DOI:
10.1039/D0QO00422G
(Review Article)
Org. Chem. Front., 2020,
7, 2107-2144
One-electron oxidative dehydrogenative annulation and cyclization reactions
Received
4th April 2020
, Accepted 14th June 2020
First published on 15th June 2020
Abstract
One-electron oxidation (OEO) can deeply change the structure and reactivity of organic molecules. Rings exist ubiquitously in natural products and pharmaceutical molecules. The oxidative dehydrogenative annulation/cyclization reaction has become one of the most straightforward and powerful tools to construct ring-containing molecules. This review focuses on the recent advances in OEO involved oxidative dehydrogenative annulations and cyclizations for the intermolecular and intramolecular construction of valuable ring structures.
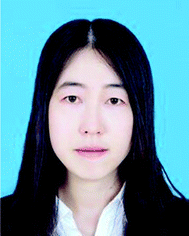 Xiazhen Bao | Xiazhen Bao received her BSc from Northwest Normal University in 2013 and PhD from Lanzhou University in 2018 under the supervision of Prof. Bo Zhou. Subsequently, she joined the research group of Prof. Congde Huo in 2019 at Northwest Normal University as a postdoctoral fellow. Her research interests focus on radical chemistry and medicinal chemistry. |
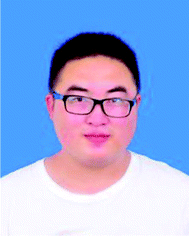 Wei Jiang | Wei Jiang received his BSc from Northwest Normal University in 2017. He is currently an MSc student in the group of Prof. Congde Huo at Northwest Normal University. His research focuses on the development of new double oxidative dehydrogenative (DOD) cyclization reactions. |
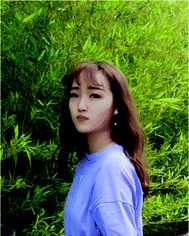 Jia Liang | Jia Liang received her BSc from Qilu Normal University in 2018. She is currently an MSc student in the group of Prof. Congde Huo at Northwest Normal University. Her research focuses on the development of new oxidative dehydrogenative coupling reactions. |
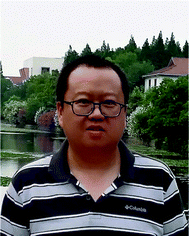 Congde Huo | Congde Huo received his BSc (Lanzhou University) in 2000 and PhD (Lanzhou University) in 2005 under the supervision of Prof. Zhong-Li Liu. He was a research associate at Hong Kong Polytechnic University (2005–2007) and a postdoctoral fellow at McGill University (2007–2009) under the supervision of Prof. Tak-Hang Chan. He then joined Northwest Normal University in 2009, and is currently a professor of organic chemistry. His research interests cover the development of single electron transfer (especially one-electron oxidation) involved efficient and novel reactions and the exploration of their mechanisms. He is also generally interested in green chemistry and medicinal chemistry. |
1. Introduction
Carbon–hydrogen bonds are ubiquitous in organic molecules and the direct functionalization of C–H bonds has been identified as a promising synthetic strategy for the formation of new carbon–carbon bonds and carbon–hetero bonds.1 Oxidative dehydrogenative coupling means a combination of one X–H bond (X: carbon or hetero-atom) with another Y–H bond (Y: carbon or hetero-atom) for the construction of a new X–Y bond directly.2 Actually, this kind of transformation to construct C–C bonds could date back to the early 1960s.3 Along with the prevalence of green chemistry and sustainable chemistry, great efforts have been devoted to explore atom-economical and step-economical strategies to achieve direct functionalizations of C–H bonds. Thus, the oxidative dehydrogenative coupling protocol was recognized by chemists again and has gained widespread attention and witnessed explosive growth in recent years.4
Single electron transfer (SET), as one of the simplest elemental reactions, has a deep influence on the structure and reactivity of organic molecules. One-electron oxidation (OEO) is stated to take one electron away from the substrate that undergoes further transformations.5 As shown in Scheme 1, there are usually two pathways to generate reactive radical intermediates or carbocation intermediates from neutral organic compounds through OEO processes. Pathway 1: substrates lose one electron first to form radical cation intermediates. Then a deprotonation process occurs to deliver radical species. Or a dehydrogenation process occurs to deliver the corresponding carbocation species. Pathway 2: substrates lose one proton first to generate carbanion intermediates. Then an OEO process occurs to deliver radical species. One more OEO process of the radical species could occur to deliver the corresponding carbocation species (Scheme 1). When organic molecules are one-electron oxidized to their radical cations, the dissociation energies of their α-C–H bonds are remarkably weakened and the acidity of their α-C–H bonds is amazingly enhanced.6 Therefore, OEO processes are really beneficial for C–H bond activation. OEO involved oxidative dehydrogenative C–H functionalization has become one of the most appealing synthetic strategies in recent years.7
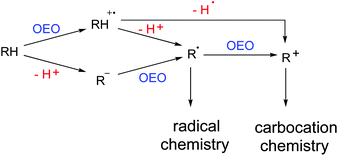 |
| Scheme 1 | |
Rings are the most privileged scaffolds existing ubiquitously in natural products and pharmaceutical molecules, which exhibit attractive physiological and biological activities. They are also indispensable chemical building blocks and transition-metal ligands.8 Hence, the development of simple and efficient synthetic methodologies for cyclic compounds attracts intense attention all the time.9 The oxidative dehydrogenative annulation/cyclization reaction has become one of the most straightforward and powerful tools to construct ring-containing molecules. This review will mainly focus on the recent advances in OEO involved oxidative dehydrogenative annulations and cyclizations for the intermolecular and intramolecular construction of valuable ring structures.
2. Intermolecular oxidative dehydrogenative annulation
2.1. Construction of five-membered rings
2.1.1. Oxidative dehydrogenative [2 + 3]-annulation.
Five-membered heterocycles are highly important structural motifs, which widely exist in natural products, pharmaceuticals and fundamental materials. Therefore, great efforts have been devoted to the construction of these heterocycles from readily available substrates. Among these methods, intermolecular [3 + 2]-annulation is one of the most straightforward approaches to construct five-membered heterocycles.
In 2014, the Rueping group reported a visible-light mediated oxidative [3 + 2]-cycloaddition of N-substituted hydroxylamines with alkenes for the construction of isoxazolidines. The aerobic oxidation of hydroxylamines to nitrones can be achieved through photoredox catalysis under oxidant-free conditions. The proposed mechanism involved single electron transfer and the following deprotonation of N-substituted hydroxylamines to afford nitrone intermediates, which are immediately blocked by alkenes yielding [3 + 2]-cycloaddition products (Scheme 2).10
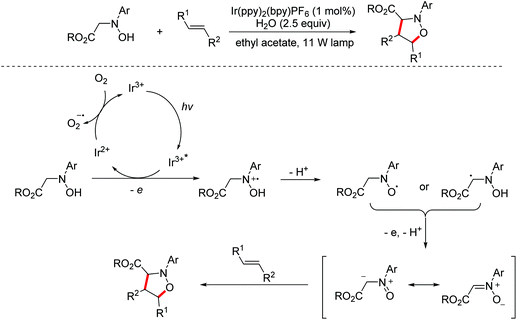 |
| Scheme 2 | |
In 2014, the Xie group developed a biomimetic strategy for the synthesis of pyrrole derivatives from commercially available amino acid esters through multiple dehydrogenations, deamination, and oxidative cyclization promoted by a co-catalyst system of Cu(II) and Mn(III) (Scheme 3).11 In addition, this biomimetic strategy has made a valuable contribution to the total synthesis of lycogarubin C and CPA.
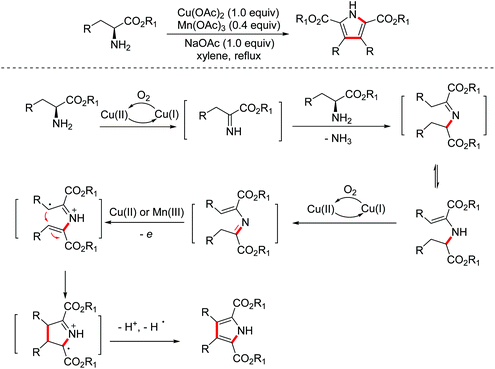 |
| Scheme 3 | |
In 2014, the Xiao group developed a visible-light induced aerobic oxidation/[2 + 3]-cycloaddition/aromatization cascade reaction between glycine esters and isocyanides to construct diverse 1,5-disubstituted imidazoles.12 The reaction proceeded through a photocatalytic oxidative dehydrogenation of glycine esters to generate imine intermediates, which were then captured by isocyanides via a formal [2 + 3]-cycloaddition with subsequent proton transfer/aromatization to produce the desired products (Scheme 4).
