DOI:
10.1039/D3AN00822C
(Critical Review)
Analyst, 2023,
148, 3432-3451
Analytical chemistry of carbonyl compounds in indoor air
Received
23rd May 2023
, Accepted 3rd July 2023
First published on 4th July 2023
Abstract
Carbonyl compounds are ubiquitous in outdoor and indoor air. Due to the high electronegativity of the oxygen atom, they are polar in nature and the C
O group opens possibilities for many types of chemical reactions. Their physical and chemical properties are additionally influenced by substituents and conjugated double bonds. The concentration ranges are also highly variable. Formaldehyde can reach 100 ppb or more in indoor air, but reaction products such as 4-oxopentanal (4-OPA) are in the lower ppb range or even below 1 ppb. Another point concerns the dynamics of carbonyls. When examining the emission of formaldehyde in test chambers, an equilibrium concentration is usually established, so that changes over time can be neglected during the measurement. On the other hand, many substances and scenarios are subject to strong fluctuations in concentration over short time periods. The analysis is also made more difficult by the fact that different methods are often required for saturated carbonyls, unsaturated carbonyls and dicarbonyls. This work focuses on aprotic carbonyl compounds such as aldehydes, ketones, lactams and pyrones with relevance for the indoor environment that do not contain any other reactive groups. The range of interesting compounds has grown significantly in recent years, notably through the derivation of health-based guide values, as well as through investigations into new products, human activities and human emissions from the skin and respiratory gas. Classical and modern analysis methods are discussed, which can be considered for the respective research question. Many small molecules require derivatization as a first step, followed by separation via gas chromatography or HPLC. Substance-specific detection without chromatographic separation is routinely used for formaldehyde. With some limitations, the identification of carbonyls in multicomponent mixtures is possible using online mass spectrometry. In particular, proton-transfer-reaction mass spectrometry (PTR-MS) has established as a method with high sensitivity and high time resolution.
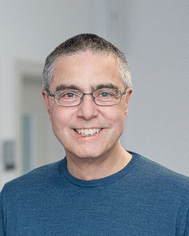 Tunga Salthammer | Tunga Salthammer holds a diploma in Chemistry, received a Dr rer. nat. in Physical Chemistry from the Technical University of Braunschweig and was appointed as a Professor in 2012. He joined the Fraunhofer WKI in 1990 and is the deputy director of the institute. Since 2007, he has been an Adjunct Professor at the Queensland University of Technology in Brisbane. He has been a Visiting Professor at the Technical University of Denmark and at Tsinghua University in Beijing. His research interests include VOC/SVOC emission studies, indoor chemistry, airborne particles, and settled dust. |
1 Introduction
To date, thousands of organic compounds have been analytically detected in indoor air. A systematic compilation is just as impossible as it is useless, since the spectrum of substances changes over time for a variety of reasons such as substance substitution, legislation and lifestyle. In addition, many substances are not directly introduced into the room air, but are formed by biotic and abiotic chemical processes.
In order to still get an overview, classical environmental chemistry distinguishes between the concepts media-related, sector-related, substance-related1 and effect-related.2 A media-related concept is used when specific matrices such as air or dust are to be examined. This is the case with surveys. Sectors relate to defined product groups (e.g. building materials, furniture) and processes (e.g. cooking, combustion, ozone reactions), while effect-related concepts focus on exposure and health aspects. Substance-related concepts can relate to individual chemicals such as formaldehyde and radon, but also to substance groups such as polychlorinated biphenyls, polycyclic aromatic hydrocarbons and phthalates.
This review is substance-related and deals with carbonyl compounds that are of importance for the indoor environment. Only aprotic substances that do not have a functional group from which hydrogen atoms can be split off as protons are discussed. Esters are also not taken into account, but only aldehydes, ketones, lactams and pyrones with the chemical structures shown in Fig. 1.
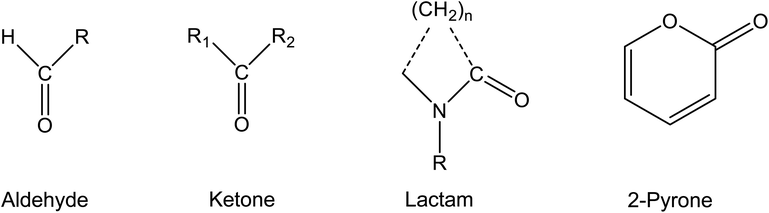 |
| Fig. 1 Chemical structures of carbonyl compounds. | |
The question of whether such work is necessary arises from history and the current situation. In the early years, formaldehyde was almost exclusively discussed.3 A summary of historical methods for determining formaldehyde in different phases can be found in Walker.4 With the beginning of systematic room air measurements, attention was drawn to solvents such as 2-butanone (MEK) and other volatile organic compounds (VOCs).5–7 The saturated and unsaturated aldehydes came into focus with investigations into oxidation reactions of unsaturated hydrocarbons and fatty acids through thermal influences, atmospheric oxygen and ozone.8 Over time, more relevant carbonyl compounds were identified, in particular through studies on wood and wood-based materials,9,10 textile floor coverings,11,12 fragrances,13 3D printers,14,15 cooking and frying,16 but also through reactions on the human skin surface.17
From an analytical point of view, the problem is that carbonyl compounds are chemically very different. Formaldehyde, the simplest aldehyde, is a typical VVOC (very volatile organic compound) with a boiling point of −20 °C; benzophenone is an SVOC (semi-volatile organic compound) with a boiling point of +305 °C. The concentrations can also cover several orders of magnitude. Some substances like 4-oxopentanal (4-OPA) and 6-methyl-5-hepten-2-one (6-MHO) are common products in the reaction of unsaturated hydrocarbons with ozone. Usually, they do not occur in high concentrations, but they are subject to fast dynamics. On the other hand, formaldehyde can cover a wide concentration range from sub-ppb to sub-ppm (note that ppb and ppm refer to volume mixing ratios) and the concentration changes over time take place within seconds as well as within days.
The spectroscopic properties of the C
O group limit the possibilities for direct analysis of carbonyl compounds using UV/VIS and infrared (IR). Therefore, mass spectrometric and chromatographic methods are common. In the past, polarography and ion chromatography have also been considered for the analysis of airborne aldehydes.18 It is advantageous that targeted derivatization reactions are possible via the C
O group. Nevertheless, analytical methods are often used today that are hardly suitable for the respective target compounds, and at the same time the results of analytical measurements are sometimes misunderstood or misinterpreted. This article offers an up-to-date overview of the analytical determination of organic carbonyl compounds in indoor air and discusses which methods can be applied for the respective task and which cannot.
2 Carbonyls relevant for the indoor environment
The available literature was evaluated in order to obtain an overview of the organic carbonyl compounds mainly found indoors. In particular, original papers, reviews and the results of environmental surveys were considered. Table 1 represents the result of this search with a total of 54 compounds. The selection is purely analytical and must not be interpreted in terms of health. This means that the substances listed in Table 1 are often detected in the indoor environment, but this is not automatically associated with a health risk. Of course, there are many other carbonyl compounds that can or have been identified in the indoor environment, usually in traces. This is especially true for the oxidation products of terpenes.19
Table 1 CAS numbers, molecular properties and preferred analytical method of the selected carbonyl compounds (T = Tenax TA, BC = black carbon). Only experimentally determined boiling points at standard atmospheric pressure are given. The respective literature sources are not listed
Compound |
CAS |
MW (g mol−1) |
BP (°C) |
Preferred method |
Formaldehyde |
50-00-0 |
30.03 |
−20 |
DNPH,35 Hantzsch36 |
Acetaldehyde |
75-07-0 |
44.05 |
21 |
DNPH35 |
Propanal |
123-38-6 |
58.08 |
48 |
DNPH35 |
Butanal |
123-72-8 |
72.11 |
75 |
DNPH35 |
2-Methyl propanal |
78-84-2 |
72.11 |
64 |
TD(T)-GC/MS37 |
Pentanal |
110-62-3 |
86.13 |
103 |
DNPH,35 TD(T)-GC/MS37 |
3-Methyl butanal |
590-86-3 |
86.13 |
93 |
DNPH35 |
Hexanal |
66-25-1 |
100.16 |
130 |
DNPH,35 TD(T)-GC/MS37 |
Heptanal |
111-71-7 |
114.19 |
153 |
TD(T)-GC/MS37 |
Octanal |
124-13-0 |
128.21 |
171 |
TD(T)-GC/MS37 |
Nonanal |
124-19-6 |
142.24 |
195 |
TD(T)-GC/MS37 |
Decanal |
112-31-2 |
156.26 |
212 |
TD(T)-GC/MS37 |
Undecanal |
112-44-7 |
170.29 |
225 |
TD(T)-GC/MS37 |
Acrolein |
107-02-8 |
56.06 |
52 |
TD(BC)-GC/MS25 |
Methacrolein |
78-85-3 |
70.09 |
73 |
TD(BC)-GC/MS38 |
cis-2-Butenal |
15798-64-8 |
70.09 |
104 |
TD(T)-GC/MS37 |
trans-2-Butenal |
123-73-9 |
70.09 |
102 |
TD(T)-GC/MS37 |
3-Methyl-2-butenal |
107-86-8 |
84.12 |
134 |
TD(T)-GC/MS37 |
trans-2-Pentenal |
1576-87-0 |
84.12 |
124 |
TD(T)-GC/MS37 |
3-Methyl-2-pentenal |
3592-19-6 |
98.14 |
|
TD(T)-GC/MS37 |
trans-2-Hexenal |
6728-26-3 |
98.14 |
147 |
TD(T)-GC/MS37 |
trans-2-Heptenal |
18829-55-5 |
112.17 |
166 |
TD(T)-GC/MS37 |
trans-2-Octenal |
2548-87-0 |
126.20 |
|
TD(T)-GC/MS37 |
trans-2-Nonenal |
18829-56-6 |
140.22 |
189 |
TD(T)-GC/MS37 |
trans-2-Decenal |
3913-81-3 |
154.25 |
229 |
TD(T)-GC/MS37 |
trans-2-Undecenal |
53448-07-0 |
168.28 |
245 |
TD(T)-GC/MS37 |
Furfural |
98-01-1 |
96.08 |
162 |
TD(T)-GC/MS37 |
Benzaldehyde |
100-52-7 |
106.12 |
179 |
DNPH,35 TD(T)-GC/MS37 |
2,5-Dimethyl benzaldehyde |
5779-94-2 |
134.17 |
245 |
DNPH,35 TD(T)-GC/MS37 |
Glyoxal |
107-22-2 |
58.04 |
50 |
PFBHA39–41 |
Methylglyoxal |
78-98-8 |
72.06 |
72 |
PFBHA39–41 |
Diacetyl |
431-03-8 |
86.02 |
88 |
TD(T)-GC/MS37 |
4-Oxopentanal (4-OPA) |
626-96-0 |
100.12 |
|
PFBHA,41 PTR-MS17 |
Pinonaldehyde |
2704-78-1 |
168.23 |
|
TD(T)-GC/MS37 |
trans-Cinnamaldehyde |
14371-10-9 |
132.16 |
253 |
TD(T)-GC/MS37 |
Geranial |
141-27-5 |
152.24 |
229 |
TD(T)-GC/MS37 |
Neral |
106-26-3 |
152.24 |
228 |
TD(T)-GC/MS37 |
Amyl cinnamal |
122-40-7 |
202.29 |
284 |
TD(T)-GC/MS37 |
Lilial |
80-54-6 |
204.31 |
275 |
TD(T)-GC/MS37 |
Acetone |
67-64-1 |
58.08 |
56 |
DNPH35 |
2-Butanone (MEK) |
78-93-3 |
72.11 |
80 |
TD(T)-GC/MS37 |
3-Buten-2-one (MVK) |
78-94-4 |
70.09 |
81 |
TD(BC)-GC/MS38 |
2-Pentanone |
107-87-9 |
86.13 |
102 |
TD(T)-GC/MS37 |
4-Methyl-2-pentanone (MIBK) |
108-10-1 |
100.16 |
116 |
TD(T)-GC/MS37 |
3-Octanone |
106-68-3 |
128.21 |
168 |
TD(T)-GC/MS37 |
6-Methyl-5-hepten-2-one (6-MHO) |
110-93-0 |
126.20 |
173 |
PTR-MS17 |
Geranylacetone |
3796-70-1 |
194.31 |
256 |
TD(T)-GC/MS37 |
Cyclohexanone |
108-94-1 |
98.15 |
156 |
TD(T)-GC/MS37 |
Acetophenone |
98-86-2 |
120.15 |
202 |
TD(T)-GC/MS37 |
Benzophenone |
119-61-9 |
182.22 |
305 |
TD(T)-GC/MS37 |
1-Methyl-2-pyrrolidone |
872-50-4 |
99.13 |
203 |
TD(T)-GC/MS37 |
1-Ethyl-2-pyrrolidone |
2687-91-4 |
113.16 |
213 |
TD(T)-GC/MS37 |
Caprolactam |
105-60-2 |
113.16 |
270 |
TD(T)-GC/MS37 |
Coumarin |
91-64-5 |
146.14 |
302 |
TD(T)-GC/MS37 |
In detail, the selection is based on the following references: formaldehyde;20,21 acetaldehyde;22,23 other saturated aldehydes (C3–C11);24 acrolein;25 methacrolein;16 other unsaturated aldehydes (C4–C11);8 pinonaldehyde;26 furfural;9,27 glyoxal, methylglyoxal;28 diacetyl;29 acetone, MEK, MIBK, 2-pentanone, 3-octanone;30 MVK;31 benzaldehyde, cyclohexanone, acetophenone, benzophenone;32 2,5-dimethyl benzaldehyde;33 geranial, neral, lilial, trans-cinnamaldehyde, amyl cinnamal;13 geranylacetone, 4-OPA, 6-MHO;17 caprolactam;15 1-methyl-2-pyrrolidone, 1 ethyl-2-pyrrolidone.34
3 The carbonyl (C
O) group
3.1 Electronic structure and spectroscopy
In carbonyl groups, the carbon atom is approximately sp2 hybridized and forms a σ-bond to the oxygen atom. The remaining p orbital of carbon overlaps with a p orbital of the oxygen atom to form a π-bond. In addition, two non-bonding (n) orbitals remain at the oxygen atom (see Fig. 2).42
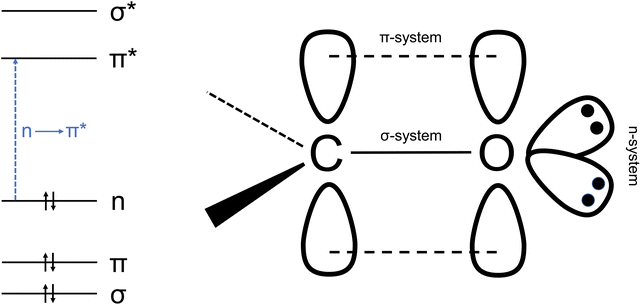 |
| Fig. 2 Electronic structure of the carbonyl group and qualitative view of the energy level scheme. | |
The absorption of electromagnetic radiation in the visible (VIS) and ultraviolet (UV) range causes electronic excitation. Organic molecules with an isolated C
O group have an absorption band in the UV range between 250 nm and 350 nm. This corresponds to an n → π* transition, i.e. the promotion of an electron from a nonbonding orbital on oxygen to the antibonding π* molecular orbital. In this process, charge is shifted from the electronegative oxygen atom to the delocalized π* orbital, which is associated with a change in the dipole moment. In the case of formaldehyde, the dipole moment of the first excited singlet and triplet state is smaller than that in the ground state.43 Transitions with n → π* character are forbidden for reasons of symmetry, so the transition intensities are usually small.44 For formaldehyde, the maximum of the UV absorption is at 294 nm, the peak cross section is 8.4 × 10−20 cm2 per molecule at 296 K.45 For the higher aldehydes and ketones with an isolated C
O group, the cross sections of the n → π* transition are in a similar range, but the maxima are shifted towards shorter wavelengths, which is due to the electron-donating alkyl groups.46 The absorption spectra of various aldehydes are discussed by Calvert and Pitts.47 Due to the simplicity of structure, the rotational and vibrational bands of formaldehyde can be resolved, allowing this molecule to be selectively excited.
