DOI:
10.1039/D3VA00358B
(Critical Review)
Environ. Sci.: Adv., 2024,
3, 1097-1110
Bioremediation – the recent drift towards a sustainable environment
Received
22nd November 2023
, Accepted 16th May 2024
First published on 18th May 2024
Abstract
The release of untreated effluents into waterbodies poses a major threat to the environment and human health. The increasing ratio of demands to rate of supply due to the ever-growing population has resulted in the need for large-scale and efficient manufacturing. One of the pitfalls of the fast-paced industrialisation of textiles is the current negligence towards environmental safety and health concerns. Textile dyes, especially azo dyes, are one of the most toxic industrial pollutants. To date, many conventional treatment methods such as aeration lagoons, filtration, sedimentation, flocculation, and coagulation have been used for degradation. Nevertheless, modern techniques such as bioremediation, phytoremediation, and mycoremediation have been proven to be more efficient and feasible due to their eco-friendly nature. Bioremediation is the process of degradation of effluents using microbes. There are two bioremediation strategies: i.e., ex situ and in situ. In situ bioremediation involves the biological degradation of contaminants to benign products onsite. In the ex situ process, pollutants are removed from the contamination site, and then treated. Bioventing, biosparging, bioslurping, land farming, biopiles, and windrows are some techniques that have been in practice. Various microbiological, ecological, and geological factors affect the rate of bioremediation. To achieve accurate results, the maintenance of an optimal functional range is necessary. Technological advancements have led to new remediation techniques, i.e., nanobioremediation. This review includes insights on the impacts of azo dyes; the principles of bioremediation and its strategies, advantages, and limitations; and future prospects involving nanobioremediation.
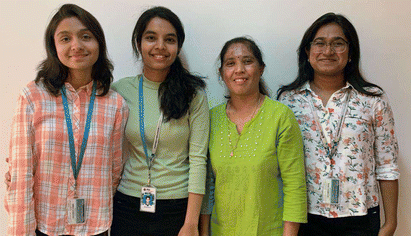 From left, Urvi S. Katti, Prajna R., Kavitha R. V. and Sanjana M. | Sanjana M. is an undergraduate student currently pursuing a Bachelor's degree in Biotechnology (BTech) at PES University, Bengaluru. Throughout her academic journey, she has actively engaged in coursework that spans fundamental principles to advanced applications within the realm of biotechnology. Beyond the classroom, she completed a summer internship in Biocon Biologics in the QC Microbiology Dept. She has been an active participant in club activities and departmental co-curricular activities at her college. In addition to her pursuits in the field of Life Sciences, Sanjana M. is a professionally trained classical singer. She has been learning Carnatic classical music for the last 15 years, and she finished her senior grade examinations in the same. She has actively participated in numerous performances at various venues. Sanjana M. looks forward to the opportunity to contribute to the scientific community and is excited about the potential impact of her work in the field of biotechnology. |
| Prajna R. Prajna R. is an undergraduate student currently pursuing a Bachelor's degree in Biotechnology (BTech) at PES University, Bengaluru. Throughout her undergraduate studies, she has consistently demonstrated good skillsets in her coursework. Recognizing the importance of hands-on experience, she actively engaged in research projects, collaborating with professors and fellow students in cutting-edge studies. She actively participates in the university's club activities and co-curricular events. She also completed a summer internship at Bal Pharma Limited Bangalore in the QC Microbiology department. Beyond her academic and professional pursuits, Prajna R. is a trained classical singer, and has been singing for the last 15 years. She finished her junior grade examinations in Carnatic and Hindustani classical music. She has showcased her performances in various prestigious venues, including All India Radio Bangalore. Prajna R. envisions herself pursuing a master's degree in the field of biotechnology, and is assured to make significant contributions to the field as she embarks on the next chapter of her scientific career. |
| Urvi S. Katti Urvi S. Katti is a dedicated BTech student pursuing biotechnology at PES University, Bengaluru. With a strong grasp of the fundamentals, she is committed to contributing to the field with advanced thinking. Urvi has gained valuable internship experience at Metropolis Diagnostics and Healthcare, where she worked with a diverse range of real-time samples, conducting critical analyses and reviewing various microbial properties. Additionally, she has attended courses on genomics and NGS analysis, as well as workshops on bioinformatics and data sciences, ensuring that she stays updated with the latest technology in the field. Alongside her academic pursuits, Urvi is an enthusiastic participant in cultural activities, and has been professionally trained in Carnatic Music and Bharatanatyam. She finished her junior examinations in the same. With a determination to make a difference in research, Urvi S. Katti is a promising and accomplished student. |
| Kavitha R. V. Prof. R. V. Kavitha obtained her BE, Chemical Engineering Degree from MS Ramaiah Institute of Technology and MTech specialization in Chemical Engineering from Dayananda Sagar College of Engineering, Bangalore. She is presently pursuing her PhD on the topic “Production of bacteriorhodopsin” under the Guidance of Dr Suresh, Professor, Chemical Engineering Department, R. V. College of Engineering, Bangalore. Prof. R. V. Kavitha was the Co-Principal Investigator for MODROBS for the Upgradation of Downstream Process Technology Lab in the Biotechnology Department, funded by AICTE, and also received a fund of Rs. 8 lakhs in the year 2010. She has accumulated a total of 14 years of teaching experience and industrial exposure. She also has published 11 research papers in national and international journals, and 30 papers in national and international conferences. She is a Life Member in the Indian Society for Technical Education and Zero Waste Society. She is also a reviewer/editorial board member for many reputed national and international journals and is a coordinator, responsible for organizing many conferences, faculty development programs, seminars, symposiums, workshops, and guest talks, for biotechnology students. Involved in organizing many industrial visits to Biocon, Pall Life Sciences, Mangalore Fertilizers Ltd, Mangalore, Petroleum Refineries, CFTRI, Mysore, pharma companies in Goa and Bangalore such as Cipla, SmithKline Beecham, Merck Millipore, Sami Labs Kumar Organic Products Ltd, Narayana Hrudayalaya and Netralaya, GRB Foods Pvt. Ltd to BTech Students, which in turn helped them to obtain internships, projects, and placements. She was a convener and a coordinator, responsible for organizing a “National Conference on Emerging Trends in Bioprocessing & Simulation” at the PESIT campus, Bangalore. She was involved every year as a coordinator in the event named Bootstrap, an awareness program for PESU students. Areas of specialization and subjects taught: chemical engineering and biotechnology, metabolic engineering, life sciences, and environmental biotechnology. Biochemical equipment design and drawing using CAED, chemical engineering thermodynamics, momentum transfer, unit operations, bioprocess and bioreaction engineering, biokinetics, lab to industrial scaling, fluid mechanics, mechanical operations, heat transfer, mass transfer, process control and automation, downstream process technology, environmental biotechnology, metabolic engineering, fermentation technology, microbiology, bioprocess calculations, economics and plant design, operations research, and pollution control and safety. |
Environmental significance
Waste management and pollution control are important concerns in today's world. Azo dyes are one of the leading pollutants, and their degradative effects on marine flora, fauna, and the well-being of humans and animals is alarming. Effective, faster, and eco-friendly methods of remediation are the call of the hour. It has been validated that bioremediation is an apt method of remediation due to its massive scope. This review aims to provide a detailed account of the same, highlighting bioremediation strategies, upcoming research areas such as nanobioremediation, and future prospects. Studies were compared by compiling data that address the above topics.
|
Introduction
In the world of molecular biology and ecology, there is an appreciable amount of progress that has led to the discovery of more efficacious microbial processes. Bioremediation is one such accomplishment, and it harnesses certain bacteria that have evolved to exhibit enzymatic properties and possess the capability to significantly break down and decolourise industrial azo dyes.
Bioremediation is the controlled degradation of organic waste with the assistance of bioremediators to decrease waste concentration levels so that they are within the limits pre-established by regulatory boards. Biological agents such as fungi, bacteria, and archaea are used as bioremediators. Hazardous substances are detoxified or degraded through the biodegradative activities of microorganisms. These microbes break down the toxic compounds present in the waste through inclusion in their metabolic processes. Usually, this type of degradation is the result of the collective activity of various organisms. These microbes restore the original environment, and also prevent further pollution.