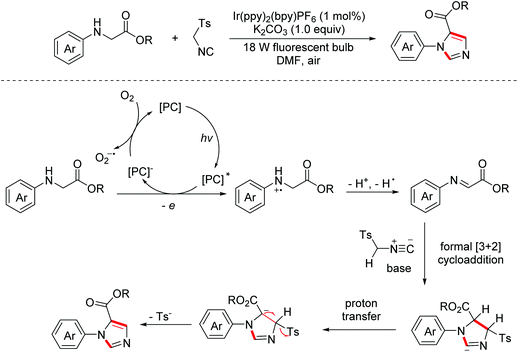 |
| Scheme 4 | |
In 2015, the Liu group disclosed a copper-catalyzed sequential aerobic oxidation/[2 + 3]-cycloaddition/oxidative aromatization cascade reaction of glycine esters with α-diazo compounds through imine intermediates to construct 1,2,3-triazoles (Scheme 5).13
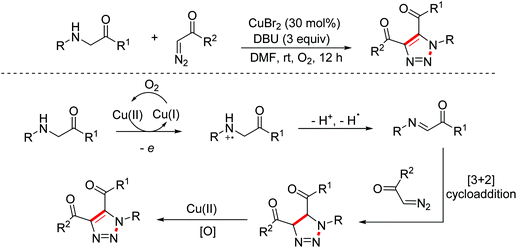 |
| Scheme 5 | |
In 2015, the Huo group developed a copper-catalyzed aerobic oxidative dehydrogenative formal [2 + 3]-annulation of glycine derivatives with α-angelicalactone for the construction of biologically significant multi-substituted pyrrolidone derivatives.14 The proposed mechanism for this process is presented in Scheme 6. Firstly, electrophilic iminium ion intermediates were formed under copper-catalyzed aerobic conditions, which then underwent nucleophilic addition with nucleophilic α-angelicalactone. Then, intramolecular nucleophilic substitution and deprotonation occurred to produce pyrrolidone derivatives. Afterward, a visible light-induced version of this transformation was reported by the Zhang group in 2018.15
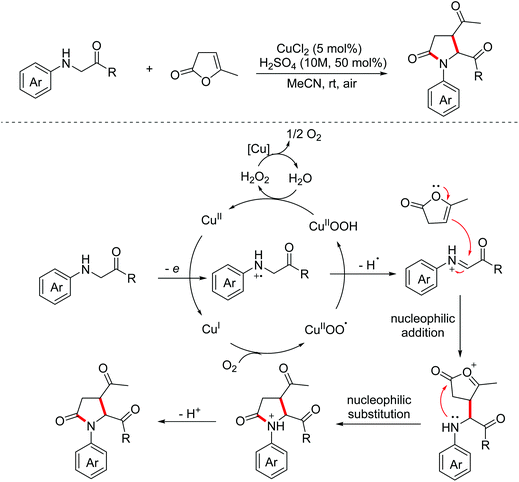 |
| Scheme 6 | |
The fully substituted 4,5-biscarbonyl imidazole moiety is an important core of some natural products due to its widespread biological and pharmacological activities. In 2017, the Wang group reported a copper-catalyzed aerobic oxidative reaction between glycine derivatives and 5-alkoxyoxazoles for accessing tetra-substituted 4,5-biscarbonyl imidazoles via the [2 + 3]-annulation/aromatization cascade annulation process.16 The process featured a similar oxidative cyclization mechanism (Scheme 7).
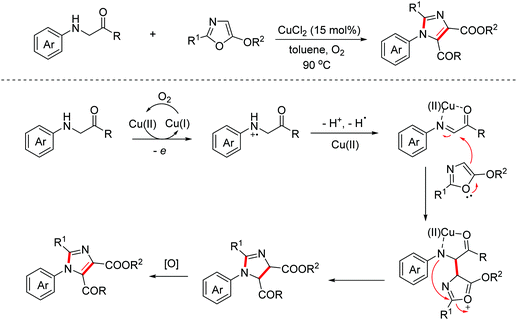 |
| Scheme 7 | |
Enols are useful building blocks in organic synthetic chemistry and they can be in situ generated from the corresponding ketones. In 2019, the Baidya group disclosed the synthesis of clausenamide analogues from N-arylglycine derivatives with α-keto esters via oxidative cross-dehydrogenative [2 + 3]-annulation.17 The mechanistic investigation proposed that the reaction proceeded through a nucleophilic addition of electrophilic imines (generated from N-arylglycines via Cu(II)-catalyzed oxidation) with nucleophilic enols (generated from ketones under base catalysis). Then a cyclization process occurred to yield pyrrolones (Scheme 8).
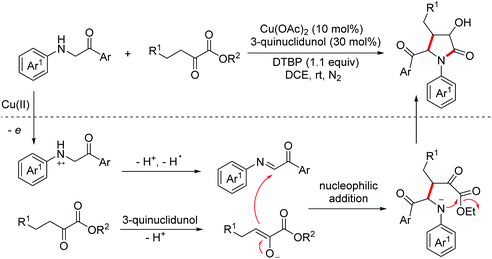 |
| Scheme 8 | |
Imidazolidines represent an important class of five-membered nitrogen-containing heterocycles with a broad spectrum of biological activities. Aziridines are important synthetic synthons, which can serve as masked 1,3-dipoles to participate in formal [3 + 2]-annulation with dipolarophiles. In 2017, the Punniyamurthy group reported a stereospecific Cu(II)/TBHP catalyzed one-pot reaction of N-alkylanilines with aziridines for the construction of 1,3-imidazolidines.18 The proposed mechanism for this process is presented in Scheme 9. Initially, the SN2 reaction of aziridines and N-alkylanilines took place to deliver the pivotal intermediates, which then underwent the OEO process and the following intramolecular nucleophilic cyclization via the Cu(II)/TBHP catalytic cycle to yield the desired 1,3-imidazolidines.
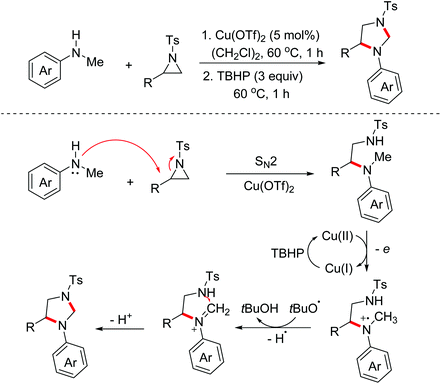 |
| Scheme 9 | |
In 2018, the Huo group developed an aerobic oxidative dehydrogenative formal [2 + 3]-annulation of glycine derivatives with aziridines to produce highly functionalized imidazolidine derivatives.19 The reaction also began with the SN2 ring-opening step. However, the authors found that the subsequent cyclization did not proceed through the classical copper induced aerobic oxidative dehydrogenative catalytic cycle after careful mechanism studies. An auto-oxidation involved mechanism with the following Brønsted acid promoted intramolecular SN1 type nucleophilic process was proposed (Scheme 10).
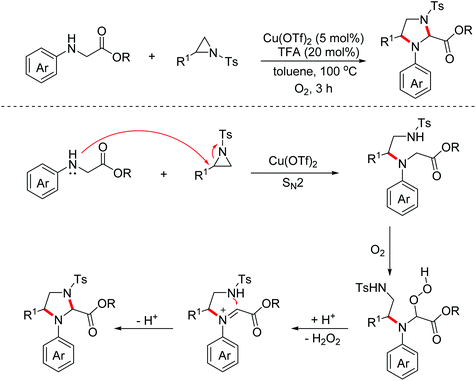 |
| Scheme 10 | |
In 2020, a visible-light-induced aerobic oxidative [2 + 3]-cycloaddition between glycine derivatives and styrene oxides for the construction of 1,3-oxazolidines was reported by the Zhang group.20 The process involved a photo-active EDA complex and no external photoredox catalyst was needed. A series of control and UV-Vis absorption spectroscopy experiments proved that glycine esters first interacted with benzyl iodides, which were in situ generated from styrene oxides in the presence of HI, to form the corresponding EDA complexes. Then, the key intermediates with charge separation were formed under visible-light irradiation with oxidation of the EDA complex. Finally, the nucleophilic addition/intramolecular nucleophilic substitution/deprotonation process occurred to give 1,3-oxazolidines (Scheme 11).
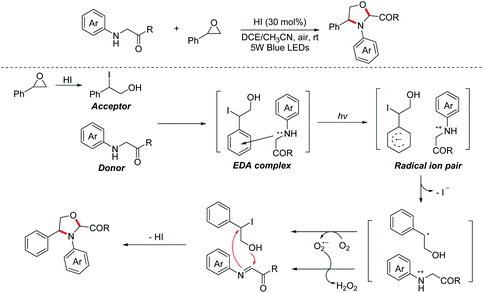 |
| Scheme 11 | |
In 2020, Miao and Yang et al. reported a copper-catalyzed annulation of oxime acetates with α-amino acid ester derivatives for the synthesis of 4-pyrrolin-2-one derivatives. Oxime acetates act as internal oxidants and 1,3-dinucleophilic species simultaneously in this reaction.21 The key imine intermediates were generated by one-electron oxidation and enamino-copper intermediates were delivered by one-electron reduction. Then the transformation proceeded through nucleophilic addition, intramolecular cyclization and oxidation process between in situ generated enamino-copper and imine intermediates to give the desired heterocyclic products (Scheme 12).
