DOI:
10.1039/D3QI01268A
(Research Article)
Inorg. Chem. Front., 2023,
10, 5782-5788
Making one VIV substitution for Mo on classical [MoV2O4]2+ group: the first heterobimetallic Mo–V subunit in polyoxomolybdate–bisphosphonate family†
Received
6th July 2023
, Accepted 17th August 2023
First published on 18th August 2023
Introduction
Polyoxometalates (POMs) are a large exceptional class of oxygen cluster compounds mainly consisting of early transition metals of group V and VI (Mo, W, V, Nb, Ta, etc.) in their high oxidation states, which have a diverse structural and compositional range as well as a great variety of catalytic,1–5 electrochemical,6–8 medical,9–12 fluorescent13–15 and magnetic properties16–18 on the molecular level. Grafting organic moieties onto POMs by covalent bonds can unite the advantages of the inorganic metal oxide cluster and the organic components with rich functionalities to enrich the structure of POMs and enhance compatibility with other organic substrates or media.19–21 Recently, POMos incorporating BP have been an important subclass of POMs and possesses potential application prospects for the prominent work of many chemists.22 However, we find that the types of topological structures are very limited and often only change the ligands in POMos–BP complexes, possibly due to the main precursor of most POMos–BP complexes being the dication [MoV2O4]2+
23 and the majority of the basic building units being the following: [MoV2O4],24 [MoVI2O6],25 [MoVI3O8] (Scheme 1a–l).26 (Here, a basic building unit is well-defined as part of the integral structure rather than an isolated cluster.) These restricted basic building units hinder the generation of novel compounds within the POMos–BP family and significantly hamper the structural and preparative chemistry of the POMos–BP family, ultimately influencing the performance through structure–function relationships. It is a huge challenge to further boost the development of POMos–BP systems with desired structures.
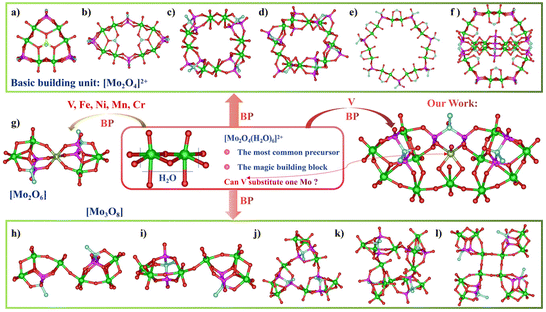 |
| Scheme 1 The design concept of achieving a new heterobimetallic building unit in this work and previous works: (a–f) the classical wheel shaped clusters based on basic building unit [Mo2O4]2+, (g) the transition metal element M doped Mo4(BP)2M (M = VIV, CrIII, FeIII, MnII) based on [MoVI2O6], (h–l) the various structures of POMos–BP members based on [MoVI3O8] and our work. | |
To resolve the above-mentioned challenge, it is thus vastly essential to elaborate a library of basic building units with distinct compositional and structural characteristics. Inspired by previous reports about molybdenum/vanadium (Mo/V) or Mo–V mixed clusters for the similar coordination geometry, ionic radius and valence state between Mo and V,27–30 we deem it feasible and valuable to explore more new building units based on one V in place of one Mo in [MoV2O4] units in the family of POMos–BPs (Scheme 1). Meanwhile, the substitution pattern of V retaining the V
O with redox-active sites may have much better catalytic oxidation activity than the simple chelating coordination doping of V into Mo clusters like other transition metals.
The main objective of the present work is to investigate whether it is feasible to construct the hetero-metallic binuclear building unit [MoVVIVO4] in a POMos–BP system. Additionally, this work aims to confirm the significance of the V-replacing mode with V
O by contrasting its catalytic oxidation activity with that of other V-doping without V
O through an organic model reaction. Fortunately, by examining various reaction conditions, we were able to isolate novel Mo–V–BP clusters with an unusual building unit [MoVVIVO2(OH)2(H2O)]3+ retaining V
O (compound 1), as well as a fresh illustration of straightforward V coordination doping free of V
O (compound 2) for comparison.
