Enhancing community well-being in African drylands through technology-based solutions in the water–energy–food–ecosystems nexus
Received
4th July 2023
, Accepted 22nd October 2023
First published on 23rd October 2023
Abstract
The environmental challenges in African drylands, including water scarcity, limited energy access, and food shortages, are interconnected and have significant implications for the region's sustainability and the well-being of its communities. To address these issues, comprehensive and sustainable management plans are urgently needed. This critical review delves into the water–energy–food–ecosystem (WEFE) nexus, emphasizing the importance of integrated technologies to improve water and energy efficiency while mitigating environmental impacts. Key databases were systematically reviewed, including ScienceDirect, Scopus, and ProQuest as well as various international documents, such as those accessible in the FAO Corporate Document Repository. Our emphasis was on WEFE technologies, their applications, research outcomes, and relevance in African arid regions. The study examined recent decade initiatives to bridge the research gap, emphasizing the need to consider interrelationships and broader impacts of technical solutions in water, energy, and food sectors. This analysis brought together natural and technological advancements within the WEFE nexus, yielding valuable insights. By considering significant case studies addressing WEFE challenges, we ascertained the substantial potential of WEFE nexus technologies in mitigating African dryland challenges. Additionally, the critical factors that significantly influence the adoption of these transformative technologies are identified. The outcomes of this study underscore the importance of proactively integrating innovative WEFE technologies within the natural based solutions. Closing knowledge gaps, integrating solutions, and fostering innovation are essential steps in addressing the complex challenges these regions face. These efforts lead to enhanced water, energy, and food systems, fostering regional sustainability and resilience, ultimately securing a more promising and sustainable future for vulnerable communities.
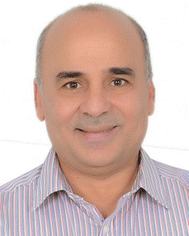 Thameur Chaibi | Thameur Chaibi has worked for nearly three decades in the fields of science, technology, higher education, and research. His career has been marked by significant roles, including positions as a senior expert at the African Union Commission (AUC) and GIZ. Currently, he serves as the Director of Research at the National Research Institute for Agricultural Engineering, Water, and Forestry (INRGREF). He holds a PhD in Agricultural Engineering and Climate Technologies from (SLU) in Sweden. He actively contributes to various research projects in the areas of water-energy-food security nexus and agricultural innovation and technologies. He is follow of the World Academy of Sciences (TWAS), the African Academy of Sciences (AAS), the Islamic World Academy of Sciences (IAS), and the French Water Academy. |
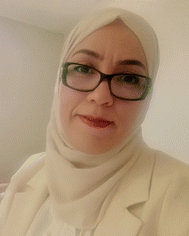 Meriem Soussi | Meriem Soussi obtained her PhD in Energy Engineering (Energy Systems optimization) since 2016 at the National School of Engineering of Tunis. She is currently a Researcher at the National Research Institute for Rural Engineering, Water, and Forestry (INRGREF). With a career spanning over ten years in higher education, her primary research interests revolve around energy processes used in Agriculture and innovative renewable energy applications. Additionally, she actively participates in projects addressing the Water-Energy-Food-Energy (WEFE) Nexus issue. |
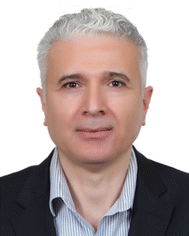 Ali Karnib | Ali Karnib is a water resources expert with a focus on sustainable development. He has worked with the United Nations and other international organizations on projects related to integrated water resources management, the water-energy-food-ecosystems Nexus, and climate change mitigation and adaptation. He has published over 60 scientific articles and international reports and serves on the editorial board of the Water Resources Management Journal. |
Water impact
The paper emphasizes the promotion of water, energy, food, and ecosystem (WEFE)-based technologies for arid areas in Africa. It advocates for a comprehensive and integrated approach that aims to enhance water efficiency, promote conservation measures, advance water treatment methods, foster sustainable agricultural practices, harness the potential of renewable energy sources, and improve both environmental sustainability and the overall well-being of communities.
|
1. Introduction
Dryland ecosystems are mainly characterized by water scarcity, extreme rainfall variability, and low agricultural productivity. The available water resources are often inadequate in quality to be used for drinking or even for agricultural purposes. Dryland regions in Africa constitute 43% of the overall land area, encompassing 75% of the agricultural land and inhabited by 50% of the population.1 Around 75% of Africans experiencing poverty live in countries with a minimum of 25% of the population residing in arid regions, where poverty is strongly concentrated.2 Drylands often have poor institutional support, limited infrastructure, undeveloped financial markets, and minimal chances for commercial expansion. The population living in dryland regions of Africa is anticipated to surge from 460 million to nearly 800 million by 2030. This increase is expected to strengthen the pressure and the competition for natural resource use.3
Low access to water resources and reliable modern energy in drylands constitutes the main barriers to socioeconomic well-being of the communities as well as to agriculture development and food security.4 According to the Food and Agriculture Organization (FAO), irrigated lands account for over 40% of the world's food production.5 Therefore, improving water control and irrigation processes in sub-Saharan and Northern Africa where merely 4% to 28% of farming land is under irrigation, is a key solution for boosting agricultural production.5–7 Environmental pressures and climate change, along with the growing economy and populations, strengthen the necessity to assess and analyse the complex interlinkages between water, energy and ecosystems.8,9
The purpose of this paper is to analyse the water–energy–ecosystems nexus approach and contribute to the identification of some technology-based solutions to improve well-being and achieve water, energy, and food security, particularly in communities living in African drylands.
2. Overview of water and energy resources in African drylands
2.1. Water scarcity
Africa is renowned for its diverse and unique ecosystems, which range from hot deserts to cool woodlands. This broad range of ecosystems is heavily influenced by the fluctuating availability of rainfall and freshwater resources, both spatially and over time.10 The subregion of Southern Africa is particularly vulnerable to irregular inter-annual rainfall and frequent, severe droughts, especially in the southwest.11 Rainfall in Eastern Africa varies in quantity and distribution, with mean annual ranges spanning from 220 mm in Djibouti to 1200 mm in Rwanda.12,13 The region experiences considerable variability in rainfall over both time and place. This includes frequent episodes of flooding or drought, and complex seasonality with variations occurring within short distances of tens of kilometres.14
The wettest sub-region of Africa is Central Africa, but there are dry regions along the Sahelian zone in Chad and northern Cameroon, resulting in a climate that can vary from tropical dry to wet to equatorial.15 Most rain in the West Africa sub-region falls in the coastal area, with less rain falling inland. Rainfall in the north consistently decreases to the Sahel belt (100–200 mm per year), whereas in some parts of the Sahara it might be completely absent and average annual rainfall can be as low as 20–30 mm per year.16 Northern Africa is the most arid sub-region in Africa, characterized by extremely limited water resources and a rainfall that ranges annually between 50 and 1000 mm in the southern and the north-western areas, respectively.17
Some countries predominantly comprise drylands, such as South Sudan, Chad, Niger, Mali, and Botswana, while others, including Northern African countries, Kenya, Ghana, and Namibia, have substantial dryland parts that occupy a part of their total land area.18
Per the conclusions of the Intergovernmental Panel on Climate Change (IPCC),19 droughts are projected to occur more frequently over the next decades, exacerbating existing water scarcity issues across the continent. Populations in Africa face various challenges related to water scarcity, including high rates of poverty, an excessive reliance on nature for water supply, which could be a health risk in terms of water-borne diseases, poor nutrition, food shortages, sharing of water sources with livestock and wildlife, and long distances to water sources. These challenges can impact the well-being of populations and exacerbate existing socioeconomic inequalities.20–22
2.2. Drylands and energy resources
It is well known that access to energy is crucial for both economic growth and the achievement of human and societal well-being. In sub-Saharan Africa (SSA), energy poverty is considered one of the most prominent challenges; nearly 1 billion of the population lack access to electricity and still depend on polluting fossil resources for cooking. More than half of the African countries still have an electrical access rate below 60%.23,24
Some countries in the drylands of Africa like South Sudan (7.2%), Chad (11%), and Niger (16%) have access rates of less than 20% with severe lack of a modern form of energy access and rely on biomass which is critical for many livelihood needs including construction material, feed for camels and goats, cooking, and heating.25
Despite a slight improvement in the availability of electricity in dryland areas, the accessibility of clean cooking fuels and technologies (CCFT) has not improved, leading to a 10% increase in the number of people seeking CCFT, which rose to about 940 million in 2020 due to population growth. This makes Africa the sole continent experiencing a rise in the number of inhabitants lacking CCFT.26 As a result, millions of people continue to rely on harmful fossil resources and biomass including charcoal, fuel, kerosene, wood, agricultural leftovers, and animal excrement. The use of these resources in basic stoves poses significant risks in polluting indoor air, greatly exacerbating various diseases and even causing numerous fatalities.27–29 This problem is compounded by the absence of availability of modern energy technologies and inadequate ventilation in most rural homes.
Addressing this reliance and finding cleaner alternatives is crucial for public health and environmental sustainability. Actually, renewable clean energy resources are abundant and widely available across the continent, with over 40% of global solar irradiation falling on Africa.30 The continent has wind and solar resources in both its north and southern parts, large hydro resources in most regions, biomass resources throughout much of the continent, and geothermal resources in its eastern part.31 The continent has the potential to be at the forefront of renewable energy transition even though all these resources are only slightly exploited. Significant efforts have been made over the past two decades to integrate renewable energy technologies for power generation on the continent and nearly 2% of the worldwide funding in this field has been directed towards Africa, with significant regional disparities.32
Stand-alone systems and mini-grids of less than 10 megawatts are two examples of distributed renewable energy solutions that are constantly evolving to increase the availability of electrical generation and distribution in off-grid areas.33 The most prominent technologies to achieve that are solar photovoltaic (PV) followed by hydro power.
By 2040, these emerging decentralized systems are projected to provide electricity access to 140 million Africans.34,35
3. Ecosystems in the context of drylands
The pressure on rainfed farming in dryland agroecosystems in Africa is a major concern for subsistence farmers. Dryland communities have a longstanding experience of adaptation to water scarcity through many ways of dealing with aridity and variability, and this knowledge may provide valuable lessons and insight for other areas that will face similar difficulties.36 This includes water harvesting and conservation, water and energy supply and management, and land management practices, such as cultivating drought-resistant crops or adopting conservation agriculture practices.37–39
The adaptation of technical measures to ensure efficient water resource management is expected to vary depending on economic, social, and environmental conditions. Moreover, it is influenced by technology accessibility and availability across all sectors and levels, ranging from local to national.17 However, the implementation and the development of the technology remain constrained and hindered by insufficient resources and limited knowledge in several African countries.
3.1. Water harvesting and conservation
The practice of increasing the productivity of dryland agriculture has a long history and a lot of experience in using nature-based solutions, recognized as a key tool for balancing WEF needs while promoting sustainable ecosystems. Farmers have developed a variety of methods for conserving rainwater to counteract drought.40 For instance, traditional irrigation in North Africa utilized “foggara” or “khettara” (Fig. 1(a)). In this system, the water flow is gravity-driven. It reaches the surface at the opening of underground channels, where it is used for drinking and irrigation. Underground water transportation reduces water loss due to evaporation and prevents sediment accumulation caused by sandstorms.41,42 However, this conventional system has been abandoned as a result of the substantial reduction of the groundwater table driven by an excessive supply of water and poor maintenance practices in favour of newer technologies, enabling water withdrawal that exceeds natural recharge.43 Other ancient rainwater harvesting methods known as Meskat systems were of particular interest and commonly used in the Maghreb region.44 The Meskat was described as a flat piece of land with a slight slope and few or no drainage channels.45,46
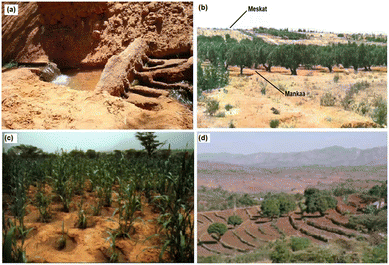 |
| Fig. 1 Nature-based solutions for water harvesting and conservation. (a) El Meghaier foggara in the oasis of Timimoun, Algeria.48 (b) General view of the Meskat–Mankaa system in Central Tunisia, with the catchment and cropping area.49 (c) Zai technique for soil and water conservation in the Sahel zones of West Africa (photo by Djibril S. Dayamba, ICRAF-Mali). (d) Fanja juu technique used in Eastern Africa.50 | |
The land is prepared for rainwater collection (Meskat), and the water is channelled towards a different parcel directly below (Mankaa). This plot of land is known as the collector, and it is where crops are planted (Fig. 1(b)). Unfortunately, the state of these systems has deteriorated as a result of the exhaustive agricultural progress that has occurred since the mid-century.47
Another example comes from western Sahel (Burkina Faso, Niger, Mali), where the Zai or Tassa (ancestral planting pits) is used as an effective method for improving the management of degraded lands and lowering soil erosion, vegetation loss, and biodiversity loss in addition to improving crop productivity, livelihoods and soil fertility and rehabilitating degraded drylands51 (Fig. 1(c)).