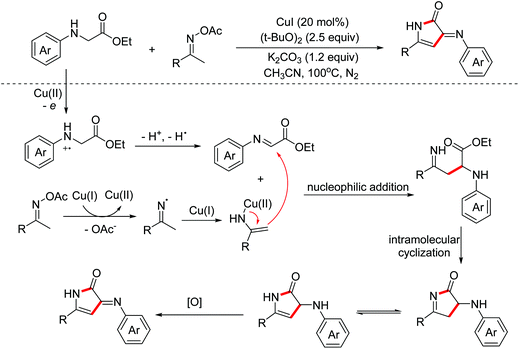 |
| Scheme 12 | |
Pyrazoles are kinds of crucial bioactive motifs, which are widely present in synthetic medicines and natural products. In 2019, the Huang group explored a Cu(I)-catalyzed oxidative formal [3 + 2]-annulation of ketoxime acetates with tetrahydroisoquinolines to construct isoquinoline-fused pyrazoles (Scheme 13).22
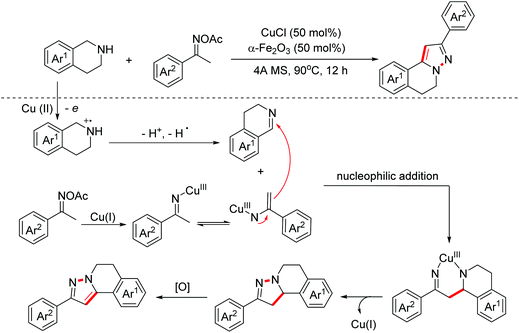 |
| Scheme 13 | |
Benzoheterocycle motifs exist in some important bioactive natural products and are associated with widespread bioactivity. In 2017, the Lei group developed an electro-oxidative [3 + 2]-annulation between phenols and N-acetylindoles under undivided electrolytic conditions to synthesize benzofuroindolines.23 The two substrates were evenly oxidized by an anode to yield the relevant oxidized intermediates first. Then the two intermediates underwent direct cross-coupling with the following intramolecular cyclization/deprotonation to form benzofuroindolines. The chemical oxidants were avoided and H2 was the only by-product (Scheme 14). In 2020, Zhang and Wang et al. also reported an electro-oxidative annulation of phenols with electron-deficient alkenes to achieve 2,3-dihydrobenzofuran skeletons.24
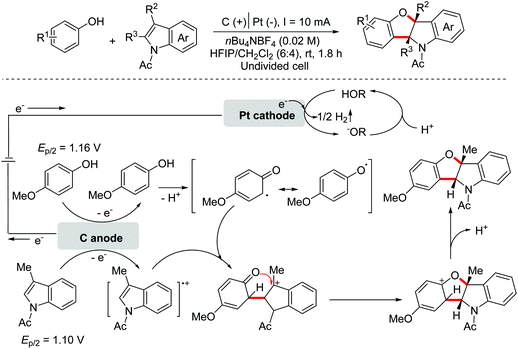 |
| Scheme 14 | |
In 2020, a synthetic method of 2-diarylaminoindolo[2,3-b]indoles via a copper-catalyzed [3 + 2]-annulation reaction of diarylamines with indoles was reported by the Zhang group.25 The possible mechanism is illustrated in Scheme 15. The dimerization of diphenylamine occurred first through a one-electron oxidation involved process. Then the dimers were one-electron oxidized again to generate radical cation intermediates. Subsequently, with the following reaction steps, polycyclic products were achieved (Scheme 15).
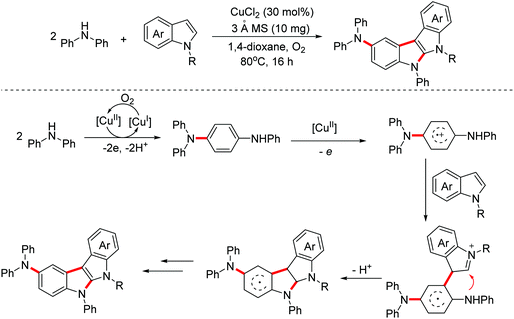 |
| Scheme 15 | |
Benzothiophenes are important sulfur-containing heterocycles with promising pharmacological activity. In 2018, the Huo group realized an auto-oxidation mediated intermolecular oxidative [3 + 2]-annulation reaction of thiophenols with alkynes to construct complex benzothiophene derivatives.26 Several control experiments indicated that sulfur-centered radicals were involved as the key intermediates in the process and the following radical annulation occurred to deliver the targeted benzothiophenes (Scheme 16).
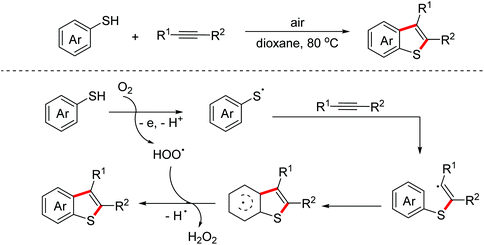 |
| Scheme 16 | |
2.1.2. Oxidative dehydrogenative [2 + 2 + 1]-annulation.
Nitrogen/oxygen-containing heterocyclic moieties, such as oxazoles and pyrroles, are universal skeletons in natural products which possess significant and extensive biological activities. The synthesis of these compounds is a hot topic in organic synthesis. In 2012, the Jiao group demonstrated a Cu(II)-mediated aerobic oxidative dehydrogenative [2 + 2 + 1]-annulation of aldehydes, amines and molecular oxygen to synthesize oxazole derivatives with the removal of six hydrogen atoms and the functionalization of four C(sp3)–H bonds (Scheme 17).27 In 2015, the Pan group accomplished a facile synthesis of 2,5-disubstituted oxazoles via a copper-catalyzed cascade reaction of alkenes with azides.28 Subsequently, TsOH/I2-mediated and copper-catalyzed oxidative dehydrogenative annulations of amines and alkynes to construct substituted oxazoles were developed by the Chen and Jiao groups, in 2017 and 2018 respectively.29
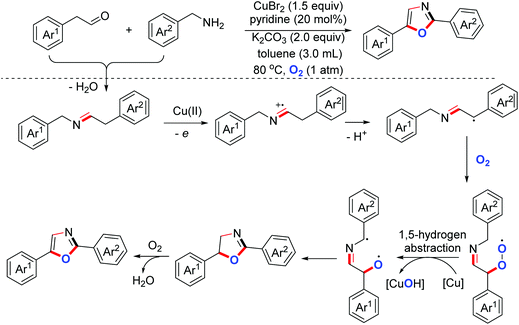 |
| Scheme 17 | |
1H-Pyrrol-2(3H)-ones, an important class of nitrogen heterocycles, represent the key structural motifs in many valuable biologically active molecules and natural products. In 2016, Tu and Zhang et al. reported a CuO/TEMPO-mediated multi-step cascade process of diarylethanones and primary amines to access multi-aryl 1H-pyrrol-2(3H)-ones.30 Moreover, the AIE (Aggregation Induced Emission) effects of these compounds were also evaluated by the authors (Scheme 18).
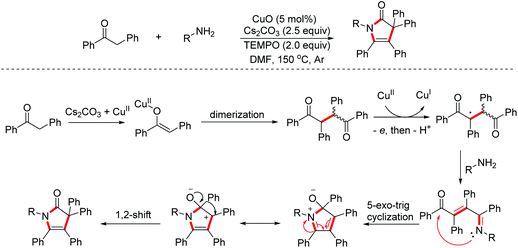 |
| Scheme 18 | |
Pyrroles are a vital class of aromatic heterocycle compounds, which can be found in many bioactive natural products and significantly important pharmaceuticals. In 2017, the Zhao group explored a Cu(I)-catalyzed oxidative annulation of diverse amines, alkyne esters and maleimides to synthesize fully substituted dihydropyrroles via the cleavage of C(sp2)–H/N–H bonds.31 The proposed mechanism indicated that the key radical intermediates were formed through one-electron oxidation of imine intermediates, which were derived from in situ generated enamines. Subsequently, intramolecular cyclization and the following oxidation occurred to yield pyrroles (Scheme 19). In 2018, a similar Mn(OAc)3-promoted oxidative annulation of alkenes with amines/alkyne esters or enaminone esters to deliver polysubstituted dihydropyrroles was developed by the Zhang group.32
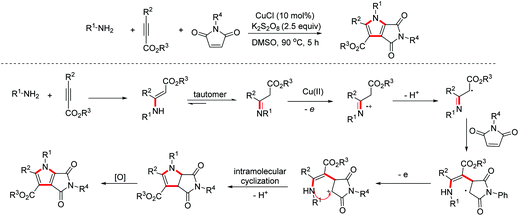 |
| Scheme 19 | |
2.1.3. Oxidative dehydrogenative [4 + 1]-annulation.
In 2018, the Xu group explored an electrochemical dehydrogenative [4 + 1]-annulation of N-allyl amides with 1,3-dicarbonyl compounds to obtain pyrrolidines.33 Mechanistic experiments suggested that carbon centered radical intermediates were obtained from malonates through deprotonation and the following OEO process in the presence of redox-active catalysts. Later, radical intermediates were added to the alkenyl moiety of N-allyl amides generating radical adducts, which then underwent step-wise transformations to deliver pyrrolidine derivatives (Scheme 20).
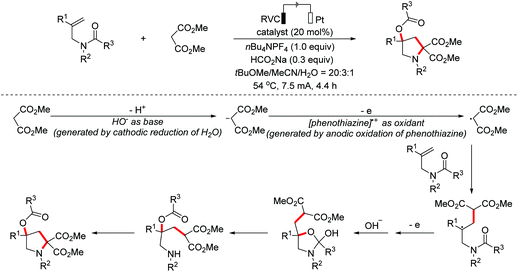 |
| Scheme 20 | |
2.2. Construction of six-membered rings
2.2.1. Oxidative dehydrogenative [4 + 2]-annulation.
Quinoline derivatives exist in a number of natural products and synthetic pharmaceuticals possessing diverse biological activities.34 Many different strategies for the synthesis of quinolines have been developed. Among them, the direct oxidative dehydrogenative [4 + 2]-annulation of various substituted aniline derivatives with olefins is one of the most atom-economical strategies to construct decorated quinolines.
