DOI:
10.1039/C6QM00240D
(Chemistry Frontiers)
Mater. Chem. Front., 2017,
1, 208-211
Nanoarchitectonics: a navigator from materials to life
Received
29th September 2016
, Accepted 23rd October 2016
First published on 7th November 2016
Abstract
Nanoarchitectonics is a new concept for the fabrication of functional material systems through harmonization of various actions including atomic/molecular-level manipulation, chemical reactions, self-assembly and self-organization and their modulation by external fields/stimuli. This working principle and target-scales are exactly the same as those for biological systems where every aspect of living systems is heavily dependent on physicochemical occurrences at the nano–micro scale length. Therefore, nanoarchitectonics can work as a navigator to convert conventional materials into life-like high-level functional systems. It would be a key concept in the breakthrough of materials innovation in the near future.
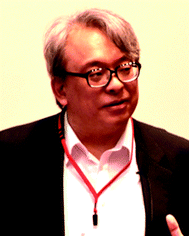
Katsuhiko Ariga
| Katsuhiko Ariga received his PhD degree from the Tokyo Institute of Technology. He is currently the Director of the Supermolecules Group, and the Principal Investigator of the World Premier International (WPI) Research Centre for Materials Nanoarchitectonics (MANA), the National Institute for Materials Science (NIMS). His research is oriented to supramolecular chemistry, surface science, and functional nanomaterials (Langmuir–Blodgett film, layer-by-layer assembly, self-organized materials, sensing and drug delivery, molecular recognition, mesoporous material, etc.) and he is now trying to combine them into a unified field. |
Technology to architectonics
As Pasteur demonstrated in the so-called swan-neck flask experiment, living creatures cannot spontaneously emerge from non-living matters. So, we cannot create living systems from life-less materials, although living creatures can be regarded as the ultimate functional systems due to their incredibly high specificity and efficiency even under ambient mild conditions. One of our greatest endeavours in science is to create functional materials with bio-like high functions from non-living components. How can we complete this impossible mission?
Due to the rapid progress in science, we currently know that activities of bio-systems are fundamentally based on combinations of nano-sized chemical phenomena such as molecular recognitions, energy transfer, and self-assembly. Therefore, efforts to control nano-sized systems and their assemblies would open up ways to our dreams. Our strongest tool for the regulation of nano-worlds is believed to be nanotechnology, where various techniques to fabricate nano-sized structures are included. Although they enable us to control functions with nano-components, most of them work as separate actions. This feature is much different from those observed in bio-systems where various types of processes are working together in fantastic harmonization. Therefore, more advanced concepts exceeding individual technologies is required for approaches towards living-creature-like highly functional systems.
It could be architectonics rather than individual techniques or technology. Masakazu Aono proposed a key term, nanoarchitectonics, at the 1st International Symposium on Nanoarchitectonics Using Suprainteractions in 2000.1 Unlike conventional nanofabrication strategies, material fabrication with nanoarchitectonics is accomplished with concerted harmony with various actions including atomic/molecular-level manipulation, chemical reactions, self-assembly and self-organization and their modulation by external fields/stimuli.2 This concept is now introduced to various research fields from basic materials production/organization3 to advanced applications4 including bio-related fields.5
Harmony at the nanoscale
Another important difference between nanotechnology and nanoarchitectonics would be a way of thinking for precision. Pursuing structural precision is an important task in nanotechnology. Scientists in nanotechnology usually try to achieve higher and higher structural precision. Better nanotechnology means technology with higher structural precision. This way of thinking on precision is effective for rigid and stable materials such as inorganic nanomaterials in static states and less disturbed conditions, such as ultrahigh vacuum and low temperatures. Therefore, most of the advanced nanotechnology examples such as atom manipulations are achieved for less-dynamic objects under well-controlled conditions.
In sharp contrast, nanoarchitectonics does not try to accomplish too precise structural control and strict regulation of phenomena. This way of thinking on precision is especially suited to nano-scale systems with certain dynamisms.6 When systems of materials include features of dynamism, the difference between micro-scale and nanoscale phenomena becomes critical. In macroscopic and microscopic scales, structural fabrications can be basically carried out according to their blueprint. Even when dynamic motions are included, simple Newtonian mechanics can mostly explain their behaviours in microscopic scale. Therefore, the functions and properties of materials are basically expectable with high precision.
