DOI:
10.1039/D0QO00510J
(Review Article)
Org. Chem. Front., 2020,
7, 3067-3099
Recent advances in the synthesis of bridgehead (or ring-junction) nitrogen heterocycles via transition metal-catalyzed C–H bond activation and functionalization
Received
30th April 2020
, Accepted 31st July 2020
First published on 5th August 2020
Abstract
Bridgehead nitrogen (or ring-junction nitrogen) heterocycles are one of the most privileged scaffolds in synthetic chemistry and medicinal chemistry. For decades, transition metal-catalyzed C–H activation has provided straightforward access to bridgehead nitrogen heterocycles in an atom-economical manner. Palladium, rhodium, ruthenium, iridium, cobalt, nickel, silver, and copper catalysts were used for the successful synthesis of these skeletons. Herein, we summarize recent advances that are made in the synthesis of bridgehead nitrogen heterocycles via diverse transition metal-catalyzed C–H activations and C–H functionalizations. This review covers the period from May 2004 to April 2020.
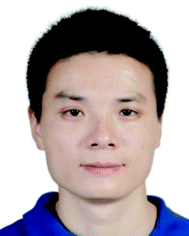 Biao Nie | Biao Nie received his MS from China Pharmaceutical University in 2010. He joined the HEC Pharm Group in 2010 where his research interests were focused on drug synthesis. He then joined Jiang's group as a PhD candidate under the supervision of Professor Huanfeng Jiang in 2016, where his primary research focus is on transition-metal-catalyzed C–H functionalization and its applications in drug synthesis. |
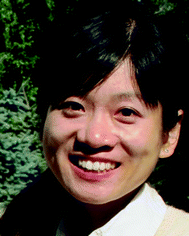 Wanqing Wu | Wanqing Wu received her PhD from Peking University with Professor Zhen Yang and Chi-Sing Lee in 2010. And then she joined Professor Huanfeng Jiang's group as a postdoctoral researcher at South China University of Technology (SCUT). In 2014, she was promoted to Professor and her research interests include the development and applications of carbon-heteroatom triple bond transformations. |
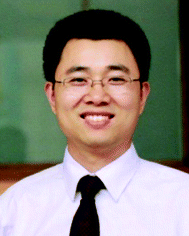 Yingjun Zhang | Yingjun Zhang obtained his BSc degree in 2001 and his PhD in 2007 from Hunan University. He was a postdoctoral researcher at Okayama University of Science, Japan (2007–2008) before joining the HEC Pharm Group as a research chemist. He was a group leader on drug discovery (2008–2010), before being promoted to director (2010-) taking in charge of the drug discovery program. |
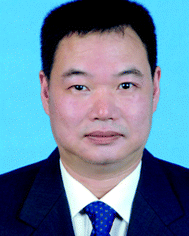 Huanfeng Jiang | Huanfeng Jiang received his PhD from the Shanghai Institute of Organic Chemistry (SIOC) with Professor Xiyan Lu in 1993. And then he joined the Guangzhou Institute of Chemistry as a research fellow. In 2003, he moved to the South China University of Technology (SCUT) as the Leading Professor of Chemistry. He received Chinese Chemical Society-BASF Young Investigator's Award in 2002 and the National Natural Science Funds for Distinguished Young Scholar in 2006. His research interests focus on synthetic methodology, and green and sustainable chemistry. |
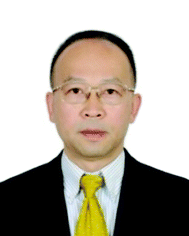 Ji Zhang | Ji Zhang received his academic training from the South China Normal University (BS, 1983), Beijing Normal University, China (MS. 1987) and obtained his Ph.D (1997) from the University of Victoria, Canada with Prof. Reginald H. Mitchell. He took positions as a research chemist at Abbott Laboratories in Chicago (1997–2000), associate research fellow of Pfizer Global Research & Development at Ann Arbor, Michigan (2000–2007), and principal scientist of Bristol-Myers Squibb at Princeton/New Brunswick, New Jersey, USA (2007–2011). Currently, he is a Chief Scientific Officer of HEC Pharm Group in China. |
1. Introduction
Bridgehead nitrogen (ring-junction nitrogen) heterocycles are among the most privileged cores in natural products (Fig. 1)1 and therapeutic pharmaceuticals2 (Fig. 2). These scaffold architectures play important roles in chemical biology and drug discovery. For example, rutaecarpine,3 amaryllidaceae alkaloids,4 luotonin A and B,5 (+)-austamide,6 lamellarin D,7 and camptothecin8 have diverse biological activities. Alprazolam,9 midazolam,10 vardenafil,11 sitagliptin,12 ponatinib,13 dolutegravir,14 filgotinib,15 upadacitinib,16 baloxavir marboxil,17 and remdesivir18 were approved to treat a variety of serious diseases. In particular, the FDA has recently approved the emergency use of remdesivir for COVID-19.19 Consequently, these heterocycles have attracted great attention from synthetic chemists and medical scientists.20 In order to obtain these scaffolds efficiently, various methods involving cycloaddition reactions,21 cyclization reactions,22 reduction reactions,23 multicomponent reactions,24 cyclization of N-acyliminium,25 transition-metal-catalyzed cyclizations26etc. have been developed. Among these methods, transition metal catalyzed C–H activation provided a straightforward way to access bridgehead nitrogen heterocycles in an atom-economical manner, by a facile one-pot synthesis of complex molecules without the requirement of substrate prefunctionalization.27 One of these examples has been shown in the synthesis of two imidazopyridine drug molecules: alpidem and zolpidem (Scheme 1).28
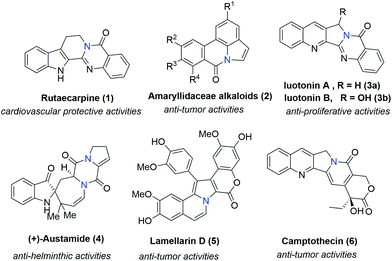 |
| Fig. 1 Some natural products bearing bridgehead nitrogen heterocycle scaffolds. | |
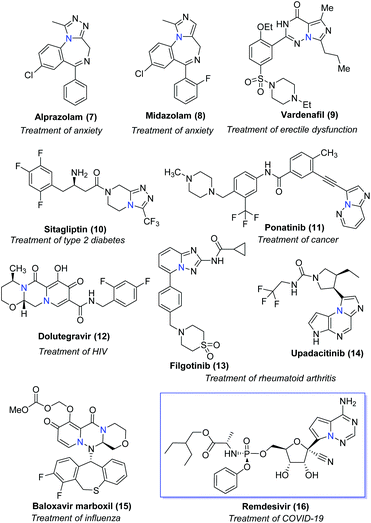 |
| Fig. 2 Some launched pharmaceuticals containing bridgehead nitrogen heterocycles moieties. | |
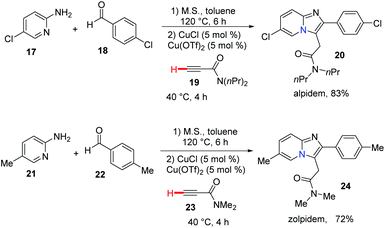 |
| Scheme 1 One pot synthesis of alpidem and zolpidem via copper-catalyzed C–H functionalization reactions. | |
Ferraccioli29 and Guo30 reviewed palladium-catalyzed and copper-catalyzed C–H functionalization reactions for the construction of heterocycles respectively. In 2016, various transition metal-catalyzed heterocycle syntheses via C–H activation were reviewed by Wu and co-workers.31 However, their coverage is not specific to bridgehead nitrogen heterocycles. Meanwhile, the Sharma group covered transition-metal-catalyzed synthesis of imidazopyridines.32 Zhang et al. summarized copper-catalyzed C–H functionalizations for the synthesis of bridgehead nitrogen heterocycles.33 Yet these reviews were limited to one type of heterocycle nucleus or to a particular metal catalysis. Due to the importance of bridgehead nitrogen heterocycles and the rapid development of C–H activation methodologies, a timely review which is specific to the synthesis of bridgehead nitrogen heterocycles via diverse transition metal-catalyzed C–H activations and functionalizations would be desirable and useful for the exploration of new reactions and beneficial for drug discovery.
Therefore, we present this review organized according to different kinds of metals for the C–H activation and functionalization, further subdividing according to the fused nitrogen heterocycle structure (Fig. 3). This review covers the time period from the year 2004 to 2020. The notable features of this review are as follows: (i) various transition metal catalysts including Pd, Rh, Ru, Ir, Co, Ni, Ag and Cu are involved; (ii) diverse kinds of bridgehead nitrogen heterocycles are covered; (iii) C–H bond activation including C(sp2)–H, C(sp)–H, and C(sp3)–H is depicted; (iv) selected examples, mechanisms and potential applications (particularly in drug discovery) of reactions are discussed.
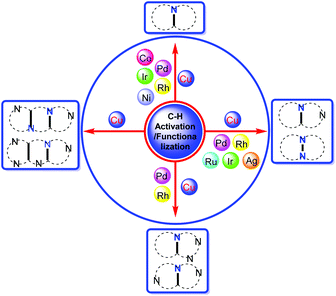 |
| Fig. 3 A concise summary of the preparation of different kinds of bridgehead nitrogen heterocycles by various transition metal-catalyzed C–H bond activations and functionalizations. | |
2. Transition metal catalyzed synthesis of bridgehead nitrogen heterocycles
2.1 Palladium-catalyzed synthesis of bridgehead nitrogen heterocycles
Knochel and co-workers developed a novel palladium-catalyzed intramolecular cyclization leading to condensed N-heterocycles 26 (Scheme 2).34 These polyheterocycles are frequently found as the cores of both natural products and drug molecules; the preparation of the starting N-arylpyrrole 25 proved to be extremely simple. This cyclization involved a Pd-catalyzed C(sp3)–H (benzylic type) activation step and screening indicated that the ligand p-Tol3P and the base Cs2CO3 are superior to the other ligand/base combinations explored for this transformation.
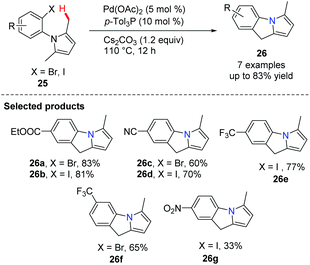 |
| Scheme 2 Palladium-catalyzed intramolecular cyclization. | |
Oestreich reported a C-2 alkenylation of indoles with substituted alkenes by utilizing a Pd(OAc)2-pyridine ligand system in 2012 (Scheme 3).35 The key step here is an endo cyclization of alkenes onto indoles temporarily tethered to the indole nitrogen atom by an amide linkage.
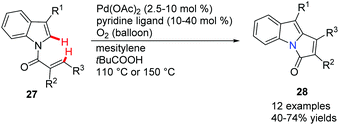 |
| Scheme 3 Palladium-catalyzed intramolecular cyclization. | |
Lautens and co-workers developed a palladium-catalyzed/norbornen-mediated complex for the synthesis of 5,6-dihydro-pyrrolo[2,1-a]isoquinoline derivatives 31via the C(sp2)–H functionalization reaction of pyrazoles 29 and aryl iodides 30 (Scheme 4).36 These skeletons have been found in some natural and biologically active compounds, including lettowianthine and lamellarin D.
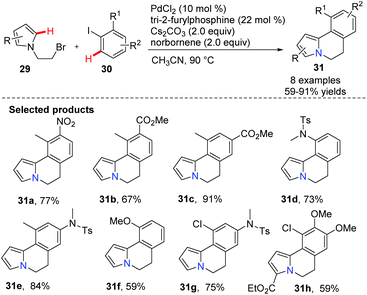 |
| Scheme 4 Palladium-catalyzed alkylation/direct arylation sequence. | |
Recently, Hu and co-workers described a dehydrogenative Heck annulation reaction of indolizine 32 with diaryl acetylene 33via dual C(sp2)–H bond cleavage (Scheme 5).37 A detailed mechanistic study confirmed that C–H bond metalation of the 7-position of the indolizine is the rate-limiting step. By optimizing and screening various additives and solvents, they found that the ligand 2,6-difluorobenzoic acid was the most efficient additive and DMSO the ideal solvent. This protocol has provided a simple and straightforward route to arylpyrrolo[2,1,5-cd]indolizines 34 under base-free conditions. In order to avoid regioisomers, symmetric alkynes were used.
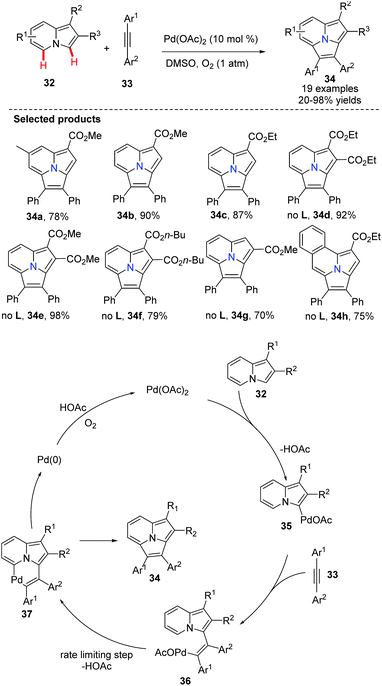 |
| Scheme 5 Dual C–H activation and a plausible mechanism. | |
Chang discovered a Pd(OAc)2-catalyzed cyclization of N-(2-halobenzyl)-substituted pyrroles 38 to afford polycyclic compounds 39 (Scheme 6).38 The reaction of substrates bearing electron-deficient groups on the pyrrole was found to undergo cyclization with faster rates compared to those bearing electron-donating groups. This method has provided an efficient route to the pyrroloindoles 39 which are difficult to obtain using the conventional Friedel–Crafts approach.
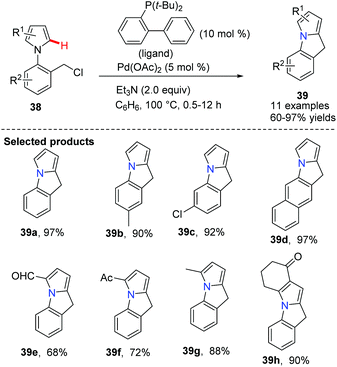 |
| Scheme 6 Pd-Catalyzed synthesis of pyrroloindoles. | |
Booker-Milburn and co-workers pioneered a palladium-catalyzed C(sp2)–H activation/cascade strategy for the synthesis of polyheterocycles 42 from readily available materials 40 (Scheme 7).39 In order to avoid the furan moiety undergoing conjugate addition with the oxidant, a sterically bulky quinone 41 was used as an oxidizing agent. It was found that a short reaction time, typically 30 minutes, at an elevated temperature favoured this transformation. A large range of polycyclic compounds containing pyrrol, indole, furan and thiophene, such as 42a to 42h, were prepared in one step.
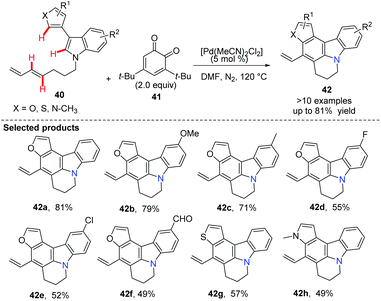 |
| Scheme 7 Synthesis of polyheterocycles by the C–H activation cascade sequence. | |
In the proposed mechanism, compound 40 undergoes C–H activation by coordination of the palladium(II) center to the diene 43. Then, the intermediate 44 is formed by the cyclization by syn-carbometalation of the diene, which is followed by an attack of the second heterocyclic ring onto this electrophilic complex to give 45. Next, dehydrogenation of 45 leads to 46. Finally, the oxidation of the product 46 delivers 42 under mild reaction conditions (Scheme 8).
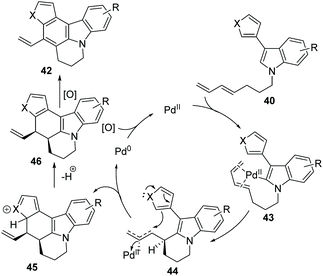 |
| Scheme 8 A plausible mechanism for the synthesis of polyheterocycles. | |
Xu, Loh and co-workers reported a feasible and efficient one-pot approach for the synthesis of pyrroloisoindolone derivatives 48 using N-vinyl acetamides by palladium-catalyzed intramolecular C(sp3)–H activation (Scheme 9).40 The strategy offered straightforward access to valuable nitrogen-containing products 48a–48k under mild reaction conditions. A proposed mechanism suggested that this is an intramolecular Heck cyclization involving 5-exo cyclization, C(sp3)–H activation and reductive elimination during the catalytic cycles.
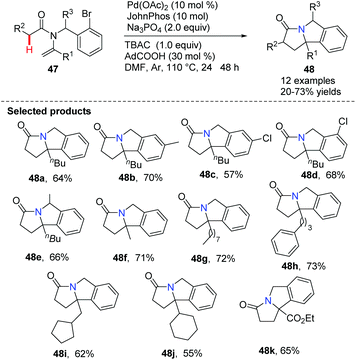 |
| Scheme 9 Palladium-catalyzed domino coupling reaction. | |
Verma and co-workers disclosed the palladium-catalyzed Sonogashira coupling conjoined C(sp2)–H activation for the syntheses of pyrrolo[1,2-a]quinoline derivatives 51.41 It is important to avoid the uncyclized product 52 during the tandem transformation. After an extensive screening of Pd(II) sources [PdCl2, Pd(OAc)2, Pd2(dba)3 and PdCl2(PPh3)2], additives such as LiCl and CuI, solvents (CH3CN, DMF, DMSO, DMA) and bases (Et3N, K3PO4, KOtBu, NaOAc), the best and general combination for these substrates was determined to be 5 mol% of PdCl2(PPh3)2, 3.0 equiv. of NaOAc, 2.0 equiv. of LiCl in DMA at 120 °C for the tandem cyclization. This method was also used for the synthesis of pyrrolo[1,2-a]quinolines when 1-(2-bromophenyl)-pyrrole and terminal alkynes were used under the same reaction conditions (Scheme 10).
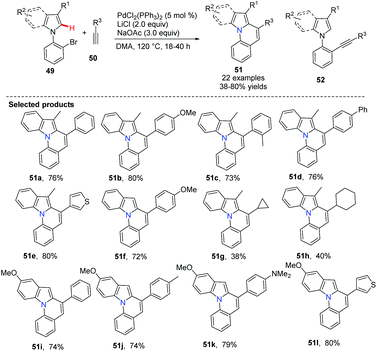 |
| Scheme 10 Palladium-catalyzed tandem coupling reaction and cyclization. | |
Substituted pyrazolo[1,5,a]pyridines are important molecules which are often employed as indole isosteres due to their relatively high metabolic stability in drug discovery. In 2011, Charette and co-workers reported the study of a domino direct alkynylation and cyclization of N-iminopyridinium ylides using alkenyl bromides and alkenyl iodides (Scheme 11).42 Compared with previously reported synthetic methods, this protocol is greatly advantageous because only a two-step process is required. The selected screening of reaction conditions found silver benzoate to be the most favourable silver salt; a P(4-MeOPh)3/PdBr2 ratio of 3
:
1 provided the best results. Solvent choice was examined showing that 1,4-dioxane is ideal and running the reaction at 125 °C for 16 h provided the best general outcome for the substrates involved.