George M. Robinson, who worked as a petroleum engineer at US Microbes, was the first to use bioremediation on large scale to clean up an oil spill in Santa Barbara, California. For a very long time, natural bioremediation has been used for clearing out waste water, but the targeted and controlled use for degradation of hazardous wastes has only been brought into the spotlight in recent times.
Dyes are an integral part of different industries such as textile, leather, printing, paint, and cosmetics. Incipiently, dyes of only natural origin were used, but with increasing demand for production and cost-effectiveness, synthetically produced dyes were introduced into the market. Azo dyes are synthetically produced organic colourants that contain an R–N
N–R′ functional group. Presently, more than 3000 varieties of azo dyes are available on the market, and they constitute up to 65% of the commercial dye market.41
The problem with these dyes is that they are often disposed without treatment. Because of their chemical composition involving aromatic rings, azoic linkages, and amino groups, azo dyes are very deleterious in nature, and they are persistent in aquatic environments, which affects the quality of water. The residence of azo dyes in water bodies affects the photosynthetic ability of plants by reducing the amount of dissolved oxygen and the penetration of light. They cause the production of chlorophyllase and abscisic acid, which results in the degradation of chlorophyll, and they also reduce the germination rate. Similarly, they affect aquatic fauna by causing stunted growth, and morbidity in gills, liver, intestines, and muscles. Azo dyes are classified as carcinogenic compounds, and because those with nitro groups can be mutagenic, they are harmful to humans and animals. There is no end to industrialisation, and nature has never failed to remediate all the taints caused by these ongoing activities.1
The bacterial degradation of azo dyes typically involves a two-step process. Initially, the azo bonds undergo reductive cleavage by azo reductase enzymes, which results in the formation of toxic aromatic amines. This is followed by the degradation of these amines. Although this process can be implemented under aerobic and anaerobic conditions, aerobic medium is more favourable (Fig. 1).3
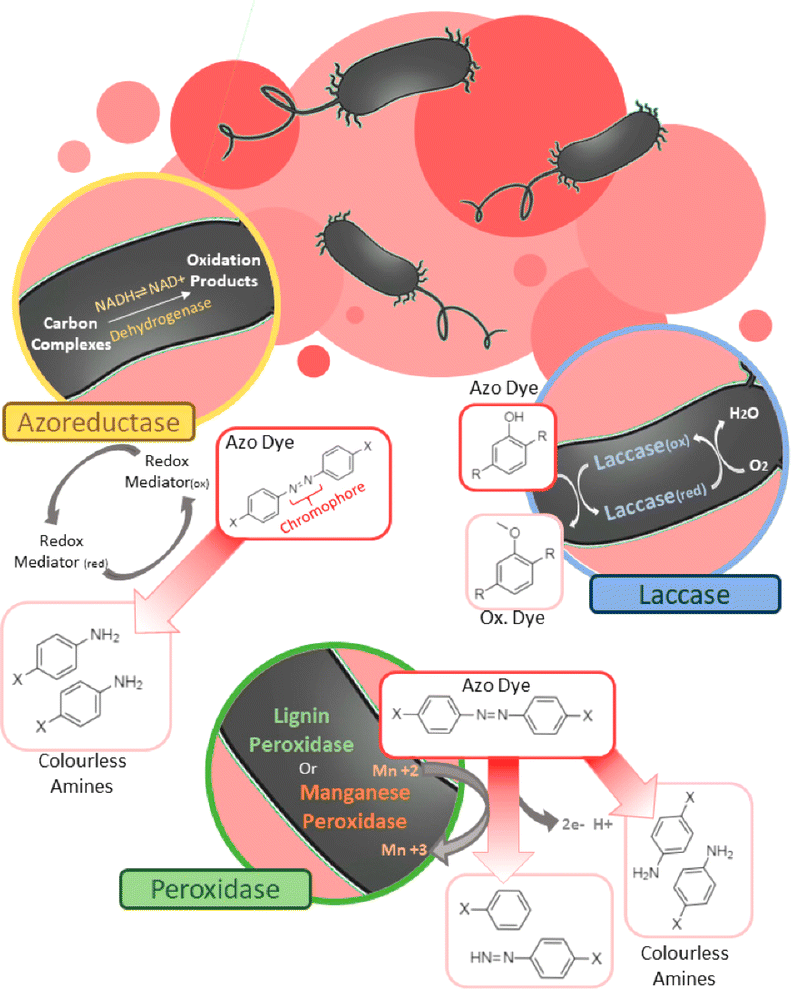 |
| Fig. 1 Three primary bacterial enzymatic mechanisms for degrading azo chromophore groups are illustrated. First, the azoreductases, depicted in yellow, facilitate enzymatic degradation using NADH as a crucial reducing agent to cleave azo bonds. This process generates aromatic amines, which lead to discoloration of the medium. Moving clockwise, we observe the catalytic reaction cycle mediated by laccase, represented in blue. This cycle results in the generation of oxidized substrates, eliminating the formation of potentially toxic amines, and notably, does not require cofactors. Lastly, the green highlighted peroxidase enzymes, including lignin peroxidase and manganese peroxidase, are employed for dye degradation, and play a pivotal role in the overall azo chromophore degradation process.30 | |
Recently a large-scale assessment of the Yamuna River was carried out for major pollutants. Samples from 11 different locations were obtained, and these samples were tested for nitrate, phosphate, chloride, sulphate, and silicon. The levels of pollutants were measured before and after treatment with microalgae, and the results were recorded. Six different algal species were used, and after 30 days of treatment with the selected algae species, a significant reduction in pollutants was observed, with Scenedesmus spp. carrying out the highest reduction in nitrate (86.31%) and phosphate (99.21%). This study highlights the sustainable and green nature of algal systems for environmental waste removal.35
Effects of azo dyes
Impact on marine organisms
Azo dyes are a severe potential risk to the ecosystem and cause numerous health hazards. Due to their highly thermostable and photostable nature, these dyes are resistant to biodegradation and tend to prevail in the atmosphere for long periods of time. High amounts of these dyes in marine bodies disrupt the biological process of photosynthesis by decreasing the reoxygenation capacity of the collecting water and occluding the sunlight so that it does not reach the water surface. Lengthy exposure to azo dyes causes immense amounts of toxicity due to the accumulation of dye deposits in aquatic life forms. The end products of these dyes are mutagenic and carcinogenic, and DNA damage results when they are ingested and metabolised by marine organisms.2
With persistent exposure to these dyes, marine fish are affected by genotoxicity, such as increasing erythrocytic micronuclei formation, which induces detrimental effects on the immune system. The azo dyes profoundly impact water bodies by elevating the chemical and biological demand for oxygen. The toxic components of the azo dye effluents enter the bodies of marine organisms, eventually make their way through the food chain, and ultimately reach humans, causing several somatic disorders such as renal damage, hypertension, and cramps.4
When the fish Cyprinus carpio is exposed to sublethal concentrations of azo dyes, the feeding pattern, absorption rates, and conversion are strongly affected. When we compare feeding rates and effluent concentrations, we see that they are inversely proportional. There is a drastic reduction in the protein, carbohydrate, and lipid content of these fish due to decreased food consumption. The proteinaceous edible eel Mastacembelus armatus is similarly affected, whereby toxic effluents cause an imbalance of ionic concentrations in the vital bodily organs, leading to a decrease in the concentrations of chloride and sodium ions and an increase in calcium, magnesium, and potassium ions. There are other adverse effects on marine organisms, namely, shrunken glomeruli and increased periglomerular space, disruption of the gut microbiome, and disorganisation in the architecture of the gills and lungs.5 Hence, physical, chemical, and biological factors exert their influence on the accumulation of these contaminants by depleting the oxygen concentration and disrupting the biological potency of aquatic biota.4,5
Impact on human health
Azo dyes are compounds that are abundantly found in nature. These dyes are released as effluents into water bodies, and through biomagnification, can reach humans. The impact of these dyes on human health are a great cause for concern because they are linked to splenic sarcomas, bladder cancer, dermatitis, and disorders related to the central nervous system. Benzidine-based dyes are used in paper manufacturing, leather industries, and textile and dye manufacturing industries. These dyes have been found to cause urinary bladder cancer in humans and are tumorigenic in various animals. Workers in these industries often develop occupational asthma, dermatitis, allergic conjunctivitis, and several other allergic reactions. These reactive dyes form immunoglobulin E (IgE) when they react with human serum albumin, which leads to the above-mentioned allergic reactions. Inhalation due to exposure to dust in these industries results in irritation to the eyes and skin.7
The most worrisome factor associated with these dyes is their genotoxicity, and studies have shown their mutagenic potential. The mutagenicity of Disperse Orange 1 dye has caused DNA damage, base pair substitution, and frameshift mutation.24 Another example is Sudan I dye, which is an azo-lipophilic compound. Despite a ban on its use, it can still be found in some food and textile industries. Once it enters the human body, it is enzymatically converted into tumorigenic aromatic amine by the intestinal microbiome.8 Despite the banning of more than 4000 dyes after the establishment of their toxicity, more than 100 of these dyes are still in use in several industries. This is due to the demand for inexpensive raw materials and labour.4,5
Impact on plants
Azo dyes from the textile industries are discharged into water bodies, and this can alter the chemical and biological parameters of the water. This largely impacts the growth of algae by affecting several growth parameters such as pigment concentration and nutrients. One such example is indigo dye, and it has been observed that it reduces the biomass production and overall growth of the freshwater microalga Scenedesmus quadricauda.
