DOI:
10.1039/C6RA11218H
(Paper)
RSC Adv., 2016,
6, 75133-75137
A novel self-terminated Prins strategy for the synthesis of tetrahydropyran-4-one derivatives and their behaviour in Fisher indole synthesis†
Received
30th April 2016
, Accepted 31st July 2016
First published on 4th August 2016
Abstract
A novel self-terminated Prins strategy has been developed for the synthesis of 2-substituted tetrahydropyran-4-one derivatives through a condensation of 3-(phenylthio)but-3-en-1-ol with carbonyl compounds in the presence of 5 mol% of Sc(OTf)3 under mild conditions. A few products were subsequently transformed into the corresponding indoles by Fisher-indole synthesis.
Introduction
Tetrahydropyrans of varied substitution pattern are embodied as an integral part of numerous biologically active natural products (Fig. 1).1
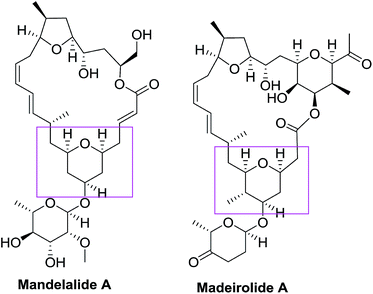 |
| Fig. 1 Examples of tetrahydropyranol containing natural products. | |
Generally, the tetrahydropyrans are prepared from aldol-type cyclizations,2 hetero-Diels–Alder reactions,3 Japp–Maitland reactions,4 oxa-Michael reactions,5 and Petasis–Ferrier rearrangements.6 By employing these strategies, a wide range of tetrahydropyran-4-one substructures have been synthesized. The direct approach for the synthesis of tetrahydropyran-4-ones involves the cyclization of enol ethers and enol acetates with in situ generated oxocarbenium ions.7–9 In addition, Prins cyclization has successfully been employed for the synthesis of tetrahydropyranols, which could subsequently be oxidized into the corresponding tetrahydropyranones.10 However, there have been no reports on the direct synthesis of tetrahydropyranones by the condensation of thiol functionalized homoallylic alcohol with aldehydes.
Results and discussions
Following our interest on Prins type cyclizations,11 we herein report an efficient strategy for the direct synthesis of 2-substituted tetrahydropyran-4-ones by a self termination of Prins cyclization. The requisite precursor, 3-(phenylthio)but-3-en-1-ol (1) was prepared by treating homopropargyl alcohol with thiophenol in the presence of Pd(OAc)2 in THF (Scheme 1).12
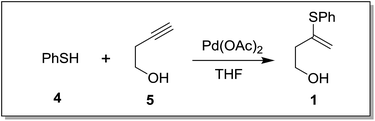 |
| Scheme 1 Preparation of starting materials. | |
In a preliminary experiment, 3-(phenylthio)but-3-en-1-ol (1) was treated with benzaldehyde (2) in the presence of 5 mol% Sc(OTf)3 in dichloromethane. Interestingly, the reaction proceeded well at room temperature and the corresponding 2-phenyl tetrahydropyran-4-one 3a was obtained in 87% yield (Scheme 2).
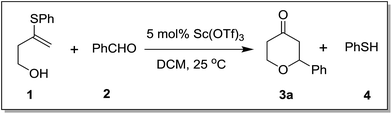 |
| Scheme 2 Synthesis of 2-phenyltetrahydropyran-4-one. | |
The efficiency of other Lewis acids such as InCl3, InBr3, Yb(OTf)3, TMSOTf and BF3·OEt2 was investigated and the results are presented in Table 1.
Table 1 Optimization of the reaction conditions
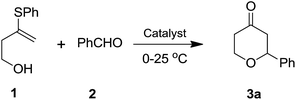
|
Entry |
Catalysta |
mol% |
Solvent |
Time (h) |
Yieldb (%) |
Reactions were performed in 1 mmol scale. Yield refers to pure product after chromatography. |
a |
Sc(OTf)3 |
5 |
DCM |
6 |
87 |
b |
Sc(OTf)3 |
5 |
THF |
7 |
30 |
c |
Sc(OTf)3 |
5 |
CH3CN |
7 |
20 |
d |
InCl3 |
10 |
DCM |
8 |
25 |
e |
InBr3 |
5 |
DCM |
6 |
40 |
f |
Yb(OTf)3 |
10 |
DCM |
6 |
30 |
g |
TMSOTf |
5 |
DCM |
7 |
35 |
h |
BF3·OEt2 |
5 |
DCM |
8 |
25 |
Among them, 5 mol% Sc(OTf)3 was found to be the most effective in terms of conversion.
To our surprise, the most frequently used Lewis acids such as TMSOTf and BF3·OEt2 were also found to be less effective for this conversion. To know the effect of solvent, the reaction was performed in different solvents such as THF, acetonitrile and DCM. Of these, dichloromethane gave the best results.