Fatondji et al.52 revealed that employing solely Zai in Niger led to doubling the water use efficiency and raising the grain yield by 3 to 4 times compared with flat planting.
More common terracing technologies such as Fanya juu are used by smallholder farmers living on steep mountain slopes of East Africa (Kenya, Tanzania, Ethiopia) (Fig. 1(d)). This technology is found advantageous for managing flooding as well as avoiding soil erosion and landslides.53 However, many of these techniques are disappearing from use54,55 mainly due to inadequate maintenance, encroachments, obstruction of the recharge pathways and urbanization.
3.2. Water efficiency and productivity
Agricultural ecosystems are the main sources of food, yet they are also the largest consumers of water resources on a global scale. The water consumption of African ecosystems accounts for a substantial portion ranging between 70% and 90% of the available water in agriculture. This fluctuation is contingent on varying climate conditions and the economic development level of the country.56 The current irrigation efficiency in most of the African countries is only 0.3 to 0.4, which is significantly lower than the 0.7 to 0.9 efficiency in developed countries.5,57,58 This is due to the outdated technological and managerial practices employed by the aging irrigation infrastructure, which has deteriorated significantly over time. In African dryland countries, the majority of rural households will continue to rely on agriculture irrigated by natural rainfall, which is characterized by limited infrastructure, challenges in water management and fertilizer use.59 These combined factors result in lower productivity, particularly in terms of crop water productivity, consequently affecting the overall yield and sustainability of the farming system.
3.3. Wastewater treatment and reuse
Treatment of wastewater for use as a substitute for freshwater is already recognized as a significant water conservation and environmental preservation approach that also preserves agricultural production.60 Wastewater reuse can be a key element of effective management of wastewater and water resources. It offers a sustainable alternative to managing wastewater that significantly reduces the environmental effects of discharging wastewater into surface waters.61
Reusing reclaimed water has been integrated into the National Water Resources Strategy of a number of dryland countries in Africa, particularly those in the northern part.17 For instance, more than a third of Tunisia's wastewater treatment facilities offer irrigation to farmland or recharge aquifers in regions experiencing excessive groundwater extraction.17,62
However, a significant difficulty continues to be the lack of maintenance that caused many wastewater treatment plants to stop operating.63 Despite the fact that there is a considerable measure of potential for adopting wastewater reuse for irrigation in arid regions of Africa, this technique has not been fully adopted in almost the majority of the continent's dryland countries due to socioeconomic and technological constraints.64 In addition to aridity, several factors have been identified to affect the broader implementation of reuse. These encompass proximity to the source, retrofitting existing infrastructure instead of installing new ones, the scale of reuse, cost considerations, source quality, public health aspects, readiness within the community, and public confidence and awareness alongside the regulatory framework and guidelines governing reuse.65,66
4. The WEFE nexus approach
IWRM is defined as “a process which promotes the coordinated development and management of water, land and related resources, in order to maximize the resultant economic and social welfare in an equitable manner without compromising the sustainability of vital ecosystems”.67
The management of the water–energy–food nexus is a complicated issue with numerous challenges, especially given the significant shifts in the climate conditions, population growth, and resulting urbanization. Balancing these factors requires careful consideration and integrated approaches for sustainable resource management. Fig. 2 illustrates the diverse factors influencing the WEFE sectors, showcasing the intricate interconnections of their various aspects and highlighting composite indicators crucial for enhancing the well-being of communities in African drylands.
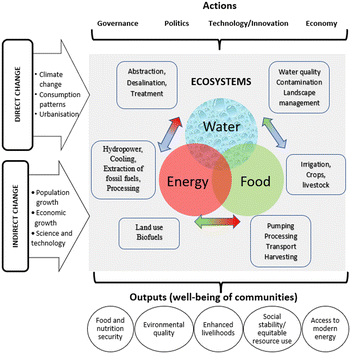 |
| Fig. 2 The nexus approach for well-being of communities in African drylands. | |
While many studies have focused on water availability and demands for human needs, there is a need to consider the impacts of WEF interactions on other aspects such as the environment, biodiversity, and ecosystems.20,68–70 This is important to guarantee the long-term sustainability of WEF systems and to minimize negative impacts on natural resources. Regarding the synergies between agricultural water demand and hydropower generation, there is still much to be explored and optimized to achieve maximum benefits from these interactions. This is particularly relevant in areas where both water and energy are scarce and where there are competing demands for these resources.71 To achieve sustainable water targets, there is a need for a comprehensive and integrated approach that encompasses water reuse, conservation, policy mechanisms, and infrastructure improvements, supported by strong institutions and funding. This requires an interdisciplinary and collaborative effort that integrates biodiversity and healthy ecosystems into water, energy, agriculture, and other sectors, and that involves stakeholders from various sectors and levels of governance. As rapid demographic and economic changes are prevalent in Africa, it is crucial for future food systems to transition from existing producing and consuming patterns towards healthier sustainable food and food products.72
While food systems benefit from water, climate stability, biodiversity, and healthy ecosystems, they can also negatively impact these elements.73 Agri-food systems are the primary cause of biodiversity loss and contribute significantly to greenhouse gas emissions and water pollution.74 However, there are options available to mitigate future impacts, such as agroecology in production and dietary shifts in consumption. The changes in food systems have a significant impact on ecosystems and other nexus elements, and improvements can be made in various stages, from production to waste. However, the relationship between each stage and other elements of the nexus is complex, and trade-offs and synergies must be carefully considered to ensure positive outcomes for biodiversity and ecosystem protection.75 For example, agricultural production contributes to biodiversity loss and freshwater pollution, while it also provides essential nutrients for nutritional health.76 Therefore, how goals are set to achieve outcomes at each stage is crucial for the overall nexus outcomes for biodiversity and ecosystems protection.
The use of a nexus approach may be essential in effectively managing water, energy, and food security in regions with limited water resources, such as drylands, where competing demands and limited funding can present significant challenges.
By adopting the WEFE nexus as a framework, decision-makers can benefit from a comprehensive model that facilitates the evaluation of adaptation strategies and informed decision-making.77,78
The Agenda for Sustainable Development,79 the agenda 2063 of the African Union80 and the Technology and Innovation Strategy for Africa 2024 (STISA-2024)81 acknowledge the interconnectedness of the various aspects of water security and are very pro-integration of water problems across sectors using methods like the Integrated Water Resources Management (IWRM).
The following section will discuss technological innovations that might play a substantial role in addressing the challenges and opportunities of the WEFE nexus, specifically in the context of African drylands.
5. Innovative technology-based solutions in the WEFE nexus
In African dryland region, the WEFE program is particularly interested in solutions that are easy to use, adaptable, and affordable, have immediate advantages and benefits for smallholder farmers and other actors in the value chain, and also have net positive impacts on ecosystems and natural resources, particularly water and energy. Some of the potential innovation themes to consider are the following.
5.1. Energy irrigation nexus
Energy irrigation nexus covers innovations that develop new, water-efficient methods or improve the efficiency of those that are currently used. Examples include the following techniques.
5.1.1. Agricultural PV.
One of the promising hybrid solutions combining food with energy production is called agrivoltaics (AgriPV). This concept combines the production of crops and energy concurrently on the same cultivating land, resulting in the optimized utilization of agricultural land, water, and incident radiation. The land use efficiency expressed by the land equivalency ratio (LER) in the case of AgriPV can increase by up to 90% compared to conventional agricultural use.82,83
Depending on the climate and the choice of crop variety, the sharing of light between these two types of production may enable a higher crop yield. In some cases, it may even be mutually beneficial as vegetation evapotranspiration creates a cooler microclimate under the PV panels, which allows decreasing the panels' temperatures and increasing their performance and service life.84–87 The experiments conducted by the Barron-Gafford research team have described the impact from the agrivoltaic system installed in the dryland of Arizona (USA) using this technology on some agricultural species adapted for dryland environments and proved that compared to conventional PV system conditions, PV panels were approximately 9 °C cooler during the day, which allows a 3% increase in the system performance.86
Barron-Gafford et al.86 focused also on the eco-physical function and the biomass production of vegetation under AgriPV and proved that the total crop production has doubled compared to that of conventional agriculture.86
AgriPV results are promising and encouraging in terms of soil water saving and renewable energy and food production. Installing energy-efficient solar powered systems in African drylands is considered as a key solution to improve well-being especially in rural areas where 37% of inhabitants lack access to electricity and agriculture stands out as the primary source of both employment and income.82,88 However, it is important to further explore various species and delve into optimizing the conception and configuration of solar systems. This investigation will grant a deeper comprehension of the intricate balance and interplay between energy production and crop yield.
At present, the construction of pilot AgriPV projects in Kenya, Uganda, Tanzania, Mali, and Gambia is either in the planning stage, in progress or has recently been completed.17,82,89 The primary goal of these pilot projects is to demonstrate the operational and economic feasibility of agrivoltaics, emphasizing the efficiency and the effectiveness of the combined three-fold-land use. Ultimately, the adoption of AgriPV aims to bolster ecologically and socioeconomically sustainable development in these African countries. An additional objective is to thoroughly analyze the impacts of AgriPV structures on crop yields and the financial value they bring to farmers.9,82,90,91
Many other examples that are more efficient in water and energy use are the following.
5.1.2. Solar powered irrigation systems (SPIS).
A solar powered irrigation system is a technically advanced option that can substitute for conventional energy sources. It holds numerous benefits, offering an eco-friendly substitute for fossil resources and enabling the advancement of low-carbon irrigated agriculture.92 If properly managed and coupled with modern energy-efficient irrigation techniques, including drip irrigation or sprinkler systems, SPIS allow for improved and more effective water utilization, reducing water loss due to evaporation or runoff, and potentially improve food production.
The reduced water pollution driven by a decrease in groundwater consumption can help to preserve and improve the biodiversity of wetlands, lakes, and rivers. Burney et al.6 investigated an SPIS using a drip system in the West African Sudano-Sahelian zone. They revealed that this technology is more affordable than alternative technologies and considerably increases household earnings and nutritional consumption, especially amidst the arid season.
Farmers in North Africa are increasingly considering using these systems due to the significant potential for developing SPIS. In Tunisia, for example, there are about 13
000 shallow wells widespread in Tunisia with a potential of about 24 MWc.93
However, there has been abuse of groundwater resources because farmers in this region perceive these systems as just an energy option to the detriment of the water security.
Mekki et al.94 reported a rise in the illicit use of SPIS in South Tunisia's Kebili region, particularly in the areas disconnected from the ancient oasis, during the period from 2015 and 2020. This increase was marked by the deployment of 2400 SPIS systems (Fig. 3), resulting in substantial adverse impacts on the local environment and the agricultural sector's sustainability.
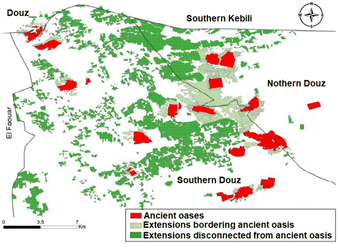 |
| Fig. 3 Spatial distribution of irrigated areas and main abstraction from SPIS in Kebili region (Tunisia).94 | |
Chaibi95 emphasized the negative effects on the ecosystems driven by the over-exploitation of deep aquifers, which generated a flow of more salinized water from the soil's surface to the subsurface (Fig. 4).
