DOI:
10.1039/C9RA03146D
(Paper)
RSC Adv., 2019,
9, 17347-17357
One-pot construction of diverse and functionalized isochromenoquinolinediones by Rh(III)-catalyzed annulation of unprotected arylamides with 3-diazoquinolinediones and their application for fluorescence sensor†
Received
27th April 2019
, Accepted 27th May 2019
First published on 3rd June 2019
Abstract
A facile and efficient Rh(III)-catalyzed annulation of arylamides with 3-diazoquinolinediones for the construction of diverse and highly functionalized isochromenoquinolinediones is described. Furthermore, the methodology is applicable for delivering various relevant molecules such as pyridopyranoquinolindiones, thienopyranoquinolinones, and indolopyranoquinolinone. The reaction proceeds via cascade C–H activation, carbene insertion, and intramolecular lactonization. The reaction exhibits high atom economy, good functional group tolerance, and high regioselectivity. The synthesized compound can also behave as a potent fluorescence sensor for Fe3+ ion.
Introduction
Molecules bearing isochromenones or quinolinones are widely found in bioactive natural products, synthetic molecules, and pharmaceuticals.1,2 They are also used as valuable building blocks for the synthesis of medicines and functional materials.3,4 Among them, isochromenones containing a six-membered lactone ring possess potent biological functions such as anticancer, antimicrobial, anti-inflammatory, anticoagulant, and anti-HIV activities (Fig. 1A).5 The nitrogen-containing heterocycles, quinolinones also have a broad range of biological properties including anticancer, antibiotic, antiviral, antibacterial, and antihypertensive activities (Fig. 1B).6
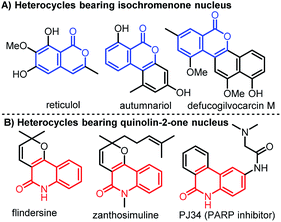 |
| Fig. 1 Biologically active heterocycles bearing isochromenone or quinolinone moiety. | |
Owing to their importance and usefulness, various approaches for the synthesis of isochromenones or quinolinones have been developed. The typical methods for the preparation of isochromenones include nickel-catalyzed decarbonylative addition of anhydrides to alkynes,7 Rh(III)-catalyzed reaction of phosphonium ylides or benzamides with diazocarbonyl compounds,8 N-heterocyclic carbene/Lewis acid-catalyzed dimerization of 2-formylcinnamates,9 and Ru(II)-catalyzed electrooxidative [4 + 2] reaction of benzyl alcohols with alkynes.10 The representative strategies for the synthesis of quinolinones include Rh-catalyzed C–H bond activation of anilines with CO and alkynes,11 Rh-catalyzed decarbonylative coupling of isatins with alkynes,12 Ru-catalyzed cyclization of anilides with propiolates or acrylates,13 Pd-catalyzed cascade reaction of anilines with acrylates,14 direct oxidation C–H amidation of amides.15 In addition, Rh(III)-catalyzed annulation of benzamides with diazo compounds for isoquinolinones was reported (Scheme 1a).16 Although a number of methods for the synthesis of isochromenones and quinolinones or isoquinolinones have been demonstrated, the direct construction of heterocycles bearing both isochromenones and quinolin-2-ones are only reported sporadically.17 Recently, the reported Pd(II)-catalyzed carbonylation of alkynes is one such example (Scheme 1b).17 However, there is no example for the direct construction of highly functionalized isochromenoquinolinediones by Rh(III)-catalyzed annulation of unprotected arylamides with 3-diazoquinolinediones.
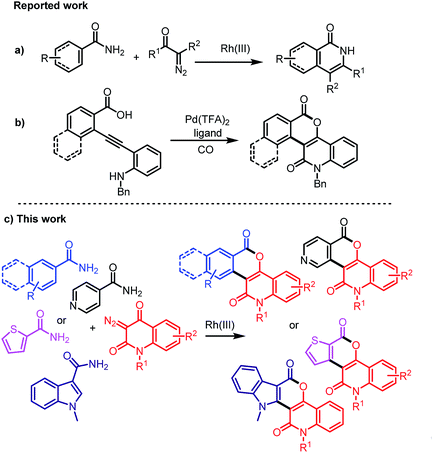 |
| Scheme 1 Representative strategies for the construction of isoquinolinones, isochromenoquinolinones, and their derivatives. | |
As a part of our ongoing studies on the development of new synthetic methodologies for the construction of heterocycles utilizing diazo compounds,18 this paper describes an efficient Rh(III)-catalyzed annulation of unprotected arylamides, isonicotinamide, thiophene-2-carboxamide, and indole-3-carboxamide with various 3-diazoquinoline-2,4-diones for the construction of diverse and functionalized heterocycles bearing isochromenones and quinolinones (Scheme 1c).
Results and discussion
Our investigation commenced with the reaction of primary benzamide (1a) with 3-diazo-1-methylquinoline-2,4-dione (2a) in the presence of different catalysts, additives, and solvents (Table 1). The initial reaction with 2.5 mol% [RuCl2(p-cymene)]2 and 20 mol% Cu(OAc)2 under reflux in 1,2-dichloroethane for 12 h provided the N–H insertion product 3a′ (48%), along with a trace amount of 3a (entry 1). However, with 2.5 mol% of [RhCp*Cl2]2 and 20 mol% Cu(OAc)2, 3a was isolated in 64% yield as the sole product (entry 2). Importantly, [RhCp*Cl2]2 showed superior catalytic activity than [RuCl2(p-cymene)]2 for the synthesis of 3a. To our delight, an increase in the amount of Cu(OAc)2 to 100 mol% improved the yield of 3a to 72% (entry 3). Encouraged by this result, we screened other additives such as CsOAc, AgNO3, AgSbF6, Ag2CO3, and AgOAc for this reaction, along with 2.5 mol% [RhCp*Cl2]2 (entries 4–8). Among these, AgOAc was found to be the best additive for this transformation. Importantly, increasing the amount of AgOAc to 200 mol% not only reduced the reaction time to 8 h, but also increased the yield of 3a to 84% (entry 9). However, decreasing the loading of AgOAc to 20 mol% decreased the yield of 3a to 52% (entry 10). In addition, decreasing the loading of [RhCp*Cl2]2 to 1.0 mol% in the presence of 200 mol% AgOAc provided 3a in 70% yield (entry 11). However, decreasing the reaction temperature to room temperature did not afford any product (entry 12). On the other hand, 3a was not obtained in the absence of AgOAc or [RhCp*Cl2]2 (entries 13–14). Further reactions using other nonpolar or polar solvents such as toluene, 2,2,2-trifluoroethanol (TFE), acetonitrile, and ethanol provided 3a in lower yields (45–68%) (entries 15–18). The structure of 3a was identified by the analysis of its spectral data. The 1H NMR spectrum of 3a revealed the characteristic N-methyl peak at δ 3.80 ppm and 8 aromatic proton peaks at δ 9.60, 8.40, 8.34, 7.86, 7.67, 7.89, 7.42, and 7.35 ppm. The 13C NMR spectrum of 3a exhibited peaks due to the carbonyl carbon of a conjugated lactone moiety at δ 160.7 ppm and a conjugated amide carbonyl carbon at δ 160.2 ppm. The structure of 3a was further confirmed by single-crystal X-ray crystallographic analysis of the structurally related compound 3j (Fig. 2).
Table 1 Optimization of the reaction conditionsa
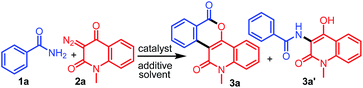
|
Entry |
Catalyst (mol%) |
Additive (mol%) |
Solvent |
Temp. |
Time |
Yieldb (%) |
3a
|
3a′
|
Reaction conditions: 1a (0.5 mmol), 2a (0.5 mmol) in solvent (5 mL) under N2.