Even though the classical dication building block [MoV2O4]2+ is recurrent for the design of various Mo–oxygen clusters30 and the incorporation of transition metal elements M into Mo4(BP)2M (M = VIV, CrIII, FeIII, MnII) has previously been reported,31–36 herein the unprecedented heterometallic binuclear building unit [MoVVIVO2(OH)2(H2O)]3+ was first obtained in the POMs–BP family (Scheme 1). Notably, the desired compound 1 containing the fundamental heterobimetallic building unit [MoVVIVO2(OH)2(H2O)]3+ obtained by substituting one Mo in the traditional [MoV2O4] dimer was characterized by single-crystal X-ray diffraction (SXRD), powder X-ray diffraction (PXRD), FT-IR, XPS, and TGA. Furthermore, as a proof-of-concept, the catalytic oxidation activities of 1 and 2 for the oxidation of dibenzothiophene (DBT) using H2O2 as an oxidant were examined. By using the molecular models 1 and 2 as atomically precise building blocks, this study enriches the POMos–BP family's basic building blocks and opens new possibilities for the design of catalytic activity sites for Mo–V clusters.
Results and discussion
The treatment of [MoV2O4(H2O)4]2+ aqueous media and risedronic acid (H5L: H2PO3C(OH)(CH2-3-C5NH4)PO3H2) with different V precursors at 60 °C for 3 h afforded two polyoxomolybdovanadates, 1 [N(CH3CH2)4]7(NH4)13{[MoVVIVO2(OH)2(H2O)H2L3−][Mo5O15H2L3−]2}3·2Cl·24H2O and 2 (NH4)9Na(H2O)2[NaVIII(MoVI2O6)2L2Cl(H2O)2]2·2H2O, by adjusting the pH with different alkaline substances and counter cations. (The synthetic details are shown in the ESI.†) The polyanions in 1 can be briefly described as a centrosymmetric cluster in which a novel heterometallic binuclear unit [MoVVIVO2(OH)2(H2O)H2L3−] is united to two pentamolybdate cluster-based building units [Mo5O15H2L3−]3− on a mirror plane (Fig. 1a). The dimer of [MoVVIVO2(OH)2(H2O)]3+ can be viewed as a substitution of one V on [MoV2O4]2+ for one Mo. Bond valence sums (BVS) of 1 confirm that the oxidation states of V and Mo in the dinuclear unit are +4 and +5, which is consistent with the results of XPS (Fig. S4–S6†). It is important to emphasize that BVS supports the assignment of two μ2-O between Mo and V of [MoVVIVO2(OH)2(H2O)]3+ as OH, demonstrating that the two μ2-O have relatively stronger basicity than the other oxygen atoms. This, in turn, leads to a new trivalent [MoVVIVO2(OH)2(H2O)]3+ dimer unit. The VIV ion bearing one terminal V
O bond adopts a square-pyramidal geometry with two V–O bonds from two phosphonato groups of one BP ligand and two V–O bonds from [MoVVIVO2(OH)2(H2O)]3+. The MoV with Mo
O of [MoVVIVO2(OH)2(H2O)]3+ ion is coordinated by four μ2-O atoms and one H2O molecule to furnish a distorted octahedral geometry. Remarkably, the protonation of two doubly bridging oxygen atoms prolonged the associated Mo–O distances (0.208 nm) and induced conspicuous trans influences, resulting in the formation of two shorter μ2-O doubly bridging Mo–O(Mo) (0.177 nm) distances, which is exceedingly unusual for trans influence in POMos chemistry. Meanwhile, the shorter Mo