Infrared spectroscopy offers an excellent opportunity for the analysis of carbonyl compounds, which is used in atmospheric chemistry for monitoring formaldehyde.48 The excitation of the C
O stretching vibration is associated with a large change in the dipole moment.49 As a result, the IR band of the isolated C
O group, which is in the wave number range from 1700 cm−1 to 1800 cm−1, has a particularly high intensity. Fig. 3 shows the theoretical IR spectrum of formaldehyde in the gas phase with the six possible normal modes according to the selection rules. The C
O band is at 1746 cm−1.50 In general, electron-donating neighboring groups result in a shift to lower wave numbers and electron-withdrawing neighboring groups result in a shift to higher wave numbers. However, the IR spectroscopic differences are usually too small to be able to distinguish between carbonyl compounds in the room air.
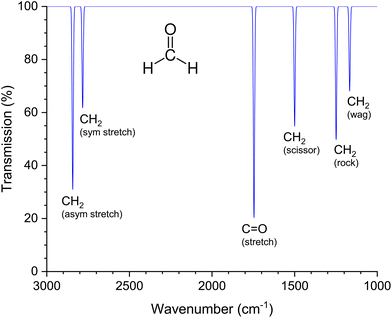 |
| Fig. 3 Simulated IR spectrum of formaldehyde in the gas phase. The wavenumbers of the six normal modes were taken from the publication by Nakanaga et al.50 The terms in brackets refer to different types of molecular vibrations (sym stretch: symmetrical stretching; asym stretch: antisymmetrical stretching; stretch: linear stretching; scissor: scissoring oder bending, rock: rocking; wag: wagging). | |
3.2 Chemistry
The C
O double bond is highly polar due to the different electronegativity of C and O and the easy polarizability of the π bond. Thus, because of its strong partial positive charge, the carbonyl carbon is a prominent electrophilic center in the aldehydes and ketones and is easily attacked by nucleophiles. Conversely, the oxygen is a nucleophilic center that is attacked by acid cations, for example H+. In the presence of an α-hydrogen atom, they undergo keto–enol tautomerism. So the chemistry of carbonyl compounds is diverse.42
Fig. 4 shows five reactions that are important from an analytical point of view. Hemiacetals or hemiketals are formed with alcohols, which then react further with the elimination of water to form acetals and ketals. The reaction can be catalysed by acids and bases. However, even in the absence, a noticeable reaction is observed if the alcohol is used as the solvent. This is important when preparing analytical standards in methanol. The Hantzsch reaction originally served to synthesize 1,4-dihydropyridines and pyridines.42 However, it has been shown that the dihydrolutidines synthesized with aldehydes and ketones are chromophores with broad absorption bands in the visible range and some of them also fluoresce. This makes these compounds interesting for analytical applications. The reaction of aldehydes and ketones with hydrazines to form hydrazones and the reaction of aldehydes with certain hydrazones also find wide attention in analytical chemistry. The resulting compounds have broad absorption bands in the UV and/or VIS. Oximes are formed when aldehydes and ketones react with hydroxylamine or its derivatives. It should be noted that only the formal reaction equations are shown in Fig. 4. The exact mechanisms can be found in organic chemistry textbooks.42,51 Other derivatization reactions for the analysis of aldehydes and ketones have been published,18,52 but these have largely lost their importance and will not be discussed here.
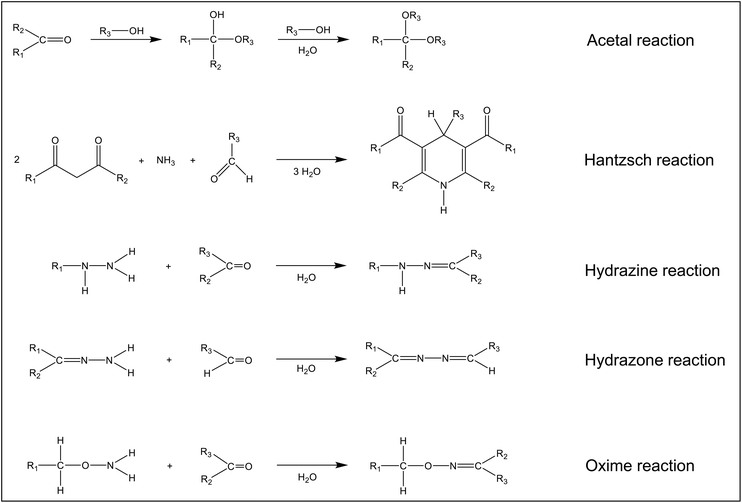 |
| Fig. 4 Chemical reactions of carbonyl compounds relevant to the analytical chemistry of these compounds. | |
4 Colorimetry
4.1 UV/VIS spectrometry and fluorescence
The simple colorimetric methods have the general disadvantage that no substance separation takes place. In the case of substance mixtures, one must therefore ensure that the wavelength selected for detection, whether in absorption or emission, is specific to the target compound, which is essentially formaldehyde. The application of the Hantzsch reaction to formaldehyde analysis with 2,4-pentanedione (acetyl acetone) and ammonium acetate using UV spectrometry of the formed 3,5-diacetyl-1,4-dihydrolutidine (DDL) (see Fig. 5) was first published by Nash.53 Belman54 suggested using the fluorescence of DDL for formaldehyde analysis because of higher selectivity and sensitivity. The full photophysical properties of DDL (absorption coefficient, fluorescence quantum yield, thermal and photochemical degradation) were later described in detail.55 DDL shows a broad absorption band at 412 nm and a fluorescence band at 510 nm. The derivatives of other carbonyl compounds with 2,4-pentanedione and ammonium acetate do not fluoresce, so the detection is specific for formaldehyde.56 However, the fluorescence quantum yield is strongly temperature dependent,57 so that attention must be paid to temperature constancy during the analysis. Salthammer55 showed that the fluorescence quantum yield of DDL decreases by 11% when the temperature is increased from 20 °C to 30 °C.
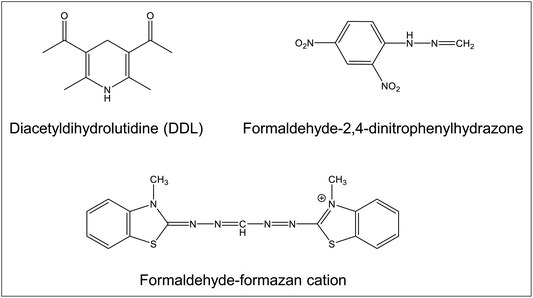 |
| Fig. 5 Chromophores resulting from the reaction of formaldehyde with acetyl actone (2,4-pentanedione), DNPH and MBTH. | |
Sampling is carried out by passing formaldehyde laden air through an absorber bottle where formaldehyde is quantitatively trapped in distilled water. The typical collected air volume is 60–80 l with an air flow rate of 2 l min−1. Then the reagents are added and at 40 °C the reaction is complete in a few minutes. This so-called acetyl acetone method is available as a European standard.58 The analytical details and comparisons with other methods are published in several papers,20,52,59–61 the full procedure can be found in EN 717-1.58 In Europe, EN 717-1 is recommended for determining the release of formaldehyde from wood-based materials in test chambers.
At this point, two publications must be addressed separately. Roffael's book62 deals with the role of formaldehyde in relation to wood and wood-based materials. Not only the historical and current methods for determining formaldehyde are discussed, but also the numerous international standards. Dugheri et al.63 provide an excellent overview of sampling and analysis methods for formaldehyde. Active and passive sampling devices are listed in detail, as well as the various analysis techniques.
The fast course of the Hantzsch reaction and the simple fluorimetric detection also make the acetyl acetone method interesting for automated online analysis with high time resolution. Corresponding devices are commercially available and their suitability for the sensitive formaldehyde analysis indoors and outdoors has been proven.20,64–66 When used in the field, however, the disadvantage is that the relatively large bottles with reagents also have to be carried along. With the conventional acetylacetone method, quantification limits (LOQ) of around 1 ppb (1.2 μg m−3) can be reached. The online method is even more sensitive at 0.3–0.5 ppb.
A completely different picture emerges with the MBTH method. Here, aldehydes react with 3-methyl-2-benzothiazolinone hydrazone (MBTH) to form a colored formazon cation (see Fig. 5).67,68 Sampling is carried out directly in an aqueous solution of MBTH. Ammonia iron(III) sulfate and acetic acid are then added to complete the reaction. Similar to acetyl acetone, MBTH is suitable for automated analysis. Goebel et al.,69 as well as Toda et al.70 present devices for the near real time measurement of gaseous formaldehyde. However, the detection technique is UV/VIS spectrometry at 628–629 nm, which makes the method non-specific. With the MBTH method, a sum value is always determined for different aldehydes, only the calibration is done with formaldehyde. This aspect is often ignored or misinterpreted. Therefore it can be assumed that formaldehyde concentrations measured with the MBTH method tend to be too high. This can cause problems when comparing formaldehyde data measured with different methods.71 Chan et al.72 argue that concentrations of aliphatic aldehydes are generally lower than formaldehyde, but this is not necessarily the case. Fig. 6 shows the results of a study by Giesen et al.73 It is a comparison of aldehyde measurements with 13 different materials (samples) in the air of environmental test chambers. The MBTH method suggests a formaldehyde concentration, which in fact results from the sum of several aldehyde components. Similar problems also arise for other colorimetric methods, which are not discussed here.20,52
 |
| Fig. 6 Results of aldehyde measurements with DNPH and MBTH in test chambers on 13 different materials. Experimental details and data are published by Giesen et al.73 | |
4.2 Enzymatic detection of formaldehyde
For an initial estimation of the formaldehyde concentration in the indoor environment, quick tests are sometimes used, which are available in pharmacies and drugstores and can also be handeled by non-experts. The principle is often based on an enzymatic reaction with a color change, the assessment is made optically against reference colors that are assigned to the corresponding concentration ranges. Such quick tests are quite suitable for an initial screening. However, one must always be aware that this is a very rough method that neither allows an exact concentration determination nor a health-related assessment.
