DOI:
10.1039/D1TB01817E
(Paper)
J. Mater. Chem. B, 2022,
10, 2561-2569
Induction of mesenchymal stem cell differentiation by co-culturing with mature cells in double-layered 2-methacryloyloxyethyl phosphorylcholine polymer hydrogel matrices†
Received
20th August 2021
, Accepted 25th November 2021
First published on 25th November 2021
Abstract
The effects of differentiated cells on stem cell differentiation were analyzed via co-culturing using a cell-encapsulated double-layered hydrogel system. As a polymer hydrogel matrix, a water-soluble zwitterionic polymer having both a 2-methacryloyloxyethyl phosphorylcholine unit and a p-vinylphenylboronic acid unit (PMBV), was complexed spontaneously with poly(vinyl alcohol) (PVA) under mild cell culture conditions. The creep modulus of the hydrogel was controlled by changing the composition of the polymer in the solution. Mouse mesenchymal stem cells (MSCs), C3H10T1/2 cells, were encapsulated into PMBV/PVA hydrogels and cultured. In the PMBV/PVA hydrogel with a lower creep modulus (0.40 kPa), proliferation of C3H10T1/2 cells occurred, and the formation of cell aggregates was observed. On the other hand, a higher creep modulus (1.7 kPa) of the hydrogel matrix prevented cell proliferation. Culturing C3H10T1/2 cells encapsulated in the PMBV/PVA hydrogel in the presence of bone morphogenetic protein-2 increased the activity of intracellular alkaline phosphatase (ALP). This indicated that C3H10T1/2 cells differentiated into mature osteoblasts. When the C3H10T1/2 cells encapsulated in the PMBV/PVA hydrogel were cultured in combination with the mature osteoblasts in the hydrogel by a close contacting double-layered hydrogel structure, higher ALP activity was observed compared with the cells cultured separately. It was considered that the differentiation of C3H10T1/2 cells in the hydrogel layer was induced by cytokines diffused from mature osteoblasts encapsulated in another hydrogel layer. It could be concluded that the PMBV/PVA hydrogel system provides a good way to observe the effects of the surrounding cells on cell function in three-dimensional culture.
1. Introduction
In stem cell-based regenerative medicine, it is necessary to culture stem cells in three dimensions (3Ds) to produce cell aggregates and induce differentiation.1–5 Cells may communicate with each other via various bioactive molecules, such as cytokines. Cell aggregates are beneficial for the diffusion of these bioactive molecules because the cells are in close contact within the aggregate.6–8 Therefore, cell–cell interactions are stronger. Thus, it is necessary to understand the effects of the co-culture of cell aggregates on differentiation.9,10 Further control of stem cell differentiation can be achieved. Cell proliferation and differentiation depend on the surrounding circumstances. When cell culture is carried out in 3Ds, suitable polymer hydrogel matrices are required. Recently, polymer hydrogels bearing zwitterionic polar groups have been studied as cell culture substrates.11–17 In our previous research, a zwitterionic polymer hydrogel system for cell encapsulation and culturing has been examined.11 That is, water-soluble poly[2-methacryloyloxyethyl phosphorylcholine (MPC)-co-n-butyl methacrylate (BMA)-co-p-vinylphenyl boronic acid (VPBA)] (PMBV) was mixed with poly(vinyl alcohol) (PVA) in an aqueous medium, and a hydrogel (PMBV/PVA hydrogel) was spontaneously formed by reversible covalent bond formation between the VPBA units and hydroxyl groups in PVA.11,18,19 The hydrogel encapsulated mesenchymal stem cells (MSCs) under mild conditions. Cell proliferation can be regulated by the elastic modulus of the PMBV/PVA hydrogel matrix.20 The PMBV/PVA hydrogel was dissociated by the addition of a low-molecular-weight sugar.21 The encapsulated cells were easily recovered. Another important property of the PMBV/PVA hydrogel is the self-adhesion and formation of a layer-by-layer structure.22,23 It is known that the states of two cells cooperate with each other in a cell co-culture system.24
In this study, by adhering two PMBV/PVA hydrogel layers encapsulated in different states of the cells (stem cells and mature cells), we analyzed the effects of mature cells on stem cell differentiation. This is important to clarify the characteristics of the cell-growth environment when stem cells are used as a cell source.
2. Experimental
2.1 Materials and reagents
MPC was purchased from NOF Co., Tokyo, Japan, which was synthesized using a reported procedure.25,26 BMA was obtained from FUJI FILM Wako Pure Chemical Co., Ltd (Osaka, Japan). VPBA and 2,2′-azobisisobutyronitrile (AIBN) were obtained from Kanto Chemical Co., Inc. (Tokyo, Japan). PVA (NH-18S, polymerization degree of 2100) was obtained from Nippon Synthetic Chemical Industry Co., Ltd (Tokyo, Japan). A water-insoluble poly(MPC-co-BMA) (Lipidure-PMB) was purchased from NOF Co.26 Human recombinant bone morphogenetic protein-2 (BMP-2) was purchased from R&D Systems, Inc. (Minneapolis, MN, USA). Dulbecco's modified Eagle's medium (DMEM), fetal bovine serum (FBS), and phosphate-buffered saline (PBS) were purchased from Thermo Fisher Scientific (Waltham, MA, USA). Dulbecco's modified Eagle's medium (DMEM) containing 10% FBS and 1.0% penicillin was prepared. Trypsin (0.05% solution) containing 1 mmol L−1N,N,N′,N′-ethylenediamine tetraacetic acid (EDTA) was purchased from Invitrogen (Tokyo, Japan). C3H10T1/2 cells were purchased from RIKEN BRC through the National Bio-Resource Project of MEXT, Japan. Other reagents and solvents were commercially available extra-pure grade reagents and were used without further purification.
