Adsorption of copper by naturally and artificially aged polystyrene microplastics and subsequent release in simulated gastrointestinal fluid†
Received
15th August 2023
, Accepted 16th January 2024
First published on 17th January 2024
Abstract
Microplastics, especially aged microplastics can become vectors of metals from environment to organisms with potential negative effects on food chain. However, a few studies focused on the bioavailability of adsorbed metals and most studies related to aged microplastics used artificial method that cannot entirely reflect actual aging processes. In this study, virgin polystyrene was aged by ozone (PS-O3), solar simulator (PS-SS) and lake (PS-lake) to investigate adsorption of Cu by virgin, artificially and naturally aged microplastics and subsequent release in simulated gastrointestinal fluids (SGF). Characterization results show carbonyl was formed in PS-O3 and PS-SS, and the oxidation degree was PS-O3 > PS-SS > PS-lake. However, Cu adsorption capacity followed this order PS-lake (158 μg g−1) > PS-SS (117 μg g−1) > PS-O3 (65 μg g−1) > PS-virgin (0). PS-O3 showed highest Cu adsorption capacity at 0.5 h (71 μg g−1), but it dropped dramatically later (10 μg g−1, 120 h), because PS-O3 could break up and the adsorbed Cu released in solutions subsequently. For PS-lake, precipitation of metallic oxides contributes to the accumulation of Cu. The addition of dissolved organic matter (DOM) could occupy adsorption sites on PS and compete with Cu, but also can attach PS and adsorb Cu due to its rich functional groups. The simultaneous ingestion of microplastics with food suggested that adsorbed Cu is solubilized mostly from aged PS to SGF.
Environmental significance
Microplastics are present in all aquatic environments and undergo various ageing processes. They can act as vectors for organic and inorganic micropollutants such as heavy metals that might affect the metabolism of living organisms. This study aims to gain a better understanding of how ageing processes and the dissolved organic matter influence copper adsorption and its bioavailability to living organisms. The results show that the aging of microplastics in lake water leads to the formation of metallic oxides on their surface contributes to the accumulation of Cu. The in vitro digestion of these particles in the presence of food shows that Cu is mainly released in the gastric fluid and could have deleterious effects on aquatic fauna.
|
Introduction
Microplastics have drawn widespread attention due to their occurrence in different ecosystems ranging from waters and sediments, to soils and air.1–3 Microplastics are generally considered to be plastic particles less than 5 mm in diameter, which are formed due to weathering and breakdown of plastics.4,5 Due to the small size and poor degradation rate, microplastics can be mistakenly considered as food and ingested by a wide range of organisms with adverse biological effects.6,7
Besides the direct adverse physical effects caused by the microplastics alone, the organic and inorganic additives and adsorbed exogenous contaminants can also constitute a threat to organisms.8,9 Microplastics can act as vectors of contaminants such as possibly toxic metals from surrounding environment to the bodies of organisms and can disrupt the food chain.10,11 On the one hand, metals and metalloids are widely incorporated in plastic itself.12 On the other hand, microplastics can accumulate metals from environment.13 Concentrations of metals in microplastics are typically on the order of a few ng g−1 to a few μg g−1.12,14,15 In natural aquatic environment, dissolved organic matter (DOM) is ubiquitous due to the degradation of plants and animals residuals.16,17 When microplastics enter the environment, they can interact with DOM, and affects the adsorption of metals because the DOM not only affects the surface charge properties, but could also contains functional groups such as hydroxyl, carboxyl, and amino that can form complexes with metals.8,18 Therefore, it is important to investigate the adsorption of metals on microplastics in the presence of DOM. In addition, most present research studied the adsorption of metals by microplastics, but studies related to the bioavailability of adsorbed metals to organisms is comparatively much scarcer.19–23 Therefore, it is important to investigate the adsorption of metals by microplastics and subsequently release in biological fluids, to assess the contribution of microplastics as a source of metals for aquatic organisms such as fish.
Microplastics released in the environment are susceptible to photo-degradation by ultraviolet radiation, thermal degradation, mechanical abrasion, biodegradation, and oxidation reactions.24,25 These processes can change the physical and chemical properties of microplastics, including morphology, hydrophobicity, mechanical strength, molecular weight, oxidation degree, etc.26,27 Many studies suggested that aging processes can increase the adsorption capacity of metals on microplastics because the processes can increase the surface area, hydrophilicity and carbonyl groups of microplastics.28,29 The aging of microplastics in natural environment is considered to be very slow.30 Thus, most studies related to aged microplastics carried out the research through artificial aging method, including for instance mechanical agitation, UV and thermal treatments, which are not able to accurately reflect the actual aging processes in natural environment.24,26,31 Therefore, exploration of the transformation of microplastics and their adsorption/release of metals mechanisms in natural aquatic environment is significant to help us to better assess the fate and behavior of microplastics in natural environment.
The aims of the present study are to better assess how the weathering of microplastics affects (i) the adsorption of a trace metal (i.e. Cu) and (ii) its subsequent release in gastric fluids and potential bioavailability for aquatic organisms. To understand these processes, series of experiments were carried out with polystyrene (PS) microplastics. PS was selected as the model plastic because it widely used in food and industrial packaging, heat preservation box, and building insulation.32–34 Moreover expanded polystyrene (EPS) is a plastic marine debris common in oceans around the world. EPS's unique “foam” structure, made up of thin layers, is more vulnerable to fragmentation than bulk plastics such as PE and PP.35 Therefore, PS tends to form small MPs, or even nanoplastics, when exposed to sunlight. The size of polystyrene MPs observed in water samples was often in the range associated with microplankton algae, between 20 μm and 200 μm, which makes them easy to be ingested by aquatic organisms.36 Copper (Cu) was selected as the target trace metal, because it is widely found in natural waters, as it originates from antifouling paint and tailings that are not disposed of properly.37–39 Cu is an essential micronutrient for living organisms only within a concentration range but it can be toxic above the concentration threshold.40,41 In vertebrates, Cu is present in many enzymes and glycoprotein. This trace metal is also necessary for hemoglobin synthesis and is important for nervous system function. Virgin PS microparticles were artificially aged by ozonation and incubation in a solar simulator. Ozone was used to stimulate atmospheric aging in tropospheric ozone and the solar simulator was used to stimulate the solar radiation on the earth. In addition, PS microparticles were naturally aged in a lake to assess natural weathering in an aquatic environment. The adsorption behavior and mechanisms of Cu on virgin and aged PS were compared to probe into the aging effects on the adsorption of metals by microplastics. The desorption and coingestion of PS particles with food in simulated gastric fluids (SGF) were also studied to investigate the release and bioavailability of adsorbed Cu in virgin and aged PS.
