D. Young, Increase in CFC-11 emissions from eastern China based on atmospheric observations, Nature, 2019, 569, 546–550 CrossRef CAS PubMed.
- N. R. P. Harris, S. A. Montzka and P. A. Newman, Report on the International Symposium on the Unexpected Increase in Emissions of Ozone-Depleting CFC-11, SPARC Newsletter, 2019, 53, 9–18 Search PubMed.
- R. D. Russotto and T. P. Ackerman, Changes in clouds and thermodynamics under solar geoengineering and implications for required solar reduction, Atmos. Chem. Phys., 2018, 18, 11905–11925 CrossRef CAS.
- S. Madronich, S. Tilmes, B. Kravitz, D. MacMartin and J. Richter, Response of surface ultraviolet and visible radiation to stratospheric SO2 injections, Atmosphere, 2018, 9, 432 CrossRef CAS.
- C. M. Olsen, A. C. Green, N. Pandeya and D. C. Whiteman, Trends in melanoma incidence rates in eight susceptible populations through 2015, J. Invest. Dermatol., 2019, 139, 1392–1395 CrossRef CAS PubMed.
- D. M. Holman, M. B. Freeman and M. L. Shoemaker, Trends in melanoma incidence among non-hispanic whites in the United States, 2005 to 2014, JAMA Dermatol., 2018, 154, 361–362 CrossRef PubMed.
- J. F. Aitken, D. R. Youlden, P. D. Baade, H. P. Soyer, A. C. Green and B. M. Smithers, Generational shift in melanoma incidence and mortality in Queensland, Australia, 1995–2014, Int. J. Cancer, 2018, 142, 1528–1535 CrossRef CAS PubMed.
- L. Sacchetto, R. Zanetti, H. Comber, C. Bouchardy, D. H. Brewster, P. Broganelli, M. D. Chirlaque, D. Coza, J. Galceran, A. Gavin, M. Hackl, A. Katalinic, S. Laronningen, M. W. J. Louwman, E. Morgan, T. E. Robsahm, M. J. Sanchez, L. Tryggvadottir, R. Tumino, E. Van Eycken, S. Vernon, V. Zadnik and S. Rosso, Trends in incidence of thick, thin and in situ melanoma in Europe, Eur. J. Cancer, 2018, 92, 108–118 CrossRef CAS PubMed.
- K. Greveling, M. Wakkee, T. Nijsten, R. R. van den Bos and L. M. Hollestein, Epidemiology of lentigo maligna and lentigo maligna melanoma in the Netherlands, 1989–2013, J. Invest. Dermatol., 2016, 136, 1955–1960 CrossRef CAS PubMed.
- C. Matas-Nadal, J. Malvehy, J. R. Ferreres, A. Boada, D. Bodet, S. Segura, M. Salleras, A. Azon, S. Bel-Pla, X. Bigata, A. Campoy, N. Curcó, J. Dalmau, M. Formigon, A. Gonzalez, M. Just, E. Llistosella, M. E. Nogues, R. Pedragosa, J. A. Pujol, M. Sabat, J. A. Smandia, P. Zaballos, S. Puig and R. M. Martí, Increasing incidence of lentigo maligna and lentigo maligna melanoma in Catalonia, Int. J. Dermatol., 2019, 58, 577–581 CrossRef PubMed.
- D. C. Whiteman, A. C. Green and C. M. Olsen, The growing burden of invasive melanoma: projections of incidence rates and numbers of new cases in six susceptible populations through 2031, J. Invest. Dermatol., 2016, 136, 1161–1171 CrossRef CAS PubMed.
- C. Garbe, U. Keim, T. Eigentler, T. Amaral, A. Katalinic, B. Holleczek, P. Martus and U. Leiter, Time trends in incidence and mortality of cutaneous melanoma in Germany, J. Eur. Acad. Dermatol. Venereol., 2019, 33, 1272–1280 CrossRef CAS PubMed.
- F. Bray, J. Ferlay, I. Soerjomataram, R. L. Siegel, L. A. Torre and A. Jemal, Global cancer statistics 2018: GLOBOCAN estimates of incidence and mortality worldwide for 36 cancers in 185 countries, Ca-Cancer J. Clin., 2018, 68, 394–424 CrossRef PubMed.
- C. Karimkhani, A. C. Green, T. Nijsten, M. A. Weinstock, R. P. Dellavalle, M. Naghavi and C. Fitzmaurice, The global burden of melanoma: results from the Global Burden of Disease Study 2015, Br. J. Dermatol., 2017, 177, 134–140 CrossRef CAS PubMed.
- M. Arnold, E. de Vries, D. C. Whiteman, A. Jemal, F. Bray, D. M. Parkin and I. Soerjomataram, Global burden of cutaneous melanoma attributable to ultraviolet radiation in 2012, Int. J. Cancer, 2018, 143, 1305–1314 CrossRef CAS PubMed.
- D. E. O'Sullivan, D. R. Brenner, P. J. Villeneuve, S. D. Walter, P. A. Demers, C. M. Friedenreich and W. D. King, Estimates of the current and future burden of melanoma attributable to ultraviolet radiation in Canada, Prev. Med., 2019, 122, 81–90 CrossRef PubMed.
- M. Arnold, M. Kvaskoff, A. Thuret, P. Guenel, F. Bray and I. Soerjomataram, Cutaneous melanoma in France in 2015 attributable to solar ultraviolet radiation and the use of sunbeds, J. Eur. Acad. Dermatol. Venereol., 2018, 32, 1681–1686 CrossRef CAS PubMed.
- I. Savoye, C. M. Olsen, D. C. Whiteman, A. Bijon, L. Wald, L. Dartois, F. Clavel-Chapelon, M. C. Boutron-Ruault and M. Kvaskoff, Patterns ofultraviolet radiation exposure and skin cancer risk: the E3N-SunExp Study, J. Epidemiol., 2018, 28, 27–33 CrossRef PubMed.
- C. M. Olsen, N. Pandeya, M. H. Law, S. MacGregor, M. M. Iles, B. S. Thompson, A. C. Green, R. E. Neale and D. C. Whiteman, Does polygenic risk influence associations between sun exposure and melanoma? A prospective cohort analysis, Br. J. Dermatol., 2019 DOI:10.1111/bjd.18703.
- D. C. Whiteman, P. G. Parsons and A. C. Green, p53 expression and risk factors for cutaneous melanoma: a case-control study, Int. J. Cancer, 1998, 77, 843–848 CrossRef CAS PubMed.
- D. C. Whiteman, M. Stickley, P. Watt, M. C. Hughes, M. B. Davis and A. C. Green, Anatomic site, sun exposure, and risk of cutaneous melanoma, J. Clin. Oncol., 2006, 24, 3172–3177 CrossRef PubMed.
- D. C. Whiteman, P. Watt, D. M. Purdie, M. C. Hughes, N. K. Hayward and A. C. Green, Melanocytic nevi, solar keratoses, and divergent pathways to cutaneous melanoma, J. Natl. Cancer Inst., 2003, 95, 806–812 CrossRef PubMed.
- M. C. Cameron, E. Lee, B. P. Hibler, C. A. Barker, S. Mori, M. Cordova, K. S. Nehal and A. M. Rossi, Basal cell carcinoma: Epidemiology; pathophysiology; clinical and histological subtypes; and disease associations, J. Am. Acad. Dermatol., 2019, 80, 303–317 CrossRef PubMed.
- P. Adelson, G. R. Sharplin, D. M. Roder and M. Eckert, Keratinocyte cancers in South Australia: incidence, geographical variability and service trends, Aust. N. Z. J. Public Health, 2018, 42, 329–333 CrossRef PubMed.
- A. Pondicherry, R. Martin, I. Meredith, J. Rolfe, P. Emanuel and M. Elwood, The burden of non-melanoma skin cancers in Auckland, New Zealand, Australas. J. Dermatol., 2018, 59, 210–213 CrossRef PubMed.
- C. Anselmo Lima, M. Sampaio Lima, A. Maria Da Silva, M. A. Prado Nunes, M. M. Macedo Lima, M. Oliveira Santos, D. Lyra and C. Kleber Alves, Do cancer registries play a role in determining the incidence of non-melanoma skin cancers?, Eur. J. Dermatol., 2018, 28, 169–176 Search PubMed.
- C. M. Oh, H. Cho, Y. J. Won, H. J. Kong, Y. H. Roh, K. H. Jeong and K. W. Jung, Nationwide trends in the incidence of melanoma and non-melanoma skin cancers from 1999 to 2014 in south korea, Cancer Res. Treat., 2018, 50, 729–737 CrossRef PubMed.
- Z. C. Venables, P. Autier, T. Nijsten, K. F. Wong, S. M. Langan, B. Rous, J. Broggio, C. Harwood, K. Henson, C. M. Proby, J. Rashbass and I. M. Leigh, Nationwide incidence of metastatic cutaneous squamous cell carcinoma in England, JAMA Dermatol., 2019, 155, 298–306 CrossRef PubMed.
- E. C. Noels, L. M. Hollestein, S. van Egmond, M. Lugtenberg, L. P. J. van Nistelrooij, P. J. E. Bindels, J. van der Lei, R. S. Stern, T. Nijsten and M. Wakkee, Healthcare utilization and management of actinic keratosis in primary and secondary care: a complementary database analysis, Br. J. Dermatol., 2019, 181, 544–553 CrossRef CAS PubMed.
- J. H. Lee, Y. H. Kim, K. D. Han, Y. M. Park, J. Y. Lee, Y. G. Park and Y. B. Lee, Incidence of actinic keratosis and risk of skin cancer in subjects with actinic keratosis: A population-based cohort study, Acta Derm.-Venereol., 2018, 98, 382–383 CrossRef PubMed.
- S. C. Flohil, R. J. van der Leest, E. A. Dowlatshahi, A. Hofman, E. de Vries and T. Nijsten, Prevalence of actinic keratosis and its risk factors in the general population: the Rotterdam Study, J. Invest. Dermatol., 2013, 133, 1971–1978 CrossRef CAS PubMed.
- P. J. Dziunycz, E. Schuller and G. F. L. Hofbauer, Prevalence of actinic keratosis in patients attending general practitioners in Switzerland, Dermatology, 2018, 234, 214–219 CrossRef PubMed.
- C. Ferrándiz-Pulido, M. Lera-Imbuluzqueta, C. Ferrándiz and M. J. Plazas-Fernandez, Prevalence of Actinic Keratosis in Different Regions of Spain: The EPIQA Study, Actas Dermo-Sifiliogr., 2018, 109, 83–86 CrossRef PubMed.
- H. Yeung, M. L. Baranowski, R. A. Swerlick, S. C. Chen, J. Hemingway, D. R. Hughes and R. Duszak, Use and cost of actinic keratosis destruction in the Medicare part B fee-for-service population, 2007 to 2015, JAMA Dermatol., 2018, 154, 1281–1285 CrossRef PubMed.
- D. A. Tran, A. C. Coronado, S. Sarker and R. Alvi, Estimating the health care costs of non-melanoma skin cancer in Saskatchewan using physician billing data, Curr. Oncol., 2019, 26, 114–118 CAS.
- C. K. Park, K. Fung, P. C. Austin, S. J. Kim, L. G. Singer, N. N. Baxter, P. A. Rochon and A. W. Chan, Incidence and Risk Factors of Keratinocyte Carcinoma After First Solid Organ Transplant in Ontario, Canada, JAMA Dermatol., 2019, 155, 1041–1048 CrossRef PubMed.
-
Organ procurement and transplantation network, Organ transplants in United States set sixth consecutive record in 2018, 2019, [cited 13 September 2019]; available from: https://optn.transplant.hrsa.gov/news/organ-transplants-in-united-states-set-sixth-consecutive-record-in-2018/ Search PubMed.
- J. C. van der Leun, R. D. Piacentini and F. R. de Gruijl, Climate change and human skin cancer, Photochem. Photobiol. Sci., 2008, 7, 730–733 RSC.
- R. D. Piacentini, L. S. Della Ceca and A. Ipina, Climate change and its relationship with non-melanoma skin cancers, Photochem. Photobiol. Sci., 2018, 17, 1913–1917 RSC.
- H. Slaper, G. J. Velders, J. S. Daniel, F. R. de Gruijl and J. C. van der Leun, Estimates of ozone depletion and skin cancer incidence to examine the Vienna Convention achievements, Nature, 1996, 384, 256–258 CrossRef CAS PubMed.
- D. Gutierrez, J. V. Gaulding, A. F. Motta Beltran, H. W. Lim and E. N. Pritchett, Photodermatoses in skin of colour, J. Eur. Acad. Dermatol. Venereol., 2018, 32, 1879–1886 CrossRef CAS PubMed.
- K. J. Rutter, I. Ashraf, L. Cordingley and L. E. Rhodes, Quality of life and psychological impact in the photodermatoses: a systematic review, Br. J. Dermatol., 2019 DOI:10.1111/bjd.18326.
- A. Swaminathan, S. L. Harrison, N. Ketheesan, C. H. A. van den Boogaard, K. Dear, M. Allen, P. H. Hart, M. Cook and R. M. Lucas, Exposure to solar UVR suppresses cell-mediated immunization responses in humans: The Australian Ultraviolet Radiation and Immunity Study, J. Invest. Dermatol., 2019, 139, 1545–1553 CrossRef CAS PubMed.
- E. Valencia-Vera, J. Aguilera, A. Cobos, J. L. Bernabo, V. Perez-Valero and E. Herrera-Ceballos, Association between seasonal serum folate levels and ultraviolet radiation, J. Photochem. Photobiol., B, 2019, 190, 66–71 CrossRef CAS PubMed.
- F. Rezvan, M. Khabazkhoob, E. Hooshmand, A. Yekta, M. Saatchi and H. Hashemi, Prevalence and risk factors of pterygium: a systematic review and meta-analysis, Surv. Ophthalmol., 2018, 63, 719–735 CrossRef PubMed.
- D. R. Garzon-Chavez, E. Quentin, S. L. Harrison, A. V. Parisi, H. J. Butler and N. J. Downs, The geospatial relationship of pterygium and senile cataract with ambient solar ultraviolet in tropical Ecuador, Photochem. Photobiol. Sci., 2018, 17, 1075–1083 RSC.
- P. Song, H. Wang, E. Theodoratou, K. Y. Chan and I. Rudan, The national and subnational prevalence of cataract and cataract blindness in China: a systematic review and meta-analysis, J. Glob. Health, 2018, 8, 010804 CrossRef PubMed.
- A. Bastawrous, W. Mathenge, J. Nkurikiye, K. Wing, H. Rono, M. Gichangi, H. A. Weiss, D. Macleod, A. Foster, M. Burton and H. Kuper, Incidence of visually impairing cataracts among older adults in Kenya, JAMA Netw. Open, 2019, 2, e196354 CrossRef PubMed.
