DOI:
10.1039/C5QO00372E
(Research Article)
Org. Chem. Front., 2016,
3, 335-338
N-Heterocyclic carbene catalyzed dehydrogenative coupling of enals: synthesis of monobactams†
Received
14th November 2015
, Accepted 5th January 2016
First published on 7th January 2016
Abstract
The first NHC-catalyzed dehydrogenative coupling of enals is described. With a suitable combination of NHC precatalyst, base, and solvent, the reaction proceeded smoothly to yield a wide range of monobactams. These substituted monobactams have been synthesized for the first time, and they are versatile synthetic intermediates toward other useful complex molecules.
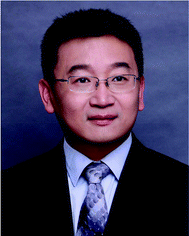 Jian Wang | Prof. Jian Wang received his PhD degree in synthetic chemistry from the University of New Mexico in 2007 under the supervision of Professor Wei Wang. He then moved to The Scripps Research Institute for his postdoctoral study with Professor Peter G. Schultz. In 2009, he began his independent academic career at the Department of Chemistry at National University of Singapore (NUS). In early 2013, Dr Wang moved to Tsinghua University and joined the faculty of the School of Medicine and the School of Pharmaceutical Sciences. His research program focuses on synthetic methodology (NHC catalysis) and drug discovery. |
Introduction
In nature, nucleotide transhydrogenase of Escherichia coli catalyzes the hydride transfer between reduced nicotinamide adenine dinucleotide (NADH) and reduced nicotinamide adenine dinucleotide phosphate (NADPH) coupled to translocation of protons across the cytoplasmic membrane.1 Inspired by nature's behavior, a variety of metal-promoted or catalyzed hydride transfer methods (e.g. hydrogenation) of imines or alkenes have been reported.2 Soon after that, organocatalytic hydride transfer reactions received some attention from chemists due to their environmentally benign features.3 Although N-heterocyclic carbene (NHC) catalyzed benzoin or Stetter reactions of aldehydes have been extensively studied,4 examples of carbene promoted or catalyzed hydride transfer are extremely few. In 2006, the Scheidt group reported the first NHC-catalyzed hydroacylation of activated ketones with aldehydes through a hydride transfer strategy (Scheme 1a).5 Later in 2013, Chen et al. disclosed an unprecedented 1,5-hydride transfer from the C–H group of an aldehyde to an activated alkene under a cascade amine and NHC catalysis.6 To the best of our knowledge, there are no further reports regarding the NHC-catalyzed hydride transfer process.
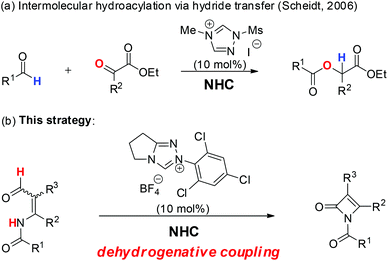 |
| Scheme 1 (a) NHC-catalyzed intermolecular hydroacylation; (b) NHC-catalyzed intermolecular dehydrogenative coupling. | |
The monobactam core is the key structural element of the most widely recognised family of antimicrobial agents.7 As shown in Fig. 1,7 Aztreonam is used primarily to treat infectious caused by Gram-negative bacteria; Tigemonam inhibited peptidoglycan subunit synthesis and transport; Tabtoxin is the precursor to the antibiotic tabtoxinine β-lactam. Nocardicin A exhibited a comparatively potent antimicrobial activity against Gram-negative organisms, especially Pseudomonas aeruginosa. Given the importance of monobactam in medicinal chemistry, the prospect of rapidly generating new monobactam-containing frameworks appeared particularly interesting. Among these known synthetic approaches, hydroxamate cyclization,8 enolate–imine condensation,9 carbene–imine reaction,10 isocyanate–alkene cycloaddition,11 and ketene–imine cycloaddition (Staudinger reaction),12 are the most commonly used tools for the construction of monobactams. In particular, the Staudinger reaction method has provided more economical entries to monobactam, mainly due to the readily availability of their starting materials. As part of a program aimed at developing new routes for the rapid buildup of monobactam derivatives, we considered a new reaction design in which an initial hydride transfer would ultimately result in the formation of the β-lactam ring.13 Herein, we disclose a novel intramolecular NHC-catalyzed dehydrogenative coupling reaction of enals for the synthesis of monobactams via a hydride transfer process (Scheme 1b).14