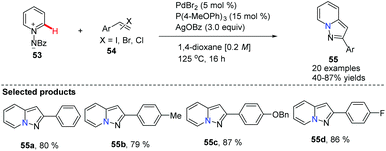 |
| Scheme 11 Domino direct alkynylation and cyclization of N-iminopyridinium ylides with alkenyl bromides or iodides. | |
Charette and co-workers conducted mechanistic studies and confirmed the formation of alkynes from alkenyl iodides, by a competition reaction. It was found that the reaction proceeded quickly once the alkyne was generated, and thus alkynes were identified as optional starting materials for this transformation (Scheme 12 and 13).
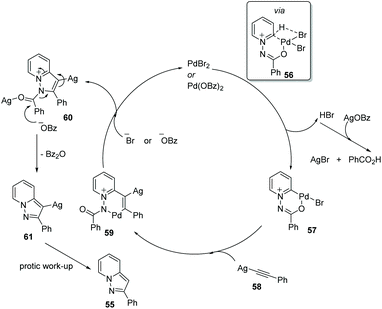 |
| Scheme 12 Proposed catalytic cycle involving the C–H activation. | |
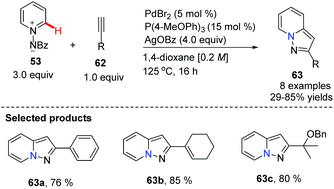 |
| Scheme 13 Domino direct alkynylation and cyclization of N-iminopyridinium ylides with alkynes. | |
Direct C(sp2)–H azidation, followed by N–N bond formation to give novel bridgehead nitrogen heterocycles was rarely observed until 2013 when Jiao and co-workers first showcased a novel Pd-catalyzed azidation of arylpyridines via C(sp2)–H activation using azides as an external nitrogen source (Scheme 14).43 This study is significant because it provides a concise, alternative approach to bioactive pyrido[1,2-b]indazoles from readily available arylpyridines.
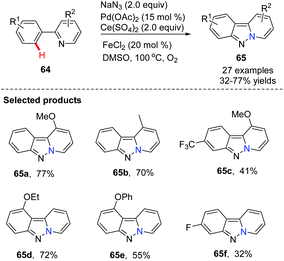 |
| Scheme 14 Palladium-catalyzed nitrogenation reaction. | |
SanMartin, Dominguez and co-workers developed a new strategy for the rapid construction of a series of pyrazolo[1,5-f]phenanthridines 67 from simple starting materials, such as acetophenones and arylhydrazines 66.44 The cyclization by intramolecular biaryl bond formation is the key step, accomplished by direct C–H activation utilizing Pd(OAc)2 as the catalyst (Scheme 15).
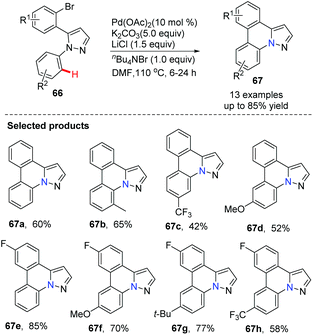 |
| Scheme 15 Synthesis of pyrazolo[1,5-f]phenanthridines by direct arylation. | |
Chen, Wu and co-workers developed a novel approach for the synthesis of indazolo[3,2-b]quinazolinone derivatives 70via palladium-catalyzed C–H activation/intramolecular amination of 2-aryl-3-(arylamino)quinazolinones 68 in moderate to excellent yields (Scheme 16).45 Furthermore, the potential utility of the products was demonstrated as a new class of blue fluorophores for fluorescent materials. Preliminary mechanistic studies suggested that a palladacycle dimer could be the key intermediate, which underwent a cascade cyclometalation and C–H amination sequence.
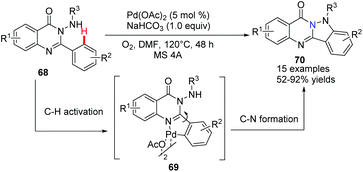 |
| Scheme 16 Synthesis of indazolo[3,2-b]quinazolinone derivatives via Pd-catalyzed C–H activation/intramolecular amination. | |
In 2019, Sawant et al. reported that under Pd-catalyzed conditions, azomethine imine 75 reacted with nitroolefins 74, giving bridgehead nitrogen containing heterocycles 76.46 A one-pot, four component approach (Method B) also gave this potential pharmaceutically useful polyheterocyclic skeleton from readily available 2-azidobenzaldehyde 71, isocyanide 72, aryl sulfonyl hydrazide 73 and nitroolefins 74 (Scheme 17).
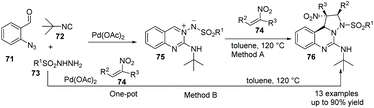 |
| Scheme 17 Pd-Catalyzed cyclocondensation of azomethine imine. | |
2.3. Rhodium-catalyzed synthesis of bridgehead nitrogen heterocycles
Utilizing Rh as a catalyst, silver and copper salts as co-catalysts, Wu, Zhang and Huang developed a one-step synthesis of indolo[2,1-a]isoquinolines 79via a double C–H annulation cascade (Scheme 18). Interestingly, they employed triazene 77 as an internally cleavable directing group.47 The kinetic isotope effects suggested that the alkyne insertion step is rate limiting for the second C–N annulation.
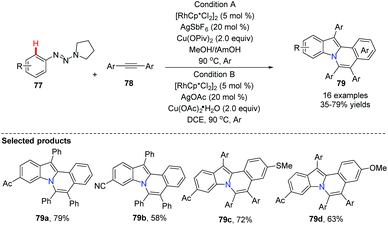 |
| Scheme 18 Synthesis of indolo[2,1-a]isoquinolines via a triazene-involved C–H annulation cascade. | |
Li and co-workers pioneered the study of Rh-catalyzed double oxidative coupling of primary benzamides and 2.0 equiv. of symmetrical alkynes,48 providing the bridgehead nitrogen heterocycle 82 in good to excellent yields (71–93%) via double C–H activation and oxidative coupling (Scheme 19). Since isoquinolones were prepared from secondary benzamides and alkynes under the almost identical conditions, this method was found to be a valuable improvement for building novel bridgehead nitrogen heterocycles from quinazolones or dihydroquinazolinones with alkynes.
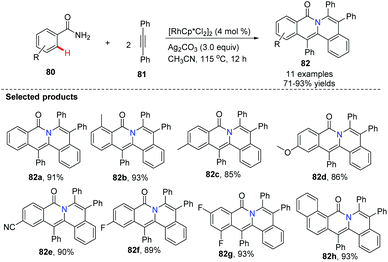 |
| Scheme 19 Rh(III)-Catalyzed double oxidative coupling between primary benzamides and alkynes. | |
Recently, a novel approach to bridgehead nitrogen-fused indolones has been developed by Cheng's group (Scheme 20).49 A rhodium-catalyzed reaction of aroyl sulfoxonium ylides 83 and anthranils 84 proceeded well to build three bonds in one pot, giving desired products 86 in moderate to good yields. A tentative mechanistic study suggested that the catalytic cycle involves Rh promoted C–H activation and ortho-amination of α-aroyl sulfoxonium ylides. Finally, the second indole ring was constructed via intermediate 85 by the Aldol condensation.
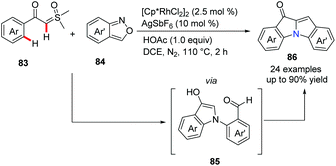 |
| Scheme 20 Rh-Catalyzed coupling reaction of sulfoxonium ylides and anthranils. | |
Li and co-workers developed a Rh-catalyzed oxidative coupling of 5-aryl-1H-pyrazoles 87 with diphenyl-acetylenes 88.50 In this transformation, pyrazoles acted as an ideal directing group to facilitate C–H bond activation to access novel heterocycles with good to excellent yields, although only symmetrical alkynes were studied (Scheme 21).
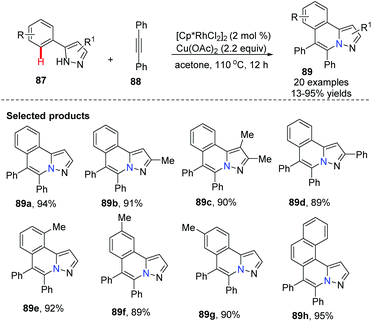 |
| Scheme 21 Rh(III)-Catalyzed C–C/C–N coupling between aryl-pyrazoles with alkynes. | |
Imidazo[2,1-a]isoquinolines are bridgehead nitrogen fused heterocycles found in many drugs and bioactive compounds. In 2018, Song et al. reported an efficient and practical approach via Rh(III)-catalyzed [4 + 2] annulation of 2-arylimidazoles 90 and α-diazoketoesters 91 (Scheme 22).51 Obviously, under acidic reaction conditions, the decarboxylation products 92 were obtained in good to excellent yields when t-butyl ester was used.
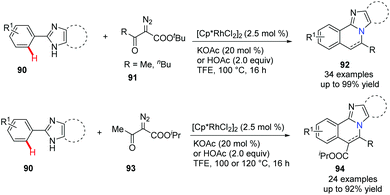 |
| Scheme 22 Rh(III)-Catalyzed annulation of 2-arylimidazoles with diazoketoesters to access imidazoisoquinolines. | |
It is well known that benzoazepine derivatives are valuable compounds with significant biological and pharmaceutical activities. Meanwhile, imidazole is an often-found essential scaffold in the drug discovery program. In 2019, Zhang, Fan and their co-workers developed a novel and efficient method for combining both benzoazepine and imidazole pharmacophores together.52 The Rh(III)-catalyzed C(sp2)–H activation and functionalization of 2-phenylimidazole and methyleneoxetanone via [4 + 3] annulation gives the desired coupling products, fused heterocycles 97, in moderate to good yields (Scheme 23). This is a reliable and atom-economical approach from simple and inexpensive starting materials and is expected to find wide application.
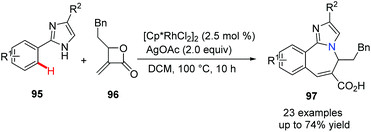 |
| Scheme 23 Rh(III)-Catalyzed C–H functionalization and annulation to prepare benzoazepine using methyleneoxetanone. | |
Sulfoxonium ylides have been introduced recently as convenient carbene precursors which are much safer compared with the analogous diazo compounds. In 2018, Ellman et al. developed a Rh(III)-catalyzed coupling reaction between C-alkenyl azoles and sulfoxonium ylides and in situ cyclodehydration gave substituted bridgehead nitrogen-fused [5,6]bicyclic heterocycles 100 with complete regioselectivity (Scheme 24).53 A proposed mechanism suggests that the C–H functionalizations of C-alkenyl azoles and sulfoxonium ylides were involved.
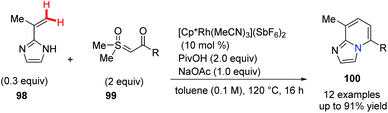 |
| Scheme 24 Rh(III)-Catalyzed C–H functionalization of C-alkenyl azoles with sulfoxonium ylides. | |
Diazepine and quinoxaline are the most important privileged scaffolds in drug discovery. Recently, Sun et al. developed a powerful and versatile approach to these bridgehead nitrogen fused heterocycles.54 Notably, under the Rh(III) catalyst, the annulation of indolo anilines and diazo compounds proceeded well via the free amine assisted C–H activation, followed by amidation leading to the diazepino[1,7-a]indole 104 or 105 in good to excellent yields. On the other hand, when Ru(II) was used as the catalyst, the coupling reaction involves the formation of a Ru–carbene complex, followed by –NH2 group insertion and cascade cyclization via a metallo-ene type reaction, a further β-hydride elimination, giving the indolo[1,2-a]quinoxaline 106 or 107 as the major product in good to excellent yields (Scheme 25).
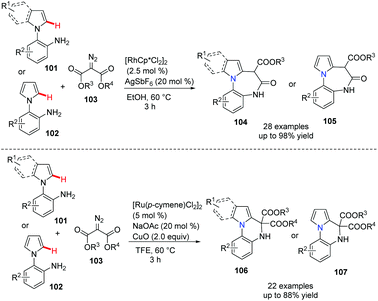 |
| Scheme 25 Rh(III) or Ru(II)-controlled divergent annulation to diazepine and quinoxaline derivatives. | |
In 2017, Ellman's group disclosed a novel approach to synthesize privileged [5,6]-bicyclic heterocycles 110 with bridgehead nitrogen by Rh(III)-catalyzed C–H activation of C-alkenyl azoles (Scheme 26).55 Since one of the reactants can be alkenyl imidazoles, pyrazoles, or triazoles, it could provide the heterocycle products with nitrogen incorporated at different sites. On the other hand, the coupling partners, such as alkyne and diazoketone, also give azolopyridines with various substitution patterns which make the new method very attractive for medicinal chemistry.
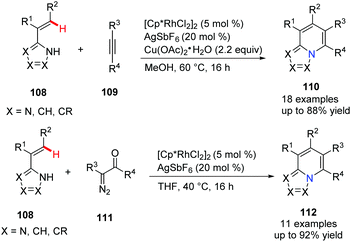 |
| Scheme 26 Rh(III)-Catalyzed C–H activation of C-alkenyl azoles to access bicyclic bridgehead nitrogen heterocycles. | |
In 2020, Li, Wu and co-workers reported a novel and practical approach to benzimidazo[1,2-a]quinolones 115.56 The cascade reaction from imidamides and anthranils involves a Rh(III)-catalyzed C–H activation, followed by a intermolecular amination and cyclization process (Scheme 27).
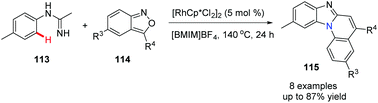 |
| Scheme 27 Rh(III)-Catalyzed C–H activation to access benzimidazoquinolines. | |
Bicyclic [5,6]-bridgehead nitrogen fused heterocycles are privileged pharmacophores as FDA-approved drug and clinical candidates. In 2018, Ellman and his group first reported Rh(III)-catalyzed imidoyl C–H activation for the preparation of substituted azolopyrimidines 121 (Scheme 28).57 The annulations of N-azolo imines with sulfoxonium ylides, diazoketones and alkynes gave the desired bridgehead nitrogen fused heterocycles in good to excellent yields. This novel approach could have a valuable application in drug discovery.
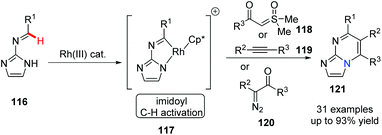 |
| Scheme 28 Synthesis of pyrazolopyrimidines via Rh(III)-catalyzed imidoyl C–H activation. | |
Three-component coupling reactions from aldehydes, aminopyrazoles and newly readily available sulfoxonium ylides enable access to complex molecules under straightforward conditions with a short reaction time. Ellman's group further developed a successful Rh(III)-catalyzed protocol to the general synthesis of many pyrazolo[1,5-a]pmidines 125 in good yields (Scheme 29).58 Notably, good functional group compatibility is an great advantage for this versatile and efficient transformation, and it was suggested that Rh(III)-catalyzed imidoyl C–H activation was involved in annulation (Scheme 30). On the other hand, in a one-pot, stepwise reaction, formyl ylide 126 was first utilized smoothly.
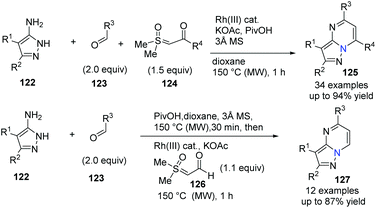 |
| Scheme 29 Three-component coupling of aminopyrazoles, aldehyde and sulfoxonium ylides to access pyrazolopyrimidines. | |
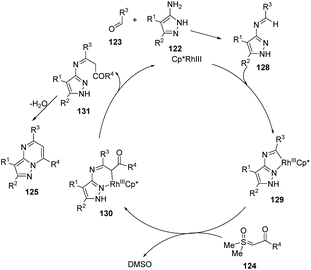 |
| Scheme 30 Proposed mechanism for annulation. | |
Imidazo[1,2-c]quinazolines are widely present in drug molecules. Several methods that utilized substituted anilines as the starting materials have been developed to build these scaffolds. Different from these known methods, Cheng reported a Rh(III), and Ag(I)-co-catalyzed annulation via ortho-C–H activation of 2-arylimidazoles (Scheme 31).59 A series of fused heterocycles 134 were prepared in good to excellent yields from simple and readily available 2-arylimidazoles 132 and 1,4,2-dioxazol-5-ones 133 which provided rhodium carbene species by the extrusion of CO2.
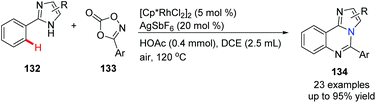 |
| Scheme 31 Rh(III)Ag(I)-cocatalyzed annulation via C–H activation of arylimidazoles. | |
2.4. Ruthenium-catalyzed synthesis of bridgehead nitrogen heterocycles
Yi and co-workers reported a cationic ruthenium–hydride complex for C–H activation of benzocyclic amines to access tricyclic compounds 137.60 Substituted quinolines and quinoxalines could be formed via the N–H and C(sp2)–H functionalization reaction of benzocyclic amines and terminal alkynes (Scheme 32).
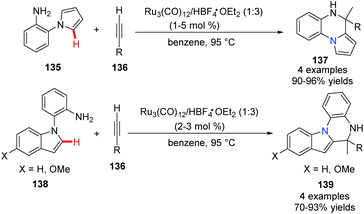 |
| Scheme 32 Coupling reaction of benzocyclic amines and alkynes to form tricyclic heterocycles. | |
In 2012, Ackermann and co-workers reported using 5 mol% of cationic ruthenium(II), 20 mol% AgSbF6 as a promoter, and Cu(OAc)2·H2O/air as the oxidant to promote an oxidative alkyne annulation process with substituted 1H-pyrazoles (Scheme 33).61 This method generated the bridgehead heterocycles 142 from readily available starting materials with high functional group tolerance.
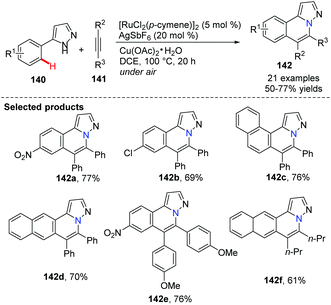 |
| Scheme 33 Oxidative alkyne annulations with pyrazoles. | |
In 2014, Peng and co-workers applied the ruthenium-catalyzed oxidative cross-coupling/annulation of quinazolones with symmetric alkynes to the synthesis of 8H-isoquinolino[1,2-b]quinazolin-8-one derivatives 145 (Scheme 34).62 After screening of reaction conditions we found that using 2.2 equiv. of Cu(OAc)2 as the oxidant, and 2.0 equiv. of Na2CO3 as the base in toluene at 90 °C for 16 h provided the best results for these fused polycyclic heteroarenes with good to excellent yields, although moderate regioselectivities were obtained when unsymmetrical alkynes were used as reactants.
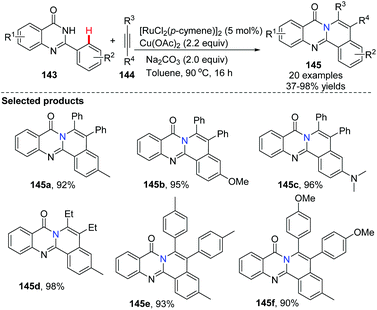 |
| Scheme 34 Ru-Catalyzed cross-coupling/annulations of quinazolones with alkynes. | |
Slightly different from Peng's approach, Nagaiah demonstrated Ru-catalyzed aerobic oxidative dehydrogenation followed by cross-coupling/annulation of dihydroquinazolinones 146, instead of the use of quinazolinones.63 The method avoided the use of Cu(OAc)2 as the oxidant and purification of intermediates. This synthetic method provided a facile route to access a class of highly functionalized, N-fused polycyclic compounds 148. When unsymmetrically, disubstituted alkynes were used, two regioisomeric products were obtained (Scheme 35).