Feldbrügge et al.74 describe the formaldehyde dehydrogenase catalyzed reaction of formaldehyde with NAD+ to formic acid and NADH. A diaphorase enzyme then transfers NADH and a colorless tetrazolium salt into NAD+ and a colored formazan derivative. A further development of this method was published by Monkawa et al.,75 who used the water soluble tetrazolium salt WST-8. In principle, it is also possible to determine the formaldehyde concentration by means of absorption measurements, although optical comparison has prevailed in the quick tests for reasons of simplicity.
5 Chromatography
5.1 HPLC-UV
The most important method for the analytical determination of low-molecular-weight carbonyl compounds in ambient air worldwide is DNPH. The exact procedures for sampling, separation of signals by HPLC and analysis are specified in ISO 16000-335 and can be read there. It is common practice that derivatization with 2,4-dinitrophenylhydrazine (see Fig. 4) is performed directly during sampling on DNPH impregnated silica cartridges. This can lead to the release of acetonitrile into the room air, so that VOC sampling using Tenax TA must not be carried out at the same time. The hydrazone formed with formaldehyde is shown in Fig. 5. The analysis is carried out by means of HPLC-UV (360 nm) after the formed hydrazones have been eluted from the cartridge with acetonitrile. The different hydrazones absorb light between 340 nm and 427 nm.52 Typical sampling times are 5–60 min at an air flow rate of 0.5–1.5 l min−1. To achieve a limit of quantification (LOQ) of 1–2 μg m−3, an air collection volume of at least 60 l is required. The European standard EN 1651676 “Construction products: Assessment of release of dangerous substances – determination of emissions into indoor air” stipulates the use of ISO 16000-3 for formaldehyde, acetaldehyde, propanal and butanal. Based on the DNPH reaction, Aiello and McLaren77 have developed an automated sampling analysis system that enables the sensitive determination of formaldehyde, acetaldehyde, propanal, acetone and benzaldehyde over 2 h cycles. The DNPH method is sensitive to nitrogen oxides78 and ozone,79 so these compounds must be removed before the sampled air reaches the cartridge. Williams et al.80 showed that carbon monoxide reacts with DNPH and interferes with the determination of acetone. DNPH-coated precolumns can also be used to remove interfering aldehydes and ketones from sampled air. This principle is applied in the gas chromatographic reactive sorption concentration (RSC) method.81
However, the DNPH method specified according to the 2022 version of ISO 16000-335 is far from being suitable for all carbonyl compounds. The standard applies to the saturated linear C1–C6 aldehydes, 3-methylbutanal, acetone, benzaldehyde, the methyl benzaldehydes and 2,5-dimethyl benzaldehyde. Acrolein and 2-butenal (crotonaldehyde) cannot be determined according to ISO 16000-3.35 Ho et al.82 examined the hydrazone derivatives of the unsaturated carbonyls acrolein, methacrolein, 2-butenal and 3-buten-2-one (MVK) and found that the DNPH is not suitable for these compounds. This is due in particular to the formation of dimers and trimers. However, Ho et al.83 also applied the DNPH method to the analysis of dicarbonyls and C7–C10 aldehydes. Salthammer and Mentese61 compared analytical methods for the determination of carbonyl compounds and found a very good agreement between the acetyl acetone and the DNPH method for formaldehyde. In contrast, when sampling on impregnated cartridges, the DNPH method gave lower results for pentanal and hexanal in comparison with thermal desorption gas chromatography/mass spectrometry (TD-GC/MS) using Tenax TA as the adsorbent. The reason for this lies in the increasing hydrophobicity of the aldehyde with the chain length. The formation of water from the condensation reaction increasingly wets the silica surfaces of the cartridge as the reaction progresses. DNPH is also only slightly soluble in water.
The DNPH method is very well suited for passive sampling, whereby furfural and the higher aldehydes up to C11 (undecanal) can also be analytically recorded. Birmili et al.24 determined the collection rates of a badge type passive sampler in a test chamber versus active methods according to ISO 16000-3 and TD-GC/MS for a total of 14 aldehydes. Villanueva et al.84 applied a cylinder type passive sampler with integrated ozone scubber for the analysis of formaldehyde and acetaldehyde in air. These and other types of passive samplers are discussed by Dugheri et al.63 Note that sampling rates only apply to a specific type of passive sampler and must therefore be determined individually.
Many variants of the DNPH method are known in the literature. Chi et al.85 present a method for 32 carbonyl compounds by HPLC and electrospray ionization (ESI) tandem mass spectrometry (MS/MS) after derivatization with DNPH. A gradient capillary electrochromatography method was developed by Feng and Zhu86 to measure 12 carbonyls in indoor air. Zhang et al.87 optimized a DNPH-HPLC-MS method for the simultaneous determination of 30 atmospheric carbonyls. These and other methods have their place in special tasks, for example in the analysis of exhaust gas, but are rarely used to examine indoor air quality.
5.2 TD-GC/MS
Similar to the HPLC method discussed in the previous section, sampling on adsorbents with subsequent thermal desorption (TD) and GC/MS analysis is also standardized with ISO 16000-6.37 VOCs are defined as compounds that elute between n-hexane and n-hexadecane on a 5% phenyl 95% methyl polysiloxane phase capillary column. Tenax TA is the preferred adsorbent for this retention range. Organic compounds eluting before n-hexane are defined as VVOCs and organic compounds eluting after n-hexadecane are defined as SVOCs. For compounds with higher vapor pressures than that of n-hexane (approx. 16 kPa at 20 °C), other sorbents such as graphitized carbon, carbon molecular sieve and multiple sorbents are preferred. An air collection tube is normally filled with 200 mg Tenax TA, typical collection rates are 4–6 l at an air flow rate of 100–150 ml min−1. The desorption of the collected molecules takes place at 300 °C in an inert gas stream. A quadrupole mass filter is standard for detection and quantification after gas chromatographic separation.88 Identification is based on a retention index versus internal and external standards and using the electron ionization mass spectrum (70 eV). If a six-membered transition state is possible after excitation, carbonyl compounds undergo rearrangement and fragmentation reactions. However, identification with the help of spectral libraries usually succeeds without problems.89 ISO 16000-637 generally requires a limit of quantification (LOQ) of at least 1 μg m−3 or less. This can be achieved with the usual sampling volumes of 4–6 l. An important issue concerns the accuracy and repeatability of ISO 16000-6 measurements. Wilke et al.90 evaluated the results of six round robin tests carried out in test chambers with a typical VOC spectrum and found a relative standard deviation of 28%. It must also be stated at this point that there are alternative definitions for VVOCs, VOCs and SVOCs, for example from the World Health Organization (WHO) and the U.S. EPA.31,91
The thermal desorption process is also ideal for the passive detection of indoor air pollutants. In the axial collection type, single sorbent filled stainless steel tubes are fitted with a cap containing a fine mesh gauze that defines the sample surface. The other end of the tube is capped and kept sealed. Radial collectors are cylindrical and comprise a sorbent sampling cartridge housed in a porous polymer that allows sampling along and around the whole surface.92 When sampling in the field, it must be remembered that Tenax TA (2,6-diphenyl oxide polymer) decomposes in the presence of ozone, nitrogen oxides and other reactive gases to form carbonyl compounds.93,94
From Table 1 it can be seen that the carbonyl compounds relevant for indoor environments cover a wide boiling point range between approximately −20 °C and +305 °C, but only a small proportion can be analyzed using the DNPH method. In Fig. 7 the retention indices of some carbonyl compounds are plotted in comparison to n-hexane and n-hexadecane. The horizontal red lines mark the boundary between VVOC/VOC and VOC/SVOC. The indices were calculated based on an alkane series for non-isothermal conditions.88 Strictly speaking, MEK is a VVOC and benzophenone is an SVOC, but these substances can be determined analytically after sampling on Tenax TA using TD-GC/MS according to ISO 16000-6.37 Even 2-methylpropanal (not displayed in Fig. 7), which is a VVOC with a retention index of around 500, can be analyzed using TD-GC/MS. Pentanal, although a C5 compound, already belongs to the group of VOCs. A comparison with the DNPH method has shown that aldehydes from C5 can be determined more precisely using TD-GC-MS with sampling on Tenax TA.61 Benzaldehyde and its methyl derivatives are qualified for analysis according to ISO 16000-3, but can be determined more easily and precisely according to ISO 16000-6. Acetone is clearly a VVOC and can be reliably determined using the DNPH method. The general problem with VVOCs is that not all substances are covered by one sampling technique.31 Schieweck et al.38 have developed a thermal desorption method for C3–C6 compounds with sampling on graphitized carbon, which also works for various carbonyl compounds (see Table 1). A medium-polarity capillary column proved to be suitable for the gas chromatographic separation. Richter et al.95 recommend combinations of graphitized carbon black, carbon molecular sieve and Tenax GR for the sampling of C1–C6 VVOCs with gas chromatographic separation on a polar capillary column. Even et al.96 have compared several gas chromatographic methods for VVOC analysis and conclude that water removal is the greatest practical challenge.
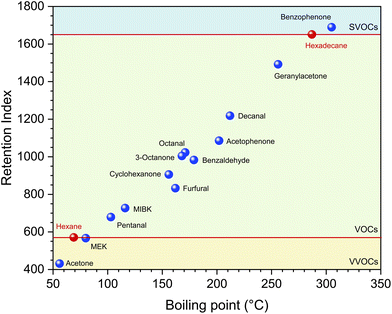 |
| Fig. 7 Gas chromatographic retention indices of several carbonyl compounds versus boiling point in comparison to n-hexane and n-hexadecane. The indices were determined against a series of alkanes using a laboratory-specific method for non-isothermal conditions.88 | |
Particular analytical problems are caused by the unsaturated compounds and dicarbonyls.82,97 Various methods have been published for acrolein, the simplest unsaturated aldehyde, which are summarized by Schieweck et al.25 The authors conclude that TD-GC/MS after sampling on graphitized carbon black is the most practical method for acrolein, because it is robust, easy-to-handle and very suitable for routine analyzes and surveys. With an air collection volume of 4 l a LOQ of 0.3 μg m−3 can be achieved. Sampling on graphitized black carbon with subsequent TD-GC/MS analysis also allows the determination of methylacrolein and MVK.38 The higher unsaturated aldehydes starting from butenal can be determined using TD-GC/MS and with Tenax TA as a sorbent. Fig. 8 shows a multistandard. The substances were dissolved in methanol, spiked onto Tenax TA and the solvent was evaporated in an inert gas flow. The compounds shown in red color and in italics are used only to identify their retention time on the capillary gas chromatographic column. The analytical details are provided in the figure caption.
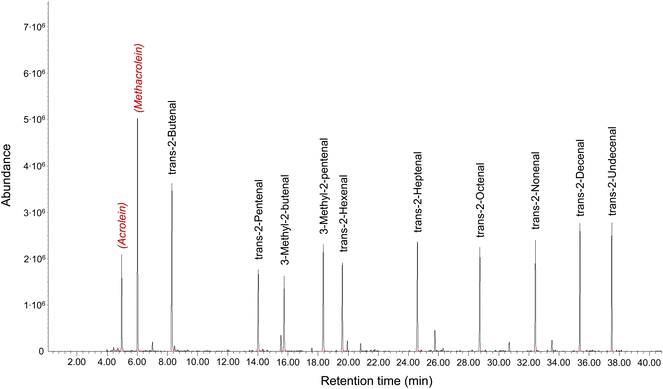 |
| Fig. 8 Chromatogram of a multistandard of unsaturated aliphatic aldehydes for analysis according to ISO 16000-6.37 Acrolein and methacrolein (in red) only serve to identify them on the capillary column via the retention time. Analytical parameters: GC: Agilent 7890; MS: 5977A; TD: Markes Unity TD100; Trap: −30 °C/300 °C; column: 5 MS Ultra inert 60 m × 250 μm × 0.25 μm; carier gas: He; oven: 32 °C → 300 °C; Mode: scan. | |
An alternative sampling technique is to collect air in stainless steel canisters. The contained VOCs are then pre-concentrated and injected into a GC/MS for separation, identification and quantification. Method TO-15A98 of the U.S. EPA for ambient air provides a list of organic compounds that can be determined in this way, including acrolein, acetone, MEK, MIBK and 2-hexanone. With appropriate validation, other carbonyl compounds can also be determined according to TO-15A.