2.2 Synthesis of PMBV
Free-radical polymerization was used to synthesize PMBV.18,20 All monomers were dissolved in ethanol. AIBN was added to the solution as an initiator in the polymerization glass tubing. The concentrations of the monomer and initiator were [monomer] = 0.50 mol L−1 and [AIBN] = 5.0 mmol L−1. After bubbling with argon gas for 15 min, the glass tubing was sealed, and polymerization was carried out at 60 °C for 6 h. The tubing was opened, and the reaction solution was added dropwise to a diethyl ether/chloroform (80/20 (v/v)) mixture with a 15 time larger volume to reprecipitate the polymer. After standing for 1 h, the mixture was washed again with the same solvent. The precipitated polymer was filtered through a glass filter, placed in a desiccator, and dried under reduced pressure overnight. The polymer was then dissolved in water and dialyzed against a large amount of water for three days using a Spectra/Por® dialysis membrane (molecular weight cutoff: 3500; Funakoshi Co., Ltd, Tokyo, Japan) at room temperature (approximately 25 °C). Finally, the polymer solution was lyophilized overnight to obtain the polymer powder.
2.3 Characterization of PMBV
The composition of each monomer unit in the polymer was analyzed by nuclear magnetic resonance spectroscopy (1H-NMR, α-400, JEOL Co., Tokyo, Japan). Ethanol-d6 was used as the deuterated solvent. The number of integrations was 32 for polymer characterization. The representative 1H-NMR spectrum is shown in Fig. S1 (ESI†).
Fourier-transform infrared spectroscopy (FT-IR, FT-IR 6300, Jasco Co., Ltd, Tokyo, Japan) was used to identify the functional groups in the polymer. The resolution was 4.0 cm−1, while the number of integrations was 64. A representative FT-IR spectrum is presented in Fig. S2 (ESI†).
The molecular weights of the polymers were determined by gel permeation chromatography (GPC, JASCO Co., Ltd, Tokyo, Japan) equipped with an SB-804HQ column (Shodex, Tokyo, Japan). A methanol/water mixed solution of 70/30 (v/v) with LiBr (10 mmol L−1) was used as the eluent. The measurements were performed at a flow rate of 0.50 mL min−1 at 25 °C. Poly(ethylene glycol) (TSK standard PEG, Tosoh Co., Tokyo, Japan) was used as a standard sample to calculate the weight-averaged molecular weight (Mw) and number-average molecular weight (Mn). The molecular dispersity (Mw/Mn) was then calculated.
Synthetic results of the PMBV are summarized in Table 1.
Table 1 Synthetic results of PMB
Polymer |
Composition (MPC/BMA/VPBA)a |
Yield (%)b |
Molecular weight (kDa)c |
Dispersity |
Solubilityd |
In feed |
In polymer |
M
n
|
M
w
|
M
w/Mn |
In DMEM(+,+) |
Determined by 1H-NMR spectroscopy in ethanol-d6 in 25 °C.
[monomer] = 0.5 mol L−1, [AIBN] = 5 mmol L−1 at 60 °C for 15 h.
Determined by GPC in 70 vol% methanol aqueous solution containing 10 mmol L−1 LiBr at 25 °C.
Evaluated in DMEM containing 10% FBS and 1.0% penicillin (DMEM(+,+)) at 25 °C. [Polymer] = 1.0 mg mL−1.
|
PMBV-1 |
0.60/0.30/0.10 |
0.73/0.21/0.06 |
56 |
13 |
25 |
2.5 |
++ |
PMBV-2 |
0.60/0.20/0.20 |
0.81/0.07/0.12 |
66 |
7.1 |
15 |
2.1 |
++ |
PMBV-3 |
0.80/0.10/0.10 |
0.93/0/04/0.03 |
65 |
12 |
42 |
3.5 |
++ |
2.4 Preparation of PMBV/PVA hydrogels
PMBV was dissolved in DMEM and sterilized through a filter with a filter pore size of 0.45 μm. PVA was initially dissolved in PBS. The desired concentration of PVA was obtained by adding DMEM to the PVA solution. The PMBV/PVA hydrogel was prepared by mixing 1.5 mL of a 5.0 wt% PMBV solution in which PMBV was dissolved in DMEM and 1.5 mL of a 2.5 wt% PVA solution and pipetting several times. Since the gelation process is based on the formation of molecular complexes based on the chemical equilibrium between PMBV and PVA, the formed hydrogel was allowed stand overnight to reach an equilibrium state.18 It was then transferred to a nylon cell strainer (pore diameter: 40 μm) (Corning Brand, FALCON, NY, USA). The swelling ratio of the PMBV/PVA hydrogel was measured in a 6-well cell-culture plate prefilled with DMEM (5.0 mL) in the wells and immersed in an excess amount of DMEM. The swelling ratio was measured continuously over 14 days. Assuming that the mass of the hydrogel before immersion is W0 and that the mass of the hydrogel at time t is Wt, the swelling ratio is expressed as follows:
2.5 Evaluation of the rheological properties of PMBV/PVA hydrogels
In this study, the creep modulus, which represents static viscoelasticity, was measured and used to evaluate the strength of the hydrogel. A creep meter (RE2-33005B; Yamaden Co., Ltd, Tokyo, Japan) was used to measure the rheological properties of the hydrogels.20 One mL of the PMBV/PVA hydrogel was prepared in a 24-well cell-culture plate coated with the water-insoluble poly(MPC-co-BMA) (Lipidure-PMB, NOF Co.) with a fraction of MPC units of 0.30 in the polymer. A 2.0-N load cell was used for the measurement with the creep meter. The entire test lasted for 120 s. For the first 60 s, the hydrogel was compressed with a load of 0.10 N using a contact sensor with a diameter of 8 mm, then recovered for the next 60 s. The program step and speed were set to 0.010 mm s−1 and 0.50 mm s−1, respectively. For the creep chart, the creep modulus of the PMBV/PVA hydrogel was calculated using a 6-parameter model.27
A PMBV solution (5.0, 6.0, 8.0, and 10 wt%) and PVA solution (2.5, 3.0, 4.0, and 5.0 wt%) were prepared in DMEM. A PMBV/PVA hydrogel was prepared by mixing 0.50 mL of the PMBV solution and 0.50 mL of the PVA solution. The mixing ratios of the PMBV and PVA solutions were fixed at PMBV/PVA = 2/1 (v/v). The concentration of PMBV in the polymer hydrogel was 2.5 wt%–5.0 wt%. The change in the creep modulus of the PMBV/PVA hydrogel when the concentration of PMBV in the gel was increased was measured using a creep meter.