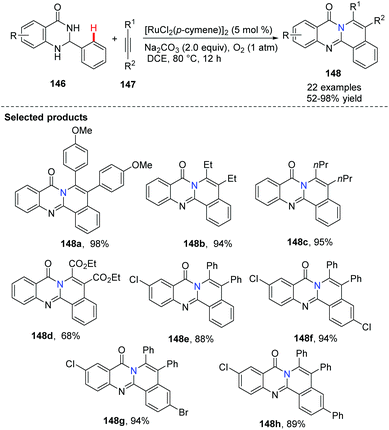 |
| Scheme 35 Amide-directed cross-coupling/annulation. | |
The use of N-arylphthalazine-1,4-dione 148 to construct bridgehead nitrogen fused and functionalized phthalazines has received some investigation recently. It was reported that a Ru-promoted oxidative alkenylation of N-aryl pyridazinediones and N-aryl phthalazinediones with acrylates resulted in indazole derivatives 151 in good to excellent yields (Scheme 36).64 In addition, it was found that Rh(III)-catalyzed annulations of N-arylphthalazine-1,4-dione with α-diazo carbonyl compounds gave either the [4 + 1] or [4 + 2] annulation product, depending on the additive in the reaction conditions.
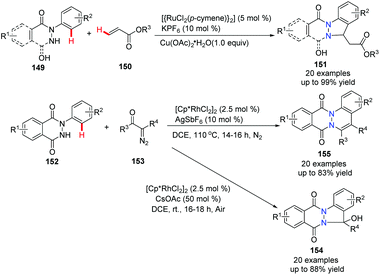 |
| Scheme 36 Ru(II) or Rh(III)-catalyzed annulation of N-arylphthalazinedione with acrylates or diazo carbonyl compounds. | |
Similarly, under different reaction conditions, using either Ru(II) or Ir(III) catalyst for C–H activation and annulation with 1,3-diketone-2-diazo compounds, N-arylphthalazine-1,4-diones were converted to phthalazinocinnolinediones 158 in good to excellent yields, and phenylquinazolinones were transformed to isoquinolinoquinazolinones under mild conditions respectively (Scheme 37).65
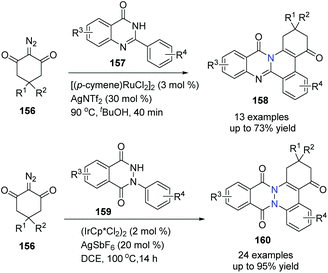 |
| Scheme 37 Ru(II)/Ir(III)-catalyzed C–H bond activation/annulation of cyclic amides to prepare bridgehead nitrogen heterocycles. | |
2.5. Iridium-catalyzed synthesis of bridgehead nitrogen heterocycles
The Sames group successfully demonstrated a novel intramolecular oxidative cross-coupling of C(sp3)–H bonds and alkenes under neutral and iridium-catalyzed conditions (Scheme 38).66 It is noteworthy that when optically pure proline derivative 163 serves as the starting material, it gave bridgehead nitrogen heterocycle 164 with excellent chiral purity (>99% ee).
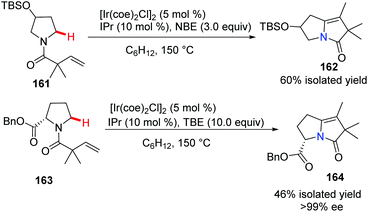 |
| Scheme 38 Iridium-catalyzed oxidative cyclization. | |
The indole skeleton exists in various biologically active natural products and medicines, therefore much effort has been expended to develop novel and catalytic approaches via C(sp2)–H bond activation. Ellman and Bergman pioneered the studies of the Rh-catalyzed enantioselective reaction using N-allylindoles to access N-fused bridgehead tricycles with chiral phosphoramidites as ligands.67 Recently, Shibata and co-workers developed an intramolecular and highly enantioselective C–H activation of N-alkenylindoles that utilized iridium.68 A para-anisoyl group at the 3-position of the indoles operated as an efficient directing group (see 166a to 166d), and the combination of cationic iridium(I) and chiral diphosphine ligands achieved excellent desired product 166 with up to 97% ee (Scheme 39).
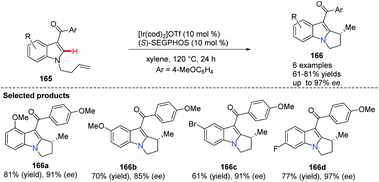 |
| Scheme 39 Intramolecularalkylations of N-alkenylindoles at the C-3 position using the directing group approach. | |
Sulfoxonium ylides have recently received increased attention; they serve as novel carbene surrogates catalyzed by transition metals for the rapid construction of bridgehead nitrogen heterocycles. In 2019, Aïssa and his group found that when catalyzed by an iridium catalyst, without the assistance of a directing group, chemo-specific cyclization of α-carbonyl sulfoxonium ylides 167 for the functionalization of the pyrrolic C–H bond occurred, giving bicyclic 5–5 and 5–6 systems 168 in good to excellent yields (Scheme 40) respectively.69
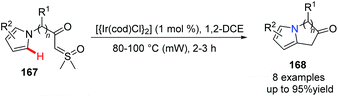 |
| Scheme 40 Ir-Catalyzed functionalization of the pyrrolic-C–H bond to prepare bridgehead nitrogen heterocycles. | |
The use of α-diazo carbonyl compounds for Rh-, Ru- or Pd-catalyzed carbene insertion/cyclization has been investigated by many academic labs. Although the Ir-catalyzed carbenoid functionalization has been reported for the synthesis of heterocycles, its use to access bridgehead nitrogen fused polycycles is limited. In 2018, Sakhuja et al. reported an Ir-promoted annulation of 1-arylindazolones with α-diazo carbonyl compounds, providing indazolone-fused cinnolines 172 in good to excellent yields (Scheme 41).70 Obviously, the Ir(I) species facilitates the N–H oxidative addition of 1-arylindazolone and affords the five-membered Ir(III) intermediate 171 which reacts with α-diazo carbonyl compounds, to generate the final desired product.
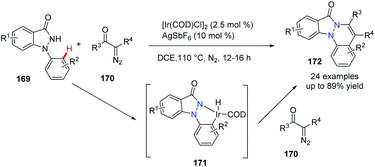 |
| Scheme 41 Ir-Catalyzed annulation to access indazolone-fused cinnolines. | |
2.6. Cobalt-catalyzed synthesis of bridgehead nitrogen heterocycles
Yoshikai and co-workers reported a cobalt-N-heterocyclic carbene (NHC)-catalyzed intramolecular hydroarylation reaction on an indole to give dihydro-pyrrolo-indole and tetrahydropyridoindole derivatives (Scheme 42).71 Notably, by careful choice of the NHC ligands, the selective formation of 5-exo174 and 6-endo product 175 was controlled well. This method provided an efficient route to access a class of highly functionalized tricyclic compounds with good yields and regioselectivity.
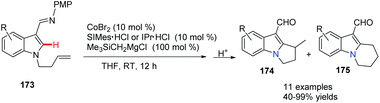 |
| Scheme 42 Intramolecular hydroarylation leading to dihydropyrrolo-indole and tetrahydropyridoindole. | |
The authors proposed that the reaction was initiated by chelation-assisted oxidative addition of the C–H bond to a cobalt species to give key intermediate 176. Subsequent insertion of the olefin into the Co–H bond leads to either six-membered 178 or seven-membered 180 intermediates, which undergo reductive elimination to give the title compound 174 or 175. The olefin insertion step is probably the regioselectivity determining step of the reaction (Scheme 43).
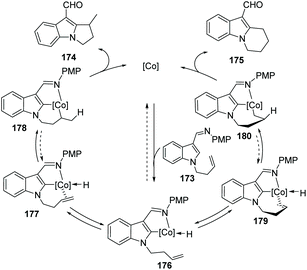 |
| Scheme 43 Proposed reaction mechanism. | |
Kanai and co-workers recently innovated the utility of a cationic, high-valent cobalt(III) complex for the C(sp2)–H bond functionalization of N-carbamoyl indoles 181.72 The C-2 indole alkenylation/annulation sequence proceeded smoothly in the presence of a Cp*Co(III) complex, giving the corresponding pyrrolo-indolone compounds 183 in 58–89% yields (Scheme 44).
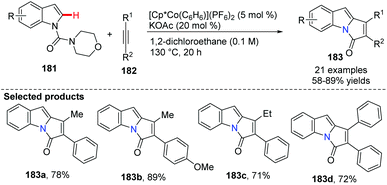 |
| Scheme 44 Directed C–H alkenylation/annulation. | |
Glorius and co-workers accomplished a cobalt(III)-catalyzed directed C(sp2)–H functionalization of various 2-arylpyridines 184 with carbene precursors 185 in 2015.73 The dual role of cobalt(III) catalyst as a transition metal and a Lewis acid might be vital to the C–H activation step and sequential cyclization (Scheme 45). This method provided novel bridgehead nitrogen heterocycles 186 efficiently with broad structural diversity, as the ortho-pyridine substrates are crucial directing groups for the arene C–H bond activation.
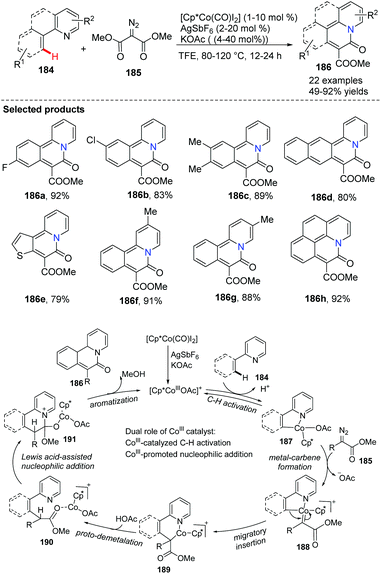 |
| Scheme 45 Directed C–H bond functionalization of 2-arylpyridines with carbene precursors and a putative mechanism. | |
2.7. Nickel-catalyzed synthesis of bridgehead nitrogen heterocycles
Hiyama and co-workers reported intramolecular cyclization of alkenyl pyridone derivatives 192 using Ni(cod)2/AlMe3 (as a Lewis acid) catalysis in 2009.74 The intramolecular addition proceeded mainly in an exo-trig fashion to afford predominantly bicyclic substituted pyridone 193 along with the minor isomer 194 in combined high yields and good regioselectivity (Scheme 46).
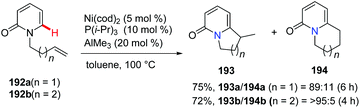 |
| Scheme 46 Intramolecular alkenylation leading to bicyclic pyridone derivatives. | |
2.8. Silver-catalyzed synthesis of bridgehead nitrogen heterocycles
Wu and co-worker described a silver and copper co-catalyzed three-component reaction of 2-alkynylbenzaldehyde, sulfonohydrazide, and a tertiary amine, which provides a novel and efficient route for the generation of H-pyrazolo[5,1-a]isoquinolines 198 (Scheme 47).75 The reaction process involved the following steps: (i) Silver-catalyzed cyclization of 2-alkynylbenzaldehyde and sulfonohydrazide. (ii) Copper-catalyzed oxidation of an aliphatic C–H bond of tertiary amines, and subsequent intermolecular cyclization/aromatization. The reaction occurs under mild conditions and provides excellent results employing simple operations.
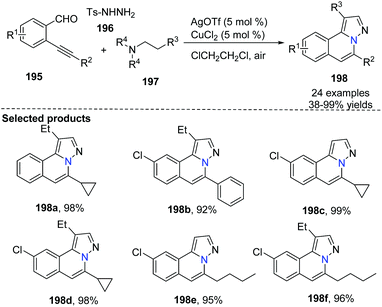 |
| Scheme 47 Synthesis of H-pyrazolo[5,1-a]isoquinolines catalyzed by Ag(I)/Cu(II). | |
An example applied to N-iminoisoquinolinium ylides, which generated in situ ylides as directing groups for further C–H activation transformations, was developed by Chen, Peng and co-workers in 2012.76 Without using a palladium(II) catalyst, they described a catalytic pathway in which silver triflate (10 mol%) and copper iodide (10 mol%) co-promoted tandem alkynyl active cyclization of N-iminoisoquinolinium ylides and bromoalkynes to form H-pyrazolo[5,1-a]isoquinolines 202 in good yields under mild reaction conditions (Scheme 48). The C–H bond activation mechanism is given in Scheme 49, suggesting that N-iminoisoquinolinium ylides are ideal building blocks for the synthesis of novel bridgehead nitrogen heterocycles.
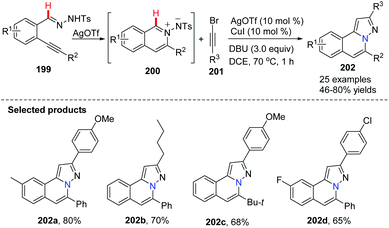 |
| Scheme 48 AgOTf/CuI co-catalyzed tandem alkynylative cyclization reaction to form H-pyrazolo[5,1-a]isoquinolines. | |
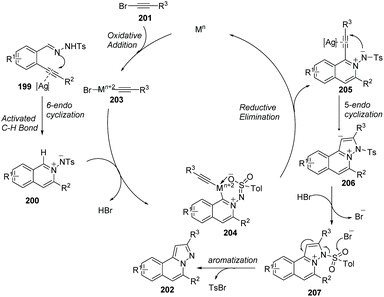 |
| Scheme 49 Plausible mechanism involved the formation of N-iminoisoquinolinium ylides and C–H activation. | |
It should be noted that construction of benzimidazo-isoquinoline fused polycycles via radical type cyclization is not highly efficient. Recently, Wei, Yu and their co-workers reported a silver-catalyzed decarboxylative radical cascade cyclization.77 In the presence of K2S2O8/AgNO3, the reaction of N-methacryloyl-2-phenylbenzoimidazole 208 with carboxylic acids 209 gave desired heterocycle 211 under mild reaction conditions (Scheme 50). Based on the mechanistic study, it is believed that radical intermediate 210 was generated after releasing CO2 from the corresponding carboxylic acid, followed by the addition of the reactive radical to the aromatic C
C bond.
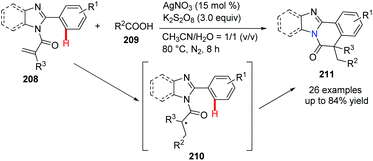 |
| Scheme 50 Silver salt promoted decarboxylative radical cyclization to access bridgehead nitrogen heterocycles. | |
2.9. Copper-catalyzed synthesis of bridgehead nitrogen heterocycles
Metal-catalyzed modern C–C cross coupling reactions and C–N bond forming reactions, such as the modern Ullmann reaction and palladium or copper-catalyzed C–H functionalization, have provided a powerful tool for the synthesis of nitrogen-containing heterocycles in a novel and simple way. In contrast to palladium, rhodium, nickel and other transition metals, copper and copper salts are inexpensive and exhibit relatively low toxicity. Over the past decade, modern organocopper chemistry has developed from stoichiometry to the current catalytic processes. It was found that nitrogen-containing ligands can increase the catalytic activity, solubility and stability of the copper–amine complex which serves to promote catalytic reaction, shifting these complexes from heterogeneous to homogeneous catalysis. Modern organocopper chemistry has become a toolbox for chemists in all areas (pharmaceutical, materials science, dyes, etc…).
Tomás and co-workers exploited a copper(I)-catalyzed regioselective cyclization via addition of vinyldiazoacetates 213 to electron-deficient pyridines 212,78 leading to indolizine derivatives 214 with several reaction features (Scheme 51), including mild reaction conditions and a simple set up with inexpensive and readily available materials. A proposed mechanism is illustrated in Scheme 52. Initially, copper(I) alkenyl carbene 215 reacts with pyridine via Michael 1,4-addition, forming activated pyridine intermediate dipole 216, which subsequently undergoes cyclization to generate the copper(III) metallacycle 217. Metallacycle 217 suffers from reductive elimination forming a new C–C double bond, producing the Cu(I) complex 218 Finally, with the release of CuBr the desired product 219 is delivered to end the catalytic cycle. Notably, when simple benzo-fused pyridines, such as quinoline and isoquinoline, were used as the starting materials, they reacted with vinyldiazoacetate 213, affording pyrrolo[1.2-a]quinolone 214g and pyrrolo[2,1-a]isoquinoline 214h in good yields.
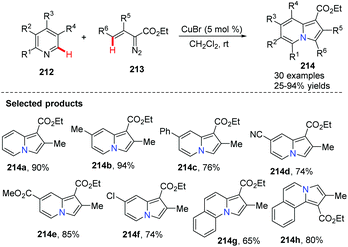 |
| Scheme 51 Synthesis of indolizine derivatives. | |
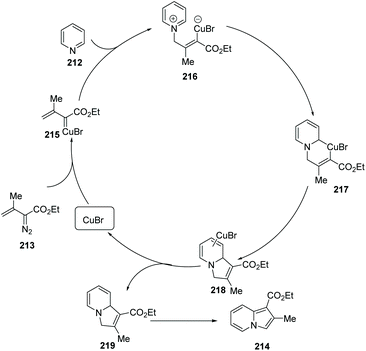 |
| Scheme 52 Proposed mechanism for the Cu(I) catalyzed synthesis of indolizine derivatives. | |
Utilizing Cu(I) and Ag(I) as catalysts, a highly efficient route for the synthesis of novel pyrrolo or indolo[1,2-a]quinolones 223 was developed from gem-dibromovinyls 220 and sulphonamides 221 (Scheme 53).79 It is worth mentioning that, for the cyclization process, the Csp2–H bond of ortho five-membered heterocycles does not require prefunctionalization. This method is simple, convenient and more efficient as it can be accomplished in a one-pot fashion in a short reaction time with diminished catalyst loading.
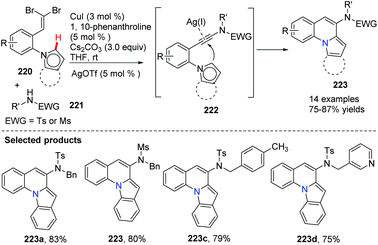 |
| Scheme 53 Synthesis of pyrrolo-/indolo[1,2-a]quinolines. | |
The group of Jia reported an indolizine synthesis by oxidative cross-coupling and cyclization of 2-(pyridin-2-yl)acetate 224 and alkenes 225via a Cu(OAc)2/I2 mediated reaction (Scheme 54).80 The control experiment using TEMPO as a radical scavenger suggested that a radical pathway is likely and a plausible mechanism is depicted in Scheme 55. A series of 1,3-di- and 1,2,3-trisubstituted indolizines 226 were easily prepared in modest to excellent yields.