Isolated yields after column chromatography.
|
1 |
[RhCl2(p-cym)]2 (2.5) |
Cu(OAc)2 (20) |
DCE |
Reflux |
12 |
Trace |
48 |
2 |
[RhCp*Cl2]2 (2.5) |
Cu(OAc)2 (20) |
DCE |
Reflux |
12 |
64 |
0 |
3 |
[RhCp*Cl2]2 (2.5) |
Cu(OAc)2 (100) |
DCE |
Reflux |
12 |
72 |
0 |
4 |
[RhCp*Cl2]2 (2.5) |
CsOAc (100) |
DCE |
Reflux |
12 |
67 |
0 |
5 |
[RhCp*Cl2]2 (2.5) |
AgNO3 (100) |
DCE |
Reflux |
12 |
68 |
0 |
6 |
[RhCp*Cl2]2 (2.5) |
AgSbF6 (100) |
DCE |
Reflux |
12 |
74 |
0 |
7 |
[RhCp*Cl2]2 (2.5) |
Ag2CO3 (100) |
DCE |
Reflux |
12 |
76 |
0 |
8 |
[RhCp*Cl2]2 (2.5) |
AgOAc (100) |
DCE |
Reflux |
12 |
80 |
0 |
9
|
[RhCp*Cl
2
]
2
(2.5)
|
AgOAc (200)
|
DCE
|
Reflux
|
8
|
84
|
0
|
10 |
[RhCp*Cl2]2 (2.5) |
AgOAc (20) |
DCE |
Reflux |
12 |
52 |
0 |
11 |
[RhCp*Cl2]2 (1.0) |
AgOAc (200) |
DCE |
Reflux |
12 |
70 |
0 |
12 |
[RhCp*Cl2]2 (2.5) |
AgOAc (200) |
DCE |
rt |
12 |
0 |
0 |
13 |
[RhCp*Cl2]2 (2.5) |
— |
DCE |
Reflux |
12 |
0 |
0 |
14 |
— |
AgOAc (20) |
DCE |
Reflux |
12 |
0 |
0 |
15 |
[RhCp*Cl2]2 (2.5) |
AgOAc (20) |
Toluene |
100 °C |
12 |
68 |
0 |
16 |
[RhCp*Cl2]2 (2.5) |
AgOAc (20) |
TFE |
Reflux |
12 |
65 |
0 |
17 |
[RhCp*Cl2]2 (2.5) |
AgOAc (20) |
CH3CN |
Reflux |
12 |
45 |
0 |
18 |
[RhCp*Cl2]2 (2.5) |
AgOAc (20) |
EtOH |
Reflux |
12 |
58 |
0 |
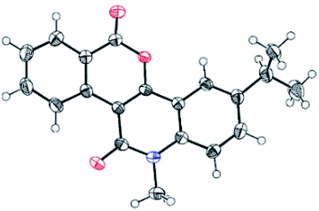 |
| Fig. 2 X-ray structure of compound 3j (CCDC 1878962). | |
With the optimized conditions in hand, we further explored the generality of the reactions by employing diversely substituted 3-diazoquinoline-2,4-diones 2b–2o (Table 2). The reactions of 1a with N-substituted 3-diazoquinoline-2,4-diones 2b–2h bearing N-chloropropyl, N-but-3-en-1-yl, N-benzyl, N-allyl, N-prenyl, N-cinnamyl, and N-trans,trans-farnesyl groups were successfully carried out to produce the desired products 3b–3h in 62–82% yields. In addition, reactions of other 3-diazoquinolindiones 2i–2o bearing various substituents on the benzene ring were successful. Treatment of 1a with 3-diazo-N-methylquinolindione compounds 2i–2k bearing electron-donating groups such as 6-Me, 6-i-Pr, and 7-OMe provided the desired products 3i–3k in 74%, 81%, and 68% yields, whereas that with diazo compounds 2l–2o bearing electron-withdrawing groups such as 5-Cl, 6-Cl, 6-Br, and 7-Cl afforded the corresponding products 3l–3o in 79–85% yields.
Table 2 Synthesis of diverse fused isochromenoquinolinediones 3b–3o by the reaction of 1a with 2b–2o
To demonstrate the versatility of this protocol, further reactions of various benzamides 1b–1m with 3-diazo-N-methylquinoline-2,4-dione (2a) were investigated (Table 3). Treatment of 2a with benzamides 1b–1d bearing electron-donating groups such as 4-Me, 4-OMe, and 4-Ph on the benzene ring provided 4a–4c in 75%, 72%, and 78% yields, respectively, whereas the reactions with benzamides 1e–1i bearing electron-withdrawing groups such as 4-Cl, 4-Br, 4-COMe, 4-CO2Me and 4-CN afforded 4d–4h in 62–86% yields. However, the reaction of 1j bearing a strong electron-withdrawing –NO2 group at 4-position of the benzene ring did not afford the expected product 4i. On the other hand, the reactions of ortho-substituted benzamides 1k and 1l with 2a afforded the desired products 4j and 4k in 76% and 74% yields, respectively. In addition, when 3,4-dichlorobenzamide (1m) bearing two electron-withdrawing groups on the benzene ring was allowed to react with 2a, the desired product 4l was produced in 84% yield. In this case, other possible regioisomers were not observed.
Table 3 Synthesis of diverse isochromenoquinolinediones 4a–4l by the reaction of 1b–1m with 2a
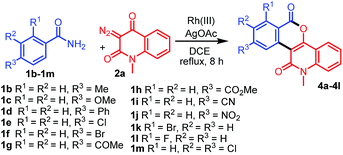
|
Reaction time = 18 h.
|
|
After demonstrating the generality and versatility of this methodology, we investigated the possibility of using other arylamides 1n–1p bearing polyaromatic and heteroatomic rings with diazo compounds (Scheme 2). For example, the reaction of 2-naphthamide (1n) with 2a or 2d provided 5 and 6 in 76% and 71% yields, respectively, while that of isonicotinamide (1o) bearing a N-heteroaromatic ring with 2a or 2l afforded the desired products 7 and 8 in 48% and 58% yields, respectively. On the other hand, treatment of thiophene-2-carboxamide (1p) bearing an S-heteroaromatic ring with 2a or 2j led to the formation of the expected products 9 and 10 in 62% and 63% yields, respectively.
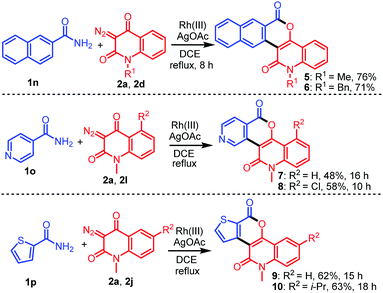 |
| Scheme 2 Construction of diverse fused heterocycles 5–10 bearing polyaromatic and heteroaromatics by the reaction of 1n–1p with 2a, 2d, 2l, or 2j. | |
As an application of this protocol, diversely oriented polycyclic heterocycle 11 was next prepared in 62% yield employing 1-methyl-1H-indole-3-carboxamide (1q) with diazo compound 2a under standard reaction conditions (Scheme 3).
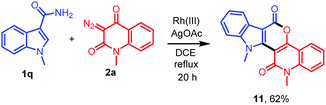 |
| Scheme 3 Construction of polycyclic heterocycle 11 by the reaction of 1q with 2a. | |
The chemoselectivity of the reactions of different benzamides with diazo compound 2a was investigated under standard conditions (Scheme 4). Treatment of two different benzamides 1a (0.5 mmol) and 1c (0.5 mmol) with diazo compound 2a (0.5 mmol) for 8 h afforded the products 3a and 4b in 40% and 28% yields, respectively (Scheme 4, eqn (1)). On the other hand, the reaction of benzamides 1a (0.5 mmol) and 1f (0.5 mmol) afforded 3a and 4e in 37% and 42% yields, respectively (Scheme 4, eqn (2)). These results indicated that benzamide 1f bearing the electron-withdrawing group on the benzene ring is more chemoselective than the unsubstituted benzamide 1a and the benzamide 1c bearing electron-donating groups.
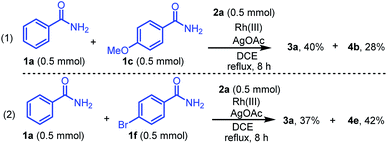 |
| Scheme 4 Chemoselectivity of different benzamides 1a, 1c, or 1f with diazo compound 2a. | |
To elucidate the reaction mechanism, some deuterium labelling experiments were performed (Scheme 5). The H/D exchange experiment was first carried out with 2.5 mol% Rh(III) and AgOAc (2 equivalents) of 1a (Scheme 5a). A significant deuterium exchange (65%) was observed at each of the two ortho positions of the benzamide to afford the product 1a′.19a This result suggested that the rhodation–proto(deuterio)derhodation process is reversible at the ortho positions. A kinetic isotope effect (KIE) experiment was next carried out (Scheme 5b).19b A significant primary KIE value (KH/KD = 3.5) was observed via1H NMR analysis, which indicated that C–H bond cleavage occurs during the rate-determining step.
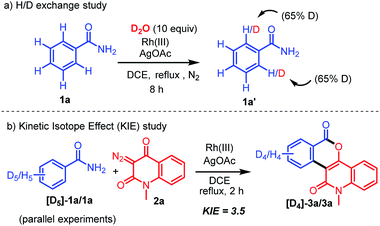 |
| Scheme 5 Deuterium labelling experiments. | |
On the basis of the control experiments and available literature,20 a plausible mechanism for the formation of 3a is depicted in Scheme 6. Initially, an active catalyst [RhCp*(OAc)2] is generated through anion exchange from [RhCp*Cl2]2 and AgOAc. A coordination reaction between the activated catalyst and 1a gives rhodacyclic intermediate Avia a concerted metalation/deprotonation pathway. Then, diazo compound 2a reacts with intermediate A to form carbene intermediate B through the release of nitrogen. Subsequently, the migratory insertion of the carbene group into the Rh–C bond delivers the eight membered rhodacycle intermediate C that is protonated by AcOH to yield the intermediate D, with regeneration of Rh(III) catalyst for the next catalytic cycle. Finally, rapid lactonization of D furnishes 3a.21
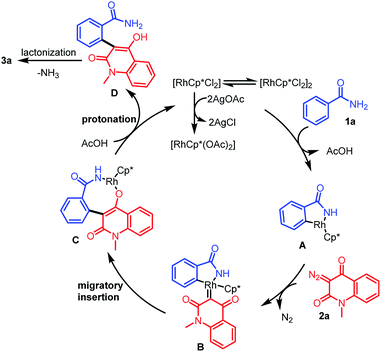 |
| Scheme 6 Reaction mechanism for the formation of 3a. | |
The synthesized compounds were evaluated as fluorescent sensor for sensing Fe3+ ions. Currently, the use of fluorescent molecular probes emitting in the visible region has become quite significant and have attracted considerable attention in academic and industrial research.22 Initially, the compound 4a (10 μM) was optimized in seven different solvents: 1,4-dioxane, dimethyl sulfoxide (DMSO), 1,2-dichloroethane (DCE), acetonitrile (ACN), dichloromethane (DCM), tetrahydrofuran (THF) and ethanol (EtOH) (Fig. S1†). Among these solvents, the compound 4a in 1,4-dioxane emitted the highest florescence maximum at 395 nm wavelength with intensity 3725 a.u. upon excitation at 350 nm. Hence, we examined the fluorescent properties of some of the synthesized compounds, viz.3a, 3g, 3j, 3l, 4a, 4c, 4d, 5, 7, and 10 in 1,4-dioxane.
