DOI:
10.1039/C5QI00227C
(Research Article)
Inorg. Chem. Front., 2016,
3, 348-353
Discoveries from a phenanthroline-based dynamic combinatorial library: catenane from a copper(I) or copper(II) template?†
Received
30th October 2015
, Accepted 7th December 2015
First published on 4th January 2016
Abstract
We report here a DCL study of a phenanthroline-based building block focusing on catenane formation with copper templates. Two [2]catenanes have been amplified by using Cu+ as a template from the DCLs that contain no interlocked compounds in the absence of copper. In addition, an unexpected Cu2+ template effect on the [2]catenane formation was discovered. The observed Cu2+ template effect was found to originate from the in situ reduction of the divalent metal to Cu+.
 Ho Yu Au-Yeung | Ho Yu Au-Yeung was born and raised in Hong Kong. He obtained his BSc (1st Hons) and MPhil from the Chinese University of Hong Kong, and later a PhD from the University of Cambridge as a Croucher Scholar with Prof. Jeremy K. M. Sanders. He then joined the group of Prof. Christopher J. Chang at the University of California, Berkeley as a Croucher Postdoctoral Fellow. In 2013, he started his independent research as an Assistant Professor in the Department of Chemistry, the University of Hong Kong. Research in his group is centred on supramolecular chemistry and molecular recognition. |
Introduction
Dynamic combinatorial chemistry (DCC),1 a selection approach for assembling molecular building blocks under thermodynamic control through reversible linkages, has been demonstrated to be an efficient strategy for the discovery of unexpected yet successful molecular receptors,2 biological ligands/inhibitors3 or complex molecular topologies,4 whose structures and syntheses could be unpredictable and/or unattainable. For example, Sanders and co-workers have recently reported a series of elegant examples of complex interlocked architectures including catenanes,5 Solomon links6 and trefoil knots,7 that are otherwise unpredicted and extremely challenging to synthesize, from aqueous disulfide dynamic combinatorial libraries (DCLs) using simple linear building blocks that can engage in aromatic donor–acceptor and hydrophobic interactions. If molecules having structures that are predesigned to favour catenane formation are used as building blocks in DCL, complex catenanes and other unexpected but potentially highly complicated molecular topologies could be obtained from this self-directing and self-selecting assembly approach. Since the orthogonal ligand arrangement from the tetrahedral phenanthroline–Cu+ coordination is one of the most successful preorganization strategies for catenane assembly,8 we propose the use of building blocks derived from the phenanthroline ligand will efficiently generate DCLs of interlocked structures plus other complex topologies when templated by Cu+, allowing the study of complex molecular topologies under thermodynamically-controlled conditions. We report here our initial study on the use of a phenanthroline-based dialdehyde building block for the generation of dynamic combinatorial libraries of catenanes9 using hydrazone as the exchange reaction. The DCL behaviour, amplification of two [2]catenanes by using Cu+ template and their characterisation are described. We also discovered an unexpected Cu2+ template effect on the assembly of the [2]catenanes. Further experiments on the coordination chemistry of model systems revealed that the observed template effect from Cu2+ originates from the Cu+ generated by the in situ reduction of the divalent metal ion. This unexpected template effect of Cu2+ was also applied in the assembly of catenanes under non-DCL conditions. It is believed that the studies of the Cu+–phenanthroline coordination motif under DCL conditions could offer a unique opportunity for studying the assembly of catenane and other possible, but not necessarily predictable or imaginable, complex molecular topologies under thermodynamic control, and eventually unveil the rules governing the self-assembly process of these complicated molecular entities.
Results and discussion
Building block designs and synthesis
The structure and synthesis of the phenanthroline-based building block 1 is depicted in Scheme 1. Building block 1 is designed to coordinate to Cu+ with the bidentate phenanthroline in a tetrahedral geometry and the terminal aldehydes are for hydrazone exchange with hydrazide building blocks. Two dihydrazide building blocks were synthesized and studied. Dihydrazide 2 has a flexible tetraethyleneglycol linker, whereas 3 contains the π-rich dioxynaphthalene unit for possible recognition of complementary π-deficient aromatics.