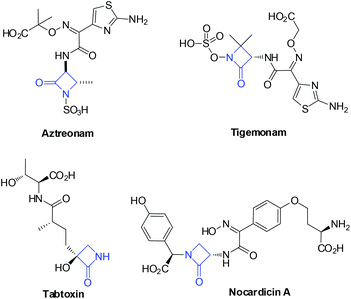 |
| Fig. 1 Selected examples of monobactam. | |
Results and discussion
We initiated our studies by investigating the intramolecular reaction of 1a under a variety of conditions. The model reaction of 1a in the presence of K2CO3 as a base additive and THF as a solvent was conducted at room temperature (Table 1, entries 1–7). As outlined in entry 3, cat. C resulted in a good yield (80%). However, cat. G afforded no product using similar conditions (entry 7). Various other reaction parameters were evaluated in order to improve the efficiency of this reaction and to maximize the yield of the desired product 2a. Different base additives are summarized in Table 1 (entries 8–16). Optimal results were obtained with 20 mol% of KHCO3 in THF (entry 8, 84%). Further screening of reaction media indicated no further improvement of yield (entries 17–22). Lowering reaction concentration led to a slight improvement in chemical yield (entry 23, 87%, 12 h). When a 5 mol% of cat. C was applied, a good chemical yield was still achieved at a plausible time (entry 24, 12 h).
Table 1 Optimization of reaction conditionsa
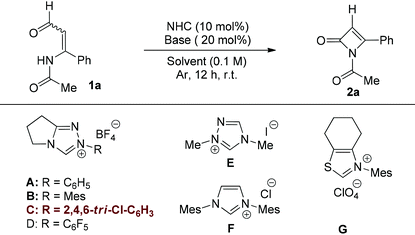
|
Entry |
NHC |
Solvent |
Base |
Yield of 2a b (%) |
Conditions: 1 (0.2 mmol, Z/E mixture), cat. C (10 mol%), KHCO3 (0.04 mmol), THF (2.0 mL), 12 h.
Isolated yield after flash chromatography.
THF (1.0 mL).
Cat. C (5 mol%), 12 h.
|
1 |
A |
THF |
K2CO3 |
56% |
2 |
B |
THF |
K2CO3 |
61% |
3 |
C |
THF |
K2CO3 |
80% |
4 |
D |
THF |
K2CO3 |
50% |
5 |
E |
THF |
K2CO3 |
44% |
6 |
F |
THF |
K2CO3 |
36% |
7 |
G |
THF |
K2CO3 |
Trace |
8 |
C |
THF |
KHCO3 |
84% |
9 |
C |
THF |
Na2CO3 |
59% |
10 |
C |
THF |
Cs2CO3 |
78% |
11 |
C |
THF |
CsOAc |
75% |
12 |
C |
THF |
KOAc |
61% |
13 |
C |
THF |
NaOAc |
42% |
14 |
C |
THF |
DBU |
73% |
15 |
C |
THF |
DIPEA |
26% |
16 |
C |
THF |
DMAP |
37% |
17 |
C |
1,4-Dioxane |
KHCO3 |
80% |
18 |
C |
CH3CN |
KHCO3 |
64% |
19 |
C |
iPr2O |
KHCO3 |
Trace |
20 |
C |
Toluene |
KHCO3 |
39% |
21 |
C |
DCM |
KHCO3 |
46% |
22 |
C |
CHCl3 |
KHCO3 |
Trace |
23
|
C
|
THF
|
KHCO
3
|
87%
|
24c,d |
C |
THF |
KHCO3 |
74% |
Having the optimized conditions in hand, we then turned our attention to the substrate scope of enal 2 (Table 2). First, enals with differently substituted alkyl (e.g. Me, tBu) or aryl (e.g. Ph) moieties in the R1 position were tested and all gave good yields (2a–c, 77–87%). In addition, this new method can be applied to a range of substrates with different substitution patterns of the aromatic ring in the R2 position. Both electron-donating (2d, 2k, 2l) and -withdrawing (2e–j, 2o–p) substituents on the phenyl unit were tolerated. Notably, enals containing a naphthyl ring (2q and 2r) and a heteroaryl (2s and 2t) substituent at the R2 position also reacted well, leading to the desired products with moderate to good yields (63–86%). Pleasingly, the substrate containing a bulky alkyl group in the R2 position could be tolerated without any loss of yield (Table 2, 2u, 76%, 12 h). It should be noted that the above used enals all had a Z/E mixture. But the experimental results indicated that both Z/E converted to the same desired products via an inherent tautomerization. Furthermore, enals with substituents in both R2 and R3 positions could also be tolerated, but generally afforded the desired product with low chemical yields (Table 2, 2v and 2w).
