DOI:
10.1039/D4RA01514B
(Review Article)
RSC Adv., 2024,
14, 14374-14391
Recent progress of electrospun nanofibers as burning dressings
Received
27th February 2024
, Accepted 25th April 2024
First published on 1st May 2024
Abstract
Burns are a global public health problem, which brings great challenges to public health and the economy. Severe burns often lead to systemic infection, shock, multiple organ failure, and even death. With the increasing demand for the therapeutic effect of burn wounds, traditional dressings have been unable to meet people's needs due to their single function and many side effects. In this context, electrospinning shows a great prospect on the way to open up advanced wound dressings that promote wound repairing and prevent infection. With its large specific surface area, high porosity, and similar to natural extracellular matrix (ECM), electrospun nanofibers can load drugs and accelerate wound healing. It provides a promising solution for the treatment and management of burn wounds. This review article introduces the concept of burn and the types of electrospun nanofibers, then summarizes the polymers used in electrospun nanofiber dressings. Finally, the drugs (plant extracts, small molecule drugs and nanoparticles) loaded with electrospun burn dressings are summarized. Some promising aspects for developing commercial electrospun burn dressings are proposed.
1 Introduction
The skin is the largest organ in the human body, covering the surface of the body and accounting for approximately 10% of body weight.1 Burns are injuries to the skin caused by high temperatures (flame or scald), electricity, chemicals, friction, or radiation.2 The healing process of burn wounds is similar to that of most other wounds, and both go through a complex process. The wound healing process usually includes four stages: hemostasis, inflammation, cell proliferation, and tissue remodelling.3 Mild burns can recover quickly with the aid of some medications. However, severe burns are often accompanied by complications such as bacterial infection, shock, and organ failure. Burns reduce the immunomodulatory function of the patient's skin, making the wound vulnerable to bacterial infection. Bacterial infection can cause an increase in wound exudate, which seriously hinders wound healing and brings huge challenges to wound treatment.4
When the skin damage is too severe and the autologous repair ability is not enough to restore the original structure and function, the traditional method of skin transplantation is generally used. In the past few decades, the survival rate of burn patients has been significantly improved due to the application of various skin grafts.5 Despite widespread use, there are problems with limited donors for autologous skin grafts and immune rejection of allogeneic transplants.6,7 With the development of biotechnology, the emergence of multifunctional wound dressings and nanofiber scaffold materials has alleviated the problem of skin transplantation to a certain extent. Wound dressings can cover the wound and provide a temporary barrier against external bacterial infections.8 It can also serve as an induction template to guide skin cell recombination, host tissue infiltration, and integration, and has a significant effect on wound healing.9 Traditional dressings such as gauze and bandages are often used in clinical practice to assist in wound treatment. They can absorb wound exudate and blood, while also providing mechanical protection. However, the disadvantage of traditional dressings is that they do not have antiinfection ability and are prone to adhesion to the wound, causing secondary damage to the patient, which is not conducive to wound healing. The ideal wound dressing should have the following properties: (1) absorb excess wound exudate and blood; (2) provide a moist environment for wound healing; (3) good breathability; (4) protect the wound from infection by external microorganisms and harmful substances; (5) good biocompatibility and degradability; (6) removing the dressing will not cause secondary damage to the patient's wound.
In addition to traditional dressings, common types of dressings include sponges, films, foams, hydrogels, hydrocolloids, and nanofiber composites.10–12 Different types of dressings have different characteristics and can be selected for specific types of wounds. Sponge dressing has a highly porous structure, which can absorb a lot of water and provide a moist environment for wound healing.13 However, sponge dressings exhibit weak mechanical strength and may cause skin maceration. Film dressings are breathable and can reduce patient pain.14 However, this type of dressing has weak water absorption, which can easily cause the accumulation of wound exudate and easily adhere to the wound.15 Hydrogels are hydrophilic macromolecular networks that can absorb and retain wound exudates, and promote fibroblast proliferation and glial cell migration.16 The mechanical stability of hydrogel becomes worse after water absorption and swelling. As a nanoscale material that is constantly being promoted for use, nanofibers are increasingly used as wound dressings. Nanofibers with nanoscale structures are similar to natural extracellular matrix, possessing good drug loading and delivery capabilities, and being able to absorb more wound exudates than other types of dressings.17–19 The nanofibers prepared by electrospinning have good mechanical and biological properties.
The preparation methods of nanofibers include drawing methods, template synthesis, self-assembly, microphase separation, and electrospinning.20–24 Electrospinning technology is widely used in the preparation of nanofibers because of its low cost, high efficiency, and simple operation. Electrospinning is an efficient method for preparing fibres with diameters between nanometres and micrometres.25 The structure of electrospun nanofibers can be adjusted to adapt to various applications.26–28 For example, in the biomedical field, electrospun nanofibers are used in wound dressings, tissue engineering scaffolds, and drug delivery.29–33 Electrospun nanofiber wound dressings have many outstanding advantages. Firstly, electrospun nanofibers have a larger specific surface area, providing more adhesion and growth sites for cells.34 Secondly, electrospun nanofibers have high porosity, maintaining good breathability and moisture permeability while blocking the invasion of external pathogens.35,36 Finally, electrospun nanofibers have a controllable structure and can serve as drug carriers to load various bioactive ingredients and drugs to improve wound healing efficiency.37–40 In addition, nanofiber wound dressings can also control the rate and time of drug release. Therefore, electrospun nanofibers show great potential in the field of biomedical applications.
In the “PubMed” and “Web of Science” databases, the relevant literature in the past ten years is searched by searching for the keywords “wound dressing” and “electrospun wound dressing”. The search results are shown in Fig. 1. From the search results, it can be seen that there are thousands of literatures on wound dressings in both databases each year, and there is an increasing trend over time. Especially after 2018, the growth rate of related literature has increased significantly, which shows that the research on wound dressing has become a hot topic in the field of biomedicine. At the same time, the literature on electrospun nanofiber dressings also shows an increasing trend. It shows that with the development of electrospun nanofiber technology, the wound dressing prepared by nanofiber has been recognized by people. The main work of this paper is to review the common types of electrospun nanofibers, the polymers used, and various drugs with therapeutic or antimicrobial activity wrapped in electrospun nanofiber dressings.
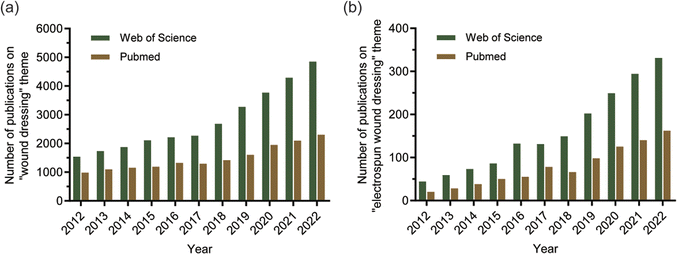 |
| Fig. 1 The literature related to the past ten years was searched in the databases of “PubMed” and “Web of Science”. (a) The number of literatures retrieved based on the theme “wound dressing”; (b) the number of literatures retrieved based on the theme “electrospun wound dressing”. | |
2 Burn and wound healing
2.1 Burn
Burns are an underestimated form of trauma that can affect anyone, anytime and anywhere.41 According to the severity of burns, they can be divided into four levels: first-degree burns, second-degree burns, third-degree burns, and fourth-degree burns.11,42 First-degree burns only involve the epidermis, accompanied by redness and mild pain, which subsides within 48–72 hours.43 This degree of burn does not require special treatment and will not leave scars. Second-degree burns are divided into superficial second-degree burns and deep second-degree burns. Superficial second-degree burns involve the epidermis and part of the dermis, accompanied by pain.44 Deep second-degree burns involve the surface layer of skin and the deeper dermis because some pain receptors are destroyed and pain is felt slowly. This degree of burn requires surgical treatment and can form scars. If the wound is not infected, it will take 3–4 weeks to heal. Third-degree burns run through the entire dermis. Because nerve endings are damaged, they usually don't feel pain and need to be protected from infection.41 Third-degree burns usually need to be treated by skin grafting. In addition, if the burn depth involves deep tissue, it is called a fourth-degree burn. It combines the characteristics of second and third-degree burns, penetrating from the epidermis to the subcutaneous tissue layer, damaging some muscles or bones.45 Fig. 2 shows the depth of each level of burn. In general, superficial burns, like normal mechanical injuries, surgical wounds, etc., undergo a natural wound healing process without much intervention. The difference, however, is that severe burns can disrupt the structural and functional integrity of the skin, increasing the likelihood of bacterial infection and bringing about a range of systemic reactions.46 Patients with extensive deep burns often develop severe systemic inflammation, hypermetabolism, and catabolic responses, and if not treated with prompt surgical intervention, they can die from multiple organ failure.47,48 In addition, burns can result in hypertrophic scars, which not only affect aesthetics but may also limit normal body function. The key to burn wound management is to prevent infection as well as to improve the scarring caused by burns. Therefore, the addition of antimicrobial drugs to burn dressings is the preferred method compared to normal wound dressings, and drugs with anti-inflammatory activity can also be added to inhibit scarring due to excessive inflammation.
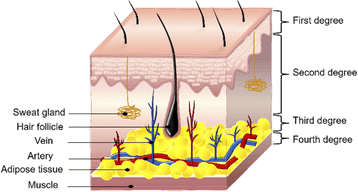 |
| Fig. 2 Burns depth and level. Burns are classified into four grades based on the size and depth of skin damage: first-degree, second-degree, third-degree, and fourth-degree burns. | |
2.2 Healing process of burn wounds
Wound healing is a dynamic and complex process that is coordinated by various cells, cytokines, growth factors, and neuroendocrine mechanisms.49 Skin wound healing is usually divided into four overlapping phases: hemostasis, inflammation, proliferation, and remodeling (Fig. 3).50 When tissues and cells of the body are damaged, inflammatory mediators activate the host's vascular and leukocyte responses for hemostasis and inflammation. The release of various cytokines allows neutrophils to accumulate in the wound and remove bacteria and damaged tissue.51,52 After some time, neutrophils gradually decrease and macrophages increase. Macrophages and neutrophils begin to phagocytose bacteria and nonfunctional host cells. Macrophages secrete fibroblast growth factor (FGF), platelet-derived growth factor (PDGF), transforming growth factor-β (TGF-β), interleukin-1 (IL-1), and tumour necrosis factor (TNF) to further stimulate capillary angiogenesis and fibroblast proliferation. The proliferation, migration, and remodelling of endothelial cells is an important process of angiogenesis.53 New capillaries and hyperplastic fibroblasts form granulation tissue, which is then remodeled and matures to form a scar. During the tissue remodelling phase, the wound undergoes epithelial regeneration via keratinized cells and extracellular matrix accumulation via fibroblasts and endothelial cells.54
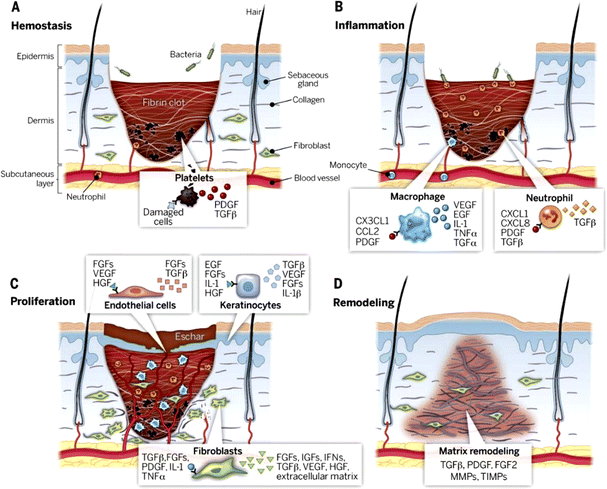 |
| Fig. 3 Stages of wound healing: (A) hemostasis, (B) inflammation, (C) proliferation, (D) remodelling. Reproduced from ref. 50 with permission from Science, copyright 2003. | |
There are many factors that affect the wound healing process, and bacterial infection is one of the most important. Bacterial infections can increase wound exudate and interfere with the normal growth of granulation tissue.55 Bacterial infections may also prolong or prevent wound healing by maintaining the inflammatory phase of the healing process. It has been reported that about 75% of burn patients die from bacterial infections.56 Therefore, antibacterial dressings can be used to assist in the treatment of burns. A qualified dressing can not only provide a suitable environment for wound healing but also have excellent anti-infection ability.
The researchers tried different methods to produce wound dressings with antibacterial activity, including the use of materials with inherent bactericidal activity, the improvement of surface structure, or the addition of antimicrobial agents.57 In recent years, people have been trying to study a variety of bioactive compounds, combining these compounds with films, sponges, foams, hydrogels, and nanofibers to make advanced dressings. Advanced dressing has a better effect on promoting wound healing than traditional dressing. Among them, the preparation process of electrospun nanofiber dressing is simple and the cost is low, so it has a good development prospect in the field of wound treatment and management.