However, down-sizing of systems with certain dynamic natures to nanoscale regions could result in various unpredictable features. In a nanoscale regime, uncontrollable disturbances and fluctuations such as thermal/statistical fluctuations have significant effects. Mutual interactions between nano-components often make the situation much more unpredictable. One input signal may cause perturbations to surrounding moieties, resulting in additional mutual interactions. The relationship between input and output is far from a one to one scheme.
In dynamic nanoscale systems, harmony would be a crucial factor. Instead of connecting individual interactions and phenomena precisely in series, harmonizing various issues together leading to soft, flexible, adjustable, adaptable and compatible functions is a crucial process in dynamic nanoscale worlds. Nanoarchitectonics is more appropriate for these tasks than nanotechnology.
Sharing features with life
The best harmony of functional materials is probably achieved in biological systems where huge numbers of components are working together under unavoidable and substantial thermal fluctuations. One day, I discussed this matter with a young scientist in Singapore (Dr Dalton Tay at the Nanyang Technological University). He kindly provided me with an interesting chart that depicts the relationship between the size and the complexity level in biological systems (Fig. 1, as a modified chart). He said that every aspect of living systems is heavily dependent on the organization, biochemical composition and physicochemical occurrences at the nano–micro scale length. In particular, a border line between non-living matters and living entities exists at scale regimes from nanometers to submicrometers. Harmonized assemblies based on interactive events including molecular recognition and self-assembly at these scale regions convert functional materials into living creatures. The working principle and target-scales are exactly the same as those in nanoarchitectonics. Nanoarchitectonics may be capable of creating living creatures from component materials. Our dreams may come true with nanoarchitectonics.
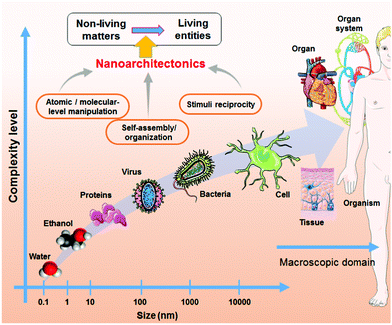 |
| Fig. 1 Relationship between the size and the complexity level in biological systems. Nanoarchitectonics may work between non-living matters and living entities. The original chart was given by Dr Tay and modified. Illustrations adapted under the terms of the CC-BY-3.0 license (http://creativecommons.org/licenses/by/3.0/). Copyright 2016, Servier. | |
From an atom-level switch to brain-like computing
It is important to extend this concept to the design and fabrication of general functional materials, systems, and devices. One example is shown in Fig. 2, where all-inorganic atomic-level switches can work as artificial synapses, and furthermore, huge networking of the atomic switch units creates brain-like computing systems through harmonizing ion/atom motions (artificial synapses) and electrical outputs (brain-like computing). Harmony in these examples appears as assembled chemical and physical actions in a self-regulated balance.
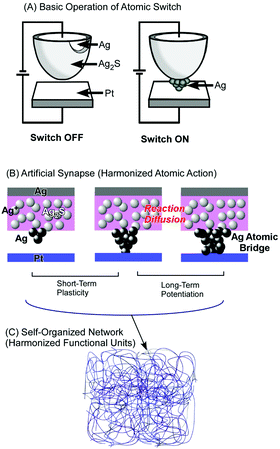 |
| Fig. 2 Examples of bio-like functions upon the nanoarchitectonics approach: (A) simple atomic switches exhibit (B) artificial synapse functions and form (C) a neuron-network-like harmonized information process device. | |
An atomic switch utilizes chemical processes in the nanoscale.7 Two electrodes (Ag2S and Pt) are set with ca. 1 nm gap, and application of a negative bias to the Pt electrode induces reduction of Ag cations to Ag at the Ag2S electrodes through a tunnel current. The formation of clusters of several Ag atoms turns this switch on by establishing a conduction path. In turn, application of positive bias oxidizes Ag atoms to an Ag ion, resulting in the switch-off of the system. This switching system is based on various chemical and physical processes such as redox reaction and atom diffusion while electrical switches in our house are based simply on the physical contact.