Furthermore, these water bodies are often a significant source of irrigation, and they supply water to agricultural sectors in large cities of developing countries. This leads to the accretion of azo dyes in very high concentrations, resulting in the collision and stabilisation of azo dyes with soil particles in the long term, which alters the soil conditions and negatively affects seed germination and overall plant growth. This can be due to dissolved solids in textile effluents, which have notably affected the growth of paddy plants. Some of these dyes are mutagenic and cause a decrease in the levels of dissolved oxygen, which suppresses elongation and complete development of the root and shoot system.46 Rates of arginine ammonification and potential nitrification have greatly decreased with the introduction of azo dyes to the soil. There is a notable reduction in nitrogen fixation and oxidation of ammonia due to the significant reduction of microorganisms such as Nitrobacter that are directly involved in nitrogen transformation processes, and ammonia-oxidising bacteria. The activity of urease enzymes in N cycling has also been observed to gradually decrease due to azo dyes.48,49 Furthermore, high levels of dissolved solids drastically decrease chlorophyll levels, because these solids increase the levels of abscisic acid, and this results in the inhibition of chlorophyll production.4,5
Principles of bioremediation
Bioremediation is a very cost-effective process with a high acceptance rate by the public. This method is not technically demanding, but experience and proficiency are required to implement the process after taking into consideration the conditions of the bioremediation site. After examining the color and appearance of the effluent, native microorganisms with the ability to degrade the azo dyes can be selected and isolated. These isolates can be used for bioremediation, or they can be further modified, which involves genetically engineering these native isolates to increase their biodegradation capacity. This produces hybrid or engineered strains that have an advanced capacity for biodegradation.45
In bioremediation, either indigenous species or species of microbes that are isolated from an area other than the contaminated area can be used. Bioaugmentation is the process of biodegradation using microbial isolates to treat the contaminant. Major bioaugmentation is carried out where there is excess petroleum hydrocarbon contamination. This method is lucrative only when the inoculum contains microbial strains that degrade the pollutants present at that specific site and compete with predators and other abiotic factors. There are numerous factors that affect the proliferation of the desired remediating bacteria, such as the nature, structure, and concentration of the pollutants; the environment of the physical site; and the behaviour of the native microbial species.27
For many years, oil-degrading microorganisms were used to remediate areas contaminated by oil. The reasoning behind this is that the native indigenous species present in the contaminated site are incompetent to degrade the complex oil mixture. Bioaugmentation can also be considered when foreign microorganisms can effectively degrade hydrocarbons at a rapid rate, strive against indigenous species, and survive the exotic environment. The selection of foreign microorganisms that flawlessly satisfy the requirements of bioaugmentation is a pivotal process.50
Enzymatic action on the contaminants is required for bioremediation to occur. Hence, permissible conditions for microbial growth must be present. To meet these requirements, the environmental condition of the contaminated site is often altered accordingly. This brings us to another type of bioremediation referred to as biostimulation. Biostimulation is the process in which the existing bacterium in the native environment is made competent to bioremediate. Biodegradation can be restrained by many factors such as temperature, pH, nutrients, availability of oxygen, and the concentration of the contaminant. Therefore, in the process of biostimulation, the nutrient additives can be either organic or inorganic. The most common nutrient additives are phosphorus, oxygen, nitrogen, and carbon. Natural or synthetic enzymes are used so that the scope of biodegradation is maximised (Fig. 2).
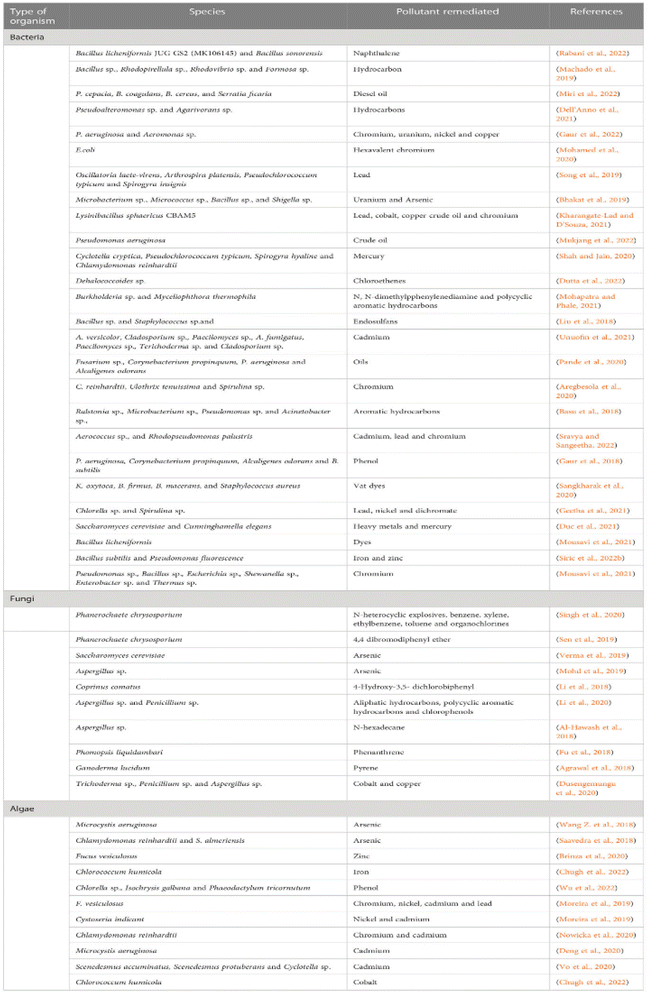 |
| Fig. 2 List of microorganisms used for the bioremediation of various pollutants such as hydrocarbons, dyes, and heavy metals.36 | |
Phytoremediation
Phytoremediation is the degradation of toxic effluents such as textile dyes and heavy metals by the use of photosynthetic plants and their associated microbes.40 It has been observed that species of plants adapt and metabolise toxic contaminants that are present in their environment for their survival. This property of some plant species can be utilised for the greater good, with detoxification carried out because the surface area of plant roots is sufficiently large for absorbing the contaminants and then processing them. Currently, plant-dependent remediation is a new research area.39
The plant root system takes up the prevailing contaminants and provides a substantial surface area. This, in turn, aids the process of mobilisation and detoxification of pollutants, which is brought about by various mechanics such as elimination, containment, and degradation. These characteristic features of plants have been successfully utilised to eradicate azo dyes and colourants along with other contaminants. There is a large impact of factors such as chelating agents, the chemical nature of the pollutants, and root zone interactions on the performance and efficacy of phytoremediation.13
There are many different procedures that can be used for remediation depending on the type of contaminant, i.e., extraction, filtration, stabilisation, degradation, transformation, or volatilization. Organic contaminants such as dyes are mostly removed by degradation, volatilization, or transformation. These processes involve the breakdown of the plant-associated microbial activity (degradation) by plant metabolites (transformation), with subsequent conversion of pollutants into volatile gases and their release into the atmosphere (volatilization) (Table 1).7,15
Table 1 Synopsis and mechanism of phytoremediation techniques applied to decontaminate pollutants. This table has been reproduced from ref. 15 under the following licence: https://creativecommons.org/licenses/by-nc-nd/4.0/
Phyto technique |
Description |
Route of contaminant uptake |
Mechanism |
Applicability |
References |
Phytoextraction |