In 2011, the Mancheño group developed an oxidative Povarov/aromatization tandem reaction of glycine derivatives with alkenes to synthesize substituted quinolines employing FeCl3 as the catalyst and the (2,2,6,6-tetramethylpiperidin-1-yl)oxy (TEMPO) oxoammonium salt as the oxidant.35 The proposed mechanism is shown in Scheme 21. Firstly, iminium ions were formed via one-electron oxidation and the following dehydrogenation of glycine derivatives. Then, the nucleophilic attack on iminium intermediates by alkenes occurred to generate carbocationic species, which underwent a F–C reaction to afford the corresponding quinolines. In 2012, an FeCl3-catalyzed approach between glycine derivatives and alkynes was developed by the Hu group.36 Since 2012, the Jia group realized this tandem oxidative degenerative [4 + 2]-annulation process using various catalyst systems, including TBPA+˙/InCl3/O2, Ce(IV)/InCl3/TBPA/O2 and TBN/InCl3/O2, for the construction of substituted quinolones.37
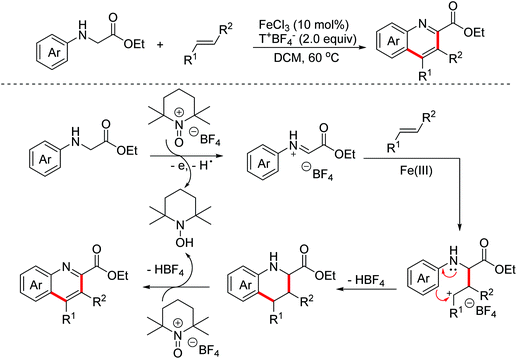 |
| Scheme 21 | |
In 2014, the Huo group discovered an unprecedented auto-oxidation coupling of glycine derivatives. The reaction was performed using organic solvents under an air atmosphere in the absence of any redox-active catalyst and chemical oxidants.38 The proposed mechanism implied that glycine esters were first auto-oxidized to generate hydroperoxide, which was then transformed into the iminium ion intermediate through the Brønsted acid-catalyzed SN1-type procedure. Subsequently, the Povarov reaction and the following aromatization process proceeded to form complex quinolines (Scheme 22). In 2015, the Huo group disclosed that CBr4 can promote this transformation more efficiently under an air atmosphere.39
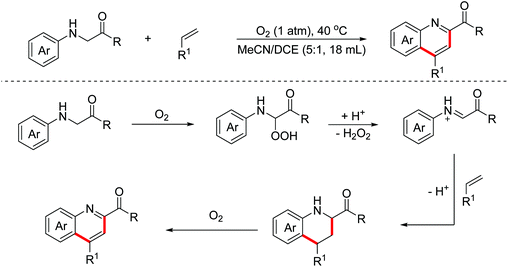 |
| Scheme 22 | |
In 2015, the Liu group developed a K2S2O8-catalyzed cross-dehydrogenative coupling of glycine derivatives with olefins.40 In this process, the SO4 radical anion abstracted one hydrogen atom from glycine derivatives first to form radical intermediates, which then were one-electron oxidized by a Cu(II) salt to afford the crucial imine intermediates. In 2016, they also reported that the Cu(OTf)2/NHPI/O2 system is efficient for this transformation.41 In 2017, this annulation reaction using a gold–oxazoline complex as a catalyst was reported by the Feng group.42
In 2016, the Zhang group accomplished a photocatalytic oxidative dehydrogenative coupling/aromatization tandem reaction of glycine esters with alkenes under an air atmosphere. It also featured the same imine pathway.43 In 2017, the Wu group demonstrated a Cu(II) salt-catalyzed C–H functionalization of glycine derivatives to deliver a variety of heterocycles.44 The process was triggered by visible light irradiation and no external photosensitizer was involved. The complexes formed in situ from Cu(II) salts with substrate amines or imines were identified as the key intermediates.
In 2017, the Jia group reported a radical cation salt-catalyzed tandem oxidative Povarov reaction of glycine esters and α-methylstyrenes for the preparation of 3,4-dihydroquinoline-3-one derivatives (Scheme 23).45
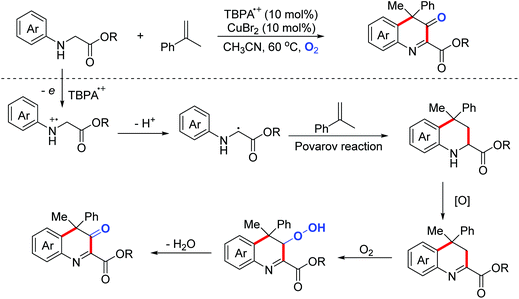 |
| Scheme 23 | |
In 2020, the Huo group developed an auto-oxidative Povarov/aromatization tandem reaction of glycine derivatives with enamides to yield quinoline derivatives. The transformation was performed using only catalytic amounts of Brønsted acid and O2. The acylamino group was used as both activating and leaving groups to improve the reaction efficiency (Scheme 24).46
 |
| Scheme 24 | |
In 2020, the Brasholz group developed a visible-light mediated oxidative dehydrogenative [4 + 2]-annulation/aromatization reaction of glycine esters with indoles to synthesize indolo[3,2-c]quinolones (Scheme 25).47
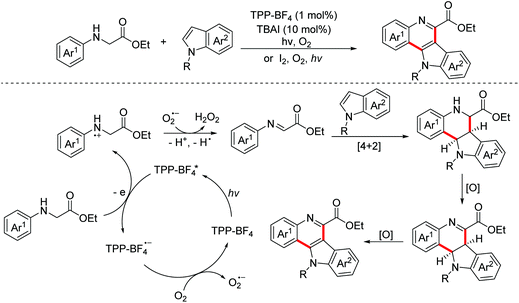 |
| Scheme 25 | |
Besides glycine derivatives, N-benzylanilines are also suitable substrates for the preparation of quinolines via a similar oxidative Povarov/aromatization tandem reaction. In 2012, the Liu group developed an iron-catalyzed oxidative annulation of N-benzyl anilines with alkynes or alkenes for the synthesis of quinoline derivatives (Scheme 26). The process proceeded through the imine intermediate pathway.48 After that, radical cation salt and Fe(OTf)3/AcOH-prompted aerobic oxidative Povarov reactions of N-benzylanilines have also been explored by the Jia and Sun groups, in 2015 and 2018 respectively.49
 |
| Scheme 26 | |
As shown above, the oxidative dehydrogenative Povarov reaction of secondary amines has been well developed. A similar tandem process using tertiary amines as the substrates was also realized. The process also involved sequential single electron transfer and dehydrogenation to generate iminium ions, and then the Povarov reaction was performed with electron-efficient alkenes to deliver the desired products.
In 2014, the Seidel group reported copper(I) catalyzed oxidative [4 + 2]-cycloaddition reactions of N-aryl tetrahydroisoquinolines with 1-vinylpyrrolidin-2-one for the synthesis of polycyclic amines via dual functionalization of both a C(sp3)–H bond and a C(sp2)–H bond (Scheme 27).50
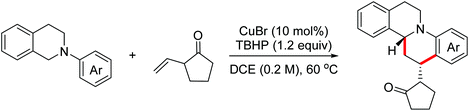 |
| Scheme 27 | |
In 2016, the Huo group demonstrated a cobalt-catalyzed aerobic oxidative dehydrogenative formal [4 + 2]-annulation reaction of N,N-dimethylanilines with dihydrofuran to obtain hexahydrofuroquinoline motifs (Scheme 28).51
 |
| Scheme 28 | |
In 2016, the Guan group reported an iron-catalyzed dehydrogenative [4 + 2]-cycloaddition reaction of tertiary anilines and enamides for the synthesis of tetrahydroquinolines with amido-substituted quaternary carbon centers (Scheme 29).52 In 2018, the Lei group also developed an electrochemical oxidative [4 + 2]-annulation of tertiary anilines with enamides to generate tetrahydroquinolines.53
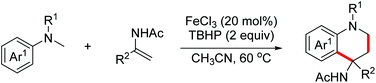 |
| Scheme 29 | |
Electron-deficient alkenes, such as maleimides, can also be used as building blocks in the oxidative dehydrogenation cross-coupling cascade of N,N-dimethylanilines. The transformation involved a single electron transfer followed by deprotonation to give α-amino alkyl radicals from tertiary amines. Subsequently, electrophilic radicals were added to the electron-deficient double bond with subsequent free-radical cyclization to generate the corresponding cyclohexadienyl radicals, which were then readily rearomatized by a second electron transfer/proton elimination leading to the final products.
In 2011, Miura and Hirano developed a copper-catalyzed oxidative annulation of N-methylanilines with electron-deficient olefins involving maleimides and benzylidene malononitriles to obtain the corresponding tetrahydroquinolines through the C(sp3)–H and C(sp2)–H bond functionalization procedure (Scheme 30).54 In 2016, the Bissember group explored a direct functionalization of α-amino C–H bonds of N-methylanilines under copper(I) photocatalysis with Brønsted acids as co-catalysts.55 Various catalytic systems were developed to achieve the transformation.56 In 2018, the Wu group developed a photo-catalyzed oxidative annulation of tertiary anilines with maleimides to synthesize tetrahydroquinolines using Ru(bpy)32+ and cobaloxime as co-catalysts under redox-neutral conditions.57 In 2018, the Sundén group reported an EDA-mediated photochemical oxidative annulation reaction for the synthesis of tetrahydroquinolines from dialkylanilines and maleimides using molecular oxygen as the terminal oxidant.58 The mechanism investigation revealed that α-aminoalkyl radicals were generated via an electron donor–acceptor (EDA) complex.