3 Electrospun nanofiber
3.1 Introduction to electrospinning technology
Electrospinning is a simple and versatile technique for preparing nanofibers containing bioactive compounds.58,59 The diameters of electrospun nanofibers range from nanometers to microns, which are two to three orders of magnitude smaller than those made by traditional dry or wet spinning methods.60–62 The electrospinning device is mainly composed of four parts: injection pump, syringe with spinneret, collection device, and high voltage power supply (Fig. 4). The specific electrospinning process is as follows. The prepared polymer solution is loaded into a syringe fixed to the syringe pump and the polymer solution is controlled at a constant flow rate to the spinneret. The high-voltage power supply applies high voltage to the spinneret, and the electrostatic charge accumulates in the polymer solution at the tip of the spinneret, gradually driving the droplet into a cone, known as the “Taylor cone”.63,64 When the strength of the electrostatic field exceeds the surface tension of the solution, the charged polymer solution ejects from the tip of the Taylor cone.26 Under the action of the electric field force between the spinneret and the collecting device, the ejected polymer solution goes through bending and whipping stages and then falls on the collecting device. With the volatilization of the solvent, the stretched and cured polymer solution is deposited on the collection device, resulting in the formation of nanofibers.65
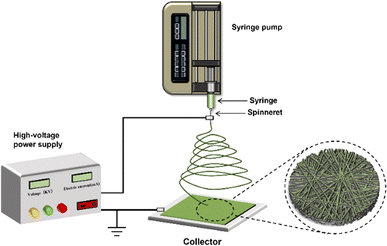 |
| Fig. 4 Electrospinning device. The electrospinning device is mainly composed of an injection pump, a syringe connected with a spinneret, a high-voltage power supply, and a collector. | |
In the process of electrospinning, the size and shape of nanofibers can be changed by changing solution parameters (polymer type, viscosity, concentration, conductivity, and surface tension), process parameters (electric field strength, electrostatic potential, acceptance distance, pore diameter) and environmental conditions (temperature, humidity, local airflow).66–70 Nanofibers with different morphological structures can realize the loading and precisely controlled release of various drugs. Sustained drug release ensures smooth drug concentration in the wound, reducing dosing frequency and improving compliance.71,72 Electrospun nanofibers have facilitated the development of drug delivery systems and show promising prospects in wound healing and skin regeneration.73,74 To provide more structures for nanofibers, many strategies have been developed to improve electrospinning technology. As shown in Fig. 5, there are various types of electrospun nanofibers: (1) single-fluid electrospinning: blending electrospinning and emulsion electrospinning; (2) double-fluid electrospinning: coaxial electrospinning and side-by-side electrospinning; (3) multi-fluid electrospinning.
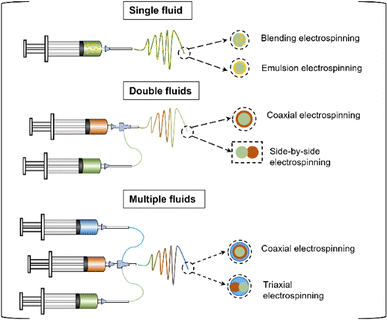 |
| Fig. 5 According to the number of fluids, electrospinning can be divided into single-fluid electrospinning (blended electrospinning and emulsion electrospinning), double-fluid electrospinning (coaxial electrospinning and side-by-side electrospinning), and multi-fluid electrospinning. | |
3.2 Single fluid electrospinning
3.2.1 Blending electrospinning. Blending electrospinning is a commonly used method for preparing blended nanofibers containing two or more different polymers.75,76 Before electrospinning, different polymers need to be added to the solvent in a certain proportion and mixed thoroughly. After testing, blending electrospinning can achieve the delivery of cell inhibitors, antibiotics, and therapeutic drugs with specific functions.77–81 The choice of solvent determines whether polymers and drugs can be fully dissolved. If the solvent that dissolves the polymer cannot dissolve the drug, it is necessary to dissolve the drug with a small amount of solvent that dissolves the drug, and then blend electrospinning with the polymer solution.63,82,83 The disadvantage of blending electrospinning is also obvious. If the drug is not fully dissolved, it will accumulate on the surface of the nanofibers and cause sudden drug release.
3.2.2 Emulsion electrospinning. Emulsion electrospinning is a simple and effective method for the preparation of core–shell nanofibers, which improves the problem of sudden drug release caused by blending electrospinning.84 Emulsion electrospinning usually uses two types of emulsions. One is a water-in-oil emulsion based on a lipophilic continuous phase and a hydrophilic liquid drop phase.85 This type of emulsion is used to encapsulate hydrophilic small molecular proteins or drugs. The emulsion is stabilized by surfactants/emulsifiers with low hydrophilic and lipophilic balance.86 The other is oil-in-water emulsion which is formed by lipophilic droplet phase and hydrophilic continuous phase. The hydrophobic drug was dissolved in the oil phase solvent and then diffused into the hydrophilic continuous phase. Compared with blending electrospinning, emulsion electrospinning has more advantages in the preparation of nanofibers containing low-solubility drugs. Compared with the drug-loaded nanofibers prepared by electrospinning of blends, the nanofibers based on emulsion electrospinning show more uniform drug distribution.87 Jiang et al. reported the electrospinning of a Pickering emulsion loaded with a natural antimicrobial agent, tea tree oil (TTO), and stabilized with ZnO/Ag nanocomposites for antimicrobial dressings.88 With the stabilization of ZnO and Ag nanoparticles, TTO could be uniformly distributed in the Pickering emulsion with good stability. The results showed that the prepared nanofiber dressings had excellent long-term bacteriostatic activity against both Gram-positive and Gram-negative bacteria, as well as good biocompatibility and blood compatibility. In addition, it effectively promoted cell proliferation and migration when the concentration of nanofiber samples was 800 μg ml−1 or less.
3.3 Double-fluid electrospinning
3.3.1 Coaxial electrospinning. Coaxial electrospinning can simultaneously use two kinds of polymer solutions to prepare nanofibers with core–shell structures. The advantage of this structure is that the drug is put into the core layer, which reduces the phenomenon of sudden drug release and prolongs the drug release time.89–91 Coaxial electrospinning can use a combination of spinnable solution and non-spinnable solution, which is generally put into the core layer. When preparing core–shell nanofibers using coaxial electrospinning, it is necessary to consider the miscibility, viscosity, and conductivity between different layers of solutions.92–94 Nanofiber burn dressings with dual drug loading of centella total glucoside and ciprofloxacin were prepared by coaxial electrospinning technique by Guo et al.95 They placed centella total glucoside into the nuclear layer to avoid sudden release while prolonging the therapeutic effect of the drug. Antimicrobial experiments and in vitro cellular experiments showed that the prepared nanofibers had excellent antimicrobial activity and the ability to promote the proliferation of fibroblasts. In addition, rat burn experiments showed that the nanofiber dressing also significantly accelerated wound healing by promoting endothelial cell proliferation and angiogenesis. In another study, Ramalingam et al. prepared core–shell nanofibers, in which polycaprolactone/gelatin loaded minocycline hydrochloride as the shell, and gelatin containing Gymnema sylvestre extract as the core layer.96 Compared with the blended nanofibers they prepared, the core–shell structure promotes the sustained release of bioactive components in the nanofibers. The plant extracts in the shell layer and the antibiotics in the core layer exerted a good synergistic effect, which together achieved antimicrobial activity and promoted cell proliferation. Experiments on second-degree burns in porcine skin demonstrated that the dressing could effectively promote collagen deposition and re-epithelialization. Coaxial electrospinning provides an effective method for encapsulating bioactive materials in biomedical nanofibers.97,98
3.3.2 Side-by-side electrospinning. Based on single-fluid electrospinning, Janus nanofibers can be prepared using parallel spinnerets. Unlike the core–shell structure, all parts of the Janus structure can be in direct contact with the external environment.99,100 Janus nanofibers can achieve biphasic controlled release during drug release. Yang et al. prepared Janus nanofiber dressing loaded with ciprofloxacin and silver nanoparticles on both sides by side-by-side electrospinning.101 Transmission electron microscopy images showed that the prepared Janus had a clear Janus structure. In vitro drug release experiments showed that the release of ciprofloxacin was almost complete within the first 30 minutes, which ensured that the strongest antimicrobial activity was achieved in the early stage of wound healing. In addition, Janus nanofibers showed good antimicrobial activity against Staphylococcus aureus and Escherichia coli due to the synergistic effect of ciprofloxacin and silver nanoparticles. In a similar study, Shi et al. prepared amphiphilic Janus nanofiber membranes loaded with copper sulfide nanoparticles, mupirocin and valsartan using polyvinyl alcohol, polylactic acid-glycolic acid.102 Their prepared Janus nanofiber membranes possessed obvious amphiphilic characteristics and were capable of sustained release of the antimicrobial agent mupirocin in a hydrophilic environment. In addition, the photothermal effect of the amphiphilic Janus nanofiber membrane can also control the release of the anti-inflammatory agent valsartan. Therefore, the wound dressing they prepared can effectively solve the problems of infection and inflammation in wound healing, providing a feasible method for clinical application. Presently, side-by-side electrospinning and the functionalizations of Janus nanofibers are hot topics in some scientific fields such as energy, electronic textiles, drug delivery, and environments.103–107
3.4 Multi-fluid electrospinning
Triaxial electrospinning can achieve more functions, such as loading drugs with different properties, longer drug release times, and solving the problem that some drugs are difficult to dissolve.108,109 Curcumin possesses several excellent antibacterial, anti-inflammatory and antioxidant activities, but it is difficult to dissolve in water. In order to improve the utilization of curcumin's active ingredients, Liu et al. prepared nanofibers with controllable thickness of the sheath layer for the controlled release of curcumin using modified triaxial electrospinning.110 They prepared nanofibers with uniform linear morphology and distinct core-sheath structure. The experimental results showed that the core-sheath nanofibers were able to precisely control the release of curcumin and showed good antibacterial activity against both Staphylococcus aureus and Escherichia coli. Triaxial electrospinning technology opens up a new way to prepare complex nanomaterials and shows great advantages in the formation of drug delivery systems with complex nanostructures.111
4 Polymer used for making electrospun nanofiber burn dressings
The selection of polymers for electrospun nanofibers is crucial for burn wound management as it affects the mechanical properties and therapeutic activity of dressings.112 There are differences in viscosity, nanofiber morphology, biocompatibility, and mechanical strength of polymer spinning solutions from different sources.113 Both natural polymers and synthetic polymers are feasible choices. Natural polymers have better biocompatibility, while synthetic polymers have better mechanical properties.114 Table 1 summarizes the natural and synthetic polymers used in the preparation of electrospun nanofiber burn dressings. These polymers mainly include natural ones such as chitosan,115–120 gelatin,121–123 silk fibroin,118,124,125 sodium alginate,126,127 and synthetic polymers such as poly(ε-caprolactone),128–132 poly(vinyl alcohol),119,125,133 poly(ethylene oxide),120,127,131 poly(vinyl pyrrolidone),132 polyurethane126 and polylactic acid.134
Table 1 Polymers for preparation of electrospun nanofibers
4.1 Natural polymers
4.1.1 Chitosan. Chitosan (CS) is a natural polymer derived from chitin. It has excellent biocompatibility, biodegradability, antioxidant, and antibacterial activity.135 Due to its unique biological activity, CS is widely used in drug delivery, wound dressings, and tissue engineering scaffolds.136–138 It is not easy to prepare nanofibers from a single CS solution using electrospinning technology. To solve this problem, Shabunin et al. added chitin nanofibril to the CS solution, which significantly improved the formation rate of CS nanofibers.115 The wound dressing has two layers, and the outer layer is composed of polyamide nanofibers to provide mechanical strength for the wound dressing and prevent the invasion of external bacteria. The inner layer is composed of CS mixed with chitin nanofibril, which is mainly responsible for sterilizing and absorbing wound exudate. Liu et al. chose polycaprolactone (PCL) with excellent mechanical properties to blend with chitosan to improve the physical properties of chitosan.116 The PCL/CS graft copolymer has both the biological activity of CS and the mechanical stability of PCL. The results of clinical experiments showed that PCL/CS nanofiber dressing had better antibacterial activity and mechanical properties than traditional dressing.Unlike most other polysaccharides, CS is a natural cationic polymer that can be blended with other negatively charged natural or synthetic polymers.139 The antibacterial activity of chitosan due to the interaction between the positive charge of chitosan and the negative charge on the surface of bacteria, resulting in the rupture of bacterial biofilm.140 Mirhaj et al. designed a bilayer wound dressing simulating the skin tissue structure, with chitosan/polyethylene glycol containing advanced platelet-rich fibrin (A-PRF) in the upper layer and chitosan electrospun nanofibers loaded with L-arginine in the lower layer.117 The results showed that the prepared bilayer dressing possessed excellent antimicrobial activity and potential to promote blood vessel formation. This was attributed to the synergistic antimicrobial effect of the positively charged L-arginine with the equally positively charged chitosan. Studies have shown that arginine has the ability to stimulate vascular regeneration and enhance collagen synthesis.141 Therefore, the L-arginine in this wound dressing together with A-PRF promotes angiogenesis and accelerates wound healing.