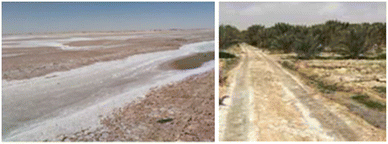 |
| Fig. 4 Photos illustrating obvious salt accumulation in the oasis of Kebili caused by illicit SPIS use.95 | |
To ensure the successful implementation of an SPIS project, adopting integrated approaches such as considering the water–energy–food nexus and promoting sustainable livelihoods is essential. These approaches help bridge knowledge deficiencies related to SPIS effectiveness and feasibility. Additionally, more efforts must be made to cooperate and systematically structure the development of professional competences. This will facilitate the conception, implementation, and development of these energy-efficient irrigation systems.
5.1.3. Hydroponic and aquaponic systems.
Hydroponic refers to a soilless cultivation system that provides a plant with the adequate nutrient solution through a water solution circulating in a closed loop.96–98 Hydroponic systems, suitable for fruit and vegetable cultivation, use less water, less pesticides and fertilizers which leads to the production of crops of high quality and high nutritional value.
They are considered as environment-friendly thanks to their low greenhouse gas emissions. For instance, Martinez-Mate et al.99 found that the gas emissions of a hydroponic system were about 0.11 kg CO2 while a conventional system generated about 0.23 kg CO2.99 Additionally, these technologies can extend the growing season to enable year-round output and allow the control of plant diseases, especially viruses, which is particularly advantageous in tropical areas where infestations are a major concern.100
Aquaponics is a production system of aquatic organisms and plants,101 in which the effluent of aquaculture is the source of the nutriments necessary to optimal plant growth. Nutrient uptake by plant roots guarantees the remediation of aquaculture water.
Aquaponics has been perceived as a sustainable technology thanks to the economic and environmental advantages it presents, mainly: a high water use efficiency, an increased production efficiency of plants and aquatic organisms, and the non-use of pesticides/herbicides or antibiotics.101
It was highlighted through several research studies that soilless agricultural systems allow effective land management, reducing arable land by 10% to 25%, and have a high potential in ensuring food security compared to conventional agriculture systems.102–104 Hydroponic and aquaponic systems guarantee higher quality food products, high value and food diversity (protein and greens) and greater food safety.105–107 Cultivation in hydroponic systems is acknowledged for faster growth rates by about 30–50% than conventional cultivation. Aquaponics, producing at the same time vegetables and high nutrition value fishes, are considered as sustainable and intensive food production systems.98,107–109
During recent years, aquaponics technology features and design have evolved to enable its rapid transition to industrial-scale production in many nations worldwide, but its uptake in Africa remains relatively limited. Despite the potential advantages of aquaponics in Africa, barriers to its widespread adoption include a lack of technical knowledge, inadequate infrastructure, and lack of funding. Some African countries such as Egypt, Nigeria, Kenya and South Africa have successfully adopted and developed this technology efficiently. According to Van der Heijden et al.,110 an aquaponic system in Egypt produced 5–7.5 tons of Nile tilapia fish, 3.2 tons of basil, 2.6 tons of chives, and 7.5 tons of lettuce annually. In Nigeria, an aquaponic system produced 160 kg m−3 catfish and 43 kg (four months) of pumpkins.105 Zipporah et al.111 used three cultivations and compared their growth in an aquaponic system for intensive production of Nile tilapia in Kenya. The system produced 1.1 kg m−2Amaranthus, 1.3 kg m−2Cucurbita and 1.6 kg m−2Artemisia; the nutrient uptake depended on the crop species and the highest extraction was during the fruiting stage. The experimental results proved the reliability of aquaponic systems under semi-arid climate conditions.
The recorded data related to energy consumption of soilless systems is minimal in the literature, particularly in Africa. Barbosa et al.102 demonstrated that the energy consumption of a hydroponic system (per kilogram) is 82 ± 11 times higher than that of a conventional lettuce production system in Arizona. The electrical consumption is also considered as the second most cost-intensive component category in aquaponic systems as it covers the requirements of heating and cooling systems as well as operation components (pumps, fans, inverters…).109,112 For this reason and in order to reduce water heating energy expenses, it is recommended to match the choice of the plants and/or the fishes with the local environmental conditions, particularly ambient air temperature, and to use passive techniques of water heating either directly by a residual heat or by solar energy or by installing soilless units inside greenhouses.98,102,104,113 Alternatively, fossil fuel electricity can be substituted with photovoltaic electricity to feed aquaponic and hydroponic systems, which improves the energy efficiency of these WEFE nexus solutions.
Aquaponics technology is an interdisciplinary approach that involves several fields, including aquaculture, microbiology, ecology, horticulture, and agriculture. To further advance this technology, more research is needed on the appropriate selection of the parameters of conception and the components of the hydroponic system as well as the most suitable combination of fish, plant, and microorganism species.101,113 It is also necessary to control various parameters such as water pH,114 nutrient and nitrogen levels, and feed quantity and type.101 To promote the adoption of aquaponics by small-scale farms in African drylands, further research should focus on developing low-cost aquaponic systems.
5.2. Ecological wastewater treatment
This covers developments in wastewater cleaning technologies with minimal energy requirements to lower pollution, agricultural contamination, soil deterioration, and related health risks. The ecosystems of wetlands may offer a range of functions essential for human well-being. For farmers and pastoralists, they serve as important resources by providing them with access to grazing areas or arable land during droughts or other dry seasons.115 Ecological wastewater treatment processes can also be designed to recover resources such as phosphorus, nitrogen and other nutrients, which are valuable for agriculture. These recovered resources can be used as fertilizers, which can reduce the dependence on chemical fertilizers and improve soil health and crop quality.116 Moreover, harvested reeds of constructed wetlands can be used either to produce biogas or biofuel or as an energy source for combustion processes, which improves the energy security and reduces the dependency on fossil fuels.116–118 Additional advantages include water filtering, water storage, flood control, outdoor recreation, processing of carbon and other nutrients, and support for plants and animals.119
Constructed wetlands (CW), integrating natural materials and processes, have yet to gain widespread adoption in Africa. Nevertheless, there are instances of studies that have achieved success proving the technical efficiency as well as the sustainable impact of this technology.
For the past 25 years, CW have been built in many small, dryland communities throughout sub-Saharan Africa, including Kenya, Tanzania and Uganda, and have proven to be reliable, efficient, and affordable120,121 (Fig. 5).
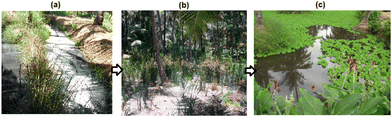 |
| Fig. 5 Consecutive treatment steps of a CW system constructed in Kiembeni (Mombasa, Kenya). (a) Surface flow biofilter using vetiver grass to entrap fats, oils and scum, (b) sub-surface horizontal flow with washed gravel planted with Typha grass, and (c) open water ponds with flowering plants and vetiver grass.122 | |
den Haring's122 study from 2015 to 2020, focusing on CWs implemented in Kiembeni, Kenya, demonstrated the effective removal of specific pollutants, such as total suspended solids (TSS), chemical oxygen demand (COD), and biochemical oxygen demand (BOD), at impressive rates of 89%, 97% and 94%, respectively.
Several other studies conducted across Africa suggest evidence that CWs reduce contaminant loading by a removal rate of 75% to 84% TSS, 86% to 96% COD, and 78.5 to 98% BOD.19,123,124
Based on three years of CW experimentation in the north of Tunisia, Ergaieg and Ben Miled118 emphasized that this treatment process has the capacity to produce effluents characterised by a higher quality and meeting irrigation standards and thus offers a practical alternative to the energy-intensive tertiary treatment of sewage.
Given that, CW technology is acknowledged for its cost-effectiveness, reduced overall expenditure, and seamless integration under Africa's arid and hot climatic conditions, particularly in remote locations,125 more efforts should be made to improve local wetland management and support local actors with sufficient capacity to construct CW and awareness raising. Along with additional funding and public support for the maintenance of the existing CW and the creation of new ones, additional research on suitable plant species, CW designs and construction is required.
5.3. Saline water use for agriculture
This includes innovative techniques for cleaning brackish water or poor-quality water to be used to increase freshwater resources for agricultural usage.
Examples include the deployment of solar desalination systems in water–energy–food nexus projects when combined with methods for growing crops.126 However, these systems have only been used in experimental and pilot projects, particularly in North Africa and island countries where saltwater is the primary source of irrigation water.127–129
In 2016, a pilot project was implemented in central eastern Tunisia to desalinate 200 m3 per day for agriculture purposes. This project, conceived as part of a comprehensive climate change adaptation strategy, aimed to reduce the salinity level of the water from 4.8 g L−1 to 0.2 g L−1, followed by mixing it with well water to achieve a concentration of 1.5 g L−1 (ref. 130) (Fig. 6). The project also involved the installation of a 640 m3 water reservoir. Presently, this initiative benefits 60 farmers who use it for irrigating their greenhouse crops. The results of the effectiveness assessment of this pilot project revealed that even without rigorous irrigation management, some farmers achieved a twofold or even threefold increase in yields during the first year of operating a desalination unit for irrigation. Chaibi and Jilar131 conducted an experimental study in a widespan greenhouse in Tunisia to demonstrate the efficiency of integrating a desalination process on the roof of its southern façade. They found that the desalination process installed on 50% of the roof surface was effective in harnessing the incident solar radiation concentrated within the closed greenhouse, allowing the production of the total annual irrigation water required by a low canopy crop.
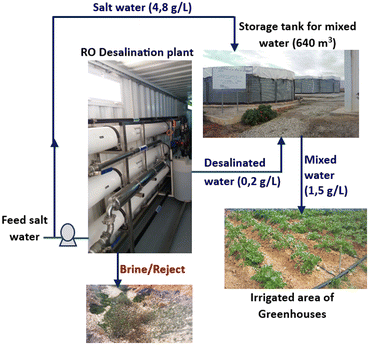 |
| Fig. 6 Agriculture desalination plant: Gounet-Mehdia (Tunisia). | |
However, this water desalination is still expensive for agriculture and sometimes presents environmental problems, such as how to manage the rejected brine, which frequently contaminates groundwater and increases its salinity.132 Currently, the most likely option for cost-effective use of solar desalination for irrigation purposes is associated to the intensive horticulture for high value irrigated crops, grown in coastal areas where the brine could be discharged without environmental adverse impacts.133
Furthermore, water desalination systems are also considered as energy consuming processes, as they employ pumping and water treatment components that consume considerable amounts of conventional fossil electricity. Depending on the desalination process, the required energy for the production of 1 m3 of water ranges between 1.5 to 3.5 kWh of electrical energy and 6 to 12 kWh of thermal energy.134 Therefore, considering the shortage of fossil fuels and their increasing prices, desalination processes were found to be energy-extensive and uneconomic.7,135
Developing advanced systems using waste heat recovery approaches or coupling desalination processes to renewable energy systems (solar, geothermal or wind energy) constitutes a great alternative to lower reliance on fossil fuels and to strengthen the sustainability of this WEFE nexus technology.134–140 Other alternative solutions for the sustainability of agricultural production in drylands where water quality is scarce includes irrigation with saline water. Thus, using saline water for irrigation offers a potentially effective way to address the water–energy–food–ecosystems (WEFE) nexus.
It can increase the amount of water that is available, enhance food security in areas where freshwater is limited, and reduce the amount of energy and expenses involved in withdrawing, transporting, and treating freshwater.141,142 For instance, Karlberg143 performed an experimental investigation of an economic drip irrigation system using saline water (6 dS m−1) in semi-arid South Africa and proved successful crop yields from two consecutive tomato crops.143
However, it is recommended to study the soil properties and its suitability for saline water irrigation before starting a saline water project. Meticulous management of saline water irrigation systems is also crucial to avoid increasing soil salinization that leads to decreased yields.
Finally, a particular study of cultivation is required to select the adequate crop species with suitable salinity tolerance.141–143
5.4. Digital Earth observation
This encompasses innovations in Earth observation (EO) science, products, and applications to enhance the understanding of the WEFE nexus approaches. These applications possess the distinct capability to monitor several processes including crop and vegetation water demand, water quality, surface water, groundwater mapping and exploitation, hydrological modelling and natural risk management.144
A hydrological model primarily relying on EO data can generate outputs that significantly improve local water management and offer valuable insights to local farmers and land users. These insights may include recommendations related to soil moisture management or irrigation, and early warnings in case of impending dry periods or droughts.14
EO is also instrumental in gathering crucial information about groundwater resources including their locations, levels, soil moisture, water quality and potential sources of contamination.