O (0.172 nm) double bonds trans to a long Mo–OH2 (0.235 nm) bond are also attributed to trans influences. The successful replacement of Mo with V indicates that [MoVVIVO4]+ is a possible basic building block (in some ways similar to the second building units of metal–organic frameworks) for the synthesis of novel Mo–V mixed clusters functionalized by BP or other organic groups. The anion cluster skeleton can also be viewed as two [Mo5O15H2L3−]3− building units systematically linked to a binuclear unit [MoVVIVO2(OH)2(H2O)H2L3−]. The [Mo5O15H3L2−]2− subunit is composed of five Mo octahedra in a corner- and edge-sharing arrangement and forms a hollow shell occupied by the H2L3− ligand (Fig. 1d and g). As far as we know, these fascinatingly structured subunit [MoVVIVO2(OH)2(H2O)]3+ dimer and pentamolybdate cluster basic building block [Mo5O15] are both first reported here. In POMos chemistry, the pure inorganic hendeca-nuclear Mo-clusters with one Mo atom bridging two [Mo5O15] are novel. The two types of H2L3− ligands exhibit distinct penta-coordination modes, as shown in Fig. 1e and f, in which two phosphonato groups from another H2L3− ligand adopt the 2.110 mode and 5.221 bridging mode (Harris notation).37 To compare the catalytic activity of the Mo–V–BP clusters with distinct V doping modes, the chelating–coordinating compound 2 devoid of V
O double bonds was synthesized by varying the reaction conditions. As depicted in Fig. 2, the cluster anion 2 can be described as two units of [(MoVI2O6)C6H5NCH3C(O)(PO3)2]4− linked by one VIII cation and exhibits isostructural characteristics with two previous POMos–BP derivatives based on different BPs.38 The V–O bond lengths in this anion range from 0.194 to 0.204 nm, meaning that vanadyl (V
O) is not present.39 The [MoVI2O6] structural unit is composed of two Mo octahedra in a face-sharing mode which are stabilized by two phosphonato groups and the hydroxyl of HL4− ligands via P–O–Mo and C–O–Mo covalent bonding. The PO3 group adopts the 3.120 coordination mode and the OH group bound to the C atom is found to be deprotonated, which was probably from the higher pH value of the solution.
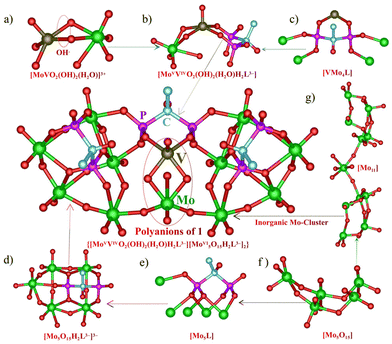 |
| Fig. 1 Schematic illustration of the structures of the polyanions in 1 and its subunits (the pyridine ring of L was deleted for clarity): (a) [MoVVIVO2(OH)2(H2O)]3+, (b) [MoVVIVO2(OH)2(H2O)H2L3−], (c) [VMo4L], (d) [Mo5O15H2L3−]3−, (e) [Mo5L], (f) [Mo5O15], (g) [Mo11]. | |
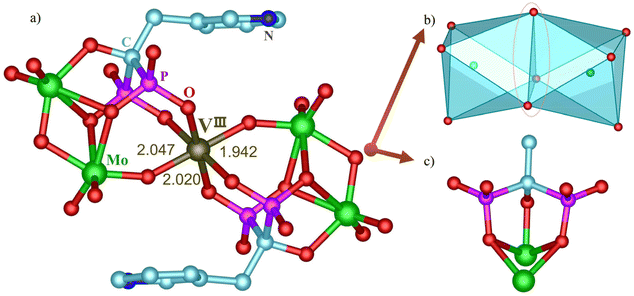 |
| Fig. 2 Schematic illustrations of the structures of (a) [(MoVI2O6)C6H5NCH3C(O)(PO3)2]4− in 2, (b) the polyhedron of [MoVI2O6] structural unit, and (c) the coordination mode of two phosphonato groups and hydroxyl of HL4− ligand. | |
As in previous reports, the Mo/V mixed-addendum Keggin-type POMs [H3+xPVxMo12−xO40] show better catalytic activities than pure [PMo12O40]3−, which indicates that the V atom may play a key function during the catalytic reaction process.40 The catalytic reaction route may also include the prevalent oxygen atom transfer (OAT) via the Mars–Van Krevelen mechanism.41–44 Hence, the remaining V
O double bonds in Mo–V catalysts may have more activity than those in other Mo–V clusters. In view of this, the atomically precise Mo–V clusters 1 and 2 are chosen as model catalysts to check the catalytic activity by the oxidation of dibenzothiophene (DBT) with H2O2 as an oxidant. The target product was obtained by column chromatography and analyzed by 1H NMR.