Materials and methods
Materials
Granulated PS was bought from Carl Roth Gmbh & Co. Micro-PS (PS-virgin) was obtained by grinding granulated PS by Retsch MM400 vibro-mill. Ten grams of granulated PS was pre cooled in liquid nitrogen for 10 min to prevent PS from being melted when grinding. The grinding process was set as 50 Hz, 2 min. Cu2+ stock solution (1000 mg L−1 in water with HNO3) was purchased from Sigma-Aldrich. Suwannee River natural organic matter (SRNOM, 2R101N) and Upper Mississippi River natural organic matter (UMRNOM, 1R110N) were purchased from the International Humic Substances Society. The SGF simulates the gastrointestinal fluid of fishes (Oncorhynchus mykiss), and the preparation of SGF was described in our previous study.42 The SGF composition is showed in Table S1.† The food used in coingestion experiments were freeze-dried Gammarus pulex (JBL®) obtained from a fish store, and Lemna minor which was collected from a pond and dried at 40 °C for 3 days. The food was grounded into powder using a porcelain mortar and pestle.
Artificial and in situ aging methods
Virgin PS was aged by 3 different methods: ozone (PS-O3), solar simulator (PS-SS) and natural process (lake) (PS-lake). For PS-O3, PS particles were introduced in a glass tube and an ozone generator (Innovatec, CMG 3–5, Germany) was used to create a continuous flow of ozone (3 g h−1, 5 h). For PS-SS, a solar simulator with Xe lamp (ATLAS AMETEK, Inc., NEX 1700, USA) was used to simulate the light aging caused by solar radiation of PS-virgin (irradiance wavelength: 300–800 nm, chamber temperature: 30 °C, irradiance power: 1600 W m−2, irradiance time: 60 d). Considering an annual average peak sun hours per day of 4, the aging induce by solar simulator corresponds to the equivalent of 1.6 year of sun exposure. For PS-lake, virgin PS was put in nylon mesh bags of 100 μm, the bags were sealed by needle work and food sealing clips. To remove PS-virgin particles <100 μm, the nylon mesh bags with PS-virgin were put in water with rotation overnight. After that, the bags were attached on a rope attached to a floating platform anchored at 570 m of the shore, and the depth of the bags were about 5 cm under the water (46°30′0.819′′ N and 6°39′39.007′′ E, LéXPLORE, Lake Geneva, Switzerland; https://lexplore.info). The PS particles inside the bag can float on the surface of the lake and were weathered by sunlight. The particles very incubated in the lake during 30 days.
Characterization methods
The morphology of virgin and aged PS particles was observed by scanning electron microscopy (ZEISS GeminiSEM 300, Germany), equipped with EDX detector (Oxford Inst.) for elements scanning. Contact angle values of ultrapure water were measured using a goniometer (EasyDrop, Krüss, Germany) to measure surface tension between virgin and aged PS particles with water. The functional groups of virgin/aged PS particles were analyzed by ATR-FTIR spectrometer (PerkinElmer Spectrum Two, USA). The size distribution of virgin and aged PS particles was measured using a particle size analyzer (Beckman Coulter LS 13 320, USA).
Batch adsorption and desorption experiments
To evaluate the impact of the pH on Cu adsorption, the pH value (3.0–5.0) of the Cu solution was adjusted by adding 1 mol L−1 NaOH, because Cu will precipitate above pH of 6 and Cu tend not to be adsorbed under strong acid conditions.43 The pH was adjusted to optimum value (pH 5.0) in other adsorption experiments. The virgin and aged PS particles (10 g L−1) were used for Cu (5 mg L−1; Cu solution volume: 100 mL) adsorption and stirred for 3 days in an orbital shaker (160 rpm, room temperature). In natural aquatic environments, the general pH condition varied from 6 to 8, and the general Cu concentration varied from the order of ng L−1 to μg L−1.44,45 In this study, the Cu concentration was set to 5 mg L−1 that is higher than that in natural aquatic environments to increase the Cu adsorption capacity of microplastics in a short time and better to observe the Cu adsorption and desorption behavior of PS particles.46,47 Cu2+ will precipitate above pH of 6.2 considering that the Ksp of Cu(OH)2 is 2.2 × 10−20, and Cu tend to not be adsorbed under strong acid conditions.48 Therefore, the pH conditions were set to 3–5 in this study.46,47 After adsorption, the PS particles were removed by PTFE filters (0.45 μm). The adsorption experiments over time were performed after adsorption for 0.5, 1, 5, 24, 48, 72, 96, 120 h. To evaluate the impacts of dissolved organic matter (DOM) on the Cu adsorption on virgin and aged PS, SRNOM and UMRNOM were dissolved in ultrapure water to prepare DOM stock solution (200 mg L−1) and the pH was adjusted to 7.0 using 1 mol L−1 NaOH. Then the DOM stock solution was diluted appropriately to 0, 10, 50, 100 mg L−1 for Cu adsorption (Cu: 5 mg L−1, pH = 5, 100 mL, 3 d). The concentrations of Cu were measured by ICP-OES (Shimadzu ICPE-9000, Japan). All experimental data was the average of measurements of triplicate samples and the results were expressed as mean ± standard deviation.
After adsorption of Cu, virgin and aged PS particles were collected by filtration using a Büchner funnel (cellulose filter, 0.45 μm). Then the particles were dried in an oven (30 °C) prior to desorption in simulated gastric fluid. The desorption of Cu from virgin and aged particles was performed in SGF for 1, 4.5, 20, 44, 68, 92, 116 h (PS: 10 g L−1, 100 mL). To assess different co-ingestion scenarios, different ratio of food (food
:
PS = 2
:
1, 5
:
1 and 10
:
1) and virgin or aged PS particles were added in the SGF (PS: 5 g L−1, 50 mL, 24 h). After digestion, the food and PS mixture was removed by filtration (PTFE filter, 0.45 μm), and the digestate sample was diluted 100 times before analysis by ICP-MS/MS (Agilent Technologies LC Infinity II ICP-MS Triple Quad 8900, USA).
Results and discussion
Characterization of virgin and aged PS particles
SEM was used to observe the morphology of virgin and aged PS samples, contact angle values of ultrapure water on virgin/aged PS was measured to compare their hydrophobicity and ATR-FTIR was used to analyze changes of functional groups.
Fig. S1† shows the SEM images of virgin and aged PS particles. From Fig. S1(a), (c), (e) and (g),† it can be seen that the virgin and aged PS particles have irregular shape and various size. Fig. S1(b), (d), (f), and (h)† show the details of the surface of the particles. For PS-O3, the surface is rough and likely due to the erosion caused by ozone. For PS-SS, cracks appeared at the surface, which were generated by the oxidation of ultraviolet. Similar results were observed by Huffer et al., and they explained that polymers such as the polystyrene are particularly susceptible to crack development due to their relatively open structure.26 For PS-lake, the surface was smoother than PS particles exposed to other weathering, which may be due to the wash of lake water.