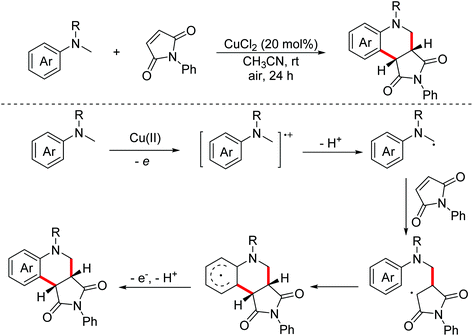 |
| Scheme 30 | |
In 2018, the Lei group reported a photoinduced oxidative [4 + 2]-annulation of imines with alkenes for accessing multi-substituted 3,4-dihydroisoquinoline derivatives with high regioselectivity and trans-diastereoselectivity utilizing a dual photoredox/cobaloxime catalytic system.59 Initially, alkene radical cation intermediates were delivered through one-electron oxidation of substrate alkenes by the excited photosensitizer. Then radical addition with imines occurred to generate relatively stable benzyl radicals, which underwent radical cyclization leading to the final isoquinoline derivatives (Scheme 31).
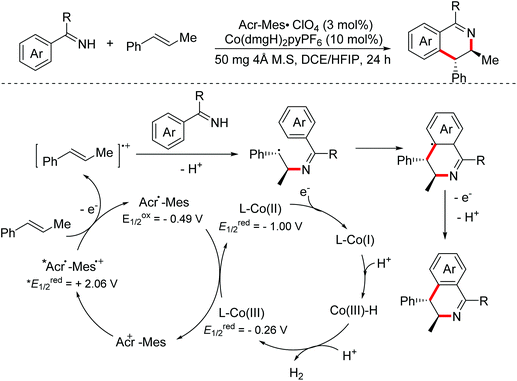 |
| Scheme 31 | |
In 2020, Weng, Chiang and Lei et al. developed an electrochemical oxidative dehydrogenative [4 + 2]-annulation of indole derivatives to construct polycyclic indoline derivatives (Scheme 32).60
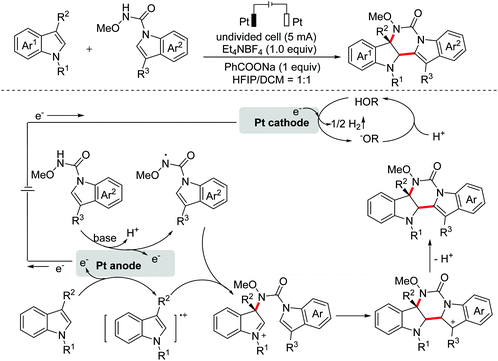 |
| Scheme 32 | |
In 2020, Tang and Pan et al. reported an electrochemical catalyzed [4 + 2]-annulation of alkynes with 1,3-dicarbonyl compounds to synthesize 1-naphthol derivatives via the C-centered radical-mediated intramolecular cyclization process (Scheme 33).61
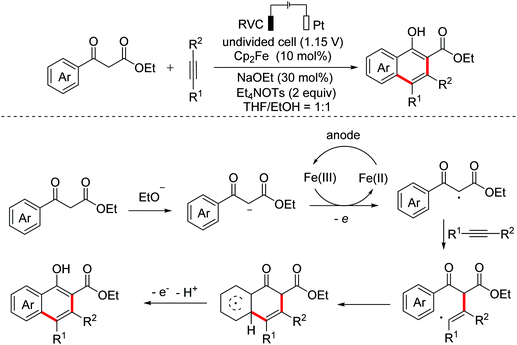 |
| Scheme 33 | |
Quinoline-fused lactones are important subunits in a lot of bioactive natural and synthetic products. In 2015, the Huo group accomplished an oxidative dehydrogenative coupling of glycine derivatives with 2,3-dihydrofuran using the dual catalyst system CuCl2–H2SO4 (Scheme 34).62 This methodology could achieve the synthesis of the analogue of bioactive luotonin A. Afterwards, similar tandem processes, using the TBPA+˙/O2 and photoredox catalyst systems, were achieved by the Jia and Li groups in 2016 and 2018, respectively.63
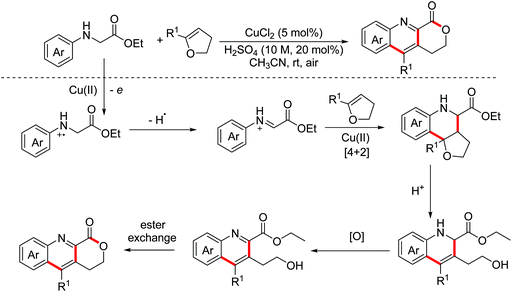 |
| Scheme 34 | |
In 2013, the Mancheño group reported an iron-catalyzed homo-condensation of glycine esters to construct dihydroquinazolines using the TEMPO oxoammonium salt as the oxidant.64 The mechanistic study confirmed that an iminium ion was formed first, which was then trapped by another glycine ester molecule (Scheme 35). A radical cation salt-induced homo-condensation of glycine derivatives was also developed by the Jia group in 2016.65
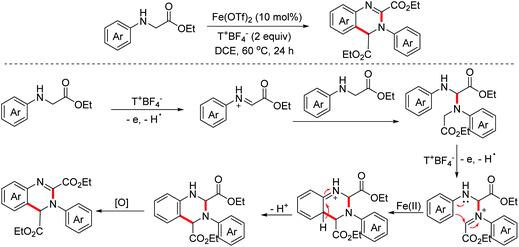 |
| Scheme 35 | |
In 2017, the Jia group achieved a radical cation salt-triggered dual removable activating group enabled Povarov homo-condensation reaction of N-aryl amino esters to deliver the desired quinoline-4-carboxylate esters.66 Control experiments revealed that a radical-mediated process was involved (Scheme 36).
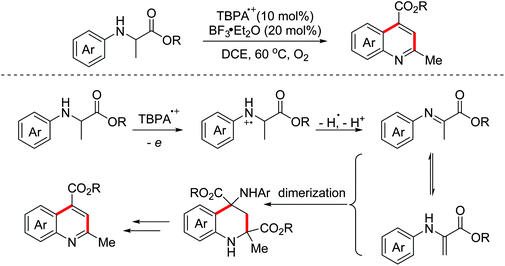 |
| Scheme 36 | |
Pyrimidinethiones are the core structure of numerous natural products and possess promising pharmacological properties. In 2014, the Sun group developed a photo-assisted metal-free multi-component dehydrogenative condensation of aryl aldehydes, thioureas and THF (or THP, 1,4-dioxane) to afford the corresponding pyrimidinethiones.67 In this transformation, dihydrofuran was first formed by dehydrogenative oxidation of THF, which could undergo a nucleophilic addition/annulation with the in situ formed imine derivatives to deliver pyrimidinethiones (Scheme 37).
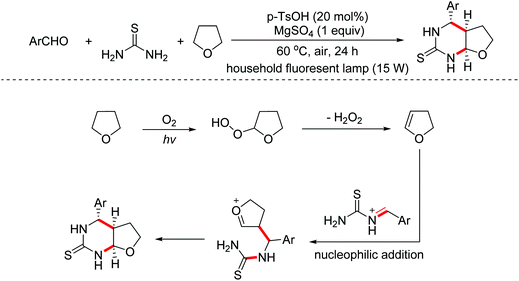 |
| Scheme 37 | |
In 2018, the Chen group reported an FePc-catalyzed biomimetic oxidative coupling annulation of tetrahydrocarbolines enabling the rapid construction of isochromanoindolenines (Scheme 38).68
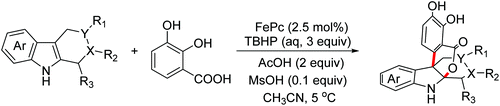 |
| Scheme 38 | |
In 2015, the Liu group reported a copper-catalyzed oxidative Povarov reaction between N-alkyl N-methylanilines and saturated oxa- and thiacyclic compounds to achieve the preparation of tetrahydroquinoline derivatives via activating inert sp3 C–H bonds.69 In this process, saturated tetrahydrofurans were oxidized by Cu(II)/TBHP to form dihydrofurans. In the meantime, N-alkylanilines underwent one-electron oxidation and dehydrogenation to deliver imine intermediates (Scheme 39).
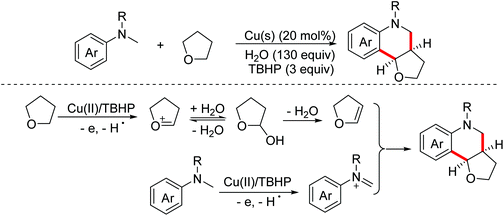 |
| Scheme 39 | |
Recently, the Huo group put forward the concept of the dual oxidative-dehydrogenative (DOD) annulation strategy. Compared with the cross-dehydrogenative coupling (CDC) reaction, the DOD annulation reaction activates four C–H bonds in substrates simultaneously. In 2015, the Huo group developed an iron-catalyzed DOD [4 + 2] tandem annulation of glycine derivatives with tetrahydrofurans for the synthesis of highly valuable quinoline fused lactones (Scheme 40).70
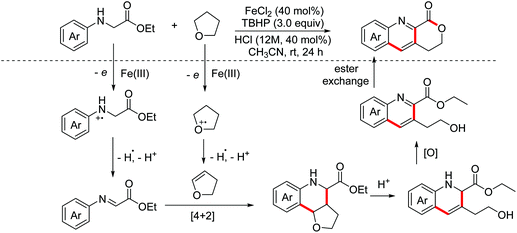 |
| Scheme 40 | |
In 2016, the Huo group disclosed a CBr4-promoted DOD reaction of glycine derivatives with dioxane for the synthesis of complex quinoline motifs (Scheme 41). This work proved again that CBr4 can be an efficient reagent to initiate radical reactions under mild reaction conditions. The key imine and dioxene intermediates were formed via the oxidation of glycine esters and dioxane by a bromine radical.71
 |
| Scheme 41 | |
The utility of the DOD strategy was further established by the Huo group. They successfully exploited a Cu(II)/DDQ/O2 system-catalyzed DOD tandem reaction of readily available glycine derivatives and ethylbenzenes in 2018.72 The mechanism study revealed that the active imines and styrenes were obtained through the oxidation of Cu(II)/O2 and DDQ respectively. Then the Cu(II)-promoted [4 + 2] Povarov reaction and the following oxidative aromatization occurred to achieve the quinolone derivatives (Scheme 42).