The effects of time and solvent on the product catalyzed by 1 were investigated using dibenzothiophene as a substrate. Table 1 provides an overview of the selected examples. The optimal conditions are 0.2 mmol of substrate, 0.001 mmol of catalyst, 0.8 mmol H2O2 (30% aqueous), 80 °C, 12 h, and 2 mL CH3CN. The catalytic properties of compound 1 were assessed by screening compounds 1 and 2 and a blank under standard conditions (0.2 mmol thioether, thioanisole, and 4-chlorothioanisole), as shown in Table 1. In entry 1 of Table 2, the blank reaction and compound 2 were performed on dibenzothiophene, with only 22% and 35% conversions and 0% and 25% selectivities, respectively. The conversion and selectivity were low. However, compound 1 has good conversion and selectivity for sulfone, which suggests that V
O played a key role in the catalytic performance of POMos–BP. When expanded to diphenyl thioether, thioanisole, and 4-chlorothioanisole, compound 1 still exhibits good conversion and selectivity.
Table 1 Effect of reaction conditions for the oxidation of DBT with H2O2
a

|
Entry |
Solvent |
Time (h) |
Conversionb (%) |
Select for sulfoneb (%) |
Reaction conditions: 0.2 mmol of dibenzothiophene, 0.001 mmol of compound 1, 0.8 mmol H2O2 (30% aqueous), 80 °C, 2 mL of solvent.
Conversion: isolated yield.
|
1 |
CH3CN |
6 |
39 |
>99 |
2
|
CH
3
CN
|
12
|
95
|
>99
|
3 |
CH3CN |
18 |
96 |
>99 |
4 |
H2O |
12 |
Trace |
Trace |
5 |
DMF |
12 |
Trace |
Trace |
6 |
EtOH |
12 |
57 |
>99 |
7 |
EA |
12 |
68 |
72 |
8 |
DCM |
12 |
27 |
9 |
9 |
1,4-Dioxane |
12 |
90 |
63 |
Table 2 The oxidation of sulfides using different catalystsa
To verify the universality of 1 under the optimized reaction conditions for the high selectivity of sulfone, different substituent sulfides were used as substrates in the catalytic reactions. As shown in Scheme 2, all substrate expansion experiments indicated that the selectivity for sulfone was higher than 90%. The conversion rate decreases slightly as the steric hindrance of the S atom increases and the selectivity stays high with the increasing steric hindrance of the S atom.
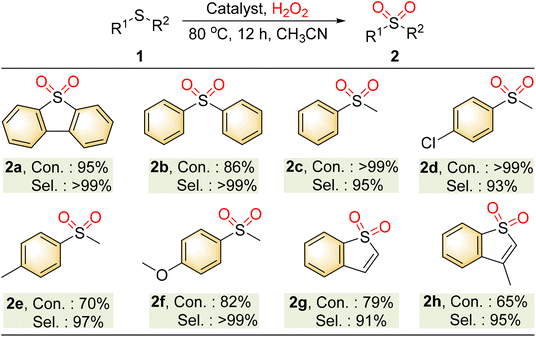 |
| Scheme 2 Substrate expansion for catalytic oxidation of thioether by 1. | |
Conclusions
In summary, the first molybdovanadate cluster (1) modified by BP with the unusual [MoVVIVO4] building unit was successfully synthesized based on the substitution of one V for one Mo in the classical [MoV2O4] building unit. More significantly, we demonstrated that the V
O in 1 plays a pivotal role in the deep oxidation of dibenzothiophene into sulfone by comparing the atomically precise 1 and 2 as molecular model catalysts. This work enriches the basic building units of the POMos–BP family and may shed new light on the assembly and catalytic activity site design of Mo–V clusters. Further studies of the assembly mechanism, other novel Mo–V clusters containing the [MoVVIVO4] building unit using this approach, and potential catalytic activities toward other organic model reactions are underway in our laboratory and will be reported in due course.