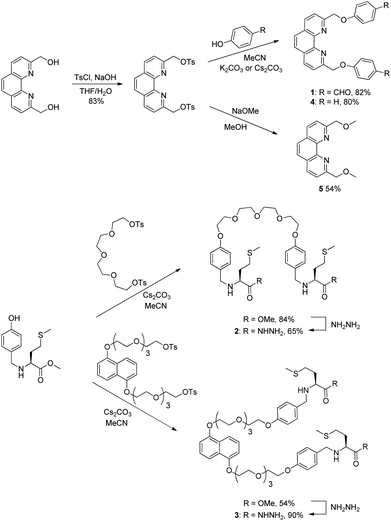 |
| Scheme 1 Synthesis of the dialdehyde building block 1, the dihydrazides 2 and 3, and the phenanthroline derivatives 4 and 5. | |
DCL studies and Cu+-templated catenane formation
The first hydrazone DCL was prepared by dissolving 1 mM each of the building blocks 1 and 2 in CHCl3/MeOH/CF3COOH (10
:
10
:
1). The library solution was analysed by LCMS after 3 days. The HPLC chromatogram showed that the [1 + 1] macrocycle M1 is the dominant species in the DCL, and it represents 96% of the library materials with the remaining 4% as the [2 + 2] macrocycle M2 (Fig. 1). Further analysis of the DCL at later time points (i.e. 5 and 7 days) revealed the same library composition, suggesting that the library has reached thermodynamic equilibrium (Scheme 2).
 |
| Fig. 1 HPLC analysis of a 1 mM DCLs of 1 and 2 (a) in the absence of a template and in the presence of 0.5 mM of (b) Cu+ and (c) Cu2+. Absorbance was monitored at 285 nm. | |
 |
| Scheme 2 Generation of hydrazone DCL from dialdehyde 1 and dihydrazide 2. The [2]catenane C1·Cu+ was amplified by the Cu+ template. | |
When the DCL was prepared in the presence of 0.5 mM Cu+, a library of a different composition was obtained. Addition of Cu+ to the DCL templated the formation of a new species (C1·Cu+), which was not detectable in the metal-free DCL, up to 71% at the expense of other library members. The pure sample of C1·Cu+ can be obtained by preparative HPLC or flash column chromatography for further analysis (see the ESI† for details). Results from ESI-MS, HRMS and MS/MS studies on C1·Cu+ are consistent with an interlocked structure (Fig. 2). The mass spectrum of C1·Cu+ shows peaks at m/z = 571.4 [C1 + Cu + 3H]4+, 761.5 [C1 + Cu + 2H]3+ and 1141.3 [C1 + Cu + H]2+ that correspond to the tetra-, tri- and di-cation of C1·Cu+ respectively. Fragmentation of the ion at m/z = 1141.3 [C1 + Cu + H]2+ directly resulted in daughter ions at m/z = 1109.3 and 1171.3 that correspond to [M1 + H]+ and [M1 + Cu]+. The observation that a [2 + 2] species directly fragment to a [1 + 1] and other smaller species without any other fragments with m/z larger than that of the [1 + 1] macrocycle is typical for a catenane, as other topologies such as a [2 + 2] macrocycle or a molecular figure-of-eight tied by Cu+ coordination should produce larger fragments such as those derived from the [2 + 2] or [2 + 1] building block combination in the MS/MS experiments.4a–c,5,10 Furthermore, to further confirm the catenane topology, the metal-free C1 obtained from treatment of C1·Cu+ with NaCN was also analysed by MS and MS/MS. The mass spectrum of the sample showed a peak at m/z = 1132.3 [C1 + 2Na]2+ that corresponds to the expected copper free C1. MS/MS analysis of C1 again resulted in only M1 (m/z = 1131.4 [M1 + Na]+) and its fragments (m/z = 1088.3, 1040.4, 1013.3 and 987.3) as the only species observed in the MS/MS experiment (Fig. 2). No larger species than that derived from M1 was observed in the tandem mass spectrum, further confirming the catenane topology of C1 and that a [2]catenane was successfully amplified by the Cu+ template from the dynamic library upon the anticipated phenanthroline–Cu+ coordination. 1H NMR studies on C1·Cu+ revealed multiple sets of overlapped resonances in the aromatic region, suggesting the presence of different inequivalent conformations of the catenane. Indeed, the 1H NMR spectrum of M1 does show at least six different sets of aromatic signals that are identified as the phenylene protons from the corresponding COSY spectrum (Fig. S19 and S20†), suggesting that both the syn and anti isomers of the hydrazone are present in M1 (and also most likely in C1·Cu+) that lead to the inequivalent aromatic chemical environments.