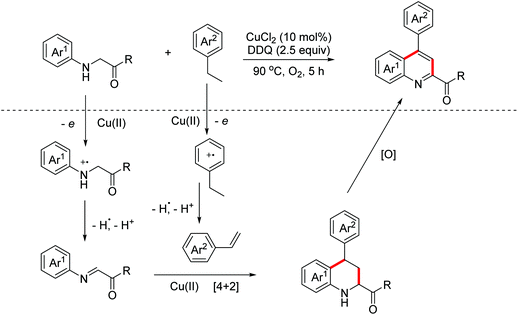 |
| Scheme 42 | |
Quinoline derivatives bearing a quaternary carbon center exhibit excellent bioactivities. In 2019, the Huo group disclosed a Cu(II)/DDQ/O2-catalyzed cascade reaction of N-arylglycine derivatives with cumene derivatives for the synthesis of substituted 3,4-dihydroquinoline-3-one derivatives (Scheme 43).73
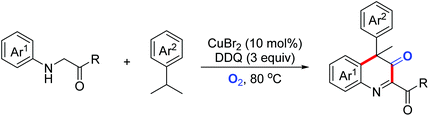 |
| Scheme 43 | |
2.2.2. Oxidative dehydrogenative [3 + 3]-annulation.
The oxidative dehydrogenative [3 + 3]-annulation is another promising strategy for the construction of six-membered heterocyclic compounds. In 2016, the Han group developed a Cu(II)/TEMPO system catalyzed [3 + 3]-annulation of commercially available amidines and saturated ketones for the synthesis of pyrimidines.74 The proposed mechanism showed that saturated ketones were oxidized to enones via oxidative dehydrogenation first, which then underwent intermolecular conjugate addition with amidines followed by intramolecular condensation and finally aromatization to produce the desired pyrimidines (Scheme 44).
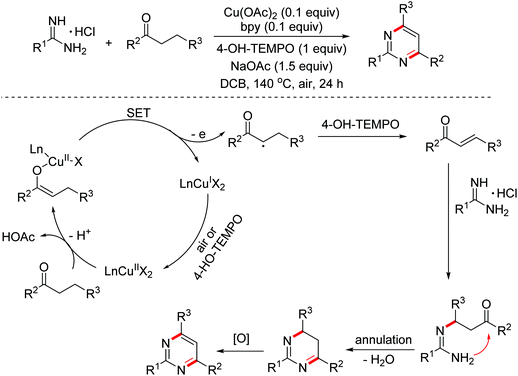 |
| Scheme 44 | |
In 2017, the Fan group presented a Cu(II)-catalyzed one-pot cascade reaction of saturated ketones with electron-deficient enamines for the synthesis of functionalized pyridines under an air atmosphere.75 The oxidation of saturated ketones and the following Michael addition were involved in this transformation (Scheme 45).
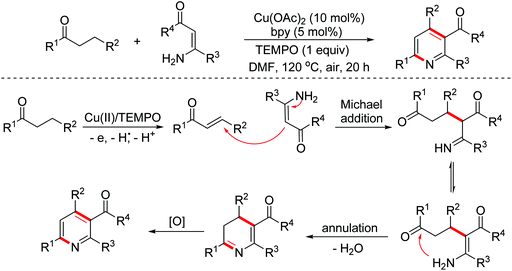 |
| Scheme 45 | |
The oxidative dehydrogenative functionalization of C(sp3)–H for the construction C–C bonds is mainly focused on C(sp3)–H adjacent to heteroatoms other than C(sp2)–H bonds. In 2017, the Yan group employed 1,3-diarypropenes as substrates to achieve the syntheses of bio-active pyranonaphthoquinones through DDQ-promoted C(sp3)–H and C(sp2)–H functionalization.76 In the key step, 1,3-diarypropenes were oxidized by DDQ through SET followed by dehydrogenation to generate allylic cations, which were then attacked by nucleophilic lawsones (Scheme 46).
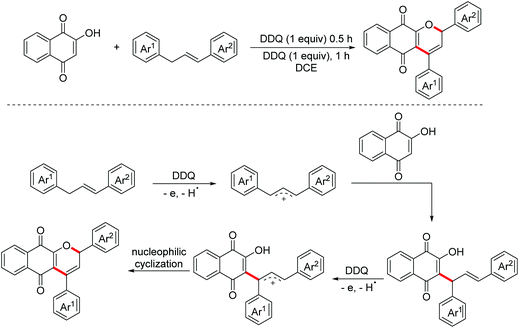 |
| Scheme 46 | |
In 2019, the Huo group developed an oxidative dehydrogenative formal [3 + 3]-annulation of benzylhydrazines with aziridines for the synthesis of highly functionalized tetrahydrotriazines.77 The plausible mechanism is shown in Scheme 47. In the presence of Cu(OAc)2 and O2, benzylhydrazines were oxidized to deliver nucleophilic hydrazones. Subsequently, nucleophilic ring opening of aziridines with hydrazones occurred. Finally, intramolecular oxidative amination occurred to deliver the desired triazines (Scheme 47).
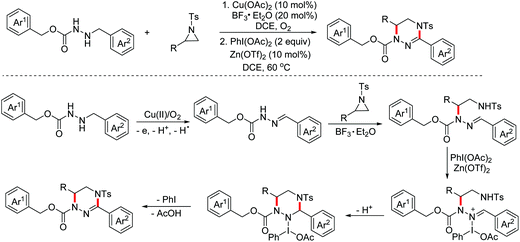 |
| Scheme 47 | |
2.2.3. Other examples.
Hantzsch esters, 1,4-dihydropyridine derivatives, are one of the most important heterocycles in biologically active and naturally occurring molecules. In 2014, the Jia group reported a radical cation salt prompted cascade reaction between glycine derivatives and β-ketoesters for the construction of 1,4-dihydropyridines using the fragment-reassembly strategy (Scheme 48).78 A radical pathway was proposed for this transformation. In 2016, the Le group reported a similar approach to access 1,4-dihydropyridine derivatives by a copper-catalyzed aerobic cascade oxidative coupling/cyclization.79 Different from Jia's work, iminium intermediates were proposed as the key intermediates.
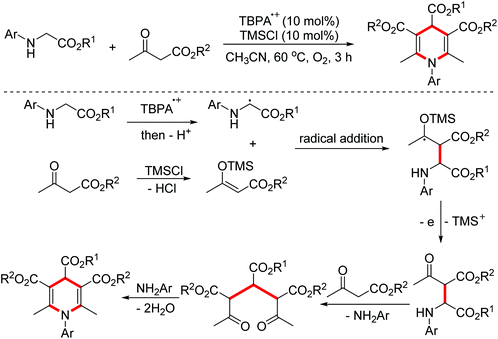 |
| Scheme 48 | |
2.3. Construction of small rings
Cyclopropanes are useful building blocks that can undergo a variety of transformations for the preparation of pharmaceuticals and functional materials. The synthesis of this synthon has evoked considerable interest and resulted in the development of different synthetic strategies. In 2015, the Antonchick group developed a copper-catalyzed direct oxidative cyclopropanation of electron-deficient alkenes with acetophenone derivatives (Scheme 49).80
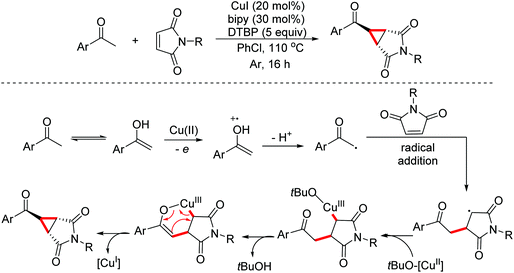 |
| Scheme 49 | |
In 2018, the Li group developed an aziridination of substituted alkenes through electrochemical catalytic oxidation employing hexafluoroisopropanol sulfamate as the nucleophilic nitrogen source.81 A proposed mechanism for this reaction is shown in Scheme 50. The alkenes were oxidized by the anode to their radical cations first, which were then attacked by nucleophilic HfsNH2. The adducts underwent second anodic oxidation/intramolecular nucleophilic ring closure leading to the final products (Scheme 50).