Author contributions
Y. F. Bi and B. K. Chen conceived and designed the project. X. Y. Ren, G. Zhang, and L. L. Dai performed the experiments and characterization. X. Y. Ren wrote the original draft. G. P. Yang, Y. F. Bi and B. K. Chen provided supervision, validated the experimental results, and reviewed and edited the manuscript. G. P. Yang and B. K. Chen provided funding support.
Conflicts of interest
The authors declare no competing financial interest.
Acknowledgements
This work was financially supported by the National Natural Science Foundation of China (22171122), the Liaoning Revitalization Talents Program (XLYC2007130), and Talent Scientific Research Fund of Liaoning Petrochemical University (2016XJJL-019).
References
- B. J. Xu, Q. Xu, Q. Z. Wang, Z. Liu, R. K. Zhao, D. D. Li, P. T. Ma, J. P. Wang and J. Y. Niu, A copper-containing polyoxometalate-based metal-organic framework as an efficient catalyst for selective catalytic oxidation of alkylbenzenes, Inorg. Chem., 2021, 60, 4792–4799 CrossRef CAS PubMed.
- X. N. Zou, D. Zhang, T. X. Luan, Q. Li, L. Li, P. Z. Li and Y. Zhao, Incorporating photochromic triphenylamine into a zirconium-organic framework for highly effective photocatalytic aerobic oxidation of sulfides, ACS Appl. Mater. Interfaces, 2021, 13, 20137–20144 CrossRef CAS PubMed.
- J. Li, D. Zhang, Y. N. Chi and C. W. Hu, Catalytic application of polyoxovanadates in the selective oxidation of organic molecules, Polyoxometalates, 2022, 1, 9140012 CrossRef.
- J. L. Wang, J. P. Cao, Z. Y. Du, X. M. Liu, J. N. Li, Q. D. Ping, T. Y. Zang and Y. Xu, Four novel Z-shaped hexanuclear vanadium oxide clusters as efficient heterogeneous catalysts for cycloaddition of CO2 and oxidative desulfurization reactions, Chin. Chem. Lett., 2023, 34, 106917 CrossRef CAS.
- Y. Zhang, X. Wang, Y. Wang, N. Xu and X. L. Wang, Anderson-type polyoxometalate-based sandwich complexes bearing a new “V”-like bis-imidazole-bis-amide ligand as electrochemical sensors and catalysts for sulfide oxidation, Polyoxometalates, 2022, 1, 9140004 CrossRef.
- D. J. Zang, Y. C. Huang, Q. Li, Y. J. Tang and Y. G. Wei, Cu dendrites induced by the Anderson-type polyoxometalate NiMo6O24 as a promising electrocatalyst for enhanced hydrogen evolution, Appl. Catal., B, 2019, 249, 163–171 CrossRef CAS.
- L. M. Chen, Z. Q. Zhang, Y. Ma, Y. M. Wang, H. H. Xiao, M. Xu, Y. Y. Huang and G. H. Yuan, Tuning ionic conduction and structure stability of ammonium vanadate by intercalating polyaniline molecules for advanced aqueous zinc-ion batteries, Inorg. Chem. Front., 2023, 10, 1926–1937 RSC.
- Q. Zhang, F. Y. Li and L. Xu, Application of polyoxometalates in third-generation solar cells, Polyoxometalates, 2023, 2, 9140018 CrossRef.
- J. W. Zhang, Y. C. Huang, G. Li and Y. G. Wei, Recent advances in alkoxylation chemistry of polyoxometalates: From synthetic strategies, structural overviews to functional applications, Coord. Chem. Rev., 2019, 378, 395–414 CrossRef CAS.