This information is invaluable for reducing water losses, improving irrigation efficiency and developing effective water management strategies aimed at managing, protecting and ensuring the sustainability of these resources.145–148
Moreover, EO data stands as a valuable tool for environmental protection and promoting human well-being. It offers critical insights into ecosystem changes and their impacts on water energy and food systems, enabling identification of areas requiring conservation efforts and support decision-making for resource management.149 EO data can also assess the potential for energy storage systems, optimize their operation, and evaluate their environmental impact. This is critical for the development of renewable energy projects and contributes to environmental protection by reducing our reliance on fossil fuels.148
As for food security, EO data plays a significant role in monitoring crops, planning land use, and responding to disasters, ultimately enhancing agricultural practices. Farmers benefit from timely and accurate information about agricultural advancement, crop health, and yield, empowering them to make well-informed decisions regarding the use of fertilizers and pesticides, and to respond quickly to natural disasters that may disrupt food production, contributing to a more resilient food supply.150,151
The Global Monitoring for Environment and Security and Africa (GMES and Africa) initiative (along with several others such as PUMA, EUMETCast, TIGER and AMESD) was initiated by the African Union in collaboration with the European Commission aiming to bolster sustainable resource management and infrastructure development in Africa to empower the continent to effectively utilize Earth observation data for informed policymaking.152 However, while these initiatives have made significant strides in enhancing accessibility to EO data and its use for the global environment and human well-being, their utilization and application still fall below their full potential in African coutries.153–155
6. Assessing the relevance and impact of WEFE nexus technical solutions
The WEFE nexus approach acknowledges the interconnected nature which means that changes made in one area can have significant effects on the others.
As a result, putting into practice the technology solutions that address the nexus might have a variety of effects on various sectors. The impacts of the WEF nexus solutions appropriate for adoption in Africa's drylands are detailed in the following sections and summarized in Table 1. The benefits of the solutions are indicated by a positive sign based on the level of advantage, while the drawbacks are indicated by a minus sign.
Table 1 The impact of selected WEFE nexus solutions
WEFE nexus solutions |
Water |
Energy |
Food |
Ecosystems |
References |
−: negative; +: positive; the number of + indicates the strength of the benefits in the various sectors. |
(1) Nature-based solutions (NbS)
|
++ |
+ |
+ |
+++ |
FAO,72 IPBS,73 A. M. N. Masoud et al.116 |
(2) Energy irrigation nexus
|
■ AgriPV |
+++ |
+++ |
++ |
++ |
A. E. Cheo et al.,82 P. Santra et al.,84 G. A. Barron-Gafford et al.,86 L. J. Walston et al.,87 Fraunhofer Institute,90 A. Ali Omer et al.156 |
■ Solar powered irrigation systems |
++ |
+++ |
++ |
++ |
J. A. Burney et al.,6 S. Mohammed Wazed et al.,92 I. Mekki et al.157 |
■ Hydroponic and aquaponic systems |
+++ |
− |
+++ |
+++ |
D. I. Pomoni et al.,98 E. O. Benjamin et al.,109 K. Tokunaga et al.,112 J. Dalsgaard et al.,158 H. El-Essawy et al.,159 G. Crini and E. Lichtfouse160 |
(3) Ecological wastewater treatment (constructed wetlands) |
+++ |
++ |
+ |
+++ |
A. M. N. Masoud et al.,116 E. Tilgalis and L. Grinberga,117 K. Ergaieg and T. Ben Miled118 |
(4) Water use for agriculture
|
■ Water desalination |
+++ |
− |
++ |
+ |
E. T. Sayed et al.,136 U. Yermiyahu et al.161 |
■ Saline water use |
++ |
++ |
+ |
− |
L. Karlberg and F. W. T. Penning de Vries,162 L. Karlberg,143 A. F. Abou-Hadid163 |
6.1. Water sector
Nature-based systems (NbS) can provide benefits for increasing water availability at a local scale during dry periods by naturally storing water and reducing soil erosion and runoff. However, these systems may not be scalable enough to meet the growing demand for water and may not be effective in extreme conditions, such as droughts or floods.
On the other hand, effective management of water resources can be achieved through the utilization of SPIS systems coupled with smart management methods, desalination processes or the use of saline water. However, it is crucial to carefully consider the environmental risks, such as the overuse of aquifers, water quality issues, groundwater contamination, and the proper disposal of brine by-products. Therefore, it is imperative to safeguard ecosystems and the environment by addressing these issues affecting the WEFE nexus balance even if it means making trade-offs with the benefits of the technology.
Furthermore, hydroponic and aquaponic systems are effective water-saving technologies that contribute to strengthening food security.
Studies have shown that these systems can save irrigation water by up to 95% compared to conventional processes. Nevertheless, it remains imperative to address potential challenges in advanced irrigation to ensure efficient water resource management and achieve optimal plant growth.
In the same context, the ecological wastewater treatment systems are considered effective solutions for improving the well-being of rural regions in African drylands suffering from water scarcity by providing water for irrigation. However, it is important to note that implementing and maintaining these systems can be resource-intensive, especially in rural areas lacking technical expertise and funding.
African AgriPV systems hold promise for enhancing sustainable water management, energy efficiency, cost savings in transportation, and rural electrification.
However, they may compete for land with traditional agriculture, raising food production concerns, and pose challenges in remote rural maintenance, with initial costs higher than that of some other WEFE technologies like water-efficient irrigation systems.
6.2. Energy sector
NbS for energy, such as solar or wind power, rely on the availability of natural resources. These solutions provide many environmental benefits, making them a sustainable alternative to conventional fossil fuels that are contributing to climate change and air pollution. However, the effectiveness of these solutions in remote rural communities can be influenced by the necessity for energy storage options that can reliably align with varying energy demands over time.
Reducing the freshwater consumption through AgriPVs and saline water irrigation is an energy efficient technology as it allows reducing electrical consumption and costs associated with water withdrawal and transportation.
SPIS and AgriPV systems are also electric energy generators covering the agricultural needs especially in rural areas with no access to the electricity grid.
Moreover, AgriPV systems have improved performance as PV panels operate at lower temperatures, which leads to higher panel efficiency and longer service life.
Compared to conventional wastewater treatment plants that consume huge amounts of electricity, CWs are identified as low-energy consuming and even zero electricity systems producing resources useful to reduce the dependency on fossil fuels.116–118
Soilless agricultural systems and desalination processes were found to be energy-extensive.98,102,113 However, substituting the fossil fuel electricity they consume with solar PV electricity and integrating passive water heating techniques in soilless systems may offer an effective solution to improve their energy efficiency.98,102,113
Further research is needed to develop renewable energy solutions adapted to the African climate conditions and accessible for low to moderate incomes.
6.3. Food sector
Using NbS in African drylands can bring multiple benefits to the environment and local communities when integrated into food systems. These solutions can offer cost-effective and sustainable alternatives that enhance soil health and fertility, protect biodiversity, and reduce dependency on synthetic inputs. However, implementing NbS may face challenges in scaling up to fulfil the growing food requirements globally. This is due to the typically higher labour and time requirements associated with NbS compared to conventional farming methods, potentially resulting in reduced overall efficiency. Additionally, NbS are more susceptible to climate change, such as droughts and floods, and less effective in preventing crop diseases or pests when compared to synthetic alternatives. This susceptibility to environmental factors can reduce crop yields, presenting a challenge to meet growing food demand.
Soilless systems contribute to strengthening food security by providing significantly higher yields compared to conventional agriculture systems. In particular, aquaponics producing vegetables and high nutrition value fishes are considered as sustainable and intensive food production systems allowing reduction of malnutrition and poverty in African drylands.98,107–109 Further efforts are required to decrease the high investment and operation costs to enhance the economic sustainability of soilless agricultural systems.
SPIS systems as well as AgriPV, desalination and saline water systems allowing higher water efficiency and availability contribute to increased irrigated crop yields and improved sustainable water and food management in Africa.82,90 However, the use of saline water requires a rigorous study and selection of the crops species with suitable salinity tolerance.
Similarly, AgriPV systems are suitable for selected lower-growing and shade-tolerant crops. The protecting and shielding effects they provide reduce heat and light stress and prevent depression in photosynthesis, which leads to enhanced crop quality and production.82,86
6.4. Ecosystem sector
NbS are generally more environmentally friendly than modern solutions, as they rely on natural processes and materials rather than synthetic chemicals or energy-intensive machinery that have negative environmental impacts such as water pollution, soil degradation, and deforestation.
Constructed wetlands are considered as sustainable and ecological wastewater treatment plants as they are based on the use of vegetation and natural processes aiming to reduce soil and groundwater contamination from pollutants and pathogens and restore the natural water ecosystem.116,118
Innovative renewable energy processes such as SPIS, AgriPVs or solar-coupled soilless systems allow reduction of fossil electricity and the generated greenhouse gas emissions in the environment. AgriPVs are considered as a triple land use technology providing at the same time the production of crops, electrical energy, and rainwater harvesting. Furthermore, AgriPVs provide essential ecosystem services related to agriculture such as producing foods and engaging in animal husbandry while ensuring water and soil conservation, carbon sequestration, crop protection and reduction of plant stress and biodiversity conservation.82,87 Therefore, AgriPVs are considered as a sustainable solution that strengthens synergies between ecosystem services. Soilless technologies are also considered as environment-friendly systems reducing arable land, generating low gas emissions, and generating a complete ecosystem between water and crops/fishes with developed synergies between the ecosystem's organisms, fish/plants and bacteria. This interconnected ecosystem boosts both crop and fish production, demonstrating a harmonious and sustainable relationship between human intervention and nature.
6.5. Enablers and drivers for selected WEFE nexus technologies
The drivers and barriers for selected WEFE nexus technologies in the African continent, outlined in Table 2, are complex and interrelated, showing significant differences based on the specific context and country in Africa.
Table 2 The enablers and barriers of the selected WEFE nexus solutions
WEFE nexus solutions |
Enablers/drivers |
Barriers/challenges |
References |
(1) Energy irrigation nexus
|
■ AgriPV |
- Clean technology- Lowers electricity costs - Independency from electricity rates - Secure investment |
- Investment volume- Specific crops - Performance decrease over time - Maintenance issues - Trust in technology |
82, 84, 86, 87, 90156, 164 |
|
■ Solar powered irrigation systems |
|
- Performance decrease over time- Overexploitation of groundwater - Trust in technology |
6, 92, 157 |
|
■ Hydroponic and aquaponic systems |
- Sustainable food production system using the circular economy concepts- Soilless solution - Reduction of inputs and waste |
- Investment volume (costs of set-up, fish feed…)- Energy and management costs - Lack of expertise and long-term planning - Trust in technology |
98, 104109, 112 158, 159, 160, 165 |
|
(2) Wastewater treatment
|
■ Conventional wastewater treatment |
- Removal of toxic contaminants- Protection of water resources |
- High initial capital cos- High energy consumption |
116
|
|
■ Ecological wastewater treatment (constructed wetlands) |
- Ecological and cost-effective solution- Allows the recovery of resources (phosphorus, nitrogen) valuable for agriculture as fertilizers - Reduces soil and groundwater contamination - Produces energy (methane gas) |
- High maintenance costs- Vulnerability to changes in climatic conditions - Inadequate expertise and technical support - The need for huge land areas and rigorous geological analysis of the site - Trust in CW potential |
116, 117, 118 |
|
(3) Water use for agriculture
|
■ Water desalination |
- A solution to water scarcity- Effective process |
- High investment and operation costs- High energy consumption - Management of the rejected brine |
136, 161 |
|
■ Saline water use |
- Strengthens drought resilience- Food production in areas of freshwater scarcity - Nutrient recovery/reduction of synthetic fertilizer use - Enhances biodiversity |
- Specific crops- Risks of pollution of groundwater and related ecosystems - Efficiency depends on the soil type, potential evaporation, precipitation, and irrigation management techniques |
143, 162, 163 |
The adoption of WEFE nexus technologies is driven by various factors, mainly the environmental issues related to climate change and land degradation along with population growth and inadequate infrastructure in African drylands. Climate change, a pressing issue, acts as a catalyst, demanding innovative solutions to tackle shifting weather patterns, prolonged droughts, and frequent extreme events. Moreover, land degradation combined with changing climate conditions also presents a critical concern that affects both agricultural productivity and ecosystem health. These WEFE nexus technologies empower communities and regions to enhance resource efficiency, diversify resources, protect agriculture, promote renewable energy, restore ecosystems, enable data-driven decision-making, and support adaptive planning. Collectively, these elements are critical for building climate resilience in the face of climate-related threats.