2.6 Adhesion between the PMBV/PVA hydrogels
A two-step preparation process was carried out to ensure the adhesion of the hydrogel layers. The PMBV/PVA hydrogel (2.0 mL) was prepared by mixing 1.0 mL of the 5.0 wt% PMBV solution and 1.0 mL of the 2.5 wt% PVA solution in a cell-culture dish with a diameter of 35 mm. After the entire solution was gently stirred to form a hydrogel, it was then allowed to stand overnight to equilibrate the gelation reaction. Subsequently, 1.0 mL of the 5.0 wt% PMBV solution and 1.0 mL of the 2.5 wt% PVA solution were mixed, and one drop of a trypan blue solution was added to prepare the solution. The concentrations of PMBV and PVA in the hydrogel were 2.5 wt% and 1.25 wt%, respectively. The solution was placed on top of the first hydrogel layer to prepare a double-layered PMBV/PVA hydrogel with a stained layer. It was left for a few days. An adhesion state between the PMBV/PVA hydrogel layers was observed.
The change in the creep modulus of the PMBV/PVA hydrogel upon adhesion was evaluated. As the first hydrogel layer, the PMBV/PVA hydrogel was prepared by mixing 0.50 mL of a 10 wt% PMBV solution and 0.50 mL of a 5.0 wt% PVA solution in a 24-well cell culture plate. As the second PMBV/PVA hydrogel, 0.50 mL of a 5.0 wt% PMBV solution and 0.50 mL of a 2.5 wt% PVA solution were mixed together. The hydrogels were allowed to stand overnight. The second hydrogel was overlayered on the first hydrogel. These two hydrogels adhered to each other. The creep modulus of the PMBV/PVA hydrogel was measured using a creep meter.
2.7 Preparation of the cell suspension with C3H10T1/2 cells
Mouse MSCs, C3H10T1/2 cells, were used as model cells.11,20 C3H10T1/2 cells were cultured in a cell culture dish until a confluence of 90% was reached. After the adhered cells were washed with 6.0 mL of PBS in the dish, 2.0 mL of a 0.05% trypsin solution containing 1 mmol L−1 EDTA was added and incubated at 37 °C until cell detachment was complete. DMEM (4.0 mL) was added to stop trypsinization. The cells were suspended in DMEM and transferred to a conical tube. After centrifugation, the supernatant was removed, and DMEM was added to prepare the cell suspension and adjust the density of the cells in the suspension.
2.8 Evaluation of the cell aggregate growth
The PMBV/PVA hydrogels with different elastic moduli for culturing C3H10T1/2 cells were prepared by changing the concentration of PMBV solution and PVA solution in a 24-well cell culture plate. The surface of the cell culture plate was coated with the water-insoluble Lipidure-PMB to prevent the adhesion of cells to the surface of the dish.26,28
Encapsulation of C3H10T1/2 cells in the hydrogel was carried out as follows. 100 μL of cell suspension was mixed with 0.5 mL of 5.0 wt% PMBV solution, and then 0.5 mL of 2.5 wt% PVA solution was mixed. The mixed solution was gently pipetted several times to prepare the PMBV/PVA hydrogel for the encapsulation of cells. The total volume of the PMBV/PVA hydrogel was 1.1 mL and the density of cells in the hydrogel was adjusted at 5.0 × 105 cells per mL. This procedure could obtain the PMBV/PVA hydrogel with 0.40 kPa elastic modulus. On the other hand, to obtain a 1.7 kPa elastic modulus hydrogel, the same procedure was done using 6.0 wt% PMBV solution and 3.0 wt% PVA solution. C3H10T1/2 cells were 3D-cultured in these hydrogels under normal cell-culturing conditions. The cell culture in the PMBV/PVA hydrogel was carried out at 37 °C in a 5.0% CO2 atmosphere. Furthermore, in the PMBV/PVA hydrogel, in which the proliferation of C3H10T1/2 cells and formation of cell aggregates were confirmed, the diameter of the cell aggregates was measured for each culture day. The morphology of the encapsulated C3H10T1/2 cells was observed using a phase contrast microscope (BX60; Olympus Co., Ltd, Tokyo, Japan).
2.9 Measurement of the number of cells in a cell aggregate
The number of cells in the cell aggregates formed in the PMBV/PVA hydrogel was evaluated by comparison with cell aggregates prepared by the conventional hanging drop method.29,30 The cell aggregates were encapsulated in the PMBV/PVA hydrogel and cultured, and then the radius of the cell aggregate was measured. The procedure used in the hanging drop method is as follows (see Fig. S3, ESI†). The cells were counted and the cell density was adjusted to 2.5 × 106 cells per mL. A cell culture dish with a diameter of 60 mm was obtained. PBS (5 mL) was placed at the bottom of the dish, which functioned as a hydration chamber. The lid was inverted, and 10 μL was added dropwise to the bottom of the lid. The lid was inverted in a bottom chamber filled with PBS. The cells were cultured at 37 °C in a 5.0% CO2 atmosphere for 24 h to prepare the cell aggregates. The cell aggregates were encapsulated on a PMBV/PVA hydrogel with a creep modulus of 0.40 kPa and cultured overnight. The hydrogel was prepared by mixing equal volumes of 5.0 wt% PMBV solution and 2.5 wt% PVA solution. The radii of the cell aggregates were measured.