Translocates and concentrates contaminants from soil via plant roots into harvestable plant parts, e.g., shoots |
Uptake by plant roots |
Absorption or uptake via dissolution in water or through cation pumps; accumulation or sequestration |
Inorganic pollutants, e.g., metals |
McCutcheon and Schnoor51 (2003) |
Marmiroli et al.52 (2006) |
Ali et al.53 (2013) |
Phytofiltration |
Plants with extensive root systems are used for removal of pollutants from water |
Uptake by plant roots (rhizofiltration), young plant seedlings (blastofiltration), or excised plant shoots (caulifiltration) |
Filtration, sorption, or precipitation of pollutants surrounding the root zone |
Inorganic pollutants, e.g., metals |
Marmiroli et al.52 (2006) |
Macek et al.54 (2009) |
Al-Baldawi et al.55 (2013) |
Ali et al.53 (2013) |
Phytostabilization |
Immobilizes pollutants and reduces their bioavailability |
Uptake by plant roots |
Sorption, precipitation, or complexation in the rhizosphere |
Inorganic pollutants, e.g., metals |
Marmiroli et al.52 (2006) |
Macek et al.54 (2009) |
Ali et al.53 (2013) |
Phytotransformation |
Breakdown of organic contaminants through plant metabolic activities or plant enzymes |
Uptake by plant roots or metabolism within the root zone |
Absorption by the root system, resulting in metabolic or enzymatic transformation within or external to plants |
Organic pollutants or xenobiotics |
Marmiroli et al.52 (2006) |
Macek et al.54 (2009) |
Al-Baldawi et al.55 (2013) |
Ali et al.53 (2013) |
Rhizodegradation |
Degrades pollutants by soil-dwelling microbes in the rhizosphere due to stimulation of microbial activity by plant secretions |
Transformation within the root zone |
Secretion of root exudates or enzymes around root zones and subsequent microbial degradation of xenobiotics |
Organic pollutants or xenobiotics |
Al-Baldawi et al.55 (2013) |
Ali et al.53 (2013) |
Phytovolatilization |
Transforms pollutants into volatile forms or gas phases so that they can subsequently be released in the atmosphere through transpiration |
Uptake of water-soluble pollutants by plant roots |
Modification of pollutants during vascular translocation from roots to leaves |
Organic pollutants or xenobiotics |
Marmiroli et al.52 (2006) |
Macek et al.54 (2009) |
Ali et al.53 (2013) |
Mycoremediation
Mycoremediation is an alternative form of bioremediation using different species of fungi. This method is less expensive compared to other methods of biodegradation in practice. Some forms of fungi have been found to be effective under extreme temperatures and radioactive environments where conventional methods fail. Fungi are known to be superior in this process because they produce lignolytic enzymes that play a key role in the degradation of organic pollutants, azo dyes, and heavy metals. Phenol oxidases, peroxidases, azoreductases, and laccases are the major extracellular enzymes produced by fungi that degrade lignin- and cellulose-rich compounds that are excessively present in textile effluents. Phanerochaete chrysosporium, commonly known as white rot fungus, is one such example of a highly creditable organism that is very significant in the degradation process. Fungal biomass is employed to decolourise dyes because of its adsorption properties, which thereby increase the process of dye degradation. It was determined via microscopic diagnosis that fungal spores, but not fungal hyphae, absorb dyes. The dye degradation involves three major processes, namely, biosorption, bioaccumulation, and biodegradation. This absorption process is inversely proportional to the cell mass or enzyme concentrations.31,42
It has been noted that there is synergy between mycoremediation and the process of phytoremediation, which is a plant-based approach. There is a symbiotic relationship between mycorrhizal fungi and plant roots that is beneficial for both organisms.16
Nano bioremediation (NBR)
Nanoparticles (NPs)
Nanoparticles are defined as matter within the size range of 1 to 100 nm in diameter. Due to their much smaller size, their physical and chemical properties dramatically differ when compared to bulk material. Due to their small size and ability to produce quantum effects, nanoparticles are endowed with unique visible properties. Nanoparticles have recently taken the spotlight due to their cost-effectiveness, efficiency, and environmental sustainability. Various methods can be used to synthesize nanoparticles, but biological synthesis is advantageous due to the low cost of production, ease of synthesis, and ability to control the final material dimensions.18,19
Biogenic production of nanoparticles
The biosynthesis of nanoparticles is a bottom-up approach that can be used for inexpensive production. Microbial enzymes and plant phytochemicals that possess reducing or antioxidant properties can assist in breaking down metal compounds into their respective nanoparticles.18
Production of nanoparticles by bacteria
The new biosynthetic plan for nanoparticle production is the use of bacteria and bacterial enzymes to catalyse the reactions that produce nanoparticles. Because of the metal-binding capacity and the S-layer of bacterial cells, they are capable of the synthesis of various metallic nanoparticles such as those composed of gold, platinum, titanium dioxide, silver, and cadmium sulphate. Comparatively pure nanoparticles without the presence of any cellular material can be synthesised in large quantities by extracellular enzymes. Important parameters such as growth conditions, enzymatic processes, and cellular activities can be optimised and regulated to control the properties of the nanoparticles produced. However, further research and study is required to increase our understanding regarding the exact reactions and enzymes involved in this biosynthesis.18,22
Production of nanoparticles by fungi
Satisfactory anabolism of nanoparticles by fungi on a large scale has been observed. The major objective of using fungi to produce nanoparticles is that fungi possess the unique ability to release extracellular enzymes that can control the synthesis of the nanoparticles. Fungi also emit substantial amounts of proteins that boost the production of nanoparticles. Further advancements in the field of genetic engineering can assist in refining the properties of the nanoparticles so that they are economically viable.18,47
Nanobioremediation techniques
Advancements in technology have led to many significant improvements with respect to detoxification of the environment. One example is nanosized zero-valent ions that are able to break down organic pollutants such as chlorpyrifos and atrazine. It has been proven that the efficacy of phytoremediation by plants has been increased by the usage of nanotechnology (Fig. 3).