Additionally, population growth in Africa's rapidly growing population places immense pressure on water, energy, and food resources, making WEFE technologies essential to efficiently manage and utilize these resources to meet the growing demand. Inadequate infrastructure in many African regions can also hinder efficient resource management by limiting distribution, causing losses, and restricting access to essentials like clean water and reliable energy. However, WEFE technologies offer a solution to bridge these gaps by providing localized, efficient, and modular solutions that reduce the dependence on extensive infrastructure. These drivers can exacerbate existing challenges related to water, energy, and food security and make it more difficult to achieve sustainable development goals.
Although the potential for progress is possible, there are obstacles that could impede these efforts.
These obstacles include political and economic instability, insufficient financial resources, and conflicting priorities among stakeholders.
Political instability and economic uncertainties pose persistent challenges capable of disrupting the long-term planning and investment essential for WEFE solutions.
Moreover, the development and implementation of WEFE technologies rely heavily on substantial financial resources, underscoring the importance of funding and effective investment mechanisms from both public and private sectors for sustainability. Additionally, the diversity of stakeholders involved may harbour divergent priorities, potentially hindering collaborative efforts.
7. Conclusion and recommendations
This review explores the urgent challenges faced by African drylands: including water scarcity, climate variability, and low agricultural productivity affecting nearly half of Africa's population. Our research methodology encompassed systematic reviewed studies in sub-Saharan and North African arid regions. This comprehensive approach relies on extensive database searches to access the significance of WEFE technologies in African arid regions. This examination underscores the importance of understanding interconnections and broader implications while integrating insights from both natural phenomena and technological progress.
The critical literature review goes beyond previous assessments by exploring the role of proposed WEFE technologies in African drylands. This is a significant contribution since the ‘WEFE nexus’ is often discussed but is insufficiently applied, especially in African climates. The literature we assessed tends to prioritize technical solutions for individual WEFE components, often overlooking comprehensive assessments of the entirety of the system, including the environment and ecosystem dynamics.
The technologies discussed in this paper highlight renewable energy's potential to optimize water and energy efficiency, enhance food security, and reduce environmental impact. Pilot projects in Africa confirm their practicality in elevating rural living standards and promoting environmental sustainability.
Analysing technical solutions provides valuable insights into addressing critical challenges. NbS offer promise in addressing climate change and resource loss, but face limitations in scale, climate resilience, and regulations.
Innovative solutions such as AgriPV and hydroponic and aquaponic systems hold promise in arid regions since they might offer multifaced solutions to the challenges of water scarcity, energy needs, and food security. However, they might require comprehensive scientific research and extensive field testing. Water desalination for agriculture purposes offers innovative pathways to address water scarcity and food security. This technology becomes particularly relevant when coupled with renewable energy sources and effective environmental management practices. Effective water resource management can be achieved using SPIS along with smart management methods. However, careful consideration is needed to address environmental risks like overuse of aquifers, water quality, and groundwater contamination. Ecological wastewater treatment is identified as low-energy consuming and effective for improving the well-being of rural regions suffering from water scarcity. Nevertheless, they can be resource-intensive, especially in rural areas lacking technical expertise and funding.
The review investigation has revealed that each of these solutions has its unique challenges and complexities, making the endeavour to address WEFE challenges in African drylands through technology multifaceted. These challenges encompass technical obstacles, sustainability concerns, and governance constraints. While the presented results emphasize the significance of WEFE technologies in African drylands, their limited application in pilot projects raises concerns about their effectiveness and scalability.
Addressing challenges in African drylands requires both additional research and the development of a comprehensive strategy that considers political, economic, and social factors as well as technology access like EO technology to inform policy and promote sustainable resource management.
Abbreviations
AgriPV | Agrivoltaics |
AMESD | African Monitoring of the Environment for Sustainable Development |
BOD | Biochemical oxygen demand |
CCFT | Clean cooking fuels and technologies |
COD | Chemical oxygen demand |
CW | Constructed wetlands |
EO | Earth observation |
EUMETCast | EUMETSAT Broadcast system for Environmental Data |
EUMETSAT | European Organization for the Exploitation of Meteorological Satellites |
FAO | Food and Agriculture Organization |
GMES | Global Monitoring for Environment and Security |
IPCC | Intergovernmental Panel on Climate Change |
IWRM | Integrated Water Resources Management |
LER | Land equivalency ratio |
NbS | Nature-based systems |
PUMA | Preparation for the use of Meteosat Second Generation in Africa |
PV | Photovoltaic |
SPIS | Solar powered irrigation systems |
SSA | Sub-Saharan Africa |
TIGER | Topologically Integrated Geographic Encoding and Referencing system |
TSS | Total suspended solids |
WEFE | Water, energy, food and ecosystems |
Author contributions
The manuscript was written with contributions from all authors. All authors have given approval to the final version of the manuscript. M. T. C. and A. K.: conceptualization, data curation, validation, writing – original draft. M. T. C. and M. S.: literature research, review, editing. M. T. C. and M. S.: writing and funding acquisition. M. T. C., A. K. and M. S.: contributed during discussion and manuscript writing.
Conflicts of interest
There are no conflicts to declare.
Acknowledgements
We would like to express our appreciation to the Environmental Science: Water Research & Technology journal for considering our work and providing the opportunity to publish our findings.
References
-
R. Cervigni and M. Morris, Confronting Drought in Africa's Drylands: Opportunities for Enhancing Resilience, World Bank and Agence Française de Développement, Washington DC, 2016, p. 282, DOI:10.1596/978-1-4648-0817-3.
-
T. Dietz, The World’s Drylands: a Classification, in The Impact of Climate Change on Drylands, with a Focus on West Africa, ed. A. J. Dietz, R. Ruben and A. Verhagen, Kluwer Academic Publishers, Dordrecht/Boston/London, Environment and Policy Series, 2004, vol. 39, pp. 19–26, DOI:10.1007/1-4020-2158-5.
- S. Lamptey, Agronomic practices in soil water management for sustainable crop production under rain fed agriculture of Drylands in Sub-Sahara Africa, Afr. J. Agric. Res., 2022, 18(1), 18–26, DOI:10.5897/ajar2021.15822.
-
R. Sims, A. Flammini, M. Puri and S. Bracco, Opportunities for agri-food chains to become energy-smart, FAO and USAID, 2015, p. 212 Search PubMed.
-
FAO and UN-Water, Progress on change in water-use efficiency-Global status and acceleration needs for SDG indicator 6.4.1., FAO and UN Water, Rome, 2021, DOI:10.4060/cb6413en.
- J. A. Burney, R. L. Naylor and S. L. Postel, The case for distributed irrigation as a development priority in sub-Saharan Africa, Proc. Natl. Acad. Sci. U. S. A., 2013, 110(31), 12513–12517, DOI:10.1073/pnas.1203597110.
-
FAO, The State of the World's Land and Water Resources: Managing Systems at Risk, FAO, 2011, [Online], Available: http://www.fao.org/3/i1688e/i1688e.pdf Search PubMed.
- T. Ding, L. Fang, J. Chen, J. Ji and Z. Fang, Exploring the relationship between water-energy-food nexus sustainability and multiple ecosystem services at the urban agglomeration scale, Sustain. Prod. Consum., 2023, 35, 184–200, DOI:10.1016/j.spc.2022.10.028.
- M. H. Yuan and S. L. Lo, Ecosystem services and sustainable development: Perspectives f1 rom the food-energy-water Nexus, Ecosyst. Serv., 2020, 46, 101217, DOI:10.1016/j.ecoser.2020.101217.
- D. Conway, A. Pereschino, S. Ardoin-Bardin, H. Hamandawana, C. Dieulin and G. Mahé, Rainfall and water resources variability in sub-Saharan Africa during the twentieth century, J. Hydrometeorol., 2009, 10(1), 41–59, DOI:10.1175/2008JHM1004.1.
- T. Mabhaudhi, S. Mpandeli, A. Madhlopa, A. T. Modi, G. Backeberg and L. Nhamo, Southern Africa’s water-energy nexus: Towards regional integration and development, Water, 2016, 8, 235, DOI:10.3390/w8060235.
- H. S. Endris,
et al., Assessment of the performance of CORDEX regional climate models in simulating East African rainfall, J. Clim., 2013, 26(21), 8453–8475, DOI:10.1175/JCLI-D-12-00708.1.
-
S. E. Nicholson, A review of climate dynamics and climate variability in eastern Africa, in The Limnology, Climatology and Paleoclimatology of the East AfricanLakes, CRC Press, 1996, pp. 25–56 Search PubMed.
- L. J. Ogallo, Relationships between seasonal rainfall in East Africa and the Southern Oscillation, J. Climatol., 1988, 8(1), 31–43, DOI:10.1002/joc.3370080104.
-
M. Tsalefac, F. Hiol, G. Mahé, A. Laraque, D. Sonwa, P. Scholte, W. Pokam, A. Haensler, T. Beyene, F. Ludwig, F. K. Mkamkam, V. Manesta Djoufack, M. Ndjatsana and C. Doumenge, Climate of Central Africa: past, present and future, in The forests of the Congo Basin - Forests and climate change, ed. C. De Wasseige, M. Tadou and R.Eba'a Atyi, Belgium, 2015, pp. 37–52 Search PubMed.
-
A. Godard and M. Tabeaud, Les Climats - Mécanismes, variabilités, répartition, Armand Colin, Paris, 4th edn, 2009 Search PubMed.
-
O. Mahjoub, M. T. Chaibi and M. A. Shamseddin, Water Resource Availability and Quality in the North Africa Region Under Climate Change, in Climate Change and Water Resources in Africa, Springer International Publishing, Cham, 2021, pp. 29–54, DOI:10.1007/978-3-030-61225-2_3.
-
A. Mirzabaev, et al., Desertification, in IPCC, 2019: Climate Change and Land: an IPCC special report on climate change, desertification, land degradation, sustainable land management, food security, and greenhouse gas fluxes in terrestrial ecosystems, ed. P. R. Shukla, J. Skea, E. Calvo
Buendia, V. Masson-Delmotte, H.-O. Pörtner, D. Roberts, P. Zhai, R. Slade, S. Connors, R. van Diemen, M. Ferrat, E. Haughey, S. Luz, S. Neogi, M. Pathak, J. Petzold, J. Portugal Pereira, P. Vyas, E. Huntley, K. Kissick, M. Belkacemi and J. Malley, Cambridge University Press, 2019, pp. 249–344, DOI:10.1017/9781009157988.005.
-
IPCC, IPCC Special Report on Climate Change, Desertification, Land Degradation, Sustainable Land Management, Food Security, and Greenhouse gas fluxes in Terrestrial Ecosystems, Geneva, Switzerland, 2018 Search PubMed.
- A. Ahmadalipour, H. Moradkhani, A. Castelletti and N. Magliocca, Future drought risk in Africa: Integrating vulnerability, climate change, and population growth, Sci. Total Environ., 2019, 662, 672–686, DOI:10.1016/j.scitotenv.2019.01.278.
- Z. W. Kundzewicz, V. Krysanova, R. E. Benestad, Ø. Hov, M. Piniewski and I. M. Otto, Uncertainty in climate change impacts on water resources, Environ. Sci. Policy, 2018, 79, 1–8, DOI:10.1016/j.envsci.2017.10.008.
- N. A. Elagib, Development and application of a drought risk index for food crop yield in Eastern Sahel, Ecol. Indic., 2014, 43, 114–125, DOI:10.1016/j.ecolind.2014.02.033.