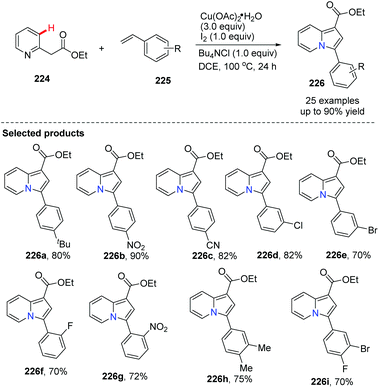 |
| Scheme 54 Synthesis of indolizine via oxidative cross-coupling/cyclization. | |
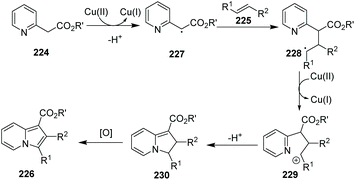 |
| Scheme 55 Plausible mechanism to form substituted indolizine. | |
Some bioactive natural products and pharmaceuticals contain the pyrrolo[1,2-a]indole core. To build this privileged structural motif, Zhou, and Deng et al. developed an environmentally benign approach to access these valuable molecules from readily available indole/tryptamine 231 and β-arylacrylic acids 232 (Scheme 56).81 This oxidative coupling reaction, a formal Michael addition and dehydration occurring in the presence of catalytic CuBr2 in CH3CN under air, turned out to be mild and scalable. This method is likely to be a powerful and versatile method for the synthesis of a large number of indole alkaloids.
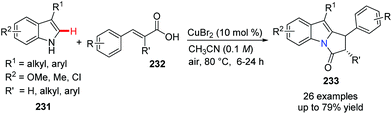 |
| Scheme 56 Cu(II)-Catalyzed oxidative coupling between indole/tryptamine and arylacrylic acids. | |
Imidazopyridine is an important pharmacophore, and it is found in many biologically active compounds. Although a variety of synthetic approaches have been developed, most of them are limited in scope and require multi-steps, have low yields, or have difficult isolations and purifications. Therefore, investigation of straightforward and green methods for the synthesis of imidazopyridines from simple and readily available starting materials is greatly desired. By using 20 mol% of Cu(OAc)2 and 10 mol% of Fe(NO3)3 as co-catalysts, without prefunctionalization of the starting material, a novel and efficient synthesis of pyrido[1,2-a]benzimidazoles 235 through direct C–H activation of N-aryl-2-aminopyridines 234 was elegantly demonstrated initially by Zhu and co-workers (Scheme 57)82 who showed that a direct intramolecular C(sp2)–H amination is practical and effective. This method should have great potential in drug research and development because the raw material N-aryl-2-aminopyridines 234 can be easily prepared from halopyridines and phenylamine.
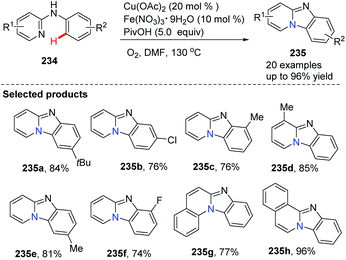 |
| Scheme 57 Efficient synthesis of pyrido[1,2-a]benz-imidazoles via direct intramolecular C(sp2)–H amination. | |
The proposed mechanism is depicted in Scheme 58: beginning with pyridyl nitrogen attack and the formation of a Cu(II) adduct 236 which then undergoes oxidization to afford more electrophilic Cu(III) intermediate 237. Afterwards, six-membered ring transition state 238 is generated and the elimination of the aromatic ring protons gives a reactive Cu(III) intermediate 239. Reductive elimination rapidly delivers the product 235.
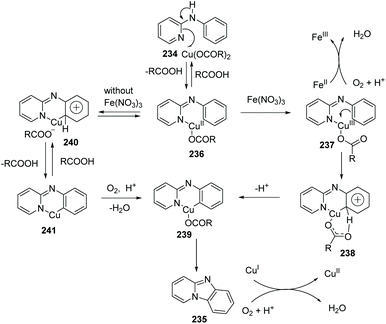 |
| Scheme 58 Proposed mechanism catalyzed by Cu(II)/Fe(III) salt. | |
In 2011, Zhu, Zhang and co-workers further exploited and developed a copper-catalyzed intramolecular dehydrogenative amino-oxygenation.83 From readily available N-allyl-2-aminopyridines and using molecular oxygen as the oxidant, they quickly and effectively prepared imidazol[1,2-a]pyridines 243. Notably, aldehyde functional groups survive without further oxidation to the corresponding acids (Scheme 59).
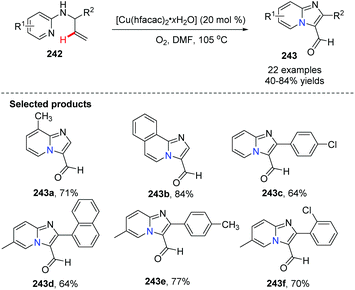 |
| Scheme 59 Synthesis of imidazo[1,2-a]pyridine-3-carbaldehyde via Cu(II) catalyzed dehydrogenative aminooxygenation. | |
A plausible mechanism is displayed in Scheme 60. The process is initiated by coordination of 242 with the copper(II) catalyst to form 244. Single-electron transfer then occurs from Cu to O2 to generate the peroxy–copper(III) intermediate 245, which undergoes insertion into the carbon–carbon double bond to form an alkyl copper(III) 246. Isomerization of the resulting exocyclic peroxy–copper(III) intermediate yields the copper(II) 247 with concurrent formation of a carbon–oxygen bond. Elimination of Cu(II)–OH releases aldehyde 248, which undergoes spontaneous aromatization to produce desired 243.
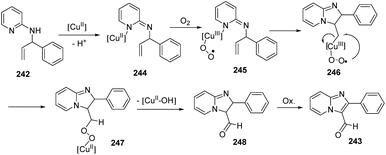 |
| Scheme 60 Plausible mechanism involved the Cu(II) catalyzed C–H activation. | |
Different from an intramolecular cyclization approach developed by Zhu's group, Hajra and co-workers reported a copper-catalyzed intermolecular cyclization reaction, as an alternative approach for the synthesis of imidazo[1,2-a]pyridines from readily available 2-aminopyridines and acetophenone in 2013 (Scheme 61).84 The reaction was performed using 1,10-phenanthroline as the ligand and ZnI2 as the additive in 1,2-dichlorobenzene, by heating in the presence of air. Using this method, zolimidine, a marketed antiulcer drug was prepared. A probable mechanism is shown in Scheme 62. Initially, imine 254 is formed by ZnI2 as a catalyst. The imine transforms to enamine 255 after tautomerization, reacting with Cu(OAc)2 to form the adduct 256, which is then readily converted into intermediate 257. Deprotonation of 257 will give 258, which is then oxidized to the reactive [Cu(III)] intermediate 259. Finally, product 251 is afforded through reductive elimination along with the formation of Cu(I) species which is oxidized by the aerobic oxygen to close the catalytic cycle.
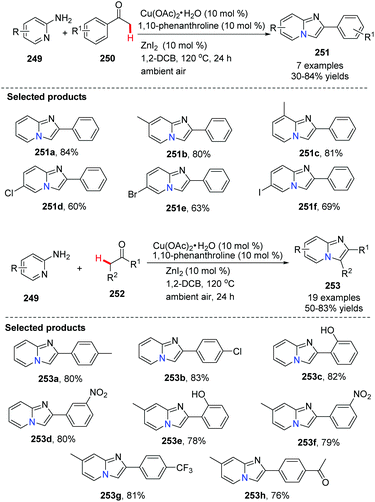 |
| Scheme 61 Synthesis of imidazo[1,2-a]pyridines. | |
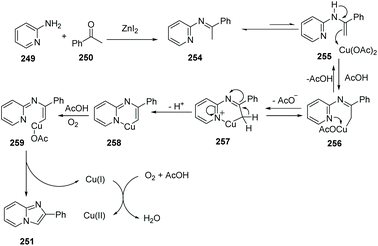 |
| Scheme 62 Probable mechanism for the synthesis of imidazo[1,2-a]pyridines. | |
Maes and co-workers reported an approach for the synthesis of pyrido[1,2-a]benzimidazoles 261 from N-phenylpyridin-2-amine 260 using Cu(OAc)2 as the catalyst in 2010 (Scheme 63).85 Reactions were performed under an atmosphere of O2, a solvent of DMSO, using a catalytic amount of 3,4,5-trifluorobenzoic acid (TFBA) as the promoter. During the catalytic cycle, reductive elimination of RCO2H from RCO2Cu(II)H yields Cu(0) (Scheme 64).
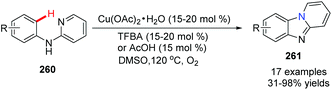 |
| Scheme 63 Synthesis of pyrido[1,2-a]benzimidazoles. | |
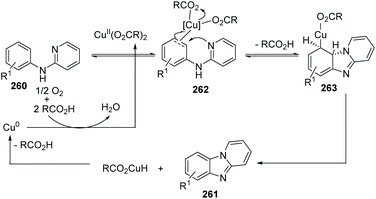 |
| Scheme 64 Proposed mechanism for the intramolecular C–H amination. | |
In 2010, Gevorgyan and co-workers developed a highly efficient method for the synthesis of imidazo[1,2-a]pyridine, imidazoquinoline and imidazoisoquinoline derivatives 267 by the copper-catalyzed three-component coupling reaction of aryl-, heteroaryl-, and alkylaldehydes with 2-aminopyridines 264 and terminal alkynes (Scheme 65).28 This reaction has been applied in a highly efficient one-pot synthesis of alpidem and zolpidem. Recently, for drug-like molecules, the use of C–H functionalization is becoming more and more important for diversification and for generating new drugs, and it should be a key transformation kept in the medicinal chemists’ toolbox.
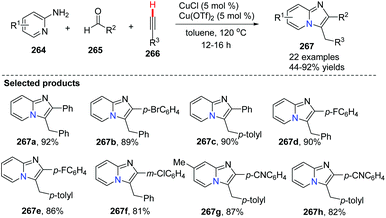 |
| Scheme 65 Copper-catalyzed one-pot synthesis of Alpidem and Zolpidem. | |
In addition to the Hajra catalytic system [Cu(II)/ZnI2/1,10-phen] for tandem imine formation-oxidative cyclization, Zhang, Su and co-workers applied a CuI-catalyzed aerobic oxidative α-amination cyclization of 2-aminopyridine and acetophenone (or unsaturated ketones) to provide [1,2-a]pyridines in 2013 (Scheme 66).86 By carefully screening reaction conditions, they found that adding 1 mol% of In(CF3SO3)3 as an additive was necessary. Without In(III) salt or CuBr2 as an additive, the reaction was not productive when NMP was used as a solvent.
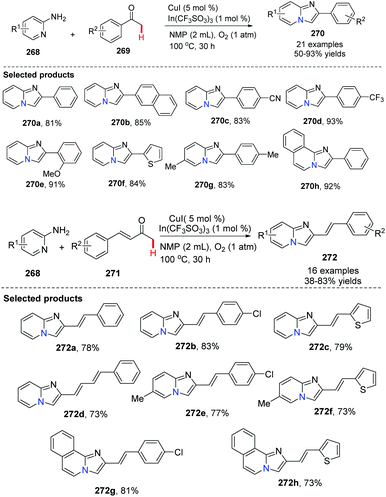 |
| Scheme 66 Synthesis of substituted imidazoheterocycles catalyzed by CuI. | |
Unlike the oxidative cyclization of arylketones with 2-aminopyridines to access imidazopyridine which is heavily being investigated and developed by two research groups, an alternative disconnection method to build the imidazopyridine core uses the pyridine and an acylated oxime. In 2013, Jiang et al. reported the novel conversion of pyridine to imidazo[1,2-a]pyridines via copper-catalyzed aerobic dehydrogenative cyclization with ketone oxime esters (Scheme 67).87 Other N-containing heteropolycycles, such as isoquinoline, also worked well in this transformation. The possible mechanism is proposed, which includes the formation of a Cu(III)-imino species 280 which is proposed to undergo reductive elimination of a six-membered Cu(III) intermediate 284. Copper-catalyzed pyridine C(sp2)–H bond and oxime ester C(sp3)–H bond activation provides the by-products H2O and CH3COOH (Scheme 68).
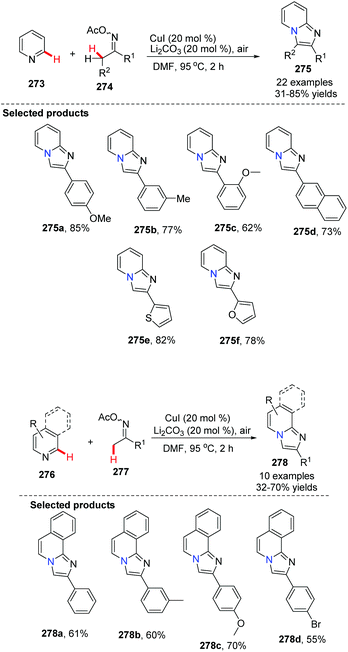 |
| Scheme 67 Conversion of pyridine to imidazo[1,2-a]pyridines. | |
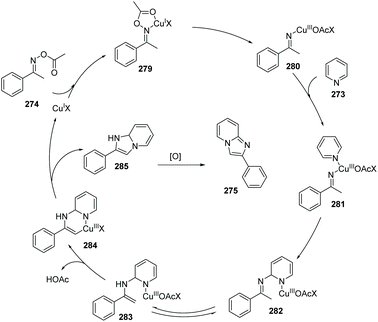 |
| Scheme 68 Plausible catalytic cycle mediated by CuI. | |
Similar to Jiang's method, and concurrent with his findings, in 2013, Fu and co-workers developed another novel and efficient copper-catalyzed aerobic oxidative C(sp2)–H functionalization of substituted pyridines with N-(alkylidene)-4H-1,2,4-triazol-4-amines, generating imidazo[1,2-a]pyridine derivatives 288 (Scheme 69).88 First, the isomerization of N-(alkylidene)-4H-1,2,4-triazol-4-amine gives 289, then coordination of the copper catalyst (CuI) provides complex 290 with the liberation of HI, afterwards treatment of 290 with HI leads to 291. Nucleophilic attack of substituted pyridine on the alkenyl moiety in 291 affords 293 with the elimination of 4H-1,2,4-triazole, and intramolecular cycloaddition, isomerization, and the final oxidation lead to the target product 288 with regeneration of the catalyst CuI (Scheme 70).
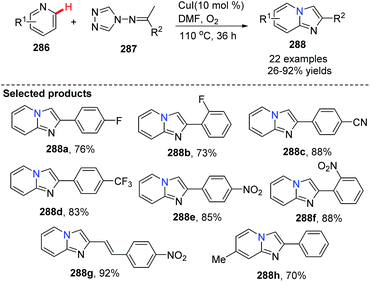 |
| Scheme 69 Copper-catalyzed aerobic oxidative synthesis of imidazo[1,2-a]pyridines. | |
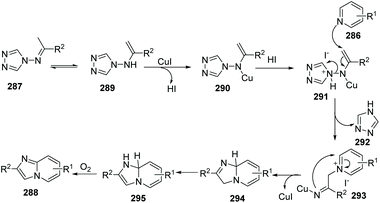 |
| Scheme 70 Possible mechanism for the Cu(I)-catalyzed synthesis of imidazo[1,2-a]pyridines. | |
In 2011, the group of Xi described CuI-catalyzed tandem coupling of 1,4-dihalo-1,3-dienes 297 with readily available azoles 296via N–H bond and C–H bond activation for the synthesis of nitrogen-bridgehead fused heterocycles 298 (Scheme 71).89 They detailed the reaction stepwise pathway via a designed experiment and found that the N–H bond was activated preferentially for the first N-alkenylation, followed by a subsequent C(sp2)–H bond alkenylation on imizole. This method provided an efficient route to the synthesis of azolopyridine derivatives.
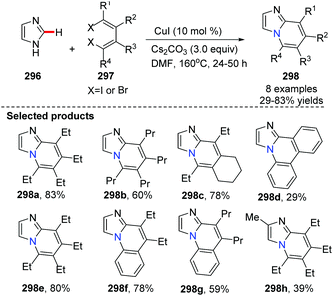 |
| Scheme 71 Tandem synthesis of substituted azolopyridines catalyzed by CuI. | |
In 2013, Jiao and co-workers independently updated a CuI (10 mol%) and Ag2CO3(10 mol%) co-catalyzed direct dehydrogenative annulation of N-iminopyridinium ylides with terminal alkynes using oxygen as the oxidant, leading to pyrazolo[1,5-a]pyridine derivatives (Scheme 72).90
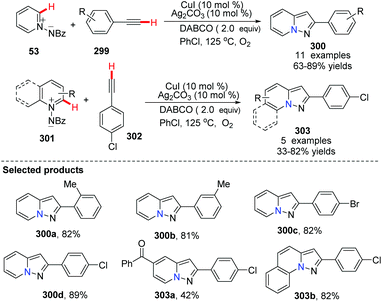 |
| Scheme 72 Copper(I)/Ag2CO3-catalyzed oxidative annulation of N-iminopyridinium ylides. | |
Fu and co-workers developed an efficient copper-catalyzed aerobic oxidative, intramolecular alkene C–H amination leading to N-heterocycles 305 utilizing cheap and readily available Cu(O2CCF3)2 as the catalyst in 2011.91 This method provided a new and useful strategy for constructing complex N-heterocycles (Scheme 73). The authors suggested the possible mechanism involving copper salt catalyzed C(sp2)–H activation (Scheme 74).
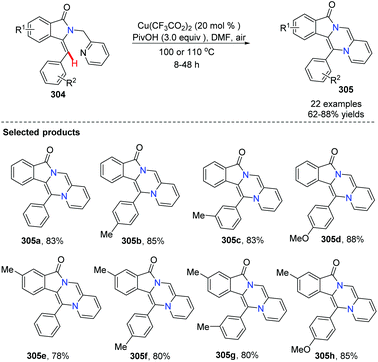 |
| Scheme 73 Synthesis of N-heterocycles via C(sp2)–H amination. | |
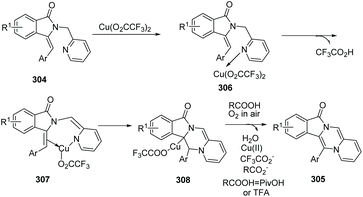 |
| Scheme 74 Possible mechanism for copper-catalyzed C(sp2)–H amination leading to N-heterocycles. | |
In 2012, Zhang and his group reported copper-catalyzed sequential Ullmann N-arylation and aerobic oxidative C–H amination for the efficient synthesis of indolo[1,2-c]quinazoline derivatives 311 (Scheme 75).92 Notably, the reaction involved C(sp3)–H bond activation, and this bond is considered to be the most chemically inert. They found that selecting R2 group is particularly critical. From a structural point of view, R2 group was aromatic or heteroaromatic. This method would be useful for the synthesis of innovative drug candidates by rapidly providing new molecular entities.