4.1.2 Gelatin. Gelatin, which is mainly extracted from collagen in animal skin, muscle, and bone, is a heterogeneous mixture of highly hydrophilic peptides.142,143 Gelatin is widely used in biomedical fields, such as biological stents, vaccine development, anticancer drugs, and wound dressings.144–146 However, gelatin needs to be blended with other polymers to enhance its mechanical properties. Poly(L-lactic-co-caprolactone) nanofibrous membranes loaded with Epigallocatechin-3-O-gallate (EGCG) were prepared by Li et al. using coaxial electrospinning, and gelatin was added to enhance the hydrophilicity and biocompatibility of nanofibrous membranes.121 The results showed that the wound dressing had good biocompatibility, antimicrobial, antioxidant, and ability to promote wound closure. Leung et al. used gelatin and polycaprolactone to prepare a chondroitin sulphate-loaded blend and coaxial nanofiber composite dressings.122 They established a pig skin burn model to test the effect of two kinds of nanofibers on wound healing. Compared with polycaprolactone/gelatin blend nanofibers, core–shell nanofibers were easier to promote cell growth and achieved controlled drug release. During the healing process of burn wounds, a large amount of exudate is produced, which can hurt wound healing. Considering this problem, Zhou et al. prepared an asymmetric wettable fibre membrane by electrospinning.123 The inner layer was composed of hydrophilic gelatin and ginsenoside Rg1, and the outer layer was a mixture of black phosphorus grafted chitosan and poly(lactic-glycolic) acid (Fig. 6). Similar to the dermis of human skin, the hydrophilic inner layer can not only remove excess wound exudate but also provide a moist environment for the wound.
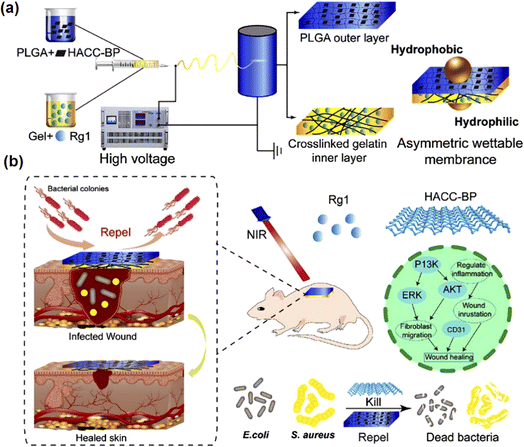 |
| Fig. 6 (a) Preparation process of the asymmetric wettable membrane. (b) The antibacterial and wound healing properties of asymmetric wettable film. Reproduced from ref. 123 with permission from Wiley, copyright 2022. | |
4.1.3 Silk fibroin. Silk fibroin (SF) is a natural macromolecular fibrin extracted from silkworm cocoons.147 Compared with other synthetic and natural polymers, SF has better biocompatibility, non-toxicity, biodegradability, resistance, and thermal stability.148,149 In addition, SF has excellent toughness and is often mixed with other polymers to improve the mechanical properties of nanofibers. Khosravimelal prepared CS/SF electrospun nanofibers containing cationic antimicrobial peptides as wound dressings.118 The combination of cationic antimicrobial peptide and chitosan can significantly improve the antibacterial effect of nanofiber dressings. SF can improve the weak mechanical properties of chitosan, which is well proved by the results of the stress–strain curve. Mollaghadimi used SF and PCL to prepare nanofiber wound dressings and added allicin to improve the antibacterial properties.124 Yerra et al. prepared electrospun nanofibers by blending silk fibroin, polyvinyl alcohol, and antibiotics.125 The wound therapeutic effect of nanofiber dressing was tested by a rat burn model. The results showed that the dressing had excellent antibacterial ability, and it also promoted the cell viability and cell adhesion of fibroblasts. SF comes into direct contact with the wound and releases antibiotics to remove pathogens. The activity of silk fibroin/polyvinyl alcohol nanofiber dressings is mainly reflected in the biodegradability of SF and the release rate of drugs.
4.1.4 Sodium alginate. Sodium alginate is a natural polysaccharide polymer extracted from brown algae.150 Because of its non-toxicity, antioxidation, biocompatibility, and biodegradability, it has aroused great interest in biomedical applications.151 The polymer synthesized from sodium alginate has weak mechanical properties and needs to be mixed with other polymers to maintain structural integrity. A series of nanofiber burn dressings based on polyurethane (PU), hydroxypropyl trimethyl ammonium chloride chitosan (HACC) and sodium alginate (SA) were prepared by Guo et al.126 The natural anionic polysaccharide SA absorbs wound exudate and maintains a moist environment, and also interacts with the cationic HACC to reduce its cytotoxicity. Cellular experiments showed that the SA-containing nanofibers significantly promoted the proliferation and migration of keratinocytes and fibroblasts. Huang et al. reported a multilayer nanofiber film, the upper and lower layers of which were poly(ethylene oxide)/sodium alginate electrospun nanofiber films, and the intermediate layer was nanofibers loaded with tetracycline hydrochloride.127 The multilayer nanofiber membrane has a regular arrangement structure and many voids, which provides an ideal environment for cell adhesion and proliferation. In addition, the fibre membrane also had an excellent drug-controlled release (release period of more than 10 days) and antibacterial effect. Sodium alginate can release bioactive substances and reduce the expression of proinflammatory factors, which shows great potential in the treatment of burns.
4.2 Synthetic polymers
4.2.1 Poly(ε-caprolactone). Poly(ε-caprolactone) (PCL) is a biodegradable, safe, and non-toxic hydrophobic synthetic polymer, which has been approved by FDA for biomedical applications.152 Because of its good mechanical properties and biocompatibility, it has been widely used as a raw material for the preparation of electrospun nanofibers. However, the electrospun nanofibers based on PCL are not hydrophilic, which may not provide an ideal environment for cell adhesion and proliferation.153 PCL needs to be blended with other hydrophilic polymers or drugs to improve hydrophilicity. Zhou et al. prepared chitosan oligosaccharide/polycaprolactone nanofiber membranes loaded with rutin and quercetin.128 The authors explained that blending chitosan oligosaccharides with PCL can improve the hydrophilicity of nanofiber membranes and provide a moist environment for wound healing. The experimental results showed that the prepared nanofiber membrane had good hydrophilic, antioxidant, and antibacterial properties, and could promote wound healing. Bayat et al. prepared kiwifruit extract (KE) loaded nanofibers by blending hydrophilic cellulose acetate with PCL and evaluated their properties of promoting burn wound healing.129 In the test of wound healing effect for more than 21 days, PCL/CA/KE nanofiber dressing had a better effect than KE. In addition, PCL/CA/KE effectively promoted the proliferation of fibroblasts. Guo et al. prepared electrospun nanofiber mats using PCL and α-lactalbumin (ALA).130 The wettability and mechanical properties of nanofiber wound dressings were improved by adjusting the mass ratio of ALA to PCL. The results proved that the prepared nanofibers had a significant effect on promoting wound healing and reducing scar.
4.2.2 Poly(vinyl alcohol). Polyvinyl alcohol (PVA) is a kind of synthetic polymer with semi-crystalline properties.154 Because of its excellent hydrophilicity, biocompatibility, and biodegradability, it is widely used in the preparation of medical biomaterials such as hydrogels, films, scaffolds, and nanofibers.155,156 Morais et al. prepared electrospun nanofiber mats by mixing PVA, CS, green propolis extract, and nystatin.119 The combination of PVA and CS makes the biodegradable nanofiber mat have better water absorption and swelling ability, which is beneficial to the absorption of wound exudate. Ramkumar et al. prepared PVA nanofiber scaffolds doped with molybdenum nanoparticles.133 The experimental results showed that the nanofiber scaffold was compatible with red blood cells and had excellent biocompatibility. The diameter of the bacteriostatic circle was more than 7 mm, which proved that it had better antibacterial performance. Their research confirms the advantages of polyvinyl alcohol nanofibers mixed with metal nanoparticles in promoting cell growth.
4.2.3 Poly(ethylene oxide). Poly(ethylene oxide) (PEO) is a kind of synthetic polymer, which shows excellent biocompatibility, biodegradability, and water solubility.157 Singh et al. used PEO, PCL, and silver sulfadiazine to prepare nanofibers with core–shell structure.131 PEO mixed with silver sulfadiazine as the core layer (Fig. 7A(a)). The prepared core–shell nanofibers showed sudden drug release in the initial stage (Fig. 7A(c)). This is because PEO tends to absorb water and expand, resulting in the release of drugs too quickly. With the gradual degradation of the core layer, the release curve gradually tended to be stable, and the long-term sustained release of silver sulfadiazine was realized. Abid et al. used CS and PEO to prepare nanofiber burn dressings loaded with ZnO nanoparticles (Fig. 7B).120 Because it was too difficult to spin with a single CS solution, the author added PEO to improve the spinnability of the CS solution. The results showed that the prepared nanofibers had good antibacterial activity, biocompatibility, and stability.
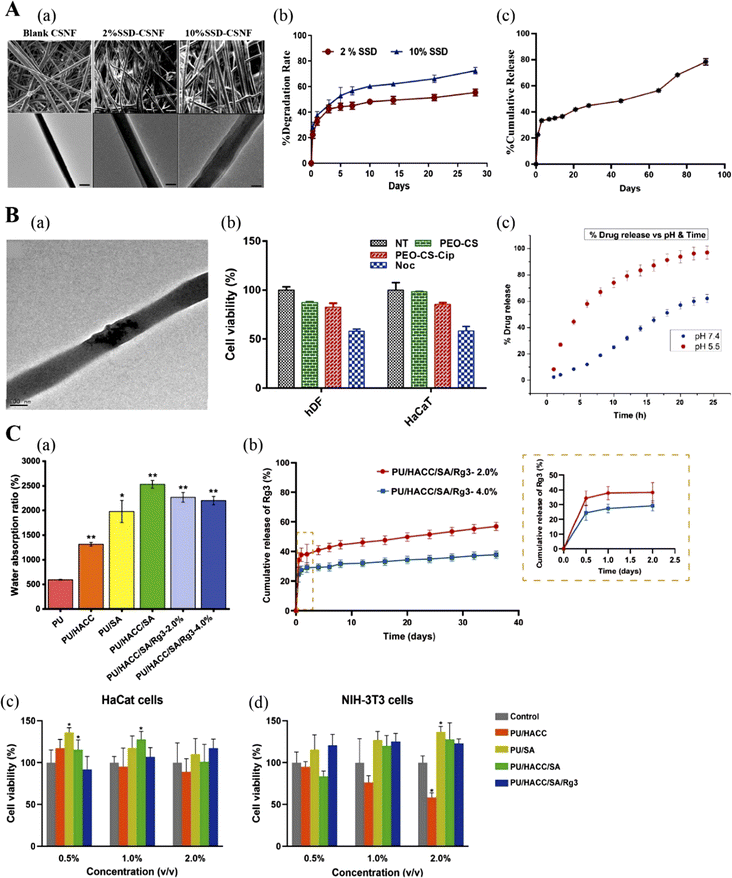 |
| Fig. 7 (A) Silver sulfadiazine (SSD) loaded core–shell nanofibers: (a) SEM and TEM images of core–shell nanofibers (CSNF), 2% SSD loaded and 10% SSD loaded core–shell nanofibers (SSD-CSNF); (b) degradation profile of 2% SSD-CSNF; (c) the release profile of 2% and 10% SSD-CSNF. Adapted from ref. 131 with permission from Elsevier, copyright 2022. (B) Poly(ethylene oxide)-chitosan nanofibers: (a) TEM image of ciprofloxacin-loaded nanofibers with a size of 100 nm; (b) cytotoxicity of nanofibers to human dermal fibroblasts and keratinocytes; (c) commutative drug release % vs. pH and time. Adapted from ref. 120 with permission from Elsevier, copyright 2019. (C) Ginsenoside Rg3-loaded polyurethane/marine polysaccharide-based nanofiber dressings: (a) water absorption; (b) release curve of ginsenoside Rg3 with time; (c) and (d) are the effects of different concentrations of nanofiber dressing extracts on the proliferation of keratinocytes and fibroblasts. Adapted from ref. 126 with permission from Elsevier, copyright 2023. | |
4.2.4 Poly(vinyl pyrrolidone). Water-soluble polymer polyvinylpyrrolidone (PVP) has excellent film-forming, biodegradability, high polar groups, and is non-toxic.158,159 Khataei et al. reported a composite nanofiber wound dressing using polyamide-6, PVP, and tea tree essential oil produced by electrospinning.132 The results of mechanical property tests showed that PVP improved the mechanical strength of the nanofibers, thus protecting the wound from external forces during the healing process. As the molecular weight of PVP increased, the nanofibers exhibited higher porosity, water vapor permeability, and surface wettability, thereby promoting cell adhesion and proliferation. In addition, the produced nanofibers containing tea tree essential oil exhibited excellent antioxidant and antimicrobial properties. This explains why the nanofiber dressings they prepared can effectively promote wound healing.