Fig. S2(a)–(d)† shows the contact angle values of ultrapure water on virgin and aged PS particles. The water contact angles of PS-virgin, PS-O3, PS-SS and PS-lake were 103.7°, 0°, 0°, and 75.7°, respectively. Generally, the substance is hydrophilic if the water contact angle is 0°–90°, and the substance is hydrophobic if the water contact angle is >90°.49 These results suggest that the aging processes increased the hydrophilicity of virgin PS particles, and PS-O3 and PS-SS had higher hydrophilicity than PS-lake. Based on previous studies, it was speculated that hydrophilic contaminants will be adsorbed more tightly on hydrophilic microplastics than hydrophobic contaminants.50 It was reported that increase of hydrophilic groups in microplastics strengthen their interactions with hydrophilic contaminants in aqueous solutions. Shen et al. found that the introduction of surfactants resulted in a higher hydrophilicity of polyethylene (PE), polypropylene (PP) and polymethylmethacrylate (PMMA) particles, and increased the Pb2+ adsorption capacity of PE, PP and PMMA.20 Peng et al. found that the aging of PS and PE particles promoted the accumulation of hydrophilic antibiotics onto aged PS and PE in aquatic environments.24 Therefore, the aging process could increase the Cu adsorption capacity by PS particles, and the more hydrophilic the particles are, the higher Cu adsorption capacity the particles would have.
Fig. S2(e)† shows the color of virgin and aged PS particles. The color of PS-virgin, PS-O3, PS-SS, and PS-lake are white, light yellow, yellow, and grey, respectively. These results further confirm that PS-virgin apparently changed after the three aging processes. Fig. S2(f)† shows the position of virgin and aged PS particles in water. The PS-virgin particles were floating on the surface of water, the aged PS particles all sank to the bottom of the water, suggesting that the aged PS particles could have a higher affinity for hydrophilic contaminants in water. Fig. S3† shows the size distribution of virgin and aged PS, for PS-Solar simulator and PS-lake, and Table S2† shows the related D10, D50 and D90 that derived from volume distribution curve, the particles size did not change, but for PS-O3, the particles size tended to decrease, suggesting the particles could break down to smaller particles.
Fig. 1 shows the ATR-FTIR spectra of virgin and aged PS. The major peaks of PS-virgin emerged at 1600, 1492, 1451, 755, and 695 cm−1 due to the skeleton vibration and deformation of C–H in the aromatic ring and main chain. Compared to PS-virgin, there are apparent new peaks in the spectra of PS-O3. The peak at 1715.51 cm−1 represents the carbonyl, implying the C–H in PS-virgin was oxidized to carbonyl, which is easier to bind with metals.51,52 For PS-SS, there is also a new peak at 1715.94 cm−1 representing the carbonyl, although the carbonyl peak in PS-SS is weaker than that in PS-O3. However, for PS-lake, there is not apparent change compared to PS-virgin. These results suggest that the oxidation degree of three aged PS was PS-O3 > PS-SS > PS-lake, which suggest that the Cu adsorption capacity by different aged PS particles may follow a similar sequence.
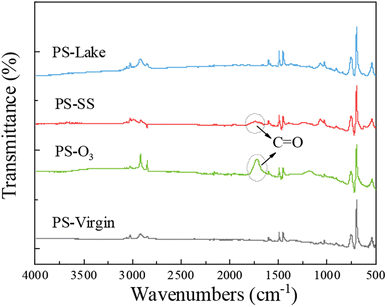 |
| Fig. 1 ATR-FTIR spectra of PS-virgin, PS-O3, PS-SS, PS-lake. | |
Adsorption of Cu onto virgin/aged PS
Three batches of adsorption experiments were conducted, to investigate the influence of pH, adsorption time and DOM on the Cu adsorption by microplastics. To evaluate the effect of different aging processes on the behavior between PS particles and metals, Cu adsorption experiments were conducted at different pH and adsorption time.
Fig. 2(a) shows the Cu adsorption capacity of virgin and aged PS at pH = 3–5. In natural aquatic environments, the general pH condition varied from 6 to 8, and the general Cu concentration varied from the order of ng L−1 to μg L−1.44,45 In this study, the Cu concentration was set to 5 mg L−1 that is higher than that in natural aquatic environments to increase the Cu adsorption capacity of microplastics in a short time. Cu2+ will precipitate above pH of 6.2 considering that the Ksp of Cu(OH)2 is 2.2 × 10−20, and Cu tend to not be adsorbed under strong acid conditions.48 Therefore, the pH conditions were set to 3–5 in this study. The PS-virgin had little adsorption capacity towards Cu. For aged PS, the Cu adsorption capacity increased as the pH increased from 3 to 5. The increase of Cu adsorption capacity with pH may be because Cu exists mainly in the form of positively charged Cu2+, Cu2+ tended to be adsorbed in higher pH condition. Because carboxylic groups were formed in aged PS, it was possible that carboxylic groups had more negative charges at higher pH.53 At pH 5, aged PS reached the highest Cu adsorption capacity, with largest Cu adsorption capacity for PS-lake (158 μg g−1), medium Cu adsorption for PS-SS (117 μg g−1), and lowest Cu adsorption capacity for PS-O3 (65 μg g−1). These results were surprising because PS-O3 had the most obvious oxidation degree and PS-lake seldom had oxidation. There was a significant decrease of Cu adsorption capacity for PS-lake when pH decreased (pH = 5: 158 μg g−1; pH = 4: 61 μg g−1; pH = 3: 30 μg g−1), and the decrease for PS-O3 and PS-SS were relatively lower, suggesting that the PS-lake was more sensitive to pH compared to PS-O3 and PS-SS.
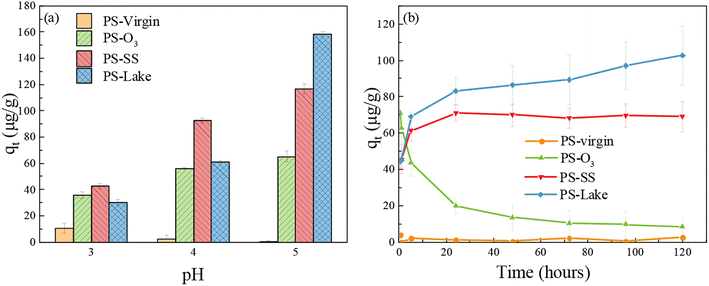 |
| Fig. 2 (a) Effect of initial pH on the Cu adsorption capacity of PS-virgin, PS-O3, PS-SS, PS-lake; (b) adsorption kinetics for the adsorption of Cu onto PS-virgin, PS-O3, PS-SS, PS-lake. | |
Fig. 2(b) shows the Cu adsorption capacity by virgin and aged PS from 0.5 h–120 h. PS-virgin had little Cu adsorption capacity. For PS-SS and PS-lake, the Cu adsorption capacity increased quickly in the first 24 h and then increasing slowly with the increase of adsorption time. The Cu adsorption capacity of PS-lake gradually increased after 24 h (103 μg g−1 at t = 120 h), and that of PS-SS reached adsorption equilibrium after 24 h (70 μg g−1). Unexpectedly, PS-O3 showed highest Cu adsorption capacity at 0.5 h (71 μg g−1), but the Cu adsorption capacity dropped dramatically with the increase of time (10 μg g−1, 120 h). The increase in PS-O3 adsorption capacity followed by a decrease is likely due to the formation of a highly oxidized and fragile layer on the surface of the particles. The DOC of the suspension containing PS-virgin was 0.05 mg L−1 whereas DOC concentration was 30 mg L−1 for PS-O3. This suggest that PS-O3 releases carboxylic acids once in contact with water. O3 is a very powerful oxidant which breaks the polymer chains and forms an oxidized layer on the surface of the PS particles which is easily eliminated in solution leaving a poorly oxidized surface with a low copper adsorption capacity. This hypothesis is also supported by the comparison of the pH before and after adsorption. For PS-virgin, PS-SS, and PS-lake, the pH did not change significantly. For PS-O3, the pH changed from 5 to 3 probably because of the carboxylic acids released in solution from PS-O3.