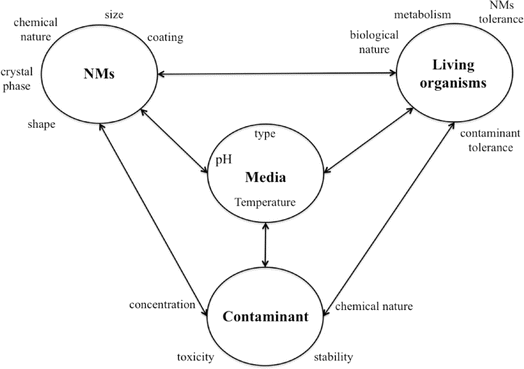 |
| Fig. 3 Various parameters that are responsible for the interactions between nanoparticles (NPs) and living organisms along with the contaminants.20 | |
Nanotechnology has been adopted in the bioremediation process, which is now known as nanobioremediation (NBR). This includes various techniques such as incineration, and physical, chemical, and enzymatic remedies. Microbe- and plant-based remediation are ineffective on certain organic contaminants that show resistance to these methods. Nanotechnology has been able to overcome this drawback by the introduction of enzymatic degradation, which makes use of nanoencapsulated enzymes for the initial degradation.18,19
Nanobioremediation of organic pollutant wastewater treatment
In water, organic chemical pollutants such as dye effluent, petroleum wastewater, antibiotics, and pharmaceuticals pose threats to human health and societal progress. The introduction of nanoparticles significantly enhances the chemical activity and adsorptive capability of surface metals on nanoadsorbents, and primarily contributes to their expanded surface area.37,38 Carbon nanotubes (CNTs) are promising materials for eliminating organic pollutants, and have rapidly become a focal point in research. CNT-based materials are applied in advanced oxidation processes (AOPs) for wastewater treatment, where they exhibit the ability to catalyse various oxidants such as hydrogen peroxide (H2O2), persulfates (PMS/PDS), ozone (O3), and ferrate/permanganate (Fe(VI)/Mn(VII)), which generates additional reactive oxygen species (ROS) to effectively eliminate organic pollutants.33,34
Nano remediation of pollutants
The need for remediating the environment has only been increasing, and it has become one of the major priorities for the sustenance of life. In addition, the developments in the field of technology have also been promising and have led to various methods that can be used to carry out the remediation of soil, wastewater, and groundwater sources.37
Conventional wastewater treatment methods can be improvised and their efficiency increased by nanotechnology. This approach is more promising, can nullify the necessity to use toxic chemicals, and can further upgrade the quality of water so that it is suitable for reuse. For example, removal of Cr(IV) from aqueous solution can be carried out by substituting conventional solvents with polypyrrole–polyaniline (PPy–PANI). Recent studies have shown that nano-sized iron particles and their derivatives exhibit high versatility to carry out this process.18
In a previous study, biosynthesized ZnO particles exhibited improved decolorization efficiency, reaching 95.46% at pH 8. This was attributed to the unique property of the nanoparticles. In contrast, in the decolorization of methylene blue dye, individual potential bacteria demonstrated an efficiency of 89.08%, and a bacterial consortium exhibited a slightly lower efficiency of 76.3%. This proves the efficiency of nanobioremediation.32
Nanoscale elemental or zero-valent metals such as iron, nickel, and palladium have been proven effective in treating contaminated sites, stabilizing metals such as chromium and arsenic, and dehalogenating organic compounds. Because of their tiny size, large surface area, and distinct chemical properties, there has been extensive research on nanoparticles and increasingly common applications (Table 2).28,37
Table 2 Comparison between the different types of bioremediations
Phytoremediation |
Mycoremediation |
Nanobioremediation |
Cost-effective |
Effective on a wide range of contaminants, including heavy metals and textile dyes |
High production costs |
Additional waste is not generated, hence disposal sites are not required |
Only a small number of fungal species have been identified as suitable for remediation |
Highly specific and can target specific pollutants at the molecular level |
Time-consuming |
Faster than phytoremediation |
Rapid remediation |
Low biomass availability and tardy growth |
Requires controlled conditions for optimal results |
Ethical concerns due to the use of engineered materials |
Strategies of bioremediation
In situ bioremediation
This is the process where contaminants are biologically degraded to attenuated products onsite. It is economical with low maintenance, and provides a long-term solution for tainted sites. Some of the techniques in this category include bioaugmentation, biostimulation, bioventing, biosparging, and bioslurping.6,10,13
Bioaugmentation.
This process involves the application of naturally occurring or genetically modified microorganisms, whether native or introduced, to contaminated and hazardous waste sites. This approach aims to expedite the elimination of unwanted compounds from the environment.29 In the process of bioaugmentation, it is necessary to carry out the characterization of microbial strains, and isolation and optimization of microorganisms.9,43
Biostimulation.
Biostimulation stands out as a highly effective, economical, and environmentally friendly remediation method. This technique involves introducing essential nutrients such as phosphorus, nitrogen, oxygen, and electron donors to heavily polluted sites, with the aim of activating existing bacteria to break down hazardous and toxic contaminants. In the realm of bioremediation, biostimulation is widely recognized as the most efficient approach for addressing hydrocarbon pollutants. The existing literature underscores the significance of biostimulation as a crucial tool in the remediation of hydrocarbons, particularly those stemming from petroleum products and their derivatives (Fig. 4).26,29
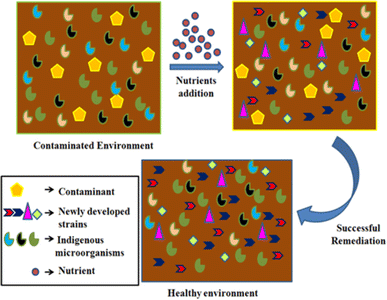 |
| Fig. 4 Illustration of the biostimulation process.29 | |
Bioventing.
Bioventing is a process whereby a controlled supply of airflow to the unsaturated regions of tainted areas stimulates the activity of indigenous bacteria. This process maintains a low air-flow rate and prevents volatility of the contaminants to the atmosphere. This technique has attained popularity amongst other in situ bioremediation techniques for remediating sites where there are light spilled petroleum products. Air injection rates and air flow rates are the key parameters of this process. Because this process eliminates the process of excavation and transportation costs of the contaminants, it favours inclination to the in situ method if constructively practiced.6,13
Biosparging.
Biosparging is a process analogous to bioventing. It involves supplying air to the saturated subsurface of the soil to revive the microbial action. Basically, the air in the saturated surface induces the movement of volatile organic compounds from the saturated surface to the unsaturated surface for effective biodegradation. The prime factors that will increase the efficacy of biosparging are soil permeability and soil biodegradability. In situ air sparging (IAS) is a process correlated to biosparging that requires high air flow rates for volatilisation of pollutants. It was previously reported that a greater than 70% overall decrease in BTEX (benzene, toluene, ethylbenzene, and xylene) indicates that biosparging as a process can be used to bioremediate BTEX-contaminated water.25 However, the air flow direction is highly unanticipated.6,13
Bioslurping.
Bioslurping is the amalgamation of three different processes, namely, bioventing, vacuum-enhanced pumping, and solid vapour extraction to achieve the remediation of soil and water by supplying indirect oxygen. Light non-aqueous phase liquid (LNAPL) recoveries are the major highlight of this technique, which is used for remediating saturated, unsaturated, and capillary zones as well. This system uses a ‘slurp’ tube that draws up the liquids from the free product layer. LNAPLs drift to the surface due to this pumping action, and thus are removed from the air and water. Apart from the complete removal of the free products, the system can further be transformed to the prevailing bioventing system for the execution of the remaining remediation process. One major concern associated with this technique is that excess moisture inhibits the permeability of the air, and it is difficult to obtain a vacuum on a deep, highly permeable site.13
Ex situ bioremediation
In this strategy of bioremediation, pollutants are removed from the contamination site, and then treated. Even after disruption occurs at the site, this technique is very effective because it provides greater control of the overall process.6,10,13
Land farming.
This is one of the simplest bioremediation techniques because it involves lesser equipment and is inexpensive. It involves the excavation of the pollutants, which may be followed by tilling. Tilling, irrigation, and the addition of nutrients are the important steps in this method. Tilling assists in the aeration of the soil, which increases the growth of aerobic bacteria and thus the rates of bioremediation. However, the additional excavation cost, larger operating area, and the subsequent reduction in microbial activity are the major disadvantages of this method.6,13
Biopiles.
In this practice, polluted soil is excavated and stacked above the ground, after which microbial activities are amplified to strengthen the bioremediation process. To achieve this, the soil undergoes aeration and is subjected to irrigation. Provisions are made for nutrient collection and beds for treatment. This is a preferred technique from an economic standpoint, and the bioremediation process can be improvised with this method by controlling all the other factors such as irrigation, nutrition, and aeration. The rate of biodegradation can be augmented with optimal hot air injection. In addition, this method is optimal for the remediation of large volumes of polluted soil in a confined space.