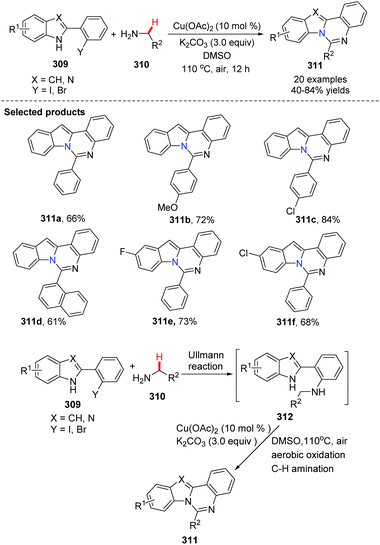 |
| Scheme 75 Convenient route to indolo[1,2-c]quinazoline derivatives. | |
Zhang, Zou and co-workers developed a method for the synthesis of a series of fused nitrogen-containing heterocycles containing indole and quinoline skeletons by a copper-catalyzed reaction from 2-arylated indoles (Scheme 76).93 The reaction involved aerobic oxidative C–H bond functionalization and aromatic C–H amination. It is worth mentioning that the reaction built fused cycles containing a quaternary carbon atom, a short and efficient synthesis accomplished via a new strategy. 18O-Labelled experiments revealed that molecular O2 is not only the oxidant, but also the reactant.
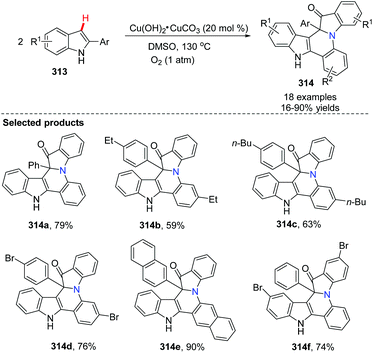 |
| Scheme 76 Oxidative cyclization of 2-arylindoles. | |
A plausible reaction mechanism was proposed as shown in Scheme 77. Firstly, the indole radical cation 315 is formed in the presence of a copper catalyst and oxygen via the electron transfer process. The subsequent deprotonating equilibrium gives the indolyl radical 316, which is oxidized by the oxygen/copper system to afford intermediate 317. The combination of intermediates 318 and 316 delivers the oxidized dimer of 2-phenylindole 319. The subsequent cyclization might occur via the oxidative addition and reductive elimination process of a Cu(I)/Cu(III) cycle, where the oxygen drives the process forward. The electrophilic metalation and reductive elimination pathway is another possible route.
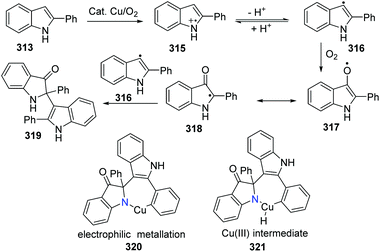 |
| Scheme 77 Plausible reaction mechanism of oxidative cyclization of 2-arylindoles. | |
In 2013, Lu, Wang and co-workers reported two concise methods for the preparation of tryptanthrins from indoles (Scheme 78).94 In the presence of CuI and oxygen, heating indoline itself in DMSO at 80 °C yielded tryptanthrin derivatives 323. On the other hand, the reaction of indoline with isatin 324 in NMP at 80 °C afforded tryptanthrin derivatives 325.
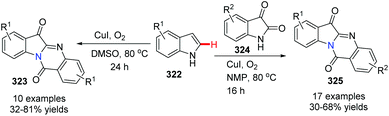 |
| Scheme 78 CuI-Catalyzed synthesis of tryptanthrin derivatives. | |
They postulated a possible mechanism as shown in Scheme 79. Initially, 322 is aerobically oxidized to indolin-3-one 326, which could further oxidize to isatin 327. Then, 327 undergoes hydrolysis to form α-oxoacetic acid 328. Copper-catalyzed decarboxylative coupling between 326 and 328 furnishes 329. Compound 329 is sequentially oxidized to imminium 330via copper-catalyzed oxidation. Then, intramolecular nucleophilic addition of the amino group to N-acylimminium provides the fused ring intermediate 331. Finally, 323 is obtained via dehydrogenative aromatization of 331.
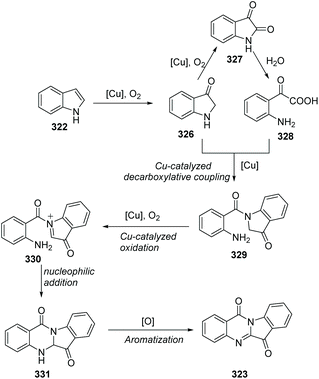 |
| Scheme 79 Proposed mechanism for the synthesis of tryptanthrin derivatives. | |
Fu and co-workers developed a CuI-catalyzed reaction of 2-(2-bromophenyl)-1H-indole 332 with α-amino acids leading to indolo-quinazoline derivatives 334 (Scheme 80).95 The reactions undergo copper-catalyzed N-arylation, aerobic oxidative dehydrogenation, intramolecular cyclization and dissociation of formic acid. The process uses cheap and readily available α-amino acids as the nitrogen source. When 2-(2-halophenyl)-1H-benzo[d]imidazole 335 is employed as the starting material, it provides benzo[4,5]imidazo[1,2-c]quinazoline derivatives 336. This method shows simplicity and practical advantages and should find wide application for the construction of other N-fused heterocycles.
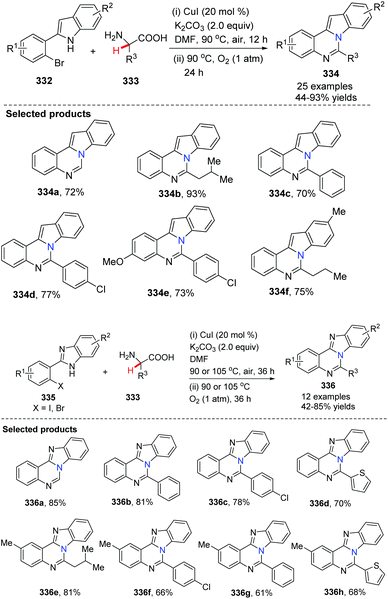 |
| Scheme 80 Copper-catalyzed synthesis of H-indolo[1,2-c]quinazoline derivatives. | |
A possible mechanism for the copper-catalyzed reaction of substituted 2-(2-bromophenyl)-1H-indoles with α-amino acids leading to benzo[4,5]imidazo[1,2-c]quinazolines is suggested in Scheme 81. Firstly, Cu-catalyzed N-arylation of α-amino acid 333 with substituted 2-(2-bromophenyl)-1H-indole 332 affords 337. And then intermediate 337 undergoes one of two domino pathways. In pathway A, oxidative elimination of formic acid from 337 gives 338. Intramolecular cyclization of 338 provides 339, and copper-catalyzed aerobic oxidative dehydrogenation of 339 affords the target product 334. In pathway B, copper-catalyzed aerobic oxidative dehydrogenation of 337 provides 340, intramolecular cyclization of 340 leads to 341, and loss of formic acid from 341 to aromatize the system gives 334.
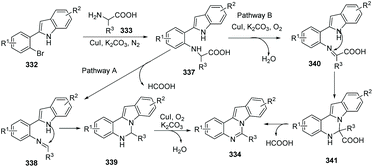 |
| Scheme 81 Possible mechanism for the synthesis of H-indolo[1,2-c]quinazoline derivatives. | |
In 2014, Ye, Zeng and co-workers developed the first Cu(I)-catalyzed direct C(sp3)–H amination of N-heteroaryl aldehydes or ketones with alkylamines leading to rapid and concise access to imidazo[1,5-a]pyridines (Scheme 82).96 A possible mechanism involving a catalytic cycle is shown in Scheme 83. Firstly, the Cu(I) ion coordinates with the pyridine N-atom and imine N-atom in 346. Since the reaction is under an oxygen atmosphere, compound 347 is generated. Subsequently, the corresponding Cu(II)-superoxo radical 347 would abstract the intramolecular hydrogen atom from the coordinated imine to produce Cu(II) intermediate 348, through a six-membered transition state. The proposed Cu(III) species 349 is then attained via isomerization/oxidation processes. Finally, the reductive elimination of the Cu(III) intermediate leads to the formation of imidazo[1,5-a]pyridine 344 with regeneration of the Cu(I) catalyst to close the catalytic cycle.
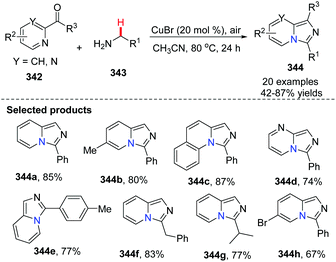 |
| Scheme 82 Synthesis of imidazo[1,5-a]pyridines via Cu(I)-catalyzed transannulation. | |
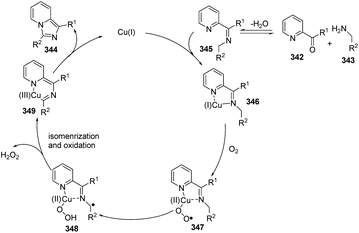 |
| Scheme 83 Proposed catalytic cycle mediated by Cu(I). | |
In 2014, Fu and co-workers developed a novel copper-catalyzed, one-pot method for the preparation of tetrahydroisoquinolino[2,1-a]-quinazolinone derivatives (Scheme 84).97 This approach utilized a sequential copper-catalyzed N-arylation and intramolecular aerobic oxidative cyclization. The first step or N-arylation was conducted under an inert atmosphere (N2) and following C(sp3)–H activation, oxidative cyclization occurred under an oxygen atmosphere.
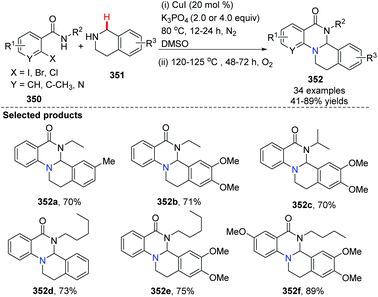 |
| Scheme 84 Synthesis of tetrahydroisoquinolino[2,1-a]quinazolinone derivatives. | |
Recently, Huang, Deng and co-workers initialized the study of cyclization of indole with oxime acetates by copper-catalyzed coupling (Scheme 85).98 A series of pyrazolo[1,5-a]indole derivatives 355 have been prepared efficiently. This novel approach elegantly tri-functionalized N1, C2 and C3 of indoles in a one-pot fashion using internal/external co-oxidation with oxygen and 15 mol% CuCl2 to promote the process. Mechanistic studies indicated that the reaction proceeds through a radical intermediate.
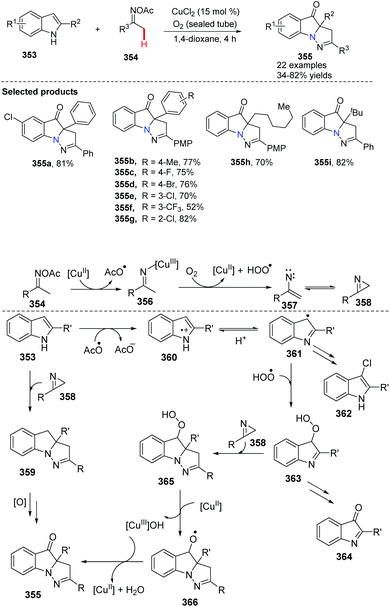 |
| Scheme 85 Cu(II)-Catalyzed aerobic oxygenation and cyclization of indoles with oxime acetates. | |
In 2012, Fu and co-workers developed an efficient copper-catalyzed aerobic oxidative intramolecular C(sp2)–H amination leading to imidazobenzimidazole derivatives (Scheme 86).99 Imidazo C(sp2)–H functionalization led to effective and convenient amination closing of the imidazole ring system. A proposed mechanism is shown in Scheme 87. Initial coordination of substrate 367 with the complex LnCu(OAc)2 and oxidative insertion in the presence of NaOAc provide the key intermediate 369. Reductive elimination affords the target product 368, releasing the Cu(II)Ln catalyst under oxygen. The authors found that yields clearly increased when phenanthroline was applied as the ligand.
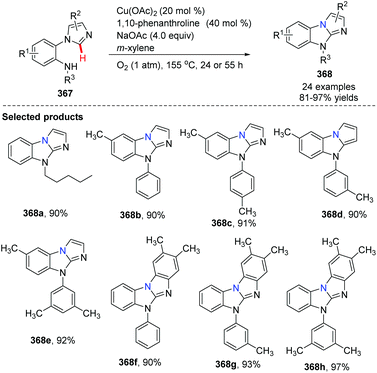 |
| Scheme 86 Synthesis of imidazobenzimidazole derivatives. | |
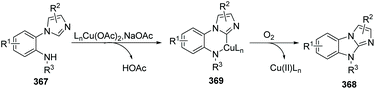 |
| Scheme 87 Possible mechanism for copper-catalyzed C–H amination. | |
Qiao et al. conveniently prepared imidazo/benzimidazo–quinazolinones 371via an intramolecular C–H amination of readily available starting materials in the presence of CuCl as the catalyst (Scheme 88).100 Interestingly, Cu(II) salts such as Cu(OAc)2 are not suitable catalysts. A possible mechanism is shown in Scheme 89. Similar to the previously proposed mechanism, coordination of substrate 370 with CuCl forms 372. Then in the presence of NaOAc, intramolecular coordination allows oxidative insertion providing copper complex 374. Further oxidative addition leads to the Cu(III) complex 375. Finally, reductive elimination gives the target product 371, releasing CuCl to close the catalytic cycle.
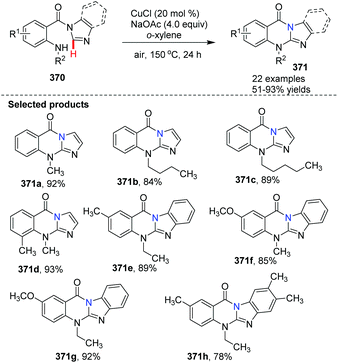 |
| Scheme 88 Synthesis of 5-substituted imidazo/benzimidazoquinazolinones. | |
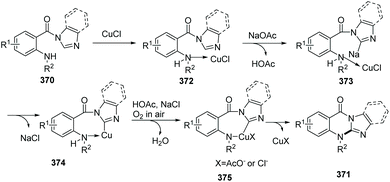 |
| Scheme 89 Possible mechanism of copper-catalyzed synthesis of substituted imidazo/benzimidazoquinazolinones. | |
Recently, Qian and co-workers described a Cu-catalyzed three-component cascade reaction, via copper-mediated click chemistry, C–H functionalization, the Goldberg amidation, the Camps cyclization and C–H arylation, providing an efficient procedure for the synthesis of nitrogen polyheterocycles (Scheme 90).101 These compounds, especially dibenzotriazolonaphthyridinones, are likely to be valuable for drug discovery programs when the triple electrophile bis(2-bromophenyl)methanone was used in the one-pot condition.
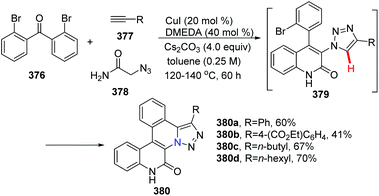 |
| Scheme 90 One-pot construction of dibenzotriazolonaphthyridinones. | |
By using the readily available substituted 2-(2-bromophenyl)-1H-indoles, imidazole and benzoimidazoles as the starting materials and inexpensive CuBr as the catalyst, in the presence of oxygen, a novel and efficient copper-catalyzed one-pot synthesis of indoloimidazoquinoline derivatives has been developed by Fu and co-workers (Scheme 91).102 This reaction involves N-arylation and an aerobic oxidative C(sp2)–H/C(sp2)–H coupling. The method provided more novel target compounds and new molecular entities for drug discovery. A proposed mechanism for the reaction is shown in Scheme 92. In the presence of CuBr and a ligand, N-arylation provides the product 384. The coordination of CuBr with 384 gives complex 385, and the addition of the 3-C–H in the indole group to 2′-C of the imidazole provides 386. Finally, oxidation and elimination give the target product 383, freeing the Cu(I) catalyst to close the catalytic cycle.
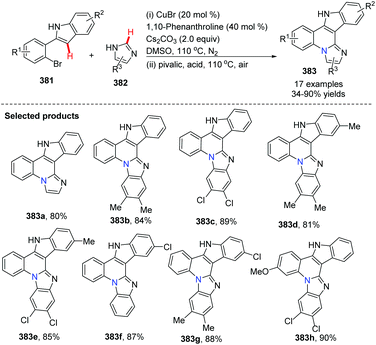 |
| Scheme 91 Synthesis of indoloimidazoquinoline derivatives. | |
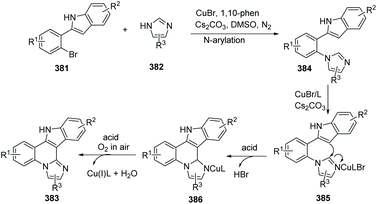 |
| Scheme 92 Possible mechanism for the copper-catalyzed synthesis of indoloimidazoquinoline derivatives. | |
In 2013, Bao and co-workers reported the synthesis of benzimidazol[1,2-a]quinazolin5(6H)-one from 2-iodo-benzamides and N-heterocycles via Cu(I)-catalyzed C–N oxidative coupling/C(sp2)–H activation/C–N formation (Scheme 93).103 When pyrazole was used as an N-aryl substrate, the product of the reaction is pyrazolo[1,5-a]-quinazolinone 392.
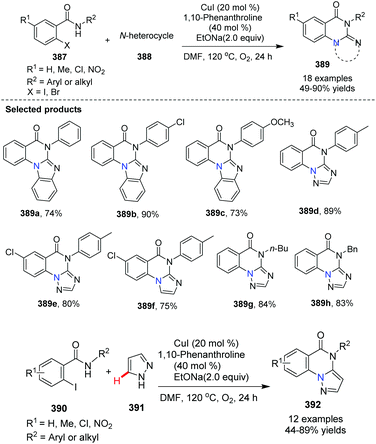 |
| Scheme 93 Synthesis of azoquinazolinones and pyrazolo[1,5-a]quinazolinones. | |
In 2014, Kumar and co-workers reported an approach for the synthesis of imidazo[1,2-a]pyrimidine derivatives from 2-aminobenzimidazole, benzaldehyde and alkynes (Scheme 94),104a using the copper salt-catalyzed multicomponent cascade coupling reaction. The reaction involves two C–N bond forming steps and one C–H bond formation. Intramolecular N–H bond and C(sp)–H bond activation is necessary to promote the reaction. In 2019, it was found by Zhang, Chai and co-workers that without adding Ag2CO3, in the presence of a catalytic amount of CuI and K2CO3, a decarboxylic multicomponent reaction of heterocyclic azoles, aldehydes and alkynecarboxylic acid in DMSO gives the corresponding bridgehead nitrogen scaffold (Scheme 95).104b It is worth mentioning that the composition of the product depends on the ratio of starting materials.When 2.0 equiv. of aldehyde were used, it gave 401 as the major product.
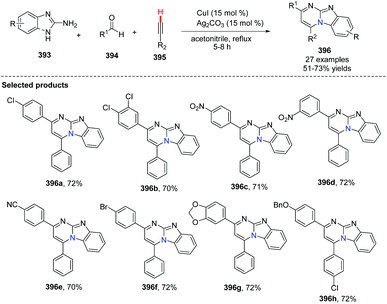 |
| Scheme 94 Synthesis of fused imidazo[1,2-a]pyrimidines. | |
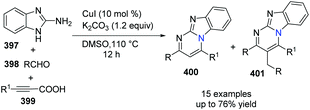 |
| Scheme 95 CuI-Catalyzed decarboxylic multicomponent reaction to prepare pyrimidobenzimidazoles. | |
Purine nucleosides analogues have been developed as drugs in the treatment of virus infections.105 Thus, the modification of purine nucleosides has increasingly attracted the interest of medicinal chemists in recent years. It is worth emphasizing that C–H bond amination reactions on purine rings remain challenging due to their inherent reduced activity in metal-catalyzed transformations as a result of nitrogen coordination which is believed to deactivate the catalyst. Additionally, the poor stability of the nucleosidic bond, which can be easily broken under harsh conditions, has limited further development of these structures.
