DOI:
10.1039/C7RA03822D
(Paper)
RSC Adv., 2017,
7, 34000-34004
1H and 19F NMR in drug stress testing: the case of voriconazole†
Received
3rd April 2017
, Accepted 26th June 2017
First published on 5th July 2017
Abstract
Stress tests form an important part of drug development, and of subsequent accreditation. Commonly the change in chemical composition over time is determined using chromatographic methods that separate the mixture components, but when structural characterization of the degradation products is required, other methods are needed. Arguably the most powerful method for determining chemical structure information is NMR spectroscopy. Here we show that NMR alone, using a combination of simple 19F, 1H, and DOSY experiments, can characterise both structure and kinetics of an intact reaction mixture, without the need for any physical separation.
Introduction
During the development of a drug, and certainly before its release to the market, it is important to have information about its stability under various conditions, since this affects both its safety and its efficacy.1 This information is commonly found by stress testing (forced degradation), i.e. subjecting the drug to harsh conditions to infer information about its long-term stability. Typical examples in solution include acid, alkaline and oxidative conditions, as well as high temperature.1 Monitoring the reaction time course under such conditions provides kinetic and structural information on the reagents, intermediates, and final products, improving the understanding of reaction mechanisms and kinetic properties.2 Common spectroscopic methods used for this purpose include mid-infrared (MIR),3 Raman,4 near-infrared (NIR),5 mass (MS)2,6 and nuclear magnetic resonance (NMR)7–12 spectroscopies.
The choice of technique depends on the target reaction and on what information it is required. NMR has some distinct advantages over other techniques, since it provides very detailed information about chemical structure, and it is non-destructive. However, it also has the disadvantage of low sensitivity, and it is far from ideal for mixtures, because of the difficulty, common to many spectroscopic methods, of assigning signals to individual species. Diffusion-ordered spectroscopy (DOSY),13,14 in which the signals from mixture components are differentiated according to the diffusion behaviour of the species involved, has been shown to be effective in the analysis of drug mixtures.15–17 An alternative approach is to use HPLC-NMR, but this involves physical separation and often requires lengthy chromatographic optimization. DOSY, in contrast, keeps the sample intact, so that interactions can be studied, and sample recovery is trivial; it can be performed on standard NMR equipment, present in most chemistry departments and pharmaceutical industry laboratories worldwide. NMR can also provide specific information about different nuclei and isotopes, which is a great advantage for fluorinated drugs. A large proportion of modern drugs, that has steadily increased over the last decade,18,19 contain at least one fluorine atom. 19F is an excellent probe in NMR, because of its strong signal, high sensitivity to changes in chemical environment, and excellent spectral resolution due to its wide chemical shift range.
In a previous study using HPLC, only one of the voriconazole degradation products could be characterized, while the second degradation product was reported to be an unstable component that degrades into multiple species, including the degradation product already identified, during the solvent evaporation step.20 In another study Ahmed and Abdalla described the development of an HPLC methodology to isolate voriconazole and its degradation products; apart from being time-consuming to develop and validate, at the end of the process none of the products identified by the method were structurally characterized.21 Such characterization is today a regulatory requirement, since more importance is attached to the structures and to the potential toxicity of degradation products.
NMR is extensively used in pharmaceutical analysis, in all stages of the development and production of active pharmaceutical ingredients. This includes the identification, characterization, and quantification of species that arise during the manufacturing process or are generated during storage, and quality assessment of drug formulations.12,15–17,22 This article illustrates a less common application, the use of 1H and 19F NMR for stress testing. An alkaline stress test of the fluorinated drug voriconazole is performed entirely inside a standard NMR tube, without the need for separation. The two main degradation products are characterized, one of them for the first time, and a complete kinetic profile is produced.
Experimental section
Full 1H, 13C and 19F spectral assignments of voriconazole (Fig. 1) were performed, using 1D and 2D NMR experiments, prior to degradation tests (see Fig. S2–S12 in ESI†). 1H and 13C assignments were in agreement with those previously reported;19,23,24 unequivocal coupling constant assignments and 19F NMR assignments are given here for the first time.