A switching mechanism upon the atomic reaction and diffusion accomplishes bio-like functions as artificial synapse behaviours to realize short-term plasticity and long term potentiation through modulating frequencies of input pulses.8 Applications of low-frequency pulses with an interval of 20 s can form one quantized conductive channel only upon each pulse input, and cannot maintain the high conductance state for a long time. It is regarded as short-term plasticity. In contrast, applications of high frequency pulses with 2 s intervals can stabilise long-lived higher-conductance, corresponding to long-term potentiation. These switching processes are not based on simple physical contact and result from harmonized processes of atomic reaction and diffusion. We can memorize things through repeated learning in a high frequency. This human behaviour can be mimicked only at nano-level inorganic architectures.
Because atomic switch device structures can be prepared using various metallic and insulator components, atomic switch functions can be architected into a nanowire network through self-organization of nanowires with post-processes.9 The atomic switch network prepared on an integrated electrode board has a huge amount of junctions with highly non-uniform nanowire lengths. Their switching behaviours are harmonized to result in nonlinear outputs. Filament formation and dissolution can be dynamically altered through re-current connectivity changes. The entire system is adaptable to respond and complete target tasks. The prepared neural network mimics would be capable of brain-like natural computing in the future. This approach based on harmonization of numerous functional elements may overcome scaling limits in the current CMOS technology.
Future
The above mentioned systems are exemplified because they nicely couple man-made device technology and wise biofunctions. However, possibilities of the nanoarchitectonics approach are not limited to these brain-like computing systems. A wide range of usages of the nanoarchitectonics concept were already proposed from nanoscopic machineries10 to large-scale environmental systems.11 Life-like self-responsive, self-thinking and self-powered functional systems can be potentially fabricated with nanoarchitectonics. Actually, self-regulated ON/OFF-type material release systems were invented by architecting hierarchic pore-layer structures from a nano to submicron-level.12 Self-powered systems based on nanoarchitectonics can automatically convert our daily motions to an energy source for various functions.13 Harmonization of these features on the basis of the nanoarchitectonics concept would convert conventional materials to bio-like systems.14
Nanoarchitectonics can work as a navigator to convert artificial materials (even metals and semiconductors) into life-like high-level functional systems. By embracing the harmonization concepts underlying nanoarchitectonics, we are able to open a new chapter in nanomaterials science. It would be a key concept in the breakthrough of materials innovation in the near future.
Acknowledgements
I much appreciate Dr Dalton Tay for stimulative discussion. This work was partly supported by JSPS KAKENHI Grant Number JP16H06518 (Coordination Asymmetry).
Notes and references
- K. Ariga, Q. Ji, J. P. Hill, Y. Bando and M. Aono, NPG Asia Mater., 2012, 4, e17 CrossRef.
-
(a) K. Ariga, M. Li, G. J. Richards and J. P. Hill, J. Nanosci. Nanotechnol., 2011, 11, 1 CrossRef CAS PubMed;
(b) K. Ariga, Q. Ji, W. Nakanishi, J. P. Hill and M. Aono, Mater. Horiz., 2015, 2, 406 RSC;
(c) M. Aono and K. Ariga, Adv. Mater., 2016, 28, 989 CrossRef CAS PubMed;
(d) K. Ariga and M. Aono, Jpn. J. Appl. Phys., 2016, 55, 1102A6 CrossRef.