Interestingly, compound 4a emitted the highest fluorescence intensity amongst all the compounds examined (Fig. 3a). Therefore, the fluorescence response of 10 μM 4a was recorded against various metal ions in water (Fig. 3b). Indeed, as indicated in Fig. 3b, among the different metal ions, the compound 4a selectively sensed Fe3+. Following this, the fluorescence response of 4a (excitation at 350 nm) was examined against various concentrations of Fe3+ (100 to 900 μM) in water (Fig. 4a). The fluorescent intensity of compound 4a was quenched linearly by increasing Fe3+ concentration with a correlation coefficient (R2) of 0.9961 (Fig. 4b). Hence, the compound 4a can be utilized as a potent fluorescence sensor for Fe3+ ions.
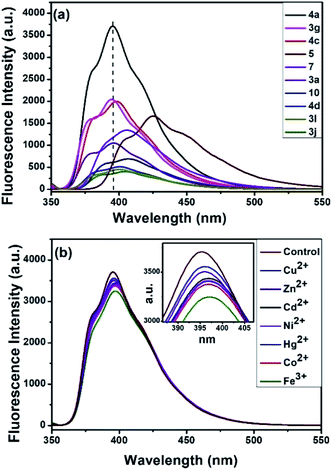 |
| Fig. 3 (a) Fluorescence responses of different compounds (10 μM, λex = 350 nm). (b) Fluorescence responses of 4a in the presence of various metal cations (10 μM, λex = 350 nm). | |
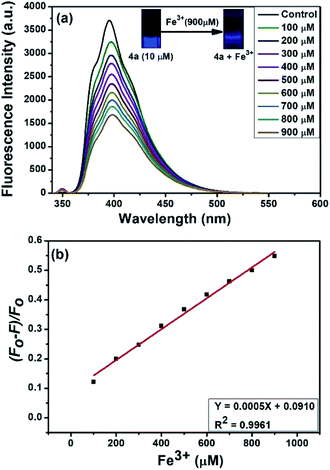 |
| Fig. 4 (a) Fluorescence spectra of 4a in the presence of different concentrations of aqueous Fe3+ (100 to 900 μM). (b) Plot of quenching efficiency versus the concentration of Fe3+ ions. | |
Conclusions
In conclusion, we have developed an efficient protocol for the Rh(III)-catalyzed direct annulation of unprotected arylamides, isonicotinamide, thiophene-2-carboxamide, and indole-3-carboxamide with various 3-diazoquinoline-2,4-diones to synthesize various isochromenoquinolinediones, pyridopyranoquinolinediones, thienopyranoquinolineones, and indolopyranoquinolinedione that can be widely used as a significant building block for the synthesis of bioactive natural products and pharmaceuticals. This protocol provides a rapid access to fused analogs of isochromenones as a one-pot procedure, and has several advantages such as high atom economy, high regioselectivity, and good tolerance of various functional groups with good yields. In addition, the synthesized compounds showed potent turn-off fluorescence sensing for Fe3+ ion.
Experimental
General information
All the reactions were carried out under nitrogen atmosphere in a 25 mL two-necked round-bottom flask with magnetic stirring. Merck silica gel plates (Art. 5554) precoated with a fluorescent indicator were used for analytical TLC analysis. Flash column chromatography was performed using silica gel 9385 (Merck). Melting points were determined using micro-cover glasses on a Fisher-Johns apparatus and are uncorrected. 1H NMR and 13C NMR spectra were recorded on Varian VNS (600 MHz and 150 MHz, respectively) spectrometers in CDCl3. Chemical shifts for protons were reported as parts per million in δ scale using solvent residual peak (CHCl3: 7.24 ppm) as internal standard. Chemical shifts of 13C NMR spectra were reported in ppm from the central peak of CDCl3 (77.00 ppm) on the δ scale. Fourier transform infrared (FT-IR) spectra were recorded on a PerkinElmer FT-IR spectrometer Spectrum Two™. High-resolution mass spectra (HRMS) were obtained with a JEOL JMS-700 spectrometer at the Korea Basic Science Institute.
General procedure for the synthesis of isochromenoquinolinone, pyridopyranoquinolinone, thienopyranoquinolinone, and indolopyranoquinolinone derivatives (3–11)
In an oven dried two-necked flask, a mixture of benzamide 1 (0.5 mmol) and 3-diazo-N-substituted quinoline-2,4-dione 2 (0.5 mmol) were dissolved in DCE (5 mL). This was then followed by addition of [RhCp*Cl2]2 (2.5 mol%), and AgOAc (2.0 equiv.) under nitrogen atmosphere. The reaction mixture was stirred under reflux condition, and the progress of the reaction was followed by TLC analysis. After completion, the reaction mixture was cooled to room temperature. The volatiles were removed in vacuo and the residue was purified by silica gel column chromatography (Hex
:
EtOAc = 1
:
5) to obtain the desired products 3–11.
N-(4-Hydroxy-1-methyl-2-oxo-1,2-dihydroquinolin-3-yl)benzamide (3a′).
Yield: 48% (70 mg); white solid; mp: 190–192 °C. IR (ATR): 3299, 1757, 1643, 1617, 1597, 1464, 1374, 1360, 1087 cm−1. 1H NMR (600 MHz, CDCl3): δ 13.11 (1H, s), 9.56 (1H, s), 8.17 (1H, d, J = 7.8 Hz), 7.97 (2H, t, J = 7.8 Hz), 7.59–7.54 (2H, m), 7.51 (2H, t, J = 7.2 Hz), 7.33–7.28 (2H, m), 3.75 (3H, s). 13C NMR (150 MHz, CDCl3): δ 166.7, 159.8, 149.0, 136.6, 132.8, 132.2, 130.5, 128.9, 127.6, 124.8, 122.6, 117.3, 113.7, 109.2, 29.99. HRMS m/z (M+): calcd for C17H14N2O3: 294.1004; found: 294.1007.
12-Methyl-6H-isochromeno[4,3-c]quinoline-6,11(12H)-dione (3a).
Yield: 84% (116 mg); white solid; mp: 286–288 °C. IR (ATR): 2923, 1754, 1625, 1503, 1466, 1340, 1254, 1234, 1202, 1025, 972, 802 cm−1. 1H NMR (600 MHz, CDCl3): δ 9.6 (1H, d, J = 8.4 Hz), 8.40 (1H, d, J = 8.4 Hz), 8.34 (1H, d, J = 7.8 Hz), 7.86 (1H, t, J = 8.4 Hz), 7.67 (1H, t, J = 8.4 Hz), 7.59 (1H, t, J = 7.8 Hz), 7.42 (1H, d, J = 9 Hz), 7.35 (1H, t, J = 7.8 Hz), 3.80 (3H, s). 13C NMR (150 MHz, CDCl3): δ 160.6, 160.1, 154.6, 139.0, 135.6, 134.7, 132.6, 129.9, 128.8, 127.2, 124.1, 122.7, 120.6, 114.2, 113.6, 105.0, 29.8. HRMS m/z (M+): calcd for C17H11NO3: 277.0739; found: 277.0738.
12-(3-Chloropropyl)-6H-isochromeno[4,3-c]quinoline-6,11(12H)-dione (3b).
Yield: 82% (138 mg); white solid; mp: 200–202 °C. IR (ATR): 3166, 2923, 2857, 1792, 1654, 1602, 1526, 1428, 1390, 1285, 1239, 1193, 1121, 994, 859 cm−1. 1H NMR (600 MHz, CDCl3): δ 9.55 (1H, d, J = 8.4 Hz), 8.39 (1H, dd, J = 8.4, 1.8 Hz), 8.34 (1H, dd, J = 8.4, 1.8 Hz), 7.85 (1H, t, J = 7.2 Hz), 7.68 (1H, J = 8.4 Hz), 7.59 (1H, t, J = 7.8 Hz), 7.50 (1H, d, J = 9.0 Hz), 7.35 (1H, t, J = 7.2 Hz), 4.53 (2H, t, J = 7.2 Hz), 3.74 (2H, t, J = 6 Hz), 2.29–2.25 (2H, m). 13C NMR (150 MHz, CDCl3): δ 160.5, 160.0, 154.7, 138.1, 135.6, 134.5, 132.8, 129.9, 128.9, 127.1, 124.4, 122.8, 120.6, 113.9, 113.8, 104.8, 42.7, 40.7, 30.3. HRMS m/z (M+): calcd for C19H14ClNO3: 339.0662; found: 339.0660.