The preparation of liquid standards for identification and quantification in GC/MS measurements can lead to a well-known artifact in carbonyl analysis. Methanol is often used as a solvent because it can be easily removed after spiking onto the respective sorbent. However, as shown in Fig. 4, carbonyl compounds react with alcohols first to form hemiacetals and then further to form acetals. Uhde and Salthammer99 observed the formation of 1,1-dimethoxy-cyclohexane from cyclohexanone in methanolic standard solutions. However, the reaction proceeds slowly under these conditions and can be prevented by storing the standard solution at −80 °C.
5.3 Other chromatographic methods
Most of the methods discussed in the following were developed for applications in outdoor air. Anderson et al.41 studied dicarbonyl compounds (glyoxal, methylglyoxal, glutaraldehyde, diacetyl, and 4-OPA) in a simulated indoor air environment. The target compounds were collected in methanol and then derivatized to oximes (see Fig. 4) with O-(2,3,4,5,6-pentafluorobenzyl) hydroxylamine (PFBHA) according to a method published by Yu et al.39 The mass spectrometric detection after gas chromatographic separation was carried out using an ion trap.100 PFBHA was used by Seaman et al.40 to determine the unsaturated aldehydes acrolein, methacrolein, MVK, 2-butenal and the dicarbonyls glyoxal and methylglyoxal. In this case, however, before the derivatization step, a formation of carbonyl-bisulfite adducts takes place. Wells and Ham used oxime formation with O-tert-butylhydroxylamine hydrochloride (TBOX) for the GC/MS detection of dicarbonyls resulting from the reaction of limonene with ozone.101
As an alternative to DNPH, dansylhydrazine (DNSH) can be used as a derivatization reagent with separation and detection via HPLC-UV for the analysis of carbonyl compounds. However, in the case of the unsaturated aldehydes, the identification of the derivatives is complex. For example, acrolein forms two mono-derivative isomers and one dimeric derivative with DNSH.102
Ho and Yu82,103 developed a technique based on derivatization with pentafluorophenyl hydrazine (PFPH) and TD-GC/MS. The carbonyl compounds are collected on Tenax TA coated with PFPH. The authors describe their method as suitable for a wide range of aliphatic aldehydes (up to C8), acrolein, furfural and dicarbonyls. Li et al.104 used collection onto solid sorbent coated with PFPH, followed by solvent extraction and GC/MS for the analysis of 20 airborne carbonyl compounds in the C1–C10 range. Pang et al.105 collected carbonyls on PFPH-coated Tenax TA, followed by solvent desorption and GC/MS analysis. The authors state that their method is reliable for 21 investigated carbonyl compounds in the C1–C9 range. When sampling takes place in a liquid medium, the relative derivatization performance between carbonyl compound and PFPH depends on the solvent.106 Bourdin and Desauziers107 used solid-phase microextraction (SPME) for the on-fiber derivatization of carbonyl compounds. The combination of a polydimethylsiloxane-divinylbenzene fiber with PFBHA proved to be suitable. For the investigated compounds formaldehyde, acetaldehyde and hexanal, acceptable to good limits of quantification were achieved using GC/MS analysis in the single ion mode (SIM) and in the extracted ion chromatogram (EIC). In general, SPME and other micromethods are well suited for screening purposes due to their easy handling.108 However, this is often offset by disadvantages in terms of sensitivity and calibration effort compared to conventional sampling techniques.
6 Molecular spectroscopy
Finlayson-Pitts and Pitts48 summarize the state-of-the-art analysis of carbonyl compounds in ambient air up to the year 2000. Many online methods have been developed for sensitive formaldehyde analysis in the ppt range. These include tunable diode laser spectroscopy (TDLS), cavity ring-down spectroscopy (CRDS), Fourier transform infrared (FTIR) and differential absorption optical spectroscopy (DOAS). Various comparison measurements with classical methods have been published: DOAS and CRDS with Hantzsch;109 DOAS and PTR-MS (see next section) with Hantzsch and DNPH.110 DOAS and FTIR with Hantzsch and DNPH.64 A work by Hanoune et al.111 comparing infrared diode laser spectroscopy with DNPH was performed indoors.
Another technique that has now become established for measuring formaldehyde in environmental test chambers and indoor air is photoacoustic spectroscopy (PAS). This involves irradiation of a sample with intermittent infrared light and detecting the periodic temperature changes in the sample as pressure fluctuations.112 The type of excitation is decisive for the selectivity and sensitivity. Conventional PAS devices work with a broadband IR source, the desired wavelength is selected with a narrow-band filter. In the case of carbonyl compounds, the less specific C–H vibrations between 2500 cm−1 and 3000 cm−1 (see Fig. 3) are usually observed. The poor resolution makes conventional PA spectroscopy unsuitable for the indoor air analysis of carbonyls. More advanced devices use laser excitation, whereby the analysis is usually limited to the well-resolved vibrational bands of formaldehyde. Hirschmann et al.113 measured the spectrum of formaldehyde from 1772 cm−1 to 1777 cm−1 by tuning a quantum cascade laser with a spectral resolution of 0.018 cm−1. The band at 1773.959 cm−1 was finally selected for analysis. The cross section of the formaldehyde C
O IR absorption band in this range is in the order of 10−18 cm2 per molecule,114 depending on the spectral integration width. This enables the specific monitoring of formaldehyde with detection limits in the sub-ppb range. Photoacoustics with quantum cascade lasers as a light source has now been further developed for routine analysis and, like the online Hantzsch method, enables specific, sensitive and precise formaldehyde analysis in indoor test chamber environments with high time resolution.
This is shown in Fig. 9 using the example of a chamber experiment with a burning candle according to EN 16738.115 The blue curve represents the time course of the formaldehyde chamber concentration, which was measured with a photoacoustic device (Gasera Ltd) and laser excitation of the C
O vibrational band at a time resolution of 10 s. The DNPH measurements (red dots) carried out for comparison correctly reflect the respective mean value over the sampling period of 60 minutes (red bars), but cannot depict the dynamics of the concentration profile.
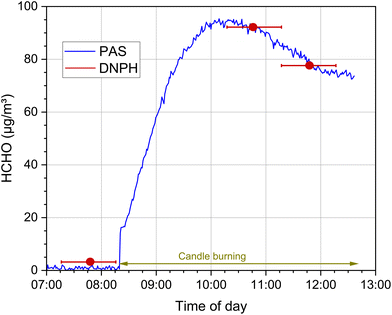 |
| Fig. 9 Time course of the formaldehyde concentration when burning a candle in a 1 m3 test chamber according to EN 16738115 (T = 23 °C, relative humidity = 50%, air exchange rate = 2 h−1, see also Salthammer et al.116 for the detailed experimental conditions). The blue curve represents the online measurement with a photoacoustic device (Gasera One), the red dots are the concentrations determined from a 60 min sampling using the DNPH method, the red lines indicate the duration of the sampling. | |
When using suitable IR laser light sources and multi-pass optical cells, classic IR spectroscopy can also be used under certain conditions for the specific determination of formaldehyde. The gas analysis to determine the formaldehyde release from wood-based materials is standardized according to ISO 12460-3,117 with the formaldehyde determination being carried out using the acetylacetone method. It is currently under discussion whether IR laser spectroscopy should also be permitted, provided the method shows equivalent test results to acetylacetone analysis.
7 Online mass spectrometry
In recent years, proton-transfer-reaction mass spectrometry (PTR-MS)118,119 has experienced increased application to indoor air issues. The reason for this is a wide range of studies on trace substances,120 chemical reactions121 and the personal chemical cloud of humans.122,123 A PTR-MS device consists of an ion source, a reaction and drift tube for transferring the proton to the target molecule (R) and a mass selective detector. In most cases, H3O+ ions are used, so the reaction proceeds according to eqn (1). A related technique is Selected Ion Flow Tube Mass Spectrometry (SIFT-MS), which is particularly applied for breath gas analysis.124 SIFT-MS employs eight reagent ions (H3O+, NO+, O2+, OH−, O−, O2−, NO2− and NO3−), which greatly facilitates the identification of isomeric organic compounds.
The [M + 1]+ ions generated in the PTR-MS drift tube can be analyzed using either a low-resolution quadrupole filter (QMS) or a high-resolution time-of-flight (ToF) detector. Quadrupoles have only limited applications and are not usually used to identify components in mixtures of substances.125 However, reaction (1) only occurs if the proton affinity of the target molecule is higher than that of water (691 kJ mol−1).126 This means that PTR-MS is hardly suitable for the analysis of formaldehyde, which has a proton affinity of 712 kJ mol−1.126 In this case increasing humidity strongly supports the back reaction.127,128 Another analytical problem is the fragmentation of molecules with longer chain lengths, which has been investigated for aldehydes and ketones by Španěl et al.129 There is no fragmentation observable up to propanal (C3). Starting with butanal (C4), the [M + 1]+ ions increasingly split off water, which is shown in eqn (2) for the hexanal example. The ratio of m/z 101.096 to m/z 83.086 is approximately 1
:
1.129,130 On the other hand, the fragmentation of aldehydes is advantageous insofar that it can be used to distinguish from ketones of the same molecular mass, which, according to the results of Španěl et al.,129 do not fragment. A comprehensive library with fragmentation reactions of organic compounds after protonation was published by Pagonis et al.131
|  | (2) |
The concentration of the target molecule [R] can be obtained from
eqn (3) if the drift time
tr in the reaction tube and the proton-transfer rate constant
kPT are known. [RH
+] and [H
3O
+] are the concentrations of the product and the primary ion, respectively. This shows the difference between PTR-MS and other analytical procedures, because there is no calibration in the classic sense using conventional statistical methods.
132 The drift time
tr has to be calculated from the respective instrument settings.
133 | 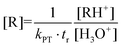 | (3) |
The reaction rate constant
kPT can only be determined experimentally to a very limited extent, since the corresponding test gas is required for this. It is much easier to calculate
kPT from the ion-dipole collision theory. With the so-called “capture theory”, Su
134 has developed parameterizations based on trajectory analyses.
Eqn (4) shows the principle. According to Langevin, the reaction constant consists of a non-polar part
kL, which only takes into account the polarizability
α, and a polar part
kμD. In practice,
kPT is obtained by multiplying
kL with the capture parameter
Kcap, which depends on the dipole moment
μD of the molecule and the temperature
T in the reaction chamber. The parametrization procedure for calculating
Kcap was originally published by Su
134 and has been summarized by Cappellin
et al.133 and Salthammer
et al.135 for PTR-MS applications.
| kPT = kL + kμD = kL(α) × Kcap(μD, T) | (4) |
The calculation according to the capture theory also raises some problems. Firstly, the polarizabilities and dipole moments for many organic compounds in the gas phase are not known or only known imprecisely. Secondly, kPT also depends on the reaction conditions in the drift tube, in particular on the ratio of the electric field strength (E) to the molecular density (N). Fig. 10 shows the kPT values for three polar molecules as a function of E/N. Note that the kPT of non polar compounds is not affected by the E/N value. E/N ratios of 100–120 Td are typical for PTR-MS measurements. Lower values result in more water clusters, higher values result in more complicated fragmentation patterns.
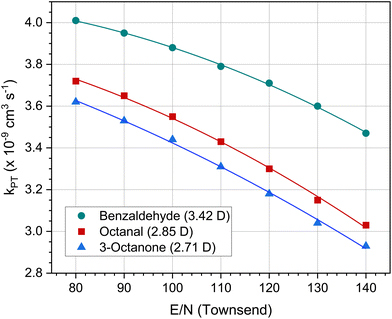 |
| Fig. 10 Dependence of the proton-transfer reaction constant kPT on the E/N ratio (electric field to molecular density) set in the PTR-MS device at 353 K for benzaldehyde, octanal and 3-octanone. The values in brackets indicate the respective dipole moment in Debye. The data were taken from Salthammer et al.135 | |
Table 2 lists carbonyl compounds for which kPT values calculated according to the capture theory (E/N = 120 Townsend) are available. The dipole moments and polarizabilities for the gas phase were calculated using quantum chemical methods. The values of Salthammer et al.135 refer to 353 K, those of Cappellin et al.133 to 363 K.