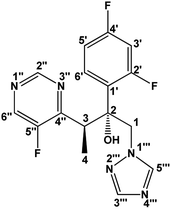 |
| Fig. 1 The structure of voriconazole, C16H14F3N5O, molar mass of 349.12 g mol−1. 1H, 13C, and 19F assignments are given below. | |
Voriconazole assignments
1H NMR (600.17 MHz, CD3OH): δ (ppm) 9.00 (1H, d, 5JH2′′F5′′ = 2.40 Hz, H2′′); 8.75 (1H, d, 3JH6′′F5′′ = 1.86 Hz, H6′′); 8.27 (1H, s, H5′′′); 7.59 (1H, s, H3′′′); 7.49 (1H, td, 3JH6′H5′ = 9.01, 4JH6′F2′ = 9.01 and 4JH6′F4′ = 6.67 Hz, H6′); 6.97 (1H, ddd, 3JH3′F2′ = 12.12, 3JH3′F4′ = 8.82 and 4JH3′H5′ = 2.52 Hz, H3′); 6.86 (1H, td, 3JH5′F4′ = 8.04 Hz, H5′); 6.39 (1H, s, OH); ∼4.85* (1H, d, H1); 4.36 (1H, d, 2JH1H1 = 14.28 Hz, H1); 4.16 (1H, q, 3JH3H4 = 6.84 Hz, H3) and 1.14 (3H, d, H4). 13C NMR (125.69 MHz, CD3OH): δ (ppm) 164.36 (1C, dd, 1JC4′F4′ = 248.2 and 3JC4′F2′ = 12.6 Hz, C4′); 160.44 (1C, dd, 1JC2′F2′ = 246.5 and 3JC2′F4′ = 11.9 Hz, C2′); 160.04 (1C, d, 2JC4′′F5′′ = 12.6 Hz, C4′′); 157.74 (1C, d, 1JC5′′F5′′ = 264.1 Hz, C5′′); 154.89 (1C, d, 4JC2′′F5′′ = 7.7 Hz, C2′′); 151.02 (1C, s, C3′′′); 146.58 (1C, d, 2JC6′′F5′′ = 22.7 Hz, C6′′); 145.90 (1C, s, C5′′′); 131.80 (1C, dd, 3JC6′F2′/4′ = 9.23 and 3JC6′F2′/4′ = 5.88 Hz, C6′); 125.36 (1C, dd, 2JC1′F2′ = 12.29 and 4JC1′F4′ = 3.75 Hz, C1′); 112.14 (1C, dd, 2JC5′F4′ = 20.88 and 4JC5′F2′ = 2.59 Hz, C5′); 105.02 (1C, t, 2JC3′F2′/4′ = 27.26 Hz, C3′); 78.70 (1C, d, 3JC2F2′ = 4.92 Hz, C2); 58.08 (1C, d, 4JC1F2′ = 4.86 Hz, C1); 39.11 (1C, d, 3JC3F5′′ = 4.95 Hz, C3) and 15.51 (1C, s, C4). 19F{1H} NMR (470.35 MHz, CD3OH): δ (ppm) −137.30 (1F, s, F5′′); −112.92 (1F, d, 4JF4′F2′ = 8.3 Hz, F4′) and −109.45 (1F, d, F2′). *Assigned from 1H–13C HSQC.