12-(But-3-en-1-yl)-6H-isochromeno[4,3-c]quinoline-6,11(12H)-dione (3c).
Yield: 68% (95 mg); white solid; mp: 180–182 °C. IR (ATR): 2995, 2917, 1824, 1769, 1539, 1597, 1479, 1375, 1254, 1097, 1023, 908, 856 cm−1. 1H NMR (600 MHz, CDCl3): δ 9.52 (1H, d, J = 7.8 Hz), 8.34 (1H, d, J = 7.2 Hz), 8.26 (1H, d, J = 8.4 Hz), 7.82–7.80 (1H, m), 7.62–7.60 (1H, m), 7.56–7.54 (1H, m), 7.35 (1H, d, J = 8.4 Hz), 7.28 (1H, t, J = 8.4 Hz), 5.94–5.80 (1H, m), 5.16–5.13 (1H, m), 5.09 (1H, d, J = 9.6 Hz), 4.37 (2H, t, J = 7.8 Hz), 2.52–2.49 (2H, m). 13C NMR (150 MHz, CDCl3): δ 160.1, 159.9, 154.4, 138.0, 135.5, 134.5, 134.1, 132.4, 129.7, 128.7, 127.0, 124.2, 122.5, 120.4, 117.4, 114.0, 113.6, 104.7, 42.0, 31.7. HRMS m/z (M+): calcd for C20H15NO3: 317.1052; found: 317.1054.
12-Benzyl-6H-isochromeno[4,3-c]quinoline-6,11(12H)-dione (3d).
Yield: 76% (134 mg); white solid; mp: 198–200 °C. IR (ATR): 2880, 1785, 1661, 1599, 1514, 1490, 1395, 1352, 1258, 1172, 1037, 841 cm−1. 1H NMR (600 MHz, CDCl3): δ 9.61 (1H, d, J = 9.0 Hz), 8.40 (1H, d, J = 8.4 Hz), 8.33 (1H, d, J = 7.8 Hz), 7.85 (1H, t, J = 7.8 Hz), 7.60 (1H, d, J = 7.8 Hz), 7.52 (1H, t, J = 7.8 Hz), 7.32–7.28 (4H, m), 7.24–7.22 (3H, m), 5.63 (2H, s). 13C NMR (150 MHz, CDCl3): δ 160.7, 160.0, 154.8, 138.4, 136.0, 135.6, 134.6, 132.5, 129.8, 128.9, 128.8, 127.4, 127.2, 126.4, 124.0, 122.8, 120.5, 115.0, 113.7, 104.8, 46.2. HRMS m/z (M+): calcd for C23H15NO3: 353.1052; found: 353.1049.
12-Allyl-6H-isochromeno[4,3-c]quinoline-6,11(12H)-dione (3e).
Yield: 64% (96 mg); white solid; mp: 165–167 °C. IR (ATR): 2988, 2894, 2014, 1917, 1766, 1469, 1382, 1244, 1101, 1054, 845 cm−1. 1H NMR (600 MHz, CDCl3): δ 9.54 (1H, d, J = 8.4 Hz), 8.36–8.35 (1H, m), 8.29 (1H, dd, J = 8.4, 1.2 Hz), 7.83–7.80 (1H, m), 7.60–7.55 (2H, m), 7.34–7.28 (2H, m), 6.01–5.95 (1H, m), 5.24–5.22 (1H, m), 5.13–5.10 (1H, m), 5.00–4.99 (2H, m). 13C NMR (150 MHz, CDCl3) δ 160.2, 160.0, 154.7, 138.3, 135.5, 134.5, 132.4, 131.5, 129.8, 128.7, 127.1, 124.0, 122.7, 120.5, 117.3, 114.7, 113.6, 104.7, 44.9. HRMS m/z (M+): calcd for C19H13NO3: 303.0895; found: 303.0894.
12-(3-Methylbut-2-en-1-yl)-6H-isochromeno[4,3-c]quinoline-6,11(12H)-dione (3f).
Yield: 81% (134 mg); white solid; mp: 195–197 °C. IR (ATR): 2881, 1753, 1661, 1590, 1497, 1391, 1257, 1166, 1134, 1031, 947, 897 cm−1. 1H NMR (600 MHz, CDCl3): δ 9.59 (1H, d, J = 8.4 Hz), 8.37 (1H, dd, J = 9.6, 1.2 Hz), 8.30 (1H, dd, J = 7.8, 1.2 Hz), 7.85–7.82 (1H, m), 7.62–7.59 (1H, m), 7.58–7.56 (1H, m), 7.34–7.29 (2H, m), 5.16–5.14 (1H, m), 4.99 (2H, d, J = 4.8 Hz), 1.91 (3H, s), 1.72 (3H, s). 13C NMR (150 MHz, CDCl3): δ 160.3, 160.1, 154.6, 138.4, 136.4, 135.5, 134.7, 132.4, 129.8, 128.7, 127.2, 124.1, 122.5, 120.5, 119.1, 114.6, 113.7, 104.9, 41.2, 25.6, 18.4. HRMS m/z (M+): calcd for C21H17NO3: 331.1208; found: 331.1210.
12-Cinnamyl-6H-isochromeno[4,3-c]quinoline-6,11(12H)-dione (3g).
Yield: 62% (117 mg); white solid; mp: 226–228 °C. IR (ATR): 2926, 1799, 1675, 1579, 1492, 1442, 1323, 1238, 1193, 1017, 962, 898 cm−1. 1H NMR (600 MHz, CDCl3): δ 9.62 (1H, d, J = 2.4 Hz), 8.41 (1H, d, J = 7.8 Hz), 8.37 (1H, d, J = 8.4 Hz), 7.89–7.86 (1H, m), 7.66–7.63 (1H, m), 7.61 (1H, t, J = 8.4 Hz), 7.48 (1H, d, J = 8.4 Hz), 7.35 (1H, t, J = 7.8 Hz), 7.31 (2H, d, J = 8.4 Hz), 7.26–7.24 (2H, m), 7.19 (1H, t, J = 7.2 Hz), 6.59 (1H, d, J = 16 Hz), 6.37–6.32 (1H, m), 5.20 (2H, d, J = 5.4 Hz). 13C NMR (150 MHz, CDCl3): δ 160.4, 160.1, 154.9, 138.4, 136.1, 135.6, 134.7, 132.8, 132.6, 129.9, 128.9, 128.5, 127.9, 127.2, 126.4, 124.2, 123.0, 122.8, 120.6, 114.7, 113.8, 104.9, 44.6. HRMS m/z (M+): calcd for C25H17NO3: 379.1208; found: 379.1211.
12-((2E,6E)-3,7,11-Trimethyldodeca-2,6,10-trien-1-yl)-6H-isochromeno[4,3-c]quinoline-6,11(12H)-dione (3h).
Yield: 72% (168 mg); white solid; mp: 85–87 °C. IR (ATR): 2880, 1771, 1658, 1599, 1515, 1394, 1357, 1354, 1131, 1170, 1070, 1035, 966, 831 cm−1. 1H NMR (600 MHz, CDCl3): δ 9.61 (1H, d, J = 7.8 Hz), 8.39 (1H, d, J = 1.8 Hz), 8.38 (1H, d, J = 1.8 Hz), 7.86–7.83 (1H, m), 7.63–7.57 (2H, m), 7.34–7.30 (2H, m), 5.17 (1H, t, J = 6 Hz), 5.02–4.97 (4H, m), 2.09–2.01 (4H, m), 1.95–1.91 (5H, m), 1.87–1.85 (2H, m), 1.61 (3H, s), 1.51 (6H, d, J = 3.6 Hz). 13C NMR (150 MHz, CDCl3): δ 160.3, 160.2, 154.6, 139.8, 138.4, 135.5, 135.4, 134.7, 132.4, 131.3, 129.8, 128.7, 127.2, 124.2, 124.1, 123.5, 122.6, 120.6, 119.1, 114.7, 113.7, 104.9, 41.2, 39.6, 39.4, 26.7, 26.2, 25.6, 17.6, 16.8, 16.01. HRMS m/z (M+): calcd for C31H33NO3: 467.2460; found: 467.2458.
3,12-Dimethyl-6H-isochromeno[4,3-c]quinoline-6,11(12H)-dione (3i).
