DOI:
10.1039/C9DT00765B
(Perspective)
Dalton Trans., 2019,
48, 9408-9421
Evolution and understanding of the d-block elements in the periodic table
Received
20th February 2019
, Accepted 6th March 2019
First published on 8th March 2019
Abstract
The d-block elements have played an essential role in the development of our present understanding of chemistry and in the evolution of the periodic table. On the occasion of the sesquicentenniel of the discovery of the periodic table by Mendeleev, it is appropriate to look at how these metals have influenced our understanding of periodicity and the relationships between elements.
Introduction
In the year 2019 we celebrate the sesquicentennial of the publication of the first modern form of the periodic table by Mendeleev (alternatively transliterated as Mendelejew, Mendelejeff, Mendeléeff, and Mendeléyev from the Cyrillic
).1 The periodic table lies at the core of our understanding of the properties of, and the relationships between, the 118 elements currently known (Fig. 1).2 A chemist can look at the periodic table and gain a deep understanding of the chemical relationships between elements and make reasoned predictions of chemical behaviour on the basis of the position within the table and the implied arrangement of valence shell electrons. The International Union of Pure and Applied Chemistry (IUPAC) is trusted with the custodianship of the periodic table and acts as the arbiter for the validation and nomenclature of new elements as well as providing the definitive list of the atomic weight of all elements.3 The latter task acquired a decidedly political aspect as the search for new elements became linked with cutting edge physics in the race for dominance in nuclear technologies.4 The naming of elements after prominent scientists also generated debate with the proposal to name element 106 Seaborgium, after Glenn Seaborg, which transgressed a previously unwritten (and unobserved in the case of einsteinium) rule that elements were not to be named after living researchers.5 However, the periodic table has achieved a cultural identity that far transcends its scientific relevance and has become one of the iconic scientific symbols recognized worldwide in all levels of society.6 It is only necessary to sketch the block structure of the periodic table for an audience to exhibit enlightenment and recognition. The arrangement of objects and concepts in rows and columns appeals to mankind's search for order in his world, and periodic tables concerning objects as diverse as fruit, vegetables, beer, cartoon characters, and superheroes abound in our connected world.7
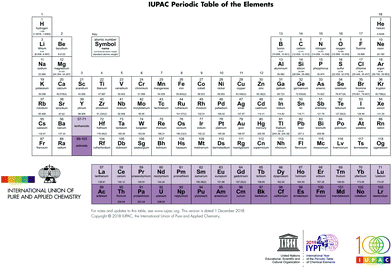 |
| Fig. 1 The modern medium length form of the periodic table (https://iupac.org/wp-content/uploads/2018/12/IUPAC_Periodic_Table-01Dec18.jpg). | |
In the commonly encountered medium or long forms of the periodic table, the central portion is occupied by the d-block elements, commonly known as the transition elements or transition metals. These elements have played a critical rôle in our understanding of modern chemistry and have proved to be the touchstones for many theories of valence and bonding. They continue to lie at the forefront of research in areas as diverse as materials science, catalysis, bioinorganic chemistry, materials science and photonic materials. However, many of these elements are scientific newcomers and their history is, in a way, also the history of the development of the periodic table. This article provides an overview of how the elements in groups 3–12 influenced the development and refinement of our modern periodic table.
Today we use the terms transition metal, transition series and transition element interchangeably and with an implicit understanding of which elements they refer to. However, these terms are, at least in English, neologisms dating to the second decade of the last century.8 In this article, I have attempted to use the less-emotive term “d-block element”. In general, the elements of group 12 are not accepted as transition elements, but rather as d-block elements with a chemistry closely related to the elements of group 2.9
The prehistory of d-block elements
The earliest metals
Some metals have been associated not only with the history of the periodic table, but also irrevocably with the ascent of Homo sapiens. From pre-history to the beginning of the Common Era, only the seven metals, gold, silver, copper, iron, mercury, tin and lead were widely known to man.10 Of these, the first five are d-block elements found in the central part of the periodic table. A few metals occur in the elemental form in the biosphere. Notable amongst these are gold, copper (Fig. 2) and silver, which were the first to be utilized in the ancient world, with gold and copper being in the historic record since at least 9000 BCE. The earliest civilizations of which we are aware prized silver and gold both as valuable fiscal resources and for their attractive appearances, leading to their use in decorative art and as a signal of privilege and status. Furthermore, the distinctive colours of metallic silver and gold resulted in their acquiring religious and mystical rôles, primarily through association with the Moon and the Sun respectively. Despite their relative scarcity, both gold and silver are widely-distributed and most civilizations and emerging civilizations would have had access to these elements in small amounts. Silver and gold are both soft and low melting, with the consequence that they found little application as mechanical tools or weapons. In contrast, copper found early application, for example as the basis of knives used in the domestic environment and the period 6000–4000 BCE is known as the copper age. Although relatively hard, pure copper itself is not hard enough to use for weapons or armour. For this development, a new technological innovation was needed – metallurgy and the isolation of elemental metals from their naturally occurring ores. Probably the first metal to be isolated by reductive pyrometallurgy was copper, most likely obtained from sulfide ores about 4000 BCE. The copper obtained from metallurgy sometimes had different properties to native copper; in particular, a much harder material was obtained from arsenic-containing ores, which we know now as the arsenic-bronzes. By refining mixtures of copper and tin ores, the harder and stronger tin-bronzes were obtained which allowed the manufacture of the new “superweapons” and the genesis of the bronze age 3500–1500 BCE. The near-contemporary epic poem, The Iliad, describes warfare using state-of-the-art bronze military technology. Although the tin-bronzes were extremely successful, they also represent a very early example of a technology limited by resource availability – tin ores were only found in very limited sites in the classical known world.
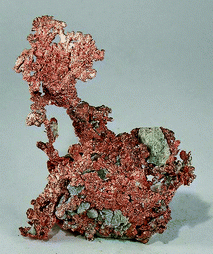 |
| Fig. 2 The earliest metals to be utilized by man were those which occurred in the elemental form, such as copper. | |
Although the core of our planet is composed of molten elemental iron, only small amounts of the elemental metal or alloys occur in the biosphere, mostly in material from meteorites. The metallurgical extraction of iron from oxides and other ores probably began in South Asia in the third millennium BCE and became incorporated in the European and Near Eastern historiography from about 2000 BCE onwards. As a technological development, iron and steels of various types represented a disruptive innovation that signalled the end of the bronze age and the commencement of the iron age.
The final metal known to antiquity was mercury, unique at the time in being liquid at ambient temperature. Mercury was probably first known in the Far East and China as early as 2000 BCE and was later well established in the early Greek and Roman cultures.
The first millennium CE
In Eurocentric histories, the first millennium CE is described as the dark ages. Although these years provided the material content for literary classics such as The Decameron,11 The Canterbury Tales,12 El Cid13 and Le Morte D'Arthur14 it did not add new metals to the centre of the periodic table. The only candidate might be zinc, which had been known in the form of alloys such as brass in the East and Europe since pre-history. However, there is little compelling evidence for the isolation of metallic zinc in this period. This almost certainly reflects the fact that zinc is volatile and pyrometallurgical conditions leading to its formation from zinc ores would also vaporize the metal. It was not until about 12th century CE that elemental zinc was deliberately prepared by smelting.
The beginning of the scientific era … up to the 18th Century CE
The period of history up to the 18th Century CE is very easy to define in terms of the discovery of the d-block elements. Nothing happened! Or more precisely, no new elements were identified, although the nature and properties of zinc compounds was clarified and expanded. Indeed, associated with my adopted city of Basel, Theophrastus Bombastus de Hohenheim (better known as Paracelsus) reintroduced the medicinal actions of zinc compounds to Western Europe, although the original observations date back to Pliny and Dioscorides.15 Indeed, the name zinc is usually credited to Paracelsus, who wrote thus: “Concerning zinc. Moreover, there is a certain metal, not commonly known, called zinc. It is of peculiar nature and origin. Many metals are adulterated in it. The metal of itself is fluid, because it is generated from three fluid primals. It does not admit of hammering, only of fusion. Its colours are different from other colours, so that it resembles no other metals in the condition of growth. Such, I say, is this metal that its ultimate matter, to me at least, is not yet fully known. It does not admit of admixture; nor does it allow the fabrications of other metals. It stands alone by itself”.16 The last two sentences come dangerously close to predating the Lavoisier definition of an element!17
The 18th Century CE and the modern definition of an element
The new elements.