Fig. 3 shows the Cu adsorption capacity onto virgin and aged PS in Cu solution with different concentration of DOM. For PS-virgin, the Cu adsorption capacity increased a little bit with the addition of DOM. For PS-O3, although there was fluctuation, the Cu adsorption capacity decreased with the addition of DOM. For PS-lake, the Cu adsorption capacity shows a decreasing trend with the increase of DOM concentration. For PS-SS, although the Cu adsorption capacity slightly decreased in DOM of 10 and 50 mg L−1, it increased significantly in 100 mg L−1 DOM (SRN: 94 μg L−1, UP: 125 μg L−1) compared with Cu solution without DOM (75 μg L−1). The addition of DOM could occupy the adsorption sites on PS particles and compete with Cu, subsequently decrease the Cu adsorption capacity. However, according to previous studies, DOM also can adsorb on nano- or microplastics and adsorb metals due to its rich functional groups such as carboxyl, carbonyl, acetal, aromatic, and phenolic, which could increase the metals adsorption on plastic particles.8 In this study, DOM on PS-SS and PS-virgin played a role of adsorbent for Cu, but DOM on PS-lake compete with Cu for adsorption sites.
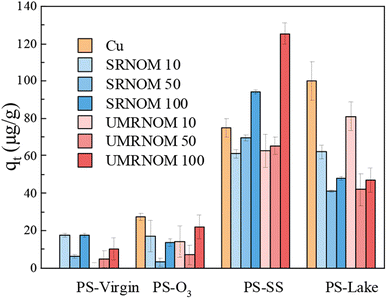 |
| Fig. 3 Adsorption capacity of Cu onto PS-virgin, PS-O3, PS-SS and PS-lake in Cu solution with DOM (concentration of DOM: 0, 10, 50, 100 mg L−1). | |
Desorption of Cu from virgin and aged PS
According to above results, PS particles showed apparent Cu adsorption capacity after aging process. The PS particles can be ingested and accumulated in the bodies of various aquatic and terrestrial organisms, and the adsorbed Cu could be released and enter the food chain. To investigate the release behavior and bioavailability of adsorbed Cu in PS particles, release kinetics and coingestion of PS particles with food SGF were investigated.
Fig. 4 shows the release of Cu in the SGF solution from virgin and aged PS at 1, 4.5, 20, 44, 68, 92, 116 h. For PS-virgin, there was no detectable Cu release in SGF, because little Cu was adsorbed on PS-virgin. The release processes were rapid for PS-lake and PS-O3 and they reached equilibrium within 1 h and 5 h, respectively. The release of Cu from PS-SS gradually increased, which showed a different trend compared to PS-O3 and PS-lake. Fig. S4† shows the Cu desorption ratio from aged PS in SGF. The adsorbed Cu in PS-O3 completely desorbed, while 72% and 58% of adsorbed Cu in PS-SS and PS-lake, respectively, were desorbed. These results suggests that aged microplastics can serve as vectors of metals such as copper from environment to organisms.
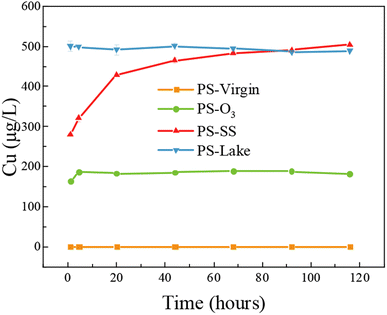 |
| Fig. 4 Release kinetics of Cu from PS-virgin, PS-O3, PS-SS and PS-lake in SGF. | |
Fig. 5 shows the release of Cu in coingestion of virgin and aged PS with either vegetal (Lemna minor) animal or (Gammarus pulex) food in SGF, and the mass ratio of food and PS particles were 2, 5, 10, respectively. For PS-virgin, there was no obvious change of Cu solubilization after the addition of PS-virgin compared to food only, because the Cu adsorption on PS-virgin was minimal. For PS-O3, coingestion with vegetal food led to a small increase of Cu solubilization but not increase with animal food. The Cu solubilization gap between animal food only and PSO3 with animal food was tiny, because the release of Cu from PS-O3 (70 μg L−1) was much lower than the Cu released from animal food. For PS-SS and PS-lake, there was a significant increase of Cu solubilization after addition of PS particles compared to animal or vegetal food only. Table S4† shows the change of dissolved Cu in SGF with the existence of PS particles compared with food only. The Cu originated from PS-SS and PS-lake increased about 9.17–204.63% compared with food only, and the increase was more significant for low food quantity and vegetal food. These results suggest that the PS-SS and PS-lake lead to greater bioavailability of Cu. The release of Cu in coingestion scenario was a little lower compared to the sum of PS particles and food for PS-SS and PS-lake. These results indicate that Cu solubilized from PS-SS and PS-lake was partly readsorbed on vegetal and animal food. This is likely because the polysaccharides and proteins in Lemna minor and Gammarus pulex contain rich functional groups such as carboxyl, amino, hydroxyl and phosphoryl that can bind with metals.54,55 Overall, the addition of PS can lead to a higher bioavailability of Cu although the animal food can act as a complexant for Cu to some extent.
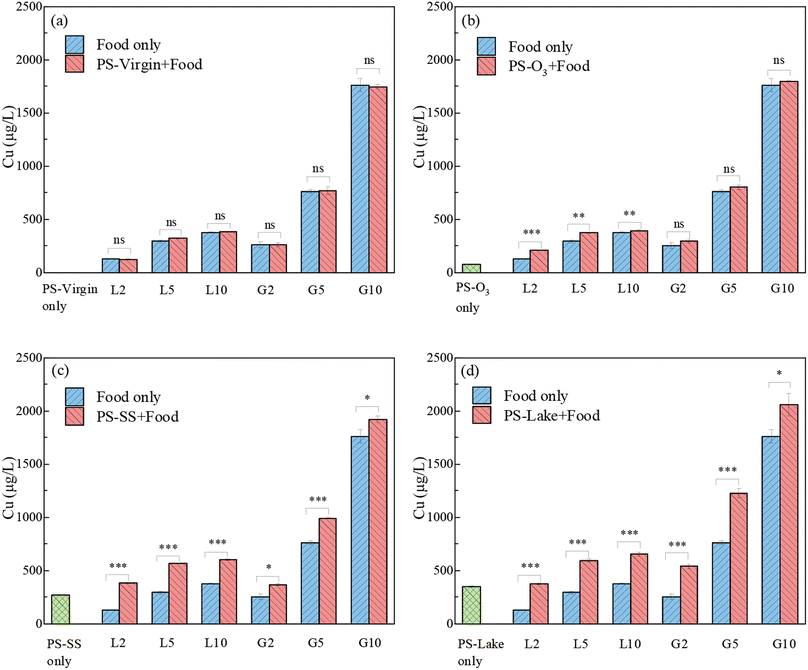 |
| Fig. 5 Release of Cu from virgin/aged PS and food in SGF. (a) PS-virgin, (b) PS-O3, (c) PS-SS and (d) PS-lake (L2, L5 and L10 represent the ratio of Lemna minor food to PS are 2 : 1, 5 : 1 and 10 : 1; G2, G5 and G10 represent the ratio of Gammarus pulex food to PS are 2 : 1, 5 : 1 and 10 : 1). Statistical analyses with Welch two-samples tests: *, **, and *** represent p value < 0.05, 0.01, and 0.001, respectively. | |
Cu adsorption and desorption mechanisms of virgin/aged PS
Generally, the adsorption capacity of metals by PS follows a positive correlation with the aging extent of PS, because the aged PS is more hydrophilic and contains more functional groups such as carboxylic groups that can combine with metals. In this study, the aging extent sequence is PS-O3 > PS-SS > PS-lake > PS-virgin according to their hydrophilicity and carbonyl content. However, for aged PS, the Cu adsorption capacity sequence is PS-lake > PS-SS > PS-O3. For PS-SS, UV-light was an important aging factor. In addition, UV-light can react with O2 and generate a bit of ozone.56 The adsorption result is comprehensible for PS-SS, because the aging of PS particles under solar simulator were apparent but weaker than those in ozone. But for PS-O3 and PS-lake, the adsorption results are surprising. To investigate the adsorption mechanisms of PS-O3 and PS-lake, further studies were carried out.