-
V. R. Nalule, Energy Access in Sub-Saharan Africa, in Energy Poverty and Access Challenges in Sub-Saharan Africa, Springer International Publishing, Cham, 2019, pp. 21–39, DOI:10.1007/978-3-319-95402-8_2.
-
A. Brew-Hammond, G. S. Mensah and O. Amponsah, Energy Poverty in Sub-Saharan Africa, in Energy Poverty, Oxford University Press, 2014, pp. 296–315, DOI:10.1093/acprof:oso/9780199682362.003.0015.
-
M. P. Blimpo and M. Cosgrove-Davies, Electricity Access in sub-Saharan Africa: Uptake, Reliability, and Complementary Factors for Economic Impact, World Bank, 2019, [Online], Available: http://hdl.handle.net/10986/31333 Search PubMed.
-
IEA, IEA (2022), SDG7: Data and Projections, Paris, 2022, [Online], Available: https://www.iea.org/reports/sdg7-data-and-projections Search PubMed.
-
World Health Observatory, Global health observatory data repository: population using solid fuels, Geneva, 2015 Search PubMed.
-
E. Puzzolo and D. Pope, Clean Fuels for Cooking in Developing Countries, in Encyclopedia of Sustainable Technologies, Elsevier, 2017, vol. 4, pp. 289–297, DOI:10.1016/B978-0-12-409548-9.10153-8.
- I. Hanif, Energy consumption habits and human health nexus in Sub-Saharan Africa, Environ. Sci. Pollut. Res., 2018, 25(22), 21701–21712, DOI:10.1007/s11356-018-2336-0.
- M. K. Abdelrazik, S. E. Abdelaziz, M. F. Hassan and T. M. Hatem, Climate action: Prospects of solar energy in Africa, Energy Rep., 2022, 8, 11363–11377, DOI:10.1016/j.egyr.2022.08.252.
-
A. Belward, B. Bisselink, K. Bódis, A. Brink, J.-F. Dallemand, A. de Roo, T. Huld, F. Kayitakire, P. Mayaux, M. Moner-Girona, H. Ossenbrink, I. Pinedo, H. Sint, J. Thielen, S. Szabó, U. Tromboni and L. Willemen, Renewable energies in Africa Current knowledge, EurCommission, 2012, DOI:10.2788/1881.
-
IRENA and AfDB, Renewable Energy Market Analysis: Africa and Its Regions, International Renewable Energy Agency and African Development Bank, Abu Dhabi and Abidjan, 2022 Search PubMed.
- C. D. Iweh, S. Gyamfi, E. Tanyi and E. Effah-Donyina, Distributed generation and renewable energy integration into the grid: Prerequisites, push factors, practical options, issues and merits, Energies, 2021, 14(5375) DOI:10.3390/en14175375.
- A. A. Ogundipe, O. Akinyemi and O. M. Ogundipe, Energy access: Pathway to attaining sustainable development in Africa, Int. J. Energy Econ. Policy, 2018, 8(6), 371–381, DOI:10.32479/ijeep.7213.
- O. K. Bishoge, G. G. Kombe and B. N. Mvile, Renewable energy for sustainable development in sub-Saharan African countries: Challenges and way forward, J. Renewable Sustainable Energy, 2020, 12, 052702, DOI:10.1063/5.0009297.
-
I. Niang, et al., Climate Change 2014: Impacts, Adaptation, and Vulnerability. Part B: Regional Aspects, in Fifth Assessment Report of the Intergovernmental Panel on Climate Change, Cambridge, United Kingdom and New York, Cambridge University Press, 2014, pp. 1199–1260 Search PubMed.
- K. M. Gebru,
et al., Adoption of road water harvesting practices and their impacts: Evidence from a semi-arid region of Ethiopia, Sustainability, 2020, 12(21), 1–25, DOI:10.3390/su12218914.
-
I. K. Mpanga, D. S. Gaikpa, E. Koomson and H. K. Dapaah, Innovations in Water Management: Agriculture, in The Palgrave Handbook of Global Sustainability, Springer International Publishing, Cham, 2022, pp. 1–23, DOI:10.1007/978-3-030-38948-2_33-1.
-
M. Ben-Hur, Water Use Efficiency in Agriculture: Opportunities for Improvement, in Encyclopedia of earth sciences series, 2011, pp. 979–984, DOI:10.1007/978-90-481-3585-1_186.
- N. Kiggundu,
et al., Rainwater harvesting knowledge and practice for agricultural production in a changing climate: A review from Uganda's perspective, Agric. Eng. Int.: CIGR J., 2018, 20(2), 19–36 Search PubMed.
- M. A. Kendouci, A. Bendida, R. Khelfaoui and B. Kharroubi, The impact of traditional irrigation (Foggara) and modern (drip, pivot) on the resource non-renewable groundwater in the Algerian Sahara, Energy Procedia, 2013, 36, 154–162, DOI:10.1016/j.egypro.2013.07.018.
- D. R. Lightfoot, Moroccan khettara: Traditional irrigation and progressive desiccation, Geoforum, 1996, 27(2), 261–273, DOI:10.1016/0016-7185(96)00008-5.
- D. R. Lightfoot, Qanats in the levant: Hydraulic technology at the periphery of early empires, Technol. Cult., 1997, 38(2), 432–450, DOI:10.2307/3107129.
-
B. Tobbi, Water harvesting: historic, existing and potentials in Tunisia, in Expert consultation on Water Harvesting for improved agricultural production, Cairo 21-25 November 1993, ed. FAO, Rome, Italy, 1994, pp. 189–201 Search PubMed.
- Runoff Harvesting for Crop Production: Practical Solutions for Dryland Agriculture, Training Manual 1. Nile Basin Initiative (NBI), Nile Equatorial Lakes Subsidiary Action Programme (NELSAP) - Regional Agricultural and Trade Programme (RATP), 2012, vol. 1, pp. 1–60 Search PubMed.
-
M. Rajouene and B. S. Asma, Impact du Système Meskat sur le Comportement de l' Olivier (Olea Europaea L., CV Chemlali) en Milieu Semi-Aride Tunisien, 2012, vol. 92, (4), pp. 510–525 Search PubMed.
-
A. Salih, J. Khouri, M. Abdulrazzak, M. Kallel and H. Adam, Rainfall water management in the Arab region: state of the art report, Cairo, Egypt, 1995 Search PubMed.
- B. Remini, The role of the gallery in the functioning of the foggara, J. Water Land Dev., 2016, 29(1), 49–57, DOI:10.1515/jwld-2016-0011.
-
N. Ben Mechlia and M. Ouessar, Water harvesting systems in Tunisia, in Indigenous water harvesting systems in West Asia and North Africa, ed. ICARDA, ICARDA, Aleppo, Syria, 2004, pp. 19–41 Search PubMed.
-
WOCAT, Where the land is greener—case studies and analysis of soil and water conservation initiatives worldwide, Hanspeter Liniger and William Critchley, 2007, vol. 28, (2), DOI:10.1659/mrd.mm028.
- M. N. Danjuma and S. Mohammed, Zai Pits System: A Catalyst for Restoration in the Dry Lands, IOSR J. Agric. Vet. Sci., 2015, 8(2), 01–04, DOI:10.9790/2380-08210104.
- D. Fatondji, C. Martius, C. L. Bielders, P. L. G. Vlek, A. Bationo and B. Gerard, Effect of planting technique and amendment type on pearl millet yield, nutrient uptake, and water use on degraded land in Niger, Nutr. Cycling Agroecosyst., 2006, 76(2–3), 203–217, DOI:10.1007/s10705-005-6209-9.
-
B. M. Mati, Overview of water and soil nutrient management under smallholder rain- fed agriculture in East Africa. Working Paper 105. Colombo, Sri Lanka, International Water Management Institute (IWMI), 2005, vol. 105, p. 81 Search PubMed.
- T. V. Pesanayi and K. N. Weaver, Collaborative learning of water conservation practices: Cultivation and expansion of a learning network around rainwater harvesting demonstration sites in the Eastern Cape, South Africa, S. Afr. J. Agric. Ext., 2016, 44(1), 131–145, DOI:10.17159/2413-3221/2016/v44n1a386.
- T. Pedzisa, L. Rugube, A. Winter-Nelson, K. Baylis and K. Mazvimavi, Abandonment of conservation agriculture by smallholder farmers in Zimbabwe, J. Sustain. Dev., 2015, 8(1), 69–82, DOI:10.5539/jsd.v8n1p69.
- S. Siebert,
et al., Groundwater use for irrigation - A global inventory, Hydrol. Earth Syst. Sci., 2010, 14(10), 1863–1880, DOI:10.5194/hess-14-1863-2010.
-
M. Svendsen, Measuring Irrigation Performance in Africa, 2009 Search PubMed.
-
FAO, AQUASTAT - FAO's Global Information System on Water and Agriculture, https://www.fao.org/aquastat/en/ Search PubMed.
-
C. Ward, R. Torquebiau and H. Xie, Improved Agricultural Water Management for Africa's Drylands, World Bank, Washington, DC, 2016, DOI:10.1596/978-1-4648-0832-6.
- N. Haruvy, Agricultural reuse of wastewater: Nation-wide cost-benefit analysis, Agric., Ecosyst. Environ., 1997, 66(2), 113–119, DOI:10.1016/S0167-8809(97)00046-7.
- S. Raghuvanshi, V. Bhakar, C. Sowmya and K. S. Sangwan, Waste Water Treatment Plant Life Cycle Assessment: Treatment Process to Reuse of Water, Procedia CIRP, 2017, 61, 761–766, DOI:10.1016/j.procir.2016.11.170.
-
W. R. and F. Ministry of Agriculture, Rapport National Rapport National, 2021, vol. 2226, pp. 1–145, [Online], Available: file:///Users/air/Desktop/Morocco_Rapport_EPT2011fr.pdf Search PubMed.
- J. R. Adewumi, A. A. Ilemobade and J. E. Van Zyl, Treated wastewater reuse in South Africa: Overview, potential and challenges, Resour., Conserv. Recycl., 2010, 55(2), 221–231, DOI:10.1016/j.resconrec.2010.09.012.
-
WWAP, The United Nations World Water Development Report 2017: Wastewater the Untapped Resources, Paris, France, 2017 Search PubMed.
- J. K. Debrah, G. K. Teye and M. A. P. Dinis, Barriers and Challenges to Waste Management Hindering the Circular Economy in Sub-Saharan Africa, Urban Sci., 2022, 6(3), 57, DOI:10.3390/urbansci6030057.
- L. F. Diaz, Waste management in developing countries and the circular economy, Waste Manage. Res., 2017, 35(1), 1–2, DOI:10.1177/0734242X16681406.
-
K. Ghebremichael, J. Eckart, K. Khatri and K. Vairavamoorthy, Integrated water resources management, 2015, p. 4, DOI:10.4324/9781315693606-54.
- E. M. Biggs,
et al., Environmental Science & Policy Sustainable development and the water – energy – food nexus: A perspective on livelihoods, Environ. Sci. Policy, 2015, 54, 389–397, DOI:10.1016/j.envsci.2015.08.002.
- M. Lafforgue and V. Lenouvel, Closing the urban water loop: Lessons from Singapore and Windhoek, Environ. Sci.: Water Res. Technol., 2015, 1(5), 622–631, 10.1039/c5ew00056d.
- R. Peeters, H. Vanderschaeghe, J. Rongé and J. A. Martens, Energy performance and climate dependency of technologies for fresh water production from atmospheric water vapour, Environ. Sci.: Water Res. Technol., 2020, 6(8), 2016–2034, 10.1039/d0ew00128g.
-
H. Hoff, Background paper for the Bonn 2011 Nexus Conference: Understanding the Nexus, in Bonn2011 Conference: The Water, Energy and Food Security Nexus, Stockholm Environment Institute, Stockholm Search PubMed.
-
FAO, The state of food and agriculture: Overcoming water challenges in agriculture, Rome, Italy, 2020 Search PubMed.
-
IPBS, The global assessment report on biodiversity and ecosystem services, 2019 Search PubMed.
-
T. Benton, C. Bieg, H. Harwatt, R. Pudassaini and L. Wellesley, Food system impacts on biodiversity loss, 2021 Search PubMed.