Conditions: 1 (0.2 mmol, Z/E mixture), cat. C (10 mol%), KHCO3 (0.04 mmol), THF (2.0 mL), 12 h.
Starting materials were recovered.
|
|
A postulated mechanism of this reaction process is depicted in Scheme 2. Reaction of enal 1a with the NHC catalyst C in the presence of KHCO3 initially forms intermediate I. Elimination of the tetrahedron intermediate I through a hydride transfer leads to the acyl azolium intermediate II. The subsequent dehydration converts intermediate II to intermediate III. Lastly, an intramolecular cycloaddition of intermediate III eventually forms the desired product 2a along with the regeneration of the NHC catalyst C.
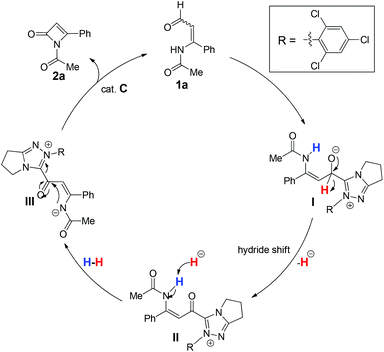 |
| Scheme 2 Plausible mechanism. | |
Conclusions
In summary, we have demonstrated that intramolecular dehydrogenative coupling of enals can be catalyzed by N-heterocyclic carbenes. A variety of monobactam products were obtained in moderate to high yields. Further investigations of the generality of this catalytic process and the development of asymmetric variants are currently ongoing and will be reported in due course.
Acknowledgements
The project described was supported by the grant from the Tsinghua University, the “Thousand Plan” Youth program of China, the Bayer Investigator Fellowship, and the Tsinghua-Peking Centre for Life Sciences (CLS).
Notes and references
-
(a) J. B. Jones, Tetrahedron, 1986, 42, 3351–3403 CrossRef CAS;
(b) E. Schoffers, A. Golebiowski and C. R. Johnson, Tetrahedron, 1996, 52, 3769–3826 CrossRef CAS;
(c)
J. McMurry and T. Begley, The Organic Chemistry of Biological Pathways, Roberts and Company Publishers, Englewood, CO, 2005 Search PubMed;
(d)
B. Alberts, D. Bray, J. Lewis, M. Raff, K. I. Roberts and J. D. Watson, Molecular Biology of the Cell, Garland, New York & London, 3rd edn, 2002 Search PubMed.
- For selected examples, see:
(a) K. Sakai, J. Ide, O. Oda and N. Nakamura, Tetrahedron Lett., 1972, 13, 1287 CrossRef;
(b) J. Schwartz and J. B. Cannon, J. Am. Chem. Soc., 1974, 96, 4721 CrossRef CAS;
(c) K. Tanaka and G. C. Fu, J. Am. Chem. Soc., 2001, 123, 11492 CrossRef CAS PubMed;
(d) K. Fuji, T. Morimoto, K. Tsutsumi and K. Kakiuchi, Chem. Commun., 2005, 3295 RSC;
(e) G. L. Moxham, H. E. Randell-Sly, S. K. Brayshaw, R. L. Woodward, A. S. Weller and M. C. Willis, Angew. Chem., Int. Ed., 2006, 45, 7618 CrossRef CAS PubMed;
(f) Z. Shen, H. A. Khan and V. M. Dong, J. Am. Chem. Soc., 2008, 130, 2916 CrossRef CAS PubMed;
(g) D. H. T. Phan, B. Kim and V. M. Dong, J. Am. Chem. Soc., 2009, 131, 15608 CrossRef CAS PubMed;
(h) A. B. Chaplin, J. F. Hooper, A. S. Weller and M. C. Willis, J. Am. Chem. Soc., 2012, 134, 4885 CrossRef CAS PubMed.