2.10 Staining procedure of alkaline phosphatase in cells
BMP-2, an inducer of osteoblasts, was used to induce the differentiation of C3H10T1/2 cells into osteoblasts.31 The PMBV/PVA hydrogel for culturing C3H10T1/2 cells was prepared as follows. A 5.0 wt% PMBV solution and 2.5 wt% PVA solution were prepared, and then 100 μL of a DMEM suspension of C3H10T1/2 cells and 500 ng of BMP-2 were added to 0.50 mL of the PMBV solution and 0.50 mL of the PVA solution, respectively. To prepare a cell-encapsulated PMBV/PVA hydrogel with a total volume of 1.1 mL, the cell seeding density was adjusted to 5.0 × 105 cells per mL. By culturing the cells encapsulated in the PMBV/PVA hydrogel for 10 days, C3H10T1/2 cells were induced to differentiate into osteoblasts. The cells encapsulated in the PMBV/PVA hydrogel were collected using the following procedure. To dissolve 1.1 mL of the PMBV/PVA hydrogel, 13.0 mL of a 5.0 wt% D-sorbitol/PBS solution was used. The D-sorbitol/PBS solution was also sterilized using a 0.22 μm-pore filter unit (MF-Millipore™ Membrane, Merck Millipore Ltd, Ireland). The mixture of the D-sorbitol solution and hydrogel was shaken gently by pipetting every 15 min for up to 60 min until the hydrogel was completely dissolved. The cell suspension was centrifuged at 1000 rpm for 3.0 min to precipitate the cells. The cells were resuspended in DMEM, seeded on a conventional cell culture dish, and incubated for 24 h to adhere to the cells. After washing the cells three times with PBS, 10% formaldehyde was added and the cells were allowed to stand at room temperature (25 °C) for 20 min to fix the cells. After washing the cells with distilled water three times, the cells were stained with an alkaline phosphatase (ALP) staining kit (Cosmo Bio Co., Ltd, Tokyo, Japan). The stained cells were observed under an optical microscope.
2.11 Determination of the activity of ALP in cells
In a 24-well cell-culture dish coated with Lipidure-PMB, 100 μL of the DMEM suspension of C3H10T1/2 cells, 0.50 mL of the PMBV solution, and 0.50 mL of the PVA solution containing 500 ng of BMP-2 were mixed together to prepare a cell-encapsulated PMBV/PVA hydrogel with a total volume of 1.1 mL. To investigate the effect of the difference in the elastic modulus of the hydrogel on the differentiation of encapsulated cells, PMBV/PVA hydrogels with creep moduli of 0.40 and 1.7 kPa were prepared. That is, the hydrogels with a creep modulus of 0.40 kPa were prepared by mixing equal amounts of 5.0 wt% PMBV solution and 2.5 wt% PVA solution, and hydrogels with a creep modulus of 1.7 kPa were prepared by mixing equal amounts of 6.0 wt% PMBV solution and 3.0 wt% PVA solution. The cell seeding density was adjusted to 5.0 × 105 cells per mL. Cells were cultured in the PMBV/PVA hydrogel for 10 days under conventional cell culture conditions. The PMBV/PVA hydrogel was then dissociated using the same procedure described above to collect the encapsulated cells. The activity of ALP in the cells was measured optically using an ALP assay kit (TRACP & ALP Assay Kit, Takara Bio, Kusatsu, Japan) according to the manufacturer's instructions.
2.12 Cell culturing in double-layered PMBV/PVA hydrogels
Co-culturing of cells with different states was carried out using a double-layered hydrogel matrix.22,23 A schematic of this process is presented in Fig. S4 (ESI†).
In the first step, a 5.0 wt% PMBV solution and 2.5 wt% PVA solution were prepared using DMEM. In a Lipidure-PMB-coated cell-culturing dish with a diameter of 35 mm, 0.50 mL of the PMBV solution, 0.50 mL of the PVA solution containing 500 ng of BMP, and 100 μL of the DMEM suspension of C3H10T1/2 cells were added to each well. The cell seeding density was adjusted to 5.0 × 105 cells per mL. Cells were cultured in the PMBV/PVA hydrogel with a creep modulus of 0.40 kPa for 10 days under normal cell culture conditions. After 10 days, the cell-encapsulated PMBV/PVA hydrogels were dissolved using D-sorbitol solution and gently shaken by pipetting every 15 min for up to 60 min until the hydrogel was completely dissolved. The cell suspension was then centrifuged at 1000 rpm for 3 min to collect the cell aggregates. After removing the supernatant solution, DMEM and 6.0 wt% PMBV solution was immediately added and suspended. After that, 3.0 wt% PVA solution was mixed to prepare a cell aggregate encapsulated PMBV/PVA hydrogel. The creep modulus of the PMBV/PVA hydrogel was adjusted to 1.7 kPa. Using the same procedure, the PMBV/PVA hydrogel encapsulated mature osteoblast cells, which were prepared by differentiation of C3H10T1/2 cells by BMP-2.31,34
Second, in a cell-culturing dish with a diameter of 35 mm coated with Lipidure-PMB, 100 μL of the DMEM suspension of C3H10T1/2 cells, 0.50 mL of the 6.0 wt% PMBV solution, and 0.5 mL of the 3.0 wt% PVA solution were mixed together to prepare a cell-encapsulated PMBV/PVA hydrogel. The creep modulus of the PMBV/PVA hydrogel was adjusted to 1.7 kPa. By placing the cell-aggregate-encapsulated PMBV/PVA hydrogel, which was prepared in the first step, a double-layered PMBV/PVA hydrogel was constructed on the cell-encapsulated PMBV/PVA hydrogel. It was possible to coculture differentiated C3H10T1/2 cells (osteoblasts) and C3H10T1/2 cells encapsulated in the PMBV/PVA hydrogel.
2.13 Evaluation of the ALP activity in double-layered and co-cultured cell aggregates
Three types of PMBV/PVA hydrogels with encapsulated cells were prepared. The PMBV/PVA hydrogel with a creep modulus of 1.7 kPa encapsulated undifferentiated C3H10T1/2 cells or their differentiated cell aggregates (osteoblast cell aggregates) prepared by the addition of BMP-2 during the 10 days of cell culture. In addition, C3H10T1/2 cells were encapsulated in the PMBV/PVA hydrogel with a creep modulus of 1.7 kPa to prepare a cell-dispersed hydrogel. These hydrogels were integrated to prepare double-layered hydrogels as follows: System A was a double-layered hydrogel with undifferentiated C3H10T1/2 cell aggregates and dispersed cells. System B was a separate hydrogel system of osteoblast cell aggregates in the PMBV/PVA hydrogel and undifferentiated C3H10T1/2 cells in the hydrogel. These hydrogels were placed without direct contact in a cell-culture dish, and cell culturing was carried out for 10 days. System C was a double-layered hydrogel containing osteoblast cell aggregates and undifferentiated C3H10T1/2 cells. After 10 days of cell culture, the PMBV/PVA hydrogel was dissociated to recover the cell aggregates in the PMBV/PVA hydrogel. The ALP activity was measured using the procedure described above.