In 2012, Guo, Fossy and co-workers reported the first synthesis of novel purine-fused polycyclics via Cu(II) salt catalyzed C(sp2)–H activation under mild conditions from 6-anilinopurine as the starting material (Scheme 96).106 A possible pathway is shown in Scheme 97. Initially, coordination of Cu(OTf)2 with substrate 402, followed by an electrophilic substitution process, yields a Cu(II) intermediate. Finally, reductive elimination delivers the target product 403 and Cu(0), which can be re-oxidized to Cu(OTf)2, to end the catalytic cycle.
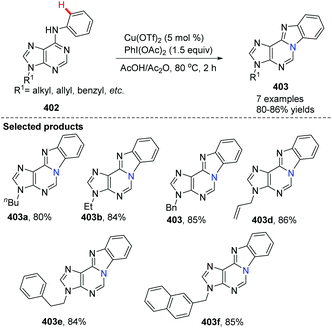 |
| Scheme 96 Copper-catalyzed synthesis of purine-fused polycyclics. | |
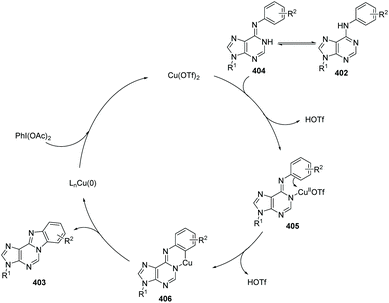 |
| Scheme 97 Plausible catalytic cycle for the synthesis of purine-fused polycyclics. | |
With the optimized conditions, they found that Cu(OTf)2 exhibited better catalytic activity than Cu(I), and thus Cu(OTf)2 was selected as the copper source of choice. PhI(OAc)2 as the oxidant was better than any other oxidants screened. Since the only by-product of the reaction is water, it is not difficult to understand why AcOH/Ac2O was chosen as the solvent, which proved to significantly improve the yield under mild conditions. They also investigated the electronic and steric effects. Results showed that substrates containing electron-withdrawing groups gave higher yields than those with electron-donating groups; substrates with substituents at the ortho position of the aniline ring gave lower yield than those with para substituents. Phenyl as a substituent is more favourable for the reaction than naphthyl as a substituent.
Lv and co-workers developed a Cu-catalyzed synthesis of fused polycycles from bis-(2-haloaryl)carbodiimides and benzimidazole (or indole) (Scheme 98).107 Benzimidazo[1,2-a]indoles were prepared from the reaction with carbodiimides. The proposed mechanism involved the C–H activation was depicted (Scheme 99).
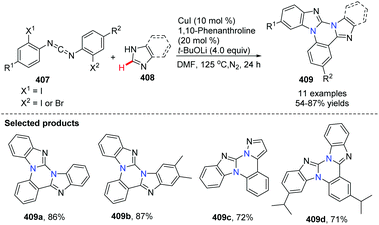 |
| Scheme 98 Approach to polycyclic benzimidazole derivatives. | |
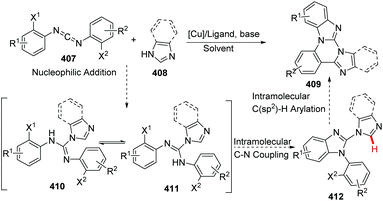 |
| Scheme 99 Proposed mechanism involved the C–H activation in the catalytic pathway. | |
3. Conclusions and outlook
Transition metal-catalyzed C–H activation is a powerful strategy for the synthesis of bridgehead nitrogen heterocycles. Diverse metal catalysis including palladium, rhodium, ruthenium, iridium, cobalt, nickel, silver, and copper have been used as efficient catalysts for the activation of different C(sp2)–H, C(sp)–H, and C(sp3)–H bonds. Among them, Pd, Rh, and Cu are three main players. In particular, Cu catalysts have demonstrated a broad spectrum of catalytic activities. As a non-precious metal, copper is inexpensive and less toxic. In view of the green chemistry principles, copper-catalyzed reactions have bright prospects. Although significant progress has been achieved in this field, further exploration is still required in many directions: (i) the development of additional C–H functionalization protocols for the synthesis of new drug scaffolds is needed. For example, only a few methods that have been reported for transition metal-catalyzed nucleoside synthesis via C–H activation. (ii) New catalysts and catalytic systems are required for the transformation of bridgehead nitrogen heterocycles, so that the number of examples using other catalysts such as Ru, Co, Ir, Ni, Ag etc. would be increased. (iii) In contrast to C(sp2)–H and C(sp)–H bonds, it is still challenging to activate C(sp3)–H bonds.108 Hence more C(sp3)–H bond activation reactions are necessary. (iv) Due to many drugs containing bridgehead nitrogen heterocycles scaffolds, scalable reactions are strongly favored by chemists. We hope that this review would be helpful for synthetic chemists to select appropriate methods for the preparation of target molecules and promote the development of new C–H activation reactions for the synthesis of bridgehead nitrogen heterocycles.
Conflicts of interest
There are no conflicts to declare.
Acknowledgements
This work was supported by the National Major Scientific and Technological Special Project for “Significant New Drugs Development” during the Thirteenth Five-year Plan Period (2018ZX09201002-001), the Key-Area Research and Development Program of Guangdong Province (2019B02021002 and 2020B010188001) and the Fundamental Research Funds for the Central Universities (x2hgD2190580).
Notes and references
-
(a)
Fundamentals of Heterocyclic Chemistry: Importance in Nature and in the Synthesis of Pharmaceuticals, ed. L. D. Quin and J. A. Tyrell, Wiley, Hoboken, NJ, 2010 Search PubMed;
(b)
Heterocyclic Chemistry, ed. J. A. Joule and K. Mills, Wiley, Chichester, WestSussex, 5th edn, 2010 Search PubMed;
(c)
Synthetic Applications of 1,3-Dipolar Cycloaddition Chemistry Toward Heterocycles and Natural Products, ed. A. Padwa and W. H. Pearson, Wiley, New York, NY, 2002 Search PubMed;
(d)
Biosynthesis of Heterocycles, From Isolation to Gene Cluster, ed. P. Diana and G. Cirrincione, Wiley, Hoboken, NJ, 2015, pp. 379–745 Search PubMed.
-
(a)
Contemporary Drug Synthesis, ed. J. J. Li, D. S. Johnson, D. R. Sliskovic and B. D. Roth, Wiley, Hoboken, NJ, 2004, pp. 189–200 Search PubMed;
(b)
P. R. Guzzo, in The Art of Drug Synthesis, ed. D. S. Johnson and J. J. Li, Wiley, Hoboken, NJ, 2007, pp. 215–223 Search PubMed.
-
(a) S. Ramesh and R. Nagarajan, Copper-Catalyzed Hydroarylation of Alkynes for the Synthesis of Fascaplysin, Rutacarpine and Granulatimide Analogues, Synthesis, 2015, 47, 3573–3582 CrossRef CAS;
(b) X. Pan and T. D. Bannister, Sequential Sonagashira and Larock Indole Synthesis Reactions in a General Strategy To Prepare Biologically Active β-Carboline-Containing Alkaloids, Org. Lett., 2014, 16, 6124–6127 CrossRef CAS PubMed;
(c) B. A. Granger, K. Kaneda and S. F. Martin, Multicomponent Assembly Strategies for the Synthesis of Diverse Tetrahydroisoquinoline Scaffolds, Org. Lett., 2011, 13, 4542–4545 CrossRef CAS PubMed;
(d) C. Zhang, C. K. De, R. Mal and D. Seidel, α-Amination of Nitrogen Heterocycles: Ring-Fused Aminals, J. Am. Chem. Soc., 2008, 130, 416–417 CrossRef CAS PubMed.
-
(a) S. De, S. Ghosh, S. Bhunia, J. A. Sheikh and A. Bisai, Intramolecular Direct Dehydrohalide Coupling Promoted by KOtBu: Total Synthesis of Amaryllidaceae Alkaloids Anhydrolycorinone and Oxoassoanine, Org. Lett., 2012, 14, 4466–4469 CrossRef CAS PubMed;
(b) M. Matveenko, O. J. Kokas, M. G. Banwell and A. C. Willis, Chemoenzymatic Approaches to Lycorine-Type Amaryllidaceae Alkaloids: Total Syntheses of ent-Lycoricidine, 3-epi-ent-Lycoricidine, and 4-Deoxy-3-epi-ent-lycoricidine, Org. Lett., 2007, 9, 3683–3685 CrossRef CAS PubMed;
(c) B. M. Trost, W. Tang and F. D. Toste, Divergent Enantioselective Synthesis of (−)-Galanthamine and (−)-Morphine, J. Am. Chem. Soc., 2005, 127, 14785–14803 CrossRef CAS PubMed;
(d) B. M. Trost and W. Tang, Enantioselective Synthesis of (−)-Codeine and (−)-Morphine, J. Am. Chem. Soc., 2002, 124, 14542–14543 CrossRef CAS PubMed;
(e) J. Jin and S. M. Weinreb, Application of a Stereospecific Intramolecular Allenylsilane Imino Ene Reaction to Enantioselective Total Synthesis of the 5,11-Methanomorphanthridine Class of Amaryllidaceae Alkaloids, J. Am. Chem. Soc., 1997, 119, 5773–5784 CrossRef CAS;
(f) J. Zhong and G. Yao, Amaryllidaceae and Sceletium alkaloids, Nat. Prod. Rep., 2019, 36, 1462–1488 RSC.
-
(a) M.-C. Tseng, Y.-W. Chu, H.-P. Tsai, C.-M. Lin, J. Hwang and Y.-H. Chu, One-Pot Synthesis of Luotonin A and Its Analogues, Org. Lett., 2011, 13, 920–923 CrossRef CAS PubMed;
(b) Y. Ju, F. Liu and C. Li, Palladium-Catalyzed Sequential Cyanation/N-Addition/N-Arylation in One-Pot: Efficient Synthesis of Luotonin A and Its Derivatives, Org. Lett., 2009, 11, 3582–3585 CrossRef CAS PubMed;
(c) A. V. Sridharan, P. Ribelles, M. T. Ramos and J. C. Menéndez, Cerium(IV) Ammonium Nitrate Is an Excellent, General Catalyst for the Friedländer and Friedländer−Borsche Quinoline Syntheses: Very Efficient Access to the Antitumor Alkaloid Luotonin, J. Org. Chem., 2009, 74, 5715–5718 CrossRef PubMed;
(d) H.-B. Zhou, G.-S. Liu and Z.-J. Yao, Short and Efficient Total Synthesis of Luotonin A and 22-Hydroxyacuminatine Using A Common Cascade Strategy, J. Org. Chem., 2007, 72, 6270–6272 CrossRef CAS PubMed;
(e) A. Servais, M. Azzouz, D. Lopes, C. Courillon and M. Malacria, Radical cyclization of N-acylcyanamides: total synthesis of luotonin A, Angew. Chem., Int. Ed., 2007, 46, 576–579 CrossRef CAS PubMed.
-
(a) P. S. Baran and E. J. Corey, A Short Synthetic Route to (+)-Austamide, (+)-Deoxyisoaustamide, and (+)-Hydratoaustamide from a Common Precursor by a Novel Palladium-Mediated Indole → Dihydroindoloazocine Cyclization, J. Am. Chem. Soc., 2002, 124, 7904–7905 CrossRef CAS PubMed;
(b) W. Ding, Q.-Q. Zhou, J. Xuan, T.-R. Li, L.-Q. Lu and W.-J. Xiao, Photocatalytic aerobic oxidation/semipinacol rearrangement sequence: a concise route to the core of pseudoindoxyl alkaloids, Tetrahedron Lett., 2014, 55, 4648–4652 CrossRef CAS;
(c) R. M. Williams, Total Synthesis and Biosynthesis of the Paraherquamides: An Intriguing Story of the Biological Diels–Alder Construction, Chem. Pharm. Bull., 2002, 50, 711–740 CrossRef CAS PubMed.
-
(a) L. Shen, N. Xie, B. Yang, Y. Hu and Y. Zhang, Design and total synthesis of Mannich derivatives of marine natural product lamellarin D as cytotoxic agents, Eur. J. Med. Chem., 2014, 85, 807–817 CrossRef CAS PubMed;
(b) Q. Li, J. Jiang, A. Fan, Y. Cui and Y. Jia, Total Synthesis of Lamellarins D, H, and R and Ningalin B, Org. Lett., 2011, 13, 312–315 CrossRef CAS PubMed;
(c) T. Ohta, T. Fukuda, F. Ishibashi and M. Iwao, Design and Synthesis of Lamellarin D Analogues Targeting Topoisomerase I, J. Org. Chem., 2009, 74, 8143–8153 CrossRef CAS PubMed;
(d) P. Ploypradith, T. Petchmanee, P. Sahakitpichan, N. D. Litvinas and S. Ruchirawat, Total Synthesis of Natural and Unnatural Lamellarins with Saturated and Unsaturated D-Rings, J. Org. Chem., 2006, 71, 9440–9448 CrossRef CAS PubMed;
(e) D. Pla, A. Marchal, C. A. Olsen, F. Albericio and M. Álvarez, Modular Total Synthesis of Lamellarin D, J. Org. Chem., 2005, 70, 8231–8234 CrossRef CAS PubMed.
-
(a) S. Yu, Q.-Q. Huang, Y. Luo and W. Lu, Total Synthesis of Camptothecin and SN-38, J. Org. Chem., 2012, 77, 713–717 CrossRef CAS PubMed;
(b) G.-S. Liu, Q.-L. Dong, Y.-S. Yao and Z.-J. Yao, Expeditious Total Syntheses of Camptothecin and 10-Hydroxycamptothecin, Org. Lett., 2008, 10, 5393–5396 CrossRef CAS PubMed;
(c) H.-B. Zhou, G.-S. Liu and Z.-J. Yao, Highly Efficient and Mild Cascade Reactions Triggered by Bis(triphenyl) oxo diphosphonium Trifluoromethanesulfonate and a Concise Total Synthesis of Camptothecin, Org. Lett., 2007, 9, 2003–2006 CrossRef CAS;
(d) R. Peters, M. Althaus, C. Diolez, A. Rolland, E. Manginot and M. Veyrat, Practical Formal Total Syntheses of the Homocamptothecin Derivative and Anticancer Agent Diflomotecan via Asymmetric Acetate Aldol Additions to Pyridine Ketone Substrates, J. Org. Chem., 2006, 71, 7583–7595 CrossRef CAS PubMed;
(e) H. Twin and R. A. Batey, Intramolecular Hetero Diels−Alder (Povarov) Approach to the Synthesis of the Alkaloids Luotonin A and Camptothecin†, Org. Lett., 2004, 6, 4913–4916 CrossRef CAS PubMed;
(f) D. L. Comins and J. M. Nolan, A Practical Six-Step Synthesis of (S)-Camptothecin, Org. Lett., 2001, 3, 4255–4257 CrossRef CAS PubMed.
-
(a) J. B. Hester Jr., A. D. Rudzik and P. F. VonVoigtlander, 8-Chloro-1-methyl-6-phenyl-4H-s-triazolo[4,3-a][1,4]benzodiazepines with substituents at C-4, J. Med. Chem., 1980, 23, 643–647 CrossRef CAS PubMed;
(b) A. Walser and G. Zenchoff, Quinazolines and 1,4-benzodiazepines. 81. s-Triazolo[4,3-a][1,4]benzodiazepines by oxidative cyclization of hydrazones, J. Med. Chem., 1977, 20, 1694–1697 CrossRef CAS PubMed.
-
(a) A. Walser and R. I. Fryer, Quinazolines and 1,4-benzodiazepines. 69. 1-Vinyl-1,4-benzodiazepin-2-ones and 1-vinylquinazolin-2(1H)-ones, J. Med. Chem., 1974, 17, 1228–1230 CrossRef CAS PubMed;
(b)
A. Walser, G. Zenchoff and R. I. Fryer, Quinazolines and 1,4-benzodiazepines. 75. 7-Hydroxyaminobenzodiazepines and derivatives, J. Med. Chem., 1976, 19, 1378–1381 Search PubMed;
(c) E. J. Trybulski, L. Benjamin, S. Vitone, A. Walser and R. I. Fryer, 2-Benzazepines. [1,2,3]Triazolo[4,5-d][2]benzazepines and dibenzo[c,f][1,2,3]triazolo[3,4-a]azepines. Synthesis and evaluation as central nervous system agents, J. Med. Chem., 1983, 26, 367–372 CrossRef CAS PubMed.
- T. B. Beghyn, J. Charton, F. Leroux, G. Laconde, A. Bourin, P. Cos, L. Maes and B. Deprez, Drug to Genome to Drug: Discovery of New Antiplasmodial Compounds, J. Med. Chem., 2011, 54, 3222–3240 CrossRef CAS PubMed.
- D. Kim, L. Wang, M. Beconi, G. J. Eiermann, M. H. Fisher, H. He, G. J. Hickey, J. E. Kowalchick, B. Leiting, K. Lyons, F. Marsilio, M. E. McCann, R. A. Patel, A. Petrov, G. Scapin, S. B. Patel, R. S. Roy, J. K. Wu, M. J. Wyvratt, B. B. Zhang, L. Zhu, N. A. Thornberry and A. E. Weber, (2R) -4-Oxo-4-[3-(Trifluoromethyl)-5,6-dihydro[1,2,4]triazolo[4,3-a]pyrazin- 7(8H)-yl]-1-(2,4,5-trifluorophenyl)butan-2-amine: A Potent, Orally Active Dipeptidyl Peptidase IV Inhibitor for the Treatment of Type 2 Diabetes, J. Med. Chem., 2005, 48, 141–151 CrossRef CAS PubMed.
- W.-S. Huang, C. A. Metcalf, R. Sundaramoorthi, Y. Wang, D. Zou, R. M. Thomas, X. Zhu, L. Cai, D. Wen, S. Liu, J. Romero, J. Qi, I. Chen, G. Banda, S. P. Lentini, S. Das, Q. Xu, J. Keats, F. Wang, S. Wardwell, Y. Ning, J. Snodgrass, M. I. Broudy, K. Russian, T. Zhou, L. Commodore, N. I. Narasimhan, Q. K. Mohemmad, J. Iuliucci, V. M. Rivera, D. C. Dalgarno, T. K. Sawyer, T. Clackson and W. C. Shakespeare, Discovery of 3-[2-(Imidazo[1,2-b]pyridazin-3-yl)ethynyl]-4-methyl-N-{4-[(4-methylpiperazin-1-yl)methyl]-3-(trifluoromethyl)phenyl}benzamide (AP24534), a Potent, Orally Active Pan-Inhibitor of Breakpoint Cluster Region-Abelson (BCR-ABL) Kinase Including the T315I Gatekeeper Mutant, J. Med. Chem., 2010, 53, 4701–4719 CrossRef CAS PubMed.