- R. Subedi, M. Karki and D. Panday, Food System and Water–Energy–Biodiversity Nexus in Nepal: A Review, Agronomy, 2020, 10(8), 1129, DOI:10.3390/agronomy10081129.
-
HLPE, Agroecological and other innovative approaches for sustainable agriculture and food systems that enhance food security and nutrition, 2019 Search PubMed.
- F. Muhirwa,
et al., Ecological balance emerges in implementing the water-energy-food security nexus in well-developed countries in Africa, Sci. Total Environ., 2022, 833, 154999, DOI:10.1016/j.scitotenv.2022.154999.
-
M. G. Jacobson, Water–energy–food nexus approaches to facilitate smallholder agricultural technology adoption in Africa, in Water - Energy - Food Nexus Narratives and Resource Securities, Elsevier, 2022, pp. 279–297, DOI:10.1016/B978-0-323-91223-5.00018-6.
- UN General Assembly, Transforming our world: the 2030 Agenda for Sustainable Development.
-
The African Union Commission, AGENDA 2063: The Africa We Want - First ten-year Implementation Plan 2013-2023, 2015 Search PubMed.
-
C. Juma and I. Serageldin, Rebooting African Development - Science, Technology, and Globalization Project Report, 2016 Search PubMed.
-
A. E. Cheo, N. Adelhardt and T. Krieger, Agrivoltaics across the Water-Energy-Food-Nexus in Africa: Opportunities and Challenges for Rural Communities in Mali, Discuss. Pap. Ser., 2022, vol. 3, pp. 1–22, DOI:10.21203/rs.3.rs-1503422/v1.
- M. Trommsdorff, J. Kang, C. Reise, S. Schindele, G. Bopp, A. Ehmann, A. Weselek, P. Hogy and T. Obergfell, Combining food and energy production: Design of an agrivoltaic system applied in arable and vegetable farming in Germany, Renewable Sustainable Energy Rev., 2021, 140, 110694, DOI:10.1016/j.rser.2020.110694.
- P. Santra, P. C. Pande, S. Kumar, D. Mishra and R. K. Singh, Agri-voltaics or solar farming: The concept of integrating solar PV based electricity generation and crop production in a single land use system, Int. J. Renew. Energy Res., 2017, 7(2), 694–699, DOI:10.20508/ijrer.v7i2.5582.g7049.
- C. Toledo and A. Scognamiglio, Agrivoltaic systems design and assessment: A critical review, and a descriptive model towards a sustainable landscape vision (three-dimensional agrivoltaic patterns), Sustainability, 2021, 13, 6871, DOI:10.3390/su13126871.
- G. A. Barron-Gafford,
et al., Agrivoltaics provide mutual benefits across the food–energy–water nexus in drylands, Nat. Sustain., 2019, 2(9), 848–855, DOI:10.1038/s41893-019-0364-5.
- L. J. Walston, T. Barley, I. Bhandari, B. Campbell, J. McCall, H. M. Hartmann and A. G. Dolezal, Opportunities for agrivoltaic systems to achieve synergistic food-energy-environmental needs and address sustainability goals, Front. Sustain. Food Syst., 2022, 6, 932018, DOI:10.3389/fsufs.2022.932018.
-
L. Cozzi, T. Gould, S. Bouckart, D. Crow, T. Y. Kim, C. McGlade, P. Olejarnik, B. Wanner and D. Wetzel, World Energy Outlook 2020, IEA 2020, pp. 213–250, DOI:10.1787/557a761b-en.
-
Fraunhofer ISE, APV-MaGa – Agrivoltaics for Mali and Gambia: Sustainable Electricity Production by Integrated Food, Energy and Water Systems, https://www.ise.fraunhofer.de/en/research-projects/apv-maga.html, (accessed Aug. 20, 2023) Search PubMed.
-
M. Trommsdorff, S. Gruber, T. Keinath, M. Hopf, C. Hermann, F. Schönberger, P. Högy, S. Zikeli, A. Ehmann, A. Weselek, U. Bodmer, C. Rösch, D. Ketzer, N. Weinberger, S. Schindele and J. Vollprecht, Agrivoltaics: Opportunities for Agri-culture and the Energy Transition, 2022, vol. 114, p. 76 Search PubMed.
- R. J. Randle-Boggis, E. Lara, J. Onyango, E. J. Temu and S. E. Hartley, Agrivoltaics in East Africa: Opportunities and challenges, AIP Conf. Proc., 2021, 2361, 1–8, DOI:10.1063/5.0055470.
- S. Mohammed Wazed, B. R. Hughes, D. O'Connor and J. Kaiser Calautit, A review of sustainable solar irrigation systems for Sub-Saharan Africa, Renew. Sustain. Energy Rev., 2018, 81, 1206–1225, DOI:10.1016/j.rser.2017.08.039.
-
H. Y. Abdelmajid and A. Ben Hmid, Etude d'Opportunité du Solaire Photovoltaïque dans les Secteurs Etude d' Opportunité du Solaire Photovoltaïque dans les Secteurs Agricole & Agroalimentaire, 2016, [Online], Available: http://www.onagri.nat.tn/uploads/Etudes/Etude-opportunite-photovoltaique.pdf Search PubMed.
- I. Mekki, I. Ferchichi, N. Taouajouti, N. Faysse and A. Zairi, Oasis extension trajectories in Kebili territory, Southern Tunisia: drivers of development and actors' discourse, New Medit, 2022, 2022(5), 85–101, DOI:10.30682/nm2205f.
-
M. T. Chaibi, Global Symposium on Sustainable Water and Energy Solutions, United Nations, Paraguay, Brazil, 2022 Search PubMed.
- N. Sharma, S. Acharya, K. Kumar, N. Singh and O. P. Chaurasia, Hydroponics as an advanced technique for vegetable production: An overview, J. Soil Water Conserv., 2018, 17(4), 364, DOI:10.5958/2455-7145.2018.00056.5.
- T. K. Upadhyay, S. Kumar Sharma, T. Kumar Upadhyay and P. Singh, Impact of Hydroponics: Present and Future Perspective for Farmer's Welfare, Sci. Technol., 2019, 5(2), 19–26 Search PubMed , [Online], Available: https://www.researchgate.net/publication/337591596.
- D. I. Pomoni, M. K. Koukou, M. G. Vrachopoulos and L. Vasiliadis, A Review of Hydroponics and Conventional Agriculture Based on Energy and Water Consumption, Environmental Impact, and Land Use, Energies, 2023, 16(4), 1690, DOI:10.3390/en16041690.
- M. A. Martinez-Mate, B. Martin-Gorriz, V. Martínez-Alvarez, M. Soto-García and J. F. Maestre-Valero, Hydroponic system and desalinated seawater as an alternative farm-productive proposal in water scarcity areas: Energy and greenhouse gas emissions analysis of lettuce production in southeast Spain, J. Cleaner Prod., 2018, 172, 1298–1310, DOI:10.1016/j.jclepro.2017.10.275.
- R. S. Velazquez-Gonzalez, A. L. Garcia-Garcia, E. Ventura-Zapata, J. D. O. Barceinas-Sanchez and J. C. Sosa-Savedra, A Review on Hydroponics and the Technologies Associated for Medium-and Small-Scale Operations, Agriculture, 2022, 12(5), 1–21, DOI:10.3390/agriculture12050646.
- B. Yep and Y. Zheng, Aquaponic trends and challenges – A review, J. Cleaner Prod., 2019, 228, 1586–1599, DOI:10.1016/j.jclepro.2019.04.290.
- G. L. Barbosa,
et al., Comparison of land, water, and energy requirements of lettuce grown using hydroponic vs. Conventional agricultural methods, Int. J. Environ. Res. Public Health, 2015, 12(6), 6879–6891, DOI:10.3390/ijerph120606879.
- P. Bradley and C. Marulanda, Simplified hydroponics to reduce global hunger, Acta Hortic., 2001, 554, 289–296, DOI:10.17660/ActaHortic.2001.554.31.
-
C. Somerville, M. Cohan, E. Pantanella and A. Stankus, Small-scale aquaponic food production, FAO Fisheries and aquaculture technical paper, FAO, 2014, vol. 589, p. 288 Search PubMed.
- V. T. Okomoda, S. A. Oladimeji, S. G. Solomon, S. O. Olufeagba, S. I. Ogah and M. Ikhwanuddin, Aquaponics production system: A review of historical perspective, opportunities, and challenges of its adoption, Food Sci. Nutr., 2022, 00, 1–9, DOI:10.1002/fsn3.3154.
- N. Mchunu, G. Lagerwall and A. Senzanje, Aquaponics in South Africa: Results of a national survey, Aquac. Rep., 2018, 12, 12–19, DOI:10.1016/j.aqrep.2018.08.001.
- N. Mchunu, G. Lagerwall and A. Senzanje, Food Sovereignty for Food Security, Aquaponics System as a Potential Method: A Review, J. Aquacult. Res. Dev., 2017, 08(07) DOI:10.4172/2155-9546.1000497.
- M. C. M. Beveridge, S. H. Thilsted, M. J. Phillips, M. Metian, M. Troell and S. J. Hall, Meeting the food and nutrition needs of the poor: The role of fish and the opportunities and challenges emerging from the rise of aquaculturea, J. Fish Biol., 2013, 83(4), 1067–1084, DOI:10.1111/jfb.12187.
-
E. O. Benjamin, D. Tzemi and D. S. Fialho, Sustainable Urban Farming in Sub-Saharan Africa: A Review of a Coupled Single-Loop Aquaponics System in Nigeria, 2021, pp. 1–34, DOI:10.20944/preprints202111.0372.v1.
-
P. G. M. Van der Heijden, F. Farrag and G. Blom-Zandstra, Bustan aquaponics: Egypt's first working commercial aquaponic farm, Aquaculture Europe, 2013, 38, (2) Search PubMed.
-
G. Zipporah, H. Waidbacher, W. Zollitsch, S. Drexler and D. Liti, The Potential of Aquaponics as Food Production and Nutrient Recovery Systems in Kenya, inInternational Scientific Days 2018, Towards Productive, Sustainable and Resilient Global Agriculture and Food Systems: Proceedings, Wolters Kluwer ČR, Prague, 2018, 1154, DOI:10.15414/isd2018.s4.21.
- K. Tokunaga, C. Tamaru, H. Ako and P. Leung, Economics of small-scale commercial aquaponics in hawai'i, J. World Aquacult. Soc., 2015, 46(1), 20–32, DOI:10.1111/jwas.12173.
- H. W. Palm,
et al., Towards commercial aquaponics: a review of systems, designs, scales and nomenclature, Aquacult. Int., 2018, 26(3), 813–842, DOI:10.1007/s10499-018-0249-z.
- R. V. Tyson, D. D. Treadwell and E. H. Simonne, Opportunities and Challenges to Sustainability in Aquaponic Systems, HortTechnology, 2011, 21(1), 6–13, DOI:10.21273/HORTTECH.21.1.6.
- L. A. Nuamah,
et al., Constructed wetlands, status, progress, and challenges. The need for critical operational reassessment for a cleaner productive ecosystem, J. Cleaner Prod., 2020, 269, 122340, DOI:10.1016/j.jclepro.2020.122340.
- A. M. N. Masoud, A. Alfarra and S. Sorlini, Constructed Wetlands as a Solution for Sustainable Sanitation: A Comprehensive Review on Integrating Climate Change Resilience and Circular Economy, Water, 2022, 14, 3232, DOI:10.3390/w14203232.
-
E. Tilgalis and L. Grinberga, Energy-efficient wastewater treatment technologies in constructed wetlands, Civ. Eng. '11 - 3rd Int. Sci. Conf. Proc., 2011, 3, pp. 263–266 Search PubMed.
- K. Ergaieg and T. Ben Miled, Full-scale hybrid constructed wetlands monitoring for decentralized tertiary treatment of municipal wastewater, Arabian J. Geosci., 2021, 14(1407) DOI:10.1007/s12517-021-07776-y.
-
S. Alexander and R. McInnes, The benefits of wetland restoration, Ramsar Scientific and Technical Briefing Note, 2012, vol. 4, DOI:10.13140/RG.2.1.3024.1124.