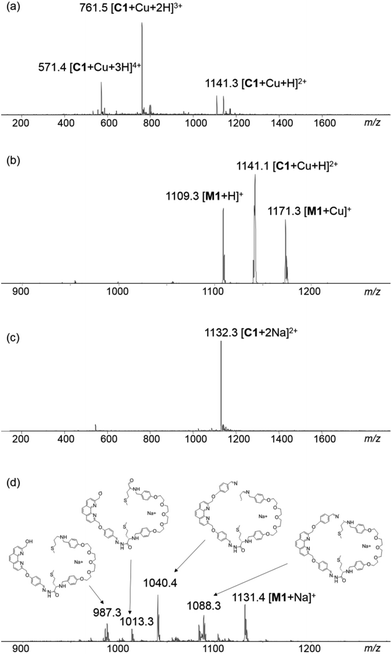 |
| Fig. 2 ESI-MS analysis of C1·Cu+ and C1. (a) The MS and (b) MS/MS spectra of C1·Cu+. The MS/MS spectrum was obtained from fragmenting the peak at m/z = 1141.3. (c) The MS of spectrum C1; and (d) the MS/MS spectrum from fragmentation of the peak at m/z = 1132.3. | |
The dynamic nature of the catenane assembly was studied by a series of equilibration experiments. First, instead of adding the Cu+ template at the beginning of the DCL preparation, the metal ion was added to a DCL that had been equilibrated for 3 days. Concentration of C1·Cu+ in the DCL gradually increased from undetectable to ca. 70% with the remaining 30% as M1, which is almost the same as that of the DCL prepared when Cu+ was introduced at the beginning of the DCL evolution (Fig. S28†). The observation that the same equilibrium position could be reached from two different starting points suggests that the library is dynamic and is under thermodynamic control.11 Second, the effect of the building block concentration on the equilibrium position and yield of C1·Cu+ was studied. DCLs with different concentrations of 1 and 2 were setup and their equilibrium compositions were compared. In accordance with the Le Châtelier's principle, an increase in the yield of C1·Cu+ was observed from 36% to 71% to 93% when the building block concentrations were increased from 0.1 mM to 1 mM to 5 mM at a building block/Cu+ ratio of 2
:
1 (Fig. S26†). Third, the effect of the Cu+ template on the building block ratio was studied. DCLs with 0.1, 0.5, 1 and 2 eq. of Cu+ relative to 1 were prepared. From 0.1 eq. to 0.5 eq. of Cu+, an increase in the yield of C1·Cu+ from 17% to 71% was observed. Further increasing the template concentration to 1 eq. and 2 eq. of Cu+ relative to 1, on the other hand, led to a decrease of the yield of C1·Cu+ from 68% to 48% respectively (Fig. S27†). Presumably, at Cu+ concentration below 0.5 eq., an increase in the concentration of Cu+ templates the formation of the 2
:
1 phenanthroline–Cu+ complex and leads to a higher yield of C1·Cu+. However, further increase in the Cu+ concentration could shift the equilibrium towards the 1
:
1 phenanthroline–Cu+ complex which favors the formation of the macrocycle instead of the [2]catenane.
A similar hydrazone DCL was also prepared from 1 and 3 under the same conditions (Scheme S4†). The library mixture was analysed by LCMS which showed the [1 + 1] macrocycle M3 as the only detectable library member. No [2 + 2] macrocycle similar to M2 was found. Similar to the DCL of 1 and 2, the presence of 0.5 mM Cu+ templates the formation of the [2]catenane C2·Cu+ to 90% (Fig. S26†). The interlocked structure of C2·Cu+ and its metal-free form C2 were also characterised by ESI-MS and MS/MS. Fragmentation behaviour similar to that of C1·Cu+ and C1 was observed, confirming the catenane topology of C2·Cu+ (Fig. S34 and S35†). Since building block 3 is designed with the π-rich dioxynaphthalene core for further molecular recognition of complementary π-deficient units, we tested if the use of the π-deficient cyclobisparaquat(p-phenylene) (CBPQT4+)12 macrocycle could amplify other interlocked compounds from the DCL. Due to the insolubility of CBPQT4+ under DCL conditions, attempts to use the π-deficient macrocycle as a template, in the form of a concentrated MeCN solution, were unsuccessful. LCMS analysis of the filtered library solution revealed the presence of only C2·Cu+ and M3, in the distribution as if the macrocyclic template was not added (Fig. S29†). No incorporation of the π-deficient unit to the π-rich library members was observed.