- B. A. Johns, T. Kawasuji, J. G. Weatherhead, T. Taishi, D. P. Temelkoff, H. Yoshida, T. Akiyama, Y. Taoda, H. Murai, R. Kiyama, M. Fuji, N. Tanimoto, J. Jeffrey, S. A. Foster, T. Yoshinaga, T. Seki, M. Kobayashi, A. Sato, M. N. Johnson, E. P. Garvey and T. Fujiwara, Carbamoyl Pyridone HIV-1 Integrase Inhibitors 3. A Diastereomeric Approach to Chiral Nonracemic Tricyclic Ring Systems and the Discovery of Dolutegravir (S/GSK1349572) and (S/GSK1265744), J. Med. Chem., 2013, 56, 5901–5916 CrossRef CAS PubMed.
- C. J. Menet, S. R. Fletcher, G. V. Lommen, R. Geney, J. Blanc, K. Smits, N. Jouannigot, P. Deprez, E. M. van der Aar, P. Clement-Lacroix, L. Lepescheux, R. Galien, B. Vayssiere, L. Nelles, T. Christophe, R. Brys, M. Uhring, F. Ciesielski and L. V. Rompaey, Triazolopyridines as Selective JAK1 Inhibitors: From Hit Identification to GLPG0634, J. Med. Chem., 2014, 57, 9323–9342 CrossRef CAS PubMed.
- P. Norman, Selective JAK inhibitors in development for rheumatoid arthritis, Expert Opin. Investig. Drugs, 2014, 23, 1067–1077 CrossRef CAS PubMed.
- D. L. Hughes, Review of the Patent Literature: Synthesis and Final Forms of Antiviral Drugs Tecovirimat and Baloxavir Marboxil, Org. Process Res. Dev., 2019, 23, 1298–1307 CrossRef CAS.
-
https://www.gilead.com/news-and-press/press-room/press-releases/2020/5/gilead-announces-approval-of-veklury-remdesivir-in-japan-for-patients-with-severe-covid19
.
-
https://www.drugs.com/news/fda-approves-emergency-remdesivir-covid-19–89997.html
.
-
(a) N.-Y. Wang, Y. Xu, W.-Q. Zuo, K.-J. Xiao, L. Liu, X.-X. Zeng, X.-Y. You, L.-D. Zhang, C. Gao, Z.-H. Liu, T.-H. Ye, Y. Xia, Y. Xiong, X.-J. Song, Q. Lei, C.-T. Peng, H. Tang, S.-Y. Yang, Y.-Q. Wei and L.-T. Yu, Discovery of Imidazo[2,1-b]thiazole HCV NS4B Inhibitors Exhibiting Synergistic Effect with Other Direct-Acting Antiviral Agents, J. Med. Chem., 2015, 58, 2764–2778 CrossRef CAS PubMed;
(b) T. Xue, S. Ding, B. Guo, Y. Zhou, P. Sun, H. Wang, W. Chu, G. Gong, Y. Wang, X. Chen and Y. Yang, Design, Synthesis, and Structure–Activity and Structure–Pharmacokinetic Relationship Studies of Novel [6,6,5] Tricyclic Fused Oxazolidinones Leading to the Discovery of a Potent, Selective, and Orally Bioavailable FXa Inhibitor, J. Med. Chem., 2014, 57, 7770–7791 CrossRef CAS PubMed;
(c) J. J. Cui, H. Shen, M. Tran-Dubé, M. Nambu, M. McTigue, N. Grodsky, K. Ryan, S. Yamazaki, S. Aguirre, M. Parker, Q. Li, H. Zou and J. Christensen, Lessons from (S)-6-(1-(6-(1-Methyl-1H-pyrazol-4-yl)-[1,2,4]triazolo[4,3-b]pyridazin-3-yl)ethyl)quinoline (PF-04254644), an Inhibitor of Receptor Tyrosine Kinase c-Met with High Protein Kinase Selectivity but Broad Phosphodiesterase Family Inhibition Leading to Myocardial Degeneration in Rats, J. Med. Chem., 2013, 56, 6651–6665 CrossRef CAS PubMed;
(d)
C. Lamberth and J. Dinges, in Bioactive Heterocyclic Compound Classes: Pharmaceuticals, ed. J. Dinges and C. Lamberth, Wiley-VCH, Weinheim, 2012, pp. 3–20 Search PubMed.
- W. Zhao, Novel Syntheses of Bridge-Containing Organic Compounds, Chem. Rev., 2010, 110, 1706–1745 CrossRef CAS PubMed.
-
J. J. Vaquero and J. Alvarez-Builla, Heterocycles Containing a Ring-Junction Nitrogen, in Modern Heterocyclic Chemistry, ed. J. Alvarez-Builla, J. J. Vaquero and J. Barluenga, Wiley-VCH, Weinheim, First edn, 2011, pp. 1989–2070 Search PubMed.
-
Comprehensive Heterocyclic Chemistry III, ed. J. Cossy, Elsevier, 2008, vol. 11 Search PubMed.
- B. H. Rotstein, S. Zaretsky, V. Rai and A. K. Yudin, Small Heterocycles in Multicomponent Reactions, Chem. Rev., 2014, 114, 8323–8359 CrossRef CAS PubMed.
- P. Wu and T. E. Nielsen, Scaffold Diversity from N-Acyliminium Ions, Chem. Rev., 2017, 117, 7811–7856 CrossRef CAS PubMed.
- I. Nakamura and Y. Yamamoto, Transition-Metal-Catalyzed Reactions in Heterocyclic Synthesis, Chem. Rev., 2004, 104, 2127–2198 CrossRef CAS PubMed.
- Reviews, see:
(a) J. Yamaguchi, A. D. Yamaguchi and K. Itami, C-H bond functionalization: emerging synthetic tools for natural products and pharmaceuticals, Angew. Chem., Int. Ed., 2012, 51, 8960–9009 CrossRef CAS PubMed;
(b) C. S. Yeung and V. M. Dong, Catalytic Dehydrogenative Cross-Coupling: Forming Carbon−Carbon Bonds by Oxidizing Two Carbon−Hydrogen Bonds, Chem. Rev., 2011, 111, 1215–1292 CrossRef CAS PubMed;
(c) K. M. Engle, T.-S. Mei, M. Wasa and J.-Q. Yu, Weak Coordination as a Powerful Means for Developing Broadly Useful C–H Functionalization Reactions, Acc. Chem. Res., 2012, 45, 788–802 CrossRef CAS PubMed;
(d) T. W. Lyons and M. S. Sanford, Palladium-Catalyzed Ligand-Directed C−H Functionalization Reactions, Chem. Rev., 2010, 110, 1147–1169 CrossRef CAS PubMed;
(e) S. R. Neufeldt and M. S. Sanford, Controlling Site Selectivity in Palladium-Catalyzed C–H Bond Functionalization, Acc. Chem. Res., 2012, 45, 936–946 CrossRef CAS PubMed;
(f) G. Song, F. Wang and X. Li, C–C, C–O and C–N bond formation via rhodium(iii)-catalyzed oxidative C–H activation, Chem. Soc. Rev., 2012, 41, 3651–3678 RSC;
(g) K. R. D. Johnson and P. G. Hayes, Cyclometalative C–H bond activation in rare earth and actinide metal complexes, Chem. Soc. Rev., 2013, 42, 1947–1960 RSC;
(h) B. Li and P. H. Dixneuf, sp2 C–H bond activation in water and catalytic cross-coupling reactions, Chem. Soc. Rev., 2013, 42, 5744–5767 RSC;
(i) K. R. Campos, Direct sp3 C–H bond activation adjacent to nitrogen in heterocycles, Chem. Soc. Rev., 2007, 36, 1069–1084 RSC;
(j) A. E. Shilov and G. B. Shul'pin, Activation of C−H Bonds by Metal Complexes, Chem. Rev., 1997, 97, 2879–2932 CrossRef CAS PubMed;
(k) D. A. Colby, R. G. Bergman and J. A. Ellman, Rhodium-Catalyzed C−C Bond Formation via Heteroatom-Directed C−H Bond Activation, Chem. Rev., 2010, 110, 624–655 CrossRef CAS PubMed;
(l) P. B. Arockiam, C. Bruneau and P. H. Dixneuf, Ruthenium(II)-Catalyzed C–H Bond Activation and Functionalization, Chem. Rev., 2012, 112, 5879–5918 CrossRef CAS PubMed;
(m) V. Ritleng, C. Sirlin and M. Pfeffer, Ru-, Rh-, and Pd-Catalyzed C−C Bond Formation Involving C−H Activation and Addition on Unsaturated Substrates: Reactions and Mechanistic Aspects, Chem. Rev., 2002, 102, 1731–1770 CrossRef CAS PubMed;
(n) Y. J. Park, J.-W. Park and C.-H. Jun, Metal−Organic Cooperative Catalysis in C−H and C−C Bond Activation and Its Concurrent Recovery, Acc. Chem. Res., 2008, 41, 222–234 CrossRef CAS PubMed;
(o) C. Jia, T. Kitamura and Y. Fujiwara, Catalytic Functionalization of Arenes and Alkanes via C−H Bond Activation, Acc. Chem. Res., 2001, 34, 633–639 CrossRef CAS PubMed;
(p) B. G. Hashiguchi, S. M. Bischof, M. M. Konnick and R. A. Periana, Designing Catalysts for Functionalization of Unactivated C–H Bonds Based on the CH Activation Reaction, Acc. Chem. Res., 2012, 45, 885–898 CrossRef CAS PubMed;
(q) J. A. Labinger and J. E. Bercaw, Understanding and exploiting C–H bond activation, Nature, 2002, 417, 507–514 CrossRef CAS PubMed;
(r)
C-H Activation, ed. J.-Q. Yu and Z.-J. Shi, Springer, Berlin Heidelberg, 2010 Search PubMed.
- N. Chernyak and V. Gevorgyan, General and efficient copper-catalyzed three-component coupling reaction towards imidazoheterocycles: one-pot synthesis of alpidem and zolpidem, Angew. Chem., Int. Ed., 2010, 49, 2743–2746 CrossRef CAS PubMed.
- R. Ferraccioli, Heterocycle Synthesis Based on Palladium-Catalyzed C-H Bond Functionalization Methods, Curr. Org. Synth., 2012, 9, 96–113 CrossRef CAS.
- X.-X. Guo, D.-W. Gu, Z. Wu and W. Zhang, Copper-Catalyzed C–H Functionalization Reactions: Efficient Synthesis of Heterocycles, Chem. Rev., 2015, 115, 1622–1651 CrossRef CAS PubMed.
-
Transition Metal-Catalyzed Heterocycle Synthesis via C-H Activation, ed. X. F. Wu, Wiley-VCH, Weinheim, 2016 Search PubMed.
- G. K. Reen, A. Kumar and P. Sharma, Recent advances on the transition-metal-catalyzed synthesis of imidazopyridines: an updated coverage, Beilstein J. Org. Chem., 2019, 15, 1612–1704 CrossRef CAS PubMed.
- Y. Zhang, B. Nie and J. Zhang, Progress in the Research of Copper Promoted C-H Functionalizations for the Synthesis of Fused Heterocycles Bearing Bridgehead Nitrogen, Chin. J. Org. Chem., 2015, 35, 2067–2085 CrossRef CAS.
- H. Ren and P. Knochel, Chemoselective Benzylic C−H Activations for the Preparation of Condensed N-Heterocycles, Angew. Chem., Int. Ed., 2006, 45, 3462–3465 CrossRef CAS PubMed.
- S. R. Kandukuri, J. A. Schiffner and M. Oestreich, Aerobic Palladium(II)-Catalyzed 5-endo-trig Cyclization: An Entry into the Diastereoselective C-2 Alkenylation of Indoles with Tri- and Tetrasubstituted Double Bonds, Angew. Chem., Int. Ed., 2012, 51, 1265–1269 CrossRef CAS PubMed.
-
(a) C. Blaszykowski, E. Aktoudianakis, C. Bressy, D. Alberico and M. Lautens, Preparation of Annulated Nitrogen-Containing Heterocycles via a One-Pot Palladium-Catalyzed Alkylation/Direct Arylation Sequence, Org. Lett., 2006, 8, 2043–2045 CrossRef CAS PubMed;
(b) B. Laleu and M. Lautens, Synthesis of Annulated 2H-Indazoles and 1,2,3- and 1,2,4-Triazoles via a One-Pot Palladium-Catalyzed Alkylation/Direct Arylation Reaction, J. Org. Chem., 2008, 73, 9164–9167 CrossRef CAS PubMed.
- H. Hu, G. Li, W. Hu, Y. Liu, X. Wang, Y. Kan and M. Ji, Synthesis of Pyrrolo[2,1,5-cd]indolizines through Dehydrogenative Heck Annelation of Indolizines with Diaryl Acetylenes Using Dioxygen
as an Oxidant, Org. Lett., 2015, 17, 1114–1117 CrossRef CAS PubMed.
- S. J. Hwang, S. H. Cho and S. Chang, Synthesis of Condensed Pyrroloindoles via Pd-Catalyzed Intramolecular C−H Bond Functionalization of Pyrroles, J. Am. Chem. Soc., 2008, 130, 16158–16159 CrossRef CAS PubMed.
- S. P. Cooper and K. I. Booker-Milburn, A Palladium(II)-Catalyzed C–H Activation Cascade Sequence for Polyheterocycle Formation, Angew. Chem., Int. Ed., 2015, 54, 6496–6500 CrossRef CAS PubMed.
- M. Wang, X. Zhang, Y.-X. Zhuang, Y.-H. Xu and T.-P. Loh, Pd-Catalyzed Intramolecular C–N Bond Cleavage, 1,4-Migration, sp3 C–H Activation, and Heck Reaction: Four Controllable Diverse Pathways Depending on the Judicious Choice of the Base and Ligand, J. Am. Chem. Soc., 2015, 137, 1341–1347 CrossRef CAS PubMed.
- S. P. Shukla, R. K. Tiwari and A. K. Verma, Palladium-Catalyzed Sonogashira-Coupling Conjoined C–H Activation: A Regioselective Tandem Strategy to Access Indolo- and Pyrrolo[1,2-a]quinolines, J. Org. Chem., 2012, 77, 10382–10392 CrossRef CAS PubMed.
- J. J. Mousseau, J. A. Bull, C. L. Ladd, A. Fortier, D. S. Roman and A. B. Charette, Synthesis of 2- and 2,3-Substituted Pyrazolo[1,5-a]pyridines: Scope and Mechanistic Considerations of a Domino Direct Alkynylation and Cyclization of N-Iminopyridinium Ylides Using Alkenyl Bromides, Alkenyl Iodides, and Alkynes, J. Org. Chem., 2011, 76, 8243–8261 CrossRef CAS PubMed.
- Q.-Z. Zheng, P. Feng, Y.-F. Liang and N. Jiao, Pd-Catalyzed Tandem C–H Azidation and N–N Bond Formation of Arylpyridines: A Direct Approach to Pyrido[1,2-b]indazoles, Org. Lett., 2013, 15, 4262–4265 CrossRef CAS PubMed.
- S. Hernández, I. Moreno, R. SanMartin, G. Gómez, M. T. Herrero and E. Domínguez, Toward Safer Processes for C−C Biaryl Bond Construction: Catalytic Direct C−H Arylation and Tin-Free Radical Coupling in the Synthesis of Pyrazolophenanthridines, J. Org. Chem., 2010, 75, 434–441 CrossRef PubMed.
- W. Yang, J. Chen, X. Huang, J. Ding, M. Liu and H. Wu, Pd-Catalyzed Intramolecular Aerobic Oxidative C–H Amination of 2-Aryl-3-(arylamino)quinazolinones: Synthesis of Fluorescent Indazolo[3,2-b]quinazolinones, Org. Lett., 2014, 16, 5418–5421 CrossRef CAS PubMed.
- A. J. Ansari, R. S. Pathare, A. Kumawat, A. K. Maurya, S. Verma, V. K. Agnihotri, R. Joshi, R. K. Metre, A. Sharon, R. T. Pardasani and D. M. Sawant, A diversity-oriented synthesis of polyheterocycles via the cyclocondensation of azomethine imine, New J. Chem., 2019, 43, 13721–13724 RSC.
- H. Sun, C. Wang, Y.-F. Yang, P. Chen, Y.-D. Wu, X. Zhang and Y. Huang, Synthesis of Indolo[2,1-a]isoquinolines via a Triazene-Directed C–H Annulation Cascade, J. Org. Chem., 2014, 79, 11863–11872 CrossRef CAS PubMed.
- G. Song, D. Chen, C.-L. Pan, R. H. Crabtree and X. Li, Rh-Catalyzed Oxidative Coupling between Primary and Secondary Benzamides and Alkynes: Synthesis of Polycyclic Amides, J. Org. Chem., 2010, 75, 7487–7490 CrossRef CAS PubMed.
- X. Wu, Y. Xiao, S. Sun, J.-T. Yu and J. Cheng, Rhodium-Catalyzed Reaction of Sulfoxonium Ylides and Anthranils toward Indoloindolones via a (4+1) Annulation, Org. Lett., 2019, 21, 6653–6657 CrossRef CAS PubMed.
- X. Li and M. Zhao, Rhodium(III)-Catalyzed Oxidative Coupling of 5-Aryl-1H-pyrazoles with Alkynes and Acrylates, J. Org. Chem., 2011, 76, 8530–8536 CrossRef CAS PubMed.
- S. Mai, Y. Luo, X. Huang, Z. Shu, B. Li, Y. Lan and Q. Song, Diversity-oriented synthesis of imidazo[2,1-a]isoquinolines, Chem. Commun., 2018, 54, 10240–10243 RSC.
- Y. Xu, L. Zhang, M. Liu, X. Zhang, X. Zhang and X. Fan, Synthesis of benzoazepine derivatives via Rh(iii)-catalyzed inert C(sp2)–H functionalization and [4+3] annulation, Org. Biomol. Chem., 2019, 17, 8706–8710 RSC.
- G. L. Hoang and J. A. Ellman, Rhodium(III)-catalyzed C–H functionalization of C-alkenyl azoles with sulfoxonium ylides for the synthesis of bridgehead N-fused [5,6]-bicyclic heterocycles, Tetrahedron, 2018, 74, 3318–3324 CrossRef CAS PubMed.
- S. Dhole, W.-J. Chiu and C.-M. Sun, Catalyst-Controlled Chemodivergent Annulation to Indolo/Pyrrolo-Fused Diazepine and Quinoxaline, Adv. Synth. Catal., 2019, 361, 2916–2925 CrossRef CAS.
- K. S. Halskov, H. S. Roth and J. A. Ellman, Synthesis of [5,6]-Bicyclic Heterocycles with a Ring-Junction Nitrogen Atom: Rhodium(III)-Catalyzed C-H Functionalization of Alkenyl Azoles, Angew. Chem., Int. Ed., 2017, 56, 9183–9187 CrossRef CAS PubMed.
- Y. Hu, T. Wang, Y. Liu, R. Nie, N. Yang, Q. Wang, G.-B. Li and Y. Wu, Practical Synthesis of Benzimidazo[1,2-a]quinolines via Rh(III)-Catalyzed C–H Activation Cascade Reaction from Imidamides and Anthranils, Org. Lett., 2020, 22, 501–504 CrossRef CAS PubMed.
- K. S. Halskov, M. R. Witten, G. L. Hoang, B. Q. Mercado and J. A. Ellman, Rhodium(III)-Catalyzed Imidoyl C–H Activation for Annulations to Azolopyrimidines, Org. Lett., 2018, 20, 2464–2467 CrossRef CAS PubMed.