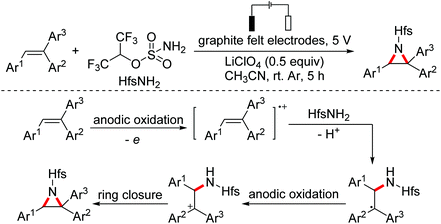 |
| Scheme 50 | |
In 2018, the Xu group developed a visible-light induced dehydrogenation/[2 + 2] cycloaddition sequence for the dual functionalization of two C(sp3)–H bonds to construct small strained cyclobutene skeletons.82 The mechanistic study proved that the elusive cyclic enamine intermediates were formed under these mild reaction conditions (Scheme 51).
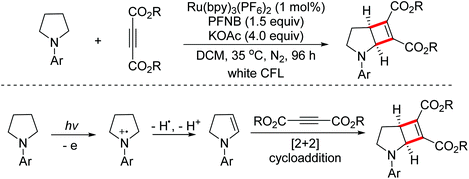 |
| Scheme 51 | |
3. Intramolecular oxidative dehydrogenative cyclization
3.1. Construction of five-membered rings
3.1.1. C–C bond formation.
Intermolecular one-electron oxidative dehydrogenative annulation has been extensively discussed as mentioned above. Compared with intermolecular cases, the development of new methodologies for the formation of new C–C bonds in an intramolecular manner has also been realized recently.
In 2013, the Ge group developed a copper-catalyzed aerobic intramolecular dehydrogenative cyclization reaction of N,N-disubstituted hydrazones through C(sp3)–H functionalization.83 A possible mechanism was proposed that hydrazines were first oxidized to form iminium ions, which then underwent tautomerization to generate the corresponding enamines. Subsequently, intramolecular cyclization/aromatization occurred to deliver the pyrazole products (Scheme 52).
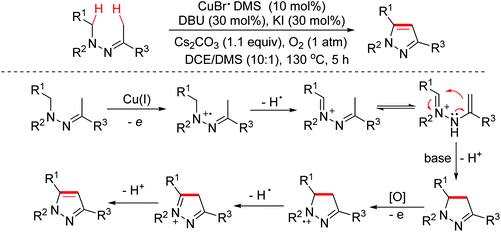 |
| Scheme 52 | |
In 2014, the Deng group reported a Cu(II)/DDQ-promoted dehydrogenative intramolecular cyclization for the straightforward synthesis of indene derivatives from (Z)-1,2,3-triaryl propylenes via OEO involved benzylic/allylic C(sp3)–H bond activation (Scheme 53).84
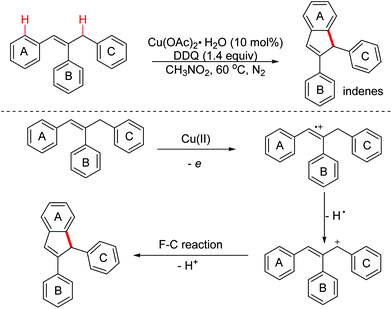 |
| Scheme 53 | |
In 2015, the Brasholz group explored a visible light induced intramolecular dehydrogenative oxidation/6π-cyclization/oxidation cascade reaction to synthesize 12-nitroindoloisoquinolines from 2-aryltetrahydroisoquinolines (Scheme 54).85
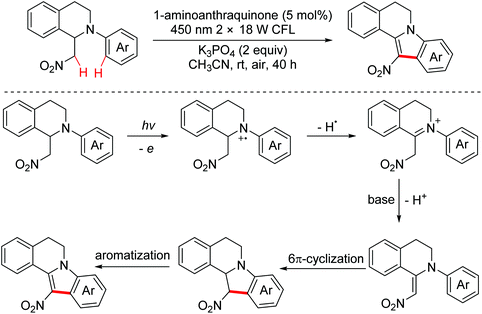 |
| Scheme 54 | |
In 2016, the Wu group described a synthetic strategy for the synthesis of highly substituted indoles via visible-light induced intramolecular C(sp2)–C(sp2) bond coupling of N-aryl enamines.86 The transformation involved one-electron oxidation and the following deprotonation to generate the key radical intermediates (Scheme 55). In 2017, an electrocatalytic dehydrogenative annulation of N-aryl enamines was developed by the Lei group.87
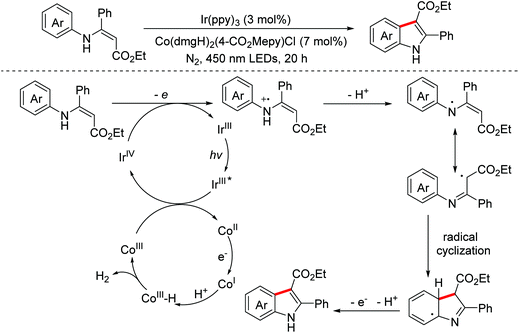 |
| Scheme 55 | |
Oxindoles are omnipresent backbones for chemical and biological applications. In 2017, the Xu group developed an electrochemical intramolecular cross-coupling reaction of C(sp3)–H and C(sp2)–H bonds from malonate amides for the straightforward construction of C3-fluorinated oxindoles using Cp2Fe as the redox catalyst.88 Mechanism research showed that in situ generated MeO− abstracted a proton of substrates to deliver their conjugated bases, which then underwent the OEO process to form the key radical intermediates (Scheme 56). Afterwards, the group employed a similar method to achieve intramolecular C(sp3)–H/C(sp2)–H cross-coupling of 1,3-dicarbonyl compounds.89
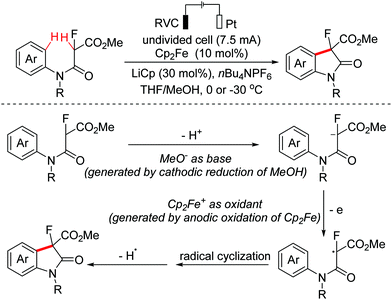 |
| Scheme 56 | |
3.1.2. C–N bond formation.
Construction of the C–N bond is always a powerful tool in organic synthetic chemistry. It is a straightforward way to synthesize nitrogen-containing compounds, which are ubiquitous motifs in medicinal and biological chemistry. Using N-centered radicals generated from N–H bond cleavage to directly form C–N bonds is an attractive approach in the synthetic community. In 2011, the Xiao group described an efficient strategy for the synthesis of highly substituted tetrahydroimidazole derivatives by means of visible-light induced intramolecular cyclization reactions of diamines.90 In this process, iminium ions were formed under visible-light promoted aerobic conditions. Then an intramolecular nucleophilic cyclization occurred to deliver tetrahydroimidazoles (Scheme 57).
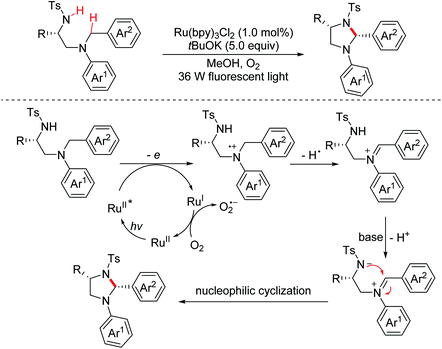 |
| Scheme 57 | |
In 2014, the Xiao group disclosed a visible-light-catalyzed intramolecular cyclization of β,γ-unsaturated hydrazones to synthesize 4,5-dihydropyrazoles (Scheme 58).91
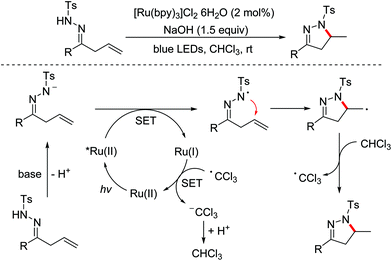 |
| Scheme 58 | |
In 2017, the Xu group demonstrated an electrochemical oxidative cleavage of N–H bonds to generate amidinyl radicals, which then underwent intramolecular cyclization to afford a host of polycyclic benzimidazoles and pyridoimidazoles (Scheme 59).92
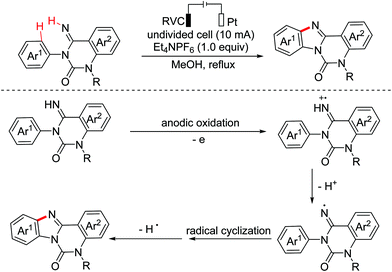 |
| Scheme 59 | |
In the same year, the Xu group also disclosed an electrochemical intramolecular oxidative amination reaction of tri- or tetrasubstituted alkenes to provide cyclic carbamates, ureas and lactams.93 The mechanistic study revealed that a nitrogen-centered radical-initiated cyclization process was involved in the transformation (Scheme 60).