2.14 Statistical analysis
All experiments were performed at least three times. The results were evaluated using Student's t-test. The statistical analyses in this study were performed using Microsoft Excel 2016 (Microsoft Corp., Redmond, WA, USA).
3. Results and discussion
3.1 Characterization of PMBV
Three types of MPC polymers having both hydrophobic and phenylboronic acid units (PMBV) were synthesized by changing the monomer fractions in the feed solution. The chemical structure of PMBV is shown in Fig. 1. The radical polymerization proceeded homogeneously, yielding polymers.11,18 The chemical structures of PMBV were confirmed by 1H-NMR and FT-IR spectroscopies. The representative 1H-NMR and FT-IR spectra are shown in Fig. S1 and S2 (ESI†), respectively. In the FT-IR spectrum, IR absorptions attributed to carbonyl, phenyl, and phosphate groups were observed at 1720, 3100, and 1050 cm−1, respectively. The polymerization results are listed in Table 1. Every PMBV was completely soluble in water, PBS, and cell culture medium (DMEM).
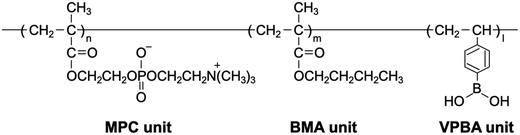 |
| Fig. 1 Chemical structure of PMBV. | |
3.2 Hydrogel formation between PMBV and PVA in an aqueous medium
By mixing the PMBV aqueous solution with the PVA aqueous solution, a hydrogel was formed at room temperature by gently mixing the solutions for 10 s.11,18 As shown in Fig. 2, the anionic form of the phenylboronic acid group reacts with dihydroxy compounds depending on the chemical structure. The PMBV/PVA hydrogel dissociates reversibly by the addition of D-sorbitol under atmospheric conditions at room temperature.20 During the gelation process, cells could be encapsulated in the PMBV/PVA hydrogel.
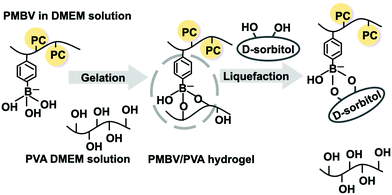 |
| Fig. 2 Hydrogel formation and the dissociation mechanism between PMBV and PVA in DMEM. Anionic form of the VPBA unit in the PMBV can react with hydroxy groups in PVA and make cross-linking points between PMBV and PVA. D-Sorbitol can dissociate the cross-linking points. PC represents the phosphorylcholine group in the MPC unit. | |
The properties of the PMBV/PVA hydrogels depended on the monomer unit composition in the PMBV and the concentrations of both polymers. When the concentrations of PMBV and PVA were adjusted to 5.0 wt%, the PMBV/PVA hydrogel formed in the case of PMBV-1 and PMBV-2. In the case of PMBV-2, the hydrogel was brittle, as shown in Fig. S5 (ESI†). PMBV-1 can form a homogeneous, transparent, and soft hydrogel. On the other hand, PMBV-3 did not form hydrogels with PVA. This was due to the lower fraction of phenylboronic acid units providing stable cross-linking with PVA. Therefore, PMBV-1 is a promising candidate for cell encapsulation. In the following experiment, we used PMBV-1 as a polymer component of the PMBV/PVA hydrogels.
The creep modulus of the PMBV/PVA hydrogel was controlled by varying the concentration of PMBV. Fig. 3 shows the concentration dependence of the creep modulus of the PMBV/PVA hydrogel. The creep modulus increased linearly with PMBV concentration. This is suitable for regulating cell behavior after encapsulation. In our previous study, the proliferation of cells encapsulated in the PMBV/PVA hydrogel strongly depended on the modulus of the hydrogel.20 The hydrogel was stable even when it was maintained in DMEM. The relative swelling ratio of the PMBV/PVA hydrogel during the immersion period in DMEM is shown in Fig. S6 (ESI†). The relative swelling ratio of the hydrogel did not change from its initial value. Although the DMEM solution contained glucose, the binding constant of glucose to PMBV was smaller than that of PVA.21 Thus, dissociation of the PMBV/PVA hydrogel hardly occurred in the DMEM solution.
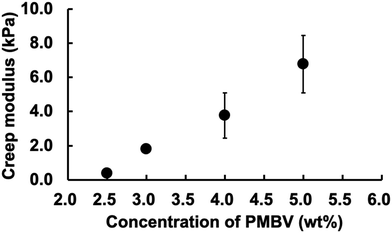 |
| Fig. 3 Relationship between the concentration of PMBV and creep modulus of the PMBV/PVA hydrogel. The composition ratio of PMBV/PVA was 2/1. | |
The PMBV/PVA hydrogels adhered when the two hydrogel layers were in contact with each other. Notably, no additional process was required because phenylboronic acid groups and hydroxyl groups that appeared at the surface could react at the interface between the two hydrogel layers.35 To evaluate the adhesion between interfaces of two hydrogel layers, molecular diffusion of the small dye was examined. Optical photo images are shown in Fig. S7 (ESI†). The PMBA/PVA hydrogel layer was in contact with the surface of another PMBV/PVA hydrogel containing a small amount of trypan blue dye. On the first day, the interface between the two hydrogel layers was clear. However, after three days, the entire hydrogel was stained by diffusion of the dye, and the interface disappeared. In addition, although two hydrogel layers of the double-layered hydrogel had different creep moduli, the creep modulus of the hydrogels became average after three days of contact. That is, when the creep moduli of the initially formed hydrogel layers were 6.8 kPa and 0.40 kPa, respectively, the value became about 3.7 kPa after three day-integration. This may indicate that the two hydrogel layers with different properties become a uniform equilibrium state with time. Therefore, adhesion of the PMBV/PVA hydrogels could be achieved spontaneously by simply contacting the two hydrogel surfaces.