CuII template catenane formation in DCL
In addition to Cu+, other metal ions including Li+, Na+, K+, Mg2+, Ca2+, Al3+, Mn2+, Fe2+, Fe3+, Co2+, Ni2+, Cd2+, Ce3+ and Er3+ were also tested as templates for the DCL of 1 and 2. While these metal ions did not perturb the equilibrium position and the same library compositions as the metal-free DCL were observed (Fig. S25†), C1·Cu+ was amplified by addition of Cu2+. While the redox switching of Cu2+/+ has been demonstrated to induce the circumrotation and conformation change of catenanes and other complex topologies with variable coordination geometry and coordination number,13 templating the [2]catenane assembly from two bidentate phenanthroline precursors by Cu2+ is not known as far as we are concerned. Compared with the Cu+ template, Cu2+ only amplifies C1·Cu+ to 44% of the library materials. The lower efficiency of Cu2+ to template C1 in the DCL may suggest that (1) Cu2+ coordination to the [2]catenane is thermodynamically less stable than that of Cu+ or (2) the mechanism of the Cu2+ template involves not only Cu2+–phenanthroline coordination.
In situ reduction of CuII to CuI
To elucidate the origin of the Cu2+ template effect on the [2]catenane assembly, the coordination chemistry of the phenanthroline derivatives 4 and 5 to Cu2+ was studied. Complexation of 4 to CuCl2 in a 2
:
1 ratio in CH2Cl2/MeOH at room temperature initially resulted in a yellow solution that gradually turned red. UV-Vis analysis showed that the red species has an absorption maximum at 445 nm which is consistent with the reported MLCT band of Cu+–phenanthroline (Fig. 3a).14 The absorption spectrum also resembles that of the independently synthesized [Cu(4)2][PF6], indicating a reduction of Cu2+ to Cu+ upon coordination of 4 under experimental conditions. Similar Cu2+ to Cu+ reduction has also been observed in cross-coupling reactions involving copper as the catalysts,15 but such effects in molecular self-assembly involving the metal is not known. By comparing the relative absorbance, a ca. 60% conversion of Cu2+ to Cu+ has been estimated, which correlates very well with the observed relative yields (71% vs. 44%) of C1·Cu+ in the corresponding DCLs. The observed in situ reduction is also supported by 1H NMR studies. The red solution obtained from a 2
:
1 mixture of 4 and CuCl2 in CD3CN showed no paramagnetically shifted resonances due to the d9 Cu2+, and that the 1H spectrum is consistent with the formation of [Cu(4)2]+ (Fig. S24†).
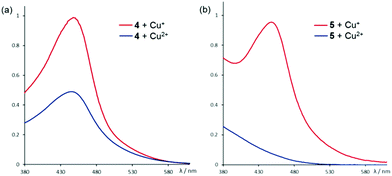 |
| Fig. 3 UV-Vis spectra of a 2 : 1 complex mixture of the phenanthroline ligands (a) 4 and (b) 5 with Cu+ (red) and Cu2+ (blue). The absorption band at 445 nm is assigned to the Cu+–phenanthroline MLCT.13 | |
Presumably, the tetrahedral coordination geometry enforced by the two phenanthrolines modulates the reduction potential of Cu2+/+ such that Cu2+ could be reduced to Cu+, possibly by protic reagents, upon coordination to the bidentate ligand under experimental conditions.15,16 The X-ray structure of [Cu(4)2][PF6] revealed the expected tetrahedral coordination geometry around the metal (Fig. 4a). In addition, the observed π-stacking interaction between the phenanthroline and the aromatic substituents may also reinforce the stability of the tetrahedral coordination motif and facilitate the in situ reduction. Indeed, the complexation reaction of 5 to Cu2+ resulted in a much less efficient reduction to Cu+ as shown by both UV-Vis and 1H NMR studies (Fig. 3b and S24†). X-ray diffraction study of the crystalline product obtained from a 2
:
1 mixture of 5 and CuCl2 showed only the 5-coordinated [Cu(5)Cl2] with a distorted square pyramidal structure (Fig. 4b). The formation of such a 1
:
1 complex suggests that Cu2+–phenanthroline coordination alone is not sufficient to explain the observed amplification of the [2]catenane in the DCL because the required preorganized ML2 complex could not be formed. Together with the UV-Vis and NMR data, it is believed that the reduction of Cu2+ to Cu+, and the subsequent formation of the tetrahedral ML2 complex constitute the major part of the observed template effect.