Comparative studies have been performed to determine the feasibility of biopiles in clay and sandy soils. Studies have reported low TPH levels in humidified biopiles due to ideal moisture content and reduced leaching. On the contrary, higher TPH concentrations have been reported in heated biopiles. Agents such as sawdust or wood chips or other organic materials are added to biopiles to boost the remediation process.
Despite the various advantages offered, the drawbacks of biopiles include a high maintenance cost, and the paucity of power supplies in outlying areas that are needed to enable the distribution of air in contaminated piles. Moreover, the overheating of air will hinder microbial activities, thus affecting bioremediation.6,13
Windrows.
This method incorporates other methods that increase the activity of hydrocarbonoclastic bacteria. This is brought about by sporadic turning of polluted soil, augmented aeration, and uniform nutrient distribution, which in turn lead to the enhancement of bacterial degradation or bioremediation. In comparison with biopile treatment methods, hydrocarbon removal is more effective and occurs at a much higher rate in windrow treatment. It was later reported that this was due to the pulverulent form of soil being used. This method of treatment also causes the progression of anaerobic zones within the soil pile, which releases methane and impedes aeration.13
Solid phase bioremediation.
This method involves the excavation of contaminated soil and arranging it into piles. Organic waste such as leaves, and agricultural, domestic, and industrial waste is incorporated. The bacterial growth within these piles is facilitated through a network of pipes for air circulation.44
Bioreactor.
A bioreactor is a vessel or an enclosed system in which unprocessed products are converted into a desired biochemical product. The extensiveness and the rate of the biodegradation is comparatively higher than that in situ remediation, as the outcomes of the instrument are easily tractable, and thereby can increase the efficacy and decrease the duration of the bioremediation. This process is also called slurry phase bioremediation. A wide range of modes are available for the operation of the bioreactor such as fed batch, batch, sequencing batch, multistage, and continuous.
The key highlight of bioremediation using bioreactors is that they have an excellent responsiveness towards the environment to promote an optimum level of growth. Substrate and inoculum concentrations, agitation and aeration rates, and pH and temperature play a vital role in the success of this apparatus. Although there are a few constraints in the process of bioremediation, they can be nullified with a bioreactor by modification of those limitations. Bioreactors are used to treat contaminated soil and water, which consist of toxic volatile organic compounds (VOCs). Genetically engineered organisms can be used for the biodegradation of various pollutants and then destroyed before the soils are sent to the landfill. This process of destruction of exotic modified genes prevents them from escaping into the surroundings. However, the downside of bioreactors is that they require a high capital investment and workforce.6,13
Factors affecting bioremediation
As discussed, the restoration of the environment can be carried out by microbial bioremediation, which depends on numerous factors. The rates of bioremediation are directly proportional to the bacterial presence in the contaminated area. It has been discovered that new bacterial strains possess a plasmid-linked ability to participate in degradation, in addition to their remarkable ability to adapt and sustain themselves in extreme or harsh habitats. One of the biggest limiting factors for microbial degradation is inter- and intraspecies competition. Usually, there is competition for the utilisation of the limited nutrients, and also in certain cases, some microbial species release metabolites that inhibit the growth of other species of microbes residing in the same environment. Hence, to devise a plan with the greatest suitability for bioremediation, a proper understanding of the bacterial community dynamics is very much necessary.12,13
Bioremediation is associated with various microbiological, ecological, and geological factors. The most predominant factor associated with bioremediation is the capacity of the organic material to provide sufficient energy for an anaerobic organism to perform the necessary degradation. This is affected by the oxidation state of carbon in the material, the lesser being more energetically efficient and reliable.10,11
Along with favourable environmental conditions, the bacterial characteristics of being Gram negative or Gram positive also exert a considerable effect. There is a need for accurate and precise identification of substrate specificity, microbial colony identification, and designing. Additionally, contaminants play a major role in altering the structure of the bacterial colonies. Some communities of bacteria form an extracellular layer of polymers surrounding them for protection, which is called a biofilm. It has been observed that the formation of a biofilm enhances the ability of the community to biodegrade.14
Along with the above-mentioned biotic factors, there are additional abiotic criteria that affect the rate of biodegradation. The nature of the contaminant is the most major one. The physical and chemical properties of these contaminants such as their concentration, molecular weight, composition, structure, and toxicity affect the biological activity of these bacterial communities. A higher concentration of contaminants can be toxic and can inhibit or limit the growth and activity of the bioremediators. Also, a very low concentration of these contaminants can suppress the activation of the genes responsible for the degradation, subsequently leading to the decreased availability or unavailability of the degrading enzymes. Hence, an optimum concentration of contaminants must be present for effective degradation.13
There are also a number of non-technical criteria that affect microbial degradation, such as the attainment of cleanup targets, cost-efficiency, risks associated with residual effluents, space limitations, and devising a pragmatic solution on the whole.12–14
Advantages of bioremediation
• Bioremediation is a natural process, and is recognised as an approved alternative for the treatment of pollutants.
• These pollutants naturally augment the growth of the microorganisms required to carry out this process, thus reducing the requirement for external aids.
• This process facilitates disposing or discarding of waste by degrading it and decreasing its toxicity.
• Bioremediation can be accomplished at sites, thus causing little interference with normal activities. As a result of this, the necessity of transport and hazards to human health associated with transportation is avoided.
• Bioremediation is a biological process, and because it requires very minimal cost, it is hence a very economical alternative.
• In situ bioremediation techniques do not require excavating polluted soil.
• The treatment of subsurface pollution is carried out more rapidly with in situ methods as compared to other pumping methods.
Disadvantages of bioremediation
• This process remains restricted only to biodegradable materials.
• There is the possibility that some of the products obtained will be more noxious than the primary compound.
• The completion of the entire process takes a long time compared to other available alternatives.
• Bioremediation is a biological process, and thus, demands a competent microbial population and a favourable environment.17
Prospects of bioremediation
From the preceding discussions, the process of bioremediation with its intrinsic techniques has proven to be highly productive in remediating contaminated sites with the aid of microorganisms, which play a critical role in the process. Genomics, proteomics, transcriptomics, and metabolomics have elevated the aspects of microbial bioremediation. Metagenomics promotes the identification of uncultured microorganisms present at the tainted site, which overpowers the early approach. Procuring genome sequences is a potential advancement in recent times that assists in understanding the metabolic functioning of the organism at cellular level. Amelioration of omics tools will certainly result in a prolific yield in the field of bioremediation. Biostimulation and bioaugmentation are two major processes that open doors for effective remediation practices. A combination of both these processes has been proven to be very successful in removing pollutants such as polyaromatic hydrocarbons (PAHs) of lower molecular weight. Furthermore, using an amalgamation of different microorganisms can assist in cleaning up the polluted environment at a faster rate as compared to using pure cultures, due to the symbiotic interactions in the environment. Biosurfactants are said to increase the accessibility of pollutants and degrade the pollutants that have existed for a long period of time by the mechanism of desorption and solubilization.13
Genetically engineered microorganisms (GEMs) are an optimistic approach in the field of bioremediation. A biocatalyst can be designed that can degrade a specific pollutant of interest by altering the metabolic and catabolic pathways, and by increasing the scope of the substrate. Metabolic pathways can be modified and extended to a wide range of unfavourable compounds that cannot otherwise be degraded by wild species, thereby increasing the degradation efficacy. The enzyme substrate specificity and enzymatic activity that aid in the degradation of specific pollutants are altered in some GEMs. The use of lux gene-based systems and GEM to produce bioluminescence assists in understanding the extent of these bioremediation processes. All the above-mentioned parameters can be used to produce genetically engineered strains that are sufficiently competent.13,23
Nanotechnology emerges as a groundbreaking discipline that serves as an intermediary in the realm of bioremediation. It engenders nano-encapsulated enzymes, which are characterized by their heightened stability and resilience against impromptu degradation. Consequently, this augmentation extends the half-life of enzymes and concurrently diminishes the costs associated with the bioremediation process, as encapsulated enzymes can be judiciously utilized multiple times. A paramount function of nanoremediation lies in its capacity to discern pollutants and contaminated sites through the adept utilization of nano-sensors.19
Zinc, copper, and silver nanoparticles have been empirically validated for their efficacious outcomes in the decomposition of organic dyes and other bioactive phytochemicals into less deleterious forms. Collectively, the potency of the bioremediation process undergoes a substantial surge when concurrently deploying the aforementioned remedial methodologies.13,18,21
Conclusion
Effluents containing textile dyes are currently a major source of pollution. The release of such untreated effluents into soil and water bodies is very detrimental to the environment, as well as human health. Azo dyes are the most widely used textile dyes, and they pose a severe threat because they have been found to be carcinogenic to humans and various animals, and they reduce the biomass and overall growth in aquatic plants. This issue can be resolved via advanced decolorization technologies. One of them is bioremediation, and it is a green alternative to traditional treatment technologies because it is a more cost-effective, non-toxic, and safer method. Treatment of the pollutants can be carried out without excavation through this process, and the need for external aids is reduced. Upcoming bioremediation technologies such as nanobioremediation have the potential to completely revolutionise the existing methods, with cost-effectiveness, feasibility, and improved performance. This technology is proving to be a more sustainable method, with an almost zero-emission approach. Overall, the advancement of research in the area of bioremediation and nanobioremediation and efforts made to rectify the cons prove that this method promises to be a more sustainable, feasible, cost-effective, and high-performance method of remediation.6,13
Abbreviations
IgE | Immunoglobulin E |
NBR | Nanobioremediation |
PPy–PANI | Polypyrrole–polyaniline |
PCBs | Polychlorinated biphenyls |
DDT | Dichlorodiphenyltrichloroethane |
TNT | Trinitrotoluene |
NDMA |
N-Nitrosodimethylamine |
VOCs | Volatile organic compounds |
GEM | Genetically engineered microorganisms |
Author contributions
PR substantially contributed to the data collection and analysis; SM and USK have critically revised the intellectual content; and RVK approved and revised the versions of the manuscript. All authors have equally contributed in the drafting of manuscript, reviewing the results, and approving the final version of the manuscript.