-
(a) K. Ariga, A. Vinu, Y. Yamauchi, Q. Ji and J. P. Hill, Bull. Chem. Soc. Jpn., 2012, 85, 1 CrossRef CAS;
(b) K. Ariga, Y. Yamauchi, G. Rydzek, Q. Ji, Y. Yonamine, K. C.-W. Wu and J. P. Hill, Chem. Lett., 2014, 43, 36 CrossRef CAS;
(c) V. Malgras, Q. Ji, Y. Kamachi, T. Mori, F.-K. Shieh, K. C. W. Wu, K. Ariga and Y. Yamauchi, Bull. Chem. Soc. Jpn., 2015, 88, 1171 CrossRef CAS;
(d) T. Govindaraju and M. B. Avinash, Nanoscale, 2012, 4, 6102 RSC;
(e) G. Rydzek, Q. Ji, M. Li, P. Schaaf, J. P. Hill, F. Boulmedais and K. Ariga, Nano Today, 2015, 10, 138 CrossRef CAS.
-
(a) H. Abe, J. Liu and K. Ariga, Mater. Today, 2016, 19, 12 CrossRef CAS;
(b) K. Ariga, K. Minami and L. K. Shrestha, Analyst, 2016, 141, 2629 RSC.
-
(a) W. Nakanishi, K. Minami, L. K. Shrestha, Q. Ji, J. P. Hill and K. Ariga, Nano Today, 2014, 9, 378 CrossRef CAS;
(b) K. Ariga, K. Kawakami, M. Ebara, Y. Kotsuchibashi, Q. Ji and J. P. Hill, New J. Chem., 2014, 38, 5149 RSC;
(c) K. Ariga, K. Minami, M. Ebara and J. Nakanishi, Polym. J., 2016, 48, 371 CrossRef CAS;
(d) S. Howorka, Langmuir, 2013, 29, 7344 CrossRef CAS PubMed;
(e) K. Ariga, Q. Ji, M. J. McShane, Y. M. Lvov, A. Vinu and J. P. Hill, Chem. Mater., 2012, 24, 728 CrossRef CAS.
- K. Ariga, J. Li, J. Fei, Q. Ji and J. P. Hill, Adv. Mater., 2016, 28, 1251 CrossRef CAS PubMed.
- T. Hasegawa, K. Terabe, T. Tsuruoka and M. Aono, Adv. Mater., 2012, 24, 252 CrossRef CAS PubMed.
- T. Ohno, T. Hasegawa, T. Tsuruoka, K. Terabe, J. K. Gimzewski and M. Aono, Nat. Mater., 2011, 10, 591 CrossRef CAS PubMed.
- E. C. Demis, R. Aguilera, H. O. Sillin, K. Scharnhorst, E. J. Sandouk, M. Aono, A. Z. Stieg and J. K. Gimzewski, Nanotechnology, 2015, 26, 204003 CrossRef CAS PubMed.
- Y. Shirai, K. Minami, W. Nakanishi, Y. Yonamine, C. Joachim and K. Ariga, Jpn. J. Appl. Phys., 2016, 55, 1102A2 CrossRef.
- K. Ariga, S. Ishihara, H. Abe, M. Lia and J. P. Hill, J. Mater. Chem., 2012, 22, 2369 RSC.
-
(a) Q. Ji, M. Miyahara, J. P. Hill, S. Acharya, A. Vinu, S. B. Yoon, J.-S. Yu, K. Sakamoto and K. Ariga, J. Am. Chem. Soc., 2008, 130, 2376 CrossRef CAS PubMed;
(b) Q. Ji, S. Acharya, J. P. Hill, A. Vinu, S. B. Yoon, J.-S. Yu, K. Sakamoto and K. Ariga, Adv. Funct. Mater., 2009, 19, 1792 CrossRef CAS.
- F. R. Fan, W. Tang and Z. L. Wang, Adv. Mater., 2016, 28, 4283 CrossRef CAS PubMed.
-
(a) M. B. Avinasha and T. Govindaraju, Nanoscale, 2014, 6, 13348 RSC;
(b) M. B. Avinash and T. Govindaraju, J. Phys. Chem. Lett., 2013, 4, 583 CrossRef CAS PubMed;
(c) M. B. Avinash, K. V. Sandeepa and T. Govindaraju, ACS Omega, 2016, 1, 378 CrossRef CAS.
|
This journal is © the Partner Organisations 2017 |
Click here to see how this site uses Cookies. View our privacy policy here.