The 18th Century CE was a turning point in the development of chemistry. In this Century, many intellectuals turned to the study of chemistry, and information was disseminated through the fledgling learned societies such as the Deutsche Akademie der Naturfischer Leopoldina (1652), the Royal Society (founded 1660), the Académie des sciences (established 1666). The understanding of the natural world, combined with the commercial imperatives of mining, the search for new materials and new medicines, resulted in numerous elements being identified or isolated. Although many of these elements were undoubtedly known previously (for example, Paracelsus talks of cobalt), the 18th Century saw the first isolation of the pure element or the first scientific description of its compounds.
Probably the first of the “new” metals of the 18th Century was cobalt, although as mentioned above it was really an “old element”. The beautiful blue colours of cobalt glazes and cobalt glasses were known since antiquity. Chemists in Sweden made enormous contributions to the discovery of elements in the 18th Century, and so it is appropriate that the honour of the (re)discovery of cobalt in 1835 goes to the Swedish chemist Georg Brandt. German metallurgists had identified a mineral in mines in Saxony, but did not systematically investigate it. The miners named this mineral Kobold, after a malevolent gnome which haunted underground places and mines, referring both to the unwanted nature of the material and its negative effects on their health, due to its occurrence with arsenic. Brandt isolated reasonably pure samples of cobalt and cobalt compounds sometime between 1735 and 1739.18 One of his major aims was to distinguish the element from bismuth, with which it occurs naturally, and it is of note that he described cobalt not as a metal but as a semi-metal. Cobalt compounds were critical to the later pioneering investigations of coordination chemistry by Alfred Werner,19 but it is also worthy of note that one of the earliest scientific descriptions of the preparation of a coordination compound, [Co(NH3)6]3+, dates back to a report from Paris at the height of the French revolution in 1798: “another rather surprising phenomenon is that, when cobalt nitrate is precipitated by excess ammonia, a precipitate is formed which is immediately re-dissolved to give a brown solution; but if this solution is immediately treated with a lot of water, a green precipitate is formed consisting only of pure cobalt oxide, which dissolves in acids, and gives solutions of a beautiful pink color; if we leave the cobalt solution in ammonia exposed to air for a long period, we can dilute it with as much water as we wish, without it forming a precipitate”.20
Platinum was known and used widely in South America in the period before the European discovery of the continent. However, little is known or documented regarding this pre-history. Although platinum most commonly occurs in the native form, it does not appear to have been documented in Europe and the earliest reference appears to date to 1557 when Julius Caesar Scaliger who described a metal which could not be melted by technology of the time.21 Indeed, this property lead Scaliger to challenge Gerolamo Cardano who in 1550 had defined a metal as “a substance that can be melted and hardens on cooling”.22 Scaliger says “… I know that in Honduras, a district between Mexico and Darien, there are mines containing a substance which it has not hitherto been possible to melt by fire or by any of the Spanish arts”.23 The first scientific description of platinum dates to 1748, when Don Antonio de Ulloa described both its hardness and that it could not be calcined.24 Although preliminary studies of the chemistry were made by Spanish and other European scientists, the occurrence of significant reserves of native platinum in Russia meant that the early systematic studies were associated with Russian chemists.25
We return to Sweden for the discovery of nickel by Axel Fredrik Cronstedt, in 1751. He identified nickel from a new mineral discovered near Halsingland and the ore Kupfernickel, which was used to make green glasses. Cronstedt isolated the new metal which he described as “hard and brittle” and “only feebly attracted by the magnet”26 and called it nickel.27 The discovery was not entirely without controversy, and a number of eminent chemists considered that nickel was not an element but rather an alloy of other metals or a modification of iron.12
The next metallic element to be isolated was manganese. Once again, compounds of manganese had been known for many years, most notably the mineral pyrolusite (MnO2) which was also variously called black magnesia and manganese, and KMnO4 which had been first described in 1659.28 By the 18th Century, there was agreement that a new metal was present, but even great scientists such as Pott, Bergman, and Scheele were unable to reduce MnO2 to its parent metal. That distinction belonged to Johan Gottlieb Gahn who obtained the new metal by the reduction of pyrolusite with carbon. Gahn never published his preparation of manganese, and his priority claim arises from correspondence with Scheele, who in June 1774 thanked him for a sample of metallic manganese.29
By the last two decades of the 18th Century, elements were being discovered with increasing frequency and by 1797, the list of d-block elements had been expanded to include molybdenum (1781), tungsten (1783), zirconium (1789), titanium (1791), yttrium (1794) and chromium (1797). These latterly identified elements have a number of aspects in common; all were found in natural minerals and often misidentified in the first analysis. Subsequent isolation of pure(ish) salts or oxides resulted in the recognition that a new element was present, and, in many cases, reduction with charcoal yielded the new metal.
The recognition that the mineral molybdena (MoS2, now molybdenite) contained a new element that he called molybdenum is due to Carl Wilhelm Scheele in 1778,30 and the reduction to the metal with carbon was achieved by Peter Jacob Hjelm in 1781.31 A similar sequence of events, albeit etymologically more complex, is found in the case of tungsten. Here, tungsten trioxide was isolated from the ore scheelite (at the time called tung sten) by Carl Wilhelm Scheele in 178132 and Torbern Bergman suggested that it contained a hitherto unknown metal.33 Two years later José and Fausto Elhuyar succeeded in isolating the new element, which they called variously wolfram or volfram, by reduction of WO3 with charcoal.34 The name Wolfram persists in the German-speaking world and in the symbol W for the element. In the case, of chromium, Johann Gottlob Lehmann's first analysis of the mineral crocoisite (also Siberian red lead or crocoite, PbCrO4) was in 1762, but he neither published his results nor identified it as containing a new metal. The material was later utilized as a pigment before the isolation of CrO3 by Louis Vauquelin in 179735 and its subsequent reduction with charcoal in 1798.36–38
The sequence of events for the remaining elements is a little more interesting. Although the new elements were identified in their oxides, their isolation needed to wait for electricity (or rather the availability of the group I metals obtained by electrolysis). Martin Heinrich Klaproth identified ZrO2 (zirkonerde, now zirconia) as the oxide of a new element in 1789,39 but the new metal was only obtained by Berzelius in 1824 by the reduction of K2ZrF6 with potassium in a reaction he described in a letter to Pierre Dulong. Titanium was identified in the mineral ilmenite by William Gregor in 179140 and named by Martin Heinrich Klaproth (who investigated rutile) in 1795 “…I shall borrow the name for this metallic substance from mythology, and in particular from the Titans, the first sons of the earth. I therefore call this new metallic genus TITANIUM”.41 It took almost another 100 years before impure titanium metal was isolated in 1887 by Lars Nilson and Otto Pettersson by the reduction of TiCl4 with sodium at high temperature and pressure.42 The story of yttrium is also a little convoluted. Carl Axel Arrhenius discovered a new mineral that he called ytterbite in 178743 and in 1794 Johan Gadolin concluded that it contained a new metal;44 it fell to Anders Gustaf Ekeberg to call the new oxide yttria.45 As it happened, the material was not a pure sample of Y2O3, but a mixture of the oxides of yttrium, terbium (called erbium at the time) and erbium (called terbium in the contemporary literature) and the mixture was renamed gadolinite. It was only in 1843 that Heinrich Rose succeeded in isolating metallic yttrium.46 If the confusion of erbium and terbium were not enough, the elemental symbol for yttrium was Yt until the 1920s when it was changed to the (present) symbol of Y.