Table 2 CAS numbers, proton affinities (PA),126 dipole moments (μD), polarizabilities (α) and proton-transfer rate coefficients kPT at 353 K135 or 363 K133 (120 Td) of carbonyl compounds
Compound |
CAS |
PA (kJ mol−1) |
μ
D (Debye) |
α (10−24 cm3) |
k
PT (10−9 cm3 s−1) |
Acetaldehyde135 |
75-07-0 |
768.5 |
2.94 |
4.45 |
3.13 |
Propanal135 |
123-38-6 |
786.0 |
2.85 |
6.14 |
3.12 |
Butanal135 |
123-72-8 |
792.7 |
2.83 |
7.90 |
3.16 |
2-Methyl propanal135 |
78-84-2 |
797.3 |
2.91 |
7.88 |
3.21 |
Pentanal135 |
110-62-3 |
796.6 |
2.85 |
9.68 |
3.22 |
Hexanal135 |
66-25-1 |
|
2.83 |
11.45 |
3.24 |
Heptanal135 |
111-71-7 |
|
2.86 |
13.25 |
3.29 |
Octanal135 |
124-13-0 |
|
2.85 |
15.04 |
3.30 |
Nonanal135 |
124-19-6 |
|
2.85 |
16.84 |
3.32 |
Decanal135 |
112-31-2 |
|
2.85 |
18.66 |
3.35 |
Acrolein (trans)135 |
107-02-8 |
797.0 |
3.43 |
6.34 |
3.54 |
Acrolein (cis)135 |
107-02-8 |
797.0 |
2.81 |
6.14 |
3.11 |
Methacrolein133 |
78-85-3 |
808.7 |
2.80 |
8.41 |
3.20 |
trans-2-Butenal135 |
123-73-9 |
|
4.20 |
8.40 |
4.11 |
trans-2-Hexenal135 |
6728-26-3 |
|
4.37 |
12.02 |
4.33 |
Furfural (trans)135 |
98-01-1 |
|
3.60 |
9.82 |
3.70 |
Furfural (cis)135 |
98-01-1 |
|
4.31 |
9.88 |
4.15 |
Benzaldehyde135 |
100-52-7 |
834.0 |
3.42 |
12.53 |
3.71 |
Glyoxal (cis)135 |
107-22-2 |
|
3.68 |
4.64 |
3.51 |
4-OPA135 |
626-96-0 |
|
2.95 |
9.73 |
3.24 |
Pinonaldehyde133 |
2704-78-1 |
|
2.26 |
18.56 |
2.96 |
Acetone135 |
67-64-1 |
812.0 |
3.11 |
6.15 |
3.29 |
MEK135 |
78-93-3 |
827.3 |
2.97 |
7.86 |
3.25 |
2-Pentanone133 |
107-87-9 |
832.7 |
2.84 |
10.07 |
3.24 |
MIBK135 |
108-10-1 |
|
2.87 |
11.36 |
3.27 |
3-Octanone135 |
106-68-3 |
|
2.71 |
14.90 |
3.18 |
6-MHO135 |
110-93-0 |
|
2.88 |
15.12 |
3.34 |
Geranylacetone135 |
3796-70-1 |
|
2.78 |
23.77 |
3.44 |
Cyclohexanone135 |
108-94-1 |
841.0 |
3.45 |
10.57 |
3.65 |
Acetophenone135 |
98-86-2 |
861.1 |
3.16 |
14.20 |
3.55 |
Benzophenone135 |
119-61-9 |
882.3 |
3.11 |
22.62 |
3.63 |
1-Methyl-2-pyrrolidone135 |
872-50-4 |
923.5 |
4.12 |
10.28 |
4.05 |
The PTR-MS method is now firmly established in the determination of airborne carbonyl compounds and is always in demand when small concentrations with high dynamics have to be monitored. However, one must not overlook the disadvantages related to calibration, which will be discussed in a later section.
8 Ion mobility spectrometry
Another analysis method that works with a drift tube is ion mobility spectrometry (IMS). The technique is widely used for routine detection of explosives and drugs. With regard to carbonyls, IMS is suitable for monitoring the production process of beer with the off-flavor target compounds diacetyl and 2,3-pentanedione.136 The molecule is ionized in the tube and moves in an electric field depending on its mass and other molecular properties with a specific drift velocity to the detector, which is often an electrometer. The drift time of the ions is usually in the millisecond range and also depends on the length of the drift tube. Sensitivity and selectivity of the IMS can be significantly increased by combination with gas chromatography and mass spectrometry.137–139
So far there have only been a few applications of the IMS in the chemical analysis of indoor air. The reason for this is that other methods such as TD-GC/MS have clear advantages in non-target analysis. IMS can always be considered when dealing with a small and defined spectrum of organic molecules. Tiebe et al.140 used IMS to detect 14 microbial volatile organic compounds (MVOCs), including various ketones, that can serve to screen for mold infestation in rooms. However, the detection limits for these carbonyls were disproportionately high at 8–14 μg m−3. A combination of gas chromatography and IMS was used by Ruzsanyi et al.141 to detect saturated and unsaturated carbonyl compounds released from the skin surface. By using a short multi-capillary column, a time resolution of about 5 min could be achieved.
There are a few other publications that deal with the application of IMS for analytical questions of indoor air.142 In conclusion it can be stated that the method certainly has some interesting features, but no significant advantages over those already discussed.
9 Discussion
Szulejko and Kim143 provide a summary of derivatization techniques for the determination of carbonyls in air, which also addresses sampling techniques such as solid phase micro extraction (SPME), which is not addressed in detail in this work. The authors conclude that despite many problems, carbonyl analysis techniques still rely heavily on the combined application of DNPH derivatization, HPLC separation and UV or MS detection. Table 1 lists analytical methods recommended for each compound. However, this does not mean that the determination must always be carried out using this method. The release of formaldehyde from wood-based materials is a slow process144 that can be easily tracked using EN 717-1.36 In contrast, 4-OPA and 6-MHO are formed and released within seconds when the skin surface is exposed to ozone, which necessitates high-resolution online detection.17Table 3 summarizes the methods discussed in this work. The table is not complete, but derivatization methods that are rarely used today, such as chromotropic acid and pararosaniline, are not considered and can be looked up in Vairavamurthy et al.52 and Szulejko and Kim.143 Moreover, some of the methods listed in Table 3 aim at detecting outdoor air species, which often requires very low detection limits. In the indoor environment, however, compliance with guide values is often important,145 which requires good precision and reproducibility, but usually does not pose an analytical challenge with regard to the detection limit. An exception is acrolein.25
Table 3 Analytical methods for the quantitative determination of carbonyl compounds in indoor and outdoor air
Method |
Comments |
Hantzsch53,54 |
Selective for formaldehyde with fluorescence detection,55 standardized by EN 717-136 |
Hantzsch online110 |
Selective for formaldehyde with fluorescence detection |
DNPH (HPLC-UV) |
Recommended for selected aldehydes and ketones, standardized by ISO 16000-335 and ASTM 5197-21146 |
DNPH (HPLC-MS)87 |
30 carbonyls |
DNPH (HPLC-MS/MS)85 |
32 carbonyl compounds |
DNSH (HPLC-UV)102 |
15 saturated and unsatured aldehydes |
PFPH (TD-GC/MS)103 |
14 carbonyls and dicarbonyls |
PFPH (GC/MS)105 |
21 carbonyls |
PFBHA (GC-MS)41 |
Dicarbonyls |
PFBHA (GC/MS)40 |
Dicarbonyls and unsaturated aldehydes |
TBOX (GC/MS)101 |
Dicarbonyls from limonene/ozone reaction |
TD-GC/MS (Tenax TA) |
Carbonyls ≥C4, standardized by ISO 16000-637 |
TD-GC/MS (carbon black)25,38 |
Saturated and unsaturated aldehydes ≤C4 |
Canister sampling (GC/MS)98 |
Acrolein, acetone, MEK, MIBK, 2-hexanone, standardized by U.S. EPA TO-15A |
MBTH73 |
Not specific, sum value for aldehydes |
TDLS111 |
Selective for formaldehyde |
DOAS109,110 |
Selective for formaldehyde |
CRDS109 |
Selective for formaldehyde |
FTIR64 |
Selective for formaldehyde |
PAS (laser excitation)113 |
Selective for formaldehyde |
PTR-MS118,147 |
Broad range of carbonyls and dicarbonyls |
SIFT-MS124 |
Broad range of carbonyls and dicarbonyls |
IMS138,139 |
Suitable for preselected carbonyls and dicarbonyls |
Other methods |
Vairavamurthy et al.,52 Szulejko and Kim143 |
One has to realize that several methods are usually required to detect a wide range of carbonyl compounds in the room air. By default, this is the DNPH method according to ISO 16000-335 in combination with the TD-GC/MS method according to ISO 16000-6.37 Some substances can be determined using both methods. As a rule of thumb, the TD-GC/MS method delivers better results from a chain length of C5.61 Other methods can be used alternatively or additionally. Today it is undisputed that unsaturated carbonyls cannot be determined quantitatively with the DNPH method. With regard to the dicarbonyls, the statements are contradictory.
Most absorption and IR spectroscopic online methods are only suitable for formaldehyde. In absorption spectroscopy, this is due to the comparatively well-resolved and red-shifted spectral structure. However, the weak n → π* transition requires long light paths, so techniques such as DOAS cannot necessarily be recommended for indoor use. A different picture emerges for photoacoustics. Quantum cascade lasers enable the targeted excitation of the C
O stretching vibration band of formaldehyde and the necessary detection limits can also be achieved.113 The technical designs of the available devices correspond to practical requirements.
The PTR-MS method requires a detailed evaluation and critical discussion. The method undoubtedly has a number of advantages when it comes to sensitivity and temporal resolution. However, even when using a time-of-flight mass detector (ToF), it is not possible to distinguish the [M + 1]+ ions from molecules of identical monoisotopic masses. This is only possible using typical fragmentation patterns. Moreover, the fragmentation reactions depend on the E/N ratio in the drift tube and increase with increasing E/N.118 This may also be the reason why different ratios of fragmentation ions are reported in the literature.131 The interpretation of fragmentation patterns becomes more difficult with increasing number of chlorine, bromine and sulfur atoms due to their natural isotopic distribution. In addition, substances such as 1-octen-3-ol and octanal show almost indistinguishable fragmentation patterns.148
The more important point concerns the calibration of PTR-MS measurements. Standard methods such as ISO 16000-335 and 16000-637 have the advantage that their calibration is traceable and they can therefore be accredited. This is not the case with the PTR-MS. The kPT values at 353 K of the three carbonyls shown in Fig. 10 are calculated and differ by 15–25% in the range from 80 Td to 140 Td. Another critical parameter in eqn (3) is the drift time tr, which is made up of measured variables such as the length of the drift tube and the drift voltage, but also of calculated variables such as the reduced ion mobility. Therefore, the PTR-MS can only be calibrated for a specific device setting. Pham et al.149 investigated the VOC release rates from 3D printed samples by coupling a microchamber/thermal extractor system with a PTR-MS. Where available, the authors use compound specific reaction rate coefficients for calibration. Otherwise a default value of kPT = 2.0 × 10−9cm3 s−1 was applied. Pagonis et al.150 used a 10 component test gas for six point calibration. For other compounds, calibration factors were determined by averaging the factors of all calibrants. Lunderberg et al.120 also performed the calibration of their PTR-MS with authentic external standards. If these were not available, the default values of the device were used; the authors estimate the error at −40% to +60%. It is clear that the use of such default values disqualifies analytical methods when it comes to health assessments of building product emissions or indoor air quality. The three cited publications120,149,150 correctly emphasize that PTR-MS is the method of choice to characterize the dynamic nature of VVOCs and VOCs and is especially suited to measuring highly volatile compounds. However, there is still a long way to go before the method can be used for tasks that require accredited measurements. This applies in particular to the polar carbonyl compounds, whose reaction constants are strongly influenced by the system settings.
10 Conclusion
For various reasons, it is important and necessary to determine the quality and quantity of carbonyl compounds in the room air. Substances such as formaldehyde20 and acetaldehyde23 are classified as carcinogenic and are subject to strict regulations, guide values have been published for various aldehydes, ketones and lactams,145 substances such as decanal,151 4-OPA, 6-MHO, geranylacetone,17 glyoxal and acroleine152 are markers for chemical reactions. Individual compounds can be specifically analyzed and with the necessary accuracy. However, the task becomes more difficult when many carbonyl compounds have to be determined in parallel and the appropriate methods have to be selected in a targeted manner. This contribution should help to choose the right analytical method and to keep the effort as low as possible but as large as necessary.