- For a comprehensive review, see: C. Zheng and S.-L. You, Chem. Soc. Rev., 2012, 41, 2498 RSC.
- Selected reviews on NHC catalysis:
(a) K. Zeitler, Angew. Chem., Int. Ed., 2005, 44, 7506 CrossRef CAS PubMed;
(b) D. Enders, O. Niemeier and A. Henseler, Chem. Rev., 2007, 107, 5606 CrossRef CAS PubMed;
(c) N. Marion, S. Diez-González and S. P. Nolan, Angew. Chem., Int. Ed., 2007, 46, 2988 CrossRef CAS PubMed;
(d) V. Nair, S. Vellalath and B. P. Babu, Chem. Soc. Rev., 2008, 37, 2691 RSC;
(e) E. M. Phillips, A. Chan and K. A. Scheidt, Aldrichimica Acta, 2009, 42, 55 CAS;
(f) J. L. Moore and T. Rovis, Top. Curr. Chem., 2010, 291, 77 CrossRef CAS PubMed;
(g) A. T. Biju, N. Kuhl and F. Glorius, Acc. Chem. Res., 2011, 44, 1182 CrossRef CAS PubMed;
(h) K. Hirano, I. Piel and F. Glorius, Chem. Lett., 2011, 40, 786 CrossRef CAS;
(i) P.-C. Chiang and J. W. Bode, TCIMeru, 2011, 149, 2 Search PubMed;
(j) V. Nair, R. S. Menon, A. T. Biju, C. R. Sinu, R. R. Paul, A. Jose and V. Sreekumar, Chem. Soc. Rev., 2011, 40, 5336 RSC;
(k) Z. Q. Rong, W. Zhang, G. Q. Yang and S.-L. You, Curr. Org. Chem., 2011, 15, 3077 CrossRef CAS;
(l) H. U. Vora and T. Rovis, Aldrichimica Acta, 2011, 44, 3 CAS;
(m) D. T. Cohen and K. A. Scheidt, Chem. Sci., 2012, 3, 53 RSC;
(n) X. Bugaut and F. Glorius, Chem. Soc. Rev., 2012, 41, 3511 RSC;
(o) A. Grossmann and D. Enders, Angew. Chem., Int. Ed., 2012, 51, 314 CrossRef CAS PubMed;
(p) J. Douglas, G. Churchill and A. D. Smith, Synthesis, 2012, 2295 CAS;
(q) J. Izquierdo, G. E. Hutson, D. T. Cohen and K. A. Scheidt, Angew. Chem., Int. Ed., 2012, 51, 11686 CrossRef CAS PubMed;
(r) S. J. Ryan, L. Candish and D. W. Lupton, Chem. Soc. Rev., 2013, 42, 4906 RSC;
(s) S. D. Sarkar, A. Biswas, R. C. Samanta and A. Studer, Chem. – Eur. J., 2013, 19, 4664 CrossRef PubMed;
(t) S. J. Connon, Angew. Chem., Int. Ed., 2014, 53, 1203 CrossRef CAS PubMed;
(u) J. Mahatthananchai and J. W. Bode, Acc. Chem. Res., 2014, 47, 696 CrossRef CAS PubMed;
(v) M. N. Hopkinson, C. Richter, M. Schedler and F. Glorius, Nature, 2014, 510, 485 CrossRef CAS PubMed;
(w) M. Binanzer, S.-Y. Hsieh and J. W. Bode, J. Am. Chem. Soc., 2011, 133, 19698 CrossRef CAS PubMed;
(x) Z. Fu, J. Xu, T. Zhu, W. Leong and Y. R. Chi, Nat. Chem., 2013, 5, 835 CrossRef CAS PubMed;
(y) K. Namitharan, T. Zhu, J. Cheng, P. Zhang, X. Li, S. Yang, B.-A. Song and Y. R. Chi, Nat. Commun., 2014, 5, 1982 Search PubMed;
(z) D. M. Flanigan, F. Romanov-Michailidis, N. A. White and T. Rovis, Chem. Rev., 2015, 15, 9307 CrossRef PubMed.