3.3 Cell proliferation in the PMBV/PVA hydrogel
Inducing cell differentiation from stem cells to produce a specific cell type requires a process of cell aggregation. During this process, communication between cells may occur with cytokines, resulting in concerted differentiation induction. In other words, a method to form high-quality cell aggregates is also necessary to create cell tissues in three dimensions. In this study, we used mouse MSCs (C3H10T1/2 cells) as the stem cell source.
When C3H10T1/2 cells suspended in DMEM were added during hydrogel formation between PMBV and PVA, cell encapsulation was achieved at 25 °C.20,34 In addition, the creep modulus of the hydrogel was regulated by changing the polymer concentration.
Fig. 4 shows a phase-contrast microscopic image of the cells in the hydrogel. The cells were evenly dispersed in hydrogels with different creep moduli. In the case of the hydrogel with a higher creep modulus, cell proliferation was not significant. On the other hand, in the case of the hydrogel with a lower creep modulus, the cells proliferated with culturing. Small aggregates were observed on day 3. The size of the aggregates increased on day 7. As shown in Fig. 5, the size of the cell aggregates increased linearly with the cell culture period in the low-creep-modulus hydrogel (0.40 kPa) and reaches approximately 78 μm in diameter for 10 days. The morphology of the cell aggregates is spherical. The morphology of each cell in the aggregates did not change. Therefore, 3D culturing of C3H10T1/2 cells in the PMBV/PVA hydrogel with a low creep modulus provided size-controlled cell aggregates under normal cell culture conditions. After cell culturing, cell aggregates can be recovered from the hydrogel matrix by the addition of D-sorbitol to the solution. We evaluated the number of cells in cell aggregates. The hanging drop method is generally used to produce cell aggregates. It can be used to obtain uniform cell aggregates easily. To evaluate the number of cells contained in the cell aggregates formed in the PMBV/PVA hydrogel, reference cell aggregates were prepared by the hanging drop method, encapsulated in the PMBV/PVA hydrogel, and cultured for 1 day. Then the radius of the cell aggregate was measured by phase contrast microscopic observation. The cell aggregate prepared from 2.5 × 104 cells by the hanging drop method was approximately 390 μm in diameter. From this result, we determined that approximately 2.2 × 102 cells were contained in the cell aggregate with a diameter of approximately 78 μm after being cultured 10 days in the PMBV/PVA hydrogel.
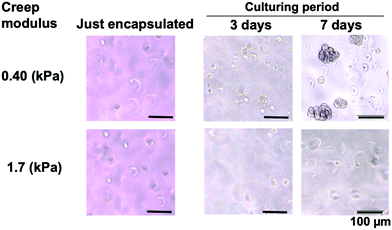 |
| Fig. 4 Morphology of C3H10T1/2 cells cultured in the PMBV/PVA hydrogels with different creep moduli. | |
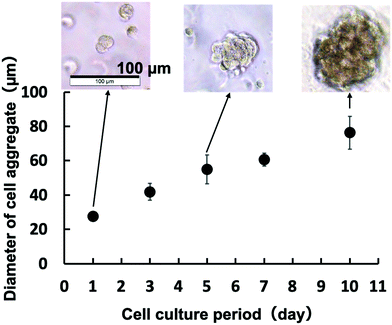 |
| Fig. 5 Relationship between the cell culture period and diameter of the C3H10T1/2 cell aggregate in the PMBV/PVA hydrogel with a creep modulus of 0.40 kPa. | |
3.4 ALP activity in cells encapsulated in the PMBV/PVA hydrogel
To clarify the functionality of MSCs in the hydrogel, the differentiation of stem cells was evaluated by the addition of cytokines. C3H10T1/2 cells differentiated into osteoblasts upon the addition of BMP-2. After the cells were cultured in the PMBV/PVA hydrogel with BMP-2 for 10 days, the cells were recovered by dissociation of the hydrogel matrix. After ALP staining, most of the cells were blue, as shown in Fig. S8 (ESI†). Cell differentiation could proceed by culturing with BMP-2 for 10 days, even when the cell culture was carried out in the hydrogel. The molecular diffusion in the PMBV/PVA hydrogel depends on the density of the polymer network.19 However, in this case, the total polymer concentration was 5.0 wt%; the remaining 95 wt% was DMEM. Molecular diffusion of cytokines occurred in the DMEM phase in the hydrogel, which was not disturbed by the polymer network. In addition, for the MPC polymer and PVA, protein binding hardly occurred. Thus, the diffusivity of cytokines may be sufficient to induce cell differentiation.
Fig. 6 shows the total activity of ALP in the cells cultured in the PMBV/PVA hydrogel. The total activity of ALP was higher after BMP-2 addition in the culture system. The ALP activity is generally recognized as an important indicator of osteoblast differentiation.31–33 The molecular mechanism of ALP activity and osteoblast calcification is elucidated when cells are stimulated by BMP-2. The creep modulus of the PMBV/PVA hydrogel was another factor that determined the increase in ALP activity in the cells. We have reported that the storage modulus of the hydrogel for the encapsulation of cells affects the cell proliferation cycle. In the case of the hydrogel with a storage modulus of 1.2 kPa, the proliferation of the encapsulated cells is arrested at the G1/G0 phase.20 The cells in this phase are sensitive to the cytokines for differentiation. Thus, the addition of cytokines resulted in a considerably more effective differentiation of the cells in the hydrogel. The same phenomenon may have occurred in this study.