Abbreviations
BP | Boiling point |
CRDS | Cavity ring down spectroscopy |
DDL | 3,5-Diacetyl-1,4-dihydrolutidine |
DNPH | 2,4-Dinitrophenylhydrazine |
DNSH | Dansylhydrazine |
DOAS | Differential optical absorption spectroscopy |
EIC | Extracted ion chromatogram |
EN | European norm |
E/N | Ratio of electric field strength and molecular density in the PTR-MS drift tube |
ESI | Electrospray ionization |
FTIR | Fourier-transform infrared |
GC/MS | Gas chromatography/mass spectrometry |
HPLC | High performance liquid chromatography |
IMS | Ion mobility spectrometry |
IR | Infrared |
ISO | International organization for standardization |
K
cap
| Capture parameter in ion-dipole collisional theory |
k
L
| Proton-transfer rate constant according to Langevin |
k
PT
| Proton-transfer rate constant |
k
μ
D
| Dipole related proton-transfer rate constant |
LOQ | Limit of quantitation |
MBTH | 3-Methyl-2-benzothiazolinone hydrazone |
MS/MS | Tandem mass spectrometry |
MVOC | Microbial volatile organic compound |
MW | Molecular weight |
NAD+ | Oxidized nicotinamide adenine dinucleotide |
NADH | Reduced nicotinamide adenine dinucleotide |
PAS | Photo acoustic spectroscopy |
PFBHA |
O-(2,3,4,5,6-Pentafluorobenzyl) hydroxylamine |
PFPH | Pentafluorophenyl hydrazine |
ppb | Parts per billion (refers to the volume mixing ratio) |
ppm | Parts per million (refers to the volume mixing ratio) |
PTR-MS | Proton-transfer-reaction mass spectrometry |
SIFT-MS | Selected ion flow tube mass spectrometry |
SIM | Single ion mode |
SPME | Solid phase micro extraction |
SVOC | Semi volatile organic compound |
TBOX |
O-tert-Butylhydroxylamine hydrochloride |
TD | Thermal desorption |
TDLS | Tunable diode laser spectroscopy |
Tenax GR | Graphitized Tenax |
UV | Ultraviolet |
VIS | Visible |
VOC | Volatile organic compound |
VVOC | Very volatile organic compound |
WST-8 | Sodium-2-(2-methoxy-4-nitrophenyl)-3-(4-nitrophenyl)-5-(2,4-disulfophenyl)-2H-tetrazolium |
Conflicts of interest
The author has no conflicts of interest to declare.
Acknowledgements
T. S. is grateful to Alexander Omelan, Sebastian Wientzek and Dr. Erik Uhde, Fraunhofer WKI, for helpful discussions and technical support.
References
-
S. Manahan, Environmental Chemistry, CRC Press, Boca Raton, FL, 2017 Search PubMed.
-
Principles of Toxicology: Environmental and Industrial Applications, ed. S. M. Roberts, R. C. James and P. L. Williams, John Wiley & Sons Inc., Hoboken, NJ, 2022 Search PubMed.
-
P. J. Walsh, C. S. Dudney and E. D. Copenhaver, Indoor Air Quality, CRC Press, Boca Raton, FL, 1984 Search PubMed.
-
J. F. Walker, Formaldehyde, Robert E. Krieger Publishing Company, Huntington, NY, 1975 Search PubMed.
- S. K. Brown, M. R. Sim, M. J. Abramson and C. N. Gray, Concentrations of volatile organic compounds in indoor air - a review, Indoor Air, 1994, 4, 123–134 CrossRef CAS.
- J. Zhang, P. J. Lioy and Q. He, Characteristics of aldehydes: concentrations, sources, and exposures for indoor and outdoor residential microenvironments, Environ. Sci. Technol., 1994, 28, 146–152 CrossRef CAS PubMed.
- T. Salthammer, Emission of volatile organic compounds from furniture coatings, Indoor Air, 1997, 7, 189–197 CrossRef CAS.
- T. Salthammer, A. Schwarz and F. Fuhrmann, Emission of reactive compounds and secondary products from wood-based furniture coatings, Atmos. Environ., 1998, 33, 75–84 CrossRef.
- M. Makowski and M. Ohlmeyer, Influences of hot pressing temperature and surface structure on VOC emissions from OSB made of Scots pine, Holzforschung, 2006, 60, 533–538 CAS.
- Y. Liu, X. Zhu, X. Qin, W. Wang, Y. Hu and D. Yuan, Identification and characterization of odorous volatile organic compounds emitted from wood-based panels, Environ. Monit. Assess., 2020, 192, 348 CrossRef CAS PubMed.
- C. J. Weschler, A. T. Hodgson and J. D. Wooley, Indoor chemistry: ozone, volatile organic compounds, and carpets, Environ. Sci. Technol., 1992, 26, 2371–2377 CrossRef CAS.
- G. C. Morrison and W. W. Nazaroff, Ozone interactions with carpet: secondary emissions of aldehydes, Environ. Sci. Technol., 2002, 36, 2185–2192 CrossRef CAS PubMed.
- J. Bartsch, E. Uhde and T. Salthammer, Analysis of odour compounds from scented consumer products using gas chromatography-mass spectrometry and gas chromatography-olfactometry, Anal. Chim. Acta, 2016, 904, 98–106 CrossRef CAS PubMed.
- P. Azimi, D. Zhao, C. Pouzet, N. E. Crain and B. Stephens, Emissions of ultrafine particles and volatile organic compounds from commercially available desktop three-dimensional printers with multiple filaments, Environ. Sci. Technol., 2016, 50, 1260–1268 CrossRef CAS PubMed.
- J. Gu, M. Wensing, E. Uhde and T. Salthammer, Characterization of particulate and gaseous pollutants emitted during operation of a desktop 3D printer, Environ. Int., 2019, 123, 476–485 CrossRef CAS PubMed.
- W. Zhang, Z. Bai, L. Shi, J. H. Son, L. Li, L. Wang and J. Chen, Investigating aldehyde and ketone compounds produced from indoor cooking emissions and assessing their health risk to human beings, J. Environ. Sci., 2023, 127, 389–398 CrossRef PubMed.
- A. Wisthaler and C. J. Weschler, Reactions of ozone with human skin lipids: Sources of carbonyls, dicarbonyls, and hydroxycarbonyls in indoor air, Proc. Natl. Acad. Sci. U. S. A., 2010, 107, 6568–6575 CrossRef CAS PubMed.
- R. Otson and P. Fellin, A review of techniques for measurement of airborne aldehydes, Sci. Total Environ., 1988, 77, 95–131 CrossRef CAS PubMed.
- A. Calogirou, B. Larsen and D. Kotzias, Gas-phase terpene oxidation products: a review, Atmos. Environ., 1999, 33, 1423–1439 CrossRef CAS.
- T. Salthammer, S. Mentese and R. Marutzky, Formaldehyde in the indoor environment, Chem. Rev., 2010, 110, 2536–2572 CrossRef CAS PubMed.
- T. Salthammer, Formaldehyde sources, formaldehyde concentrations and air exchange rates in European housings, Build. Environ., 2019, 150, 219–232 CrossRef.
- D. A. Sarigiannis, S. P. Karakitsios, A. Gotti, I. L. Liakos and A. Katsoyiannis, Exposure to major volatile organic compounds and carbonyls in European indoor
environments and associated health risk, Environ. Int., 2011, 37, 743–765 CrossRef CAS PubMed.
- T. Salthammer, Acetaldehyde in the indoor environment, Environ. Sci.: Atmos., 2023, 3, 474–493 CAS.
- W. Birmili, A. Daniels, R. Bethke, N. Schechner, G. Brasse, A. Conrad, M. Kolossa-Gehring, M. Debiak, J. Hurraß, E. Uhde, A. Omelan and T. Salthammer, Formaldehyde aliphatic aldehydes (C2-C11), furfural, and benzaldehyde in the residential indoor air of children and adolescents during the German Environmental Survey 2014–2017 (GerES V), Indoor Air, 2022, 32, e12927 CrossRef CAS PubMed.
- A. Schieweck, E. Uhde and T. Salthammer, Determination of acrolein in ambient air and in the atmosphere of environmental test chambers, Environ. Sci.: Processes Impacts, 2021, 23, 1729–1746 RSC.
- P. Wolkoff, P. A. Clausen, C. K. Wilkins and G. D. Nielsen, Formation of strong airway irritants in terpene/ozone mixtures, Indoor Air, 2000, 10, 82–91 CrossRef CAS PubMed.
- T. Salthammer and F. Fuhrmann, Release of acetic acid and furfural from cork products, Indoor Air, 2000, 10, 133–134 CrossRef CAS PubMed.
- W. Liu, J. Zhang, L. Zhang, B. J. Turpin, C. P. Weisel, M. T. Morandi, T. H. Stock, S. Colome and L. R. Korn, Estimating contributions of indoor and outdoor sources to indoor carbonyl concentrations in three urban areas of the United States, Atmos. Environ., 2006, 40, 2202–2214 CrossRef CAS.
- M. Sleiman, J. M. Logue, V. N. Montesinos, M. L. Russell, M. I. Litter, L. A. Gundel and H. Destaillats, Emissions from electronic cigarettes: Key parameters affecting the release of harmful chemicals, Environ. Sci. Technol., 2016, 50, 9644–9651 CrossRef CAS PubMed.
-
H. Fromme, Luftverunreinigungen in Innenräumen, Ecomed Medizin, Landsberg, 2021 Search PubMed.
- T. Salthammer, Very volatile organic compounds: an understudied class of indoor air pollutants, Indoor Air, 2016, 26, 25–38 CrossRef CAS PubMed.
- T. Salthammer, M. Bednarek, F. Fuhrmann, R. Funaki and S.-I. Tanabe, Formation of organic indoor air pollutants by UV-curing chemistry, J. Photochem. Photobiol., A, 2002, 152, 1–9 CrossRef CAS.
- P. Harb, N. Locoge and F. Thevenet, Emissions and treatment of VOCs emitted from wood-based construction materials: Impact on indoor air quality, Chem. Eng. J., 2018, 354, 641–652 CrossRef CAS.
- T. Salthammer, Y. Zhang, J. Mo, H. M. Koch and C. J. Weschler, Assessing Human Exposure to Organic Pollutants in the Indoor Environment, Angew. Chem., Int. Ed., 2018, 57, 12228–12263 CrossRef CAS PubMed.
-
ISO 16000-3, Indoor air – Part 3: Determination of formaldehyde and other carbonyl compounds in indoor and test chamber air - active sampling method, International Organization for Standardization, Geneva, 2022 Search PubMed.
-
EN 717-1, Wood-based panels – Determination of formaldehyde release - Part 1: Formaldehyde emission by the chamber method, Beuth Beuth Verlag, Berlin, 2004 Search PubMed.
-
ISO 16000-6, Indoor air – Part 6: Determination of organic compounds (VVOC, VOC, SVOC) in indoor and test chamber air by active sampling on sorbent tubes, thermal desorption and gas chromatography using MS or MS FID, International Organization for Standardization, Geneva, 2021 Search PubMed.
- A. Schieweck, J. Gunschera, D. Varol and T. Salthammer, Analytical procedure for the determination of very volatile organic compounds (C3-C6) in indoor air, Anal. Bioanal. Chem., 2018, 410, 3171–3183 CrossRef CAS PubMed.
- J. Yu, R. C. Flagan and J. H. Seinfeld, Identification of products containing -COOH, -OH, and -CO in atmospheric oxidation of hydrocarbons, Environ. Sci. Technol., 1998, 32, 2357–2370 CrossRef CAS.
- V. Y. Seaman, M. J. Charles and T. M. Cahill, A sensitive method for the quantification of acrolein and other volatile carbonyls in ambient air, Anal. Chem., 2006, 78, 2405–2412 CrossRef CAS PubMed.
- S. E. Anderson, L. G. Jackson, J. Franko and J. R. Wells, Evaluation of dicarbonyls generated in a simulated indoor air
environment using an in vitro exposure system, Toxicol. Sci., 2010, 115, 453–461 CrossRef CAS PubMed.
-
A. Streitwieser, C. H. Heathcock and E. M. Kosower, Introduction to Organic Chemistry, Macmillen Publishing Co. Ltd, New York, 1992 Search PubMed.
- A. D. Buckingham, D. A. Ramsay and J. Tyrrell, Electric dipole moment of formaldehyde in the first 3A2 excited state, Can. J. Phys., 1970, 48, 1242–1253 CrossRef CAS.
-
N. J. Turro, V. Ramamurthy and J. C. Scaiano, Modern Molecular Photochemistry of Organic Molecules, University Science Books, Sausalito, CA, 2010 Search PubMed.
- J. D. Rogers, Ultraviolet absorption cross sections and atmospheric photodissociation rate constants of formaldehyde, J. Phys. Chem., 1990, 94, 4011–4015 CrossRef CAS.
- R. D. Martinez, A. A. Buitrago, N. W. Howell, C. H. Hearn and J. A. Joens, The near U.V. absorption spectra of several aliphatic aldehydes and ketones at 300 K, Atmos. Environ., 1992, 26, 785–792 CrossRef.
-
J. G. Calvert and J. N. Pitts, Photochemistry, John Wiley & Sons Inc., New York, NY, 1966 Search PubMed.
-
B. J. Finlayson-Pitts and J. N. Pitts, Chemistry of the upper and lower atmosphere, Academic Press, San Diego, 2000 Search PubMed.
- H. C. Hsieh and W. C. Ermler, Ab initio vibrational transition dipole moments and intensities of formaldehyde, J. Comput. Chem., 1988, 9, 95–106 CrossRef CAS.
- T. Nakanaga, S. Kondo and S. Saëki, Infrared band intensities of formaldehyde and formaldehyde-d2, J. Chem. Phys., 1982, 76, 3860–3865 CrossRef CAS.
-
R. Brückner, Advanced Organic Chemistry - Reaction Mechanisms, Harcourt/Academic Press, San Diego, CA, 2002 Search PubMed.