- H. Zhou, H. Zhang, A. Yu and J. Xie, Association between sunlight exposure and risk of age-related macular degeneration: a meta-analysis, BMC Ophthalmol., 2018, 18, 331 CrossRef PubMed.
- V. Patra, K. Wagner, V. Arulampalam and P. Wolf, Skin microbiome modulates the effect of ultraviolet radiation on cellular response and immune function, iScience, 2019, 15, 211–222 CrossRef CAS PubMed.
- M. Zawrotniak, D. Bartnicka and M. Rapala-Kozik, UVA and UVB radiation induce the formation of neutrophil extracellular traps by human polymorphonuclear cells, J. Photochem. Photobiol., B, 2019, 196, 111511 CrossRef CAS PubMed.
- N. Schweintzger, A. Gruber-Wackernagel, E. Reginato, I. Bambach, F. Quehenberger, S. N. Byrne and P. Wolf, Levels and function of regulatory T cells in patients with polymorphic light eruption: relation to photohardening, Br. J. Dermatol., 2015, 173, 519–526 CrossRef CAS PubMed.
- R. S. Hesterberg, R. P. Amorrortu, Y. Zhao, S. Hampras, A. A. Akuffo, N. Fenske, B. Cherpelis, J. Balliu, L. Vijayan, P. K. Epling-Burnette and D. E. Rollison, T regulatory cell subpopulations associated with recent ultraviolet radiation exposure in a skin cancer screening cohort, J. Immunol., 2018, 201, 3269–3281 CrossRef CAS PubMed.
- S. Trend, A. P. Jones, L. Cha, M. N. Cooper, S. Geldenhuys, M. J. Fabis-Pedrini, W. M. Carroll, J. M. Cole, D. R. Booth, R. M. Lucas, M. A. French, S. N. Byrne, A. G. Kermode and P. H. Hart, Short-term changes in frequencies of circulating leukocytes associated with narrowband UVB phototherapy in people with clinically isolated syndrome, Sci. Rep., 2019, 9, 7980 CrossRef PubMed.
- R. M. Lucas, S. N. Byrne, J. Correale, S. Ilschner and P. H. Hart, Ultraviolet radiation, vitamin D and multiple sclerosis, Neurodegener. Dis. Manage., 2015, 5, 413–424 CrossRef PubMed.
- E. A. Holmes, R. M. Rodney Harris and R. M. Lucas, Low sun exposure and vitamin d deficiency as risk factors for inflammatory bowel disease, with a focus on childhood onset, Photochem. Photobiol., 2019, 95, 105–118 CrossRef CAS PubMed.
- J. Narbutt, P. A. Philipsen, G. I. Harrison, K. A. Morgan, K. P. Lawrence, K. A. Baczynska, K. Grys, M. Rogowski-Tylman, I. Olejniczak-Staruch, A. Tewari, M. Bell, C. O'Connor, H. C. Wulf, A. Lesiak and A. R. Young, Sunscreen applied at ≥2 mg cm−2 during a sunny holiday prevents erythema, a biomarker of ultraviolet radiation-induced DNA damage and suppression of acquired immunity, Br. J. Dermatol., 2019, 180, 604–614 CrossRef CAS PubMed.
- J. B. Travers, J. Weyerbacher, J. A. Ocana, C. Borchers, C. M. Rapp and R. P. Sahu, Acute ethanol exposure augments low-dose uvb-mediated systemic immunosuppression via enhanced production of platelet-activating factor receptor agonists, J. Invest. Dermatol., 2019, 139, 1619–1622 CrossRef CAS PubMed.
- E. M. Cela, C. D. Gonzalez, A. Friedrich, C. Ledo, M. L. Paz, J. Leoni, M. I. Gomez and D. H. Gonzalez Maglio, Daily very low UV dose exposure enhances adaptive immunity, compared with a single high-dose exposure. Consequences for the control of a skin infection, Immunology, 2018, 154, 510–521 CrossRef CAS PubMed.
- S. N. Byrne, N. Spinks and G. M. Halliday, Ultraviolet-A irradiation of C57BL/6 mice suppresses systemic contact hypersensitivity or enhances secondary immunity depending on dose, J. Invest. Dermatol., 2002, 119, 858–864 CrossRef CAS PubMed.
- S. N. Byrne, N. Spinks and G. M. Halliday, The induction of immunity to a protein antigen using an adjuvant is significantly compromised by ultraviolet A radiation, J. Photochem. Photobiol.,
B, 2006, 84, 128–134 CrossRef CAS PubMed.
- Y. J. Matthews, G. M. Halliday, T. A. Phan and D. L. Damian, Wavelength dependency for UVA-induced suppression of recall immunity in humans, J. Dermatol. Sci., 2010, 59, 192–197 CrossRef CAS PubMed.
- J. E. Manson, N. R. Cook, I. M. Lee, W. Christen, S. S. Bassuk, S. Mora, H. Gibson, D. Gordon, T. Copeland, D. D'Agostino, G. Friedenberg, C. Ridge, V. Bubes, E. L. Giovannucci, W. C. Willett, J. E. Buring and V. R. Group, Vitamin D supplements and prevention of cancer and cardiovascular disease, N. Engl. J. Med., 2019, 380, 33–44 CrossRef CAS PubMed.
- M. Barbarawi, B. Kheiri, Y. Zayed, O. Barbarawi, H. Dhillon, B. Swaid, A. Yelangi, S. Sundus, G. Bachuwa, M. L. Alkotob and J. E. Manson, Vitamin D supplementation and cardiovascular disease risks in more than 83
000 individuals in 21 randomized clinical trials: A meta-analysis, JAMA Cardiol., 2019, 4, 765–776 CrossRef PubMed.
- N. Keum, D. H. Lee, D. C. Greenwood, J. E. Manson and E. Giovannucci, Vitamin D supplementation and total cancer incidence and mortality: a meta-analysis of randomized controlled trials, Ann. Oncol., 2019, 30, 733–743 CrossRef CAS PubMed.
- J. S. Ong, P. Gharahkhani, J. An, M. H. Law, D. C. Whiteman, R. E. Neale and S. MacGregor, Vitamin D and overall cancer risk and cancer mortality: a Mendelian randomization study, Hum. Mol. Genet., 2018, 27, 4315–4322 CAS.
- W. D. Thompson, J. Tyrrell, M. C. Borges, R. N. Beaumont, B. A. Knight, A. R. Wood, S. M. Ring, A. T. Hattersley, R. M. Freathy and D. A. Lawlor, Association of maternal circulating 25(OH)D and calcium with birth weight: A mendelian randomisation analysis, PLoS Med., 2019, 16, e1002828 CrossRef PubMed.
- J. Y. Sun, M. Zhao, Y. Hou, C. Zhang, J. Oh, Z. Sun and B. L. Sun, Circulating serum vitamin D levels and total body bone mineral density: A Mendelian randomization study, J. Cell. Mol. Med., 2019, 23, 2268–2271 CrossRef CAS PubMed.
- L. Libuda, B. H. Laabs, C. Ludwig, J. Buhlmeier, J. Antel, A. Hinney, R. Naaresh, M. Focker, J. Hebebrand, I. R. Konig and T. Peters, Vitamin D and the risk of depression: a causal relationship? Findings from a Mendelian randomization study, Nutrients, 2019, 11, 1085 CrossRef CAS PubMed.
- K. Michaelsson, H. Melhus and S. C. Larsson, Serum 25-hydroxyvitamin D concentrations and major depression: a Mendelian randomization study, Nutrients, 2018, 10, 1987 CrossRef PubMed.
- N. Wang, C. Wang, X. Chen, H. Wan, Y. Chen, C. Chen, B. Han and Y. Lu, Vitamin D, prediabetes and type 2 diabetes: bidirectional Mendelian randomization analysis, Eur. J. Nutr., 2019 DOI:10.1007/s00394-019-01990-x.
- A. Havdahl, R. Mitchell, L. Paternoster and G. Davey Smith, Investigating causality in the association between vitamin D status and self-reported tiredness, Sci. Rep., 2019, 9, 2880 CrossRef PubMed.
- J. Lund-Nielsen, S. Vedel-Krogh, C. J. Kobylecki, J. Brynskov, S. Afzal and B. G. Nordestgaard, Vitamin D and inflammatory bowel disease: Mendelian randomization analyses in the Copenhagen Studies and UK Biobank, J. Clin. Endocrinol. Metab., 2018, 103, 3267–3277 CrossRef PubMed.
- R. M. Lucas, S. Yazar, A. R. Young, M. Norval, F. R. de Gruijl, Y. Takizawa, L. E. Rhodes, C. A. Sinclair and R. E. Neale, Human health in relation to exposure to solar ultraviolet radiation under changing stratospheric ozone and climate, Photochem. Photobiol. Sci., 2019, 18, 641–680 RSC.
- P. Lips, K. D. Cashman, C. Lamberg-Allardt, H. A. Bischoff-Ferrari, B. R. Obermayer-Pietsch, M. Bianchi, J. Stepan, G. El-Hajj Fuleihan and R. Bouillon, Management of Endocrine Disease: Current vitamin D status in European and Middle East countries and strategies to prevent vitamin D deficiency; a position statement of the European Calcified Tissue Society, Eur. J. Endocrinol., 2019, 180, P23–P54 CAS.
- K. A. Herrick, R. J. Storandt, J. Afful, C. M. Pfeiffer, R. L. Schleicher, J. J. Gahche and N. Potischman, Vitamin D status in the United States, 2011–2014, Am. J. Clin. Nutr., 2019, 110, 150–157 CrossRef PubMed.
- A. R. Webb, A. Kazantzidis, R. C. Kift, M. D. Farrar, J. Wilkinson and L. E. Rhodes, Meeting Vitamin D Requirements in White Caucasians at UK Latitudes: Providing a Choice, Nutrients, 2018, 10, 497 CrossRef PubMed.
- A. R. Webb, A. Kazantzidis, R. C. Kift, M. D. Farrar, J. Wilkinson and L. E. Rhodes, Colour Counts: Sunlight and Skin Type as Drivers of Vitamin D Deficiency at UK Latitudes, Nutrients, 2018, 10, 457 CrossRef PubMed.
- M. D. Farrar, A. R. Webb, R. Kift, M. T. Durkin, D. Allan, A. Herbert, J. L. Berry and L. E. Rhodes, Efficacy of a dose range of simulated sunlight exposures in raising vitamin D status in South Asian adults: implications for targeted guidance on sun exposure, Am. J. Clin. Nutr., 2013, 97, 1210–1216 CrossRef CAS PubMed.
- H. Y. Park, Y. C. Hong, K. Lee and J. Koh, Vitamin D status and risk of non-Hodgkin lymphoma: An updated meta-analysis, PLoS One, 2019, 14, e0216284 CrossRef CAS PubMed.
- I. M. Michalek, J. I. Martinsen, E. Weiderpass, J. Hansen, P. Sparen, L. Tryggvadottir and E. Pukkala, Heavy metals, welding fumes, and other occupational exposures, and the risk of kidney cancer: A population-based nested case-control study in three Nordic countries, Environ. Res., 2019, 173, 117–123 CrossRef CAS PubMed.
- R. Scragg, J. Rahman and S. Thornley, Association of sun and UV exposure with blood pressure and cardiovascular disease: A systematic review, J. Steroid Biochem. Mol. Biol., 2019, 187, 68–75 CrossRef CAS PubMed.
- L. G. Gallagher, S. Ilango, A. Wundes, G. A. Stobbe, K. W. Turk, G. M. Franklin, M. S. Linet, D. M. Freedman, B. H. Alexander and H. Checkoway, Lifetime exposure to ultraviolet radiation and the risk of multiple sclerosis in the US radiologic technologists cohort study, Mult. Scler. J., 2019, 25, 1162–1169 CrossRef PubMed.
- N. Beck, J. A. G. Balanay and T. Johnson, Assessment of occupational exposure to heat stress and solar ultraviolet radiation among groundskeepers in an eastern North Carolina university setting, J. Occup. Environ. Hyg., 2018, 15, 105–116 CrossRef CAS PubMed.
- F. O'Sullivan, J. van Geffen, M. van Weele and L. Zgaga, Annual Ambient UVB at Wavelengths that Induce Vitamin D Synthesis is Associated with Reduced Esophageal and Gastric Cancer Risk: A Nested Case-Control Study, Photochem. Photobiol., 2018, 94, 797–806 CrossRef PubMed.
-
J. Makin, K. Shaw and T. Winzenberg, Targeted programs for skin cancer prevention: An evidence check rapid review brokered by the Sax Institute (www.saxinstitute.org.au) for the Cancer Institute NSW, 2018 Search PubMed.
- D. M. Holman, H. Ding, Z. Berkowitz, A. M. Hartman and F. M. Perna, Sunburn prevalence among US adults, National Health Interview Survey 2005, 2010, and 2015, J. Am. Acad. Dermatol., 2019, 80, 817–820 CrossRef PubMed.
-
Cancer Australia, National Cancer Control Indicators: Sunburn and sun protection, 2019, [cited 27 August 2019] Search PubMed.
- B. Koster, M. Meyer, T. Andersson, G. Engholm and P. Dalum, Development in sunburn 2007–2015 and skin cancer projections 2007–2040 of campaign results in the Danish population, Medicine, 2018, 97, e12738 CrossRef PubMed.
- J. Narbutt, P. A. Philipsen, G. I. Harrison, K. A. Morgan, K. P. Lawrence, K. A. Baczynska, K. Grys, M. Rogowski-Tylman, I. Olejniczak-Staruch, A. Tewari, M. Bell, C. O'Connor, H. C. Wulf, A. Lesiak and A. R. Young, Sunscreen applied at ≥2 mg cm−2 during a sunny holiday prevents erythema, a biomarker of ultraviolet radiation-induced DNA damage and suppression of acquired immunity, Br. J. Dermatol., 2019, 180, 604–614 CrossRef CAS PubMed.
- A. R. Young, J. Narbutt, G. I. Harrison, K. P. Lawrence, M. Bell, C. O'Connor, P. Olsen, K. Grys, K. A. Baczynska, M. Rogowski-Tylman, H. C. Wulf, A. Lesiak and P. A. Philipsen, Optimal sunscreen use, during a sun holiday with a very high ultraviolet index, allows vitamin D synthesis without sunburn, Br. J. Dermatol., 2019, 181, 1052–1062 CrossRef CAS PubMed.
- R. E. Neale, S. R. Khan, R. M. Lucas, M. Waterhouse, D. C. Whiteman and C. M. Olsen, The effect of sunscreen on vitamin D: a review, Br. J. Dermatol., 2019, 181, 907–915 CrossRef CAS PubMed.