 |
| Fig. 4 Molecular structures of (a) [CuI(4)2]+ (the PF6− anion is omitted for clarity) and (b) [CuII(5)Cl2]. Selected bond lengths (Å) and angles (°) for [CuI(4)2]+: Cu1–N1 2.029(2), Cu1–N2 2.038(3), Cu1–N3 2.048(3), Cu1–N4 2.032(3); N1–Cu1–N2 82.1(8), N3–Cu1–N4 82.2(7), N1–Cu1–N3 123.5(7), N2–Cu1–N4 132.8(8); for [CuII(5)Cl2]: Cu1–N1 1.955(2), Cu1–N2 2.135(2), Cu1–O1 2.256(2); N1–Cu1–N2 80.7(9), N1–Cu1–O1 74.1(3), O1–Cu1–Cl1 90.3(9), N2–Cu1–Cl1 105.8(1). | |
Templating another [2]catenane assembly by Cu2+
We also tested if the Cu2+ template can be applied in the assembly of catenane under conditions other than that of the hydrazone DCL. We chose to study the assembly of catenane from 1 and 4,7-dioxadodecanediamine via a reductive amination reaction due to the wide applicability of imine formation in the synthesis of interlocked systems. Heating an equimolar solution of 1 and 4,7-dioxadodecanediamine (10 mM each) in the presence of 5 mM CuCl2 overnight in MeCN/CHCl3 resulted in a red solution that is consistent with the previously observed Cu2+ to Cu+ reduction. To aid analysis, the dynamic imine was reduced to the corresponding secondary amine by NaBH4. Albeit in a lower yield than if Cu+ is used as the template (58%), a [2]catenane C3·Cu+ was successfully assembled in 15% yield (Scheme 3), demonstrating the feasibility of using Cu2+ as a template for constructing molecular architectures with phenanthroline-based building blocks. The interlocked structure of C3·Cu+ has been confirmed by NMR, ESI-MS and MS/MS (Fig. S22, S23, S36 and S37†).
 |
| Scheme 3 Self-assembly of C3·Cu+ using CuCl2 as the template. | |
Conclusions
In summary, the DCL behaviour of a phenanthroline-based dialdehyde building block for catenane assembly has been studied. Two [2]catenanes have been amplified in high yields by using Cu+ as the template. Unexpectedly, it is found that Cu2+, upon in situ reduction, could also lead to the formation of the interlocked compounds under both DCL and non-DCL conditions. The discovery of the Cu2+-template effect on catenane assembly not only suggests that a larger variety of copper salts could be used for molecular self-assembly involving the versatile phenanthroline motif under different assembly conditions, but also highlights the relatively less explored application of DCC that assists the discovery of alternative templates for an assembly event. Further studies of the dynamic combinatorial assembly of other complex interlocked structures using other building blocks, and diversifying the DCL composition by increasing library complexity are currently underway.
Acknowledgements
Financial support from the URC, the University of Hong Kong under the Seed Funding programme for Basic Research (project code 201310159019) and the Croucher Foundation are gratefully acknowledged. We thank Dr Lap Szeto and Mr Kai Wang for technical assistance with X-ray crystallography and ESI-MS analysis, respectively. K. L. T. and C. C. Y. also thank the University of Hong Kong for the provision of a Postgraduate Scholarship. Y. C. T. is a recipient of the Summer Research Fellowship administered by the Faculty of Science, the University of Hong Kong.