- J. L. Liu, M. Y. Huang, X. Y. Zhang, Z. Y. Hua, Z. R. Feng, Y. Dong, T. D. Sun, X. Sun and C. X. Chen, Polyoxometalate nanomaterials for enhanced reactive oxygen species theranostics, Coord. Chem. Rev., 2022, 472, 214785 CrossRef CAS.
- Q. H. Zhuang, Z. Q. Sun, C. G. Lin, B. Qi and Y. F. Song, Latest progress in asymmetrically functionalized Anderson-type polyoxometalates, Inorg. Chem. Front., 2023, 10, 1695–1711 RSC.
- C. M. Granadeiro, D. Julião, S. O. Ribeiro, L. Cunha-Silva and S. S. Balula, Recent advances in lanthanide-coordinated polyoxometalates: from structural overview to functional materials, Coord. Chem. Rev., 2023, 476, 214914 CrossRef CAS.
- P. Yin, P. F. Wu, Z. C. Xiao, D. Li, E. Bitterlich, J. Zhang, P. Cheng, D. V. Vezenov, T. B. Liu and Y. G. Wei, A double-tailed fluorescent surfactant with a hexavanadate cluster as the head group, Angew. Chem., Int. Ed., 2011, 50, 2521–2525 CrossRef CAS PubMed.
- C. Wang, J. Ying, H. C. Mou, A. X. Tian and X. L. Wang, Multi-functional photoelectric sensors based on a series of isopolymolybdate-based compounds for detecting different ions, Inorg. Chem. Front., 2020, 7, 3882–3894 RSC.
- H. M. Zeng, W. H. Wu, C. Wang, Z. G. Jiang and C. H. Zhan, Controlled assembly and reversible transformation of tuneable luminescent Mo8-R6G hybrids, Inorg. Chem. Front., 2022, 9, 78–82 RSC.
- N. I. Gumerova and A. Rompel, Synthesis, structures and applications of electron-rich polyoxometalates, Nat. Rev. Chem., 2018, 2, 0112 CrossRef CAS.
- Y. Chen, Z. W. Guo, Y. P. Chen, Z. Y. Zhuang, G. Q. Wang, X. X. Li, S. T. Zheng and G. Y. Yang, Two novel nickel cluster substituted polyoxometalates: syntheses, structures and their photocatalytic activities, magnetic behaviors, and proton conduction properties, Inorg. Chem. Front., 2021, 8, 1303–1311 RSC.
- M. Palacios-Corella, V. Garcia-Lopez, J. C. Waerenborgh, B. J. C. Vieira, G. Minguez Espallargas, M. Clemente-Leon and E. Coronado, Redox and guest tunable spin-crossover properties in a polymeric polyoxometalate, Chem. Sci., 2023, 14, 3048–3055 RSC.
- J. H. Tong, W. H. Wang, L. D. Su, Q. Li, F. F. Liu, W. M. Ma, Z. Q. Lei and L. L. Bo, Highly selective oxidation of cyclohexene to 2-cyclohexene-1-one over polyoxometalate/metal–organic framework hybrids with greatly improved performances, Catal. Sci. Technol., 2017, 7, 222–230 RSC.
- K. Wang, Y. J. Niu, D. Y. Zhao, Y. X. Zhao, P. T. Ma, D. D. Zhang, J. P. Wang and J. Y. Niu, The polyoxovanadate-based Carboxylate Derivative K6H[VV17VIV12(OH)4O60(OOCCH2)4COO)8]·nH2O: Synthesis, crystal structure, and catalysis for oxidation of sulfides, Inorg. Chem., 2017, 56, 14053–14059 CrossRef CAS PubMed.
- H. Y. Zhang, W. L. Zhao, H. Q. Li, Q. H. Zhuang, Z. Q. Sun, D. Y. Cui, X. J. Chen, A. Guo, X. Ji, S. An, W. Chen and Y. F. Song, Latest progress in covalently modified polyoxometalates-based molecular assemblies and advanced materials, Polyoxometalates, 2023, 2, 9140011 Search PubMed.