Lavoisier and the new meaning of “element”.
By the end of the 18th Century, the chemical world was in a state of conceptual confusion. Many new elements had been identified, including some 16 d-block metals, but unfortunately, no-one knew what an element was! Slowly, the description element was evolving from a philosophical concept to a physical description. Empedocles (c. 490–c. 430 BCE) developed a philosophy with four “roots” (earth, air, fire and water) and forces of attraction and repulsion allowing all physical materials to be defined in terms of various ratios of the four “roots”. This philosophical construct was further developed by Plato (c. 428–c. 348 BCE), who also renamed the “roots” elements (στοιχεῖον, stoicheion) and established a physical model that was to survive for almost two thousand years. The beauty of this philosophical structure was that it allowed the properties of matter (hot, dry, cold, moist) to be related to the ratios of the elements contained; thus, a material composed primarily of water and earth would be cold, whereas one from fire and earth would be dry. This linking of the physical constitution with the properties was ultimately to be the downfall of the model and the confusion of heat with physical material change lay at the origin of the phlogiston debate.
The first attempt to bring some sense of order, at least in the English language, can be attributed to Robert Boyle.47 His 1661 book “The Sceptical Chymist” is a resounding attack upon Aristotelianism. In one passage, he sets the scene for a cosmology in which elements are composed of corpuscles (atoms) of a single type which can be combined to make compounds: “that I now mean by elements, as those Chymists that speak plainest do by their Principles, certain Primitive and Simple, or perfectly unmingled bodies; which not being made of any other bodies, or of one another, are the Ingredients of which all those call'd perfectly mixt Bodies are immediately compounded, and into which they are ultimately resolved: now whether there be any one such body to be constantly met with in all, and each, of those that are said to be Elemented bodies, is the thing I now question”. Nevertheless, the Sceptical Chymist is written as a discourse and is as difficult to read today as it was when it was first written and his valid scientific comments found little acceptance in the contemporary scientific community.
The breakthrough came with the publication of Traité Élementaire de Chimie (Elements of Chemistry in a New Systematic Order containing All the Modern Discoveries) by Antoine-laurent Lavoisier in 1789. This work started out as an exercise in revising the nomenclature of chemistry and delivered a modern definition of an element based upon empirical observations in. “in. The subject only furnishes us with indefinite problems, which may be solved in a thousand different ways, not one of which, in all probability, is consistent with nature. I shall therefore only add upon this subject, that if, by the term elements, we mean to express those simple and indivisible atoms of which matter is composed, it is extremely probable we know nothing at all about them; but, if we apply the term elements, or principles of bodies, to express our idea of the last point which analysis is capable of reaching, we must admit, as elements, all the substances into which we are capable, by any means, to reduce bodies by decomposition”.14 This book by Lavoisier was also the compilation of the arguments countering the then prevalent phlogiston theory.48 Another important aspect of the Lavoisier work was the identification of oxides of metals that had not yet been isolated in the elemental form, a feature that presaged the inclusion of gaps in the Mendeleev periodic table for elements that had not yet been identified. As far as the d-block is concerned, the Lavoisier list of elements included silver, cobalt, copper, iron, manganese, mercury, molybdenum, nickel, gold, platinum tungsten and zinc (Fig. 3).
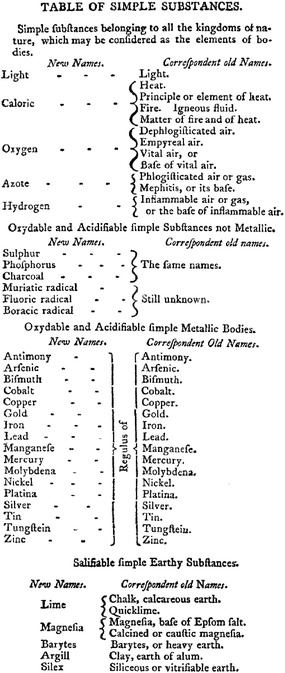 |
| Fig. 3 The table of elements prepared by Lavoisier in his 1879 book, Traité Élementaire de Chimie. | |
The 19th Century CE up to 1869
The 19th Century CE saw the fleshing out of the periodic table as ever more new elements were identified and isolated. From the point of view of the d-block metals, this period saw the establishment of vanadium (1801), niobium (1801), tantalum (1802), palladium (1802), osmium (1803), iridium (1803), rhodium (1804), cadmium (1817) and ruthenium (1844). In most cases, these were first identified in minerals and the elements were later isolated using reductive methods.
For the historian of the periodic table, the only caveat lies in the naming of the element niobium. This was originally identified in 1801 by Charles Hatchett in a mineral he named columbite49 and he subsequently named the new element columbium with the symbol Cb.50 This name was used, in particular in the American literature, until 1949, when IUPAC decided in favour of the name niobium (with symbol Nb) coined by Heinrich Rose in 1844.51
The story was not, however, quite as simple as the preceding paragraph might indicate. Although the nine new elements were successfully identified, numerous other “new elements” were proposed and either discredited or shown to be mixtures of other known and unknown elements. This fascinating part of chemical history does not belong to our story today beyond imparting some confusion in the contemporary literature! Many of these elements of fantasy belonged to the f-block, but a number of d-block elements such as phillipium, polinium, ilmenium, pelopium, davyum, nipponium and masurium were proposed and have been consigned to the annals (if not the rubbish bins) of history!52 These comments should not be regarded as critical of the skills of the contemporary chemists – in the absence of the periodic table they knew not how many elements were to be expected. Furthermore, they lacked the light that future spectroscopic methods were to bring into the chemical turmoil.
Fig. 4 presents the d-block elements known at critical points in chemical history and maybe shows why developments occurred at particular times. By 1800, enough elements were known that the contribution of Lavoisier, with the modern definition of an element, perhaps became inevitable. Similarly, by 1869, Mendeleev had a palette of elements in the 3d, 4d and 5d series that was almost complete. Only five d-block elements were not known, allowing the periodic trends within a group and within a row to be identified.
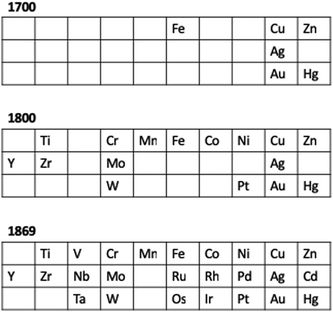 |
| Fig. 4 An overview of the elements of the 3d, 4d and 5d series known at critical points in chemical history. | |
Early attempts to bring order to the elements
Although the name of Dmitri Mendeleev is irrevocably linked with the discovery of the periodic table, there are a number of significant earlier studies which laid the basis for his work. Humans are creatures who seek order in the universe, and by the middle of the 19th Century CE, the time was probably right for the discovery of the periodic table, which would allow elements to be ordered by property according to some quantitative feature. A large number of elements were now known and the concepts of valence which were developing in parallel concentrated scientific attention on the relationships between them. The critical ingredient in this heady mix owed its genesis to work at the turn of the century. The organization principle selected was to be atomic mass. Relative atomic masses were introduced at the very beginning of the 19th Century BCE by John Dalton53,54 and Thomas Thomson55 and had been accepted in the mainstream of chemical knowledge by the 1860s.
The d-block elements in pre-Mendeleev searches for periodicity
Döbereiner.