These initial findings encouraged us to extend this process to other carbonyl compounds and the results are presented in Table 2. Interestingly, several aromatic aldehydes underwent a smooth Prins cyclization to furnish the respective substituted 2-tetrahydropyran-4-ones in good yields (entries a–g, i–k, o and p Table 2). Notably, the reaction was successful even with acid sensitive substrates like cinnamaldehyde and furfural (entries h & p, Table 2). In addition, the reaction also proceeded well with 4-hydroxybenzaldehyde without the protection of hydroxyl group (entry o, Table 2). The scope of this method was further illustrated with respect to aliphatic aldehydes such as n-valeraldehyde, isovaleraldehyde and n-hexanal (entries l–n, Table 2). In case of aliphatic aldehydes, the corresponding products were obtained relatively in lower yields than aromatic counter parts. In all the cases, the products were obtained in good to excellent yields. Finally, we examined the reactivity of ketones for example, cyclohexanone. To our delight, the corresponding spirotetrahydropyran-4-one was obtained in good yield (entry q, Table 2). However, other ketones such as 3-pentanone and acetophenone failed to give the desired product under the present reaction conditions.
Table 2 Synthesis of 2-substituted tetrahydropyran-2-one derivatives
Entry |
Aldehyde |
Producta (3) |
Time (h) |
Yieldb (%) |
All products were characterized by NMR, IR and mass spectrometry. Yield refers to pure products after chromatography. |
a |
 |
 |
7 |
87 |
b |
 |
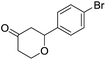 |
6 |
85 |
c |
 |
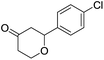 |
6 |
80 |
d |
 |
 |
7 |
78 |
e |
 |
 |
7 |
80 |
f |
 |
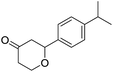 |
7 |
86 |
g |
 |
 |
8 |
82 |
h |
 |
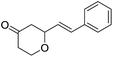 |
7 |
72 |
i |
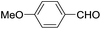 |
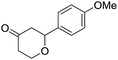 |
8 |
70 |
j |
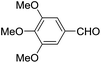 |
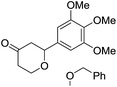 |
8 |
67 |
k |
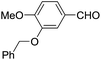 |
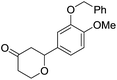 |
8 |
76 |
l |
 |
 |
7 |
72 |
m |
 |
 |
7 |
75 |
n |
 |
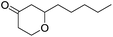 |
7 |
65 |
o |
 |
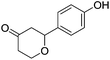 |
8 |
58 |
p |
 |
 |
6 |
75 |
q |
 |
 |
7 |
70 |
Based on our previous observations,11 we proposed a plausible reaction mechanism in Scheme 3. In the beginning, the aldehyde reacts with 3-(phenylthio)but-3-en-1-ol likely after activation with Sc(OTf)3 to generate the oxocarbenium ion (A). A subsequent attack of the internal alkene followed by a neighbouring group participation of sulfide would generate the thia-carbenium ion (B). Up on hydrolysis of B would give the desired 2-substituted tetrahydropyran-4-one (3) along with thiophenol as a by-product.
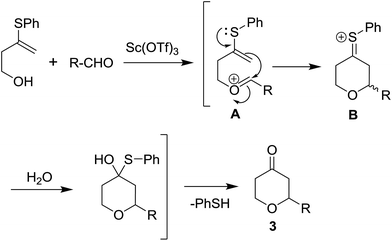 |
| Scheme 3 A plausible reaction pathway. | |
Next we were interested to demonstrate the use of tetrahydropyranones for indole synthesis. Indole derivatives are abundantly found in biologically active compounds and pharmaceuticals.13 For example, the core structure of type I is found in anti-inflammatory drug, etodolac.14 Indole type II was found to inhibit the NS5A polymerase.15 Furthermore, oxazinoindole type III exhibits potent antidepressant and antitumor properties (Fig. 2).16
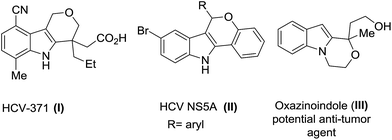 |
| Fig. 2 Biologically active indole derivatives. | |
Consequently, a few tetrahydropyran-4-ones were successfully converted into indole derivatives by means of Fischer indole synthesis (Table 3). Accordingly, treatment of 3 with phenylhydrazine (6) in the presence of acetic acid under reflux conditions afforded the indole derivative (7) as a major and dihydropyrazol (8) as a minor product (Table 3).