4.2.5 Polyurethane. Polyurethane has been widely used in the biomedical field due to its excellent biocompatibility and tensile strength.160 Guo et al. prepared composite nanofiber dressings loaded with ginsenoside Rg3 using polyurethane and marine polysaccharides (Fig. 7C).126 This composite dressing showed a significant promoting effect on the growth of fibroblasts and keratinocytes (Fig. 7C(c) and (d)). According to the results of 21 days of treatment on a third-degree burn rat model, the composite nanofiber dressing had a significant effect on promoting burn wound healing. The results indicate that composite dressings exhibit superior wound healing potential compared to conventional dressings in many aspects.
4.2.6 Polylactic acid. Polylactic acid (PLA) is a polyester polymer obtained by polymerizing lactic acid as the main raw material, which has good biocompatibility and biodegradability and shows great potential in tissue engineering and drug delivery.161 Liu et al. prepared degradable nanofiber dressings loaded with astragaloside IV using chitosan and polylactic acid.134 Since polylactic acid possesses certain hydrophobicity, it can be combined with hydrophilic chitosan, enabling the nanofiber dressings to resist the intrusion of foreign aqueous solutions while slowly absorbing wound exudate. However, the antimicrobial activity of PLA is poor, and the antimicrobial activity of the prepared dressings mainly comes from the synergistic effect of astragaloside IV and chitosan. The results showed that the wound dressing they prepared had satisfactory antibacterial and wound healing promotion effects, and was a safe and effective wound dressing.Polymers are always playing an important role in developing all kinds of medicated materials.162–164 Electrospinning initially was just a method for converting polymeric solution into solid nanofibers. Along with the fast developments of electrospinning techniques and the polymer sciences, more and more polymers will be introduced into the applications of burn dressings in the form of electrospun fibrous mats.165–170
5 Drugs loaded in electrospun nanofiber burn dressings
In order to enhance the performance of nanofiber burn dressings, various therapeutic and antimicrobial agents are incorporated into the nanofibers, including plant extracts, small molecule drugs, and nanoparticles. Table 2 Classification of nanofiber-loaded drugs according to their biological effects. These compounds have different functional performances, e.g., accelerating wound healing,171 antibacterial,172 preventing scar formation,126 and multi-function.174,175 They can be loaded into the nanofibers alone, but can also be encapsulated jointly for a synergistic effect.
Table 2 Bioactive compounds loaded on electrospun nanofiber dressings
Biological effect |
Bioactive compounds |
Ref. |
Accelerate wound healing |
Curcumin |
116 |
Ginsenoside Rg1 |
123 |
Kiwi extract |
129 |
Bromelain |
171 |
Antibacterial |
Allicin |
124 |
Berberis lycium extract |
172 |
Gymnema sylvestre extract |
96 |
Silver sulfadiazine |
131 |
Zinc oxide |
173 |
Reduce scar formation |
α-Lactalbumin |
130 |
Ginsenoside Rg3 |
126 |
Multifunction |
Quercetin/rutin |
128 |
Lavender essential oil |
174 |
Astragaloside IV |
175 |
5.1 Accelerate wound healing
5.1.1. Curcumin. Turmeric is a perennial herb widely used in Asia. Curcumin is the main bioactive component of turmeric, which has many properties, such as anti-inflammation, anti-oxidation and anti-infection.176 Persistent bacterial infection in the process of wound healing will cause long-term chronic inflammation, which will seriously affect the speed of wound healing. Curcumin, a natural active ingredient, can reduce wound inflammation by decreasing pro-inflammatory factors expression.177 Using PCL/CS as shell, zein (ZE) and curcumin (CUR) as core, Liu et al. prepared PCL/CS graft copolymer-zein-curcumin (PCL/CS-ZE-CUR) electrospun nanofibers.116 In the antibacterial experiment, the total infection rate of the traditional dressing group decreased by 13.8%, while the new nanofiber dressing group decreased by 78.2%, which proved the antibacterial activity of the new nanofiber dressing. Judging from the healing of the burn wound, PCL/CS-ZE-CUR electrospun nanofiber dressing had a better therapeutic effect than traditional dressing. Curcumin's excellent ability of anti-inflammation and scavenging oxygen free radicals can accelerate wound healing and is expected to provide beneficial improvements for wound management.
5.1.2. Ginsenoside Rg1. Ginsenoside Rg1 is a kind of protopanaxatriol saponin extracted from traditional Chinese medicine Ginseng, which has anti-inflammatory, antioxidant, and neuroprotective activities.178,179 Zhou et al. produced a double-layer structure electrospun asymmetric fibre membrane containing ginsenoside Rg1.123 In vitro, cytotoxicity tests showed that ginsenoside Rg1 could promote the migration of human umbilical vein endothelial cells and the formation of capillaries (Fig. 8A(b)). The experimental results in vivo showed that the fibre membrane could regulate inflammatory factors and growth factors, and it also achieved anti-inflammation and promoted collagen deposition (Fig. 8A(d)). The prepared asymmetric wettable membrane inhibits pro-inflammatory factors by enhancing the expression of anti-inflammatory factors, promotes macrophage M2 polarization, and inhibits macrophage M1 polarization. This also explains why the wound dressing they prepared can accelerate wound healing (Fig. 8A(c)).
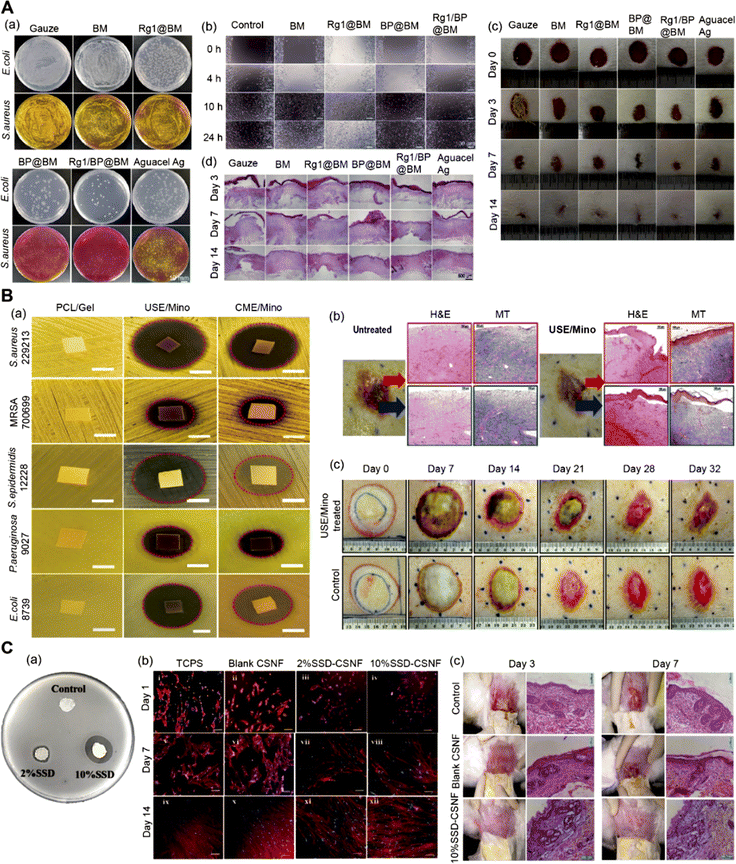 |
| Fig. 8 (A) Multifunctional nanofiber membranes containing black phosphorus/Rg1: (a) Escherichia coli and Staphylococcus aureus colonies remaining in the wound area on the third day; (b) image of umbilical vein endothelial cells in vitro wound healing experiment; (c) pictures of wounds treated with different materials on days 0, 3, 7 and 14; (d) H&E staining of wound tissues. Adapted from ref. 123 with permission from Wiley, copyright 2022. (B) Core–shell structured anti-microbial nanofiber dressings (a) disk diffusion diagram of antibacterial activity of different core–shell nanofibers; (b) histological images of wounds untreated and treated with nanofiber dressings; (c) comparative images of wound treatment results at different time intervals. Adapted from ref. 96 with permission from ACS, copyright 2021. (C) Application of core–shell nanofibers in burn treatment: (a) antibacterial effect of different core–shell nanofibers; (b) fluorescence microscope image of human dermal fibroblasts cultured on core–shell nanofibers; (c) histological sections of different groups on the 3rd and 7th day. Adapted from ref. 131 with permission from Elsevier, copyright 2022. | |
5.1.3. Kiwi extract. Kiwi extract (KE) contains a variety of bioactive components, such as phenols, vitamins C and E, which are considered to have anti-aging, anti-inflammatory, and antioxidant effects.180 Bayat et al. prepared nanofibers containing polycaprolactone, cellulose acetate (CA), and kiwifruit extract (PCL/CA/KE).129 PCL/CA/KE nanofibers have 30% higher water absorption than PCL/CA. The results of cell experiments in vitro showed that PCL/CA/KE nanofibers had no cytotoxicity and effectively promoted the proliferation and attachment of fibroblasts. The authors used a mouse burn model to test the ability of nanofiber membranes to promote wound healing. After 21 days, the ability of PCL/CA/KE to promote burn wound healing was significantly higher than that in the control group. The completion of the epithelialization process and the significant reduction of inflammatory cells better prove that PCL/CA/KE nanofiber is an effective dressing to promote burn wound healing.5.1.4 Bromelain.Pineapple is a tropical fruit loved by people around the world. Bromelain, which is extracted from the stems, leaves, and fruit of the pineapple plant, also has great appeal. Research shows that bromelain has anti-inflammatory, antioxidant, immunomodulatory, cardioprotective, and anti-cancer properties.181 In addition, bromelain is widely used in the food industry, cosmetics, and pharmaceutical industries due to its unique pharmacological and chemical properties.182 Hasannasab et al. combined the therapeutic properties of bromelain and ZnO nanoparticles with the structural properties of silk fibroin to prepare a wound dressing with antibacterial properties.171 The results of antibacterial experiments showed that bromelain did not affect the antibacterial activity of excipients, and it was ZnO nanoparticles that made the dressing have antibacterial activity. However, compared with pure silk fibroin nanofibers, bromelain can accelerate the removal of necrotic tissue to promote wound re-epithelialization.
5.2 Antibacterial
5.2.1 Allicin. Allicin is a thiosulfinate extracted from garlic. Allicin has many properties that are beneficial to human health, such as antibacterial, cholesterol-lowering, blood pressure-lowering, and anti-tumour properties.183 Some studies have shown that allicin can redox with mercaptan groups in protein and glutathione, which is crucial for the biological activity of allicin.184 All kinds of bacteria, fungi, and cells can be inhibited or killed by adjusting the concentration of allicin. Mollaghadimi added allicin to PCL and SF to make nanofiber wound dressings.124 The effect of allicin is to expand the antibacterial properties of nanofibers. In the antibacterial experiment, the diameter of the inhibitory zone of bacterial growth was measured. Compared with the sample without allicin, the inhibition band of the nanofiber containing allicin increased to more than 3 mm, showing a good antibacterial effect.
5.2.2 Berberis lycium extract. Berberis lycium is rich in phenols, alkaloids, and other bioactive compounds, which have anti-inflammatory, antibacterial, and antioxidant effects.185 Khan reported a polyvinyl alcohol/montmorillonite electrospun nanofiber containing root extract of Berberis lycium.172 The results showed that the nanofibers containing 0.8 g of extract showed the highest antibacterial activity. And the inhibitory effect on Pseudomonas aeruginosa was better than that of Staphylococcus aureus. Compared with the control group, the wound treated with nanofibers with extract showed the best healing effect and reduced inflammation. Because the extract has anti-inflammatory and antibacterial activity, it quickly enters the stage of cell proliferation after a short period of inflammation.
5.2.3 Gymnema sylvestre extract. Gymnema sylvestre is a slow-growing medicinal woody plant with effective anti-obesity and anti-diabetes activities.186 The extract also has the properties of anti-bacterial, anti-inflammatory, anti-high cholesterol, and anti-sugar. Ramalingam et al. prepared coaxial nanofibers in which the core layer was loaded with G. sylvestre extract and the shell layer was loaded with minocycline hydrochloride.96 The joint action of the extract and minocycline hydrochloride enhances the permeability of the bacterial cell wall, so it shows an obvious synergistic effect on Gram-positive bacteria (Fig. 8B(a)). The prepared core–shell nanofiber mats effectively promoted wound healing compared with the untreated control group (Fig. 8B(b) and (c)).
5.2.4 Silver sulfadiazine. The silver ions released from silver sulfadiazine are an effective antibacterial agent that can eliminate a variety of bacteria including Gram-positive and Gram-negative bacteria. Dressings containing silver sulfadiazine are often chosen for burn wound management.187 Singh et al. prepared core–shell nanofibers loaded with silver sulfadiazine using PEO and PCL.131 When the concentration of silver sulfadiazine is greater than 5 μg ml−1, bacterial growth will be completely inhibited (Fig. 8C(a)). The results showed that the core–shell nanofibers loaded with silver sulfadiazine had higher cell proliferation ability and no toxicity to cell growth (Fig. 8C(b)). Compared with the control group, there was no obvious inflammation in the wound images of the nanofiber group (Fig. 8C(c)).