- M. K. Matta, R. Zusterzeel, N. R. Pilli, V. Patel, D. A. Volpe, J. Florian, L. Oh, E. Bashaw, I. Zineh, C. Sanabria, S. Kemp, A. Godfrey, S. Adah, S. Coelho, J. Wang, L. A. Furlong, C. Ganley, T. Michele and D. G. Strauss, Effect of sunscreen application under maximal use conditions on plasma concentration of sunscreen active ingredients: a randomized clinical trial, JAMA, J. Am. Med. Assoc., 2019, 321, 2082–2091 CrossRef CAS PubMed.
- J. Hiller, K. Klotz, S. Meyer, W. Uter, K. Hof, A. Greiner, T. Goen and H. Drexler, Systemic availability of lipophilic organic UV filters through dermal sunscreen exposure, Environ. Int., 2019, 132, 105068 CrossRef CAS PubMed.
- J. Hiller, K. Klotz, S. Meyer, W. Uter, K. Hof, A. Greiner, T. Goen and H. Drexler, Toxicokinetics of urinary 2-ethylhexyl salicylate and its metabolite 2-ethyl-hydroxyhexyl salicylate in humans after simulating real-life dermal sunscreen exposure, Arch. Toxicol., 2019, 93, 2565–2574 CrossRef CAS PubMed.
- A. R. Lindstrom, L. A. von Schuckmann, M. C. B. Hughes, G. M. Williams, A. C. Green and J. C. van der Pols, Regular sunscreen use and risk of mortality: long-term follow-up of a skin cancer prevention trial, Am. J. Prev. Med., 2019, 56, 742–746 CrossRef PubMed.
- D. C. Whiteman, R. E. Neale, J. Aitken, L. Gordon, A. C. Green, M. Janda, C. M. Olsen, H. P. Soyer and Sunscreen Summit Policy G, When to apply sunscreen: a consensus statement for Australia and New Zealand, Aust. N. Z. J. Public Health, 2019, 43, 171–175 CrossRef PubMed.
- R. L. McKenzie and R. M. Lucas, Reassessing impacts of extended daily exposure to low level solar UV radiation, Sci. Rep., 2018, 8, 13805 CrossRef PubMed.
- R. M. Lucas, R. E. Neale, S. Madronich and R. L. McKenzie, Are current guidelines for sun protection optimal for health? Exploring the evidence, Photochem. Photobiol. Sci., 2018, 17, 1956–1963 RSC.
- M. Lehmann, A. B. Pfahlberg, H. Sandmann, W. Uter and O. Gefeller, Messages associated with low UV Index values need reconsideration, Int. J. Environ. Res. Public Health, 2019, 16, 2067 CrossRef PubMed.
- M. Lehmann, H. Sandmann, A. B. Pfahlberg, W. Uter and O. Gefeller, Erythemal UV Radiation on Days with Low UV Index Values - an Analysis of Data from the German Solar UV Monitoring Network over a Ten-year Period, Photochem. Photobiol., 2019, 95, 1076–1082 CAS.
- C. L. Ballaré, M. M. Caldwell, S. D. Flint, S. A. Robinson and J. F. Bornman, Effects of solar ultraviolet radiation on terrestrial ecosystems. Patterns, mechanisms, and interactions with climate change, Photochem. Photobiol. Sci., 2011, 10, 226–241 RSC.
- P. W. Barnes, C. E. Williamson, R. M. Lucas, S. A. Robinson, S. Madronich, N. D. Paul, J. F. Bornman, A. F. Bais, B. Sulzberger, S. R. Wilson, A. L. Andrady, R. L. McKenzie, P. J. Neale, A. T. Austin, G. H. Bernhard, K. R. Solomon, R. E. Neale, P. J. Young, M. Norval, L. E. Rhodes, S. Hylander, K. C. Rose, J. Longstreth, P. J. Aucamp, C. L. Ballaré, R. M. Cory, S. D. Flint, F. R. de Gruijl, D.-P. Häder, A. M. Heikkilä, M. A. K. Jansen, K. K. Pandey, T. M. Robson, C. A. Sinclair, S.-Å. Wängberg, R. C. Worrest, S. Yazar, A. R. Young and R. G. Zepp, Ozone depletion, ultraviolet radiation, climate change and prospects for a sustainable future, Nat. Sustain., 2019, 2, 569–579 CrossRef.
- C. E. Williamson, R. G. Zepp, R. M. Lucas, S. Madronich, A. T. Austin, C. L. Ballaré, M. Norval, B. Sulzberger, A. F. Bais, R. L. McKenzie, S. A. Robinson, D.-P. Häder, N. D. Paul and J. F. Bornman, Solar ultraviolet radiation in a changing climate, Nat. Clim. Change, 2014, 4, 434–441 CrossRef.
- K. J. Feeley, J. T. Stroud and T. M. Perez, Most ‘global' reviews of species’ responses to climate change are not truly global, Diversity Distrib., 2017, 23, 231–234 CrossRef.
- J. L. Rolando, C. Turin, D. A. Ramírez, V. Mares, J. Monerris and R. Quiroz, Key ecosystem services and ecological intensification of agriculture in the tropical high-Andean Puna as affected by land-use and climate changes, Agric., Ecosyst. Environ., 2017, 236, 221–233 CrossRef.
- K. S. Sheldon, Climate change in the tropics: ecological and evolutionary responses at low latitudes, Annu. Rev. Ecol. Syst., 2019, 50, 303–333 CrossRef.
- J. F. Bornman, P. W. Barnes, T. M. Robson, S. A. Robinson, M. A. K. Jansen, C. L. Ballaré and S. D. Flint, Linkages between stratospheric ozone, UV radiation and climate change and their implications for terrestrial ecosystems, Photochem. Photobiol. Sci., 2019, 18, 681–716 RSC.
- D. K. Gibson-Reinemer, K. S. Sheldon and F. J. Rahel, Climate change creates rapid species turnover in montane communities, Ecol. Evol., 2015, 5, 2340–2347 CrossRef PubMed.
- C. A. Deutsch, J. J. Tewksbury, R. B. Huey, K. S. Sheldon, C. K. Ghalambor, D. C. Haak and P. R. Martin, Impacts of climate warming on terrestrial ectotherms across latitude, Proc. Natl. Acad. Sci. U. S. A., 2008, 105, 6668–6672 CrossRef CAS PubMed.
- J. Knuesting, M. C. Brinkmann, B. Silva, M. Schorsch, J. Bendix, E. Beck and R. Scheibe, Who will win where and why? An ecophysiological dissection of the competition between a tropical pasture grass and the invasive weed Bracken over an elevation range of 1000 m in the tropical Andes, PLoS One, 2018, 13, e0202255 CrossRef PubMed.
-
WMO, Scientific Assessment of Ozone Depletion: 2018, Global Ozone Research and Monitoring Project-Report No. 58, Geneva, Switzerland, 2018, p. 88 Search PubMed.
- G. H. Krause, P. Jahns, A. Virgo, M. Garcia, J. Aranda, E. Wellmann and K. Winter, Photoprotection, photosynthesis and growth of tropical tree seedlings under
near-ambient and strongly reduced solar ultraviolet-B radiation, J. Plant Physiol., 2007, 164, 1311–1322 CrossRef CAS PubMed.
- S. Kataria and K. N. Guruprasad, Exclusion of solar UV radiation improves photosynthetic performance and yield of wheat varieties, Plant Physiol. Biochem., 2015, 97, 400–411 CrossRef CAS PubMed.
- S. Kataria and L. Baghel, Influence of UV exclusion and selenium on carbon fixation, nitrogen fixation and yield of soybean variety JS-335, S. Afr. J. Bot., 2016, 103, 126–134 CrossRef CAS.
- C. A. Mazza, P. I. Gimenez, A. G. Kantolic and C. L. Ballaré, Beneficial effects of solar UV-B radiation on soybean yield mediated by reduced insect herbivory under field conditions, Physiol. Plant., 2013, 147, 307–315 CrossRef CAS PubMed.
- G. T. Pecl, M. B. Araújo, J. D. Bell, J. Blanchard, T. C. Bonebrake, I.-C. Chen, T. D. Clark, R. K. Colwell, F. Danielsen, B. Evengård, L. Falconi, S. Ferrier, S. Frusher, R. A. Garcia, R. B. Griffis, A. J. Hobday, C. Janion-Scheepers, M. A. Jarzyna, S. Jennings, J. Lenoir, H. I. Linnetved, V. Y. Martin, P. C. McCormack, J. McDonald, N. J. Mitchell, T. Mustonen, J. M. Pandolfi, N. Pettorelli, E. Popova, S. A. Robinson, B. R. Scheffers, J. D. Shaw, C. J. B. Sorte, J. M. Strugnell, J. M. Sunday, M.-N. Tuanmu, A. Vergés, C. Villanueva, T. Wernberg, E. Wapstra and S. E. Williams, Biodiversity redistribution under climate change: Impacts on ecosystems and human well-being, Science, 2017, 355, eaai9214 CrossRef PubMed.
- A. Wolf, N. B. Zimmerman, W. R. L. Anderegg, P. E. Busby and J. Christensen, Altitudinal shifts of the native and introduced flora of California in the context of 20th-century warming, Global Ecol. Biogeogr., 2016, 25, 418–429 CrossRef.
- M. Dainese, S. Aikio, P. E. Hulme, A. Bertolli, F. Prosser and L. Marini, Human disturbance and upward expansion of plants in a warming climate, Nat. Clim. Change, 2017, 7, 577–580 CrossRef.
- A. Mosena, T. Steinlein and W. Beyschlag, Reconstructing the historical spread of non-native plants in the North American West from herbarium specimens, Flora, 2018, 242, 45–52 CrossRef.
- H. Wang, X. C. Ma, L. Zhang, E. Siemann and J. W. Zou, UV-B has larger negative impacts on invasive populations of Triadica sebifera but ozone impacts do not vary, J. Plant Ecol., 2016, 9, 61–68 Search PubMed.
- T. Václavík, M. Beckmann, A. F. Cord and A. M. Bindewald, Effects of UV-B radiation on leaf hair traits of invasive plants—Combining historical herbarium records with novel remote sensing data, PLoS One, 2017, 12, e0175671 CrossRef PubMed.
- P. W. Barnes, R. J. Ryel and S. D. Flint, UV screening in native and non-native plant species in the tropical alpine: Implications for climate change-driven migration of species to higher elevations, Front. Plant Sci., 2017, 8, 12–21 Search PubMed.
- M. Hock, R. W. Hofmann, C. Müller and A. Erfmeier, Exotic plant species are locally adapted but not to high ultraviolet-B radiation: a reciprocal multispecies experiment, Ecology, 2019, 100, e02665 CrossRef PubMed.
- L. Y. Watermann, M. Hock, C. Blake and A. Erfmeier, Plant invasion into high elevations implies adaptation to high UV-B environments: a multi-species experiment, Biol. Invasions, 2020, 22, 1203–1218 CrossRef.
- S. Tomiolo and D. Ward, Species migrations and range shifts: A synthesis of causes and consequences, Perspect. Plant Ecol. Evol. Syst., 2018, 33, 62–77 CrossRef.
- M. Wink, F. Botschen, C. Gosmann, H. Schäfer and P. G. Waterman, Chemotaxonomy
seen from a phylogenetic perspective and evolution of secondary metabolism, Annu. Plant Rev., 2018, 364–433 Search PubMed.
- A. Castagna, K. Csepregi, S. Neugart, G. Zipoli, K. Večeřová, G. Jakab, T. Jug, L. Llorens, J. Martínez-Abaigar, J. Martínez-Lüscher, E. Núñez-Olivera, A. Ranieri, K. Schoedl-Hummel, M. Schreiner, P. Teszlák, S. Tittmann, O. Urban, D. Verdaguer, M. A. K. Jansen and É. Hideg, Environmental plasticity of Pinot noir grapevine leaves; a trans-European study of morphological and biochemical changes along a 1500 km latitudinal climatic gradient, Plant, Cell Environ., 2017, 40, 2790–2805 CrossRef CAS PubMed.
- E. A. Tripp, Y. Zhuang, M. Schreiber, H. Stone and A. E. Berardi, Evolutionary and ecological drivers of plant flavonoids across a large latitudinal gradient, Mol. Phylogenet. Evol., 2018, 128, 147–161 CrossRef CAS PubMed.
- L. Pellissier, X. Moreira, H. Danner, M. Serrano, N. Salamin, N. M. van Dam and S. Rasmann, The simultaneous inducibility of phytochemicals related to plant direct and indirect defences against herbivores is stronger at low elevation, J. Ecol., 2016, 104, 1116–1125 CrossRef CAS.
- A. Kergunteuil, P. Descombes, G. Glauser, L. Pellissier and S. Rasmann, Plant physical and chemical defence variation along elevation gradients: a functional trait-based approach, Oecologia, 2018, 187, 561–571 CrossRef PubMed.
- X. Moreira, W. K. Petry, K. A. Mooney, S. Rasmann and L. Abdala-Roberts, Elevational gradients in plant defences and insect herbivory: recent advances in the field and prospects for future research, Ecography, 2018, 41, 1485–1496 CrossRef.
- M. J. Waterman, A. S. Nugraha, R. Hendra, G. E. Ball, S. A. Robinson and P. A. Keller, Antarctic moss biflavonoids show high antioxidant and ultraviolet-screening activity, J. Nat. Prod., 2017, 80, 2224–2231 CrossRef CAS PubMed.
- D. P. Fraser, A. Sharma, T. Fletcher, S. Budge, C. Moncrieff, A. N. Dodd and K. A. Franklin, UV-B antagonises shade avoidance and increases levels of the flavonoid quercetin in coriander (Coriandrum sativum), Sci. Rep., 2017, 7, 17758 CrossRef PubMed.
- C. L. Ballaré and A. T. Austin, Recalculating growth and defense strategies under competition: Key roles of photoreceptors and jasmonates, J. Exp. Bot., 2019, 70, 3425–3434 CrossRef PubMed.
- G. J. Sproull, M. F. Quigley, A. Sher and E. Gonzalez, Long-term changes in composition, diversity and distribution patterns in four herbaceous plant communities along an elevational gradient, J. Veg. Sci., 2015, 26, 552–563 CrossRef.
- J. Savage and M. Vellend, Elevational shifts, biotic homogenization and time lags in vegetation change during 40 years of climate warming, Ecography, 2015, 38, 546–555 CrossRef.
- Q.-W. Wang, S. Nagano, H. Ozaki, S.-I. Morinaga, J. Hidema and K. Hikosaka, Functional differentiation in UV-B-induced DNA damage and growth inhibition between highland and lowland ecotypes of two Arabidopsis species, Environ. Exp. Bot., 2016, 131, 110–119 CrossRef CAS.