Yield: 74% (107 mg); white solid; mp: 246–248 °C. IR (ATR): 2924, 1757, 1624, 1573, 1503, 1473, 1413, 1300, 1265, 1234, 1105, 1055, 975 cm−1. 1H NMR (600 MHz, CDCl3): δ 9.46 (1H, d, J = 8.4 Hz), 8.29 (1H, d, J = 7.2 Hz), 7.78 (1H, t, J = 7.8 Hz), 7.78 (1H, t, J = 7.8 Hz), 7.53 (1H, t, J = 6.6 Hz), 7.26–7.24 (1H, m), 7.10 (1H, d, J = 9.0 Hz), 3.63 (3H, s), 3.340 (3H, s). 13C NMR (150 MHz, CDCl3): 160.1, 160.0, 154.1, 136.7, 135.3, 134.6, 133.7, 132.4, 129.6, 128.5, 127.0, 123.2, 120.3, 113.9, 113.0, 104.5, 29.6, 20.7. HRMS m/z (M+): calcd for C18H13NO3: 291.0895; found: 291.0893.
3-Isopropyl-12-methyl-6H-isochromeno[4,3-c]quinoline-6,11(12H)-dione (3j).
Yield: 81% (129 mg); white solid; mp: 228–230 °C. IR (ATR): 2978, 1902, 1772, 1692, 1539, 1405, 1379, 1292, 1154, 1024, 978, 817 cm−1. 1H NMR (600 MHz, CDCl3): δ 9.57 (1H, dd, J = 8.4, 1.2 Hz), 8.37 (1H, dd, J = 7.8, 1.2 Hz), 8.12 (1H, d, J = 1.8 Hz), 7.84–7.81 (1H, m), 7.57–7.55 (1H, m), 7.53 (1H, dd, J = 8.4, 2.4 Hz), 7.31 (1H, d, J = 9.0 Hz), 3.75 (3H, s), 3.07–3.01 (1H, m), 1.31 (6H, d, J = 6.6 Hz). 13C NMR (150 MHz, CDCl3): δ 160.5, 160.3, 154.6, 143.5, 137.3, 135.5, 134.8, 131.4, 129.8, 128.6, 127.1, 121.1, 120.4, 114.3, 113.4, 104.7, 33.6, 29.7, 24.0. HRMS m/z (M+): calcd for C20H17NO3: 319.1208; found: 319.1201.
2-Methoxy-12-methyl-6H-isochromeno[4,3-c]quinoline-6,11(12H)-dione (3k).
Yield: 68% (100 mg); white solid; mp: 252–254 °C. IR (ATR): 2880, 1839, 1657, 1600, 1493, 1393, 1352, 1256, 1180, 1127, 1034, 911, 814 cm−1. 1H NMR (600 MHz, CDCl3): δ 9.48 (1H, d, J = 8.4 Hz), 8.34 (1H, dd, J = 7.8, 1.2 Hz), 8.17 (1H, d, J = 9.0 Hz), 7.83–7.80 (1H, m), 7.54–7.52 (1H, m), 6.87 (1H, dd, J = 9.0, 2.4 Hz), 6.74 (1H, d, J = 2.4 Hz), 3.90 (3H, s), 3.71 (3H, s). 13C NMR (150 MHz, CDCl3): δ 163.3, 161.0, 160.3, 154.8, 140.8, 135.5, 135.0, 129.7, 128.1, 126.7, 125.7, 120.0, 110.3, 107.3, 102.5, 98.5, 55.67, 29.72. HRMS m/z (M+): calcd for C18H13NO4: 307.0845; found: 307.0843.
4-Chloro-12-methyl-6H-isochromeno[4,3-c]quinoline-6,11(12H)-dione (3l).
Yield: 84% (130 mg); white solid; mp: 260–262 °C. IR (ATR): 2881, 2025, 1656, 1529, 1445, 1400, 1312, 1235, 1140, 1032, 911 cm−1. 1H NMR (600 MHz, CDCl3): δ 9.55 (1H, d, J = 8.4 Hz), 8.37 (1H, dd, J = 7.8, 1.2 Hz), 7.85–7.82 (1H, m), 7.61–7.59 (1H, m), 7.46 (1H, t, J = 8.4 Hz), 7.34–7.31 (2H, m), 3.77 (3H, s). 13C NMR (150 MHz, CDCl3): δ 160.0, 159.3, 154.4, 141.0, 135.0, 134.3, 132.5, 131.5, 129.5, 129.1, 127.4, 126.8, 120.4, 113.2, 111.4, 105.8, 30.8. HRMS m/z (M+): calcd for C17H10ClNO3: 311.0349; found: 311.0398.
3-Chloro-12-methyl-6H-isochromeno[4,3-c]quinoline-6,11(12H)-dione (3m).
Yield: 85% (132 mg); white solid; mp: 250–252 °C. IR (ATR): 2881, 2025, 1656, 1529, 1445, 1400, 1312, 1235, 1140, 1032, 911 cm−1. 1H NMR (600 MHz, CDCl3): δ 9.40 (1H, dd, J = 8.4, 1.2 Hz), 8.36 (1H, dd, J = 7.8, 1.2 Hz), 8.21 (1H, d, J = 2.4 Hz), 7.84–7.81 (1H, m), 7.60–7.57 (1H, m), 7.55 (1H, dd, J = 9.4, 2.4 Hz), 7.30 (1H, d, J = 8.4 Hz), 3.73 (3H, s).13C NMR (150 MHz, CDCl3): δ 160.1, 159.6, 153.3, 137.3, 135.6, 134.6, 132.5, 129.9, 129.2, 128.6, 127.2, 123.3, 120.6, 115.7, 114.5, 105.6, 29.9. HRMS m/z (M+): calcd for C17H10ClNO3: 311.0349; found: 311.0347.
3-Bromo-12-methyl-6H-isochromeno[4,3-c]quinoline-6,11(12H)-dione (3n).
Yield: 83% (146 mg); white solid; mp: 278–280 °C. IR (ATR): 2933, 1739, 1543, 1470, 1329, 1238, 1151, 1105, 1024, 970 cm−1. 1H NMR (600 MHz, CDCl3): δ 9.51 (1H, d, J = 4.2 Hz), 8.39–8.36 (2H, m), 7.85 (1H, t, J = 3.6 Hz), 7.71–7.69 (1H, m), 7.60 (1H, t, J = 4.2 Hz), 7.25 (1H, t, J = 4.2 Hz), 3.74 (3H, s). 13C NMR (150 MHz, CDCl3): δ 160.2, 159.6, 153.3, 137.7, 135.7, 135.3, 134.2, 130.0, 129.2, 127.2, 126.3, 120.6, 115.9, 114.9, 105.6, 29.9. HRMS m/z (M+): calcd for C17H10BrNO3: 354.9844; found: 354.9846.
2-Chloro-12-methyl-6H-isochromeno[4,3-c]quinoline-6,11(12H)-dione (3o).
Yield: 79% (122 mg); white solid; mp: 268–270 °C. IR (ATR): 2878, 2120, 1702, 1652, 1510, 1434, 1348, 1321, 1212, 1121, 1037, 966, 827 cm−1. 1H NMR (600 MHz, CDCl3): δ 9.52 (1H, dd, J = 8.4, 1.2 Hz), 8.38 (1H, dd, J = 7.8, 1.2 Hz), 8.23 (1H, d, J = 8.4 Hz), 7.87–7.84 (1H, m), 7.61–7.58 (1H, m), 7.39 (1H, d, J = 1.2 Hz), 7.30 (1H, dd, J = 8.4, 1.2 Hz), 3.76 (3H, s).13C NMR (150 MHz, CDCl3): δ 160.5, 159.8, 154.2, 139.6, 138.9, 135.7, 134.4, 130.0, 129.0, 127.1, 125.4, 123.3, 120.5, 114.3, 112.1, 105.0, 29.9. HRMS m/z (M+): calcd for C17H10ClNO3: 311.0349; found: 311.0349.
9,12-Dimethyl-6H-isochromeno[4,3-c]quinoline-6,11(12H)-dione (4a).
Yield: 75% (109 mg); white solid; mp: 271–273 °C. IR (ATR): 2921, 2855, 1800, 1724, 1640, 1372, 1238, 1191, 1029, 907, 836 cm−1. 1H NMR (600 MHz, CDCl3): δ 9.37 (1H, s), 8.29 (1H, dd, J = 7.8, 1.2 Hz), 8.26 (1H, d, J = 7.8 Hz), 7.65–7.62 (1H, m), 7.38 (2H, d, J = 9.0 Hz), 7.33–7.30 (1H, m), 3.77 (3H, s), 2.53 (3H, s). 13C NMR (150 MHz, CDCl3): δ 160.7, 160.1, 154.7, 146.9, 138.8, 134.6, 132.4, 130.0, 129.9, 127.2, 124.1, 122.7, 118.1, 114.1, 113.6, 104.9, 29.7, 22.6. HRMS m/z (M+): calcd for C18H13NO3: 291.0895; found: 291.0898.
9-Methoxy-12-methyl-6H-isochromeno[4,3-c]quinoline-6,11(12H)-dione (4b).