Notes and references
-
(a) J.-M. Lehn, Chem. – Eur. J., 1999, 5, 2455 CrossRef CAS
;
(b) P. T. Corbett, J. Leclaire, L. Vial, K. R. West, J.-L. Wietor, J. K. M. Sanders and S. Otto, Chem. Rev., 2006, 106, 3652 CrossRef CAS PubMed
;
(c) J.-M. Lehn, Chem. Soc. Rev., 2007, 36, 151 RSC
;
(d) S. Ladame, Org. Biomol. Chem., 2008, 6, 219 RSC
;
(e) F. B. L. Cougnon and J. K. M. Sanders, Acc. Chem. Res., 2012, 45, 2211 CrossRef CAS PubMed
.
- For recent examples:
(a) L. I. James, J. E. Beaver, N. W. Rice and M. L. Waters, J. Am. Chem. Soc., 2013, 135, 6450 CrossRef CAS PubMed
;
(b) S. Hamieh, V. Saggiomo, P. Nowak, E. Mattia, R. F. Ludlow and S. Otto, Angew. Chem., Int. Ed., 2013, 52, 12368 CrossRef CAS PubMed
;
(c) N. K. Pinkin and M. L. Waters, Org. Biomol. Chem., 2014, 12, 7059 RSC
;
(d) J. E. Beaver, B. C. Peacor, J. V. Bain, L. I. James and M. L. Waters, Org. Biomol. Chem., 2015, 13, 3220 RSC
;
(e) J. Li, P. Nowak and S. Otto, Angew. Chem., Int. Ed., 2015, 54, 833 CrossRef CAS PubMed
.
- For recent examples:
(a) M. Demetriades, I. K. H. Leung, R. Chowdhury, M. C. Chan, M. A. McDonough, K. K. Yeoh, Y.-M. Tian, T. D. W. Claridge, P. J. Ratcliffe, E. C. Y. Woon and C. J. Schofield, Angew. Chem., Int. Ed., 2012, 51, 6672 CrossRef CAS PubMed
;
(b) M. Mondal, N. Radeva, H. Köster, A. Park, C. Potamitis, M. Zervou, G. Klebe and A. K. H. Hirsch, Angew. Chem., Int. Ed., 2014, 53, 3259 CrossRef CAS PubMed
;
(c) Q.-Q. Jiang, W. Sicking, M. Ehlers and C. Schmuck, Chem. Sci., 2015, 6, 1792 RSC
;
(d) M. Mondal and A. K. H. Hirsch, Chem. Soc. Rev., 2015, 44, 2455 RSC
.
-
(a) R. T. S. Lam, A. Belenguer, S. L. Roberts, C. Naumann, T. Jarrosson, S. Otto and J. K. M. Sanders, Science, 2005, 308, 667 CrossRef CAS PubMed
;
(b) H. Y. Au-Yeung, G. Dan Pantoş and J. K. M. Sanders, Proc. Natl. Acad. Sci. U. S. A., 2009, 106, 10466 CrossRef CAS PubMed
;
(c) F. B. L. Cougnon, N. A. Jenkins, G. D. Pantoş and J. K. M. Sanders, Angew. Chem., Int. Ed., 2012, 51, 1443 CrossRef CAS PubMed
;
(d) M.-K. Chung, S. J. Lee, M. L. Waters and M. R. Gagné, J. Am. Chem. Soc., 2012, 134, 11430 CrossRef CAS PubMed
;
(e) J. Li, P. Nowak, H. Fanlo-Virgós and S. Otto, Chem. Sci., 2014, 5, 4968 RSC
;
(f) H. Wilson, S. Byrne and K. M. Mullen, Chem. – Asian J., 2015, 10, 715 CrossRef CAS PubMed
.
-
(a) H. Y. Au-Yeung, G. D. Pantoş and J. K. M. Sanders, J. Am. Chem. Soc., 2009, 131, 16030 CrossRef CAS PubMed
;
(b) H. Y. Au-Yeung, G. D. Pantoş and J. K. M. Sanders, J. Org. Chem., 2011, 76, 1257 CrossRef CAS PubMed
;
(c) F. B. L. Cougnon, H. Y. Au-Yeung, G. D. Pantoş and J. K. M. Sanders, J. Am. Chem. Soc., 2011, 133, 3198 CrossRef CAS PubMed
;
(d) F. B. L. Cougnon, N. Ponnuswamy, N. A. Jenkins, G. D. Pantoş and J. K. M. Sanders, J. Am. Chem. Soc., 2012, 134, 19129 CrossRef CAS PubMed
.