- K. Skarbø and K. VanderMolen, Maize migration: key crop expands to higher altitudes under climate change in the Andes, Clim. Dev., 2016, 8, 245–255 CrossRef.
- V. N. Ibañez, F. J. Berli, R. W. Masuelli, R. A. Bottini and C. F. Marfil, Influence of altitude and enhanced ultraviolet-B radiation on tuber production, seed viability, leaf pigments and morphology in the wild potato species Solanum kurtzianum Bitter & Wittm collected from an elevational gradient, Plant Sci., 2017, 261, 60–68 CrossRef PubMed.
- A. D. Derebe, A. Gobena Roro, B. Tessfaye Asfaw, W. Worku Ayele and A. K. Hvoslef-Eide, Effects of solar UV-B radiation exclusion on physiology, growth and yields of taro (Colocasia esculenta (L.)) at different altitudes in tropical environments of Southern Ethiopia, Sci. Hortic., 2019, 256, 108563 CrossRef.
- S. Bokhorst, S. H. Pedersen, L. Brucker, O. Anisimov, J. W. Bjerke, R. D. Brown, D. Ehrich, R. L. Essery, A. Heilig, S. Ingvander, C. Johansson, M. Johansson, I. S. Jonsdottir, N. Inga, K. Luojus, G. Macelloni, H. Mariash, D. McLennan, G. N. Rosqvist, A. Sato, H. Savela, M. Schneebeli, A. Sokolov, S. A. Sokratov, S. Terzago, D. Vikhamar-Schuler, S. Williamson, Y. Qiu and T. V. Callaghan, Changing Arctic snow cover: A review of recent developments and assessment of future needs for observations, modelling, and impacts, Ambio, 2016, 45, 516–537 CrossRef PubMed.
- G. J. McCabe and D. M. Wolock, Long-term variability in Northern Hemisphere snow cover and associations with warmer winters, Clim. Change, 2010, 99, 141–153 CrossRef.
- J. C. Hammond, F. A. Saavedra and S. K. Kampf, Global snow zone maps and trends in snow persistence 2001–2016, Int. J. Climatol., 2018, 38, 4369–4383 CrossRef.
- C. W. Thackeray, C. Derksen, C. G. Fletcher and A. Hall, Snow and Climate: Feedbacks, Drivers, and Indices of Change, Curr. Clim. Change Rep., 2019, 5, 322–333 CrossRef.
- P. Niittynen, R. K. Heikkinen and M. Luoto, Snow cover is a neglected driver of Arctic biodiversity loss, Nat. Clim. Change, 2018, 8, 997–1001 CrossRef.
- G. K. Phoenix and J. W. Bjerke, Arctic browning: extreme events and trends reversing arctic greening, Global Change Biol., 2016, 22, 2960–2962 CrossRef PubMed.
- R. Treharne, J. W. Bjerke, H. Tømmervik, L. Stendardi and G. K. Phoenix, Arctic browning: Impacts of extreme climatic events on heathland ecosystem CO2 fluxes, Global Change Biol., 2019, 25, 489–503 CrossRef PubMed.
- S. A. Robinson, D. H. King, J. Bramley-Alves, M. J. Waterman, M. B. Ashcroft, J. Wasley, J. D. Turnbull, R. E. Miller, E. Ryan-Colton, T. Benny, K. Mullany, L. J. Clarke, L. A. Barry and Q. Hua, Rapid change in East Antarctic terrestrial vegetation in response to regional drying, Nat. Clim. Change, 2018, 8, 879–884 CrossRef CAS.
- A. Coffey and M. A. K. Jansen, Effects of natural solar UV-B radiation on three Arabidopsis accessions are strongly affected by seasonal weather conditions, Plant Physiol. Biochem., 2019, 134, 64–72 CrossRef CAS PubMed.
- F. Pescheck and W. Bilger, Compensation of lack of UV screening by cellular tolerance in green macroalgae (Ulvophyceae) from the upper eulittoral, Mar. Biol., 2018, 165, 132 CrossRef.
- T. Solanki, P. J. Aphalo, S. Neimane, S. M. Hartikainen, M. Pieristè, A. Shapiguzov, A. Porcar-Castell, J. Atherton, A. Heikkilä and T. M. Robson, UV-screening and springtime recovery of photosynthetic capacity in leaves of Vaccinium vitis-idaea above and below the snow pack, Plant Physiol. Biochem., 2019, 134, 40–52 CrossRef CAS PubMed.
- T. M. Robson and P. J. Aphalo, Transmission of ultraviolet, visible and near-infrared solar radiation to plants within a seasonal snow pack, Photochem. Photobiol. Sci., 2019, 18, 1963–1971 RSC.
-
S. Ahmed, T. Griffin, S. B. Cash, W.-Y. Han, C. Matyas, C. Long, C. M. Orians, J. R. Stepp, A. Robbat and D. Xue, Global Climate Change, Ecological Stress, and Tea Production, in Stress Physiology of Tea in the Face of Climate Change, Springer, 2018, pp. 1–23 Search PubMed.
- M. E. Dusenge, A. G. Duarte and D. A. Way, Plant carbon metabolism and climate change: elevated CO2 and temperature impacts on photosynthesis, photorespiration and respiration, New Phytol., 2019, 221, 32–49 CrossRef CAS PubMed.
- P. B. Reich, S. E. Hobbie and T. D. Lee, Plant growth enhancement by elevated CO2 eliminated by joint water and nitrogen limitation, Nat. Geosci., 2014, 7, 920 CrossRef CAS.
- O. Urban, M. Hrstka, P. Holub, B. Veselá, K. Večeřová, K. Novotná, J. Grace and K. Klem, Interactive effects of ultraviolet radiation and elevated CO2 concentration on photosynthetic characteristics of European beech saplings during the vegetation season, Plant Physiol. Biochem., 2019, 134, 20–30 CrossRef CAS PubMed.
- M. A. K. Jansen, W. Bilger, É. Hideg, Å. Strid, P. Aphalo, C. Brelsford, K. Klem, A. Mátai, L. Llorens, J. Nezval, L. Nybakken, L. Ryan, A. Sharma, D. Schenke, K. A. Solhaug, V. Spunda, D. Verdaguer, Y. Yan and O. Urban, Interactive effects of UV-B radiation in a complex environment, Plant Physiol. Biochem., 2019, 134 DOI:10.1016/j.plaphy.2018.10.021.
- T. M. Robson, S. M. Hartikainen and P. J. Aphalo, How does solar ultraviolet-B radiation improve drought tolerance of silver birch (Betula pendula Roth.) seedlings?, Plant, Cell Environ., 2015, 38, 953–967 CrossRef CAS PubMed.
- C. L. Ballaré, Light regulation of plant defense, Annu. Rev. Plant Biol., 2014, 65, 335–363 CrossRef PubMed.
- J. F. Bornman, P. W. Barnes, S. A. Robinson, C. L. Ballaré, S. D. Flint and M. M. Caldwell, Solar ultraviolet radiation and ozone depletion-driven climate change: effects on terrestrial ecosystems, Photochem. Photobiol. Sci., 2015, 14, 88–107 RSC.
- R. Escobar-Bravo, C. Nederpel, S. Naranjo, H. K. Kim, M. J. Rodríguez-López, G. Chen, G. Glauser, K. A. Leiss and P. G. L. Klinkhamer, Ultraviolet radiation modulates both constitutive and inducible plant defenses against thrips but is dose and plant genotype dependent, J. Pest Sci., 2019 DOI:10.1007/s10340-019-01166-w.
- T. M. Robson, P. J. Aphalo, A. K. Banas, P. W. Barnes, C. C. Brelsford, G. I. Jenkins, T. Kotilainen, J. Labuz, J. Martínez-Abaigar, L. O. Morales, S. Neugart, M. Pieristè, N. Rai, F. Vandenbussche and M. Jansen, A perspective on ecologically relevant plant-UV research and its practical application, Photochem. Photobiol. Sci., 2019, 18, 970–988 RSC.
- C. N. Merfield, L. Winder, S. A. Stilwell, R. W. Hofmann, J. R. Bennett, J. J. Wargent and S. Hodge, Mesh crop covers improve potato yield and inhibit tomato potato psyllid and blight: The roles of mesh pore size and ultraviolet radiation, Ann. Appl. Biol., 2019, 174, 223–237 CrossRef.
- M.Á Del-Castillo-Alonso, L. Monforte, R. Tomás-Las-Heras, J. Martínez-Abaigar and E. Núñez-Olivera, Phenolic characteristics acquired by berry skins of Vitis vinifera cv. Tempranillo in response to close-to-ambient solar ultraviolet radiation are mostly reflected in the resulting wines, J. Sci. Food Agric., 2020, 100, 401, DOI:10.1002/jsfa.10068.
- S. Neugart and M. Schreiner, UVB and UVA as eustressors in horticultural and agricultural crops, Sci. Hortic., 2018, 234, 370–381 CrossRef CAS.
- A. El-Aal HA, A. M. Rizk and I. E. Mousa, Evaluation of new greenhouse covers with modified light regime to control cotton aphid and cucumber (Cucumis sativus L.) productivity, Crop Prot., 2018, 107, 64–70 CrossRef.
- C. F. Assumpção, V. S. Hermes, C. Pagno, A. Castagna, A. Mannucci, C. Sgherri, C. Pinzino, A. Ranieri, S. H. Flôres and R. AdO, Phenolic enrichment in apple skin following post-harvest fruit UV-B treatment, Postharvest Biol. Technol., 2018, 138, 37–45 CrossRef.
- K. C. Rose, P. J. Neale, M. Tzortziou, C. L. Gallegos and T. E. Jordan, Patterns of spectral, spatial, and long-term variability in light attenuation in an optically complex sub-estuary, Limnol. Oceanogr., 2019, 64, S257–S272 CrossRef CAS.
- M. Lindholm, M. Eie, D. O. Hessen, J. T. Johansen, K. Weiby and J. Thaulow, Effects of water browning on freshwater biodiversity: the case of the predatory phantom midge Chaoborus nyblaei, Hydrobiologia, 2018, 813, 33–40 CrossRef CAS.
-
P. J. Neale and R. L. Smyth, Are Warmer Waters, Brighter Waters? An Examination of the Irradiance Environment of Lakes and Oceans in a Changing Climate, in Aquatic Ecosystems in a Changing Climate, ed. D.-P. Häder and K. Gao, CRC, 2018, pp. 89–115 Search PubMed.
-
IPCC, Summary for Policymakers, in IPCC Special Report on the Ocean and Cryosphere in a Changing Climate, ed. H.-O. Pörtner, et al., https://www.ipcc.ch/site/assets/uploads/sites/3/2019/11/03_SROCC_SPM_FINAL.pdf Search PubMed.
- C. E. Williamson, P. J. Neale, S. Hylander, K. C. Rose, F. L. Figueroa, S. A. Robinson, D.-P. Häder, S.-Å. Wängberg and R. C. Worrest, The interactive effects of stratospheric ozone depletion, UV radiation, and climate change on aquatic ecosystems, Photochem. Photobiol. Sci., 2019, 18, 717–746 RSC.
- C. E. Williamson, E. P. Overholt, J. A. Brentrup, R. M. Pilla, T. H. Leach, S. G. Schladow, J. D. Warren, S. S. Urmy, S. Sadro, S. Chandra and P. J. Neale, Sentinel responses to droughts, wildfires, and floods: Ultraviolet radiation and the consequences for lakes and their ecosystem services, Front. Ecol. Environ., 2016, 14, 102–109 CrossRef.
- C. E. Williamson, S. Madronich, A. Lal, R. G. Zepp, R. M. Lucas, E. P. Overholt, K. C. Rose, S. G. Schladow and J. Lee-Taylor, Climate change-induced increases in precipitation are reducing the potential for solar ultraviolet radiation to inactivate pathogens in surface waters, Sci. Rep., 2017, 7, 13033 CrossRef PubMed.
- A. J. Tucker, C. E. Williamson, K. C. Rose, J. T. Oris, S. J. Connelly, M. H. Olson and D. L. Mitchell, Ultraviolet radiation affects invasibility of lake ecosystems by warm-water fish, Ecology, 2010, 91, 882–890 CrossRef PubMed.
- A. J. Tucker and C. E. Williamson, The invasion window for warmwater fish in clearwater lakes: the role of ultraviolet radiation and temperature, Divers. Distrib., 2014, 20, 181–192 CrossRef.
- A. J. Tucker, C. E. Williamson and J. T. Oris, Development and application of a UV attainment threshold for the prevention of warmwater aquatic invasive species, Biol. Invasions, 2012, 14, 2331–2342 CrossRef.
- L. Caputo, P. Huovinen, R. Sommaruga and I. Gomez, Water transparency affects the survival of the medusa stage of the invasive freshwater jellyfish Craspedacusta sowerbii, Hydrobiologia, 2018, 817, 179–191 CrossRef CAS.
- L. G. A. Barboza, A. D. Vethaak, B. R. Lavorante, A.-K. Lundebye and L. Guilhermino, Marine microplastic debris: An emerging issue for food security, food safety and human health, Mar. Pollut. Bull., 2018, 133, 336–348 CrossRef CAS PubMed.
- C. M. Rochman, C. Brookson, J. Bikker, N. Djuric, A. Earn, K. Bucci, S. Athey, A. Huntington, H. McIlwraith, K. Munno, H. De Frond, A. Kolomijeca, L. Erdle, J. Grbic, M. Bayoumi, S. B. Borrelle, T. Wu, S. Santoro, L. M. Werbowski, X. Zhu, R. K. Giles, B. M. Hamilton, C. Thaysen, A. Kaura, N. Klasios, L. Ead, J. Kim, C. Sherlock, A. Ho and C. Hung, Rethinking microplastics as a
diverse contaminant suite, Environ. Toxicol. Chem., 2019, 38, 703–711 CrossRef CAS PubMed.
- E. E. Burns and A. B. A. Boxall, Microplastics in the aquatic environment: Evidence for or against adverse impacts and major knowledge gaps, Environ. Toxicol. Chem., 2018, 37, 2776–2796 CrossRef CAS PubMed.
- M. R. Hossain, M. Jiang, Q. Wei and L. G. Leff, Microplastic surface properties affect bacterial colonization in freshwater, J. Basic Microbiol., 2019, 59, 54 CrossRef CAS PubMed.
-
P. Tsiota, K. Karkanorachaki, E. SyranidouM. Franchini and N. Kalogerakis, Microbial degradation of HDPE secondary microplastics: Preliminary Results, paper presented at Proceedings of the International Conference on Microplastic Pollution in the Mediterranean Sea, Springer International Publishing, Cham, 2018 Search PubMed.