Conflicts of interest
The corresponding author confirms on behalf of all the other authors that there are no criteria that might raise an issue or question of bias in the above paper.
Acknowledgements
The authors endorse the Department of Biotechnology, PES University, Bangalore, India.
References
- M. Ikram, M. Naeem, M. Zahoor, A. Rahim, M. M. Hanafiah, A. A. Oyekanmi, A. B. Shah, M. H. Mahnashi, A. Al Ali, N. A. Jalal and F. Bantun, Biodegradation of azo dye methyl red by pseudomonas aeruginosa: Optimization of process conditions, Int. J. Environ. Res. Public Health, 2022, 19(16), 9962 CrossRef CAS PubMed.
- L. H. Ahlström, C. S. Eskilsson and E. Björklund, Determination of banned azo dyes in consumer goods, TrAC, Trends Anal. Chem., 2005, 24(1), 49–56 CrossRef.
- L. R. Pinheiro, D. G. Gradíssimo, L. P. Xavier and A. V. Santos, Degradation of azo dyes: bacterial potential for bioremediation, Sustainability, 2022, 14(3), 1510 CrossRef CAS.
-
N. Puvaneswari
J. Muthukrishnan, P. Gunasekaran, Toxicity Assessment and Microbial Degradation of Azo, Dyes, 2006 Search PubMed.
- S. Gita, A. Hussan and T. G. Choudhury, Impact of textile dyes waste on aquatic environments and its treatment, Environ. Ecol., 2017, 35(3C), 2349–2353 Search PubMed.
- M. Vidali, Bioremediation. an overview, Pure Appl. Chem., 2001, 73(7), 1163–1172 CAS.
- B. Lellis, C. Z. Fávaro-Polonio, J. A. Pamphile and J. C. Polonio, Effects of textile dyes on health and the environment and bioremediation potential of living organisms, Biotechnol. Res. Innov., 2019, 3(2), 275–290 CrossRef.
- K. Rehman, T. Shahzad, A. Sahar, S. Hussain, F. Mahmood, M. H. Siddique, M. A. Siddique and M. I. Rashid, Effect of Reactive Black 5 azo dye on soil processes related to C and N cycling, PeerJ, 2018, 6, e4802 CrossRef PubMed.
- I. P. Thompson, C. J. Van Der Gast, L. Ciric and A. C. Singer, Bioaugmentation for bioremediation: the challenge of strain selection, Environ. Microbiol., 2005, 7(7), 909–915 CrossRef CAS PubMed.
- Y. Luka, B. K. Highina and A. Zubairu, Bioremediation: A solution to environmental pollution-a review, Am. J. Eng. Res., 2018, 7(2), 101–109 Search PubMed.
- J. Srivastava, R. Naraian, S. J. Kalra and H. Chandra, Advances in microbial bioremediation and the factors influencing the process, Int. J. Environ. Sci. Technol., 2014, 11, 1787–1800 CrossRef CAS.
- R. Boopathy, Factors limiting bioremediation technologies, Bioresour. Technol., 2000, 74(1), 63–67 CrossRef CAS.
- C. C. Azubuike, C. B. Chikere and G. C. Okpokwasili, Bioremediation techniques–classification based on site of application: principles, advantages, limitations and prospects, World J. Microbiol. Biotechnol., 2016, 32, 1–8 CrossRef CAS PubMed.
- G. Kebede, T. Tafese, E. M. Abda, M. Kamaraj and F. Assefa, Factors influencing the bacterial bioremediation of hydrocarbon contaminants in the soil: mechanisms and impacts, J. Chem., 2021, 2021, 1–7 CrossRef.
- U. Tahir, A. Yasmin and U. H. Khan, Phytoremediation: Potential flora for synthetic dyestuff metabolism, J. King Saud Univ. Sci., 2016, 28(2), 119–130 CrossRef.
- L. Singh, Biodegradation of synthetic dyes: a mycoremediation approach for degradation/decolourization of textile dyes and effluents, J. Appl. Biotechnol. Bioeng., 2017, 3(5), 430–435 Search PubMed.
-
I. Sharma, Bioremediation techniques for polluted environment: concept, advantages, limitations, and prospects, in Trace Metals in the Environment-New Approaches and Recent Advances, IntechOpen, 2020 Search PubMed.
- G. Pandey, Prospects of nanobioremediation in environmental cleanup, Orient. J. Chem., 2018, 34(6), 2838 Search PubMed.
- K. K. Yadav, J. K. Singh, N. Gupta and V. J. Kumar, A review of nanobioremediation technologies for environmental cleanup: a novel biological approach, J. Mater. Environ. Sci., 2017, 8(2), 740–757 CAS.
- E. Vázquez-Núñez, C. E. Molina-Guerrero, J. M. Peña-Castro, F. Fernández-Luqueño and M. G. de la Rosa-Álvarez, Use of nanotechnology for the bioremediation of contaminants: A review, Processes, 2020, 8(7), 826 CrossRef.
-
M. Mishra, S. K. Singh and A. Kumar, Role of omics approaches in microbial bioremediation, in Microbe Mediated Remediation of Environmental Contaminants, Woodhead Publishing, 2021, vol. 1, pp. 435–445 Search PubMed.
-
V. N. Kalpana and V. D. Rajeswari. Biosynthesis of metal and metal oxide nanoparticles for food packaging and preservation: a green expertise, in Food biosynthesis, Academic Press, 2017, vol. 1, pp. 293–316 Search PubMed.
- S. Kulshreshtha, Genetically engineered microorganisms: a problem solving approach for bioremediation, J. Biorem. Biodegrad., 2013, 4(4), 1–2 Search PubMed.