Considering PS-O3 was obtained in an extreme oxidation condition, the particles could break up and decrease the Cu adsorption capacity subsequently.57 Fig. S5† shows the total organic carbon (TOC) released from microplastics of the adsorption system after filtration. The PS-virgin (0.05 mg L−1) and PS-lake (0.31 mg L−1) released little TOC, compared to PS-SS (3 mg L−1) and especially PS-O3 (30 mg L−1). In Fig. 2(b), Cu was quickly adsorbed by PS-O3 in 0.5 h and reached the highest adsorption capacity (71 μg g−1), but then Cu released dramatically with the increase of adsorption time. These results suggest that the PS-O3 has obvious Cu adsorption capacity due to the carbonyl or more carboxylic groups, but the entire particle would be broken in solution and the adsorbed Cu will fall off from the PS-O3 particles subsequently. These results also can explain the reason that the adsorbed Cu in PS-O3 released completely in SGF. It has been reported that the C–C bonds in the main chains of the polymers can be cleaved and lead to the fragmentation of the particles under exposure to ozone.57,58 The research on PS-O3 helps us understand the fate of microplastics under extreme oxidizing conditions.
In most previous studies, the ultraviolet light, erosion and flush of water are considered the main causes for the aging of microplastics, which lead to the increase of the specific surface area and functional groups of microplastics.59–61 Consequently, the adsorption capacity of metals by aged microplastics increased obviously compared to virgin microplastics. In this study, the aging duration for PS-lake was only one month, which was not long enough to cause the apparent change of functional groups in PS. However, it was surprising that PS-lake had highest Cu adsorption capacity compared to other aged PS. Given that there was no obvious carbonyl peak in ATR-FTIR results of PS-lake, some other factors came into play. Fig. 6 shows the elements distribution of PS-lake after adsorption of Cu. As we can see, the distribution of Cu was obviously overlapped with that of Si, Al, Mg, Fe and O, which implied that the compounds that contained oxide of Si, Al, Mg, Fe are associated to the adsorption of Cu in PS-lake. In natural environment, after microplastics enter in water bodies, some abundant elements such as Si, Al, Mg, Fe can gradually precipitate as quartz (SiO2) and mica KAl2(AlSi3O10)(OH)2 (including small quantity of Mg, Fe and other metals), on the surface of microplastics.62,63 These metallic oxides can subsequently act as adsorbents and accumulate more metals.64–67 The adsorbed Cu released rapidly and completely in SGF because the metallic oxides on PS-lake dissolved rapidly in SGF. These results suggest that the metals adsorption behavior of aged microplastics is complex in natural waters, besides the aging effects caused by ultraviolet light, water erosion, the precipitation of metallic oxides can also lead to the accumulation of more metals.
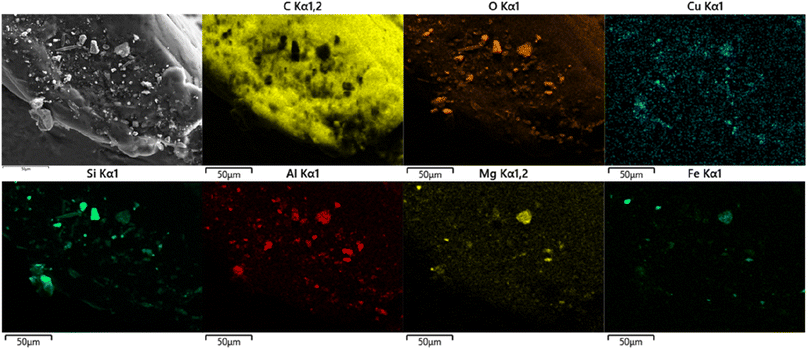 |
| Fig. 6 SEM images of PS-lake and its corresponding EDX mappings for C, O, Cu, Si, Al, Mg and Fe. | |
Environmental implication
In this study, virgin microplastics were artificially aged by ozone, solar simulator and were naturally aged in a lake to investigate the fate of microplastics and their interactions with metals. The aged microplastics can combine with Cu, and lead to greater bioavailability of Cu. In natural aquatic environments, the general Cu concentration varies from the order of ng L−1 to μg L−1, which is lower than the Cu concentration in this study, thus the accumulation of Cu in microplastics may be less.44 However, the exposure time of microplastics in natural environments is much longer than in this study, which means the aging duration and the accumulation time of Cu is much longer. Moreover, the aging of microplastics will be affected by multiple factors in natural environments due to the long exposure to solar irradiation, mechanical abrasion, mineral sedimentation, etc. and these results could be extrapolated to other metals (e.g. Zn, Cd, Pb, Ni) that are positive ions.68 It can therefore be concluded that microplastics can act as vectors of metals from environment to organisms and potentially impact their metabolism. An increase in the intake of certain metallic micronutrients such as Cu, Ni and Zn can have deleterious effects on the metabolism of certain organisms.69 In addition, increased exposure of aquatic organisms to metals such as Cd and Pb can have devastating consequences for ecosystems.70
In future studies, it is meaningful to investigate a detailed mechanism of copper ion adsorption in natural water environments. The experiments demonstrate that the absorption capacity of plastic particles after ozone treatment peaks at 0.5 hours and then dramatically decreases due to ozone-induced aging of the plastic, leading to particle fragmentation. However, it needs further investigation whether the copper on the smaller plastic particles re-dissolved back into the solution or continued to adhere to the plastic particles.
Conflicts of interest
The authors have no conflict of interest to declare.