- A. Banerjee, B. S. Bassil, G. V. Roschenthaler and U. Kortz, Diphosphates and diphosphonates in polyoxometalate chemistry, Chem. Soc. Rev., 2012, 41, 7590–7604 RSC.
- F. Sécheresse, A. Dolbecq, P. Mialane and E. Cadot, {Mo2O2X2}2+ (X=O, S), a magic building block for the design of wheel shaped metalates, C. R. Chim., 2005, 8, 1927–1938 CrossRef.
- J. D. Compain, A. Dolbecq, J. Marrot, P. Mialane and F. Sécheresse, Characterization of tetra, dodeca and tetradeca MoV polyoxometalate wheels structured by etidronate ligands, C. R. Chim., 2010, 13, 329–335 CrossRef CAS.
- A. Banerjee, F. S. Raad, N. Vankova, B. S. Bassil, T. Heine and U. Kortz, Polyoxomolybdodiphosphonates: examples incorporating ethylidenepyridines, Inorg. Chem., 2011, 50, 11667–11675 CrossRef CAS PubMed.
- O. Oms, T. Benali, J. Marrot, P. Mialane, M. Puget, H. Serier-Brault, P. Deniard, R. Dessapt and A. Dolbecq, Fully oxidized and mixed-valent polyoxomolybdates structured by bisphosphonates with pendant pyridine groups: synthesis, structure and photochromic properties, Inorganics, 2015, 3, 279–294 CrossRef CAS.
- D. Liu, B. K. Chen, J. Li, Z. G. Ling, P. H. Li, Y. C. Chi and C. W. Hu, Imidazole-functionalized polyoxometalate catalysts for the oxidation of 5-hydroxymethylfurfural to 2,5-diformylfuran using atmospheric O2, Inorg. Chem., 2021, 60, 3909–3916 CrossRef CAS PubMed.
- T. Zhang, L. K. Yan, S. Cong, W. Guan and Z. M. Su, Prediction of second-order nonlinear optical properties of Wells–Dawson polyoxometalate derivatives [X–C(CH2O)3P2M′3M15O59]6−(X = NO2, NH2, and CH3, M′ = V and Nb, M = W and Mo), Inorg. Chem. Front., 2014, 1, 65–70 RSC.
- X. L. Lu, Y. V. Geletii, T. Cheng and C. L. Hill, Role of multiple vanadium centers on redox buffering and rates of polyvanadomolybdate-Cu(II)-catalyzed aerobic oxidations, Inorg. Chem., 2023, 62, 5822–5830 CrossRef CAS PubMed.
- Y. C. Huang, J. W. Zhang, J. X. Ge, C. Sui, J. Hao and Y. G. Wei, [V4Mo3O14(NAr)3(μ2-NAr)3]2−: the first polyarylimido-stabilized molybdovanadate cluster, Chem. Commun., 2017, 53, 2551–2554 RSC.
- J. C. Liu, J. W. Zhao, C. Streb and Y. F. Song, Recent advances on high-nuclear polyoxometalate clusters, Coord. Chem. Rev., 2022, 471, 214734 CrossRef CAS.
- L. J. Zhang, J. M. Sun, Y. S. Zhou, S. ul Hassan, E. B. Wang and Z. H. Shi, A general synthesis approach in action: preparation and characterization of polyoxomolybdenum(VI) organophosphonates through oxidative Mo–Mo bond cleavage in {MoV2O4}, CrystEngComm, 2012, 14, 4826–4833 RSC.
- A. Saad, G. Rousseau, H. El Moll, O. Oms, P. Mialane, J. Marrot, L. Parent, I.-M. Mbomekallé, R. Dessapt and A. Dolbecq, Molybdenum bisphosphonates with Cr(III) or Mn(III) Ions, J. Cluster Sci., 2013, 25, 795–809 CrossRef.