In 1829 Johann Wolfgang Döbereiner published an article entitled “Versuch zu einer Gruppierung der elementaren Stoffe nach ihrer Analogie” (“Attempts to group elements by their similarities”) in which he noticed that some elements which showed similar chemical properties could be arranged in groups of three (triads) such that the atomic weight of the middle element was the arithmetic mean of the first and last members.56 Although the most successful triads were identified in the main group elements, he nevertheless grouped iron, chromium and manganese in a triad (based on the metal oxides). He seemed to have reservations about a second triad of nickel, copper and zinc based on discrepancies in their densities. More interestingly, he identified triads in the 4d and 5d elements, grouping palladium, rhodium, and pluranium together as well as platinum, iridium, and osmium. Pluranium was almost certainly a mixture of various 4d elements and can be ignored. He seems to have had a good chemical intuition on what was needed and the final sentence of the article reads “whether zinc and cadmium, antimony and bis muth, gold and tungsten or tungsten and tantalum etc. belong together, … and which may be missing analog parts of it, I dare not decide”. Although the mathematical groupings were correct, it was only in the vertical relationships (Li, Na, K; Cl, Br, I) that he anticipated the broader periodic table. The horizontal relationships between the transition elements reflected sequential filling of the d-orbitals rather than a jump in principal quantum number. Indeed, Leopold Gmelin pointed out that the differences in atomic masses of the triads involving the d-block elements were much smaller than those in the main group, predating the concepts of periodicity within groups and within rows of the periodic table.57
Cooke.
The ideas of Döbereiner can be found in a publication by Josiah Cooke Jr. from 1854 in which some relationships between d-block elements such as chromium and molybdenum are identified on the basis of chemical similarity and patterns in the atomic weights.58 This paper has been largely ignored by historians of the periodic table, in part due to its length and obscurity of argument. Nevertheless, the final paragraph is revealing “…we can make a table which shall contain, not only every known substance, but also every possible one, and when by means of a few general formulae we shall be able to express all the properties of matter … we shall be able to calculate, nay, predict, its properties with absolute certainty”.
de Chancourtois.
By the 1860s, numerous scientists in Europe were independently thinking about the arrangement of the elements by atomic weight and trying to understand trends and similarities in the chemistry of different. One of the earliest such arrangements, and also one of that is less familiar to the modrn chemist, is that of the geologist Émile Béguyer de Chancourtois. He developed a rather complex three-dimensional system with the elements arranged helically in atomic weight order onto a cylinder in his Mémoire sur un classement naturel des corps simples ou radicaux appelé vis tellurique. The circumference of the cylinder comprised 16 elements.59 This description is rather complicated and it is exceptionally unfortunate that the diagrams essential to understanding the relationships of the elements were omitted from the original publication53 and two subsequent articles in the same year.60,61 Around the cylinder, the 17th element appeared below the first one and the vertical relationships generate groups of elements. Within these vertical groups, familiar patterns emerge with the group 1 and group 2 elements forming recognizable groups. Unfortunately, the case for the d-block elements is not so rewarding, and, at best, they find positions with a vertical relationship to the group n + 10 elements, echoing the later A and B group nomenclature (Fig. 5).
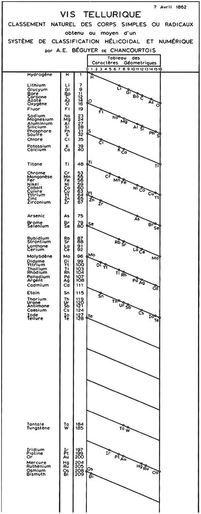 |
| Fig. 5 The vis tellurique of de Chancourtois in which chemical groups have a vertical relationship. | |
Odling.
Probably the next earliest periodic table in the modern sense is that due to William Odling (Fig. 6).62 In this periodic table, the primary arrangement is on the basis of atomic weight, with the modern groups arranged horizontally. The positioning of the d-block elements offers a little chemical insight into their relationships, although these elements are not discussed in any detail in the text. Nevertheless, the further relationships of the Döbereiner type could be discerned, and in particular Cr, Mo and W (as well as V) were assigned to the same triad, as were Ti and Zr, Zn, Cd and Hg, and Au and Ag. It is worth noting, that the atomic weight for vanadium seems anomalous, leading to its being placed in what would become the 4d series and that the first entry for Pt in the table (with a mass of 106.5) is a misprint for Pd. It is also of interest that the “noble metals”, rhodium, ruthenium, platinum, palladium, iridium and osmium are grouped together. The numerology in the paper is perfectly understood in terms of modern quantum theory, but results in the pairing of elements in triads related by the A and B group relationships that emerged in the post-Mendeleev period (for example molybdenum and selenium, chromium and sulfur or silicon and titanium). These pairings made some sense in terms of valence, but not in terms of chemical behaviour. The modern reader of this paper should also beware that some of the atomic symbols may be unfamiliar; notwithstanding the typographical error for Pd, the symbol L is used for lithium, G for beryllium (glucinium), Ro for rhodium and Cb for niobium. In the absence of quantum theory in 1864, one can only sympathize with the final sentences of the publication “doubtless some of the arithmetical relations exemplified in the foregoing tables and remarks are simply accidental; but taken altogether, they are too numerous and decided not to depend upon some hitherto unrecognized general law”. Perceptive words indeed.
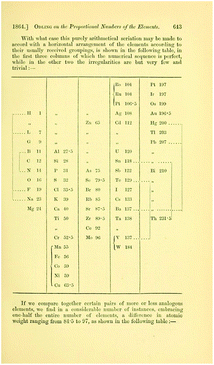 |
| Fig. 6 The table from Odling showing the periodic behaviour within each (horizontal) group (retrieved from https://www.biodiversitylibrary.org/item/52516#page/675/mode/1up).53 | |
Newlands.
In 1865, John Newlands, also arranged elements in order of their atomic weights and commented “… that the numbers of analogous elements generally differ by 7 or some multiple of seven; in other words, members of the same group stand to each other in the same relation as the extremities of one or more octaves in music. … This peculiar relationship I propose to provisionally term the “Law of Octaves”.63 The success of this original publication for the d-block elements is open to interpretation. The elements, cobalt, nickel, palladium, platinum and iridium are placed in an octet with hydrogen, fluorine, chlorine, bromine and iodine. Similarly, copper and silver are placed with the present day group 1, zinc with group 2, chromium, yttrium and tantalum with group 3, titanium, zirconium, tungsten and mercury with group 14, manganese, molybdenum and niobium with group 15 and iron, rhodium and ruthenium, gold and osmium with group 16. Some of this rather wild grouping is due to anomalies in the atomic numbers, but it gives an image of a pattern sought on the basis of mathematics rather than chemical similarity. Again, the modern reader needs again to be cautious of the atomic symbols Ro and G as well as Bo (for boron) and Di for didymium (a metal subsequently shown to be a mixture of various f-block elements).
The Mendeleev era
In 1869, within a few months of each other, Dmitri Mendeleev and Lothar Meyer independently published very similar periodic tables that we recognize as the first of the “modern” arrangements of the elelements. The elements were arranged by their atomic masses. Both Mendeleev and Meyer recognized that in order to maintain chemical periodicity, certain elements had to be placed at a different position in the periodic table than their atomic mass would suggest. Although this was the basis for much debate at the time, we now recognize that the sequence of atomic masses does not exactly follow the atomic number (only quantified as the number of protons in the nucleus of an element in 1913 by Moseley64–66). Modern periodic tables are always ordered by atomic number rather than atomic mass.
Meyer
It might seem somewhat perverse to commence a section headed the Mendeleev era by talking about an arch-rival, Julius Lothar Meyer. However, as mentioned on a number of occasions, the time was right for the discovery of the periodic table in the 1860s and Meyer was thinking along almost identical lines to Mendeleev. At the same time as Mendeleev was developing his periodic table, Lothar Meyer was doing the same thing in Breslau. One of the earliest versions was published in 1864 in his book Die Modernen Theorien der Chemie, but did not include any of the d-block elements.67 For the second edition of his book in 1868, Meyer had prepared an expanded version of his table with 52 elements in it, including manganese, ruthenium, platinum, iron, rhodium, iridium, cobalt, palladium, osmium, copper, silver, gold, itatanium, zirconium, tantalum, zinc, cadmium, mercury, molybdenum, vanadium (Vd) and tungsten. This periodic table is very similar indeed to that published by Mendeleev in 1869. Unfortunately, in the same way that the critical diagrams were omitted from the article by De Chancourtois, this revised periodic table from Meyer got omitted from the book. It was only discovered and published by Karl Seubert in 1895, after the death of Meyer himself.68 If the 1868 version had been published, we might have celebrated the year of Meyer's periodic table in 2018. Maybe the most fitting memorial is that Seubert collected the work of both Mendeleev and Meyer in what is, I believe, the first book to be published on the periodic table “Das natürliche System der chemischen Elemente” in 1895.69 It is from this publication that we can see the full 1868 periodic table of Meyer (Fig. 7).