Table 3 Fischer indole synthesis
The structure of 8c was confirmed by X-ray crystallography (Fig. 3).17
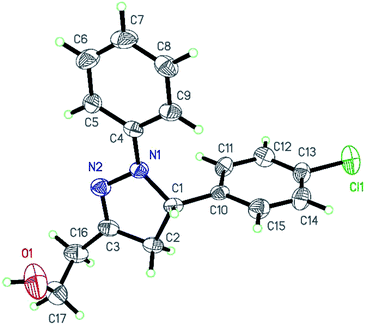 |
| Fig. 3 ORTEP diagram of 8c. | |
Furthermore, compounds 3n and 3q were subjected to Fisher-indole reaction conditions, but the expected products were not obtained under the present reaction conditions. After the formation of hydrozone, the decomposition of the reaction mixture was observed.
Conclusion
In summary, we have developed a novel strategy for the direct synthesis of 2-substituted tetrahydropyran-4-one derivatives using a catalytic amount of Sc(OTf)3 through a self termination of Prins cyclization. The synthetic utility of these tetrahydropyran-4-ones is exemplified by means of Fischer indole synthesis. This method is operationally simple and works with a diverse range of carbonyl compounds with broad substitution patterns.
Experimental
Typical procedure
To a mixture of 3-(phenylthio)but-3-en-1-ol (0.5 mmol) and aldehyde/ketone (0.6 mmol) in anhydrous DCM (5 mL) was added Sc(OTf)3 (5 mol%) at 0 °C. The resulting mixture was allowed to stir at room temperature for the specified time. After completion, the reaction mixture was quenched with water and the organic layer was separated and the aqueous layer was extracted with dichloromethane (2 × 5 mL). The combined organic phases were washed with brine, dried over anhydrous Na2SO4 and concentrated in vacuo. The resulting crude mixture was purified by silica gel column chromatography (100–200 mesh) using ethyl acetate/hexane gradient mixture to afford the pure product 3.
Acknowledgements
S. Rehana Anjum thanks CSIR-SRF, New Delhi, India for the award of a senior research fellowship.
Notes and references
-
(a) A. B. Smith, R. J. Fox and T. M. Razler, Acc. Chem. Res., 2008, 41, 675 CrossRef CAS PubMed;
(b) P. A. Wender, B. A. Loy and A. J. Schrier, Isr. J. Chem., 2011, 51, 453 CrossRef CAS PubMed;
(c) K. S. Yeung and I. Paterson, Chem. Rev., 2005, 105, 4237 CrossRef CAS PubMed;
(d) K. Watanabe, J. Li, N. Veeraswamy, A. Ghosh and R. G. Carter, Org. Lett., 2016, 18, 1744 CrossRef CAS PubMed.
- S. Das, L. S. Li and S. C. Sinha, Org. Lett., 2004, 6, 123 CrossRef CAS PubMed.
-
(a) M. Johannsen and K. A. Jorgensen, J. Org. Chem., 1995, 60, 5757 CrossRef CAS;
(b) S. E. Schaus, J. Branalt and E. N. Jacobsen, J. Org. Chem., 1998, 63, 403 CrossRef CAS;
(c) A. G. Dossetter, T. F. Jamison and E. N. Jacobsen, Angew. Chem., Int. Ed., 1999, 38, 2398 CrossRef CAS;
(d) C. F. Thompson, T. F. Jamison and E. N. Jacobsen, J. Am. Chem. Soc., 2001, 123, 9974 CrossRef CAS PubMed;
(e) M. Anada, T. Washio, N. Shimada, S. Kitagaki, M. Nakajima, M. Shiro and S. Hashimoto, Angew. Chem., Int. Ed., 2004, 43, 2664 CrossRef.
-
(a) F. R. Japp and W. Maitland, J. Chem. Soc., 1904, 85, 1473 RSC;
(b) P. A. Clarke and W. H. C. Martin, Org. Lett., 2002, 4, 4527 CrossRef CAS PubMed;
(c) P. A. Clarke and W. H. C. Martin, Tetrahedron, 2005, 61, 5433 CrossRef CAS.
-
(a) C. F. Nising and S. Brase, Chem. Soc. Rev., 2008, 37, 1218 RSC;
(b) H. Kim and J. Hong, Org. Lett., 2010, 12, 2880 CrossRef CAS PubMed;
(c) S. Chandrasekhar, B. Roy, S. Pradhan and T. K. Ghosh, J. Org. Chem., 2012, 77, 9840 CrossRef PubMed;
(d) H. Yao, J. Ren and R. Tong, Chem. Commun., 2013, 49, 193 RSC.
-
(a) N. A. Petasis and S. P. Lu, Tetrahedron Lett., 1996, 37, 141 CrossRef CAS;
(b) A. B. Smith, P. R. Verhoest, K. P. Minbiole and J. J. Lim, Org. Lett., 1999, 1, 909 CrossRef CAS PubMed;
(c) A. B. Smith, K. P. Minbiole, P. R. Verkoest and T. J. Beauchamp, Org. Lett., 1999, 1, 913 CrossRef CAS PubMed.