- G. L. Hoang, A. D. Streit and J. A. Ellman, Three-Component Coupling of Aldehydes, Aminopyrazoles, and Sulfoxonium Ylides via Rhodium(III)-Catalyzed Imidoyl C–H Activation: Synthesis of Pyrazolo[1,5-a]pyrimidines, J. Org. Chem., 2018, 83, 15347–15360 CrossRef CAS PubMed.
- X. Wu, S. Sun, S. Xu and J. Cheng, Rh-Catalyzed Annulation of ortho-C−H Bonds of 2-Arylimidazoles with 1,4,2-Dioxazol-5-ones toward 5-Arylimidazo[1,2-c]quinazolines, Adv. Synth. Catal., 2018, 360, 1111–1115 CrossRef CAS.
- C. S. Yi and S. Y. Yun, Scope and Mechanistic Study of the Ruthenium-Catalyzed ortho-C−H Bond Activation and Cyclization Reactions of Arylamines with Terminal Alkynes, J. Am. Chem. Soc., 2005, 127, 17000–17006 CrossRef CAS PubMed.
- W. Ma, K. Graczyk and L. Ackermann, Ruthenium-Catalyzed Alkyne Annulations with Substituted 1H-Pyrazoles by C–H/N–H Bond Functionalizations, Org. Lett., 2012, 14, 6318–6321 CrossRef CAS PubMed.
- H. Lu, Q. Yang, Y. Zhou, Y. Guo, Z. Deng, Q. Ding and Y. Peng, Cross-coupling/annulations of quinazolones with alkynes for access to fused polycyclic heteroarenes under mild conditions, Org. Biomol. Chem., 2014, 12, 758–764 RSC.
- R. Lingayya, M. Vellakkaran, K. Nagaiah and J. B. Nanubolu, Ruthenium as a Single Catalyst for Two Steps: One-Pot Ruthenium(II)-Catalyzed Aerobic Oxidative Dehydrogenation of Dihydroquinazolinones and Cross-Coupling/Annulation to give N-Fused Polycyclic Heteroarenes, Asian J. Org. Chem., 2015, 4, 462–469 CrossRef CAS.
-
(a) M. Gholamhosseyni and E. Kianmehr, A ruthenium-catalyzed alkenylation–annulation approach for the synthesis of indazole derivatives via C–H bond activation, Org. Biomol. Chem., 2018, 16, 5973–5978 RSC;
(b) P. Karishma, C. K. Mahesha, D. S. Agarwal, S. K. Mandal and R. Sakhuja, Additive-Driven Rhodium-Catalyzed [4+1]/[4+2] Annulations of N-Arylphthalazine-1,4-dione with α-Diazo Carbonyl Compounds, J. Org. Chem., 2018, 83, 11661–11673 CrossRef CAS PubMed.
- P. Cai, E. Zhang, Y. Wu, T. Fang, Q. Li, C. Yang, J. Wang and Y. Shang, Ru(II)/Ir(III)-Catalyzed C–H Bond Activation/Annulation of Cyclic Amides with 1,3-Diketone-2-diazo Compounds: Facile Access to 8H-Isoquinolino[1,2-b]quinazolin-8-ones and Phthalazino[2,3-a]cinnoline-8,13-diones, ACS Omega, 2018, 3, 14575–14584 CrossRef CAS PubMed.
- B. DeBoef, S. J. Pastine and D. Sames, Cross-Coupling of sp3 C−H Bonds and Alkenes: Catalytic Cyclization of Alkene−Amide Substrates, J. Am. Chem. Soc., 2004, 126, 6556–6557 CrossRef CAS.
-
(a) R. K. Thalji, J. A. Ellman and R. G. Bergman, Highly Efficient and Enantioselective Cyclization of Aromatic Imines via Directed C−H Bond Activation, J. Am. Chem. Soc., 2004, 126, 7192–7193 CrossRef CAS;
(b) H. Harada, R. K. Thalji, R. G. Bergman and J. A. Ellman, Enantioselective Intramolecular Hydroarylation of Alkenes via Directed C−H Bond Activation, J. Org. Chem., 2008, 73, 6772–6779 CrossRef CAS PubMed.
- T. Shibata, N. Ryu and H. Takano, Very Important Publication: Iridium-Catalyzed Intramolecular Enantioselective C–H Alkylation at the C-2 Position of N-Alkenylindoles, Adv. Synth. Catal., 2015, 357, 1131–1135 CrossRef CAS.
- D. Clare, B. C. Dobson, P. A. Inglesby and C. Aïssa, Chemospecific Cyclizations of α-Carbonyl Sulfoxonium Ylides on Aryls and Heteroaryls, Angew. Chem., Int. Ed., 2019, 58, 16198–16202 CrossRef CAS PubMed.
- C. K. Mahesha, D. S. Agarwal, P. Karishma, D. Markad, S. K. Mandal and R. Sakhuja, Iridium-catalyzed [4+2] annulation of 1-arylindazolones with α-diazo carbonyl compounds: access to indazolone-fused cinnolines, Org. Biomol. Chem., 2018, 16, 8585–8595 RSC.
- Z. Ding and N. Yoshikai, Cobalt-Catalyzed Intramolecular Olefin Hydroarylation Leading to Dihydropyrroloindoles and Tetrahydropyridoindoles, Angew. Chem., Int. Ed., 2013, 52, 8574–8578 CrossRef CAS PubMed.
- H. Ikemoto, T. Yoshino, K. Sakata, S. Matsunaga and M. Kanai, Pyrroloindolone Synthesis via a Cp*CoIII-Catalyzed Redox-Neutral Directed C–H Alkenylation/Annulation Sequence, J. Am. Chem. Soc., 2014, 136, 5424–5431 CrossRef CAS PubMed.
- D. Zhao, J.-H. Kim, L. Stegemann, C. A. Strassert and F. Glorius, Cobalt(III)-catalyzed directed C-H coupling with diazo compounds: straightforward access towards extended π-systems, Angew. Chem., Int. Ed., 2015, 54, 4508–4511 CrossRef CAS PubMed.
- Y. Nakao, H. Idei, K. S. Kanyiva and T. Hiyama, Direct Alkenylation and Alkylation of Pyridone Derivatives by Ni/AlMe3 Catalysis, J. Am. Chem. Soc., 2009, 131, 15996–15997 CrossRef CAS PubMed.
- S. Li and J. Wu, Synthesis of H-Pyrazolo[5,1-a]isoquinolines via Copper(II)-Catalyzed Oxidation of an Aliphatic C−H Bond of Tertiary Amine in Air, Org. Lett., 2011, 13, 712–715 CrossRef CAS PubMed.
- P. Huang, Q. Yang, Z. Chen, Q. Ding, J. Xu and Y. Peng, Metal Cocatalyzed Tandem Alkynylative Cyclization Reaction of in Situ Formed N-Iminoisoquinolinium Ylides with Bromoalkynes via C–H Bond Activation, J. Org. Chem., 2012, 77, 8092–8098 CrossRef CAS PubMed.
- K. Sun, S.-J. Li, X.-L. Chen, Y. Liu, X.-Q. Huang, D.-H. Wei, L.-B. Qu, Y.-F. Zhao and B. Yu, Silver-catalyzed decarboxylative radical cascade cyclization toward benzimidazo[2,1-a]isoquinolin-6(5H)-ones, Chem. Commun., 2019, 55, 2861–2864 RSC.
- J. Barluenga, G. Lonzi, L. Riesgo, L. A. López and M. Tomás, Pyridine Activation via Copper(I)-Catalyzed Annulation toward Indolizines, J. Am. Chem. Soc., 2010, 132, 13200–13202 CrossRef CAS PubMed.
- S. E. Kiruthika, A. Nandakumar and P. T. Perumal, Synthesis of Pyrrolo-/Indolo[1,2-a]quinolines and Naphtho[2,1-b]thiophenes from gem-Dibromovinyls and Sulphonamides, Org. Lett., 2014, 16, 4424–4427 CrossRef CAS PubMed.
- R.-R. Liu, J.-J. Hong, C.-J. Lu, M. Xu, J.-R. Gao and Y.-X. Jia, Indolizine Synthesis via Oxidative Cross-Coupling/Cyclization of Alkenes and 2-(Pyridin-2-yl)acetate Derivatives, Org. Lett., 2015, 17, 3050–3053 CrossRef CAS.
- W. Cao, J. Fan, L. Yan, G. Zeng, J. Ma, Y. Wang, Y. Zhou and X. Deng, Divergent Reactivity in CuII-Catalyzed Oxidative Coupling between Indole/Tryptamine Derivatives and β-Arylacrylic Acids, Org. Lett., 2019, 21, 9506–9511 CrossRef CAS PubMed.
- H. Wang, Y. Wang, C. Peng, J. Zhang and Q. Zhu, A Direct Intramolecular C−H Amination Reaction Cocatalyzed by Copper(II) and Iron(III) as Part of an Efficient Route for the Synthesis of Pyrido[1,2-a]benzimidazoles from N-Aryl-2-aminopyridines, J. Am. Chem. Soc., 2010, 132, 13217–13219 CrossRef CAS PubMed.
- H. Wang, Y. Wang, D. Liang, L. Liu, J. Zhang and Q. Zhu, Copper-catalyzed intramolecular dehydrogenative aminooxygenation: direct access to formyl-substituted aromatic N-heterocycles, Angew. Chem., Int. Ed., 2011, 50, 5678–5681 CrossRef CAS PubMed.
- A. K. Bagdi, M. Rahman, S. Santra, A. Majee and A. Hajra, Copper-Catalyzed Synthesis of Imidazo[1,2-a]pyridines through Tandem Imine Formation-Oxidative Cyclization under Ambient Air: One-Step Synthesis of Zolimidine on a Gram-Scale, Adv. Synth. Catal., 2013, 355, 1741–1747 CrossRef CAS.
- K.-S. Masters, T. R. M. Rauws, A. K. Yadav, W. A. Herrebout, B. Van der Veken and B. U. W. Maes, On the Importance of an Acid Additive in the Synthesis of Pyrido[1,2−a]benzimidazoles by Direct Copper-Catalyzed Amination, Chem. – Eur. J., 2011, 17, 6315–6320 CrossRef CAS PubMed.
- Y. Zhang, Z. Chen, W. Wu, Y. Zhang and W. Su, CuI-Catalyzed Aerobic Oxidative α-Aminaton Cyclization of Ketones to Access Aryl or Alkenyl-Substituted Imidazoheterocycles, J. Org. Chem., 2013, 78, 12494–12504 CrossRef CAS.
- H. Huang, X. Ji, X. Tang, M. Zhang, X. Li and H. Jiang, Conversion of Pyridine to Imidazo[1,2-a]pyridines by Copper-Catalyzed Aerobic Dehydrogenative Cyclization with Oxime Esters, Org. Lett., 2013, 15, 6254–6257 CrossRef CAS PubMed.
- J. Yu, Y. Jin, H. Zhang, X. Yang and H. Fu, Copper-Catalyzed Aerobic Oxidative C−H Functionalization of Substituted Pyridines: Synthesis of Imidazopyridine Derivatives, Chem. – Eur. J., 2013, 19, 16804–16808 CrossRef CAS PubMed.
- Q. Liao, L. Zhang, S. Li and C. Xi, Domino N−H/C−H Bond Activation: Copper-Catalyzed Synthesis of Nitrogen-Bridgehead Heterocycles Using Azoles and 1,4-Dihalo-1,3-dienes, Org. Lett., 2011, 13, 228–231 CrossRef CAS PubMed.
- S. Ding, Y. Yan and N. Jiao, Copper-catalyzed direct oxidative annulation of N-iminopyridinium ylides with terminal alkynes using O2 as oxidant, Chem. Commun., 2013, 49, 4250–4252 RSC.
- J. Lu, Y. Jin, H. Liu, Y. Jiang and H. Fu, Copper-Catalyzed Aerobic Oxidative Intramolecular Alkene C–H Amination Leading to N-Heterocycles, Org. Lett., 2011, 13, 3694–3697 CrossRef CAS PubMed.
- P. Sang, Y. Xie, J. Zou and Y. Zhang, Copper-Catalyzed Sequential Ullmann N-Arylation and Aerobic Oxidative C–H Amination: A Convenient Route to Indolo[1,2-c]quinazoline Derivatives, Org. Lett., 2012, 14, 3894–3897 CrossRef CAS PubMed.
- P. Sang, Y. Xie, J. Zou and Y. Zhang, Copper–Catalyzed Activation of Dioxygen: Oxidative Cyclization of 2−Arylindoles, Adv. Synth. Catal., 2012, 354, 1873–1878 CrossRef CAS.
- C. Wang, L. Zhang, A. Ren, P. Lu and Y. Wang, Cu-Catalyzed Synthesis of Tryptanthrin Derivatives from Substituted Indoles, Org. Lett., 2013, 15, 2982–2985 CrossRef CAS PubMed.
- Q. Liu, H. Yang, Y. Jiang, Y. Zhao and H. Fu, General and efficient copper-catalyzed aerobic oxidative synthesis of N-fused heterocycles using amino acids as the nitrogen source, RSC Adv., 2013, 3, 15636–15644 RSC.
- M. Li, Y. Xie, Y. Ye, Y. Zou, H. Jiang and W. Zeng, Cu(I)-Catalyzed Transannulation of N-Heteroaryl Aldehydes or Ketones with Alkylamines via C(sp3)–H Amination, Org. Lett., 2014, 16, 6232–6235 CrossRef CAS PubMed.
- H. Tian, H. Qiao, C. Zhu and H. Fu, Copper-catalyzed N-arylation and aerobic oxidation: one-pot synthesis of tetrahydroisoquinolino[2,1-a]quinazolinone derivatives, RSC Adv., 2014, 4, 2694–2704 RSC.
- H. Huang, J. Cai, X. Ji, F. Xiao, Y. Chen and G.-J. Deng, Internal Oxidant-Triggered Aerobic Oxygenation and Cyclization of Indoles under Copper Catalysis, Angew. Chem., Int. Ed., 2016, 55, 307–311 CrossRef CAS PubMed.
- X. Wang, Y. Jin, Y. Zhao, L. Zhu and H. Fu, Copper-Catalyzed Aerobic Oxidative Intramolecular C–H Amination Leading to Imidazobenzimidazole Derivatives, Org. Lett., 2012, 14, 452–455 CrossRef CAS PubMed.
- L. Chen, C. Li, X. Bi, H. Liu and R. Qiao, Copper-Catalyzed Aerobic Oxidative Synthesis of 5-Substituted Imidazo/Benzimidazoquinazolinones through Intramolecular C–H Amination, Adv. Synth. Catal., 2012, 354, 1773–1779 CrossRef CAS.
- W. Qian, H. Wang and J. Allen, Copper-catalyzed domino cycloaddition/C-N coupling/cyclization/(C-H arylation): an efficient three-component synthesis of nitrogen polyheterocycles, Angew. Chem., Int. Ed., 2013, 52, 10992–10996 CrossRef CAS PubMed.
- M. Wang, Y. Jin, H. Yang, H. Fu and L. Hu, Copper-catalyzed N-arylation and aerobic oxidative C–H/C–H coupling: one-pot synthesis of indoloimidazoquinoline derivatives, RSC Adv., 2013, 3, 8211–8214 RSC.
- D. Chen, Q. Chen, M. Liu, S. Dai, L. Huang, J. Yang and W. Bao, Cascade synthesis of azoquinazolinones by Cu(I)-catalyzed C–N coupling/C–H activation/C–N formation reactions under O2, Tetrahedron, 2013, 69, 6461–6467 CrossRef CAS.
-
(a) A. Kumar, M. Kumar, S. Maurya and R.-S. Khanna, Regioselective Synthesis of Fused Imidazo[1,2-a]pyrimidines via Intramolecular C–N Bond Formation/6-Endo-Dig Cycloisomerization, J. Org. Chem., 2014, 79, 6905–6912 CrossRef CAS PubMed;
(b) J. Wu, H. Luo, T. Wang, H. Sun, Q. Zhang and Y. Chai, Diverse synthesis of pyrimido[1,2-a]benzimidazoles and imidazo[2,1-b]benzothiazoles via CuI-catalyzed decarboxylic multicomponent reactions of heterocyclic azoles, aldehydes and alkynecarboxylic acids, Tetrahedron, 2019, 75, 1052–1063 CrossRef CAS.
-
(a) M. D′hooghe, K. Mollet, R. De Vreese, T. H. M. Jonckers, G. Dams and N. De Kimpe, Design, Synthesis, and Antiviral Evaluation of Purine-β-lactam and Purine-aminopropanol Hybrids, J. Med. Chem., 2012, 55, 5637–5641 CrossRef PubMed;
(b) M. J. Sofia, W. Chang, P. A. Furman, R. T. Mosley and B. S. Ross, Nucleoside, Nucleotide, and Non-Nucleoside Inhibitors of Hepatitis C Virus NS5B RNA-Dependent RNA-Polymerase, J. Med. Chem., 2012, 55, 2481–2531 CrossRef CAS PubMed;
(c) C.-H. Wu, C.-J. Wang, C.-P. Chang, Y.-C. Cheng, J.-S. Song, J.-J. Jan, M.-C. Chou, Y.-Y. Ke, J. Ma, Y.-C. Wong, T.-C. Hsieh, Y.-C. Tien, E. A. Gullen, C.-F. Lo, C.-Y. Cheng, Y.-W. Liu, A. A. Sadani, C.-H. Tsai, H.-P. Hsieh, L. K. Tsou and K.-S. Shia, Function-Oriented Development of CXCR4 Antagonists as Selective Human Immunodeficiency Virus (HIV)-1 Entry Inhibitors, J. Med. Chem., 2015, 58, 1452–1465 CrossRef CAS PubMed;
(d) C. Tintori, A. L. Fallacara, M. Radi, C. Zamperini, E. Dreassi, E. Crespan, G. Maga, S. Schenone, F. Musumeci, C. Brullo, A. Richters, F. Gasparrini, A. Angelucci, C. Festuccia, S. Delle Monache, D. Rauh and M. Botta, Combining X-ray Crystallography and Molecular Modeling toward the Optimization of Pyrazolo[3,4-d]pyrimidines as Potent c-Src Inhibitors Active in Vivo against Neuroblastoma, J. Med. Chem., 2015, 58, 347–361 CrossRef CAS PubMed.
- G.-R. Qu, L. Liang, H.-Y. Niu, W.-H. Rao, H.-M. Guo and J. S. Fossey, Copper-Catalyzed Synthesis of Purine-Fused Polycyclics, Org. Lett., 2012, 14, 4494–4497 CrossRef CAS PubMed.
- G. Yuan, H. Liu, J. Gao, K. Yang, Q. Niu, H. Mao, X. Wang and X. Lv, Copper-Catalyzed Domino Addition/Double Cyclization: An Approach to Polycyclic Benzimidazole Derivatives, J. Org. Chem., 2014, 79, 1749–1757 CrossRef CAS PubMed.
- J. He, M. Wasa, K. S. L. Chan, Q. Shao and J.-Q. Yu, Palladium-Catalyzed Transformations of Alkyl C–H Bonds, Chem. Rev., 2017, 117, 8754–8786 CrossRef CAS PubMed.
|
This journal is © the Partner Organisations 2020 |
Click here to see how this site uses Cookies. View our privacy policy here.