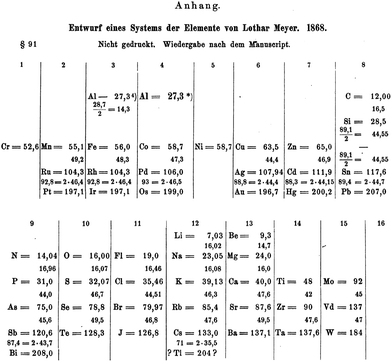 |
| Fig. 7 The expanded periodic table from Meyer that was prepared in 1868 but only published in 1895.68 | |
Mendeleev's periodic table
Mendeleev's periodic table was first published in Russia in 186970 and shortly afterwards abstracted in German in the same year (Fig. 8).71 These early versions of his periodic table were arranged such that the “groups” were horizontal. The success of the Mendeleev formulation, and the reason usually cited for his being given priority for the discovery of the periodic table, is his identification of gaps for hitherto unidentified elements.
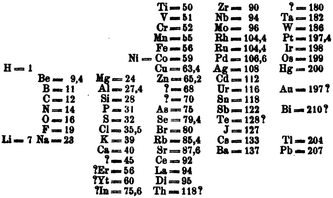 |
| Fig. 8 The version of the periodic table that Mendeleev published in Z. Chem. in 1869.64 | |
Fig. 8 sheds light onto his placing of the d-block elements. Firstly, he identifies unknown elements of atomic mass 45 and 180, the former of which can probably be identified with scandium, although this is not certain, and the latter with hafnium. The vertical columns correspond to the rows of the modern periodic table and the genesis of the 3d, 4d and 5d-blocks are seen. In the horizontal rows we see a great deal of chemical insight in placing the elements in the familiar groups. A combination of uncertainty in atomic mass, utilizing atomic mass instead of atomic number and not being aware of the missing five d-block metals, there are a number of anomalies in the table. Most notably is the placing of the elements platinum, rhodium, iridium, osmium, mercury and gold in the incorrect groups.
The periodic table was an ongoing piece of research for Mendeleev, and by 1871 (Fig. 9)72 he had rotated the arrangement through 90° to give the familiar modern arrangement of vertical groups and horizontal rows. Also notable in this version is the explicit inclusion of group numbers. Copper, silver and gold are included with group 1, predating the subsequent A and B nomenclature. Once again, this grouping makes chemical sense with all of the group 1 and group 11 elements exhibiting a characteristic +1 valence state. Similarly, zinc, cadmium and mercury are grouped together with the modern group 2 elements, reflecting the common +2 valence exhibited by these elements. The elements of groups 3–7 are associated with those of the modern groups 13–17. Once again, these assignments are based on good chemical analogies, for example [CrO4]2– and [SO4]2–, TiCl4 and SiCl4 as well as [MnO4]− and [ClO4]−. The later d-block elements are grouped together in a Group VIII that has no modern analogy (the inert gases of the modern group 18 had not been discovered in 1869). In the German literature at the end of the 19th Century CE, these elements were generally described as übergangsmetalle (transitional metals). Also notable is the placing of the group 11 elements in Mendeleev's groups 1 and 8.
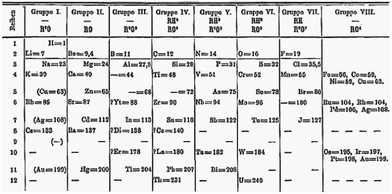 |
| Fig. 9 The 1871 version of Mendeleev's periodic table with the more familiar arrangement of groups and periods. | |
What belongs where – the origins of the A/B paradigm
The noble gases.
The Mendeleev periodic table had two features which have impact on our interest in the d-block elements. The first is his collection together of the “odds and sods” of the later transition elements into the chemically irrational Group VIII. Developments in science outside the d-block elements were to render this arrangement redundant. The discovery of the noble gases by Ramsay73 was the catalyst for the first major revision of the periodic table. The noble gases were seen to be a problem and Ramsay championed their inclusion in the periodic table as a new group. The incorporation of the new elements was not easy and Mendeleev was not initially convinced that elements which did not form compounds should be included in his periodic table.74 In an article entitled “Uber die Stellung der Elemente der seltenen Erden im periodischen System von Mendelejeff” Bohuslav Brauner presents an extended form of the periodic table (Fig. 10), in which the lanthanoids are grouped together in a new arrangement and the noble gases are placed at the left hand side and labelled group 0.75
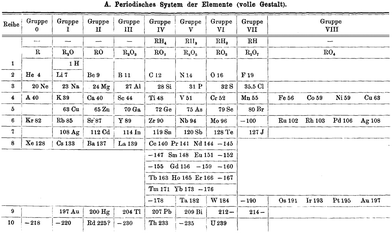 |
| Fig. 10 The Brauner periodic table from 1902 in which the lanthanoids have a special position and the noble gases are incorporated as a new group 0. | |
In chemical terms, group 0 is actually rather more logical than the present group 18. The placing of the noble gases in group 18 is a natural consequence of the ordering by atomic number, whereas the nomenclature group 0 is a good indication that these elements are primarily encountered in the elemental (oxidation state 0) form. In 1904, Ramsay relocated them to group 8 and moved them to the right-hand side (Fig. 11) where they were again grouped with those pesky late d-block elements.76 where they remain today.
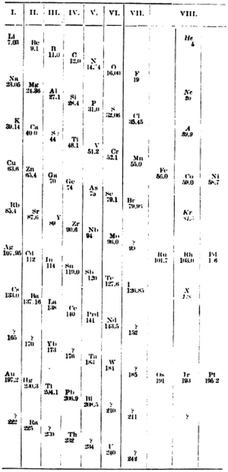 |
| Fig. 11 The Ramsay inclusion of the noble gases in group VIII of the periodic table.76 | |
A publication without Werner is like a meal without wine77
For much of the 20th Century CE, the chemistry of the d-block elements was synonymous with coordination chemistry. The father of coordination chemistry was Alfred Werner and his pioneering studies at the University of Zürich not only culminated in his being awarded the 1913 Nobel Prize for chemistry, but also laid the basis for our present day understanding of the field. Did Werner also make any significant contributions to the development of the periodic table? This appears to be a relatively undocumented aspect of his chemical oeuvre, but one that is extremely relevant to this article. In 1905, Werner published two quite remarkable articles entitled “Beitrag zum Ausbau des periodischen Systems” (Contribution to the expansion of the periodic systems)78 and “Zur periodischen Anordnung der Elemente” (about the periodic arrangement of the elements),79 the latter being a response to a critical comment on the first publication by Abegg, who stated “so, if my view, which differs from Mr. Werner's, regarding the arrangement of the periodic system is in the final place a matter of taste, it can certainly be said that the metals of the iron group have a completely natural place in the 8th group together with the noble gases”.80 For us, this long-forgotten chemical dispute is less important than the content of the first of Werner's 1905 papers. In this publication he addresses the problem of where to place the d-block elements (see the next section also) and proposes a periodic table (Fig. 12) that is almost a twin to the modern table presented in Fig. 1.