Yield: 72% (110 mg); white solid; mp: 273–275 °C. IR (ATR): 2922, 2853, 1925, 1799, 1729, 1640, 1562, 1466, 1382, 1245, 1044, 975, 880 cm−1. 1H NMR (600 MHz, CDCl3): δ 9.14 (1H, d, J = 2.4 Hz), 8.32 (1H, dd, J = 8.4, 1.8 Hz), 8.27 (1H, d, J = 9.0 Hz), 7.67–7.64 (1H, m), 7.40 (1H, d, J = 7.8 Hz), 7.33 (1H, t, J = 7.8 Hz), 7.09 (1H, dd, J = 9.0 Hz), 3.99 (3H, s), 3.78 (3H, s). 13C NMR (150 MHz, CDCl3): δ 165.4, 160.8, 159.8, 155.3, 138.9, 137.1, 132.6, 131.8, 124.2, 122.8, 117.7, 114.2, 113.7, 113.4, 109.0, 104.8, 55.8, 29.7. HRMS m/z (M+): calcd for C18H13NO4: 307.0845; found: 307.0842.
2-Methyl-9-phenyl-6H-isochromeno[4,3-c]quinoline-6,11(12H)-dione (4c).
Yield: 78% (137 mg); white solid; mp: 263–265 °C. IR (ATR): 3062, 1757, 1656, 1599, 1386, 1253, 1180, 1030, 1018, 846 cm−1. 1H NMR (600 MHz, CDCl3): δ 9.89 (1H, d, J = 1.8 Hz), 8.42 (1H, d, J = 8.4 Hz), 8.33 (1H, dd, J = 8.4, 1.2 Hz), 7.82 (1H, dd, J = 8.4, 1.2 Hz), 7.77–7.75 (2H, m), 7.67–7.64 (1H, m), 7.50–7.47 (2H, m), 7.43–7.40 (2H, m), 7.34 (1H, t, J = 7.2 Hz), 3.80 (3H, s). 13C NMR (150 MHz, CDCl3): δ 160.7, 160.1, 154.9, 148.1, 139.6, 139.0, 135.1, 132.6, 130.4, 129.0, 128.7, 127.7, 127.5, 125.5, 124.1, 122.8, 119.1, 114.2, 113.6, 104.0, 29.8. HRMS m/z (M+): calcd for C23H15NO3: 353.1052; found: 353.1055.
9-Chloro-12-methyl-6H-isochromeno[4,3-c]quinoline-6,11(12H)-dione (4d).
Yield: 84% (130 mg); white solid; mp: 284–286 °C. IR (ATR): 3106, 2881, 2017, 1658, 1539, 1459, 1352, 1226, 1165, 1073, 1037, 909, 878 cm−1. 1H NMR (600 MHz, CDCl3): δ 9.67 (1H, d, J = 1.8 Hz), 8.34–8.31 (2H, m), 7.72–7.69 (1H, m), 7.55 (1H, dd, J = 8.4, 2.4 Hz), 7.44 (1H, d, J = 8.4 Hz), 7.38–7.35 (1H, m), 3.81 (3H, s). 13C NMR (150 MHz, CDCl3): δ 160.4, 159.5, 155.4, 142.8, 139.2, 136.0, 133.1, 131.3, 129.3, 127.0, 124.2, 122.9, 118.8, 114.3, 113.3, 104.0, 29.8. HRMS m/z (M+): calcd for C17H10ClNO3: 311.0349; found: 311.0347.
9-Bromo-12-methyl-6H-isochromeno[4,3-c]quinoline-6,11(12H)-dione (4e).
Yield: 86% (152 mg); white solid; mp: 276–278 °C. IR (ATR): 2926, 1737, 1638, 1577, 1553, 1379, 1309, 1466, 1238, 1115, 1038, 910 cm−1. 1H NMR (600 MHz, CDCl3): δ 9.84 (1H, d, J = 2.4 Hz), 8.33 (1H, dd, J = 8.4, 1.2 Hz), 8.22 (1H, d, J = 8.4 Hz), 7.72–7.68 (2H, m), 7.43 (1H, d, J = 8.4 Hz), 7.38–7.35 (1H, m), 3.80 (3H, s). 13C NMR (150 MHz, CDCl3): δ 160.3, 159.6, 155.3, 139.2, 135.9, 133.0, 132.2, 131.7, 131.2, 130.0, 124.2, 122.9, 119.2, 114.3, 113.3, 103.9, 29.8. HRMS m/z (M+): calcd for C17H10BrNO3: 354.9844; found: 354.9846.
9-Acetyl-12-methyl-6H-isochromeno[4,3-c]quinoline-6,11(12H)-dione (4f).
Yield: 74% (118 mg); white solid; mp: 290–292 °C. IR (ATR): 3118, 2923, 1761, 1743, 1732, 1642, 1584, 1560, 1417, 1382, 1252, 1121, 1058 cm−1. 1H NMR (600 MHz, CDCl3): δ 10.24 (1H, s), 8.46 (1H, d, J = 8.4 Hz), 8.34 (1H, d, J = 8.4 Hz), 8.14 (1H, dd, J = 8.4, 1.8 Hz), 7.71 (1H, t, J = 7.2 Hz), 7.46 (1H, d, J = 2.4 Hz), 7.39 (1H, t, J = 7.2 Hz), 3.83 (3H, s), 2.78 (3H, s). 13C NMR (150 MHz, CDCl3): δ 197.9, 160.5, 159.4, 155.9, 142.7, 139.1, 135.2, 133.0, 130.2, 127.8, 127.3, 124.2, 123.3, 123.0, 114.4, 113.3, 104.4, 29.8, 27.1. HRMS m/z (M+): calcd for C19H13NO4: 319.0845; found: 319.0844.
Methyl 12-methyl-6,11-dioxo-11,12-dihydro-6H-isochromeno[4,3-c]quinoline-9-carboxylate (4g).
Yield: 78% (130 mg); white solid; mp: 290–292 °C. IR (ATR): 3136, 2953, 1785, 1720, 1623, 1592, 1465, 1385, 1236, 1146, 1059 cm−1. 1H NMR (600 MHz, CDCl3): δ 10.25 (1H, s), 8.46 (1H, d, J = 7.8 Hz), 8.34 (1H, d, J = 7.8 Hz), 8.22 (1H, d, J = 8.4 Hz), 7.70 (1H, t, J = 9.0 Hz), 7.45 (1H, d, J = 8.4 Hz), 7.38 (1H, t, J = 7.8 Hz), 4.00 (3H, s), 3.83 (3H, s). 13C NMR (150 MHz, CDCl3): δ 166.1, 160.4, 159.5, 155.0, 139.1, 136.3, 134.8, 133.0, 130.0, 129.2, 128.4, 124.1, 123.4, 122.9, 114.3, 113.4, 104.5, 52.8, 29.8. HRMS m/z (M+): calcd for C19H13NO5: 335.0794; found: 335.0797.
12-Methyl-6,11-dioxo-11,12-dihydro-6H-isochromeno[4,3-c]quinoline-9-carbonitrile (4h).
Yield: 62% (93 mg); white solid; mp: 295–297 °C. IR (ATR): 3482, 3110, 2237, 1747, 1635, 1618, 1586, 1556, 1501, 1479, 1403, 1305, 1259, 1138, 1033 cm−1. 1H NMR (600 MHz, CDCl3): δ 10.03 (1H, s), 8.47 (1H, d, J = 8.4 Hz), 8.33 (1H, d, J = 7.8 Hz), 7.81 (1H, d, J = 8.4 Hz), 7.74 (1H, t, J = 7.8 Hz), 7.47 (1H, d, J = 8.4 Hz), 7.39 (1H, t, J = 7.8 Hz), 3.82 (3H, s). 13C NMR (150 MHz, CDCl3): δ 160.1, 158.7, 155.6, 139.4, 135.4, 133.5, 131.5, 131.0, 130.5, 124.2, 123.1, 119.1, 117.7, 114.5, 113.1, 110.1, 103.5, 29.9. HRMS m/z (M+): calcd for C18H10N2O3: 302.0691; found: 302.0689.
7-Bromo-12-methyl-6H-isochromeno[4,3-c]quinoline-6,11(12H)-dione (4j).
Yield: 76% (134 mg); white solid; mp: 276–278 °C. IR (ATR): 3124, 1733, 1650, 1629, 1524, 1463, 1282, 1239, 1100, 1020, 912 cm−1. 1H NMR (600 MHz, CDCl3): δ 9.74 (1H, dd, J = 8.4, 1.2 Hz), 8.33 (1H, dd, J = 8.4, 1.2 Hz), 7.89 (1H, t, J = 7.8, 0.6 Hz), 7.71–7.68 (1H, m), 7.62 (1H, t, J = 8.4 Hz), 7.42 (1H, d, J = 8.4 Hz), 7.37 (1H, t, J = 7.2 Hz), 3.80 (3H, s). 13C NMR (150 MHz, CDCl3): δ 160.3, 156.7, 154.9, 139.1, 137.8, 135.7, 135.2, 133.0, 126.4, 125.0, 124.2, 122.8, 118.9, 114.2, 113.1, 104.2, 29.9. HRMS m/z (M+): calcd for C17H10BrNO3: 354.9844; found: 354.9846.
7-Fluoro-12-methyl-6H-isochromeno[4,3-c]quinoline-6,11(12H)-dione (4k).