Voriconazole degradation
A European Pharmacopoeia Reference Standard of voriconazole was purchased from Sigma-Aldrich. The alkaline stress test was performed in methanol-d3, because of the good voriconazole solubility in, and the solvent stability to, alkali. Methanol-d3 was preferred to methanol-d4 in order to avoid losing exchangeable hydrogens (OH and NH in this case). There is no consensus in the literature as to what concentration of alkali to use for such a stress test;1,25,26 here voriconazole and NaOH were used in the proportion 1
:
2 (24 mM
:
48 mM) in methanol-d3 at 10 °C. The alkali concentration most commonly used in stress testing is 0.1 M,1 under such conditions voriconazole degrades very rapidly, so a slightly lower concentration was used here in order to obtain a convenient degradation rate with sharp 19F NMR signals. Acid and thermal stress tests were also performed, but no degradation was seen in the first, and for the thermal degradation the kinetics differed between different batches of DMSO-d6 and voriconazole (see ESI†). All NMR experiments were performed using a Bruker AVANCE III spectrometer operating at a 500 MHz 1H frequency, equipped with a 5 mm BBO probe; full experimental data and all experimental parameters can be downloaded from DOI: 10.17632/9pc5pyrp7c.1. In order to acquire quantitative NMR data, the recovery delay (d1) was set to 20 s, 5 times the largest T1 measured for the compounds of interest.
1H DOSY with solvent suppression
1H DOSY data were acquired using the Oneshot45 experiment27,28 (Bruker code is available via the following link: http://nmr.chemistry.manchester.ac.uk/?q=node/264) with presaturation of the hydroxyl residual proton signal of methanol-d3. The diffusion experiment was performed using a sample in a 3 mm NMR tube,29,30 to minimize convection, with a net diffusion-encoding gradient pulse width (δ) of 2.4 ms, a diffusion delay (Δ) of 60 ms, and sixteen nominal gradient amplitudes ranging from 4.8 to 38.5 G cm−1. The delay for gradient recovery (d16) and duration of the gradient purge pulse (p19) were fixed at 0.2 and 0.6 ms, respectively. The experiments were carried out at a nominal probe temperature of 10 °C, and the nominal power level used for presaturation was 84 μW. 19F DOSY data were acquired using the Oneshot pulse sequence,13 with the same parameters as for the 1H DOSY spectrum. The processing of DOSY experiments was carried out using the free DOSY Toolbox.31 1H DOSY processing used a 32k data table, phase correction, baseline correction, and reference deconvolution with a 3 Hz wide Lorentzian target lineshape. 19F DOSY processing was identical except for a 10 Hz Lorentzian target lineshape.
Results and discussion: voriconazole alkaline degradation
Voriconazole is relatively sensitive to alkali, leading to rapid degradation at room temperature, so the study was performed at 10 °C to allow appropriate time resolution for the reaction kinetics. From the 1H NMR spectra, it was clear that virtually all voriconazole had been degraded after 17 h as only signals from degradation products, one of which showed some rather broad signals (Fig. 2), were seen. The 19F NMR spectra (Fig. 3) showed clearly that at least three new signals related to the major degradation product(s) appeared.
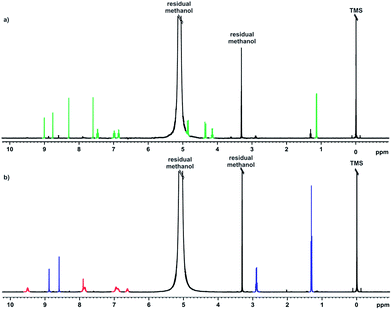 |
| Fig. 2 1H NMR spectra of alkaline stress testing; (a) time 0, and (b) after 17 h. Signals shown in green are from voriconazole, in red from degradation product 1, and in blue from degradation product 2, both here and in subsequent figures. | |
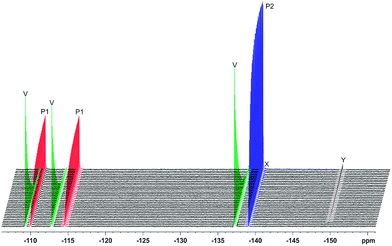 |
| Fig. 3 19F NMR spectra during a 17 h alkaline stress test, showing the consumption of voriconazole (V), the formation of major degradation products 1 (P1) and 2 (P2), and minor degradation products X and Y. Spectra are shown at half hour intervals. | |
The 1H and 19F DOSY experiments recorded at the end of the stress test (Fig. 4 and 5) showed that two main degradation products were generated, and that both are fluorinated. The agreement of diffusion coefficients between the experiments makes it easy to correlate the proton and fluorine spectra for each component. Degradation product 1 shows a lower diffusion coefficient (i.e. product 1 is a larger compound) and has two fluorine resonances, while degradation product 2 is smaller and has a single fluorine resonance.