- A. Vairavamurthy, J. M. Roberts and L. Newman, Methods for determination of low molecular weight carbonyl compounds in the atmosphere: A review, Atmos. Environ., 1992, 26, 1965–1993 CrossRef.
- T. Nash, The colorimetric estimation of formaldehyde by means of the Hantzsch reaction, Biochem. J., 1953, 55, 416–421 CrossRef CAS PubMed.
- S. Belman, The fluorimetric determination of formaldehyde, Anal. Chim. Acta, 1963, 29, 120–126 CrossRef CAS.
- T. Salthammer, Photophysical properties of 3,5-diacetyl-1,4-dihydrolutidine in solution: application to the analysis of formaldehyde, J. Photochem. Photobiol., A, 1993, 74, 195–201 CrossRef CAS.
- S. Dong and P. K. Dasgupta, Fast fluorometric flow injection analysis of formaldehyde in atmospheric water, Environ. Sci. Technol., 1987, 21, 581–588 CrossRef CAS PubMed.
-
J. R. Lakowicz, Principles of fluorescence spectroscopy, Springer Science+Business Media LLC, New York, NY, 2006 Search PubMed.
-
EN 717-1, Wood-based panels - determination of formaldehyde release. Part 1: formaldehyde emission by the chamber method, Beuth Verlag, Berlin, 2005 Search PubMed.
- T. Kleindienst, P. Shepson, C. Nero, R. Arnts, S. Tejada, G. Mackay, L. Mayne, H. Schiff, J. Lind, G. Kok, A. Lazrus, P. Dasgupta and S. Dong, An intercomparison of formaldehyde measurement techniques at ambient concentration, Atmos. Environ., 1988, 22, 1931–1939 CrossRef CAS.
- M. Risholm-Sundman, A. Larsen, E. Vestin and A. Weibull, Formaldehyde emission - comparison of different standard methods, Atmos. Environ., 2007, 41, 3193–3202 CrossRef CAS.
- T. Salthammer and S. Mentese, Comparison of analytical techniques for the determination of aldehydes in test chambers, Chemosphere, 2008, 73, 1351–1356 CrossRef CAS PubMed.
-
E. Roffael, Formaldehyde in nature, in wood and in wood-based panels, DRW-Verlag, Leinfelden-Echterdingen, 2017 Search PubMed.
- S. Dugheri, D. Massi, N. Mucci, G. Marrubini, G. Cappelli, A. Speltini, M. C. Bonferoni and G. Arcangeli, Exposure to airborne formaldehyde: Sampling and analytical methods - A review, Trends Environ. Anal. Chem., 2021, 29, e00116 CrossRef CAS.
- C. Hak,
et al., Intercomparison of four different in situ techniques for ambient formaldehyde measurements in urban air, Atmos. Chem. Phys., 2005, 5, 2881–2900 CrossRef CAS.
- U. Riess, U. Tegtbur, C. Fauck, F. Fuhrmann, D. Markewitz and T. Salthammer, Experimental setup and analytical methods for the non-invasive determination of volatile organic compounds, formaldehyde and NO in exhaled human breath, Anal. Chim. Acta, 2010, 669, 53–62 CrossRef CAS PubMed.
- N. Aldag, J. Gunschera and T. Salthammer, Release and absorption of formaldehyde by textiles, Cellulose, 2017, 24, 4509–4518 CrossRef CAS.
- E. Sawicki, T. R. Hauser, T. W. Stanley and W. Elbert, The 3-methyl-2-benzothiazolone hydrazone test. sensitive new methods for the detection, rapid estimation, and determination of aliphatic aldehydes, Anal. Chem., 1961, 33, 93–96 CrossRef CAS.
- A. P. Altshuller and L. J. Leng, Application of the 3-methyl-2-benzothiazolone hydrazone method for atmospheric analysis of aliphatic aldehydes, Anal. Chem., 1963, 35, 1541–1542 CrossRef CAS.
- R. Goebel, A. Krug and R. Kellner, Spectrophotometric flow injection analysis of formaldehyde in aqueous solutions using 3-methyl-2-benzthiazolinone hydrazone, Fresenius’ J. Anal. Chem., 1993, 347, 491–494 CrossRef CAS.
- K. Toda, K.-I. Yoshioka, K. Mori and S. Hirata, Portable system for near-real time measurement of gaseous formaldehyde by means of parallel scrubber stopped-flow absorptiometry, Anal. Chim. Acta, 2005, 531, 41–49 CrossRef CAS.
- S. Huang, W. Wei, L. B. Weschler, T. Salthammer, H. Kan, Z. Bu and Y. Zhang, Indoor formaldehyde concentrations in urban China: Preliminary study of some important influencing factors, Sci. Total Environ., 2017, 590–591, 394–405 CrossRef CAS PubMed.
- W. H. Chan, S. Shuang and M. M. F. Choi, Determination of airborne formaldehyde by active sampling on 3-methyl-2-benzothiazolinone hydrazone hydrochloride-coated glass fibre filters, Analyst, 2001, 126, 720–723 RSC.
- R. Giesen, T. Schripp, D. Markewitz, B. Meyer, H. Schwab, E. Uhde and T. Salthammer, Comparison of methods for the determination of formaldehyde in air, Anal. Lett., 2016, 49, 1613–1621 CrossRef CAS.
-
R. Feldbrügge, K. P. Rindt and A. Borchert, in Advances in Molecular and Cell Biology, ed. E. E. Bittar, B. Danielsson and L. Bülow, JAI Press Inc., Greenwich, CT, 1996, vol. 15B, pp. 451–459 Search PubMed.
- A. Monkawa, T. Gessei, Y. Takimoto, N. Jo, T. Wada and N. Sanari, Highly sensitive and rapid gas biosensor for formaldehyde based on an enzymatic cycling system, Sens. Actuators, B, 2015, 210, 241–247 CrossRef CAS.
-
EN 16516, Construction products: Assessment of release of dangerous substances – Determination of emissions into indoor air, European Committee for Standardization, Brussels, 2017 Search PubMed.
- M. Aiello and R. McLaren, Measurement of airborne carbonyls using an automated sampling and analysis system, Environ. Sci. Technol., 2009, 43, 8901–8907 CrossRef CAS PubMed.
- U. Karst, N. Binding, K. Cammann and U. Witting, Interferences of nitrogen dioxide in the determination of aldehydes and ketones by sampling on 2,4-dinitrophenylhydrazine-coated solid sorbent, Fresenius’ J. Anal. Chem., 1993, 345, 48–52 CrossRef CAS.
- R. R. Arnts and S. B. Tejada, 2,4-Dinitrophenylhydrazine-coated silica gel cartridge method for determination of formaldehyde in air: identification of an ozone interference, Environ. Sci. Technol., 1989, 23, 1428–1430 CrossRef CAS.
- J. Williams, H. Li, A. B. Ross and S. P. Hargreaves, Quantification of the influence of NO2, NO and CO gases on the determination of formaldehyde and acetaldehyde using the DNPH method as applied to polluted environments, Atmos. Environ., 2019, 218, 117019 CrossRef CAS.
-
V. G. Berezkin and Y. S. Drugov, Gas chromatography in air pollution analysis, Elsevier Science Publishers B.V., Amsterdam, 1991 Search PubMed.
- S. S. H. Ho, K. Ho, W. Liu, S. Lee, W. Dai, J. Cao and H. Ip, Unsuitability of using the DNPH-coated solid sorbent cartridge for determination of airborne unsaturated carbonyls, Atmos. Environ., 2011, 45, 261–265 CrossRef CAS.
- S. S. H. Ho, H. S. S. Ip, K. F. Ho, L. P. T. Ng, W. Dai, J. Cao, C. S. Chan and L. B. Ho, Evaluation of hazardous airborne carbonyls on a university campus in southern China, J. Air Waste Manage. Assoc., 2014, 64, 903–916 CrossRef CAS PubMed.
- F. Villanueva, I. Colmenar, R. Mabilia, C. Scipioni and B. Cabañas, Field evaluation of the Analyst® passive sampler for the determination of formaldehyde and acetaldehyde in indoor and outdoor ambient air, Anal. Methods, 2013, 5, 516–524 RSC.
- Y. Chi, Y. Feng, S. Wen, H. Lü, Z. Yu, W. Zhang, G. Sheng and J. Fu, Determination of carbonyl compounds in the atmosphere by DNPH derivatization and LC-ESI-MS/MS detection, Talanta, 2007, 72, 539–545 CrossRef CAS PubMed.
- Y.-L. Feng and J. Zhu, Separation and determination of carbonyl compounds in indoor air using two-step gradient capillary electrochromatography, Anal. Sci., 2004, 20, 1691–1695 CrossRef CAS PubMed.
- X. Zhang, Y. Kong, J. Cao, H. Li, R. Gao, Y. Zhang, K. Wang, Y. Li, Y. Ren and W. Wang, A sensitive simultaneous detection approach for the determination of 30 atmospheric carbonyls by 2,4-dinitrophenylhydrazine derivatization with HPLC-MS technique and its preliminary application, Chemosphere, 2022, 303, 134985 CrossRef CAS PubMed.
-
H.-J. Hübschmann, Handbook of GC/MS: Fundamentals and Applications, WILEY-VCH, Weinheim, 2015 Search PubMed.
-
F. W. McLafferty and F. Turecek, Interpretation of mass spectra, University Science Books, Mill Valley, CA, 1993 Search PubMed.
- O. Wilke, W. Horn, M. Richter and O. Jann, Volatile organic compounds from building products - results from six round robin tests with emission test chambers conducted between 2008 and 2018, Indoor Air, 2021, 31, 2049–2057 CrossRef CAS PubMed.
- T. Salthammer, TVOC-Revisited, Environ. Int., 2022, 167, 107440 CrossRef CAS PubMed.
- E. Woolfenden, Sorbent-based sampling methods for volatile and semi-volatile organic compounds in air, J. Chromatogr. A, 2010, 1217, 2674–2684 CrossRef CAS PubMed.
- P. A. Clausen and P. Wolkoff, Degradation products of Tenax TA formed during sampling and thermal desorption analysis: Indicators of reactive species indoors, Atmos. Environ., 1997, 31, 715–725 CrossRef CAS.
- J. G. Klenø, P. Wolkoff, P. A. Clausen, C. K. Wilkins and T. Pedersen, Degradation of the adsorbent Tenax TA by nitrogen oxides, ozone, hydrogen peroxide, OH radical, and limonene oxidation products, Environ. Sci. Technol., 2002, 36, 4121–4126 CrossRef PubMed.
- M. Richter, E. Juritsch and O. Jann, Determination of recovery rates of adsorbents for sampling very volatile organic compounds (C1-C6) in dry and humid air in the sub-ppb range by use of thermal desorption gas chromatography-mass spectrometry, J. Chromatogr. A, 2020, 1626, 461389 CrossRef CAS PubMed.
- M. Even, E. Juritsch and M. Richter, Measurement of very volatile organic compounds (VVOCs) in indoor air by sorbent-based active sampling: Identifying the gaps towards standardisation, TrAC, Trends Anal. Chem., 2021, 140, 116265 CrossRef CAS.
- R. Schulte-Ladbeck, R. Lindahl, J.-O. Levin and U. Karst, Characterization of chemical interferences in the determination of unsaturated aldehydes using aromatic hydrazine reagents and liquid chromatography, J. Environ. Monit., 2001, 3, 306–310 RSC.
-
U.S. Environmental Protection Agency, Method TO-15A: Determination of volatile organic compounds (VOCs) in air collected in specially prepared canisters and analyzed by gas chromatography-mass spectrometry (GC-MS), U.S. EPA Office of Research and Development, Washington, D.C., 2019 Search PubMed.
- E. Uhde and T. Salthammer, Impact of reaction products from building materials and furnishings on indoor air quality - a review of recent advances in indoor chemistry, Atmos. Environ., 2007, 41, 3111–3128 CrossRef CAS.
- C. D. Forester and J. R. Wells, Yields of carbonyl products from gas-phase reactions of fragrance compounds with OH radical and ozone, Environ. Sci. Technol., 2009, 43, 3561–3568 CrossRef CAS PubMed.
- J. Wells and J. E. Ham, A new agent for derivatizing carbonyl species used to investigate limonene ozonolysis, Atmos. Environ., 2014, 99, 519–526 CrossRef CAS PubMed.
- J. Herrington, L. Zhang, D. Whitaker, L. Sheldon and J. J. Zhang, Optimizing a dansylhydrazine (DNSH) based method for measuring airborne acrolein and other unsaturated carbonyls, J. Environ. Monit., 2005, 7, 969–976 RSC.
- S. S. H. Ho and J. Z. Yu, Determination of airborne carbonyls: comparison of a thermal desorption/GC method with the standard DNPH/HPLC method, Environ. Sci. Technol., 2004, 38, 862–870 CrossRef CAS PubMed.