- S. Bikangaga, M. P. Picchi, S. Focardi and C. Rossi, Perceived benefits of littoral wetlands in Uganda: A focus on the Nabugabo wetlands, Wetlands Ecol. Manage., 2007, 15(6), 529–535, DOI:10.1007/s11273-007-9049-3.
-
M. S. Businge, J. Nyunja, S. O. Ochola, B. Pengra and E. A. Ochieng, Kenya Wetland Atlas, 2012 Search PubMed.
-
S. den Haring, Constructed Wetlands in a Community Setting in Mombasa, Kenya, in Constructed Wetlands for Wastewater Treatment in Hot and Arid Climates. Wetlands: Ecology, Conservation and Management, ed. A. Stefanakis, Springer, Cham, 2022, pp. 137–152, DOI:10.1007/978-3-031-03600-2_8.
- R. O. B. Makopondo, L. K. Rotich and C. G. Kamau, Potential Use and Challenges of Constructed Wetlands for Wastewater Treatment and Conservation in Game Lodges and Resorts in Kenya, Sci. World J., 2020 DOI:10.1155/2020/9184192.
- F. M. Zahui, J. M. P. Ouattara, M. Kamagaté, L. Coulibaly and A. I. Stefanakis, Effect of plant species on the performance and bacteria denstity profile in vertical flow constructed wetlands for domestic wastewater treatment in a tropical climate, Water, 2021, 13, 3485, DOI:10.3390/w13243485.
- A. I. Stefanakis, Constructed wetlands for sustainable wastewater treatment in hot and arid climates: Opportunities, challenges and case studies in the Middle East, Water, 2020, 12, 1665, DOI:10.3390/W12061665.
- M. Soussi, M. T. Chaibi, M. Buchholz and Z. Saghrouni, Comprehensive Review on Climate Control and Cooling Systems in Greenhouses under Hot and Arid Conditions, Agronomy, 2022, 12, 626, DOI:10.3390/agronomy12030626.
- M. T. Chaibi, An overview of solar desalination for domestic and agriculture water needs in remote arid areas, Desalination, 2000, 127(2), 119–133, DOI:10.1016/S0011-9164(99)00197-6.
-
J. Martínez Beltrán and S. Koo-Oshima, Water desalination for agricultural applications, in Proceedings of the FAO Expert Consultation on Water Desalination for Agricultural Applications, FAO, Rome, Italy, 2006 Search PubMed.
- M. Chaibi, Thermal Solar Desalination Technologies for Small-Scale Irrigation, Am. J. Energy Res., 2013, 1(2), 25–32, DOI:10.12691/ajer-1-2-1.
-
K. Ergaieg, N. Farhani, J. Ben Nasr, N. Rehaiem and D. Ghanmi, Irrigation water desalination potential by reverse osmosis: Gounat case study in Tunisia, 3rd International Conference on Integrated Environmental Management for Sustainable Development, 2018 Search PubMed.
- M. T. Chaibi and T. Jilar, System design, operation and performance of roof-integrated desalination in greenhouses, Sol. Energy, 2004, 76(5), 545–561, DOI:10.1016/j.solener.2003.12.008.
-
J. El Kharraz, Desalination as an alternative to alleviate water scarcity and a climate change adaptation option in the MENA region, in partnership with Regional Program Energy Security and Climate Change Middle East and North Africa of Konrad-Adenauer-Stiftung (KAS-RE), 2020, [Online], Available: https://www.kas.de/remena Search PubMed.
- S. Burn,
et al., Desalination techniques - A review of the opportunities for desalination in agriculture, Desalination, 2015, 364, 2–16, DOI:10.1016/j.desal.2015.01.041.
- A. G. Olabi, K. Elsaid, M. K. H. Rabaia, A. A. Askalany and M. A. Abdelkareem, Waste heat-driven desalination systems: Perspective, Energy, 2020, 209, 118373, DOI:10.1016/j.energy.2020.118373.
- K. Elsaid, E. Taha Sayed, B. A. A. Yousef, M. Kamal Hussien Rabaia, M. Ali Abdelkareem and A. G. Olabi, Recent progress on the utilization of waste heat for desalination: A review, Energy Convers. Manage., 2020, 221, 113105, DOI:10.1016/j.enconman.2020.113105.
- E. T. Sayed, A. G. Olabi, K. Elsaid, M. Al Radi, R. Alqadi and M. Ali Abdelkareem, Recent progress in renewable energy based-desalination in the Middle East and North Africa MENA region, J. Adv. Res., 2023, 48, 125–156, DOI:10.1016/j.jare.2022.08.016.
-
M. T. Chaibi and A. M. El-nashar, A Review of Solar Thermal Energy Technologies for Water Desalination, 2009, DOI:10.1007/978-3-642-01150-4.
- N. Shekarchi and F. Shahnia, A comprehensive review of solar-driven desalination technologies for off-grid greenhouses, Int. J. Energy Res., 2019, 43(4), 1357–1386, DOI:10.1002/er.4268.
- H. Jouhara and A. G. Olabi, Editorial: Industrial waste heat recovery, Energy, 2018, 160, 1–2, DOI:10.1016/j.energy.2018.07.013.
- M. H. Nour, A. Ghanem, M. Buchholz and A. Nassar, Greenhouse based desalination for brackish water management using bittern evaporative cooling technique, Water Sci. Technol.: Water Supply, 2015, 15(4), 709–717, DOI:10.2166/ws.2015.025.
- R. M. Shelake, U. S. Kadam, R. Kumar, D. Pramanik, A. K. Singh and J. Y. Kim, Engineering drought and salinity tolerance traits in crops through CRISPR-mediated genome editing: Targets, tools, challenges, and perspectives, Plant Commun., 2022, 3(6), 100417, DOI:10.1016/j.xplc.2022.100417.
- A. Hamdy, S. Abdel-Dayem and M. Abu-Zeid, Saline water management for optimum crop production, Agric. Water Manag., 1993, 24(3), 189–203, DOI:10.1016/0378-3774(93)90023-4.
-
L. Karlberg, Irrigation With Saline Water Using Low-cost Drip-Irrigation Systems in Sub-Saharan Africa, PhD thesis, KTH, 2005 Search PubMed.
- R. W. Healy, W. M. Alley, M. A. Engle, P. B. McMahon and J. D. Bales, The Water-Energy Nexus — An Earth Science Perspective, Geol. Surv. Circ., 2015, 1407, 122, DOI:10.3133/cir1407.
- K. Sawaya, Extending satellite remote sensing to local scales: land and water resource monitoring using high-resolution imagery, Remote Sens. Environ., 2003, 88, 144–156, DOI:10.1016/j.rse.2003.04.0006.
- R. Hakimdavar,
et al., Monitoring water-related ecosystems with earth observation data in support of Sustainable Development Goal (SDG) 6 reporting, Remote Sens., 2020, 12(10), 1–26, DOI:10.3390/rs12101634.
- G. D'Urso,
et al., Earth Observation products for operational irrigation management in the context of the PLEIADeS project, Agric. Water Manag., 2010, 98(2), 271–282, DOI:10.1016/j.agwat.2010.08.020.
- S. Vanino, P. Nino, C. De Michele, S. F. Bolognesi and G. Pulighe, Earth Observation for Improving Irrigation Water Management: A Case-study from Apulia Region in Italy, Agric. Agric. Sci. Procedia, 2015, 4, 99–107, DOI:10.1016/j.aaspro.2015.03.012.
- A. Andries, S. Morse, R. J. Murphy, J. Lynch and E. R. Woolliams, Using Data from Earth Observation to Support Sustainable Development Indicators: An Analysis of the Literature and Challenges for the Future, Sustainability, 2022, 14, 1191, DOI:10.3390/su14031191.
- S. H. Qader, J. Dash, V. A. Alegana, N. R. Khwarahm, A. J. Tatem and P. M. Atkinson, The role of earth observation in achieving sustainable agricultural production in arid and semi-arid regions of the world, Remote Sens., 2021, 13(17), 1–27, DOI:10.3390/rs13173382.
- G. Laneve, S. Saquella, W. Huang and R. Orsi, Sino-Eu Earth Observation Data to Support the Monitoring and Management of Agricultural Resources, Int. Geosci. Remote Sens. Symp., 2022, 4372–4375, DOI:10.1109/IGARSS46834.2022.9884557.
- C. Lavaysse, P. Roudier, V. Venkatachalam, J. Van't Klooster and M. Clerici, On the use of the estation developed in the GMES & Africa EU Project: Results from the user survey, Atmosphere, 2021, 12(2), 1–15, DOI:10.3390/atmos12020258.
- C. Nakalembe, I. Becker-Reshef, R. Bonifacio, G. Hu, M. L. Humber, C. J. Justice, J. Keniston, K. Mwangi, F. Rembold, S. Shukla, F. Urbano, A. K. Whitcraft, Y. Li, M. Zappacosta, I. Jarvis and A. Sanchez, A review of satellite-based global agricultural monitoring systems available for Africa, Glob. Food Sec., 2021, 29, 100543, DOI:10.1016/j.gfs.2021.100543.
- C. Ifejika Speranza, F. Olufunmilayo Akinyemi, D. Baratoux, J. Benveniste, N. Ceperley, F. Driouech and J. Helmschrot, Enhancing the Uptake of Earth Observation Products and Services in Africa Through a Multi-level Transdisciplinary Approach, Surveys in Geophysics, 2023, 44, 7–41, DOI:10.1007/s10712-022-09724-1.
-
D. Mazvimavi, B. Maathuis, D. Kirugara and D. Fernandez, Water resources management, 1986, DOI:10.13140/2.1.5041.4724.
- A. Ali Omer,
et al., Water evaporation reduction by the agrivoltaic systems development, Sol. Energy, 2022, 247, 13–23, DOI:10.1016/j.solener.2022.10.022.
- I. Mekki, I. Ferchichi, N. Taoujouti, N. Faysse and A. Zairi, Analyse de l'extension des palmeraies oasiennes et de son impact sur les ressources en eau souterraine dans la région de Kébili, sud-ouest de la Tunisie, Ann. l'INRGREF, 2021, 22, 123–143 Search PubMed.
- J. Dalsgaard, I. Lund, R. Thorarinsdottir, A. Drengstig, K. Arvonen and P. B. Pedersen, Farming different species in RAS in Nordic countries: Current status and future perspectives, Aquac. Eng., 2013, 53, 2–13, DOI:10.1016/j.aquaeng.2012.11.008.
- H. El-Essawy, P. Nasr and H. Sewilam, Aquaponics: a sustainable alternative to conventional agriculture in Egypt – a pilot scale investigation, Environ. Sci. Pollut. Res., 2019, 26(16), 15872–15883, DOI:10.1007/s11356-019-04970-0.
- G. Crini and E. Lichtfouse, Advantages and disadvantages of techniques used for wastewater treatment, Environ. Chem. Lett., 2019, 17(1), 145–155, DOI:10.1007/s10311-018-0785-9.
- U. Yermiyahu, A. Tal, A. Ben-Gal and A. Bar-Tal, Rethinking Desalinated Water Quality and Agriculture, Science, 2007, 318, 920–921 CrossRef CAS PubMed.
- L. Karlberg and F. W. T. Penning de Vries, Exploring potentials and constraints of low-cost drip irrigation with saline water in sub-Saharan Africa, Phys. Chem. Earth, 2004, 29, 1035–1042, DOI:10.1016/j.pce.2004.08.004.
- A. F. Abou-Hadid, The use of saline water in agriculture in the Near East and North Africa region: Present and future, J. Crop Prod., 2003, 7(1–2), 299–323, DOI:10.1300/J144v07n01_11.
- T. Brudermann, K. Reinsberger, A. Orthofer, M. Kislinger and A. Posch, Photovoltaics in agriculture: A case study on decision making of farmers, Energy Policy, 2013, 61, 96–103, DOI:10.1016/j.enpol.2013.06.081.
- K. A. Obirikorang, W. Sekey, B. A. Gyampoh, G. Ashiagbor and W. Asante, Aquaponics for Improved Food Security in Africa: A Review, Front. Sustain. Food Syst., 2021, 5, 1–10, DOI:10.3389/fsufs.2021.705549.
|
This journal is © The Royal Society of Chemistry 2024 |
Click here to see how this site uses Cookies. View our privacy policy here.