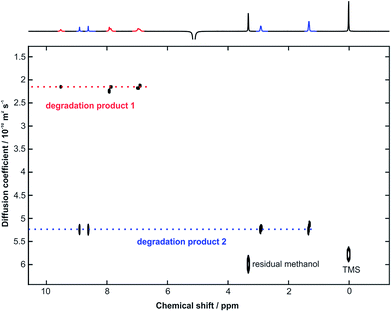 |
| Fig. 4 500 MHz 1H DOSY spectrum, with the least attenuated 1D spectrum shown at the top, acquired after 17 h of monitoring of a sample containing 24 mM voriconazole, and 48 mM NaOH in CD3OH at 10 °C. | |
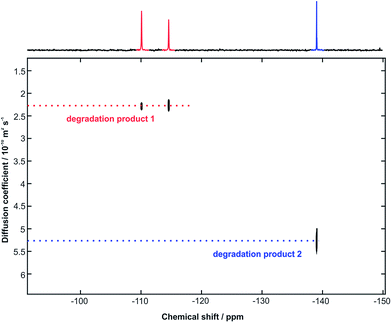 |
| Fig. 5 470 MHz 19F DOSY spectrum, with the least attenuated 1D spectrum shown at the top, acquired immediately following the 1H DOSY experiment of Fig. 4. | |
The alkaline stress testing showed two main degradation products. However, expansion of the 19F NMR spectrum (Fig. 6) revealed the presence of two minor products (X and Y). Degradation product 1 is in exchange between keto and enol tautomers, with the latter form favoured under alkaline conditions. This explains the broad extra signals observed in the 19F spectrum, which arise from the disfavoured keto form.
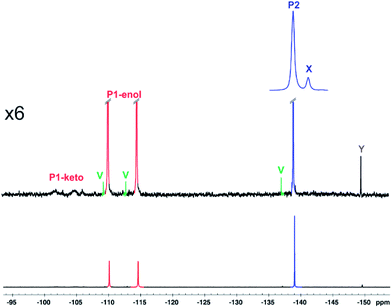 |
| Fig. 6 Expansion of 19F NMR spectrum acquired after 24 h with the same sample as Fig. 4. | |
19F NMR spectra were acquired every 10 min during the reaction time course, and the signal integrals versus reaction time course were fitted (Fig. 7) to the first order kinetic scheme of Fig. 8. The voriconazole degrades into products 1 and 2 with a rate constant of 0.27 h−1; product 1 is present in keto and enol forms that are in an equilibrium that is slow on the chemical shift timescale but fast on the timescale of reaction (kketo→enol ∼ 70 s−1), while product 2 decays further to two minor unidentified degradation products, X and Y with first order rate constants of 0.0085 and 0.0028 h−1, respectively (Fig. 8). Confirmation of the structures of products 1 and 2 is provided in the ESI.†
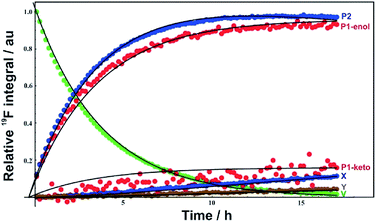 |
| Fig. 7 Voriconazole degradation profile under alkaline conditions, showing the relative concentrations of voriconazole (V), major degradation products 1 (P1) and 2 (P2), and two minor degradation products (X), (Y). Points show experimental measurements, solid lines the result of fitting to the kinetic scheme shown in Fig. 8. | |
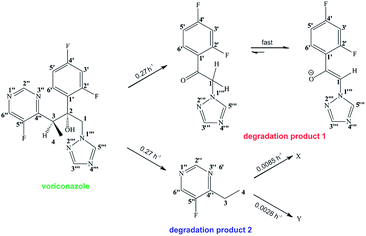 |
| Fig. 8 Proposed pathway for alkaline degradation of voriconazole. | |
Conclusion
The main purpose of this article is to illustrate the utility of NMR as a tool in monitoring drug degradation without the need for physical separation. The advantages of NMR are particularly marked for fluorinated drugs, since 19F shows excellent spectral resolution because of the sensitivity of its chemical shift to local environment. 1H and 19F DOSY spectra are helpful in providing information on the number and nature of compounds involved in the degradation process. Here a detailed analysis of the reaction time course as followed by 19F NMR revealed the presence of minor degradation products, and allowed us to identify and quantitate a pathway for the alkaline degradation of voriconazole that offers very good agreement with experimental data. The identities and assignments of the major degradation products were determined as described in the ESI,† with the structure of degradation product 2 being reported for the first time.