5.2.5 Zinc oxide. The tremendous development of nanotechnology has led to a wide range of applications of metal and its oxide nanoparticles in the fields of biology, medicine, chemistry and environment.188 Among the many metal and its oxide nanoparticles, zinc oxide nanoparticles have attracted attention for their many distinctive properties. In the biomedical field, zinc oxide nanoparticles exhibit excellent anticancer, antibacterial, and antioxidant properties, as well as the potential to promote wound healing. 189 A hyaluronic acid-silk fibroin core–shell nanofiber dressing with ZnO encapsulated in the core layer was designed for burn treatment by Hadisi et al.170 The results showed that the addition of ZnO improved the antimicrobial properties of the nanofiber dressing, which inhibited both Escherichia coli and Staphylococcus aureus, with an antimicrobial effect of more than 14 days. Although high concentration of ZnO will have better antibacterial effect, it will also be toxic to the cells. In vitro and in vivo studies have shown that 3 wt% ZnO promotes cell proliferation, collagen deposition, and effectively improves burn wound healing. ZnO nanoparticles possess significant antimicrobial properties and are not prone to the problem of bacterial resistance, and thus can be used as a viable alternative to antibiotics.
5.3 Reduce scar formation
5.3.1 α-Lactalbumin. Alpha-lactalbumin (ALA) is an acidic small molecule globular protein found in mammalian whey.190 Its main function is to promote the synthesis of lactose in the mammary gland. The abundant tryptophan in α-lactalbumin is an important precursor for the synthesis of the neurotransmitter serotonin. Serotonin can promote the proliferation and migration of fibroblasts and keratinocytes, thereby promoting wound healing.191 Guo et al. explored the role of ALA in accelerating burn wound healing and reducing scar formation by synthesizing electrospun nanofiber pads of α-lactalbumin and polycaprolactone.130 The results showed that the wound treated with ALA/PCL dressing showed higher collagen deposition and collagen fibres were more mature than other groups. Studies have shown that mature collagen fibres can promote extracellular matrix reconstruction and skin tissue growth.192 Compared with the control group, myofibroblasts were significantly reduced in the ALA/PCL nanofiber mat group. In addition, on day 12 after treatment, collagen I had a higher expression level than collagen III. All of the above results can prove that ALA/PCL nanofiber pads can significantly promote wound healing and reduce scars.
5.3.2 Ginsenoside Rg3. 20(R)-ginsenoside Rg3 is a tetracyclic triterpene saponin present in red ginseng and has a wide range of pharmacological activities, such as cardiovascular regulation, antioxidant and anti-cancer.193 An experiment by Tang et al. showed that ginsenoside Rg3 could significantly reduce the expression of interleukin-6 (IL-6), connective tissue growth factor (CTGF), tumour necrosis factor α (α-TNF) and α-smooth muscle actin (α-SMA), and enhance the expression of anti-fibrosis genes such as transforming growth factor-β (TGF-β).194 Thus, reducing the production of collagen and the accumulation of extracellular matrix. Guo et al. prepared composite nanofibers blended with polyurethane and marine polysaccharides loaded with ginsenoside Rg3 and tested their ability to inhibit scar formation.126 The results confirmed that the nanofiber dressing loaded with ginsenoside Rg3 can effectively adjust the ratio of type I collagen and type III collagen to inhibit the formation of wound scars. The composite nanofiber dressing has great advantages in promoting burn skin healing and inhibiting scar formation.
5.4 Multifunction
5.4.1 Quercetin/rutin. Quercetin is a bioactive substance produced by the hydrolysis of rutin, and it is also the most studied flavonoid. Studies have shown that quercetin/rutin can remove oxidizing substances produced by inflammation and promote wound healing.195,196 Zhou et al. reported a new nanofiber membrane composed of polycaprolactone, chitosan oligosaccharide, and quercetin/rutin.128 The antioxidant experiment showed that quercetin/rutin had a certain oxygen-free radical scavenging ability. The antibacterial activity of nanofiber membrane was studied with Staphylococcus aureus and Escherichia coli. The results showed that the inhibitory effect of the nanofiber membrane on Staphylococcus aureus was better than that of Escherichia coli. This result may be due to the unique outer membrane structure of E. coli that blocks the infiltration of external drug molecules. All in all, this new type of nanofiber membrane has good antibacterial and antioxidant activity but also has a certain hydrophilic ability, so it is an ideal burn dressing.
5.4.2 Lavender essential oil. Lavender has a long history of use, and its essential oil shows excellent pharmacological properties, which has attracted special attention.197 Lavender essential oil is considered to be one of the most commonly used over-the-counter herbs for the treatment of mental disorders, anxiety, and depression.198 Studies have shown that lavender essential oil can enhance the activity of proteins involved in wound tissue remodelling and accelerate wound contraction.199 Sofi et al. prepared polyurethane electrospun nanofibers loaded with lavender essential oil and silver nanoparticles simultaneously.174 Lavender essential oil improves the hydrophilicity of polyurethane rice fibre, provides an environment similar to the extracellular matrix for cell proliferation, and improves the vitality of fibroblasts. The results of antibacterial activity showed that lavender essential oil and silver nanoparticles had significant synergistic antibacterial effects. All in all, lavender essential oil has potential therapeutic value for burn wound healing, and it has great potential in the preparation of wound dressings.
5.4.3 Astragaloside IV. Astragaloside IV is one of the important bioactive substances in the traditional Chinese medicine plant Astragalus membranaceus, which has been proven to have the properties of anti-inflammation, anti-oxidation, anti-cancer, anti-hypertension, and anti-fibrosis.200,201 Astragaloside IV can inhibit oxidative stress and extracellular matrix deposition.202 During the wound healing process, Astragaloside IV promotes wound healing and reduces scar formation by reducing collagen deposition and reducing the expression of inflammation. Zhang et al. prepared silk fibroin/gelatin electrospun nanofibers loaded with Astragaloside IV and tested their effect on wound treatment.175 The results showed that the ratio of I/III collagen in the nanofiber dressing group loaded with Astragaloside IV was close to that of normal skin, which decreased the expression level of α-smooth muscle actin and inhibited scar formation. Silk fibroin/gelatin nanofiber dressings loaded with Astragaloside also accelerate wound healing by promoting angiogenesis, increasing the number of wound macrophages, and improving local anti-inflammation.
6 Conclusions and perspectives
Burns, as an uncontrollable accidental injury, seriously affect the physical and mental health of patients. Nanofiber dressings are often used for burn wound management. Electrospinning is widely used in the preparation of nanofibers due to its simple operation and low cost. Electrospun nanofibers show great potential in promoting wound healing due to their unique structure and physicochemical properties. First of all, this review introduces the common types of electrospun nanofibers. With the continuous improvement of electrospinning technology, there are blending electrospinning, emulsion electrospinning, coaxial electrospinning, side-by-side electrospinning, and triaxial electrospinning. Among them, the nanofibers with core–shell structures can control the rate of drug release and achieve long-term continuous drug delivery. Secondly, the polymers used for electrospinning nanofibers are reviewed, including natural polymers and synthetic polymers. Natural polymers are generally extracted from plants or animals, which have good biocompatibility and similar characteristics to natural tissue matrix, showing the potential to promote skin regeneration. However, the film-forming properties of natural polymers are poor and need to be improved by blending with other polymers. Researchers usually choose synthetic polymers with strong mechanical properties and natural polymers to improve the overall properties of electrospun nanofibers. Finally, the bioactive substances wrapped in nanofiber dressings are introduced in this paper. Nanofibers have biological activity by adding antibiotics, natural active substances, and nanoparticles, which can prevent bacterial infection and accelerate wound healing at the same time.
There are still many challenges in the application of electrospun nanofibers in burn wound dressings. Microbial infection and persistent inflammation will affect the speed of wound healing. Considering the complexity of the burn wound healing process, it is very important to choose a suitable dressing. At present, people are not only paying attention to the antibacterial activity of nanofiber dressings and their ability to accelerate wound healing but also whether the dressings can inhibit scarring. Researchers try to use a variety of materials to make nanofiber dressings have more functions, but it still requires a large number of clinical trials to prove the effectiveness and safety of nanofiber dressings. The application of nanofibers in biomedicine is expanding, and it also shows a great prospect in wound management. This review provides some reference for the application of nanofiber dressing in clinical burn treatment. In the future, it is necessary to further improve the preparation methods of nanofibers and find more bioactive substances to improve the therapeutic effect and reduce the cost. As a special applied field, wound dressing is able to always rely on the appearances of new electrospinning techniques,202–206 and also its combinations with electrospraying and other traditional chemical and physical methods.207–217
Author contributions
Conceptualization, S. Z., W. Y. and D.-G. Y.; methodology, S. Z., W. Y., W. G., Y. L. and D.-G. Y.; writing—original draft preparation, S. Z. and W. Y.; writing—review and editing, D.-G. Y. and P. L.; visualization, W. G. and Y. L.; supervision, D.-G. Y. and P. L.; project administration, D.-G. Y. and P. L.; funding acquisition, P. L. All authors have read and agreed to the published version of the manuscript.
Conflicts of interest
The authors declare no conflict of interest.
Acknowledgements
The financial support provided by the following funds is appreciated: the Shanghai Natural Science Foundation (No. 21ZR1459500), the Shanghai Industrial Collaboration Project (HCXBCY-2023-042), the Municipal Commission of Health and Family Planning Foundation of Shanghai (No. 202140413), the Natural Science Foundation of Shandong Province (No. ZR2021MH129), and the Medical Health Science and Technology Innovation Plan of Jinan (No. 202134037).
References
- L. F. Qi, C. L. Zhang, B. Wang, J. B. Yin and S. F. Yan, Macromol. Biosci., 2022, 22, e2100475 CrossRef PubMed.
- Z. Y. Li, F. Zhou, Z. Y. Li, S. Y. Lin, L. Chen, L. X. Liu and Y. M. Chen, ACS Appl. Mater. Interfaces, 2018, 10, 25194–25202 CrossRef CAS.
- A. Gholipour-Kanani, S. H. Bahrami and S. Rabbani, IET Nanobiotechnol., 2016, 10, 1–7 CrossRef.
- M. M. Chen, J. Tian, Y. Liu, H. Cao, R. Li, J. H. Wang, J. L. Wu and Q. Q. Zhang, Chem. Eng. J., 2019, 373, 413–424 CrossRef CAS.
- A. Shpichka, D. Butnaru, E. A. Bezrukov, R. B. Sukhanov, A. Atala, V. Burdukovskii, Y. Y. Zhang and P. Timashev, Stem Cell Res. Ther., 2019, 10, 94 CrossRef CAS.
- B. S. Atiyeh and M. Costagliola, Burns, 2007, 33, 405–413 CrossRef PubMed.
- H. Luze, S. P. Nischwitz, C. Smolle, R. Zrim and L. P. Kamolz, Medicina, 2022, 58, 912 CrossRef PubMed.
- D. Simões, S. P. Miguel, M. P. Ribeiro, P. Coutinho, A. G. Mendonça and I. J. Correia, Eur. J. Pharm. Biopharm., 2018, 127, 130–141 CrossRef.
- Y. P. Liang, J. H. He and B. L. Guo, ACS Nano, 2021, 15, 12687–12722 CrossRef CAS PubMed.
- A. E. Stoica, C. Chircov and A. M. Grumezescu, Molecules, 2020, 25, 2699 CrossRef CAS PubMed.
- Y. X. Yao, A. D. Zhang, C. S. Yuan, X. G. Chen and Y. Liu, Biomater. Sci., 2021, 9, 4523–4540 RSC.
- K. Chen, Y. H. Li, Y. B. Li, Y. F. Tan, Y. S. Liu, W. S. Pan and G. X. Tan, J. Nanobiotechnol., 2023, 21, 237 CrossRef CAS PubMed.
- Y. F. Shi, H. J. Zhang, X. Zhang, Z. Chen, D. Zhao and J. Ma, Regener. Biomater., 2020, 7, 63–70 CrossRef CAS.
- P. I. Sandhya, P. Subramaniyan and V. G. Sujata, Wounds, 2023, 35, 41–46 CrossRef.
- E. A. Kamoun, E. S. Kenawy and X. Chen, J. Adv. Res., 2017, 8, 217–233 CrossRef CAS PubMed.
- U. N. Tak, S. Rashid, P. Kour, N. Nazir, M. I. Zargar and A. A. Dar, Int. J. Biol. Macromol., 2023, 234, 123718 CrossRef CAS.
- Z. Hadisi, J. Nourmohammadi and S. M. Nassiri, Int. J. Biol. Macromol., 2018, 107, 2008–2019 CrossRef CAS.
- X. X. Chen, R. Zhao, X. Wang, X. Li, F. Peng, Z. G. Jin, X. Gao, J. A. Yu and C. Wang, J. Biomater. Sci., Polym. Ed., 2017, 28, 162–176 CrossRef CAS PubMed.
- D. G. Yu and C. Huang, Biomolecules, 2023, 13, 1152 CrossRef CAS PubMed.
- D. Jao and V. Z. Beachley, ACS Macro Lett., 2019, 8, 588–595 CrossRef CAS PubMed.