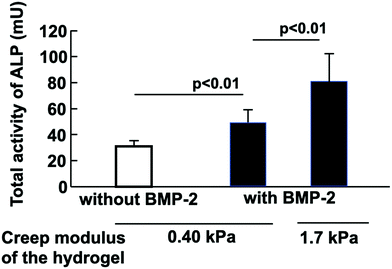 |
| Fig. 6 Total activity of ALP based on the differentiation of C3H10T1/2 cells stimulated by BMP-2 in the PMBV/PVA hydrogel. Cells were cultured for 10 days. | |
3.5 Effects of the mature cell aggregate on the differentiation of C3H10T1/2 cells
In living systems, damaged tissues are self-repaired. Undifferentiated stem cells are considered to be influenced by the surrounding mature cells at the site of injury and differentiate into cells that form optimal tissues.36–39 Thus, the relationship between mature cell tissue and undifferentiated stem cells is important for tissue regenerative medicine. We observed the induction of stem cell differentiation by coculturing mature and undifferentiated stem cells using a double-layered hydrogel system. As a characteristic of the PMBV/PVA hydrogel, the phenylboronic acid group remained on the surface, while the hydroxyl group self-adhered by forming a bond between the other PMBV/PVA hydrogel layers. In other words, when cells with different states are included in the two PMBV/PVA layers, the cell response induced by the molecular communication between the cells in the indirect contact state can be evaluated.
Fig. 7 shows the total activity of ALP in the double-layered hydrogels, encapsulating undifferentiated MSCs (C3H10T1/2 cells) and their aggregates (A), differentiated C3H10T1/2 cell aggregates, and undifferentiated C3H10T1/2 cells (C). Differentiated C3H10T1/2 cell aggregates were prepared by the addition of BMP-2 and cultured for 10 days; that is, mature osteoblast cell aggregates could be obtained. The total ALP activity was 2.4 times larger in the case of (C) than that in (A). The mature osteoblast cell aggregates induced the differentiation of C3H10T1/2 cells. When the two hydrogels containing osteoblast aggregates and C3H10T1/2 cells were separately placed (B) on the cell-culturing dish and co-cultured, the ALP activity was not significantly different from that of the double-layered hydrogel of (A). These results show that the close placement of these cells by the adhesion between the two hydrogel layers is important for the effective differentiation of C3H10T1/2 cells by mature osteoblast cell aggregates. Thus, mature cells can induce differentiation of the surrounding undifferentiated cells.40,41 This could be explained by the small distance of molecular diffusion and the high concentration of cytokines that induce cell differentiation from mature cells and reach undifferentiated cells.
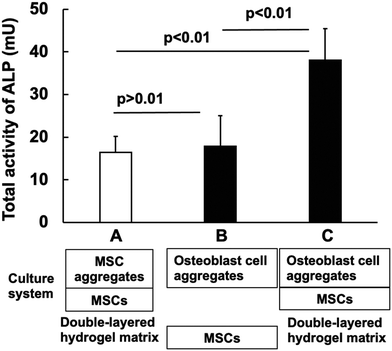 |
| Fig. 7 Total activity of ALP based on the differentiation of MSCs (C3H10T1/2 cells) stimulated by mature osteoblast cell aggregates in the double-layered hydrogel system cultured for 10 days. (A) Double-layered hydrogels containing MSC aggregates and MSCs, (B) two hydrogels containing osteoblast cell aggregates and MSCs, respectively, and (C) double-layered hydrogels containing osteoblast aggregates and MSCs. | |
In differentiating stem cells into target cells in a culture environment, it is common to use a single cytokine.42,43 On the other hand, if some cytokines secreted by mature cell aggregates can be naturally utilized, tissues can be produced more efficiently. The zwitterionic polymer hydrogel system with excellent properties including cytocompatibility, reversible gelation under mild conditions, maintenance of encapsulated cell activity, self-adhesion properties, and molecular diffusion properties may be used to design a matrix that induces differentiation of stem cells.
4. Conclusions
A hydrogel containing cells could be formed from a polymer with a phosphorylcholine group, a phenylboronic acid group, and a polymer with hydroxyl groups. The hydrogels could integrate, yielding a layered structure via self-adhesion. The differentiation of stem cells encapsulated in hydrogels is induced by cytokines. When mature cell aggregates and stem cells were encapsulated in the two hydrogel layers, respectively, after they were adhered, the stem cells differentiated efficiently.
Conflicts of interest
There are no conflicts to declare.
Acknowledgements
A part of this research was supported by a Grant-in-Aid for Scientific Research on Innovative Areas ‘‘Nanomedicine Molecular Science’’ (No. 2306) from the Ministry of Education, Culture, Sports, Science, and Technology of Japan, and Grant-in-Aid for Scientific Research (B) (26282135) from Japan Society for the Promotion Science.
References
- L. Feng, S. Liang, Y. Zhou, Y. Luo, R. Chen, Y. Huang, Y. Chen, M. Xu and R. Yao, ACS Biomater. Sci. Eng., 2020, 6, 2995–3004 CrossRef CAS PubMed.
- A. M. Hilderbrand, E. M. Ovadia, M. S. Rehmann, P. M. Kharkar, C. Guo and A. M. Kloxin, Curr. Opin. Solid State Mater. Sci., 2016, 20, 212–224 CrossRef CAS PubMed.
- S. V. Hadjiantoniou, D. Sean, M. Ignacio, M. Godin, G. W. Slater and A. E. Pelling, J. R. Soc., Interface, 2016, 13, 20160613 CrossRef PubMed.
- N. Betriu, C. Jarrosson-Moral and C. E. Semino, Biomolecules, 2020, 10, 684 CrossRef CAS.
- B. M. Bijonowski, S. I. Daraiseh, X. Yuan and T. Ma, Tissue Eng., Part A, 2019, 25, 575–587 CrossRef CAS.
- C. R. Correia, I. M. Bjørge, J. Zeng, M. Matsusaki and J. F. Mano, Adv. Healthcare Mater., 2019, 8, e1901221 CrossRef PubMed.
- S. J. Singer, Science, 1992, 255, 671–677 CrossRef.
- S. Sart, Y. Liu, T. Ma and Y. Li, Tissue Eng., Part A, 2014, 20, 2666–2679 CrossRef CAS.
- N. E. Ryu, S. H. Lee and H. Park, Cells, 2019, 8, 1620 CrossRef CAS PubMed.