Acknowledgements
The authors would like to thank Karine Vernez, Sylvain Coudret, Yann Lavanchy, and Lucie Navratilova for their assistance in sample analyses. The authors would also like to thank Pierre Rossi, Caroline Gachet, Eya Damergi and Tobias Borgmeyer for handling the microplastics, as well as Guillaume Cunillera and Sébastien Lavanchy for installing microplastics in Platform LéXPLORE, Lake Geneva – Switzerland. The authors would also like to thank China Scholarship Council and EPFL for the financial support.
References
- X. Z. Lim, Microplastics are everywhere - but are they harmful?, Nature, 2021, 593(7857), 22–25 CrossRef CAS PubMed.
- L. Su, S. M. Sharp, V. J. Pettigrove, N. J. Craig, B. Nan, F. Du and H. Shi, Superimposed microplastic pollution in a coastal metropolis, Water Res., 2020, 168, 115140 CrossRef CAS PubMed.
- W. Yuan, J. A. Christie-Oleza, E. G. Xu, J. Li, H. Zhang, W. Wang, L. Lin, W. Zhang and Y. Yang, Environmental fate of microplastics in the world's third-largest river: Basin-wide investigation and microplastic community analysis, Water Res., 2022, 210, 118002 CrossRef CAS PubMed.
- G. Bhagwat, T. K. A. Tran, D. Lamb, K. Senathirajah, I. Grainge, W. O'Connor, A. Juhasz and T. Palanisami, Biofilms Enhance the Adsorption of Toxic Contaminants on Plastic Microfibers under Environmentally Relevant Conditions, Environ. Sci. Technol., 2021, 55(13), 8877–8887 CrossRef CAS PubMed.
- W. Cao, J. Gong, M. Qin, L. Qin, S. Fang, J. Li, S. Tang, Z. Tan, X. Li and Z. Chen, Biodegradable Microplastics Affect the Wheatgrass Traits, Fe Plaque Development Involved in Sb Accumulation, and Microbial Community Functions in Antimony-Contaminated Riparian Wetlands, ACS Sustain. Chem. Eng., 2022, 10(18), 5847–5858 CrossRef.
- M. B. Ahmed, M. S. Rahman, J. Alom, M. S. Hasan, M. A. H. Johir, M. I. H. Mondal, D. Y. Lee, J. Park, J. L. Zhou and M. H. Yoon, Microplastic particles in the aquatic environment: A systematic review, Sci. Total Environ., 2021, 775, 145793 CrossRef CAS PubMed.
- L. Li, Y. Luo, R. Li, Q. Zhou, W. J. G. M. Peijnenburg, N. Yin, J. Yang, C. Tu and Y. Zhang, Effective uptake of submicrometre plastics by crop plants via a crack-entry mode, Nat Sustainability, 2020, 3(11), 929–937 CrossRef.
- F. Abdolahpur Monikh, M. G. Vijver, Z. Guo, P. Zhang, G. K. Darbha and W. Peijnenburg, Metal sorption onto nanoscale plastic debris and trojan horse effects in Daphnia magna: Role of dissolved organic matter, Water Res., 2020, 186, 116410 CrossRef CAS PubMed.
- M. P. Johansen, T. Cresswell, J. Davis, D. L. Howard, N. R. Howell and E. Prentice, Biofilm-enhanced adsorption of strong and weak cations onto different microplastic sample types: Use of spectroscopy, microscopy and radiotracer methods, Water Res., 2019, 158, 392–400 CrossRef CAS PubMed.
- J. Wang, X. Guo and J. Xue, Biofilm-Developed Microplastics As Vectors of Pollutants in Aquatic Environments, Environ. Sci. Technol., 2021, 55(19), 12780–12790 CAS.
- Y. L. Liao and J. Y. Yang, Microplastic serves as a potential vector for Cr in an in-vitro human digestive model, Sci. Total Environ., 2020, 703, 134805 CrossRef CAS PubMed.
- M. Filella and A. Turner, Observational Study Unveils the Extensive Presence of Hazardous Elements in Beached Plastics from Lake Geneva, Front. Environ. Sci., 2018, 6, 1 CrossRef.
- M. Mohsen, Q. Wang, L. Zhang, L. Sun, C. Lin and H. Yang, Heavy metals in sediment, microplastic and sea cucumber Apostichopus japonicus from farms in China, Mar. Pollut. Bull., 2019, 143, 42–49 CrossRef CAS PubMed.
- L. A. Holmes, A. Turner and R. C. Thompson, Adsorption of trace metals to plastic resin pellets in the marine environment, Environ. Pollut., 2012, 160(1), 42–48 CrossRef CAS PubMed.
- A. Turner, Heavy metals, metalloids and other hazardous elements in marine plastic litter, Mar. Pollut. Bull., 2016, 111(1–2), 136–142 CrossRef CAS.
- J. Ma, H. Zhou, S. Yan and W. Song, Kinetics studies and mechanistic considerations
on the reactions of superoxide radical ions with dissolved organic matter, Water Res., 2019, 149, 56–64 CrossRef CAS PubMed.
- Z. Guo, D. Kodikara, L. S. Albi, Y. Hatano, G. Chen, C. Yoshimura and J. Wang, Photodegradation of Organic Micropollutants in Aquatic Environment: Importance, Factors and Processes, Water Res., 2022, 118236 Search PubMed.
- Z.-L. Ye, J. Zhang, J. Cai and S. Chen, Investigation of tetracyclines transport in the presence of dissolved organic matters during struvite recovery from swine wastewater, Chem. Eng. J., 2020, 385, 123950 CrossRef CAS.
- Y. Zhou, Y. Yang, G. Liu, G. He and W. Liu, Adsorption mechanism of cadmium on microplastics and their desorption behavior in sediment and gut environments: The roles of water pH, lead ions, natural organic matter and phenanthrene, Water Res., 2020, 184, 116209 CrossRef CAS.
- M. Shen, B. Song, G. Zeng, Y. Zhang, F. Teng and C. Zhou, Surfactant changes lead adsorption behaviors and mechanisms on microplastics, Chem. Eng. J., 2021, 405, 126989 CrossRef CAS.
- B. Munier, L. I. Bendell and M. Amitava, Macro and micro plastics sorb and desorb metals and act as a point source of trace metals to coastal ecosystems, PLoS One, 2018, 13(2), e0191759 CrossRef CAS PubMed.
- O. H. Fred-Ahmadu, G. Bhagwat, I. Oluyoye, N. U. Benson, O. O. Ayejuyo and T. Palanisami, Interaction of chemical contaminants with microplastics: Principles and perspectives, Sci. Total Environ., 2020, 706, 135978 CrossRef CAS PubMed.
-
C. Catrouillet, C. L. C. D. Veslud and J. Gigault, In Metals and microplastics, a multi-contamination in the environment: which planetary distribution?, Goldschmidt Conference 2021 Search PubMed.
- P. Liu, L. Qian, H. Wang, X. Zhan, K. Lu, C. Gu and S. Gao, New Insights into the Aging Behavior of Microplastics Accelerated by Advanced Oxidation Processes, Environ. Sci. Technol., 2019, 53(7), 3579–3588 CrossRef CAS.