Acknowledgements
The authors gratefully acknowledge grants #2015/08541-6 and #2014/25903-6, São Paulo Research Foundation (FAPESP), for providing financial support for this research, and for T. M. B. scholarships (#2014/12776-6 and BEPE, #2015/19229-3); the CNPq for fellowships to R.R. and C. F. T.; CAPES (PEV project #88881.030355/2013–01), the Institute of Chemistry of UNICAMP, and the University of Manchester for NMR facilities; and the EPSRC (grant number EP/K039547).
Notes and references
- M. Blessy, R. D. Patel, P. N. Prajapati and Y. K. Agrawal, J. Pharm. Anal., 2014, 4, 159 CrossRef CAS.
- K. M. Roscioli, X. Zhang, S. X. Li, G. H. Goetz, G. Cheng, Z. Zhang, W. F. Siems and H. H. Hill Jr, Int. J. Mass Spectrom., 2013, 336, 27 CrossRef CAS.
- M. T. Drexler, D. A. Foley, H. W. Ward and H. J. Clarke, Org. Process Res. Dev., 2015, 19, 1119 CrossRef CAS.
- I. M. Clegg, J. Pearce and S. T. Content, Appl. Spectrosc., 2012, 66, 151 CrossRef CAS PubMed.
- S. M. de Lima, B. F. A. Silva, D. V. Pontes, C. F. Pereira, L. Stragevitch and M. F. Pimentel, Fuel, 2014, 115, 46 CrossRef.
- Q. Fu, J. Tang, M. Cui, Z. Zheng, Z. Liu and S. Liu, J. Chromatogr. B: Anal. Technol. Biomed. Life Sci., 2015, 990, 169 CrossRef CAS PubMed.
- D. A. Foley, E. Bez, A. Codina, K. L. Colson, M. Fey, R. Krull, D. Piroli, M. T. Zell and B. L. Marquez, Anal. Chem., 2014, 86, 12008 CrossRef CAS PubMed.
- M. A. Bernstein, Magn. Reson. Chem., 2016, 54, 422 CrossRef PubMed.
- N. M. Do, M. A. Olivier, J. J. Salisbury and C. B. Wager, Anal. Chem., 2011, 83, 8766 CrossRef CAS PubMed.
- I. M. Clegg, C. M. Gordon, D. S. Smith, R. Alzaga and A. Codina, Anal. Methods, 2012, 4, 1498 RSC.
- D. A. Foley, J. Wang, B. Maranzano, M. T. Zell, B. L. Marquez, Y. Xiang and G. L. Reid, Anal. Chem., 2013, 85, 8928 CrossRef CAS PubMed.
- R. M. Maggio, N. L. Calvo, S. E. Vignaduzzo and T. S. Kaufman, J. Pharm. Biomed. Anal., 2014, 101, 102 CrossRef CAS PubMed.