-
(a) J. E. Dalgard and S. D. Rychnovsky, J. Am. Chem. Soc., 2004, 126, 15662 CrossRef CAS PubMed;
(b) J. E. Dalgard and S. D. Rychnovsky, Org. Lett., 2005, 7, 1589 CrossRef CAS PubMed.
-
(a) G. S. Cockerill, P. Kocienski and R. J. Treadgold, J. Chem. Soc., Perkin Trans. 1, 1985, 2093 RSC;
(b) W. J. Morris, D. W. Custar and K. A. Scheidt, Org. Lett., 2005, 7, 1113 CrossRef CAS PubMed;
(c) W. Tu and P. E. Floreancig, Angew. Chem., Int. Ed., 2009, 48, 4567 CrossRef CAS PubMed;
(d) C. Sun, Y. Zhang, P. Xiao, H. Li, X. Sun and Z. Song, Org. Lett., 2014, 16, 984 CrossRef CAS PubMed.
-
(a) L. Liu and P. E. Floreancig, Org. Lett., 2010, 12, 4686 CrossRef CAS PubMed;
(b) H. H. Jung and P. E. Floreancig, Tetrahedron, 2009, 65, 10830 CrossRef CAS PubMed;
(c) G. R. Peh and P. E. Floreancig, Org. Lett., 2015, 17, 3750 CrossRef CAS PubMed.
-
(a) C. Olier, M. Kaafarani, S. S. Gastaldi and M. P. Bertrand, Tetrahedron, 2010, 66, 413 CrossRef CAS;
(b) M. Pastor and I. M. Yus, Curr. Org. Chem., 2012, 16, 1277 CrossRef;
(c) X. Han, G. R. Peh and P. E. Floreancig, Eur. J. Org. Chem., 2013, 1193 CrossRef CAS;
(d) K. Zheng, X. Liu, S. Qin, M. Xie, L. Lin, C. Hu and X. Feng, J. Am. Chem. Soc., 2012, 134, 17564 CrossRef CAS PubMed.
-
(a) B. V. S. Reddy, S. Gopal Reddy, M. Durgaprasad, M. P. Bhadra and B. Sridhar, Org. Biomol. Chem., 2015, 13, 8729 RSC;
(b) B. V. S. Reddy, V. Swathi, S. Manisha, M. PalBhadra, B. Sridhar, D. Satyanarayana and B. Jagadeesh, Org. Lett., 2014, 16, 6267 CrossRef PubMed;
(c) B. V. S. Reddy, M. Durgaprasad and B. Sridhar, J. Org. Chem., 2015, 80, 653 CrossRef PubMed.
-
(a) H. Kuniyasu, A. Ogawa, K. Sato, I. Ryu, N. Kambe and N. Sonoda, J. Am. Chem. Soc., 1992, 114, 5902 CrossRef CAS;
(b) V. P. Aninkov, S. S. Zalesskiy, N. P. Orlov and I. P. Beletskaya, Russ. Chem. Bull., 2006, 55, 2109 CrossRef;
(c) Y. Ichinose, K. Wakamatsu, K. Nozaki, J. L. Birbaum, K. Oshima and K. Utimoto, Chem. Lett., 1987, 1647 CrossRef CAS.
-
(a) M. Lounasmaa and A. Tolvanen, Nat. Prod. Rep., 2000, 17, 175 RSC;
(b) M. Somei and F. Yamada, Nat. Prod. Rep., 2005, 22, 73 RSC;
(c) S. Cacchi and G. Fabrizi, Chem. Rev., 2005, 105, 2873 CrossRef CAS PubMed;
(d) G. R. Humphrey and J. T. Kuethe, Chem. Rev., 2006, 106, 2875 CrossRef CAS PubMed.
-
(a) S. Akai, H. Nemoto and M. Egi, Heterocycles, 2008, 76, 1537 CrossRef CAS;
(b) S. Vyas, P. Trivedi and S. C. Chaturvedi, Acta Pol. Pharm., 2009, 66, 201 CAS.
- C. A. Coburn, B. J. Lavey, M. P. Dwyer, J. A. Kozlowski and S. B. Rosenblum, WO2012050848A1, 2012.
- C. A. Demerson, G. Santroch, L. G. Humber and M. P. Charest, J. Med. Chem., 1975, 18, 577 CrossRef CAS PubMed.
- CCDC 1440304 contains supplementary crystallographic data for the structure 8c.
Footnote |
† Electronic supplementary information (ESI) available: Copies of 1H and 13C NMR spectra of products. CCDC 1440304. For ESI and crystallographic data in CIF or other electronic format see DOI: 10.1039/c6ra11218h |
|
This journal is © The Royal Society of Chemistry 2016 |