- C. J. Foley, Z. S. Feiner, T. D. Malinich and T. O. Höök, A meta-analysis of the effects of exposure to microplastics on fish and aquatic invertebrates, Sci. Total Environ., 2018, 631–632, 550–559 CrossRef CAS PubMed.
- T. Gouin, R. A. Becker, A. G. Collot, J. W. Davis, B. Howard, K. Inawaka, M. Lampi, B. S. Ramon, J. Shi and P. W. Hopp, Toward the Development and Application of an Environmental Risk Assessment Framework for Microplastic, Environ. Toxicol. Chem., 2019, 38, 2087–2100 CrossRef CAS PubMed.
- Q. Wang, Y. Zhang, X. Wangjin, Y. Wang, G. Meng and Y. Chen, The adsorption behavior of metals in aqueous solution by microplastics effected by UV radiation, J. Environ. Sci., 2020, 87, 272–280 CrossRef PubMed.
- L. M. Hernandez, E. G. Xu, H. C. E. Larsson, R. Tahara, V. B. Maisuria and N. Tufenkji, Plastic teabags release billions of microparticles and nanoparticles into tea, Environ. Sci. Technol., 2019, 53, 12300–12310 CrossRef CAS PubMed.
- T. D. Malinich, N. Chou, M. S. Sepúlveda and T. O. Höök, No evidence of microplastic impacts on consumption or growth of larval Pimephales promelas, Environ. Toxicol. Chem., 2018, 37, 2912–2918 CrossRef CAS PubMed.
- C. Schür, S. Rist, A. Baun, P. Mayer, N. B. Hartmann and M. Wagner, When fluorescence is not a particle: the tissue translocation of microplastics in Daphnia magna seems an artifact, Environ. Toxicol. Chem., 2019, 38, 1495–1503 CrossRef PubMed.
- S. Seidensticker, C. Zarfl, O. A. Cirpka and P. Grathwohl, Microplastic–Contaminant Interactions: Influence of nonlinearity and coupled mass transfer, Environ. Toxicol. Chem., 2019, 38, 1635–1644 CAS.
- S. Ziajahromi, A. Kumar, P. A. Neale and F. D. L. Leusch, Effects of polyethylene microplastics on the acute toxicity of a synthetic pyrethroid to midge larvae (Chironomus tepperi) in synthetic and river water, Sci. Total Environ., 2019, 671, 971–975 CrossRef CAS.
- S. L. Schneider and H. W. Lim, Review of environmental effects of oxybenzone and other sunscreen active ingredients, J. Am. Acad. Dermatol., 2019, 80, 266–271 CrossRef CAS PubMed.
- C. L. Mitchelmore, K. He, M. Gonsior, E. Hain, A. Heyes, C. Clark, R. Younger, P. Schmitt-Kopplin, A. Feerick, A. Conway and L. Blaney, Occurrence and distribution of UV-filters and other anthropogenic contaminants in coastal surface water, sediment, and coral tissue from Hawaii, Sci. Total Environ., 2019, 670, 398–410 CrossRef CAS PubMed.
- J.-P. Fel, C. Lacherez, A. Bensetra, S. Mezzache, E. Béraud, M. Léonard, D. Allemand and C. Ferrier-Pagès, Photochemical response of the scleractinian coral Stylophora pistillata to some sunscreen ingredients, Coral Reefs, 2019, 38, 109–122 CrossRef.
- C. Corinaldesi, F. Marcellini, E. Nepote, E. Damiani and R. Danovaro, Impact of inorganic UV filters contained in sunscreen products on tropical stony corals (Acropora spp.), Sci. Total Environ., 2018, 637–638, 1279–1285 CrossRef CAS PubMed.
- C. D. Knightes, R. B. Ambrose Jr., B. Avant, Y. Han, B. Acrey, D. C. Bouchard, R. Zepp and T. Wool, Modeling framework for simulating concentrations of solute chemicals, nanoparticles, and solids in surface waters and sediments: WASP8 Advanced Toxicant Module, Environ. Modell. Softw., 2019, 111, 444–458 CrossRef PubMed.
- K. L. Nelson, A. B. Boehm, R. J. Davies-Colley, M. C. Dodd, T. Kohn, K. G. Linden, Y. Liu, P. A. Maraccini, K. McNeill, W. A. Mitch, T. H. Nguyen, K. M. Parker, R. A. Rodriguez, L. M. Sassoubre, A. I. Silverman, K. R. Wigginton and R. G. Zepp, Sunlight-mediated inactivation of health-relevant microorganisms in water: A review of mechanisms and modeling approaches, Environ. Sci.: Processes Impacts, 2018, 20, 1089–1122 RSC.
- D. Vione and A. Scozzaro, Photochemistry of surface fresh waters in the framework of climate change, Environ. Sci. Technol., 2019, 53, 7945–7963 CrossRef CAS PubMed.
- B. Avant, D. Bouchard, X. Chang, H.-S. Hsieh, B. Acrey, Y. Han, J. Spear, R. Zepp and C. D. Knightes, Environmental fate of multiwalled carbon nanotubes and graphene oxide across different aquatic ecosystems, NanoImpact, 2019, 13, 1–12 CrossRef PubMed.
- Y. Han, C. D. Knightes, D. Bouchard, R. Zepp, B. Avant, H.-S. Hsieh, X. Chang, B. Acrey, W. M. Henderson and J. Spear, Simulating graphene oxide nanomaterial phototransformation and transport in surface water, Environ. Sci.: Nano, 2019, 6, 180–194 RSC.
- A. I. Silverman, N. Tay and N. Machairas, Comparison of biological weighting functions used to model endogenous sunlight inactivation rates of MS2 coliphage, Water Res., 2019, 151, 439–446 CrossRef CAS PubMed.
- J. Wenk, M. T. Nguyen and K. L. Nelson, Natural photosensitizers in constructed unit process wetlands: Photochemical characterization and inactivation of pathogen indicator organisms, Environ. Sci. Technol., 2019, 53, 7724–7735 CrossRef CAS PubMed.
- R. G. Zepp, M. Cyterski, K. Wong, O. Georgacopoulos, B. Acrey, G. Whelan, R. Parmar and M. Molina, Biological weighting functions for evaluating the role of sunlight-induced inactivation of coliphages at selected beaches and nearby tributaries, Environ. Sci. Technol., 2018, 52, 13068–13076 CrossRef CAS PubMed.
- C. A. Gueymard, The SMARTS spectral irradiance model after 25 years: New developments and validation of reference spectra, Sol. Energy, 2019, 187, 233–253 CrossRef.
- G. X. De Hoe, M. T. Zumstein, G. J. Getzinger, I. Rüegsegger, H.-P. E. Kohler, M. A. Maurer-Jones, M. Sander, M. A. Hillmyer and K. McNeill, Photochemical transformation of poly(butylene adipate-co-terephthalate) and its effects on enzymatic hydrolyzability, Environ. Sci. Technol., 2019, 53, 2472–2481 CrossRef CAS PubMed.
- C. Chen, L. Chen, Y. Yao, F. Artigas, Q. Huang and W. Zhang, Organotin release from polyvinyl chloride microplastics and concurrent photodegradation in water: Impacts from salinity, dissolved organic matter, and light exposure, Environ. Sci. Technol., 2019, 53, 10741–10752 CrossRef CAS PubMed.
- A. Khaled, A. Rivaton, C. Richard, F. Jaber and M. Sleiman, Phototransformation of plastic containing brominated flame retardants: Enhanced fragmentation and release of photoproducts to water and air, Environ. Sci. Technol., 2018, 52, 11123–11131 CrossRef CAS PubMed.
- L. Zhu, S. Zhao, T. B. Bittar, A. Stubbins and D. Li, Photochemical dissolution of buoyantmicroplastics to dissolved organic carbon: Rates and microbial impacts, J. Hazard. Mater., 2020, 383, 121065 CrossRef CAS PubMed.
- T. Du, A. S. Adeleye, T. Zhang, C. Jiang, M. Zhang, H. Wang, Y. Li, A. A. Keller and W. Chen, Influence of light wavelength on the photoactivity, physicochemical transformation, and fate of graphene oxide in aqueous media, Environ. Sci.: Nano, 2018, 5, 2590–2603 RSC.
- Y. Gao, X. Ren, X. Zhang and C. Chen, Environmental fate and risk of ultraviolet- and visible-light-transformed graphene oxide: A comparative study, Environ. Pollut., 2019, 251, 821–829 CrossRef CAS PubMed.
- W.-C. Hou, P.-L. Lee, Y.-C. Chou and Y.-S. Wang, Antibacterial property of graphene oxide: the role of phototransformation, Environ. Sci.: Nano, 2017, 4, 647–657 RSC.
- D. Hanigan, L. Truong, J. Schoepf, T. Nosaka, A. Mulchandani, R. L. Tanguay and P. Westerhoff, Trade-offs in ecosystem impacts from nanomaterial versus organic chemical ultraviolet filters in sunscreens, Water Res., 2018, 139, 281–290 CrossRef CAS PubMed.
- X. Zhang, J. Li, W.-Y. Fan, M.-C. Yao, L. Yuan and G.-P. Sheng, Enhanced photodegradation of extracellular antibiotic resistance genes by dissolved organic matter photosensitization, Environ. Sci. Technol., 2019, 53, 10732–10740 CrossRef CAS PubMed.
- A. Singh, W.-C. Hou, T.-F. Lin and R. G. Zepp, Roles of silver–chloride complexations in sunlight-driven formation of silver nanoparticles, Environ. Sci. Technol., 2019, 53, 11162–11169 CrossRef CAS PubMed.
- J. Dean, Y. van der Velde, M. H. Garnett, K. J. Dinsmore, R. Baxter, J. S. Lessels, P. Smith, L. E. Street, J. Subke and D. Tetzlaff, Abundant pre-industrial carbon detected in Canadian Arctic headwaters: implications for the permafrost carbon feedback, Environ. Res. Lett., 2018, 13, 034024 CrossRef.
- E. A. G. Schuur, A. D. McGuire, C. Schädel, G. Grosse, J. W. Harden, D. J. Hayes, G. Hugelius, C. D. Koven, P. Kuhry and D. M. Lawrence, Climate change and the permafrost carbon feedback, Nature, 2015, 520, 171 CrossRef CAS PubMed.
-
IPCC, Special Report on the Ocean and Cryosphere in a Changing Climate. Summary for Policymakers, 2019 Search PubMed.
- M. R. Turetsky, B. W. Abbott, M. C. Jones, K. W. Anthony, D. Olefeldt, E. A. G. Schuur, C. D. Koven, A. D. McGuire, G. Grosse and P. Kuhry, Permafrost collapse is accelerating carbon release, Nature, 2019, 569, 32–34 CrossRef CAS PubMed.
- J. F. Dean, M. H. Garnett, E. Spyrakos and M. F. Billett, The potential hidden age of dissolved organic carbon exported by peatland streams, J. Geophys. Res.: Biogeosci., 2019, 124, 328–341 CrossRef CAS.
- J. C. Foereid, E. A. Zarov, I. M. Latysh, I. V. Filippov and E. D. Lapshina, Photo-exposure affects subsequent peat litter decomposition, Geoderma, 2018, 315, 104–110 CrossRef.
- J. C. Bowen, L. A. Kaplan and R. M. Cory, Photodegradation disproportionately impacts biodegradation of semi-labile DOM in streams, Limnol. Oceanogr., 2020, 65, 13–26 CrossRef CAS.
- A. Li, A. F. Aubeneau, T. King, R. M. Cory, B. T. Neilson, D. Bolster and A. I. Packman, Effects of vertical hydrodynamic mixing on photomineralization of dissolved organic carbon in arctic surface waters, Environ. Sci.: Processes Impacts, 2019, 21, 748–760 RSC.
- M. Almagro, J. Martínez-López, F. T. Maestre and A. Rey, The contribution of photodegradation to litter decomposition in semiarid Mediterranean grasslands depends on its interaction with local humidity conditions, litter quality and position, Ecosystems, 2017, 20, 527–542 CrossRef CAS.
- T. A. Day, M. S. Bliss, A. R. Tomes, C. T. Ruhland and R. Guénon, Desert leaf litter decay: Coupling of microbial respiration, water-soluble fractions and photodegradation, Global Change Biol., 2018, 24, 5454–5470 CrossRef PubMed.
- E. H. Esch, J. Y. King and E. E. Cleland, Foliar litter chemistry mediates susceptibility to UV degradation in two dominant species from a semi-arid ecosystem, Plant Soil, 2019, 440, 265–276 CrossRef CAS.
- K. I. Predick, S. R. Archer, S. M. Aguillon, D. A. Keller, H. L. Throop and P. W. Barnes, UV-B radiation and shrub canopy effects on surface litter decomposition in a shrub-invaded dry grassland, J. Arid Environ., 2018, 157, 13–21 CrossRef.
- T. A. Day, M. S. Bliss, S. K. Placek, A. R. Tomes and R. Guénon, Thermal abiotic emission of CO2 and CH4 from leaf litter and its significance in a photodegradation assessment, Ecosphere, 2019, 10, e02745 CrossRef.
- A. T. Austin, M. S. Méndez and C. L. Ballaré, Photodegradation alleviates the lignin bottleneck for carbon turnover in terrestrial ecosystems, Proc. Natl. Acad. Sci. U. S. A., 2016, 113, 4392–4397 CrossRef CAS PubMed.
-
C. L. Ballaré and A. T. Austin, UV-B Radiation and Plant Life: Molecular Biology to Ecology, in UV radiation and terrestrial ecosystems: emerging perspectives, ed. B. Jordan, CABI, Wallingford, 2017, pp. 23–38 Search PubMed.
- M. S. Méndez, M. L. Martínez, P. I. Araujo and A. T. Austin, Solar radiation exposure accelerates decomposition and biotic activity in surface litter but not soil in a semiarid woodland ecosystem in Patagonia, Argentina, Plant Soil, 2019, 445, 483–496 CrossRef.
- D. B. Hewins, H. Lee, P. W. Barnes, N. G. McDowell, W. T. Pockman, T. Rahn and H. L. Throop, Early exposure to UV radiation overshadowed by precipitation and litter quality as drivers of decomposition in the northern Chihuahuan Desert, PLoS One, 2019, 14, e0210470 CrossRef CAS PubMed.
- M. Pieristè, M. Chauvat, T. K. Kotilainen, A. G. Jones, M. Aubert, T. M. Robson and E. Forey, Solar UV-A radiation and blue light enhance tree leaf litter decomposition in a temperate forest, Oecologia, 2019, 191, 191–203 CrossRef PubMed.
- C. Wu, H. Wang, Q. Mo, Z. Zhang, G. Huang, F. Kong, Y. Liu and H. Wang, Effects of elevated UV-B radiation and N deposition on the decomposition of coarse woody debris, Sci. Total Environ., 2019, 663, 170–176 CrossRef CAS PubMed.