- J. Li, Y. L. Feng, C. J. Xie, J. Huang, J. Z. Yu, J. L. Feng, G. Y. Sheng, J. M. Fu and M. H. Wu, Determination of gaseous carbonyl compounds by their pentafluorophenyl hydrazones with gas chromatography/mass spectrometry, Anal. Chim. Acta, 2009, 635, 84–93 CrossRef CAS PubMed.
- X. Pang, A. C. Lewis and J. F. Hamilton, Determination of airborne carbonyls via pentafluorophenylhydrazine derivatisation by GC-MS and its comparison with HPLC method, Talanta, 2011, 85, 406–414 CrossRef CAS PubMed.
- M. A. Ullah, K.-H. Kim and J. E. Szulejko, The effect of solvent selection in the gas chromatographic analysis of carbonyls in air samples after derivatization with pentafluorophenyl hydrazine, Atmos. Res., 2015, 166, 101–109 CrossRef CAS.
- D. Bourdin and V. Desauziers, Development of SPME on-fiber derivatization for the sampling of formaldehyde and other carbonyl compounds in indoor air, Anal. Bioanal. Chem., 2014, 406, 317–328 CrossRef CAS PubMed.
- H. Lan, K. Hartonen and M.-L. Riekkola, Miniaturised air sampling techniques for analysis of volatile organic compounds in air, TrAC, Trends Anal. Chem., 2020, 126, 115873 CrossRef CAS.
- M. Glowania, F. Rohrer, H.-P. Dorn, A. Hofzumahaus, F. Holland, A. Kiendler-Scharr, A. Wahner and H. Fuchs, Comparison of formaldehyde measurements by Hantzsch, CRDS and DOAS in the SAPHIR chamber, Atmos. Meas. Tech., 2021, 14, 4239–4253 CrossRef CAS.
- A. Wisthaler, E. C. Apel, J. Bossmeyer, A. Hansel, W. Junkermann, R. Koppmann, R. Meier, K. Müller, S. J. Solomon, R. Steinbrecher, R. Tillmann and T. Brauers, Technical Note: Intercomparison of formaldehyde measurements at the atmosphere simulation chamber SAPHIR, Atmos. Chem. Phys., 2008, 8, 2189–2200 CrossRef CAS.
- B. Hanoune, T. LeBris, L. Allou, C. Marchand and S. L. Calvé, Formaldehyde measurements in libraries: Comparison between infrared diode laser spectroscopy and a DNPH-derivatization method, Atmos. Environ., 2006, 40, 5768–5775 CrossRef CAS.
-
K. H. Michaelian, Photoacoustic IR Spectroscopy, WILEY-VCH, Weinheim, 2010 Search PubMed.
- C. B. Hirschmann, J. Lehtinen, J. Uotila, S. Ojala and R. L. Keiski, Sub-ppb detection of formaldehyde with cantilever enhanced photoacoustic spectroscopy using quantum cascade laser source, Appl. Phys. B, 2013, 111, 603–610 CrossRef CAS.
- A. Gratien, B. Picquet-Varrault, J. Orphal, E. Perraudin, J.-F. Doussin and J.-M. Flaud, Laboratory intercomparison of the formaldehyde absorption cross sections in the infrared (1660–1820 cm−1 and ultraviolet (300–360 nm) spectral regions, J. Geophys. Res., 2007, 112, D05305 CrossRef.
-
EN 16738, Emission safety of combustible air fresheners – Test methods, European Commission for Standardization, Brussels, 2015 Search PubMed.
- T. Salthammer, J. Gu, S. Wientzek, R. Harrington and S. Thomann, Measurement and evaluation of gaseous and particulate emissions from burning scented and unscented candles, Environ. Int., 2021, 155, 106590 CrossRef CAS PubMed.
-
ISO 12460-3, Wood-based panels - Determination of formaldehyde release. Part 3: gas analysis method, International Organization for Standardization, Geneva, 2020 Search PubMed.
-
A. M. Ellis and C. A. Mayhew, Proton Transfer Reaction Mass Spectrometry: Principles and Applications, John Wiley & Sons, Chichester, 2013 Search PubMed.
- R. S. Blake, P. S. Monks and A. M. Ellis, Proton-transfer reaction mass spectrometry, Chem. Rev., 2009, 109, 861–896 CrossRef CAS PubMed.
- D. M. Lunderberg, P. K. Misztal, Y. Liu, C. Arata, Y. Tian, K. Kristensen, R. J. Weber, W. W. Nazaroff and A. H. Goldstein, High-resolution exposure assessment for volatile organic compounds in two California residences, Environ. Sci. Technol., 2021, 55, 6740–6751 CrossRef CAS PubMed.
- Y. Liu, P. K. Misztal, C. Arata, C. J. Weschler, W. W. Nazaroff and A. H. Goldstein, Observing ozone chemistry in an occupied residence, Proc. Natl. Acad. Sci. U. S. A., 2021, 118, e2018140118 CrossRef CAS PubMed.
- N. Zannoni, P. S. J. Lakey, Y. Won, M. Shiraiwa, D. Rim, C. J. Weschler, N. Wang, L. Ernle, M. Li, G. Bekö, P. Wargocki and J. Williams, The human oxidation field, Science, 2022, 377, 1071–1077 CrossRef CAS PubMed.
- N. Wang, L. Ernle, G. Bekö, P. Wargocki and J. Williams, Emission rates of volatile organic compounds from humans, Environ. Sci. Technol., 2022, 56, 4838–4848 CrossRef CAS PubMed.
- D. Smith and P. Španěl, Selected ion flow tube mass spectrometry (SIFT-MS) for on-line trace gas analysis, Mass Spectrom. Rev., 2005, 24, 661–700 CrossRef CAS PubMed.
- T. Schripp, S. Etienne, C. Fauck, F. Fuhrmann, L. Märk and T. Salthammer, Application of proton-transfer-reaction-mass-spectrometry for indoor air quality research, Indoor Air, 2014, 24, 178–189 CrossRef CAS PubMed.
- E. P. L. Hunter and S. G. Lias, Evaluated gas phase basicities and proton affinities of molecules: an update, J. Phys. Chem. Ref. Data, 1998, 27, 413–656 CrossRef CAS.
- A. Hansel, W. Singer, A. Wisthaler, M. Schwarzmann and W. Lindinger, Energy dependencies of the proton transfer reactions, Int. J. Mass Spectrom. Ion Processes, 1997, 167–168, 697–703 CrossRef.
- T. Schripp, C. Fauck and T. Salthammer, Interferences in the determination of formaldehyde via PTR-MS: What do we learn from m/z 31?, Int. J. Mass Spectrom., 2010, 289, 170–172 CrossRef CAS.
- P. Španěl, Y. Ji and D. Smith, SIFT studies of the reactions of H3O+, NO+ and O2+ with a series of aldehydes and ketones, Int. J. Mass Spectrom. Ion Processes, 1997, 165–166, 25–37 CrossRef.
- C. Warneke, J. A. de Gouw, W. C. Kuster, P. D. Goldan and R. Fall, Validation of atmospheric VOC measurements by proton-transfer- reaction mass spectrometry using a gas-chromatographic preseparation method, Environ. Sci. Technol., 2003, 37, 2494–2501 CrossRef CAS PubMed.
- D. Pagonis, K. Sekimoto and J. de Gouw, A library of proton-transfer reactions of H3O+ ions used for trace gas detection, J. Am. Soc. Mass Spectrom., 2019, 30, 1330–1335 CrossRef CAS PubMed.
-
J. W. Einax, H. W. Zwanzinger and S. Geiß, Chemometrics in Environmental Analysis, VCH Verlag, Weinheim, 1997 Search PubMed.
- L. Cappellin, T. Karl, M. Probst, O. Ismailova, P. M. Winkler, C. Soukoulis, E. Aprea, T. D. Märk, F. Gasperi and F. Biasioli, On quantitative determination of volatile organic compound concentrations using proton transfer reaction time-of-flight mass spectrometry, Environ. Sci. Technol., 2012, 46, 2283–2290 CrossRef CAS PubMed.
- T. Su, Parametrization of kinetic energy dependences of ion–polar molecule collision rate constants by trajectory calculations, J. Chem. Phys., 1994, 100, 4703–4703 CrossRef CAS.
- T. Salthammer, U. Hohm, M. Stahn and S. Grimme, Proton-transfer rate constants for the determination of organic indoor air pollutants by online
mass spectrometry, RSC Adv., 2023, 13, 17856–17868 RSC.
-
R. Barth, The chemistry of beer, John Wiley & Sons Inc., Hoboken, NJ, 2022 Search PubMed.
-
G. A. Eiceman, Z. Karpas and H. H. Hill Jr., Ion mobility spectrometry, CRC Press, Boca Raton, FL, 2014 Search PubMed.
- R. Cumeras, E. Figueras, C. E. Davis, J. I. Baumbach and I. Gràcia, Review on Ion Mobility Spectrometry. Part 1: current instrumentation, Analyst, 2015, 140, 1376–1390 RSC.
- R. Cumeras, E. Figueras, C. E. Davis, J. I. Baumbach and I. Gràcia, Review on Ion Mobility Spectrometry. Part 2: hyphenated methods and effects of experimental parameters, Analyst, 2015, 140, 1391–1410 RSC.
- C. Tiebe, H. Miessner, B. Koch and T. Hübert, Detection of microbial volatile organic compounds (MVOCs) by ion-mobility spectrometry, Anal. Bioanal. Chem., 2009, 395, 2313–2323 CrossRef CAS PubMed.
- V. Ruzsanyi, P. Mochalski, A. Schmid, H. Wiesenhofer, M. Klieber, H. Hinterhuber and A. Amann, Ion mobility spectrometry for detection of skin volatiles, J. Chromatogr. B: Anal. Technol. Biomed. Life Sci., 2012, 911, 84–92 CrossRef CAS PubMed.
- P. C. Moura and V. Vassilenko, Gas Chromatography – Ion Mobility Spectrometry as a tool for quick detection of hazardous volatile organic compounds in indoor and ambient air: A university campus case study, Eur. J. Mass Spectrom., 2022, 28, 113–126 CrossRef CAS PubMed.
- J. E. Szulejko and K.-H. Kim, Derivatization techniques for determination of carbonyls in air, TrAC, Trends Anal. Chem., 2015, 64, 29–41 CrossRef CAS.
- E. Pibiri, A. Omelan, E. Uhde and T. Salthammer, Effect of surface covering on the release of formaldehyde, acetaldehyde, formic acid and acetic acid from particleboard, Build. Environ., 2020, 178, 106947 CrossRef.
- O. Toyinbo, L. Hägerhed, S. Dimitroulopoulou, M. Dudzinska, S. Emmerich, D. Hemming, J.-H. Park and U. Haverinen-Shaughnessy, Open database for international and national indoor environmental quality guidelines, Indoor Air, 2022, 32, e13028 CrossRef PubMed.
-
ASTM D5197-21, Standard Test Method for Determination of Formaldehyde and Other Carbonyl Compounds in Air (Active Sampler Methodology), American Society for Testing and Materials, West Conshohocken, PA, 2022 Search PubMed.
- B. Yuan, A. R. Koss, C. Warneke, M. Coggon, K. Sekimoto and J. A. de Gouw, Proton-transfer-reaction mass spectrometry: applications in atmospheric sciences, Chem. Rev., 2017, 117, 13187–13229 CrossRef CAS PubMed.
- K. Buhr, S. van Ruth and C. Delahunty, Analysis of volatile flavour compounds by Proton Transfer Reaction-Mass Spectrometry: fragmentation patterns and discrimination between isobaric and isomeric compounds, Int. J. Mass Spectrom., 2002, 221, 1–7 CrossRef CAS.
- Y. L. Pham, W. Wojnowski and J. Beauchamp, Online volatile compound emissions analysis using a microchamber/thermal extractor coupled to proton transfer reaction-mass spectrometry, Anal. Chem., 2022, 94, 17354–17359 CrossRef CAS PubMed.
- D. Pagonis, D. J. Price, L. B. Algrim, D. A. Day, A. V. Handschy, H. Stark, S. L. Miller, J. de Gouw, J. L. Jimenez and P. J. Ziemann, Time-resolved measurements of indoor chemical emissions, deposition, and reactions in a university art museum, Environ. Sci. Technol., 2019, 53, 4794–4802 CrossRef CAS PubMed.
- C. J. Weschler and W. W. Nazaroff, Human skin oil: a major ozone reactant indoors, Environ. Sci.: Atmos., 2023, 3, 640–661 CAS.
- N. Beauval, M. Verrièle, A. Garat, I. Fronval, R. Dusautoir, S. Anthérieu, G. Garçon, J.-M. Lo-Guidice, D. Allorge and N. Locoge, Influence of puffing conditions on the carbonyl composition of e-cigarette aerosols, Int. J. Hyg. Environ. Health, 2019, 222, 136–146 CrossRef CAS PubMed.
|
This journal is © The Royal Society of Chemistry 2023 |
Click here to see how this site uses Cookies. View our privacy policy here.