- A. Saad, N. Anwar, G. Rousseau, P. Mialane, J. Marrot, M. Haouas, F. Taulelle, T. Mc Cormac and A. Dolbecq, Covalent attachment of thiophene groups to polyoxomolybdates or poly-oxotungstates for the formation of hybrid films, Eur. J. Inorg. Chem., 2015, 2015, 4775–4782 CrossRef CAS.
- A. Saad, W. Zhu, G. Rousseau, P. Mialane, J. Marrot, M. Haouas, F. Taulelle, R. Dessapt, H. Serier-Brault, E. Riviere, T. Kubo, E. Oldfield and A. Dolbecq, Polyoxomolybdate bisphosphonate heterometallic complexes: synthesis, structure, and activity on a breast cancer cell line, Chemistry, 2015, 21, 10537–10547 CrossRef CAS PubMed.
- H. Q. Tan, W. L. Chen, D. Liu, Y. G. Li and E. B. N. Wang, Two new cantilever-type polyoxometalates constructed from {Mo2O4}2+ fragments and diphosphonates, Dalton Trans., 2010, 39, 1245–1249 RSC.
- H. Q. Tan, W. L. Chen, D. Liu and E. B. N. Wang, A new molybdophosphate constructed from {MoV2O4(H2O)6}2+ and 1-hydroxyethylidenediphosphonate, J. Cluster Sci., 2010, 21, 147–157 CrossRef CAS.
- R. A. Coxall, S. G. Harris, D. K. Henderson, S. Parsons, P. A. Tasker and R. E. P. Winpenny, Inter-ligand reactions: in situ formation of new polydentate ligands, J. Chem. Soc., Dalton Trans., 2000, 2349–2356 RSC.
- S. S. Amin, K. D. Jones, A. J. Kibler, H. A. Damian, J. M. Cameron, K. S. Butler, S. P. Argent, M. Winslow, D. Robinson, N. J. Mitchell, H. W. Lam and G. N. Newton, Diphosphoryl-functionalized polyoxometalates: structurally and electronically tunable hybrid molecular materials, Angew. Chem., Int. Ed., 2023, 62, e202302446 CrossRef CAS PubMed.
- K. Y. Monakhov, W. Bensch and P. Kogerler, Semimetal-functionalised polyoxovanadates, Chem. Soc. Rev., 2015, 44, 8443–8483 RSC.
- X. X. Zhao, Y. P. Duan, F. Yang, W. Wei, Y. Q. Xu and C. W. Hu, Efficient mechanochemical synthesis of polyoxometalate⊂zif complexes as reusable catalysts for highly selective oxidation, Inorg. Chem., 2017, 56, 14506–14512 CrossRef CAS PubMed.
- L. M. Deusser, J. C. Petzoldt and J. W. Gaube, Kinetic model for methacrolein oxidations-Influence of cesium and vanadium on heteropolyacid catalysts CsxH3-x+y[PMo12-yVyO40], Ind. Eng. Chem. Res., 1998, 37, 3230–3236 CrossRef CAS.
- A. M. Khenkin, L. Weiner, Y. Wang and R. Neumann, Electron and oxygen transfer in polyoxometalate, H5PV2Mo10O40, catalyzed oxidation of aromatic and alkyl aromatic compounds: Evidence for aerobic Mars-van Krevelen-type reactions in the liquid homogeneous phase, J. Am. Chem. Soc., 2001, 123, 8531–8542 CrossRef CAS PubMed.
- I. Efremenko and R. Neumann, Computational insight into the initial steps of the Mars–van Krevelen Mechanism: electron transfer and surface defects in the reduction of polyoxometalates, J. Am. Chem. Soc., 2012, 134, 20669–20680 CrossRef CAS PubMed.
Footnote |
† Electronic supplementary information (ESI) available: Experiment detail, PXRD spectra of 1 and 2, XPS spectra of 1 and 2. CCDC 2277603 and 2277604. For ESI and crystallographic data in CIF or other electronic format see DOI: https://doi.org/10.1039/d3qi01268a |
|
This journal is © the Partner Organisations 2023 |
Click here to see how this site uses Cookies. View our privacy policy here.