- K. C. K. Cheng, M. A. Bedolla-Pantoja, Y. K. Kim, J. V. Gregory, F. Xie, A. de France, C. Hussal, K. Sun, N. L. Abbott and J. Lahann, Science, 2018, 362, 804–808 CrossRef CAS PubMed.
- S. G. Zhang, Adv. Cancer Res., 2008, 99, 335–362 CrossRef CAS.
- S. H. Ji and J. S. Yun, Nanomaterials, 2022, 12, 404 CrossRef CAS.
- I. Kohsari, Z. Shariatinia and S. M. Pourmortazavi, Carbohydr. Polym., 2016, 140, 287–298 CrossRef CAS.
- A. Z. Bazmandeh, E. Mirzaei, M. Fadaie, S. Shirian and Y. Ghasemi, Int. J. Biol. Macromol., 2020, 162, 359–373 CrossRef CAS.
- E. Mele, Biotechnol. Bioeng., 2023, 120, 1229–1240 CrossRef CAS PubMed.
- H. B. Wang, Y. Y. Lu, H. S. Yang, D.-G. Yu and X. H. Lu, Front. Mol. Biosci., 2023, 10, 4676 Search PubMed.
- D. G. Yu and L. Xu, Curr. Drug Delivery, 2023, 21, 360–367 CrossRef PubMed.
- A. Sadeghi, M. Zandi, M. P. Modaress and S. Rajabi, Int. J. Biol. Macromol., 2019, 132, 63–75 CrossRef CAS.
- E.-R. Kenawy, M. S. A. El-Moaty, M. Ghoneum, H. M. A. Soliman, A. A. El-Shanshory and S. Shendy, RSC Adv., 2024, 14, 4930–4945 RSC.
- D. J. Patty, A. D. Nugraheni, I. D. Ana, Y. W. Sari and Y. Yusuf, RSC Adv., 2023, 13, 34427–34438 RSC.
- Q. l. Zheng, Y. W. Xi and Y. X. Weng, RSC Adv., 2024, 14, 3359–3378 RSC.
- X. Q. Wang and C. L. Feng, WIREs Nanomed. Nanobi., 2023, 15, e1847 CrossRef CAS.
- A. Schneider, X. Y. Wang, D. L. Kaplan, J. A. Garlick and C. Egles, Acta Biomater., 2009, 5, 2570–2578 CrossRef CAS.
- Y. Q. Liu, T. Y. Li, Y. F. Han, F. J. Li and Y. Liu, Curr. Opin. Biomed. Eng., 2021, 17, 100247 CrossRef CAS.
- H. Duan, H. N. Chen, C. R. Qi, F. M. Lv, J. Wang, Y. C. Liu, Z. P. Liu and Y. Liu, Int. J. Pharm., 2024, 650, 123660 CrossRef CAS PubMed.
- B. F. Finina and A. K. Mersha, RSC Adv., 2024, 14, 5290–5308 RSC.
- J. F. Zhou, L. Z. Wang, W. J. Gong, B. Wang, D. G. Yu and Y. J. Zhu, Biomedicines, 2023, 11, 2146 CrossRef CAS.
- Y. Wang, D. G. Yu, Y. Liu and Y. N. Liu, J. Funct. Biomater., 2022, 13, 289 CrossRef CAS PubMed.
- S. Tabakoglu, D. Kołbuk and P. Sajkiewicz, Biomater. Sci., 2023, 11, 37–61 RSC.
- M. G. Jeschke, M. E. van Baar, M. A. Choudhry, K. K. Chung, N. S. Gibran and S. Logsetty, Nat. Rev. Dis. Primers, 2020, 6, 11 CrossRef PubMed.
- M. Burgess, F. Valdera, D. Varon, E. Kankuri and K. Nuutila, Cells, 2022, 11, 3073 CrossRef CAS PubMed.
- C. Johnson, Surgery, 2018, 36, 435–440 Search PubMed.
- R. M. Johnson and R. Richard, Adv. Skin Wound Care, 2003, 16, 178–187 CrossRef.
- A. Markiewicz-Gospodarek, M. Kozioł, M. Tobiasz, J. Baj, E. Radzikowska-Büchner and A. Przekora, Int. J. Environ.
Res. Public Health, 2022, 19, 3073 CrossRef.
- S. Tejiram and J. W. Shupp, Surgery, 2024, 175, 1259–1261 CrossRef.
- D. G. Greenhalgh, N. Engl. J. Med., 2019, 380, 2349–2359 CrossRef.
- P. E. Wischmeyer, Nutr. Clin. Pract., 2019, 34, 681–687 CrossRef.
- L. H. Evers, D. Bhavsar and P. Mailänder, Exp. Dermatol., 2010, 19, 777–783 CrossRef PubMed.
- B. K. Sun, Z. Siprashvili and P. A. Khavari, Science, 2014, 346, 941–945 CrossRef CAS.
- A. N. Dehkordi, F. M. Babaheydari, M. Chehelgerdi and S. R. Dehkordi, Stem Cell Res. Ther., 2019, 10, 111 CrossRef CAS.
- P. Rousselle, M. Montmasson and C. Garnier, Matrix Biol., 2019, 75, 12–26 CrossRef PubMed.
- H. J. Park, Y. L. Zhang, S. P. Georgescu, K. L. Johnson, D. Kong and J. B. Galper, Stem Cell Rev., 2006, 2, 93–102 CrossRef CAS PubMed.
- A. E. Ayadi, J. W. Jay and A. Prasai, Int. J. Mol. Sci., 2020, 21, 1105 CrossRef PubMed.
- N. Akombaetwa, A. Bwanga, P. A. Makoni and B. A. Witika, Polymers, 2022, 14, 2931 CrossRef CAS PubMed.
- Y. Liang, Y. Liang, H. Zhang and B. Guo, Asian J. Pharm. Sci., 2022, 17, 353–384 CrossRef PubMed.
- K. Vig, A. Chaudhari, S. Tripathi, S. Dixit, R. Sahu, S. Pillai, V. A. Dennis and S. R. Singh, Int. J. Mol. Sci., 2017, 18, 789 CrossRef.
- M. Roopesh, D. Davis, M. S. Jyothi, M. Vandana, B. S. Thippeswamy, G. Hegde, T. P. Vinod and R. S. Keri, RSC Adv., 2023, 13, 24320–24330 RSC.
- D.-G. Yu and J. F. Zhou, J. Pharm. Sci., 2023, 112, 2719–2723 CrossRef CAS.
- E. B. Abdelazim, T. Abed, S. S. Goher, S. H. Alya, H. A. S. El-Nashar, S. H. El-Moslamy, E. M. El-Fakharany, E. A. Abdul-Baki, M. M. Shakweer, N. G. Eissa, M. Elsabahy and E. A. Kamoun, RSC Adv., 2024, 14, 101–117 RSC.
- D.-G. Yu and J. F. Zhou, Next Mater., 2024, 2, 100119 CrossRef.
- L. T. Yao, C. P. Sun, H. Lin, G. S. Li, Z. C. Lian, R. X. Song, S. L. Zhuang and D. W. Zhang, J. Mater. Sci. Technol., 2023, 150, 114–123 CrossRef CAS.
- A. Kim, J. K. Dash and R. Patel, Membranes, 2023, 13, 183 CrossRef CAS PubMed.
- X. Y. Cao, W. Chen, P. Zhao, Y. Y. Yang and D. G. Yu, Polymers, 2022, 14, 3990 CrossRef CAS PubMed.
- M. Rahmati, D. K. Mills, A. M. Urbanska, M. R. Saeb, J. R. Venugopal, S. Ramakrishna and M. Mozafari, Prog. Mater. Sci., 2021, 117, 100721 CrossRef CAS.
- E. Ahmed, B. S. Lalia and R. Hashaikeh, Desalination, 2015, 356, 15–30 CrossRef.
- R. Augustine, S. R. U. Rehman, R. Ahmed, A. A. Zahid, M. Sharifi, M. Falahati and A. Hasan, Int. J. Biol. Macromol., 2020, 156, 153–170 CrossRef CAS PubMed.
- M. G. Lin, J. L. Shen, B. B. Wang, Y. G. Chen, C. Y. Zhang and H. Qi, RSC Adv., 2023, 13, 30680–30689 RSC.
- M. Sivan, D. Madheswaran, J. Valtera, E. K. Kostakova and D. Lukas, Mater. Des., 2022, 213, 110308 CrossRef CAS.
- M. Sivan, D. Madheswaran, S. Hauzerova, V. Novotny, V. Hedvicakova, V. Jencova, E. K. Kostakova, M. Schindler and D. Lukas, Mater. Today Chem., 2022, 26, 101025 CrossRef CAS.
- J. K. Patra, G. Das, L. F. Fraceto, E. V. R. Campos, M. D. P. Rodriguez-Torres, L. S. Acosta-Torres, L. A. Diaz-Torres, R. Grillo, M. K. Swamy, S. Sharma, S. Habtemariam and H. S. Shin, J. Nanobiotechnol., 2018, 16, 71 CrossRef.
- Y. Z. Wang, H. Kang, J. Hu, H. M. Chen, H. M. Zhou, Y. Wang and H. Z. Ke, RSC Adv., 2023, 13, 21633–21642 RSC.
- M. L. Wang, X. W. Huang, H. X. Zheng, Y. M. Tang, K. Zeng, L. Q. Shao and L. Li, J. Controlled Release, 2021, 337, 236–247 CrossRef CAS PubMed.
- C. G. Qian, Y. B. Liu, S. Chen, C. Y. Zhang, X. H. Chen, Y. H. Liu and P. Liu, Front. Bioeng. Biotechnol., 2023, 11, 5252 Search PubMed.
- S. Nagam Hanumantharao and S. Rao, Fibers, 2019, 7, 66 CrossRef.
- X. J. Chen, S. Yan, S. S. Wen, J. J. Chen, J. Q. Xu, C. Wang and X. F. Lu, J. Colloid Interface Sci., 2023, 641, 782–790 CrossRef CAS PubMed.
- W. Gao, L. Sun, X. Fu, Z. Lin, W. Xie, W. Zhang, F. Zhao and X. Chen, J. Mater. Chem. B, 2018, 6, 277–288 RSC.
- L. J. del Valle, L. Franco, R. Katsarava and J. Puiggalí, AIMS Mol. Sci., 2016, 3, 52–87 CAS.
- J. Hu, H.-Y. Li, G. R. Williams, H.-H. Yang, L. Tao and L.-M. Zhu, J. Pharm. Sci., 2016, 105, 1104–1112 CrossRef CAS PubMed.
- A. Bhattacharjee, K. Kumar, A. Arora and D. S. Katti, Mater. Sci. Eng. C, 2016, 63, 266–273 CrossRef CAS.
- J. Jalvandi, M. White, Y. Gao, Y. B. Truong, R. Padhye and I. L. Kyratzis, Mater. Sci. Eng., 2017, 73, 440–446 CrossRef CAS PubMed.
- L. Bardoňová, A. Kotzianová, K. Skuhrovcová, O. Židek, H. Vágnerová, J. Kulhánek, T. Hanová, M. Knor, J. Starigazdová, K. Mamulová Kutláková and V. Velebný, Int. J. Biol. Macromol., 2022, 194, 726–735 CrossRef PubMed.
- S. X. Kang, S. C. Hou, X. W. Chen, D. G. Yu, L. Wang, X. Y. Li and G. R. Williams, Polymers, 2020, 12, 2421 CrossRef CAS PubMed.
- M. M. Abdul Hameed, S. A. P. Mohamed Khan, B. M. Thamer, A. M. Al-Enizi, A. Aldalbahi, H. El-Hamshary and M. H. El-Newehy, J. Macromol. Sci. A, 2020, 58, 130–144 CrossRef.
- M. Buzgo, A. Mickova, M. Rampichova and M. Doupnik, in Core–Shell Nanostructures for Drug Delivery and Theranostics, ed. M. L. Focarete and A. Tampieri, Woodhead Publishing, 2018, pp. 325–347, DOI:10.1016/B978-0-08-102198-9.00011-9.
- X. Q. Li, Y. Su, S. P. Liu, L. J. Tan, X. M. Mo and S. Ramakrishna, Colloids Surf., B, 2010, 75, 418–424 CrossRef CAS PubMed.
- S. Agarwal and A. Greiner, Polym. Adv. Technol., 2011, 22, 372–378 CrossRef CAS.
- T. Jiang, D. Wang, X. Zhang, Q. Yang, Q. Huang, X. Ju, L. Li, X. Kang and C. Li, Mater. Chem. Phys., 2024, 311, 128561 CrossRef CAS.
- Y. R. Lv, Y. F. Han, Z. G. Yu, J. Chen, C. X. Li, C. Wang, P. Hu and Y. Liu, Prog. Biomater., 2022, 11, 253–261 CrossRef CAS PubMed.
- D. Han and A. J. Steckl, Chempluschem, 2019, 84, 1453–1497 CrossRef CAS PubMed.
- L. Xu, H. He, Y. T. Du, S. W. Zhang, D.-G. Yu and P. Liu, Pharmaceutics, 2023, 15, 2314 CrossRef CAS PubMed.