- F. M. Chequer, J. P. Angeli, E. R. Ferraz, M. S. Tsuboy, J. C. Marcarini, M. S. Mantovani and D. P. de Oliveira, The azo dyes Disperse Red 1 and Disperse Orange 1 increase the micronuclei frequencies in human lymphocytes and in HepG2 cells, Mutat. Res. Genet. Toxicol. Environ. Mutagen., 2009, 676(1–2), 83–86 CrossRef CAS PubMed.
- C. M. Kao, C. Y. Chen, S. C. Chen, H. Y. Chien and Y. L. Chen, Application of in situ biosparging to remediate a petroleum-hydrocarbon spill site: Field and microbial evaluation, Chemosphere, 2008, 70(8), 1492–1499 CrossRef CAS PubMed.
- G. O. Adams, P. T. Fufeyin, S. E. Okoro and I. Ehinomen, Bioremediation, biostimulation and bioaugmention: a review, Int. J. Environ. Biorem. Biodegrad., 2015, 3(1), 28–39 CAS.
- V. M. Kensa, Bioremediation-an overview, J. Ind. Pollut. Control, 2011, 27(2), 161–168 CAS.
- P. Bhatt, S. C. Pandey, S. Joshi, P. Chaudhary, V. M. Pathak, Y. Huang, X. Wu, Z. Zhou and S. Chen, Nanobioremediation: A sustainable approach for the removal of toxic pollutants from the environment, J. Hazard. Mater., 2022, 427, 128033 CrossRef CAS PubMed.
- M. Goswami, P. Chakraborty, K. Mukherjee, G. Mitra, P. Bhattacharyya, S. Dey and P. Tribedi, Bioaugmentation and biostimulation: a potential strategy for environmental remediation, J. Microbiol. Exp., 2018, 6(5), 223–231 Search PubMed.
- L. R. Pinheiro, D. G. Gradíssimo, L. P. Xavier and A. V. Santos, Degradation of azo dyes: bacterial potential for bioremediation, Sustainability, 2022, 14(3), 1510 CrossRef CAS.
- S. O. Akpasi, I. M. Anekwe, E. K. Tetteh, U. O. Amune, H. O. Shoyiga, T. P. Mahlangu and S. L. Kiambi, Mycoremediation as a Potentially Promising Technology: Current Status and Prospects—A Review, Appl. Sci., 2023, 13(8), 4978 CrossRef CAS.
- S. Modi, V. K. Yadav, A. Amari, H. Osman, C. A. Igwegbe and M. H. Fulekar, Nanobioremediation: A bacterial consortium-zinc oxide nanoparticle-based approach for the removal of methylene blue dye from wastewater, Environ. Sci. Pollut. Res., 2023, 30(28), 72641–72651 CrossRef CAS PubMed.
- L. Thangavelu and G. R. Veeraragavan, A survey on nanotechnology-based bioremediation of wastewater, Bioinorg. Chem. Appl., 2022, 2022, 1–12 Search PubMed.
- J. Peng, Y. He, C. Zhou, S. Su and B. Lai, The carbon nanotubes-based materials and their applications for organic pollutant removal: A critical review, Chin. Chem. Lett., 2021, 32(5), 1626–1636 CrossRef CAS.
- D. Kumar, S. Agrawal and D. Sahoo, Assessment of the intrinsic bioremediation capacity of a complexly contaminated Yamuna River of India: a algae-specific approach, Int. J. Phytorem., 2023, 21, 1–5 Search PubMed.
- M. S. Ayilara and O. O. Babalola, Bioremediation of environmental wastes: the role of microorganisms, Front. agron., 2023, 5, 1183691 CrossRef.
- I. Cecchin, K. R. Reddy, A. Thomé, E. F. Tessaro and F. Schnaid, Nanobioremediation: Integration of nanoparticles and bioremediation for sustainable remediation of chlorinated organic contaminants in soils, Int. Biodeterior. Biodegrad., 2017, 119, 419–428 CrossRef CAS.
- M. D. Rizwan, M. Singh, C. K. Mitra and R. K. Morve, Ecofriendly application of nanomaterials: nanobioremediation, Int. J. Nanoparticles, 2014, 1–7 Search PubMed.
- E. E. Etim, Phytoremediation and its mechanisms: a review, Int. J. Environ. Bioenergy, 2012, 2(3), 120–136 Search PubMed.
- S. D. Cunningham and D. W. Ow, Promises and prospects of phytoremediation, Plant Physiol., 1996, 110(3), 715 CrossRef CAS PubMed.
- D. Rawat, V. Mishra and R. S. Sharma, Detoxification of azo dyes in the context of environmental processes, Chemosphere, 2016, 155, 591–605 CrossRef CAS PubMed.
- V. Prakash, Mycoremediation of environmental pollutants, Int. J. Chem. Tech. Res., 2017, 10(3), 149–155 CAS.
- J. Pandey, A. Chauhan and R. K. Jain, Integrative approaches for assessing the ecological sustainability of in situ bioremediation, FEMS Microbiol. Rev., 2009, 33(2), 324–375 CrossRef CAS PubMed.
- S. B. Larsen, D. Karakashev, I. Angelidaki and J. E. Schmidt, Ex-situ bioremediation of polycyclic aromatic hydrocarbons in sewage sludge, J. Hazard. Mater., 2009, 164(2–3), 1568–1572 CrossRef CAS PubMed.
- A. Kumar, B. S. Bisht, V. D. Joshi and T. Dhewa, Review on bioremediation of polluted environment: a management tool, Int. J. Environ. Sci., 2011, 1(6), 1079–1093 Search PubMed.
- T. I. Khan and V. Jain, Effect of textile industry waste water on the growth and some biochemical parameters on Triticum aestivum, J. Environ. Pollut., 1995, 2, 47–50 Search PubMed.
- N. Durán, P. D. Marcato, M. Durán, A. Yadav, A. Gade and M. Rai, Mechanistic aspects in the biogenic synthesis of extracellular metal nanoparticles by peptides, bacteria, fungi, and plants, Appl. Microbiol. Biotechnol., 2011, 90, 1609–1624 CrossRef PubMed.
-
P. J. Colberg and L. Y. Young, Anaerobic degradation of nonhalogenated homocyclic aromatic compounds coupled with nitrate, iron, or sulfate reduction, Microbial Transformation and Degradation of Toxic Organic Chemicals, 1995, p. 307330 Search PubMed.
- J. G. Mueller, C. E. Cerniglia and P. H. Pritchard, Bioremediation of environments contaminated by polycyclic aromatic hydrocarbons, Biotechnol. Res. Ser., 1996, 6, 125–194 CAS.
- F. M. Ghazali, R. N. Rahman, A. B. Salleh and M. Basri, Biodegradation of hydrocarbons in soil by microbial consortium, Int. Biodeterior. Biodegrad., 2004, 54(1), 61–67 CrossRef CAS.
-
S. C. McCutcheon and J. L. Schnoor, Phytoremediation: transformation and control of contaminants, John Wiley & Sons, 2004 Search PubMed.
-
N. Marmiroli, M. Marmiroli and E. Maestri, Phytoremediation and phytotechnologies: a review for the present and the future, Soil and water pollution monitoring, protection and remediation, 2006, pp. 403–416 Search PubMed.
- H. Ali, E. Khan and M. A. Sajad, Phytoremediation of heavy metals—concepts and applications, Chemosphere, 2013, 91(7), 869–881 CrossRef CAS PubMed.
-
T. Macek, O. Uhlik, K. Jecna, M. Novakova, P. Lovecka, J. Rezek, V. Dudkova, P. Stursa, B. Vrchotova, D. Pavlikova and K. Demnerova, Advances in phytoremediation and rhizoremediation, in Advances in Applied Bioremediation, 2009, pp. 257–277 Search PubMed.
- I. A. Al-Baldawi, S. R. Abdullah, F. Suja, N. Anuar and I. Mushrifah, Comparative performance of free surface and sub-surface flow systems in the phytoremediation of hydrocarbons using Scirpus grossus, J. Environ. Manage., 2013, 130, 324–330 CrossRef PubMed.
|
This journal is © The Royal Society of Chemistry 2024 |
Click here to see how this site uses Cookies. View our privacy policy here.