- G. A. Morris, Diffusion-Ordered Spectroscopy (DOSY), in Encyclopedia of Magnetic Resonance, ed. R. K. Harris and R. E. Wasylishen, Wiley, Chichester, 2009, DOI:10.1002/9780470034590.emrstm0119.pub2.
- G. Dal Poggetto, D. C. Favaro, M. Nilsson, G. A. Morris and C. F. Tormena, Magn. Reson. Chem., 2014, 52, 172 CrossRef CAS PubMed.
- S. Trefi, V. Gilard, M. Malet-Martino and R. Martino, J. Pharm. Biomed. Anal., 2007, 44, 743 CrossRef CAS PubMed.
- S. Trefi, V. Gilard, S. Balayssac, M. Malet-Martino and R. Martino, J. Pharm. Biomed. Anal., 2008, 46, 707 CrossRef CAS PubMed.
- U. Holzgrabe and M. Malet-Martino, J. Pharm. Biomed. Anal., 2011, 55, 679 CrossRef CAS PubMed.
- J. Wang, M. Sánchez-Roselló, J. L. Aceña, C. del Pozo, A. E. Sorochinsky, S. Fustero, V. A. Soloshonok and H. Liu, Chem. Rev., 2014, 114, 2432 CrossRef CAS PubMed.
- J. A. Aguilar, G. A. Morris and A. M. Kenwright, RSC Adv., 2014, 4, 8278 RSC.
- A. I. H. Adams, G. Gosmann, P. H. Schneider and A. M. Bergold, Chromatographia, 2009, 69, S115 CAS.
- I. B. E. Ahmed and S. Abdalla, Eurasian J. Anal. Chem., 2010, 5, 254 Search PubMed.
- U. Holzgrabe, R. Deubner, C. Scollmayer and B. Waibel, J. Pharm. Biomed. Anal., 2005, 38, 806 CrossRef CAS PubMed.
- M. Butters, J. Ebbs, S. P. Green, J. MacRae, M. C. Morland, C. W. Murtiashaw and A. J. Pettman, Org. Process Res. Dev., 2001, 5, 28 CrossRef CAS.
- P. K. Owens, A. F. Fell and M. W. Coleman, J. Inclusion Phenom. Macrocyclic Chem., 2000, 38, 133 CrossRef CAS.
- U.S. Department of Health and Human Services, Food and Drug Administration, Center for Drug Evaluation and Research (CDER), Center for Biologics Evaluation and Research (CBER), 2003, Guidance for industry, Q1A(R2) Stability Testing of New Drugs Substances and Products, https://www.fda.gov/downloads/Drugs/GuidanceComplianceRegulatoryInformation/Guidances/UCM073369.pdf last verified on March 06, 2017.
- S. W. Baertschi and D. W. Reynolds, Introduction, in Pharmaceutical Stress Testing Predicting Drug Degradation, ed. S. W. Baertschi, Taylor & Francis Group, 2005 Search PubMed.
- M. D. Pelta, G. A. Morris, M. J. Stchedroff and S. J. Hammond, Magn. Reson. Chem., 2002, 40, S147 CrossRef CAS.
- A. Botana, J. A. Aguilar, M. Nilsson and G. A. Morris, J. Magn. Reson., 2011, 28, 270 CrossRef PubMed.
- I. Swan, M. Reid, P. W. A. Howe, M. A. Connell, M. Nilsson, M. A. Moore and G. A. Morris, J. Magn. Reson., 2015, 252, 120 CrossRef CAS PubMed.
- T. M. Barbosa, R. Rittner, C. F. Tormena, G. A. Morris and M. Nilsson, RSC Adv., 2016, 6, 95173 RSC.
- M. Nilsson, J. Magn. Reson., 2009, 200, 296 CrossRef CAS PubMed.
Footnote |
† Electronic supplementary information (ESI) available. See DOI: 10.1039/c7ra03822d |
|
This journal is © The Royal Society of Chemistry 2017 |