- A. K. Moghe and B. S. Gupta, Polym. Rev. (Philadelphia, PA, U. S.), 2008, 48, 353–377 CrossRef CAS.
- X. Y. Huang, W. L. Jiang, J. F. Zhou, D. G. Yu and H. Liu, Polymers, 2022, 14, 4947 CrossRef CAS PubMed.
- Y. T. Du, Z. L. Yang, S. X. Kang, D. G. Yu, X. R. Chen and J. Shao, Sensors, 2023, 23, 3685 CrossRef CAS PubMed.
- J. Guo, T. Wang, Z. Yan, D. Ji, J. Li and H. Pan, Int. J. Pharm., 2022, 629, 122410 CrossRef CAS PubMed.
- R. Ramalingam, C. Dhand, V. Mayandi, C. M. Leung, H. Ezhilarasu, S. K. Karuppannan, P. Prasannan, S. T. Ong, N. Sunderasan, I. Kaliappan, M. Kamruddin, V. A. Barathi, N. K. Verma, S. Ramakrishna, R. Lakshminarayanan and K. D. Arunachalam, ACS Appl. Mater. Interfaces, 2021, 13, 24356–24369 CrossRef CAS PubMed.
- H. Li, C. G. Zhao, Z. X. Wang, H. Zhang, X. Y. Yuan and D. Kong, J. Biomater. Sci., Polym. Ed., 2010, 21, 803–819 CrossRef CAS PubMed.
- Y. X. Ji, H. Zhao, H. Liu, P. Zhao and D.-G. Yu, Gels, 2023, 9, 700 CrossRef CAS PubMed.
- W. B. Chen, Z. J. Ma, X. Z. Pan, Z. L. Hu, G. P. Dong, S. F. Zhou, M. Y. Peng, J. R. Qiu and P. Gouma, J. Am. Ceram. Soc., 2014, 97, 1944–1951 CrossRef CAS.
- J. J. Li, Q. Du, J. G. Wan, D.-G. Yu, F. Tan and X. L. Yang, Mater. Des., 2024, 238, 112657 CrossRef CAS.
- J. Yang, K. Wang, D.-G. Yu, Y. Yang, S. W. A. Bligh and G. R. Williams, Mater. Sci. Eng., C, 2020, 111, 110805 CrossRef CAS PubMed.
- Y. Shi, M. Zhou, S. Zhao, H. Li, W. Wang, J. Cheng, L. Jin and Y. Wang, Mater. Des., 2023, 227, 111778 CrossRef CAS.
- Z. B. Feng, Z. Q. Zhao, Y. A. Liu, Y. K. Liu, X. Y. Cao, D.-G. Yu and K. Wang, Adv. Mater. Technol., 2023, 8, 2300021 CrossRef CAS.
- Y. Y. Yang, W. Chen, M. L. Wang, J. C. Shen, Z. Tang, Y. M. Qin and D. G. Yu, Polymers, 2023, 15, 2237 CrossRef CAS PubMed.
- H. Lv, Y. Y. Liu, P. Zhao, Y. B. Bai, W. X. Cui, S. L. Shen, Y. Liu, Z. Wang and D.-G. Yu, Appl. Catal., B, 2023, 330, 122623 CrossRef CAS.
- W. L. Song, Y. X. Tang, C. Qian, B. J. Kim, Y. Z. Liao and D.-G. Yu, Innovation, 2023, 4, 100381 CAS.
- Y. B. Bai, Y. A. Liu, H. Lv, H. P. Shi, W. Zhou, Y. Liu and D.-G. Yu, Polymers, 2022, 14, 4311 CrossRef CAS PubMed.
- E. J. Torres-Martinez, J. M. Cornejo Bravo, A. Serrano Medina, G. L. Pérez González and L. J. Villarreal Gómez, Curr. Drug Delivery, 2018, 15, 1360–1374 CrossRef CAS PubMed.
- M. L. Wang, J. S. Hou, D.-G. Yu, S. Y. Li, J. W. Zhu and Z. Z. Chen, J. Alloys Compd., 2020, 846, 156471 CrossRef CAS.
- Y. Liu, X. Chen, Y. Gao, Y. Liu, D. G. Yu and P. Liu, Biomolecules, 2022, 12, 1057 CrossRef CAS PubMed.
- K. Ghosal, R. Augustine, A. Zaszczynska, M. Barman, A. Jain, A. Hasan, N. Kalarikkal, P. Sajkiewicz and S. Thomas, React. Funct. Polym., 2021, 163, 104895 CrossRef CAS.
- A. T. Prabhu, V. Baliga, S. Bhat, S. T. Thenkondar, Y. Nayak and U. Y. Nayak, Pharmaceutics, 2023, 15, 1560 CrossRef PubMed.
- Y. Liu, S. Y. Zhou, Y. L. Gao and Y. L. Zhai, Asian J. Pharm. Sci., 2019, 14, 130–143 CrossRef PubMed.
- S. Homaeigohar and A. R. Boccaccini, Acta Biomater., 2020, 107, 25–49 CrossRef CAS PubMed.
- A. S. Shabunin, V. E. Yudin, I. P. Dobrovolskaya, E. V. Zinovyev, V. Zubov, E. M. Ivan'kova and P. Morganti, Cosmetics, 2019, 6, 16 CrossRef CAS.
- Q. Liu, L. P. Yang and Q. R. Peng, Mater. Express, 2021, 11, 1420–1437 CrossRef CAS.
- M. Mirhaj, M. Tavakoli, J. Varshosaz, S. Labbaf, S. Salehi, A. Talebi, N. Kazemi, V. Haghighi and M. Alizadeh, Carbohydr. Polym., 2022, 292, 119648 CrossRef CAS PubMed.
- S. Khosravimelal, M. Chizari, B. Farhadihosseinabadi, M. Moosazadeh Moghaddam and M. Gholipourmalekabadi, J. Mater. Sci.: Mater. Med., 2021, 32, 114 CrossRef CAS PubMed.
- M. S. Morais, D. P. F. Bonfim, M. L. Aguiar and W. P. Oliveira, J. Pharm. Innovation, 2022, 1–15, DOI:10.1007/s12247-022-09681-7.
- S. Abid, T. Hussain, A. Nazir, A. Zahir, S. Ramakrishna, M. Hameed and N. Khenoussi, Int. J. Biol. Macromol., 2019, 135, 1222–1236 CrossRef CAS PubMed.
- A. Li, L. Li, B. a. Zhao, X. Li, W. Liang, M. Lang, B. Cheng and J. Li, Int. J. Biol. Macromol., 2022, 194, 914–923 CrossRef CAS PubMed.
- C. M. Leung, C. Dhand, V. Mayandi, R. Ramalingam, F. P. Lim, V. A. Barathi, N. Dwivedi, G. Orive, R. W. Beuerman, S. Ramakrishna, Y. C. Toh, X. J. Loh, N. K. Verma, A. W. C. Chua and R. Lakshminarayanan, Biomater. Sci., 2020, 8, 3454–3471 RSC.
- L. M. Zhou, N. N. Liu, L. B. Feng, M. Y. Zhao, P. Wu, Y. F. Chai, J. Liu, P. Zhu and R. Guo, Bioeng. Transl. Med., 2022, 7, e10274 CrossRef CAS PubMed.
- B. Mollaghadimi, IET Nanobiotechnol., 2022, 16, 239–249 CrossRef PubMed.
- A. Yerra and M. M. Dadala, J. Appl. Polym. Sci., 2021, 139, 51930 CrossRef.
- X. Guo, F. F. Xiu, H. Bera, Y. F. Abbasi, Y. Chen, L. W. Si, P. X. Liu, C. W. Zhao, X. Tang, Y. Feng, D. M. Cun, X. Zhao and M. S. Yang, Carbohydr. Polym., 2023, 317, 121085 CrossRef CAS PubMed.
- Y. F. Huang, L. Wang, L. Yi, B. J. Xin and T. X. Li, J. Text. Inst., 2024, 115, 208–217 CrossRef CAS.
- L. Z. Zhou, L. Cai, H. J. Ruan, L. Zhang, J. Wang, H. J. Jiang, Y. Wu, S. W. Feng and J. Chen, Int. J. Biol. Macromol., 2021, 183, 1145–1154 CrossRef CAS PubMed.
- G. Bayat, M. Fallah-Darrehchi, P. Zahedi, A. B. Moghaddam, P. Ghaffari-Bohlouli and H. Jafari, J. Biomater. Sci., Polym. Ed., 2023, 34, 72–88 CrossRef CAS PubMed.
- X. Guo, Y. N. Liu, H. Bera, H. T. Zhang, Y. Chen, D. M. Cun, V. Fodera and M. S. Yang, ACS Appl. Mater. Interfaces, 2020, 12, 45702–45713 CrossRef CAS PubMed.
- R. Singh, P. Roopmani, M. Chauhan, S. M. Basu, W. Deeksha, M. D. Kazem, S. Hazra, E. Rajakumara and J. Giri, Int. J. Pharm., 2022, 613, 121358 CrossRef CAS PubMed.
- S. Khataei, M. H. Al-Musawi, K. Asadi, S. Ramezani, M. Abbasian and M. Ghorbani, J. Drug Delivery Sci. Technol., 2023, 82, 104310 CrossRef CAS.
- S. Ramkumar, A. S. Nivetha, S. Saravanan, R. Harchana, B. Sathyasri and N. Sammeta, J. Mater. Res., 2022, 37, 4360–4367 CrossRef CAS.
- Z. Liu, Y. Lv, G. Zheng, W. Wu and X. Che, AAPS PharmSciTech, 2023, 24, 202 CrossRef CAS PubMed.
- J. Venkatesan and S.-K. Kim, Mar. Drugs, 2010, 8, 2252–2266 CrossRef CAS PubMed.
- P. S. Bakshi, D. Selvakumar, K. Kadirvelu and N. S. Kumar, Int. J. Biol. Macromol., 2020, 150, 1072–1083 CrossRef CAS PubMed.
- S. Peers, A. Montembault and C. Ladavière, J. Controlled Release, 2020, 326, 150–163 CrossRef CAS PubMed.
- W. M. Kedir, G. F. Abdi, M. M. Goro and L. D. Tolesa, Heliyon, 2022, 8, e10196 CrossRef CAS PubMed.
- M. E. Abd El-Hack, M. T. El-Saadony, M. E. Shafi, N. M. Zabermawi, M. Arif, G. E. Batiha, A. F. Khafaga, Y. M. Abd El-Hakim and A. A. Al-Sagheer, Int. J. Biol. Macromol., 2020, 164, 2726–2744 CrossRef CAS PubMed.
- M. Kong, X. G. Chen, K. Xing and H. J. Park, Int. J. Food Microbiol., 2010, 144, 51–63 CrossRef CAS PubMed.
- H. P. Shi, S. M. Wang, G. X. Zhang, Y. J. Zhang and A. Barbul, Wound Repair Regen., 2007, 15, 66–70 CrossRef PubMed.
- H. R. El-Seedi, N. S. Said, N. Yosri, H. B. Hawash, D. M. El-Sherif, M. Abouzid, M. M. Abdel-Daim, M. Yaseen, H. Omar, Q. Shou, N. F. Attia, X. Zou, Z. Guo and S. A. M. Khalifa, Heliyon, 2023, 9, e16228 CrossRef CAS PubMed.
- M. C. Gómez-Guillén, B. Giménez, M. E. López-Caballero and M. P. Montero, Food Hydrocolloids, 2011, 25, 1813–1827 CrossRef.
- A. I. Cernencu, G. M. Vlasceanu, A. Serafim, G. Pircalabioru and M. Ionita, RSC Adv., 2023, 13, 24053–24063 RSC.
- B. Salahuddin, S. Wang, D. Sangian, S. Aziz and Q. Gu, ACS Appl. Bio Mater., 2021, 4, 2886–2906 CrossRef CAS PubMed.
- N. Sahoo, R. K. Sahoo, N. Biswas, A. Guha and K. Kuotsu, Int. J. Biol. Macromol., 2015, 81, 317–331 CrossRef CAS PubMed.
- Ł. Mazurek, M. Szudzik, M. Rybka and M. Konop, Biomolecules, 2022, 12, 1852 CrossRef PubMed.
- Z. Y. Zhou, J. Cui, S. L. Wu, Z. Geng and J. C. Su, Theranostics, 2022, 12, 5103–5124 CrossRef CAS PubMed.
- P. Du, X. Chen, Y. Chen, J. Li, Y. C. Lu, X. X. Li, K. Hu, J. F. Chen and G. Z. Lv, Heliyon, 2023, 9, e13506 CrossRef CAS PubMed.
- E. Manaila, G. Craciun and I. C. Calina, Int. J. Mol. Sci., 2022, 24, 104 CrossRef PubMed.
- M. U. A. Khan, S. I. A. Razak, S. Haider, H. A. Mannan, J. Hussain and A. Hasan, Int. J. Biol. Macromol., 2022, 208, 475–485 CrossRef CAS.