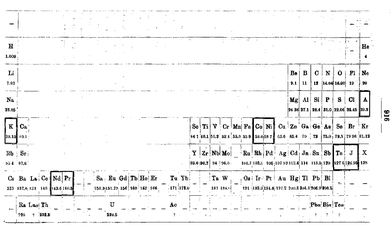 |
| Fig. 12 Werner's periodic table from 1905 with the inclusion of the d-block (and f-block) elements in an arrangement very similar to the modern long-form periodic table.78 | |
The first publication also contains a very early, and possibly the first, use of the description of the d-block elements as a transition series “Die Anordnung der Elemente in der nun vorgeschlagenen Form zeigt, dass sich die höheren, grösseren Perioden aus den kleineren durch Einschiebung von Zwiechenelementen entwickeln, welche Letzteren durch ihren Charakter eine Art Uebergangsreihe zwischen den beiden Elementen, zwischen die sie eingeschoben sind, darstellen, sodass sie gleichsam Variationen dieser Elemente bilden” (the arrangement of the elements in the proposed form shows that the higher, larger periods can be separated from the smaller ones by inserting intermediate elements which, due to their character, represent a kind of transition series between the two neighbouring elements between which they are inserted, so that they form, as it were, variations of these elements).
The years of confusion.
From about 1920 onwards, the periodic table was accepted without discussion in the chemical community and had adopted its present rôle as the core organizing principle for understanding trends and properties. It is of interest to investigate the periodic table included in H. G. Deming's General Chemistry, from 1923 (Fig. 13).81
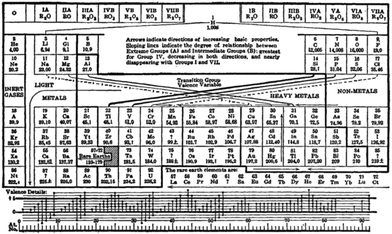 |
| Fig. 13 Deming's periodic table from 1923.76 | |
This periodic table has a number of interesting features. Firstly, the noble gases remain on the left hand side in group 0, but within a given Mendeleev group the d-block elements have been separated from the main group elements by the use of an A and B nomenclature. The nomenclature was chemically driven and drew the analogy between the typical oxides of the A and B members of a given group (for example, CrO3 and SO3, or Mn2O7 and Cl2O7).
Unfortunately, European and American usage of this nomenclature was orthogonal and the literature is exceptionally confusing. Fig. 14 shows a summary of the various nomenclature systems that were used in parallel up to the middle of the 20th Century CE. As the decision making body for nomenclature, IUPAC was prompted to act, and in 1988 issued a report predicated upon the observation “an investigation of the application of the subgroup designations A and B in all articles, which appeared between 1972 and 1981 and covered by Chemical Abstracts, revealed a completely arbitrary use of the designations”.82 In this same report, IUPAC recommended the use of the system of naming the groups with the numbers 1 to 18, that was definitively adopted in the recommendations for nomenclature in inorganic chemistry (“The Red Book”) in 199083 and 200584 and is now universally accepted.
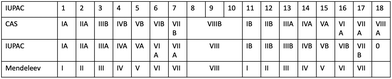 |
| Fig. 14 The various naming systems used in the course of the first part of the 20th Century CE to describe the groups in the periodic table. | |
Post-Mendeleev – filling the gaps
As noted earlier, by 1869 all of the 3d, 4d and 5d elements were known with the exception of scandium, lanthanum, hafnium, technetium and rhenium. Lanthanum we will not consider further as it should be placed as the first member of the 4f series of elements. Nevertheless, it is relevant to make an aside that the elements we know today as the lanthanoids caused considerable anguish to Mendeleev and his fellow chemists in the 19th and early 20th Century CE. These elements possessed very similar chemical properties and the literature contains numerous reports of the false identification of new elements or the claim of a new element, which was subsequently shown to be a mixture of two (or more) other elements. Something like one hundred elements were claimed to have been identified as members of this series in the period up to 1913, when Moseley demonstrated spectroscopically that there should be exactly 15 elements in the series from lanthanum to lutetium.63 The addition of the 5f elements, the actinoids, to the periodic table is primarily due to the research and insights of Glenn T. Seaborg.85
Some of these elements were only identified well into the 20th Century CE. We commented earlier that Mendeleev had identified a place for an element of between calcium and erbium in his periodic table, with an atomic mass close to 45. As early as 1870, Mendeleev had started searching for this element which was expected to have a characteristic valency of +3 and an atomic mass of ∼44 that he named eka-boron. Mendeleev used the Sanskrit prefix eka (meaning “one”) to describe an unknown element related to a known member of the periodic table. If a second member of the group was identified it was given the prefix dvi-. Mandeleev never found his eka-boron, but scandium was eventually discovered after analysis of the minerals euxenite and gadolinite by Lars Fredrik Nilson who reported in 1879 “Über Scandium, ein neues Erdmetall”.86 From analysis of the minerals euxenite and gadolinite. The identity of scandium as Mendeleev's eka-boron was made by Per Teodor Cleve in the same year of 1879.87
As early as 1869, Mendeleev had identified a new element with atomic mass approximately 180 in the triad with titanium and zirconium as the higher members. Mendeleev does not appear to have made any significant effort in identifying this eka-zirconium, but others certainly did.88,89 The element 72 was discovered and undiscovered a number of times before the definitive identification of hafnium in the mineral zircon in 1923 by Dirk Coster and Georg von Hevesy.90 In the early years of the century, Georges Urbain had isolated an element claimed to be number 72 and which he named celtium. Eventually, this material was shown on the basis of spectroscopy not to contain element 72 and the claim was eventually, and after significant controversy, decided in favour of Coster and von Hevesy.
In the 1871 periodic table, Mendeleev has a position in his group VII for an element with an atomic mass of ∼100 and also for a heavier congener with no predicted mass. The heavier member is identified with element 75 isolated from platinum ores and the minerals columbite, gadolinite and molybdenite by Walter Noddack, Ida Noddack (publishing in her maiden name of Tacke) and Otto Bergand “Die Ekamangane” and named rhenium.91 The history of the element with atomic mass ∼100, and subsequently assigned atomic number 43, is currently undergoing some revision. The element was expected to lie between molybdenum and ruthenium in group VII. Many efforts were made to identify this missing element, which was actually a rather critical test of the peridoic theory of Mendeleev. Until recently, the credit for the discovery of element 43, named technetium, belonged to Carlo Perrier and Emilio Segrè, who isolated 95mTc and 97Tc from samples of a molybdenum foil that had been irradiated in a cyclotron.92 The name technetium (Greek, τεχνητος, artificial) was given on the basis that the element was artificially prepared and did not occur in nature.
In recent years, it has been convincingly demonstrated that element 43 had been identified. In their 1925 paper “Die Ekamangane”74 Noddack, Berg, and Tacke claimed not only element 75 but also element 43, which they called masurium. They prepared the new element by the irradiation of columbite with an electron beam, although the results could not be replicated by subsequent researchers and the claim for element 43 was dismissed. More recent analysis of the original data indicate that the early team did indeed obtain element 43 in 1925.93,94
The 3d, 4d and 5d series of the periodic table were now complete!
The 6d elements – or what's in a name?.
In our journey through the periodic table, we started with the ancient elements which occurred in metallic form and were known and utilized since te beginning of human civilization. We then moved to the era of classical chemistry in which new elements were identified in naturally occurring substances. With the exception of technetium, the 3d, 4d and 5d series were populated in this manner. When we come to the 6d elements, the story is rather different. These superheavy elements are purely artificial, generated by reactions of accelerated heavy nuclei with heavy element targets. Now, the elements are predicted upon the basis of the periodic table and their anticipated electronic structure and are primarily identified not on the basis of their chemistry, but rather on the nature and energetics of their radioactive decay.
It is most unlikely that any of us will be actively involved in investigating the chemistry of these 6d elements, which are mostly known only in quantities of a few tens of atoms or high energy physics events and which have half-lives significantly shorter (typically less a few seconds) than any chemical manipulation process. Nevertheless, these elements have had significant impact outside the chemical community because the naming of elements made the transition from the scientific to the political arena. In the cold-war and post-cold war era, the preparation of a new element and the possibility of naming it according national imperative became important. Claims from competing laboratories for the synthesis of new elements, often relying on observations of only one or two events, were to be evaluated by IUPAC, with this body also being tasked with the final decision for the recommended name of the element.