Yield: 74% (109 mg); white solid; mp: 276–278 °C. IR (ATR): 3391, 3186, 1980, 1747, 1640, 1564, 1474, 1366, 1258, 1114, 1026 cm−1. 1H NMR (600 MHz, CDCl3): δ 9.74 (1H, dd, J = 8.4, 1.2 Hz), 8.33 (1H, dd, J = 8.4, 1.2 Hz), 7.89 (1H, t, J = 7.8, 0.6 Hz), 7.71–7.68 (1H, m), 7.62 (1H, t, J = 8.4 Hz), 7.42 (1H, d, J = 8.4 Hz), 7.37 (1H, t, J = 7.2 Hz), 3.80 (3H, s). 13C NMR (150 MHz, CDCl3): δ 163.9, 162.1, 160.3, 155.3 (d, J = 21 Hz), 139.1, 136.9 (d, J = 42 Hz), 136.8, 133.2, (d, J = 249 Hz), 132.9, 124.2, 122.7 (d, J = 6 Hz), 116.2 (d, J = 21 Hz), 114.2, 113.1, 109.4, 104.0, 29.8. HRMS m/z (M+): calcd for C17H10FNO3: 295.0645; found: 295.0645.
8,9-Dichloro-12-methyl-6H-isochromeno[4,3-c]quinoline-6,11(12H)-dione (4l).
Yield: 84% (144 mg); white solid; mp: 277–279 °C. IR (ATR): 2848, 1853, 1677, 1490, 1433, 1330, 1285, 1192, 1145, 1069, 984, 814 cm−1. 1H NMR (600 MHz, CDCl3): δ 9.75 (1H, s), 8.40 (1H, s), 8.28 (1H, dd, J = 7.8, 1.2 Hz), 7.71–7.68 (1H, m), 7.42 (1H, d, J = 8.4 Hz), 7.36 (1H, t, J = 7.2 Hz), 3.78 (3H, s). 13C NMR (150 MHz, CDCl3): δ 160.1, 158.4, 155.3, 141.0, 139.2, 133.8, 133.3, 133.2, 131.1, 129.1, 124.2, 123.0, 120.0, 114.4, 113.2, 103.5, 29.8. HRMS m/z (M+): calcd for C17H9Cl2NO3: 344.9959; found: 344.9962.
14-Methyl-6H-benzo[6,7]isochromeno[4,3-c]quinoline-6,13(14H)-dione (5).
Yield: 76% (124 mg); white solid; mp: 265–267 °C. IR (ATR): 2874, 1840, 1641, 1616, 1452, 1393, 1227, 1180, 1052, 952 cm−1. 1H NMR (600 MHz, CDCl3): δ 9.99 (1H, s), 8.92 (1H, s), 8.28 (1H, dd, J = 7.8, 1.8 Hz), 8.01 (1H, d, J = 8.4 Hz), 7.96 (1H, d, J = 8.4 Hz), 7.64–7.59 (2H, m), 7.55 (1H, t, J = 7.2 Hz), 7.35 (1H, d, J = 8.4 Hz), 7.30 (1H, t, J = 7.2 Hz), 3.77 (3H, s). 13C NMR (150 MHz, CDCl3): δ 160.7, 160.4, 153.5, 138.7, 136.8, 132.3, 132.5, 131.9, 129.5, 129.3, 129.1, 128.5, 127.4, 127.0, 123.9, 122.6, 118.5, 114.1, 113.6, 105.0, 29.7. HRMS m/z (M+): calcd for C21H13NO3: 327.0895; found: 327.0897.
14-Benzyl-6H-benzo[6,7]isochromeno[4,3-c]quinoline-6,13(14H)-dione (6).
Yield: 71% (143 mg); white solid; mp: 116–118 °C. IR (ATR): 2880, 1839, 1657, 1600, 1493, 1352, 1256, 1127, 1070, 963 cm−1. 1H NMR (600 MHz, CDCl3): δ 10.04 (1H, s), 8.95 (1H, s), 8.29 (1H, d, J = 7.2 Hz), 7.98–7.94 (2H, m), 7.59 (1H, d, J = 7.2 Hz), 7.52 (1H, t, J = 6.6 Hz), 7.46 (1H, t, J = 7.8 Hz), 7.20–7.24 (4H, m), 7.19–7.17 (3H, m), 5.62 (2H, s). 13C NMR (150 MHz, CDCl3): δ 161.0, 160.5, 154.0, 138.4, 136.9, 136.3, 132.4, 132.3, 132.1, 129.6, 129.3, 129.2, 129.0, 128.5, 127.5, 127.4, 127.3, 126.4, 124.1, 122.9, 118.5, 115.0, 114.0, 105.1, 46.4. HRMS m/z (M+): calcd for C27H17NO3: 403.1208; found: 403.1206.
6-Methyl-5H-pyrido[3′,4′:4,5]pyrano[3,2-c]quinoline-5,12(6H)-dione (7).
Yield: 48% (66 mg); brown solid; mp: 270–272 °C. IR (ATR): 2917, 1746, 1639, 1462, 1352, 1254, 1104, 1044, 973, 843 cm−1. 1H NMR (600 MHz, CDCl3): δ 10.84 (1H, s), 8.90 (1H, d, J = 1.2 Hz), 8.27 (1H, d, J = 7.8 Hz), 8.13 (1H, d, J = 3.6 Hz), 7.70–7.68 (1H, m), 7.42 (1H, d, J = 9.0 Hz), 7.35 (1H, t, J = 7.8 Hz), 3.80 (3H, s). 13C NMR (150 MHz, CDCl3): δ 159.8, 158.5, 155.6, 149.2, 147.9, 139.5, 133.5, 126.8, 124.1, 123.1, 121.7, 114.5, 113.1, 103.2, 29.9. HRMS m/z (M+): calcd for C16H10N2O3: 278.0691; found: 278.0694.
8-Chloro-6-methyl-5H-pyrido[3′,4′:4,5]pyrano[3,2-c]quinoline-5,12(6H)-dione (8).
Yield: 58% (90 mg); brown solid; mp: 289–291 °C. IR (ATR): 3080, 1765, 1599, 1540, 1486, 1445, 1397, 1251, 1125, 940 cm−1. 1H NMR (600 MHz, CDCl3): δ 10.81 (1H, s), 8.90 (1H, d, J = 2.7 Hz), 8.14 (1H, d, J = 2.4 Hz), 7.52 (1H, t, J = 4.2 Hz), 7.37–7.35 (2H, m), 3.80 (3H, s). 13C NMR (150 MHz, CDCl3): δ 159.2, 157.8, 155.2, 150.1, 148.9, 141.4, 132.6, 132.3, 128.5, 127.1, 126.0, 120.8, 113.5, 111.0, 104.3, 30.8. HRMS m/z (M+): calcd for C16H9ClN2O3: 312.0302; found: 312.0289.
5-Methyl-4H-thieno[3′,2′:4,5]pyrano[3,2-c]quinoline-4,11(5H)-dione (9).
Yield: 62% (87 mg); brown solid; mp: 266–268 °C. IR (ATR): 2924, 2857, 1789, 1657, 1602, 1525, 1428, 1389, 1384, 1339, 1120, 1093, 988 cm−1. 1H NMR (600 MHz, CDCl3): δ 8.52 (1H, d, J = 4.8 Hz), 8.32 (1H, d, J = 8.4 Hz), 7.96 (1H, d, J = 4.8 Hz), 7.66 (1H, t, J = 7.8 Hz), 7.42 (1H, d, J = 8.4 Hz), 7.35 (1H, t, J = 7.8 Hz), 3.79 (3H, m). 13C NMR (150 MHz, CDCl3): δ 159.6, 156.1, 155.8, 144.4, 138.9, 137.4, 132.5, 127.2, 124.1, 123.6, 123.0, 114.5, 113.7, 105.8, 29.7. HRMS m/z (M+): calcd for C15H9NO3S: 283.0303; found: 283.0300.
8-Isopropyl-5-methyl-4H-thieno[3′,2′:4,5]pyrano[3,2-c]quinoline-4,11(5H)-dione (10).
Yield: 63% (102 mg); white solid; mp: 255–257 °C. IR (ATR): 2961, 1730, 1643, 1582, 1422, 1375, 1244, 1037, 960 cm−1. 1H NMR (600 MHz, CDCl3): δ 8.53 (1H, d, J = 5.4 Hz), 8.15 (1H, d, J = 1.8 Hz), 7.96 (1H, d, J = 5.4 Hz), 7.55 (1H, dd, J = 8.4, 1.8 Hz), 7.35 (1H, d, J = 8.4 Hz), 3.77 (3H, m), 3.08–3.01 (1H, m), 1.32 (6H, d, J = 7.2 Hz). 13C NMR (150 MHz, CDCl3): δ 159.5, 156.3, 155.9, 144.6, 143.8, 137.3, 131.4, 127.2, 123.6, 121.2, 114.5, 113.6, 105.7, 33.7, 29.7, 24.0. HRMS m/z (M+): calcd for C18H15NO3S: 325.0773; found: 325.0771.
2,13-Dimethyl-2,13-dihydroindolo[2′,3′:4,5]pyrano[3,2-c]quinoline-1,8-dione (11).