- K. Zhu, H. Jia, Y. Sun, Y. Dai, C. Zhang, X. Guo, T. Wang and L. Zhu, Long-term phototransformation of microplastics under simulated sunlight irradiation in aquatic environments: Roles of reactive oxygen species, Water Res., 2020, 173, 115564 CrossRef CAS PubMed.
- T. Huffer, A. K. Weniger and T. Hofmann, Sorption of organic compounds by aged polystyrene microplastic particles, Environ. Pollut., 2018, 236, 218–225 CrossRef PubMed.
- X. Liu, Q. Deng, Y. Zheng, D. Wang and B. J. Ni, Microplastics aging in wastewater treatment plants: Focusing on physicochemical characteristics changes and corresponding environmental risks, Water Res., 2022, 221, 118780 CrossRef CAS PubMed.
- Y. Wang, X. Wang, Y. Li, J. Li, Y. Liu, S. Xia and J. Zhao, Effects of exposure of polyethylene microplastics to air, water and soil on their adsorption behaviors for copper and tetracycline, Chem. Eng. J., 2021, 404, 126412 CrossRef CAS.
- C. C. Chen, X. Zhu, H. Xu, F. Chen, J. Ma and K. Pan, Copper Adsorption to Microplastics and Natural Particles in Seawater: A Comparison of Kinetics, Isotherms, and Bioavailability, Environ. Sci. Technol., 2021, 55(20), 13923–13931 CrossRef CAS PubMed.
- S. Chen, Y. Yang, X. Jing, L. Zhang, J. Chen, C. Rensing, T. Luan and S. Zhou, Enhanced aging of polystyrene microplastics in sediments under alternating anoxic-oxic conditions, Water Res., 2021, 207, 117782 CrossRef CAS PubMed.
- A.-U.-R. Bacha, I. Nabi and L. Zhang, Mechanisms and the Engineering Approaches for the Degradation of Microplastics, ACS ES&T Engg, 2021, 1(11), 1481–1501 Search PubMed.
- X. D. Sun, X. Z. Yuan, Y. Jia, L. J. Feng, F. P. Zhu, S. S. Dong, J. Liu, X. Kong, H. Tian, J. L. Duan, Z. Ding, S. G. Wang and B. Xing, Differentially charged nanoplastics demonstrate distinct accumulation in Arabidopsis thaliana, Nat. Nanotechnol., 2020, 15(9), 755–760 CrossRef CAS PubMed.
- C. Della Torre, E. Bergami, A. Salvati, C. Faleri, P. Cirino, K. A. Dawson and I. Corsi, Accumulation and embryotoxicity of polystyrene nanoparticles at early stage of development of sea urchin embryos Paracentrotus lividus, Environ. Sci. Technol., 2014, 48(20), 12302–12311 CrossRef CAS PubMed.
- M. Jang, W. J. Shim, Y. Cho, G. M. Han, Y. K. Song and S. H. Hong, A close relationship between microplastic contamination and coastal area use pattern, Water Res., 2020, 171, 115400 CrossRef CAS.
- Y. K. Song, S. H. Hong, S. Eo, G. M. Han and W. J. Shim, Rapid Production of Micro- and Nanoplastics by Fragmentation of Expanded Polystyrene Exposed to Sunlight, Environ. Sci. Technol., 2020, 54(18), 11191–11200 CrossRef CAS.
- S. Badylak, E. Phlips, C. Batich, M. Jackson and A. Wachnicka, Polystyrene microplastic contamination versus microplankton abundances in two lagoons of the Florida Keys, Sci. Rep., 2021, 11(1), 6029 CrossRef CAS PubMed.
- C. Muller-Karanassos, A. Turner, W. Arundel, T. Vance, P. K. Lindeque and M. Cole, Antifouling paint particles in intertidal estuarine sediments from southwest England and their ingestion by the harbour ragworm, Hediste diversicolor, Environ. Pollut., 2019, 249, 163–170 CrossRef CAS PubMed.
- L. Bradney, H. Wijesekara, K. N. Palansooriya, N. Obadamudalige, N. S. Bolan, Y. S. Ok, J. Rinklebe, K. H. Kim and M. B. Kirkham, Particulate plastics as a vector for toxic trace-element uptake by aquatic and terrestrial organisms and human health risk, Environ. Int., 2019, 131, 104937 CrossRef CAS PubMed.
- M. T. Schaanning, H. C. Trannum, S. Oxnevad and K. Ndungu, Benthic community status and mobilization of Ni, Cu and Co at abandoned sea deposits for mine tailings in SW Norway, Mar. Pollut. Bull., 2019, 141, 318–331 CrossRef CAS.
- T. Punshon and B. P. Jackson, Essential micronutrient and toxic trace element concentrations in gluten containing and gluten-free foods, Food Chem., 2018, 252, 258–264 CrossRef CAS PubMed.
- H. Jeong, J. S. Ryu and K. Ra, Characteristics of potentially toxic elements and multi-isotope signatures (Cu, Zn, Pb) in non-exhaust traffic emission sources, Environ. Pollut., 2022, 292(Pt), 118339 CrossRef CAS PubMed.
- T. Masset, B. J. D. Ferrari, D. Oldham, W. Dudefoi, M. Minghetti, K. Schirmer, A. Bergmann, E. Vermeirssen and F. Breider, Vitro Digestion of Tire Particles in a Fish Model (Oncorhynchus mykiss): Solubilization Kinetics of Heavy Metals and Effects of Food Coingestion, Environ. Sci. Technol., 2021, 55(23), 15788–15796 CrossRef CAS PubMed.
- K. Y. Chen and W. Y. Zeng, Adsorption of Cu(II) by Poly-gamma-glutamate/Apatite Nanoparticles, Polymers, 2021, 13, 962 CrossRef CAS PubMed.
- G. Xing, S. Garg, C. J. Miller, A. N. Pham and T. D. Waite, Effect of Chloride and Suwannee River Fulvic Acid on Cu Speciation: Implications to Cu Redox Transformations in Simulated Natural Waters, Environ. Sci. Technol., 2020, 54(4), 2334–2343 CrossRef CAS PubMed.
- P. L. Schoon, A. de Kluijver, J. J. Middelburg, J. A. Downing, J. S. Sinninghe Damsté and S. Schouten, Influence of lake water pH and alkalinity on the distribution of core and intact polar branched glycerol dialkyl glycerol tetraethers (GDGTs) in lakes, Org. Geochem., 2013, 60, 72–82 CrossRef CAS.
- A. I. S. Purwiyanto, Y. Suteja, Trisno, P. S. Ningrum, W. A. E. Putri, Rozirwan, F. Agustriani, Fauziyah, M. R. Cordova and A. F. Koropitan, Concentration and adsorption of Pb and Cu in microplastics: Case study in aquatic environment, Mar. Pollut. Bull., 2020, 158, 111380 CrossRef CAS PubMed.
- P. P. Leal, C. L. Hurd, S. G. Sander, E. Armstrong, P. A. Fernández, T. J. Suhrhoff and M. Y. Roleda, Copper pollution exacerbates the effects of ocean acidification and warming on kelp microscopic early life stages, Sci. Rep., 2018, 8(1), 14763 CrossRef PubMed.