The IUPAC recommended names for the 6d elements (as of February 2019) are rutherfordium (Rf, 104), dubnium (Db, 105), seaborgium (Sg, 106), bohrium (Bh, 107), hassium (Hs, 108), meitnerium (Mt, 109), darmstadtium (Ds, 110), roentgenium (Rg, 111) and copernicum (Cn, 112). However, in some cases the journey to the final recommendation of the name travelled stormy waters and the controversies over the naming of elements resulted in a series of provisional publications from IUPAC explaining the process and assessing the evidence for priority of claims in the identification of new elements.95 The recent recommendations for the naming process establish that new elements may be names after “(a) mythical concepts or characters, (b) place, area or country, (c) a property of the element, and (d) a scientist”. The final agreement allowing the naming of an element after a scientist was a reversal of a 1994 recommendation that an element should not be named after a living person.
Element 104 was claimed both by researchers in Dubna (Russian Federation) and the Lawrence Radiation Laboratory (United States of America) who named it kurchatovium (Ku) and rutherfordium (Rf) respectively. Eventually IUPAC made the recommendation of rutherfordium for element 104 in 1997 (after having previously made a confusing provisional recommendation to name the element dubnium in 1994). Similarly, element 105 was claimed by Russian and American researchers who proposed naming it nielsbohrium (Ns) and hahnium (Ha) respectively, with IUPAC initially proposing joliotium and eventually deciding for dubnium (Db, which had previously been proposed and then rejected for element 104). Element 106 had an even more chequered history, with disputed claims and a proposal of the name seaborgium being hotly disputed as Glenn Seaborg was still alive at the time and IUPAC had (inconsistently) in 1994 decided that elements should not be named after living scientists. Eventually, and after a bitter debate, the name seaborgium (Sg) was recommended. The name nielsbohrium (rejected for element 105) was proposed for element 107, but IUPAC eventually decided on the shorter name bohrium (Bh), which reflects the wishes of the discoverers although yielding a linguistically (slightly) more friendly name. The name hassium (Hs) was adopted uncontroversially for element 108 (although IUPAC itself had originally proposed hahnium). Element 109 was, again without apparent controversy, named meitnerium (Mt) after Lise Meitner. However, the calm reception did not persist for long and element 110 was claimed by multiple groups with proposals of hahnium, becquerelium and darmstadtium for the name. IUPAC eventually decided in favour of darmstadtium (Ds) and at the same time recommended (without dispute) the naming of elements 111 and 112 as roentgenium (Rg) and copernicum (Cn) respectively.
And so the story of the d-block elements comes to an end (for the time being) with the establishment of copernicum as the latest member of group 12 and the last member of the 6d elements.
Conclusions
This article has traced the history of the d-block elements, with the emphasis being these metals in the context of the discovery and refinement of the periodic table. Nevertheless, the story has not been purely scientific, but one which has allowed us to look at some of the unexpected ways in which science, society and the human condition interact with one another. If anyone has learnt anything from this article, or had a “Eureka” moment in understanding or thinking about topics which have hitherto been taken for granted, then I have succeeded.
Conflicts of interest
The author has no conflicts to declare.
Acknowledgements
I would like to take the opportunity to acknowledge all of the gifted scientists and historians who have helped me in my journey through chemistry. I genuinely believe that our modern research can benefit from a reflection on what has gone before and how we have arrived at the “state-of-the-art”. Reading those forgotten works of chemical history, I can only reiterate the words of Howard Carter on first opening the tomb of Tutenkhamun: “Can you see anything?’ it was all I could do to get out the words, ‘Yes, wonderful things”.96 Those great chemists of the past, guided by smell, taste and colour achieved great things. It seems appropriate to end with the words of the last of the true alchemists, Isaac Newton “As Newton stated “If I have seen further it is by standing on the shoulders of Giants”.97
References
- The first official presentation of the periodic table was in a talk entitled “The Dependence Between the Properties of the Atomic Weights of the Elements” given to the Russian Chemical Society on the 18th March [old style, 6th March] 1869.
-
https://iupac.org/what-we-do/periodic-table-of-elements/
.
-
https://iupac.org/
.
-
D. C. Hoffman, A. Ghiorso and G. T. Seaborg, The Transuranium People: The Inside Story, Imperial College Press, London, 2000 Search PubMed.
- IUPAC, Pure Appl. Chem., 1997, 69, 2471 Search PubMed.
- A. F. Williams, Chimia, 2019 Search PubMed , in press.
-
E. C. Constable, DOI:10.26434/chemrxiv.7635905.v1.
- C. R. Bury, J. Am. Chem. Soc., 1921, 43, 1602 CrossRef CAS.
- W. B. Jensen, J. Chem. Educ., 2003, 80, 952 CrossRef CAS.
-
Metallurgy for the Non-metallurgist, ed. A. C. Reardon, ASM International, Ohio, 2nd edn, 2011, pp. 73–84 Search PubMed.
-
G. Boccaccio, The Decameron, (Ed. And Trans.) G. H. McWilliam, Penguin Books, London, 1972 Search PubMed.
-
G. Chaucer, The Canterbury Tales, Penguin Random House, London, 2005 Search PubMed.
-
Anonymous, The Poem of the Cid, A bilingual edition with parallel text, R. Hamilton and J. Perry (Trans.), Penguin Books, London, 1975 Search PubMed.
-
T. Malory, Le Morte D'Arthur, ed. J. Cowen, Penguin Books, London, 1969, Vols. 1 and 2 Search PubMed.
-
M. E. Weeks, The discovery of the elements, in J. Chem. Ed, Easton, 1956 Search PubMed.
-
A. P. T. B. von Hohenheim, The Hermetic and alchemical writings of Aureolus PhilippusTheophrastus Bombast, of Hohenheim, called Paracelsus the Great, (Ed and Trans.) A. E. Waite, J. Elliott and Co, London, 1894 Search PubMed.
-
A. Lavoisier, Elements of Chemistry in a New Systematic Order containing All the Modern Discoveries, R. Kerr (Trans.), W. Creech, Edinburgh, 1790 Search PubMed.
- G. Brandt, Acta Lit. Sci. Svec., 1735, 4, 1 Search PubMed.
- E. C. Constable and C. E. Housecroft, Chem. Soc. Rev., 2013, 42, 1429 RSC.
- Tassaert, Ann. Chim. Phys., 1798, 28, 92 Search PubMed.
-
D. McDonald and L. B. Hunt, A History Of Platinum And Its Allied Metals, Johnson Matthey, London, 1962 Search PubMed.
-
G. Cardano and De S. E. Nenci, Angeli, Milan, 2004.
-
J. C. Scaligeri, Exotericarum exercitationum liber XV. De Subtilitate ad Hieronymum Cardanum, Michael Vascosan, Paris, 1557 Search PubMed.
-
A. de Ulloa, Relación Histórica del Viage a la America Meridional, Antonio Marín, Madrid, 1748, vol. 1 and 2 Search PubMed.
-
S. Venetsky, Tales about metals, N.G. Kittell (Trans.), Mir Publishers, Moscow, 1978 Search PubMed.
- J. F. Cronstedt, K. Sven. Vetenskapsakad. Handl., 1751, 12, 287 Search PubMed.
- J. F. Cronstedt, K. Sven. Vetenskapsakad. Handl., 1754, 15, 38 Search PubMed.
-
J. R. Glauber, Teutschlands Wohlfarth, Jansson, Amsterdam, 1659 Search PubMed.
-
A. E. Nordenskiold, Scheeles nachgelassene Briefe und Aufzeichnungen, P. A. Norstedt & Soner, Stockholm, 1892, pp. 120–126 Search PubMed.
- C. W. Scheele, K. Sven. vetensk. akad. handl., 1778, 39, 247 Search PubMed.
- P. J. Hjelm, K. Sven. vetensk. akad. handl., 1790, 11, 50 Search PubMed.
- C. W. Scheele, K. Sven. vetensk. akad. handl., 1781, 2, 89 Search PubMed.