- J. T. Abatzoglou, A. P. Williams, L. Boschetti, M. Zubkova and C. A. Kolden, Global patterns of interannual climate–fire relationships, Global Change Biol., 2018, 24, 5164–5175 CrossRef PubMed.
- M. O. Andreae, Emission of trace gases and aerosols from biomass burning - an updated assessment. (Report), Atmos. Chem. Phys., 2019, 19, 8523 CrossRef CAS.
- S. J. Hart, J. Henkelman, P. D. McLoughlin, S. E. Nielsen, A. Truchon-Savard and J. F. Johnstone, Examining forest resilience to changing fire frequency in a fire-prone region of boreal forest, Global Change Biol., 2019, 25, 869–884 CrossRef PubMed.
- M. W. Jones, C. Santín, G. R. van der Werf and S. H. Doerr, Global fire emissions buffered by the production of pyrogenic carbon, Nat. Geosci., 2019, 12, 742–747 CrossRef CAS.
- C. Rumpel, Soils linked to climate change, Nature, 2019, 572, 442–443 CrossRef CAS PubMed.
- X. J. Walker, J. L. Baltzer, S. G. Cumming, N. J. Day, C. Ebert, S. Goetz, J. F. Johnstone, S. Potter, B. M. Rogers and E. A. G. Schuur, Increasing wildfires threaten historic carbon sink of boreal forest soils, Nature, 2019, 572, 520–523 CrossRef CAS PubMed.
- A. P. Williams and J. T. Abatzoglou, Recent advances and remaininguncertainties in resolving past and future climate effects on global fire activity, Curr. Clim. Change Rep., 2016, 2, 1–14 CrossRef.
- S. Wagner, R. Jaffé and A. Stubbins, Dissolved black carbon in aquatic ecosystems, Limnol. Oceanogr.
Lett., 2018, 3, 168–185 CrossRef CAS.
- A. Stubbins, J. Niggemann and T. Dittmar, Photo-lability of deep ocean dissolved black carbon, Biogeosciences, 2012, 9, 1661–1670 CrossRef CAS.
- S. R. Wilson, S. Madronich, J. D. Longstreth and K. R. Solomon, Interactive effects of changing stratospheric ozone and climate on tropospheric composition and air quality, and the consequences for human and ecosystem health, Photochem. Photobiol. Sci., 2019, 18, 775–803 RSC.
- X. Liu, X. Lyu, Y. Wang, F. Jiang and H. Guo, Intercomparison of O3 formation and radical chemistry in the past decade at a suburban site in Hong Kong, Atmos. Chem. Phys., 2019, 19, 5127–5145 CrossRef CAS.
- M. T. Pay, G. Gangoiti, M. Guevara, S. Napelenok, X. Querol, O. Jorba and C. Pérez García-Pando, Ozone source apportionment during peak summer events over southwestern Europe, Atmos. Chem. Phys., 2019, 19, 5467–5494 CrossRef CAS.
- S. Haase and K. Matthes, The importance of interactive chemistry for stratosphere–troposphere coupling, Atmos. Chem. Phys., 2019, 19, 3417–3432 CrossRef CAS.
- R. S. Williams, M. I. Hegglin, B. J. Kerridge, P. Jöckel, B. G. Latter and D. A. Plummer, Characterising the seasonal and geographical variability in tropospheric ozone, stratospheric influence and recent changes, Atmos. Chem. Phys., 2019, 19, 3589–3620 CrossRef CAS.
- S. Meul, U. Langematz, P. Kröger, S. Oberländer-Hayn and P. Jöckel, Future changes in the stratosphere-to-troposphere ozone mass flux and the contribution from climate change and ozone recovery, Atmos. Chem. Phys., 2018, 18, 7721–7738 CrossRef CAS.
- A. Anger, O. Dessens, F. Xi, T. Barker and R. Wu, China's air pollution reduction efforts may result in an increase in surface ozone levels in highly polluted areas, Ambio, 2016, 45, 254–265 CrossRef CAS PubMed.
- K. Li, D. J. Jacob, H. Liao, L. Shen, Q. Zhang and K. H. Bates, Anthropogenic drivers of 2013–2017 trends in summer surface ozone in China, Proc. Natl. Acad. Sci. U. S. A., 2019, 116, 422 CrossRef CAS PubMed.
- S. Lou, H. Liao and B. Zhu, Impacts of aerosols on surface-layer ozone concentrations in China through heterogeneous reactions and changes in photolysis rates, Atmos. Environ., 2014, 85, 123–138 CrossRef CAS.
- X. X. Tie, S. Madronich, S. Walters, D. P. Edwards, P. Ginoux, N. Mahowald, R. Y. Zhang, C. Lou and G. Brasseur, Assessment of the global impact of aerosols on tropospheric oxidants, J. Geophys. Res., 2005, 110, D03204 CrossRef.
- J. Wang, D. J. Allen, K. E. Pickering, Z. Li and H. He, Impact of aerosol direct effect on East Asian air quality during the EAST-AIRE campaign, J. Geophys. Res.: Atmos., 2016, 121, 6534–6554 CAS.
- J. Xing, J. Wang, R. Mathur, S. Wang, G. Sarwar, J. Pleim, C. Hogrefe, Y. Zhang, J. Jiang, D. C. Wong and J. Hao, Impacts of aerosol direct effects on tropospheric ozone through changes in atmospheric dynamics and photolysis rates, Atmos. Chem. Phys., 2017, 17, 9869–9883 CrossRef CAS PubMed.
- H. Liao and J. H. Seinfeld, Global impacts of gas-phase chemistry-aerosol interactions on direct radiative forcing by anthropogenic aerosols and ozone, J. Geophys. Res.: Atmos., 2005, 110, D18208 CrossRef.
- L. Pozzoli, I. Bey, S. Rast, M. G. Schultz, P. Stier and J. Feichter, Trace gas and aerosol interactions in the fully coupled model of aerosol-chemistry-climate ECHAM5-HAMMOZ:1. Model description and insights from the spring 2001 TRACE-P experiment, J. Geophys. Res.: Atmos., 2008, 113, D07308 Search PubMed.
- M. Hollaway, O. Wild, T. Yang, Y. Sun, W. Xu, C. Xie, L. Whalley, E. Slater, D. Heard and D. Liu, Photochemical impacts of haze pollution in an urban environment, Atmos. Chem. Phys., 2019, 19, 9699–9714 CrossRef CAS.
- H. Chen, B. Zhuang, J. Liu, T. Wang, S. Li, M. Xie, M. Li, P. Chen and M. Zhao, Characteristics of ozone and particles in the near-surface atmosphere in the urban area of the Yangtze River Delta, China, Atmos. Chem. Phys., 2019, 19, 4153–4175 CrossRef CAS.
- X. Lu, L. Zhang, Y. Chen, M. Zhou, B. Zheng, K. Li, Y. Liu, J. Lin, T.-M. Fu and Q. Zhang, Exploring 2016–2017 surface ozone pollution over China: source contributions and meteorological influences, Atmos. Chem. Phys., 2019, 19, 8339–8361 CrossRef CAS.
- J. M. Nicely, T. P. Canty, M. Manyin, L. D. Oman, R. J. Salawitch, S. D. Steenrod, S. E. Strahan and S. A. Strode, Changes in global tropospheric OH expected as a result of climate change over the last several decades, J. Geophys. Res.: Atmos., 2018, 123, 10774–10795 Search PubMed.
- S. A. Montzka, M. Krol, E. Dlugokencky, B. Hall, P. Jöckel and J. Lelieveld, Small interannual variability of global atmospheric hydroxyl, Science, 2011, 331, 67–69 CrossRef CAS PubMed.
- A. J. Turner, C. Frankenberg and E. A. Kort, Interpreting contemporary trends in atmospheric methane, Proc. Natl. Acad. Sci. U. S. A., 2019, 116, 2805–2813 CrossRef CAS PubMed.
- Y. Zhang, D. J. Jacob, J. D. Maasakkers, M. P. Sulprizio, J. X. Sheng, R. Gautam and J. Worden, Monitoring global tropospheric OH concentrations using satellite observations of atmospheric methane, Atmos. Chem. Phys., 2018, 18, 15959–15973 CrossRef CAS.
- S. Naus, S. A. Montzka, S. Pandey, S. Basu, E. J. Dlugokencky and M. Krol, Constraints and biases in a tropospheric two-box model of OH, Atmos. Chem. Phys., 2019, 19, 407–424 CrossRef CAS.
- J. McNorton, C. Wilson, M. Gloor, R. J. Parker, H. Boesch, W. Feng, R. Hossaini and M. P. Chipperfield, Attribution of recent increases in atmospheric methane through 3-D inverse modelling, Atmos. Chem. Phys., 2018, 18, 18149–18168 CrossRef CAS.
- M. Su, Y. Lin, X. Fan, L. Peng and C. Zhao, Impacts of global emissions of CO, NOx, and CH4 on China tropospheric hydroxyl free radicals, Adv. Atmos. Sci., 2012, 29, 838–854 CrossRef CAS.
- A. F. Bais, R. M. Lucas, J. F. Bornman, C. E. Williamson, B. Sulzberger, A. T. Austin, S. R. Wilson, A. L. Andrady, G. Bernhard, R. L. McKenzie, P. J. Aucamp, S. Madronich, R. E. Neale, S. Yazar, A. R. Young, F. R. de Gruijl, M. Norval, Y. Takizawa, P. W. Barnes, T. M. Robson, S. A. Robinson, C. L. Ballaré, S. D. Flint, P. J. Neale, S. Hylander, K. C. Rose, S.Å Wängberg, D. P. Häder, R. C. Worrest, R. G. Zepp, N. D. Paul, R. M. Cory, K. R. Solomon, J. Longstreth, K. K. Pandey, H. H. Redhwi, A. Torikai and A.M. Heikkilä, Environmental effects of ozone depletion, UV radiation and interactions with climate change: UNEP Environmental Effects Assessment Panel, update 2017, Photochem. Photobiol. Sci., 2018, 17, 127–179 RSC.
- P. M. Crill and B. F. Thornton, Whither
methane in the IPCC process?, Nat. Clim. Change, 2017, 7, 678–680 CrossRef.
- E. G. Nisbet, M. R. Manning, E. J. Dlugokencky, R. E. Fisher, D. Lowry, S. E. Michel, C. L. Myhre, S. M. Platt, G. Allen, P. Bousquet, R. Brownlow, M. Cain, J. L. France, O. Hermansen, R. Hossaini, A. E. Jones, I. Levin, A. C. Manning, G. Myhre, J. A. Pyle, B. H. Vaughn, N. J. Warwick and J. W. C. White, Very strong atmospheric methane growth in the 4 years 2014–2017: implications for the Paris Agreement, Global Biogeochem. Cycles, 2019, 33, 318–342 CrossRef CAS.
-
G. Myhre, D. Shindell, F.-M. Bréon, W. Collins, J. Fuglestvedt, J. Huang, D. Koch, J.-F. Lamarque, D. Lee, B. Mendoza, T. Nakajima, A. Robock, G. Stephens, H. Zhang, B. Aamaas, O. Boucher, S. B. Dalsøren, J. S. Daniel, P. Forster, C. Granier, J. Haigh, Ø Hodnebrog, J. O. Kaplan, G. Marston, C. J. Nielsen, B. C. O'Neill, G. P. Peters, J. Pongratz, V. Ramaswamy, R. Roth, L. Rotstayn, S. J. Smith, D. Stevenson, J.-P. Vernier, O. Wild, P. Young, D. Jacob, A. R. Ravishankara and K. Shine, Anthropogenic and Natural Radiative Forcing, in Climate Change 2013: The Physical Science Basis. Contribution of Working Group I to the Fifth Assessment Report of the Intergovernmental Panel on Climate Change, ed. T. F. Stocker, et al., Cambridge University Pressm Cambridge, United Kingdom and New York, NY, USA, 2013, p. 82 Search PubMed.
- O. Bazhenov, Increased humidity in the stratosphere as a possible factor of ozone destruction in the Arctic during the spring 2011 using Aura MLS observations, Int. J. Remote Sens., 2019, 40, 3448–3460 CrossRef.
- D. Griffin, K. A. Walker, I. Wohltmann, S. S. Dhomse, M. Rex, M. P. Chipperfield, W. Feng, G. L. Manney, J. Liu and D. Tarasick, Stratospheric ozone loss in the Arctic winters between 2005 and 2013 derived with ACE-FTS measurements, Atmos. Chem. Phys., 2019, 19, 577–601 CrossRef CAS.
- J.-P. Pommereau, F. Goutail, A. Pazmino, F. Lefèvre, M. P. Chipperfield, W. Feng, M. Van Roozendael, N. Jepsen, G. Hansen, R. Kivi, K. Bognar, K. Strong, K. Walker, A. Kuzmichev, S. Khattatov and V. Sitnikova, Recent Arctic ozone depletion: Is there an impact of climate change?, C. R. Geosci., 2018, 350, 347–353 CrossRef.
- C. Voigt, A. Dörnbrack, M. Wirth, S. M. Groß, M. C. Pitts, L. R. Poole, R. Baumann, B. Ehard, B.-M. Sinnhuber, W. Woiwode and H. Oelhaf, Widespread polar stratospheric ice clouds in the 2015–2016 Arctic winter – implications for ice nucleation, Atmos. Chem. Phys., 2018, 18, 15623–15641 CrossRef CAS.
- F. Frank, P. Jöckel, S. Gromov and M. Dameris, Investigating the yield of H2O and H2 from methane oxidation in the stratosphere, Atmos. Chem. Phys., 2018, 18, 9955–9973 CrossRef CAS.
- J. F. Dean, J. J. Middelburg, T. Röckmann, R. Aerts, L. G. Blauw, M. Egger, M. S. M. Jetten, A. E. E. de Jong, O. H. Meisel, O. Rasigraf, C. P. Slomp, M. H. in't Zandt and A. J. Dolman, Methane feedbacks to the global climate system in a warmer world, Rev. Geophys., 2018, 56, 207–250 CrossRef.
- K. W. Anthony, T. Deimling, I. Nitze, S. Frolking, A. Emond, R. Daanen, P. Anthony, P. Lindgren, B. Jones and G. Grosse, 21st-century modeled permafrost carbon emissions accelerated by abrupt thaw beneath lakes, Nat. Commun., 2018, 9, 3262 CrossRef PubMed.