- A. Chan and K. A. Scheidt, J. Am. Chem. Soc., 2006, 128, 4558 CrossRef CAS PubMed.
- C. Ma, Z.-J. Jia, J.-X. Liu, Q.-Q. Zhou, L. Dong and Y.-C. Chen, Angew. Chem., Int. Ed., 2013, 52, 948 CrossRef CAS PubMed.
- For some reviews on β-lactam antibiotics and fungicides see:
(a)
The Organic Chemistry of β-Lactams, ed. G. I. Georg, VCH Publications, New York, 1993, and references cited therein Search PubMed;
(b)
F. C. Neuhaus and N. H. Georgopapadaku, in Emerging Targets in Antibacterial and Antifungal Chemotherapy, Chapman and Hall, New York, 1992 Search PubMed;
(c)
Chemistry and Biology of β-Lactam Antibiotics, ed. R. B. Morin and M. Gorman, Academic Press, New York, 1982 Search PubMed.
- M. J. Miller, Acc. Chem. Res., 1986, 19, 49256 CrossRef.
-
(a) D. J. Hart and D. C. Ha, Chem. Rev., 1989, 89, 1447 CrossRef CAS;
(b) M. J. Brown, Heterocycles, 1989, 29, 2225 CrossRef CAS.
-
(a) L. S. Hegedus, Acc. Chem. Res., 1995, 28, 299 CrossRef CAS;
(b) M. A. Barrett and M. A. Sturgess, Tetrahedron, 1988, 44, 5615 CrossRef.
- M. Chmielewski, Z. Kaluza and B. Furman, Chem. Commun., 1996, 2689 RSC.
-
(a) H. Staudinger, Liebigs Ann. Chem., 1907, 356, 51 CrossRef CAS;
(b) C. Palomo, J. M. Aizpurua, I. Ganboa and M. Oiarbide, Eur. J. Org. Chem., 1999, 3223 CrossRef CAS;
(c) R. D. G. Cooper, B. W. Daugherty and D. B. Boyd, Pure Appl. Chem., 1987, 59, 485 CrossRef CAS.
- For our group's recent progress on NHC-catalysis, see:
(a) C. G. Zhao, F. Y. Li and J. Wang, Angew. Chem., Int. Ed., 2015 DOI:10.1002/anie.201508205;
(b) F. Y. Li and J. Wang, Angew. Chem., Int. Ed., 2015, 54, 656 CAS;
(c) Z. J. Wu, F. Y. Li and J. Wang, Angew. Chem., Int. Ed., 2015, 54, 1629 CrossRef CAS PubMed;
(d) Z. J. Wu, X. Wang, F. Y. Li, J. C. Wu and J. Wang, Org. Lett., 2015, 17, 3588 CrossRef CAS PubMed.
- For recent reviews of dehydrogenative cross-coupling, see:
(a) C. S. Yeung and V. M. Dong, Chem. Rev., 2011, 111, 1215 CrossRef CAS PubMed;
(b) J. Le Bras and J. Muzart, Chem. Rev., 2011, 111, 1170 CrossRef CAS PubMed;
(c) S. A. Girard, T. Knauber and C.-J. Li, Angew. Chem., Int. Ed., 2014, 53, 74 CrossRef CAS PubMed;
(d) C.-J. Li, Acc. Chem. Res., 2009, 42, 335 CrossRef CAS PubMed.
Footnote |
† Electronic supplementary information (ESI) available. See DOI: 10.1039/c5qo00372e |
|
This journal is © the Partner Organisations 2016 |