- T. Bergman, K. Sven. vetensk. akad. handl., 1781, 2, 95 Search PubMed.
- J. J. Elhuyar and F. Elhuyar, Extractos Real Soc. Bascongada, 1783, 46 Search PubMed.
- L. N. Vauquelin, Ann. Chim., 1798, 25, 21 Search PubMed.
- L. N. Vauquelin, Ann. Chim., 1798, 25, 194 Search PubMed.
- L. N. Vauquelin, Nicholson's J., 1798, 2, 145 Search PubMed.
- L. N. Vauquelin, Nicholson's J., 1798, 2, 387 Search PubMed.
- M. H. Klaproth, Ann. Chim. Phys., 1789, 1, 6 Search PubMed.
- W. Gregor, Crell's Ann., 1791, 15, 40 Search PubMed.
-
M. H. Klaproth, Analytical essays towards promoting the chemical knowledge of mineral substances, Cadell and Davies, London, 1801 Search PubMed.
- L. F. Nilson and S. O. Pettersson, Z. Phys. Chem., 1887, 1, 27 Search PubMed.
- B. Geijer, Crell's Ann., 1788, 9, 229 Search PubMed.
- J. Gadolin, K. Sven. vetensk. akad. handl., 1794, 15, 137 Search PubMed.
- A. G. Ekeberg, K. Sven. vetensk. akad. handl., 1797, 18, 156 Search PubMed.
- H. Rose, Ber. Akad. Wiss. Berlin, 1843, 143 Search PubMed.
-
R. Boyle, The Sceptical Chymist, Caldwell, London, 1661 Search PubMed.
-
J. H. White, The history of the phlogiston theory, E. Arnold and Co., London, 1932 Search PubMed.
- C. Hatchett, Philos. Trans. R. Soc. London, 1802, 92, 49 CrossRef.
- C. Hatchett, Ann. Phys., 1802, 11, 120 CrossRef.
- H. Rose, Ann. Phys., 1844, 139, 317 CrossRef.
-
M. Fontani, M. Costa and M. V. Orna, The lost elements, OUP, Oxford, 2015 Search PubMed.
- J. Dalton, Mem. Proc. - Manchester Lit. Philos. Soc., 1805, 1, 271 Search PubMed.
-
J. Dalton, A New System of Chemical Philosophy, Bickerstaff, London, 1808, Part 1, p. 212 Search PubMed.
-
T. Thomson, An attempt to establish the first principles of chemistry by experiment, 2 vols, Baldwin, Cradock, and Joy, London, 1825 Search PubMed.
- J. W. Döbereiner, Ann. Phys., 1829, 15, 301 CrossRef.
-
L. Gmelin, Handbuch der Chemie, Winter, Heidelberg, 4th edn, 1843, vol. 1, p. 52, p. 456 Search PubMed.
- J. P. Cooke, Jr., Am. J. Sci., 1854, 17, 387 Search PubMed.
- A. E. Beguyer De Chancourtois, C.R. Acad. Sci. Paris, 1862, 54, 757 Search PubMed.
- A. E. Beguyer De Chancourtois, C.R. Acad. Sci. Paris, 1862, 54, 840 Search PubMed.
- A. E. Beguyer De Chancourtois, C.R. Acad. Sci. Paris, 1862, 54, 967 Search PubMed.
- W. Odling, Q. J. Sci., 1864, 1, 642 Search PubMed.
- J. A. R. Newlands, Chem. News, 1865, 12, 83 Search PubMed.
- H. G. J. Moseley, Nature, 1913, 92, 554 CrossRef.
- H. G. J. Moseley, Philos. Mag. 6, 1913, 26, 1024 CrossRef.
- H. G. J. Moseley, Philos. Mag. 6, 1914, 27, 703 CrossRef CAS.
-
J. L. Meyer, Die modernen Theorien der Chemie, Maruschke & Berendt, Breslau, 1864 Search PubMed.
- K. Seubert, Z. Anorg. Allg. Chem., 1895, 9, 334 CrossRef CAS.
-
L. Meyer, D. I. Mendeleev and K. Seubert, Das natürliche System der chemischen Elemente, W. Engelmann, Leipzig, 1895 Search PubMed.
- D. I. Mendeleev, Zh. Russ. Fiz.-Khim. Obsch., 1869, 1, 60 CrossRef.
- D. Mendelejeff, Z. Chem., 1869, 12, 405 Search PubMed.
- D. Mendeleev, Liebigs Ann. Chem., 1871,(Supplementband 8), 133 Search PubMed.
- M. E. Weeks, J. Chem. Educ., 1932, 9, 2065 CrossRef.
-
E. R. Scerri, The Periodic Table. Its Story and Its Significance, Oxford University Press, Oxford, 2007 Search PubMed.
- B. Brauner, Z. Anorg. Chem., 1902, 33, 1 CrossRef.
- W. Ramsay, Sci. Am., 1904,(Suppl. 1508), 24162 CrossRef.
-
“A Meal Without Wine Is Like A Day Without Sunshine”, ed. J. A. Brillat-Savarin, The Physiology of Taste or Meditations on Transcendental Gastronomy, France, 1825 Search PubMed.
- A. Werner, Chem. Ber., 1905, 38, 914 CrossRef CAS.
- A. Werner, Chem. Ber., 1905, 38, 2022 CrossRef CAS.
- R. Abegg, Chem. Ber., 1905, 38, 1386 CrossRef CAS.
-
H. G. Deming, General chemistry, Chapman and Hall Ltd., London, 1923 Search PubMed.
- E. Fluck, Pure Appl. Chem., 1988, 60, 431 CAS.
-
G. J. Leigh, IUPAC Nomenclature of Inorganic Chemistry, Recommendations 1990, Blackwell Scientific Publications, Oxford, 1990 Search PubMed.
-
N. G. Connelly, T. Damhus, R. M. Hartshorn and A. T. Hutton, Nomenclature of Inorganic Chemistry. IUPAC Recommendations 2005, Royal Society of Chemistry, Cambridge, 2005 Search PubMed.
- G. T. Seaborg, J. Chem. Soc., Dalton Trans., 1996, 3899 RSC.
- L. F. Nilson, Chem. Ber., 1879, 12, 554 CrossRef.
- P. T. Cleve, C.R. Acad. Sci. Paris, 1879, 89, 419 Search PubMed.
- G. Hevesy, Chem. Rev., 1925, 2, 1 CrossRef CAS.
- V. P. Mel'nikov, Centaurus, 1982, 26, 317 CrossRef.
- D. Coster and G. Hevesy, Nature, 1923, 111, 79 CrossRef CAS.
- W. Noddack, I. Tacke and O. Berg, Naturwissenschaften, 1925, 13, 567 CrossRef CAS.
- C. Perrier and E. Segré, J. Chem. Phys., 1937, 5, 712 CrossRef CAS.
- P. Van Assche, Nucl. Phys., 1988, A480, 205 CrossRef CAS.
-
W. Noddack, I. Tacke and O. Berg, The Ekamanganese elements, (BLG–603) (Trans. P. van Assche), Belgium, 1988; https://inis.iaea.org/search/search.aspx?orig_q=RN:20009502 Search PubMed.
- An excellent overview and entry to the primary literature is given in: S. Hofmann, S. N. Dmitriev, C. Fahlander, J. M. Gates, J. B. Roberto and H. Sakai, Pure Appl. Chem., 2018, 90, 1773 CAS.
-
H. Carter, The Tomb of Tutankhamun, National Geographical Society, Washington DC, 2003 Search PubMed.
-
I. Newton
, in letter to Robert Hooke, 1676 reproduced in Turnbull, H.W. ed., 1959. The Correspondence of Isaac Newton: 1661–1675, Volume 1, London, UK: Published for the Royal Society at the University Press. p. 416.
|
This journal is © The Royal Society of Chemistry 2019 |
Click here to see how this site uses Cookies. View our privacy policy here.