- C. Voigt, M. E. Marushchak, M. Mastepanov, R. E. Lamprecht, T. R. Christensen, M. Dorodnikov, M. Jackowicz-Korczyński, A. Lindgren, A. Lohila, H. Nykänen, M. Oinonen, T. Oksanen, V. Palonen, C. C. Treat, P. J. Martikainen and C. Biasi, Ecosystem carbon response of an Arctic peatland to simulated permafrost thaw, Global Change Biol., 2019, 25, 1746–1764 CrossRef PubMed.
- G. Lamarche-Gagnon, J. L. Wadham, B. Sherwood Lollar, S. Arndt, P. Fietzek, A. D. Beaton, A. J. Tedstone, J. Telling, E. A. Bagshaw, J. R. Hawkings, T. J. Kohler, J. D. Zarsky, M. C. Mowlem, A. M. Anesio and M. Stibal, Greenland melt drives continuous export of methane from the ice-sheet bed, Nature, 2019, 565, 73–77 CrossRef CAS PubMed.
- B. Sulzberger, A. T. Austin, R. M. Cory, R. G. Zepp and N. D. Paul, Solar UV radiation in a changing world: roles of cryosphere–land–water–atmosphere interfaces in global biogeochemical cycles, Photochem. Photobiol. Sci., 2019, 18, 747–774 RSC.
- R. B. Neumann, C. J. Moorberg, J. D. Lundquist, J. C. Turner, M. P. Waldrop, J. W. McFarland, E. S. Euskirchen, C. W. Edgar and M. R. Turetsky, Warming effects of spring rainfall increase methane emissions from thawing permafrost, Geophys. Res. Lett., 2019, 46, 1393–1401 CrossRef.
- C. M. Singleton, C. K. McCalley, B. J. Woodcroft, J. A. Boyd, P. N. Evans, S. B. Hodgkins, J. P. Chanton, S. Frolking, P. M. Crill, S. R. Saleska, V. I. Rich and G. W. Tyson, Methanotrophy across a natural permafrost thaw environment, ISME J., 2018, 12, 2544 CrossRef CAS PubMed.
- J. Lelieveld, S. Gromov, A. Pozzer and D. Taraborrelli, Global tropospheric hydroxyl distribution, budget and reactivity, Atmos. Chem. Phys., 2016, 16, 12477–12493 CrossRef CAS.
- B. Bukosa, N. M. Deutscher, J. A. Fisher, D. Kubistin, C. Paton-Walsh and D. W. T. Griffith, Simultaneous shipborne measurements of CO2, CH4 and CO and their application to improving greenhouse-gas flux estimates in Australia, Atmos. Chem. Phys., 2019, 19, 7055–7072 CrossRef CAS.
- L. Conte, S. Szopa, R. Séférian and L. Bopp, The oceanic cycle of carbon monoxide and its emissions to the atmosphere, Biogeosciences, 2019, 16, 881–902 CrossRef CAS.
- L. Si and P. A. Ariya, Recent advances in atmospheric chemistry of mercury, Atmosphere, 2018, 9, 76 CrossRef.
- S. Falk and B.-M. Sinnhuber, Polar boundary layer bromine explosion and ozone depletion events in the chemistry–climate model EMAC v2.52: implementation and evaluation of AirSnow algorithm, Geosci. Model Dev., 2018, 11, 1115–1131 CrossRef CAS.
- Y. Luo, F. Si, H. Zhou, K. Dou, Y. Liu and W. Liu, Observations and source investigations of the boundary layer bromine monoxide (BrO) inthe Ny-Ålesund Arctic, Atmos. Chem. Phys., 2018, 18, 9789–9801 CrossRef CAS.
- S. Wang, S. M. McNamara, C. W. Moore, D. Obrist, A. Steffen, P. B. Shepson, R. M. Staebler, A. R. W. Raso and K. A. Pratt, Direct detection of atmospheric atomic bromine leading to mercury and ozone depletion, Proc. Natl. Acad. Sci. U. S. A., 2019, 116, 14479 CrossRef CAS PubMed.
- J. W. Halfacre, P. B. Shepson and K. A. Pratt, pH-dependent production of molecular chlorine, bromine, and iodine from frozen saline surfaces, Atmos. Chem. Phys., 2019, 19, 4917–4931 CrossRef CAS.
- A. Fiehn, B. Quack, I. Stemmler, F. Ziska and K. Krüger, Importance of seasonally resolved oceanic emissions for bromoform delivery from the tropical Indian Ocean and west Pacific to the stratosphere, Atmos. Chem. Phys., 2018, 18, 11973–11990 CrossRef CAS.
- D. Lee, S.-K. Min, E. Fischer, H. Shiogama, I. Bethke, L. Lierhammer and J. F. Scinocca, Impacts of half a degree additional warming on the Asian summer monsoon rainfall characteristics, Environ. Res. Lett., 2018, 13, 044033 CrossRef.
- K. Abrahamsson, A. Granfors, M. Ahnoff, C. A. Cuevas and A. Saiz-Lopez, Organic bromine compounds produced in sea ice in Antarctic winter, Nat. Commun., 2018, 9, 5291 CrossRef CAS PubMed.
- S. D. Archer, K. M. Posman, J. DeStefano, A. O. Harrison, A. Ladina, E. A. Cheff and D. P. Witt, Detection of bromoperoxidase activity in microalgae and planktonic microbial communities using aminophenyl fluorescein, Front. Mar. Sci., 2019, 6, 68 CrossRef.
- Y. Liu, D. C. O. Thornton, T. S. Bianchi, W. A. Arnold, M. R. Shields, J. Chen and S. A. Yvon-Lewis, Dissolved organic matter composition drives the marine production of brominated very short-lived substances, Environ. Sci. Technol., 2015, 49, 3366–3374 CrossRef CAS PubMed.
- R. Butler, P. I. Palmer, L. Feng, S. J. Andrews, E. L. Atlas, L. J. Carpenter, V. Donets, N. R. P. Harris, S. A. Montzka, L. L. Pan, R. J. Salawitch and S. M. Schauffler, Quantifying the vertical transport of CHBr3 and CH2Br2 over the western Pacific, Atmos. Chem. Phys., 2018, 18, 13135–13153 CrossRef CAS.
- T. Punitha, S.-M. Phang, J. C. Juan and J. Beardall, Environmental Control of Vanadium Haloperoxidases and Halocarbon Emissions in Macroalgae, Mar. Biotechnol., 2018, 20, 282–303 CrossRef CAS PubMed.
- K. R. Solomon, G. J. Velders, S. R. Wilson, S. Madronich, J. Longstreth, P. J. Aucamp and J. F. Bornman, Sources, fates, toxicity, and risks of trifluoroacetic acid and its salts: Relevance to substances regulated under the Montreal and Kyoto Protocols, J. Toxicol. Environ. Health, Part B, 2016, 19, 289–304 CAS.
- M. Ateia, A. Maroli, N. Tharayil and T. Karanfil, The overlooked short- and ultrashort-chain poly- and perfluorinated substances: A review, Chemosphere, 2019, 220, 866–882 CrossRef CAS.
- C. Jn, J. Guo, Z. Zhai and J. Zhang, The contribution of fluoropolymer thermolysis to trifluoroacetic acid (TFA) in environmental media, Chemosphere, 2019, 222, 637–644 CrossRef PubMed.
- J. Kazil, S. McKeen, S.-W. Kim, R. Ahmadov, G. A. Grell, R. K. Talukdar and A. R. Ravishankara, Deposition and rainwater concentrations of trifluoroacetic acid in the United States from the use of HFO-1234yf, J. Geophys. Res.: Atmos., 2014, 119, 14059–14079 CAS.
- Z. Wang, Y. Wang, J. Li, S. Henne, B. Zhang, J. Hu and J. Zhang, Impacts of the Degradation of 2,3,3,3-tetrafluoropropene into trifluoroacetic acid from its application in automobile air conditioners in China, the United States, and Europe, Environ. Sci. Technol., 2018, 52, 2819–2826 CrossRef CAS PubMed.
- M. Scheurer, K. Nödler, F. Freeling, J. Janda, O. Happel, M. Riegel, U. Müller, F. R. Storck, M. Fleig, F. T. Lange, A. Brunsch and H.-J. Brauch, Small, mobile, persistent: Trifluoroacetate in the water cycle – Overlooked sources, pathways, and consequences for drinking water supply, Water Res., 2017, 126, 460–471 CrossRef CAS PubMed.
- L. Zhang, H. Sun, Q. Wang, H. Chen, Y. Yao, Z. Zhao and A. C. Alder, Uptake mechanisms of perfluoroalkyl acids with different carbon chain lengths (C2-C8) by wheat (Triticum acstivnm L.), Sci. Total Environ., 2019, 654, 19–27 CrossRef CAS PubMed.
- A. G. Costa, A. U. de Carvalho, M. M. Melo and B. Soto-Blanco, Sequential administrations of trifluoroacetate induce tolerance to Palicourea maccgravii, a monofluoroacetate-containing plant, in calves, Toxicon, 2019, 160, 8–11 CrossRef CAS PubMed.
- K. Solomon, G. Velders, S. Wilson, S. Madronich, J. Longstreth, P. Aucamp and J. F. Bornman, Sources, fates, toxicity, and risks of trifluoroacetic acid and its salts: Relevance to substances regulated under the Montreal and Kyoto protocols, J. Toxicol. Environ. Health, Part B, 2016, 19, 289–304 CAS.
- I. T. Cousins, C. A. Ng, Z. Wang and M. Scheringer, Why is high persistence alone a major cause of concern?, Environ. Sci.: Processes Impacts, 2019, 21, 781–792 RSC.
- A. J. Cohen, M. Brauer, R. Burnett, H. R. Anderson, J. Frostad, K. Estep, K. Balakrishnan, B. Brunekreef, L. Dandona, R. Dandona, V. Feigin, G. Freedman, B. Hubbell, A. Jobling, H. Kan, L. Knibbs, Y. Liu, R. Martin, L. Morawska, C. A. Pope III, H. Shin, K. Straif, G. Shaddick, M. Thomas, R. van Dingenen, A. van Donkelaar, T. Vos, C. J. L. Murray and M. H. Forouzanfar, Estimates and 25-year trends of the global burden of disease attributable to ambient air pollution: an analysis of data from the Global Burden of Diseases Study 2015, Lancet, 2017, 389, 1907–1918 CrossRef.
- X. Li, L. Jin and H. Kan, Air pollution: a global problem needs local fixes, Nature, 2019, 570, 437 CrossRef CAS PubMed.
- G. McFiggans, T. F. Mentel, J. Wildt, I. Pullinen, S. Kang, E. Kleist, S. Schmitt, M. Springer, R. Tillmann, C. Wu, D. Zhao, M. Hallquist, C. Faxon, M. Le Breton, Å.M Hallquist, D. Simpson, R. Bergström, M. E. Jenkin, M. Ehn, J. A. Thornton, M. R. Alfarra, T. J. Bannan, C. J. Percival, M. Priestley, D. Topping and A. Kiendler-Scharr, Secondary organic aerosol reduced by mixture of atmospheric vapours, Nature, 2019, 565, 587–593 CrossRef CAS PubMed.
- M. Shrivastava, M. O. Andreae, P. Artaxo, H. M. J. Barbosa, L. K. Berg, J. Brito, J. Ching, R. C. Easter, J. Fan, J. D. Fast, Z. Feng, J. D. Fuentes, M. Glasius, A. H. Goldstein, E. G. Alves, H. Gomes, D. Gu, A. Guenther, S. H. Jathar, S. Kim, Y. Liu, S. Lou, S. T. Martin, V. F. McNeill, A. Medeiros, S. S. de Sá, J. E. Shilling, S. R. Springston, R. A. F. Souza, J. A. Thornton, G. Isaacman-VanWertz, L. D. Yee, R. Ynoue, R. A. Zaveri, A. Zelenyuk and C. Zhao, Urban pollution greatly enhances formation of natural aerosols over the Amazon rainforest, Nat. Commun., 2019, 10, 1046 CrossRef PubMed.
- Z. Meng, D. Dabdub and J. H. Seinfeld, Chemical coupling between atmospheric ozone and particulate matter, Science, 1997, 277, 116 CrossRef CAS.
- J. Xing, R. Mathur, J. Pleim, C. Hogrefe, C.-M. Gan, D. C. Wong, C. Wei and J. Wang, Air pollution and climate response to aerosol direct radiative effects: A modeling study of decadal trends across the northern hemisphere, J. Geophys. Res.: Atmos., 2015, 120, 12221–12236 Search PubMed.
- W. Song, Y.-L. Wang, W. Yang, X.-C. Sun, Y.-D. Tong, X.-M. Wang, C.-Q. Liu, Z.-P. Bai and X.-Y. Liu, Isotopic evaluation on relative contributions of major NOx sources to nitrate of PM2.5 in Beijing, Environ. Pollut., 2019, 248, 183–190 CrossRef CAS PubMed.
- C. Han, A. Zhao, E. Varughese and E. Sahle-Demessie, Evaluating weathering of food packaging polyethylene-nano-clay composites: Release of nanoparticles and their impacts, NanoImpact, 2018, 9, 61–71 CrossRef PubMed.
-
K. N. Fotopoulou and H. K. Karapanagioti, Degradation of various plastics in the environment, in Hazardous Chemicals Associated with Plastics in the Marine Environment, Springer, 2017, pp. 71–92 Search PubMed.
- B. Forsthuber and G. Grüll, Prediction of wood surface discoloration for applications in the field of architecture, Wood Sci. Technol., 2018, 52, 1093–1111 CrossRef CAS.
- R. Liu, H. Zhu, K. Li and Z. Yang, Comparison on the aging of woods exposed to natural sunlight and artificial xenon light, Polymers, 2019, 11, 709 CrossRef CAS PubMed.
- M. Mattonai, A. Watanabe, A. Shiono and E. Ribechini, Degradation of wood by UV light: A study by EGA-MS and Py-GC/MS with on line irradiation system, J. Anal. Appl. Pyrolysis, 2019, 139, 224–232 CrossRef CAS.
- T. H. de Almeida, D. H. de Almeida, E. Chahud, L. A. M. N. Branco, R. V. Pinheiro, A. L. Christoforo and F. A. R. Lahr, Mechanical performance of wood under artificial and natural weathering treatments, BioResources, 2019, 14, 6267–6277 Search PubMed.
- J. Buchner, M. Irle, C. Belloncle, F. Michaud and N. Macchioni, Fungal and bacterial colonies growing on weathered wood surfaces, Wood Mater. Sci. Eng., 2019, 14, 33–41 CrossRef CAS.
- S. A. Awad, C. M. Fellows and S. S. Mahini, Effects of accelerated weathering on the chemical, mechanical, thermal and morphological properties of an epoxy/multi-walled carbon nanotube composite, Polym. Test., 2018, 66, 70–77 CrossRef CAS.