Yield: 62% (102 mg); white solid; mp: 296–298 °C. IR (ATR): 2951, 1728, 1644, 1611, 1588, 1557, 1522, 1498, 1458, 1386, 1307, 1276, 1130, 1012 cm−1. 1H NMR (600 MHz, CDCl3): δ 8.44 (1H, d, J = 8.4 Hz), 8.31 (1H, d, J = 7.8 Hz), 7.67 (1H, t, J = 7.2 Hz), 7.53 (1H, d, J = 8.4 Hz), 7.46 (1H, t, J = 7.2 Hz), 7.42–7.38 (2H, m), 7.36 (1H, t, J = 7.8 Hz), 4.34 (3H, s), 3.84 (3H, s). 13C NMR (150 MHz, CDCl3): δ 159.0, 157.2, 157.0, 142.5, 141.0, 139.2, 132.7, 125.3, 124.9, 124.2, 123.2, 122.9, 121.2, 114.4, 114.21, 110.6, 103.0, 102.3, 36.4, 30.0. HRMS m/z (M+): calcd for C20H14N2O3: 330.1004; found: 330.1005.
Fluorescence sensing of compound 4a on Fe3+.
0.50 mL of compound 4a (10 μM) in 1,4-dioxane was taken in a 4 mL quartz cuvette as a fluorescent probe and was titrated with 0.50 mL of various metal ions (100 μM) in water for selectivity studies. Further, the fluorescence sensitivity of compound 4a (10 μM) was studied towards different concentrations of Fe3+ in water. All the fluorescence (Hitachi-7000 F) measurements were carried out after 5 min of incubation at room temperature at 350 nm (excitation wavelength) with 5 nm of slit width, 250 V of photomultiplier tube voltage and a scan speed of 240 nm min−1.
Conflicts of interest
There are no conflicts to declare.
Acknowledgements
This work was supported by the National Research Foundation of Korea (NRF) grant funded by the Korean government (MSIT) (2018R1A2B2004432), the Priority Research Centers Program (2014R1A6A1031189), and the Korean Ministry of Education, Science and Technology (2012M3A7B4049675).
Notes and references
-
(a) X. Luo, X. Lin, L. Salendra, X. Pang, Y. Dai, B. Yang, J. Liu, J. Wang, X. Zhou and Y. Liu, Mar. Drugs, 2017, 15, 204 CrossRef;
(b) A. R. Carroll, B. R. Copp, R. A. Davis, R. A. Keyzers and M. R. Prinsep, Nat. Prod. Rep., 2019, 36, 122–173 RSC;
(c) T. El-Elimat, H. A. Raja, M. Figueroa, J. O. Falkinham III and N. H. Oberlies, Phytochemistry, 2014, 104, 114–120 CrossRef CAS.
-
(a) T. Shiro, T. Fukaya and M. Tobe, Eur. J. Med. Chem., 2015, 97, 397–408 CrossRef CAS;
(b) I. Jarak, M. Kralj, L. Suman, G. Pavlovic, J. Dogan, I. Piantanida, M. Zinic, K. Pavelic and G. Karminski-Zamola, J. Med. Chem., 2005, 48, 2346–2360 CrossRef CAS.
-
(a) Y. Ikuma, H. Hochigai, H. Kimura, N. Nunami, T. Kobayashi, K. Uchiyama, Y. Furuta, M. Sakai, M. Horiguchi, Y. Masui, K. Okazaki, Y. Sato and H. Nakahira, Bioorg. Med. Chem., 2012, 20, 5864–5883 CrossRef CAS;
(b) P. Pahari, M. K. Kharel, M. D. Shepherd, S. G. van Lanen and J. Rohr, Angew. Chem., 2012, 124, 1242–1246 (
Angew. Chem., Int. Ed.
, 2012
, 51
, 1216–1220
) CrossRef.
-
(a) B. D. Patel and M. D. Ghate, J. Med. Chem., 2014, 74, 574–605 CrossRef CAS PubMed;
(b) E. J. Jung, B. H. Park and Y. R. Lee, Green Chem., 2010, 12, 2003–2011 RSC.
-
(a) D.-S. Lim, Y.-S. Kwak, J.-H. Kim, S.-H. Ko, W.-H. Yoon and C. H. Kim, Chemotherapy, 2003, 49, 146–153 CrossRef CAS;
(b) A. Matsumoto and P. C. Hanawalt, Cancer Res., 2000, 60, 3921–3936 CAS;
(c) T. Kawano, N. Agata, S. Kharbanda, D. Avigan and D. Kufe, Cancer Chemother. Pharmacol., 2007, 59, 329–335 CrossRef CAS.
-
(a) V. Duraipandiyan and S. Ignacimuthu, J. Ethnopharmacol., 2009, 123, 494–498 CrossRef CAS;
(b) I. S. Chen, S. J. Wu and I. L. Tsai, J. Nat. Prod., 1994, 57, 1206–1211 CrossRef CAS;
(c) S. H. Huang, M. Xiong, X. P. Chen, Z. Y. Xiao, Y. F. Zhao and Z. Y. Huang, Oncol. Rep., 2008, 20, 567–572 CAS.
- Y. Kajita, T. Kurahashi and S. Matsubara, J. Am. Chem. Soc., 2008, 130, 17226–17227 CrossRef CAS.
-
(a) Y. Li, Q. Wang, X. Yang, F. Xie and X. Li, Org. Lett., 2017, 19, 3410–3413 CrossRef CAS;
(b) R. Chen and S. Cui, Org. Lett., 2017, 19, 4002–4005 CrossRef CAS.
- H.-Y. Dang, Z.-T. Wang and Y. Cheng, Org. Lett., 2014, 16, 5520–5523 CrossRef CAS.
- M.-J. Luo, M. Hu, R.-J. Song, D.-L. He and J.-H. Li, Chem. Commun., 2019, 55, 1124–1127 RSC.
- X. Li, X. Li and N. Jiao, J. Am. Chem. Soc., 2015, 137, 9246–9249 CrossRef CAS.
- R. Zeng and G. Dong, J. Am. Chem. Soc., 2015, 137, 1408–1411 CrossRef CAS.
- R. Manikandan and M. Jeganmohan, Org. Lett., 2014, 16, 3571 Search PubMed.
- J. Wu, S. Xiang, J. Zeng, M. Leow and X.-W. Liu, Org. Lett., 2015, 17, 222–225 CrossRef CAS.
- Y. Moon, E. Jang, S. Choi and S. Hong, Org. Lett., 2018, 20, 240–243 CrossRef CAS.
- Y. Wu, P. Sun, K. Zhang, T. Yang, H. Yao and A. Lin, J. Org. Chem., 2016, 81, 2166–2173 CrossRef CAS.
- D. Ding, G. Zhu and X. Jiang, Angew. Chem., 2018, 130, 9166–9170 (
Angew. Chem., Int. Ed.
, 2018
, 57
, 9028–9032
) CrossRef.
-
(a) G. E. Park, S. M. B. Maezono, J. H. Ha, J.-J. Shim, S. H. Kim and Y. R. Lee, Adv. Synth. Catal., 2018, 360, 4523–4532 CrossRef CAS;
(b) R. S. Thombal, S.-T. Kim, M.-H. Baik and Y. R. Lee, Chem. Commun., 2019, 55, 2940–2943 RSC;
(c) R. P. Pandit and Y. R. Lee, Adv. Synth. Catal., 2015, 357, 2657–2664 CrossRef CAS;
(d) R. P. Pandit, S. H. Kim and Y. R. Lee, Adv. Synth. Catal., 2016, 358, 3586–3599 CrossRef CAS.
-
(a) J. A. Brown, S. Irvine, A. R. Kennedy, W. J. Kerr, S. Andersson and G. N. Nilsson, Chem. Commun., 2008, 1115–1117 RSC;
(b) E. M. Simmons and J. F. Hartwig, Angew. Chem., 2012, 124, 3120–3126 (
Angew. Chem., Int. Ed.
, 2012
, 51
, 3066–3072
) CrossRef.
- H. Wang and F. Glorius, Angew. Chem., 2012, 124, 7430–7434 (
Angew. Chem., Int. Ed.
, 2012
, 51
, 7318–7322
) CrossRef.
- L. Pan, J. Dong, D. Xie, Y. Li and Q. Liu, Adv. Synth. Catal., 2018, 360, 958–964 CrossRef CAS.
-
(a) B. N. G. Giepmans, S. R. Adams, M. H. Ellisman and R. Y. Tsien, Science, 2006, 312, 217–224 CrossRef CAS PubMed;
(b) A. Baker, Environ. Sci. Technol., 2001, 35, 948–953 CrossRef CAS.
Footnotes |
† Electronic supplementary information (ESI) available: NMR spectra of all products and X-ray crystallographic structure data for 3j. CCDC 1878962. For ESI and crystallographic data in CIF or other electronic format see DOI: 10.1039/c9ra03146d |
‡ R. S. and H. D. K. contributed equally to this work. |
|
This journal is © The Royal Society of Chemistry 2019 |
Click here to see how this site uses Cookies. View our privacy policy here.