- N. Ponnuswamy, F. B. L. Cougnon, G. D. Pantoş and J. K. M. Sanders, J. Am. Chem. Soc., 2014, 136, 8243 CrossRef CAS PubMed
.
- N. Ponnuswamy, F. B. L. Cougnon, J. M. Clough, G. D. Pantoş and J. K. M. Sanders, Science, 2012, 338, 783 CrossRef CAS PubMed
.
- C. O. Dietrich-Buchecker and J.-P. Sauvage, Chem. Rev., 1987, 87, 795 CrossRef CAS
.
- J. A. Berrocal, M. M. L. Nieuwenhuizen, L. Mandolini, E. W. Meijer and S. D. Stefano, Org. Biomol. Chem., 2014, 12, 6167 CAS
.
-
(a) P. Bäuerle, M. Ammann, M. Wilde, G. Götz, E. Mena-Osteritz, A. Rang and C. A. Schalley, Angew. Chem., Int. Ed., 2007, 46, 363 CrossRef PubMed
;
(b) S.-A. Poulsen, P. J. Gates, G. R. L. Cousins and J. K. M. Sanders, Rapid Commun. Mass Spectrom., 2000, 14, 44 CrossRef CAS PubMed
.
- Attempt to reverse the library composition from the catenane to the macrocycle by removal of the Cu+ template was not tested because of the incompatibility of common demetallating reagents such as CN− under acidic DCL conditions.
- For recent examples:
(a) C. Wang, S. M. Dyar, D. Cao, A. C. Fahrenbach, N. Horwitz, M. T. Colvin, R. Carmieli, C. L. Stern, S. K. Dey, M. R. Wasielewski and J. F. Stoddart, J. Am. Chem. Soc., 2012, 134, 19136 CrossRef CAS PubMed
;
(b) H. Liu, X.-Y. Li, X.-L. Zhao, Y. A. Liu, J.-S. Li, B. Jiang and K. Wen, Org. Lett., 2014, 16, 5894 CrossRef CAS PubMed
;
(c) W.-B. Hu, W.-J. Hu, X.-L. Zhao, Y. A. Liu, J.-S. Li, B. Jiang and K. Wen, Chem. Commun., 2015, 51, 13882 RSC
.
-
(a) A. Livoreil, C. O. Dietrich-Buchecker and J.-P. Sauvage, J. Am. Chem. Soc., 1994, 116, 9399 CrossRef CAS
;
(b) F. Baumann, A. Livoreil, W. Kaim and J.-P. Sauvage, Chem. Commun., 1997, 35 RSC
;
(c) A. Livoreil, J.-P. Sauvage, N. Armaroli, V. Balzani, L. Flamigni and B. Ventura, J. Am. Chem. Soc., 1997, 119, 12114 CrossRef CAS
;
(d) D. J. Cárdenas, A. Livoreil and J.-P. Sauvage, J. Am. Chem. Soc., 1996, 118, 11980 CrossRef
;
(e) F. Niess, V. Duplan and J.-P. Sauvage, J. Am. Chem. Soc., 2014, 136, 5876 CrossRef CAS PubMed
;
(f) F. Niess, V. Duplan, C. S. Diercks and J.-P. Sauvage, Chem. – Eur. J., 2015, 21, 14393 CrossRef CAS PubMed
.
- D. V. Scaltrito, D. W. Thompson, J. A. O'Callaghan and G. J. Meyer, Coord. Chem. Rev., 2000, 208, 243 CrossRef CAS
.
- G. Franc and A. Jutand, Dalton Trans., 2010, 39, 7873 RSC
.
- E. A. Ambundo, M.-V. Deydier, A. J. Grall, N. Aguera-Vega, L. T. Dressel, T. H. Cooper, M. J. Heeg, L. A. Ochrymowycz and D. B. Rorabacher, Inorg. Chem., 1999, 38, 4233 CrossRef CAS
.
Footnotes |
† Electronic supplementary information (ESI) available: Detailed synthesis, NMR, MS, HPLC, and X-ray crystallographic data. CCDC 1431350 and 1431358. For ESI and crystallographic data in CIF or other electronic format see DOI: 10.1039/c5qi00227c |
‡ These authors contributed equally. |
|
This journal is © the Partner Organisations 2016 |