- A. A. Shitole, P. Raut, P. Giram, P. Rade, A. Khandwekar, B. Garnaik and N. Sharma, Mater. Sci. Eng., C, 2020, 110, 110731 CrossRef CAS PubMed.
- J. Wang, V. Planz, B. Vukosavljevic and M. Windbergs, Eur. J. Pharm. Biopharm., 2018, 129, 175–183 CrossRef CAS PubMed.
- M. Aslam, M. A. Kalyar and Z. A. Raza, Polym. Eng. Sci., 2018, 58, 2119–2132 CrossRef CAS.
- M. A. Najim, B. I. Khalil and A. A. Hameed, Heliyon, 2022, 8, e11423 CrossRef CAS PubMed.
- Y. H. Shen, X. Yu, J. Cui, F. Yu, M. Y. Liu, Y. J. Chen, J. L. Wu, B. B. Sun and X. M. Mo, Biomolecules, 2022, 12, 1245 CrossRef CAS PubMed.
- C. Gaidău, M. Râpă, L. M. Stefan, E. Matei, A. C. Berbecaru, C. Predescu and L. Mititelu-Tartau, Fibers, 2023, 11, 87 CrossRef.
- F. H. Alshammari, Radiat. Phys. Chem., 2023, 209, 110989 CrossRef CAS.
- J. F. Zhou, Y. L. Dai, J. H. Fu, C. Yan, D. G. Yu and T. Yi, Biomolecules, 2023, 13, 1011 CrossRef CAS PubMed.
- H. Wang, J. X. Zhang, H. Liu, Z. G. Wang, G. W. Li, Q. P. Liu and C. Y. Wang, Int. J. Biol. Macromol., 2023, 253, 126294 CrossRef CAS PubMed.
- B. Mutlu, F. Çiftçi, C. B. Üstündağ and R. Çakır-Koç, Int. J. Biol. Macromol., 2023, 253, 126932 CrossRef CAS PubMed.
- N. Ajalli, M. Pourmadadi, F. Yazdian, M. Abdouss, H. Rashedi and A. Rahdar, Curr. Drug Delivery, 2023, 20, 1569–1583 CrossRef CAS PubMed.
- T. R. Zhang, L. Li, S. Chunta, W. Wu, Z. J. Chen and Y. Lu, J. Controlled Release, 2023, 354, 146–154 CrossRef CAS PubMed.
- F. L. Man, Y. Q. Yang, H. S. He, J. P. Qi, W. Wu and Y. Lu, Mol. Pharm., 2023, 20, 2579–2588 CrossRef CAS PubMed.
- M. D. Köse, N. Ungun and O. Bayraktar, Curr. Drug Delivery, 2022, 19, 547–559 CrossRef PubMed.
- D.-G. Yu and P. Zhao, Biomolecules, 2022, 12, 1234 CrossRef CAS PubMed.
- W. L. Song, Y. Zhang, C. H. Tran, H. K. Choi, D.-G. Yu and I. Kim, Prog. Polym. Sci., 2023, 142, 101691 CrossRef CAS.
- Q. Liao, E. J. Kim, Y. X. Tang, H. L. Xu, D. G. Yu, W. L. Song and B. J. Kim, J. Polym. Sci., 2023, 62, 1517–1535 CrossRef.
- M. L. Wang, R.-L. Ge, F. Y. Zhang, D.-G. Yu, Z.-P. Liu, X. Y. Li, H. Shen and G. R. Williams, Biomater. Adv., 2023, 150, 213404 CrossRef CAS PubMed.
- N. Song, S. Y. Ren, Y. Zhang, C. Wang and X. L. Lu, Adv. Funct. Mater., 2022, 32, 2204751 CrossRef CAS.
- M. Hasannasab, J. Nourmohammadi, M. M. Dehghan and A. Ghaee, Int. J. Pharm., 2021, 610, 121227 CrossRef CAS PubMed.
- A. K. Khan, S. Kaleem, F. Pervaiz, T. A. Sherazi, S. A. Khan, F. A. Khan, T. Jamshaid, M. I. Umar, W. Hassan, M. Ijaz and G. Murtaza, J. Drug Delivery Sci. Technol., 2023, 79, 3987 Search PubMed.
- Z. Hadisi, M. Farokhi, H. R. Bakhsheshi-Rad, M. Jahanshahi, S. Hasanpour, E. Pagan, A. Dolatshahi-Pirouz, Y. S. Zhang, S. C. Kundu and M. Akbari, Macromol. Biosci., 2020, 20, 1900328 CrossRef CAS PubMed.
- H. S. Sofi, T. Akram, A. H. Tamboli, A. Majeed, N. Shabir and F. A. Sheikh, Int. J. Pharm., 2019, 569, 118590 CrossRef CAS PubMed.
- D. P. Zhang, L. J. Li, Y. H. Shan, J. Xiong, Z. J. Hu, Y. Zhang and J. Q. Gao, J. Drug Delivery Sci. Technol., 2019, 52, 272–281 CrossRef CAS.
- M. Prasathkumar and S. Sadhasivam, Int. J. Biol. Macromol., 2021, 186, 656–685 CrossRef CAS PubMed.
- K. Huang, W. B. Liu, W. Y. Wei, Y. A. Zhao, P. Z. Zhuang, X. X. Wang, Y. F. Wang, Y. Hu and H. L. Dai, ACS Nano, 2022, 16, 19491–19508 CrossRef CAS PubMed.
- Y. Gao, J. T. Li, S. F. Chu, Z. Zhang, N. H. Chen, L. Li and L. Zhang, Eur. J. Pharmacol., 2020, 866, 172801 CrossRef CAS PubMed.
- J. Gao, P. Bai, Y. Y. Li, J. Z. Li, C. X. Jia, T. S. Wang, H. B. Zhao, Y. C. Si and J. X. Chen, J. Proteome Res., 2020, 19, 2676–2688 CrossRef CAS PubMed.
- A. Aires and R. Carvalho, J. Food Sci. Technol., 2020, 57, 4265–4276 CrossRef CAS PubMed.
- P. Hikisz and J. B-Slomczewska, Nutrients, 2021, 13, 4313 CrossRef CAS PubMed.
- C. Varilla, M. Marcone, L. Paiva and J. Baptista, Foods, 2021, 10, 2249 CrossRef CAS PubMed.
- J. Borlinghaus, F. Albrecht, M. C. Gruhlke, I. D. Nwachukwu and A. J. Slusarenko, Molecules, 2014, 19, 12591–12618 CrossRef PubMed.
- E. Catanzaro, D. Canistro, V. Pellicioni, F. Vivarelli and C. Fimognari, Pharmacol. Res., 2022, 177, 106118 CrossRef CAS PubMed.
- A. Sharma, N. V. Tirpude, N. Bhardwaj, D. Kumar and Y. Padwad, Inflammopharmacology, 2022, 30, 655–666 CrossRef CAS PubMed.
- R. Pothuraju, R. K. Sharma, J. Chagalamarri, S. Jangra and P. Kumar Kavadi, J. Sci. Food Agric., 2014, 94, 834–840 CrossRef CAS PubMed.
- Z. Aziz and B. Abdul Rasool Hassan, Burns, 2017, 43, 50–57 CrossRef PubMed.
- B. Sadanandan, P. Murali Krishna, M. Kumari, V. Vijayalakshmi, B. M. Nagabhushana, S. Vangala, H. K. Singh, B. R. Divya Swaroopa and V. Megala, J. Mol. Struct., 2024, 1305, 137723 CrossRef CAS.
- M. Y. Al-darwesh, S. S. Ibrahim and M. A. Mohammed, Results Chem., 2024, 7, 101368 CrossRef CAS.
- E. A. Permyakov, Biomolecules, 2020, 10, 1210 CrossRef CAS PubMed.
- A. Sadiq, A. Shah, M. G. Jeschke, C. Belo, M. Qasim Hayat, S. Murad and S. Amini-Nik, Int. J. Mol. Sci., 2018, 19, 1034 CrossRef PubMed.
- S. Q. Yan, Q. Zhang, J. N. Wang, Y. Liu, S. Z. Lu, M. Z. Li and D. L. Kaplan, Acta Biomater., 2013, 9, 6771–6782 CrossRef CAS PubMed.
- M. Y. Sun, Y. Ye, L. Xiao, X. Y. Duan, Y. M. Zhang and H. Zhang, Int. J. Mol. Med., 2017, 39, 507–518 CrossRef CAS PubMed.
- M. Y. Tang, W. B. Wang, L. Y. Cheng, R. Jin, L. Zhang, W. W. Bian and Y. G. Zhang, Iran. J. Basic Med. Sci., 2018, 21, 309–317 Search PubMed.
- E. Başaran, A. A. Öztürk, B. Şenel, M. Demirel and Ş. Sarica, Eur. J. Pharm. Sci., 2022, 172, 106153 CrossRef PubMed.
- S. Faraji, N. Nowroozi, A. Nouralishahi and J. Shabani Shayeh, Life Sci., 2020, 257, 118062 CrossRef CAS PubMed.
- W. S. Yap, A. V. Dolzhenko, Z. Jalal, M. A. Hadi and T. M. Khan, Sci. Rep., 2019, 9, 18042 CrossRef CAS PubMed.
- V. López, B. Nielsen, M. Solas, M. J. Ramírez and A. K. Jäger, Front. Pharmacol, 2017, 8, 280 CrossRef PubMed.
- R. Samuelson, M. Lobl, S. Higgins, D. Clarey and A. Wysong, J. Altern. Complementary Med., 2020, 26, 680–690 CrossRef PubMed.
- T. Q. Chen, P. Y. Yang and Y. J. Jia, Int. J. Mol. Med., 2021, 47, 4846 Search PubMed.
- L. Li, X. J. Hou, R. F. Xu, C. Liu and M. Tu, Fundam. Clin. Pharmacol., 2017, 31, 17–36 CrossRef CAS PubMed.
- W. B. Qian, X. R. Cai, Q. H. Qian, W. Zhang and D. L. Wang, J. Cell. Mol. Med., 2018, 22, 4354–4365 CrossRef CAS PubMed.
- H. P. Shi, Y. N. Liu, Y. B. Bai, H. Lv, W. Zhou, Y. Liu and D.-G. Yu, Sep. Purif. Technol., 2024, 330, 125247 CrossRef CAS.
- J. F. Zhou, T. Yi, Z. Y. Zhang, D.-G. Yu, P. Liu, L. Z. Wang and Y. J. Zhu, Adv. Compos. Hybrid Mater., 2023, 6, 189 CrossRef CAS.
- P. Zhao, K. Zhou, Y. Xia, C. Qain, D. G. Yu, Y. Xie and Y. Liao, Adv. Fiber Mater., 2024, 5 DOI:10.1007/s42765-024-00397-6.
- D.-G. Yu, W. Gong, J. Zhou, Y. Liu, Y. Zhu and X. Lu, WIREs Nanomed. Nanobiotechnol., 2024, 16, 1964 Search PubMed.
- Z.-C. Yao, C. C. Zhang, Z. Xing, Z. Ahmad, Q. P. Ding and M.-W. Chang, Chem. Eng. J., 2022, 429, 132221 CrossRef CAS.
- Y. N. Lang, B. L. Wang, M.-W. Chang, R. Y. Sun and L. F. Zhang, Colloids Surf., A, 2023, 668, 131399 CrossRef CAS.
- X. Jiang, Y. E. Zeng, C. Li, K. Wang and D. G. Yu, Front. Bioeng. Biotechnol., 2024, 12, 1354286 CrossRef PubMed.
- Y. Wang, L. Liu, Y. J. Zhu, L. Z. Wang, D.-G. Yu and L.-Y. Liu, Pharmaceutics, 2023, 15, 2561 CrossRef CAS PubMed.
- C. Huang, M. Wang, S. Yu, D. G. Yu and S. W. A. Bligh, Nanomaterials, 2024, 14, 646 CrossRef CAS PubMed.
- S. Chen, J. F. Zhou, B. Y. Fang, Y. Ying, D.-G. Yu and H. He, Macromol. Mater. Eng., 2023, 309, 2300361 CrossRef.
- L. Sun, J. F. Zhou, Y. M. Chen, D.-G. Yu and P. Liu, Front. Bioeng. Biotechnol., 2023, 11, 4 Search PubMed.
- X. H. Chen, Y. B. Liu and P. Liu, Mol. Pharm., 2024, 21, 173–182 CrossRef CAS PubMed.
- Q. Q. Lv, X. F. Ma, C. M. Zhang, J. Q. Han, S. J. He, K. M. Liu and S. H. Jiang, Int. J. Biol. Macromol., 2024, 259, 129268 CrossRef CAS PubMed.
- Y. Zhu, C. Zhang, Y. Liang, J. Shi, Q. Yu, S. Liu, D. G. Yu and H. Liu, Biomater. Sci., 2024, 17, 1643–1661 RSC.
- J. Zhou, H. Pan, W. Gong, D. G. Yu and Y. Sun, Nanoscale, 2024 10.1039/D4NR00893F.
Footnote |
† These authors contributed equally to this work. |
|
This journal is © The Royal Society of Chemistry 2024 |