- C. Han, E. Sahle-Demessie, E. Varughese and H. Shi, Polypropylene–MWCNT composite degradation, and release, detection and toxicity of MWCNTs during accelerated environmental aging, Environ. Sci.: Nano, 2019, 6, 1876–1894 RSC.
- W. Wohlleben, C. Kingston, J. Carter, E. Sahle-Demessie, S. Vázquez-Campos, B. Acrey, C.-Y. Chen, E. Walton, H. Egenolf, P. Müller and R. Zepp, NanoRelease: Pilot interlaboratory comparison of a weathering protocol applied to resilient and labile polymers with and without embedded carbon nanotubes, Carbon, 2017, 113, 346–360 CrossRef CAS PubMed.
- A. Gupta, A. Kumar, K. Sharma and R. Gupta, Application of high conductive nanoparticles to enhance the thermal and mechanical properties of wood composite, Mater. Today: Proc., 2018, 5, 3143–3149 CAS.
- M. L. Weththimuni, D. Capsoni, M. Malagodi and M. Licchelli, Improving Wood Resistance to Decay by Nanostructured ZnO-Based Treatments, J. Nanomater., 2019, 6715756 CAS.
- E. Oberhofnerová, M. Pánek, M. Podlena, M. Pavelek and I. Štěrbová, Color stabilization of Siberian and European larch wood using UVA, HALS, and nanoparticle pretreatments, Forests, 2019, 10, 23 CrossRef.
- L. Wallenhorst, L. Gurău, A. Gellerich, H. Militz, G. Ohms and W. Viöl, UV-blocking properties of Zn/ZnO coatings on wood deposited by cold plasma spraying at atmospheric pressure, Appl. Surf. Sci., 2018, 434, 1183–1192 CrossRef CAS.
- H. Shen, S. Zhang, J. Cao, J. Jiang and W. Wang, Improving anti-weathering performance of thermally modified wood by TiO2 sol or/and paraffin emulsion, Constr. Build. Mater., 2018, 169, 372–378 CrossRef CAS.
- L. Préaubert, V. Tassistro, M. Auffan, I. Sari-Minodier, J. Rose, B. Courbiere and J. Perrin, Very low concentration of cerium dioxide nanoparticles induce DNA damage, but no loss of vitality, in human spermatozoa, Toxicol. in Vitro, 2018, 50, 236–241 CrossRef PubMed.
- A. Mackevica, M. E. Olsson, P. D. Mines, L. R. Heggelund and S. F. Hansen, Dermal transfer quantification of nanoparticles from nano-enabled surfaces, NanoImpact, 2018, 11, 109–118 CrossRef.
- J. G. Clar, W. E. Platten III, E. J. Baumann Jr., A. Remsen, S. M. Harmon, C. L. Bennett-Stamper, T. A. Thomas and T. P. Luxton, Dermal transfer and environmental release of CeO2 nanoparticles used as UV inhibitors on outdoor surfaces: Implications for human and environmental health, Sci. Total Environ., 2018, 613–614, 714–723 CrossRef CAS PubMed.
- H. Nagasawa, J. Xu, M. Kanezashi and T. Tsuru, Atmospheric-pressure plasma-enhanced chemical vapor deposition of UV-shielding TiO2 coatings on transparent plastics, Mater. Lett., 2018, 228, 479–481 CrossRef CAS.
- J. Xu, H. Nagasawa, M. Kanezashi and T. Tsuru, UV-protective TiO2 thin films with high transparency in visible light region fabricated via atmospheric-pressure plasma-enhanced chemical vapor deposition, ACS Appl. Mater. Interfaces, 2018, 10, 42657–42665 CrossRef CAS PubMed.
- Y. Shi, X. Zhuang, L. Cao, S. Gou, Y. Xiong, W.-F. Lai, Z. Wang and A. L. Rogach, Copper-nanocluster-based transparent ultraviolet-shielding polymer films, ChemNanoMat, 2019, 5, 110–115 CrossRef CAS.
- Z. Zhao, A. Mao, W. Gao and H. Bai, A facile in situ method to fabricate transparent, flexible polyvinyl alcohol/ZnO film for UV-shielding, Compos. Commun., 2018, 10, 157–162 CrossRef.
- M. Wang, M. Zhang, L. Pang, C. Yang, Y. Zhang, J. Hu and G. Wu, Fabrication of highly durable polysiloxane-zinc oxide (ZnO) coated polyethylene terephthalate (PET) fabric with improved ultraviolet resistance, hydrophobicity, and thermal resistance, J. Colloid Interface Sci., 2019, 537, 91–100 CrossRef CAS PubMed.
- D. Chen, Z. Mai, X. Liu, D. Ye, H. Zhang, X. Yin, Y. Zhou, M. Liu and W. Xu, UV-blocking, superhydrophobic and robust cotton fabrics fabricated using polyvinylsilsesquioxane and nano-TiO2, Cellulose, 2018, 25, 3635–3647 CrossRef CAS.
- D. Cheng, M. He, J. Ran, G. Cai, J. Wu and X. Wang, In situ reduction of TiO2 nanoparticles on cotton fabrics through polydopamine templates for photocatalysis and UV protection, Cellulose, 2018, 25, 1413–1424 CrossRef CAS.
- M. E. El-Naggar, S. Shaarawy and A. Hebeish, Multifunctional properties of cotton fabrics coated with in situ synthesis of zinc oxide nanoparticles capped with date seed extract, Carbohydr. Polym., 2018, 181, 307–316 CrossRef CAS PubMed.
- D. K. Subbiah, G. K. Mani, K. J. Babu, A. Das and J. B. B. Rayappan, Nanostructured ZnO on cotton fabrics–A novel flexible gas sensor & UV filter, J. Cleaner Prod., 2018, 194, 372–382 CrossRef CAS.
- L. E. Román, J. Huachani, C. Uribe, J. Solís, M. Gómez, S. Costa and S. Costa, Blocking erythemally weighted UV radiation using cotton fabrics functionalized with ZnO nanoparticles in situ, Appl. Surf. Sci., 2019, 469, 204–212 CrossRef.
-
E. Louris, E. Sfiroera, G. Priniotakis, R. Makris, H. Siemos, C. Efthymiou and M. Assimakopoulos, Evaluating the ultraviolet protection factor (UPF) of various knit fabric structures, in IOP Conference Series: Materials Science and Engineering, IOP Publishing, 2018 Search PubMed.
- Y. Liang, E. Pakdel, M. Zhang, L. Sun and X. Wang, Photoprotective properties of alpaca fiber melanin reinforced by rutile TiO2 nanoparticles: A study on wool fabric, Polym. Degrad. Stab., 2019, 160, 80–88 CrossRef CAS.
- S. Nourbakhsh, M. Montazer and Z. Khandaghabadi, Zinc oxide nano particles coating on polyester fabric functionalized through alkali treatment, J. Ind. Text., 2018, 47, 1006–1023 CrossRef CAS.
- N. Zhang, M. Xu and L. Cai, Improvement of mechanical, humidity resistance and thermal properties of heat-treated rubber wood by impregnation of SiO2 precursor, Sci. Rep., 2019, 9, 982 CrossRef PubMed.
- M. Gašparík, M. Gaff, F. Kačík and A. Sikora, Color and chemical changes in teak (Tectona grandis L. f.) and meranti (Shorea spp.) Wood after thermal treatment, BioResources, 2019, 14, 2667–2683 Search PubMed.
- V. Kučerová, R. Lagaňa and T. Hýrošová, Changes in chemical and optical properties of silver fir (Abies alba L.) wood due to thermal treatment, J. Wood Sci., 2019, 65, 21 CrossRef.
- V. Jirouš-Rajković and J. Miklečić, Heat-treated wood as a substrate for coatings, weathering of heat-treated wood, and coating performance on heat-treated wood, Adv. Mater. Sci. Eng., 2019, 8621486 Search PubMed.
- H. Shen, J. Xu, J. Cao, J. Jiang, S. Zhang, J. Xue and L. Zhang, Evolution of extractive composition in thermally modified Scots pine during artificial weathering, Holzforschung, 2019, 73, 747–755 CAS.
- R. Herrera, J. Sandak, E. Robles, T. Krystofiak and J. Labidi, Weathering resistance of thermally modified wood finished with coatings of diverse formulations, Prog. Org. Coat., 2018, 119, 145–154 CrossRef CAS.
- Z. Kaplan, H. Böke, A. Sofuoglu and B. İpekoğlu, Long term stability of biodegradable polymers on building limestone, Prog. Org. Coat., 2019, 131, 378–388 CrossRef CAS.
- I. Turku, T. Kärki and A. Puurtinen, Durability of wood plastic composites manufactured from recycled plastic, Heliyon, 2018, 4, e00559 CrossRef PubMed.
- T. Ratanawilai and K. Taneerat, Alternative polymeric matrices for wood-plastic composites: Effects on mechanical properties and resistance to natural weathering, Constr. Build. Mater., 2018, 172, 349–357 CrossRef CAS.
- S. Lv, J. Gu, H. Tan and Y. Zhang, Enhanced durability of sustainable poly (lactic acid)-based composites with renewable starch and wood flour, J. Cleaner Prod., 2018, 203, 328–339 CrossRef CAS.
- C. M. Chan, S. Pratt, P. Halley, D. Richardson, A. Werker, B. Laycock and L.-J. Vandi, Mechanical and physical stability of polyhydroxyalkanoate (PHA)-based wood plastic composites (WPCs) under natural weathering, Polym. Test., 2019, 73, 214–221 CrossRef CAS.
- S. D. Varsavas and C. Kaynak, Weathering degradation performance of PLA and its glass fiber reinforced composite, Mater. Today Commun., 2018, 15, 344–353 CrossRef CAS.
- Q. Ma, L. Chen, R. Wang, R. Yang and J. Zhu, Direct production of lignin nanoparticles (LNPs) from wood using p-toluenesulfonic acid in an aqueous system at 80 °C: characterization of LNP morphology, size, and surface charge, Holzforschung, 2018, 72, 933–942 CAS.
- F. Zikeli, V. Vinciguerra, A. D'Annibale, D. Capitani, M. Romagnoli and G. Scarascia Mugnozza, Preparation of lignin nanoparticles from wood waste for wood surface treatment, Nanomaterials, 2019, 9, 281 CrossRef CAS PubMed.
- T.-C. Chang and S.-T. Chang, Multiple photostabilization actions of heartwood extract from Acacia confusa, Wood Sci. Technol., 2017, 51, 1133–1153 CrossRef CAS.
- T.-C. Chang, T.-F. Yeh and S.-T. Chang, Investigation of photo-induced discoloration on wood treated with the polyphenols from Acacia confusa Heartwood, J. Wood Chem. Technol., 2019, 1–12 Search PubMed.
- X. Yang, Y. Zhao, H. Mussana, M. Tessema and L. Liu, Characteristics of cotton fabric modified with chitosan (CS)/cellulose nanocrystal (CNC) nanocomposites, Mater. Lett., 2018, 211, 300–303 CrossRef CAS.
- M. G. da Silva, M. A. S. de Barros, R. T. R. de Almeida, E. J. Pilau, E. Pinto, G. Soares and J. G. Santos, Cleaner production of antimicrobial and anti-UV cotton materials through dyeing with eucalyptus leaves extract, J. Cleaner Prod., 2018, 199, 807–816 CrossRef.
- M. Shabbir, L. J. Rather and F. Mohammad, Economically viable UV-protective and antioxidant finishing of wool fabric dyed with Tagetes erecta flower extract: Valorization of marigold, Ind. Crops Prod., 2018, 119, 277–282 CrossRef CAS.
- P. Pisitsak, N. Tungsombatvisit and K. Singhanu, Utilization of waste protein from Antarctic krill oil production and natural dye to impart durable UV-properties to cotton textiles, J. Cleaner Prod., 2018, 174, 1215–1223 CrossRef CAS.
- R. Pandey, S. Patel, P. Pandit, S. Nachimuthu and S. Jose, Colouration of textiles using roasted peanut skin-an agro processing residue, J. Cleaner Prod., 2018, 172, 1319–1326 CrossRef CAS.
- A. Ter Halle, L. Ladirat, M. Martignac, A. F. Mingotaud, O. Boyron and E. Perez, To what extent are microplastics from the open ocean weathered?, Environ. Pollut., 2017, 227, 167–174 CrossRef CAS PubMed.
- J. P. Da Costa, A. R. Nunes, P. S. Santos, A. V. Girão, A. C. Duarte and T. Rocha-Santos, Degradation of polyethylene microplastics in seawater: Insights into the environmental degradation of polymers, J. Environ. Sci. Health, Part A: Toxic/Hazard. Subst. Environ. Eng., 2018, 53, 866–875 CrossRef CAS PubMed.
- O. S. Alimi, J. Farner Budarz, L. M. Hernandez and N. Tufenkji, Microplastics and nanoplastics in aquatic environments: aggregation, deposition, and enhanced contaminant transport, Environ. Sci. Technol., 2018, 52, 1704–1724 CrossRef CAS PubMed.
- T. M. Karlsson, M. Hassellöv and I. Jakubowicz, Influence of thermooxidative degradation on the in situ fate of polyethylene in temperate coastal waters, Mar. Pollut. Bull., 2018, 135, 187–194 CrossRef CAS PubMed.
- I. Efimova, M. Bagaeva, A. Bagaev and I. P. Chubarenko, Secondary microplastics generation in the sea swash zone with coarse bottom sediments: laboratory experiments, Front. Mar. Sci., 2018, 5, 313 CrossRef.
- M. T. Ekvall, M. Lundqvist, E. Kelpsiene, E. Šileikis, S. B. Gunnarsson and T. Cedervall, Nanoplastics formed during the mechanical breakdown of daily-use polystyrene products, Nanoscale Adv., 2019, 1, 1055–1061 RSC.
Footnotes |
† Representative Concentration Pathways are greenhouse gas concentration (not emission) trajectories adopted by the IPCC for its fifth Assessment Report (AR5) in 2014. The pathways are used for climate modelling and research. They describe four climate futures, which differ in the amount of greenhouse gases that are emitted in years to come. The four RCPs, RCP 2.6, RCP 4.5, RCP 6, and RCP 8.5, are named after a possible range of radiative forcing values in the year 2100 relative to pre-industrial values (+2.6, +4.5, +6.0, and +8.5 W m−2, respectively). |
‡ The SAM is the leading mode of southern hemisphere extratropical climate variability, describing a see-saw of atmospheric mass between the mid- and high-latitudes, with corresponding impacts on the strength of the circumpolar westerly winds. A positive SAM index corresponds to a poleward shift of the maximum wind speed, which results in weaker-than-normal westerly winds in the southern mid-latitudes. |
|
This journal is © The Royal Society of Chemistry and Owner Societies 2020 |