- H. Kim, S. H. Han, Y. M. Kook, K. M. Lee, Y. Z. Jin, W. G. Koh, J. H. Lee and K. Lee, J. Mater. Chem. B, 2020, 8, 9481–9491 RSC.
- K. Ishihara, H. Oda and T. Konno, Biomaterials, 2020, 230, 119628 CrossRef CAS PubMed.
- D. Dong, J. Li, M. Cui, J. Wang, Y. Zhou, L. Luo, Y. Wei, L. Ye, H. Sun and F. Yao, ACS Appl. Mater. Interfaces, 2016, 8, 4442–4455 CrossRef CAS PubMed.
- V. Perugini, M. Best, S. Kumar, A. L. Guildford, A. J. Bone, W. M. Macfarlane, M. Santin and G. J. Phillips, J. Mater. Sci.: Mater. Med., 2017, 29, 15 CrossRef PubMed.
- K. Ishihara, M. Abe, K. Fukazawa and T. Konno, Macromol. Biosci., 2021, 21, e2000341 CrossRef.
- C. Wachiralarpphaithoon, Y. Iwasaki and K. Akiyoshi, Biomaterials, 2007, 28, 984–993 CrossRef CAS PubMed.
- X. Montano, A. L. Lewis, S. W. Leppard and C. Lichtenstein, Biotechnol. Bioeng., 2005, 90, 770–774 CrossRef CAS.
- G. Liu, K. Iwata, T. Ogasawara, J. Watanabe, K. Fukazawa, K. Ishihara, Y. Asawa, Y. Fujihara, U.-L. Chung, T. Moro, Y. Takatori, T. Takato, K. Nakamura, H. Kawaguchi and K. Hoshi, J. Biomed. Mater. Res., Part A, 2010, 92, 1273–1282 CAS.
- T. Konno and K. Ishihara, Biomaterials, 2007, 28, 1770–1777 CrossRef CAS PubMed.
- T. Aikawa, T. Konno, M. Takai and K. Ishihara, Langmuir, 2012, 28, 2145–2150 CrossRef CAS.
- H. Oda, T. Konno and K. Ishihara, Biomaterials, 2013, 34, 5891–5896 CrossRef CAS PubMed.
- H. Oda and K. Ishihara, React. Funct. Polym., 2019, 135, 113–120 CrossRef CAS.
- B. Gao, T. Konno and K. Ishihara, J. Biomater. Sci., Polym. Ed., 2015, 26, 1372–1385 CrossRef CAS.
- B. Gao, T. Konno and K. Ishihara, Colloids Surf., B, 2013, 108, 345–351 CrossRef CAS PubMed.
- D. N. Heo, M. Hospodiuk and I. T. Ozbolat, Acta Biomater., 2019, 95, 348–356 CrossRef CAS.
- K. Ishihara, T. Ueda and N. Nakabayashi, Polym. J., 1990, 22, 355–360 CrossRef CAS.
- K. Ishihara, J. Biomed. Mater. Res., Part A, 2019, 107, 933–943 CrossRef CAS PubMed.
- G. Chao-Chi and A.-I. Yeh, Cereal Chem., 2006, 83, 179–187 CrossRef.
- K. Ishihara, Langmuir, 2019, 35, 1778–1787 CrossRef CAS.
- R. Foly, J. Visualized Exp., 2011, 51, 2720 Search PubMed.
- M. Chen, Y.-Q. Lin, S.-L. Xie, H.-F. Wu and J.-F. Wang, Biotechnol. Lett., 2011, 33, 853–858 CrossRef CAS.
- G. Rawadi, B. Vayssière, F. Dunn, R. Baron and S. Roman-Roman, J. Bone Miner. Res., 2003, 18, 1843–1853 CrossRef PubMed.
- R. T. Franceschi and B. S. Iyer, J. Bone Miner. Res., 1992, 7, 235–246 CrossRef CAS PubMed.
- G. S. Stein, J. B. Lian and T. A. Owen, FASEB J., 1990, 4, 3111–3123 CrossRef CAS PubMed.
- H. Oda, T. Konno and K. Ishihara, Biomaterials, 2015, 36, 86–91 CrossRef.
- Y. Chen, D. Diaz-Dussan, D. Wu, W. Wang, Y. Y. Peng, A. B. Asha, D. G. Hall, K. Ishihara and R. Narain, ACS Macro Lett., 2018, 7, 904–908 CrossRef CAS.
- Z. Weiliang and G. Lili, Ann. Dermatol., 2021, 33, 309–317 CrossRef PubMed.
- N. Urao, J. Liu, K. Takahash and G. Ganesh, Adv. Wound Care, 2021 DOI:10.1089/wound.2021.0065.
- Y. Xu, W. X. Zhang, L. N. Wang, Y. Q. Ming, Y. L. Li and G. X. Ni, World J. Stem Cells, 2021, 13, 753–775 CrossRef PubMed.
- S. Muthu, M. Jeyaraman, R. Jain, A. Gulati, N. Jeyaraman, G. S. Prajwal and P. C. Mishra, Stem Cell Invest., 2021, 8, 13 CrossRef PubMed.
- X. F. Ma, X. B. Ma, W. J. Qian, H. Y. Zhao, J. P. Ding and T. L. Zhao, J. Craniofac. Surg., 2020, 31, 2355–2359 CrossRef.
- K. Seo, T. Suzuki, K. Kobayashi and T. Nishimura, Anim. Sci. J., 2019, 90, 423–434 CrossRef CAS.
- A. Karadag, B. O. Oyajobi, J. F. Apperley, R. G. Russell and P. I. Croucher, Br. J. Haematol., 2000, 108, 383–390 CrossRef CAS.
- J. A. Spector, Plast. Reconstr. Surg., 2002, 109, 631–642 CrossRef PubMed.
Footnote |
† Electronic supplementary information (ESI) available. See DOI: 10.1039/d1tb01817e. |
|
This journal is © The Royal Society of Chemistry 2022 |
Click here to see how this site uses Cookies. View our privacy policy here.