- A. Tegenaw, G. A. Sorial, E. Sahle-Demessie and C. Han, Influence of water chemistry on colloid-size Cu-based pesticides particles: A case of Cu(OH)(2) commercial fungicide/bactericide, Chemosphere, 2020, 239, 124699 CrossRef CAS PubMed.
- M. Wu, G. Shi, W. Liu, Y. Long, P. Mu and J. Li, A Universal Strategy for the Preparation of Dual Superlyophobic Surfaces in Oil-Water Systems, ACS Appl. Mater. Interfaces, 2021, 13(12), 14759–14767 CrossRef CAS PubMed.
- J. He, X. Fu, F. Ni, G. Yang, S. Deng, J. P. Chen and F. Shen, Quantitative assessment of interactions of hydrophilic organic contaminants with microplastics in natural water environment, Water Res., 2022, 119024 CrossRef CAS PubMed.
- P. Henke, H. Kozak, A. Artemenko, P. Kubat, J. Forstova and J. Mosinger, Superhydrophilic polystyrene nanofiber materials generating O2((1)Delta(g)): postprocessing surface modifications toward efficient antibacterial effect, ACS Appl. Mater. Interfaces, 2014, 6(15), 13007–13014 CrossRef CAS PubMed.
- G. Bhagwat, M. Carbery, T. K. Anh Tran, I. Grainge, W. O'Connor and T. Palanisami, Fingerprinting Plastic-Associated Inorganic and Organic Matter on Plastic Aged in the Marine Environment for a Decade, Environ. Sci. Technol., 2021, 55(11), 7407–7417 CrossRef CAS PubMed.
- F. Blancho, M. Davranche, R. Marsac, A. Léon, A. Dia, B. Grassl, S. Reynaud and J. Gigault, Metal-binding processes on nanoplastics: rare earth elements as probes, Environ. Sci.: Nano, 2022, 9(6), 2094–2103 RSC.
- R. Mikutta, A. Baumgärtner, A. Schippers, L. Haumaier and G. Guggenberger, Extracellular Polymeric Substances from Bacillus subtilis Associated with Minerals Modify the Extent and Rate of Heavy Metal Sorption, Environ. Sci. Technol., 2012, 46(7), 3866–3873 CrossRef CAS PubMed.
- A. B. Saralegui, V. Willson, N. Caracciolo, M. N. Piol and S. P. Boeykens, Macrophyte biomass productivity for heavy metal adsorption, J. Environ. Manage., 2021, 289, 112398 CrossRef CAS PubMed.
- H. Claus, Ozone Generation by Ultraviolet Lamps, Photochem. Photobiol., 2021, 97(3), 471–476 CrossRef CAS PubMed.
- Y. Li, J. Li, J. Ding, Z. Song, B. Yang, C. Zhang and B. Guan, Degradation of nano-sized polystyrene plastics by ozonation or chlorination in drinking water disinfection processes, Chem. Eng. J., 2022, 427, 131690 CrossRef CAS.
- S. Kim, A. Sin, H. Nam, Y. Park, H. Lee and C. Han, Advanced oxidation processes for microplastics degradation: A recent trend, Chem. Eng. J. Adv., 2022, 9, 100213 CrossRef CAS.
- Y. K. Lee, S. Hong and J. Hur, Copper-binding properties of microplastic-derived dissolved organic matter revealed by fluorescence spectroscopy and two-dimensional correlation spectroscopy, Water Res., 2021, 190, 116775 CrossRef CAS PubMed.
- X. Wu, P. Liu, Z. Gong, H. Wang, H. Huang, Y. Shi, X. Zhao and S. Gao, Humic Acid and Fulvic Acid Hinder Long-Term Weathering of Microplastics in Lake Water, Environ. Sci. Technol., 2021, 55(23), 15810–15820 CrossRef CAS PubMed.
- J. Marsic-Lucic, J. Lusic, P. Tutman, D. Bojanic Varezic, J. Siljic and J. Pribudic, Levels of trace metals on microplastic particles in beach sediments of the island of Vis, Adriatic Sea, Croatia, Mar. Pollut. Bull., 2018, 137, 231–236 CrossRef CAS PubMed.
- L. W. Hao, H. F. Tao, R. L. Guo, W. W. Mou, B. Tian and X. F. Ma, Hydrodynamic evolution from quartz microtextures of a beach ridge in Qinghai Lake, China, Sediment. Geol., 2019, 389, 13–25 CrossRef.
- S. A. C. Furquim, L. Barbiero, R. C. Graham, J. Neto, R. P. D. Ferreira and S. Furian, Neoformation of micas in soils surrounding an alkaline-saline lake of Pantanal wetland, Brazil, Geoderma, 2010, 158(3–4), 331–342 CrossRef CAS.
- I. Khan, S. A. Awan, M. Rizwan, S. Ali, M. J. Hassan, M. Brestic, X. Zhang and L. Huang, Effects of silicon on heavy metal uptake at the soil-plant interphase: A review, Ecotoxicol. Environ. Saf., 2021, 222, 112510 CrossRef CAS PubMed.
- M. Hong, L. Yu, Y. Wang, J. Zhang, Z. Chen, L. Dong, Q. Zan and R. Li, Heavy metal adsorption with zeolites: The role of hierarchical pore architecture, Chem. Eng. J., 2019, 359, 363–372 CrossRef CAS.
- F. Aouaini, M. Ben Yahia, H. I. Alrebdi and M. A. Alothman, Interpretation of the adsorption of metals on quartz crystal based-macromolecule via advanced modeling of equilibrium isotherms, Sci. Rep., 2021, 11(1), 20031 CrossRef CAS PubMed.
- M. K. Uddin, A review on the adsorption of heavy metals by clay minerals, with special focus on the past decade, Chem. Eng. J., 2017, 308, 438–462 CrossRef CAS.
- Y. Li, Y. Feng, H. Li, Y. Yao, C. Xu, J. Ju, R. Ma, H. Wang and S. Jiang, Adsorption of metal ions by oceanic manganese nodule and deep-sea sediment: Behaviour, mechanism and evaluation, Sci. Total Environ., 2024, 908, 168163 CrossRef CAS PubMed.
- A. Pal, S. Bhattacharjee, J. Saha, M. Sarkar and P. Mandal, Bacterial survival strategies and responses under heavy metal stress: a comprehensive overview, Crit. Rev. Microbiol., 2022, 48(3), 327–355 CrossRef CAS PubMed.
- N. B. Saidon, R. Szabo, P. Budai and J. Lehel, Trophic transfer and biomagnification potential of environmental contaminants (heavy metals) in aquatic ecosystems, Environ. Pollut., 2024, 340, 122815 CrossRef CAS PubMed.
Footnote |
† Electronic supplementary information (ESI) available: Physical characterization of virgin/aged PS, pseudo-second-order kinetic model for the adsorption of Cu on PS-SS and PS-lake, Cu desorption ratio, TOC released from virgin/aged PS, composition of SGF, change of dissolved Cu in SGF with the existence of PS particles compared with food only. See DOI: https://doi.org/10.1039/d3em00354j |
|
This journal is © The Royal Society of Chemistry 2024 |
Click here to see how this site uses Cookies. View our privacy policy here.