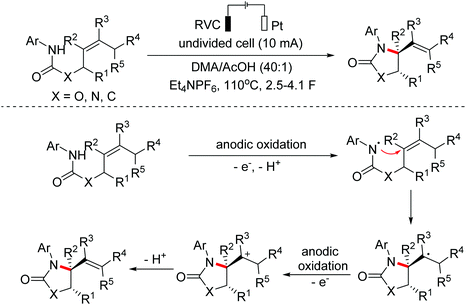 |
| Scheme 60 | |
In 2017, the Rao group explored a copper-catalyzed intramolecular C–N coupling reaction for benzoimidazo[1,2-a]indole formation. A one-electron oxidation initiated anilidyl radical pathway was proposed (Scheme 61).94
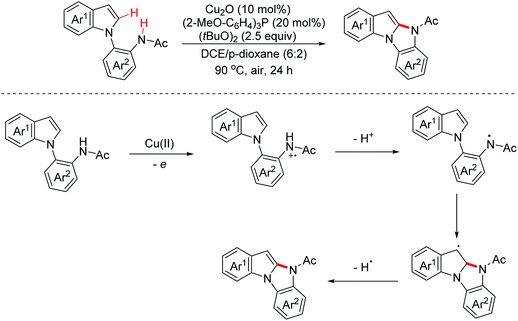 |
| Scheme 61 | |
In 2018, the Lei group reported an intramolecular electro-oxidative cross-coupling reaction of the C(sp3)–H bond and the N–H bond of amides for the construction of pyrrolidines (Scheme 62).95
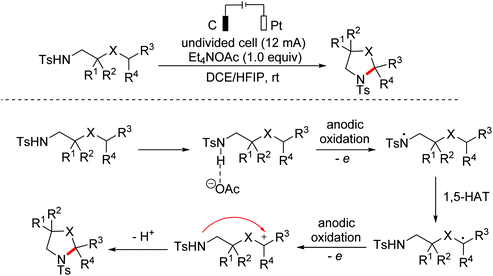 |
| Scheme 62 | |
3.1.3. C–O bond formation.
Oxazolidines, relatively stable cyclic N,O-acetals, widely exist in many active natural products and possess widespread biological and pharmaceutical activities. Therefore, many synthetic methodologies have been explored to forge these ubiquitous scaffolds. Among them, the direct oxidative functionalization of a C(sp3)–H bond next to nitrogen with alkyl O–H to construct a new C–O bond is a straightforward route. In 2006, the Okimoto group developed an electrochemical oxidative intramolecular C–H/O–H cyclization reaction of hydroquinolyl/hydroisoquinolyl alcohols toward the synthesis of fused oxazolidine derivatives.96 The key imine intermediates were formed first by one-electron oxidation and the following dehydrogenation, which were then attacked by the intramolecular hydroxyl group to complete the process (Scheme 63).
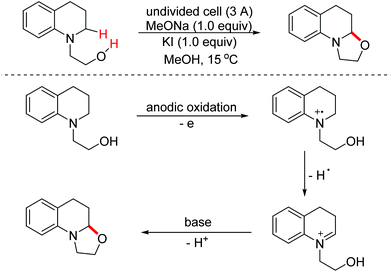 |
| Scheme 63 | |
In 2016, the Punniyamurthy group described a TBAI/T-hydro promoted oxidative cross-coupling reaction of N-alkyl C–H bonds with alkyl O–H and N–H bonds for the construction of functionalized oxazolidines and imidazolidines with high optical purity (Scheme 64).97
 |
| Scheme 64 | |
In 2017, the Waldvogel group developed an electrochemical intramolecular coupling of anilides for the synthesis of benzoxazoles via the key amidyl radical intermediates (Scheme 65).98
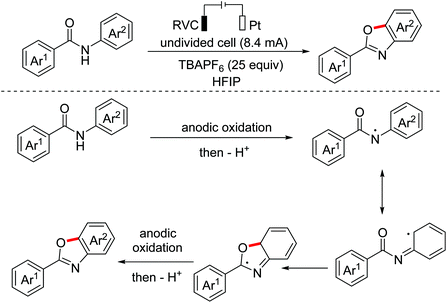 |
| Scheme 65 | |
3.1.4. C–S bond formation.
As one of the most important carbon–heteroatom bonds in organic chemistry, C–S bonds ubiquitously exist in a large number of bioactive natural products with an extensive spectrum of pharmaceutical and biological activity. In 2015, Wu and Lei et al. developed a photoredox cobalt-catalyzed C–H functionalization/C–S bond formation reaction for the construction of benzothiazoles under external oxidant-free conditions.99 The proposed mechanism involves the formation of the key sulfur-centered radical intermediates (Scheme 66).
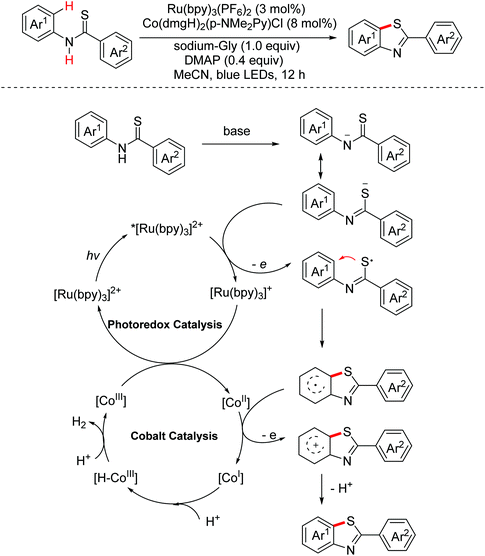 |
| Scheme 66 | |
In 2017, Song and Xu et al. disclosed a TEMPO-catalyzed electrochemical C–H thiolation reaction for the synthesis of benzothiazoles and thiazolopyridines via the thioamidyl radical intermediate pathway.100 In 2018, Wirth and Xu et al. explored the electrochemical dehydrogenative C–S bond formation in the continuous-flow method to produce thiazolopyridines via a similar procedure.101
3.2. Construction of six-membered rings
3.2.1. C–C bond formation.
Dehydrogenative C–C bond formation by converting two C–H bonds to a C–C bond is an ideal process to construct six-membered heterocyclic skeletons. Indole alkaloids are prime substructures in bioactive natural and medicinal products. The Kanai group developed a manganese(III)-catalyzed aerobic dehydrogenative intramolecular cyclization to produce ring-fused indole skeletons in 2013 (Scheme 67).102
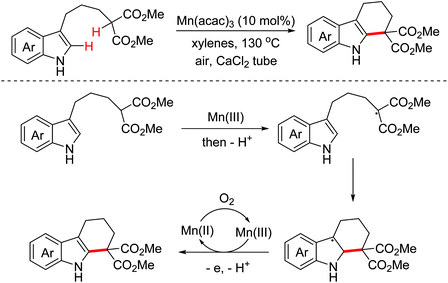 |
| Scheme 67 | |
4-Quinolone scaffolds exist in numerous marketed drug molecules. In 2015, the Long group disclosed an oxidative intramolecular Mannich reaction of secondary amines with unmodified ketones through C(sp3)–H/C(sp3)–H coupling to form valuable 4-quinolones employing TEMPO as the oxidant and tBuOK as the base (Scheme 68).103
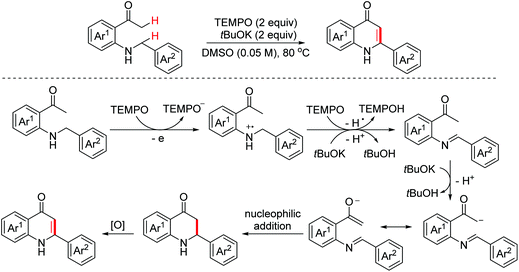 |
| Scheme 68 | |
In 2017, a CBr4 promoted intramolecular aerobic oxidative dehydrogenative arylation of aldehydes for the synthesis of xanthones and fluorenones was explored by the Huo group.104 The reaction proceeded through a radical pathway (Scheme 69).
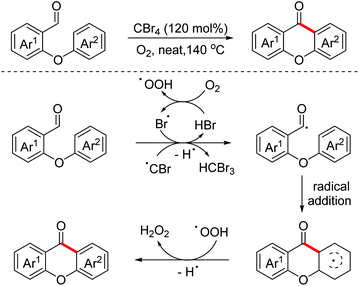 |
| Scheme 69 | |
It is worth mentioning that the activation of a C–H bond next to an oxygen atom is more difficult than the activation of a C–H bond adjacent to a nitrogen atom owing to its relatively higher oxidation potential. In 2018, the Scheidt group reported a chiral Lewis acid-catalyzed enantioselective intramolecular cross-dehydrogenative coupling to access valuable tetrahydropyrans from unfunctionalized ethers (Scheme 70).105
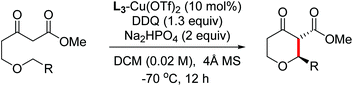 |
| Scheme 70 | |
3.2.2. C–N bond formation.
Ring-fused tetrahydroquinolines are structurally important units in various natural products. In 2013, the Toste group reported a chiral phosphate anion catalyzed enantioselective C–N bond forming reaction to produce fused tetrahydroisoquinolines via intramolecular oxidative dehydrogenative coupling.106 The formation of a tight chiral ion-pair of chiral phosphate anions and the cationic oxidant with the substrates is pivotal in the transformation (Scheme 71).
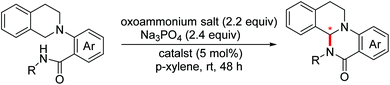 |
| Scheme 71 | |
In 2014, the Huo group demonstrated a stable radical cation salt promoted intramolecular dehydrogenative coupling reaction to deliver ring-fused tetrahydroquinoline derivatives under mild conditions.107 The OEO process was involved in the generation of the active iminium ion species (Scheme 72).
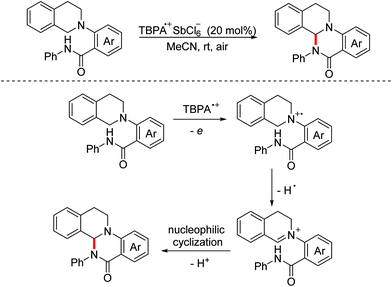 |
| Scheme 72 | |
In 2017, the Xu group developed an electrochemical catalyzed C(sp2)–H/N–H cross-coupling of biaryl aldehydes to regioselectively synthesize fused pyridines employing NH3 as a nitrogen donor.108 A plausible mechanism proposed that aldimines were formed in situ from aldehyde substrates and NH3, which then underwent anodic oxidation to generate the key aryl radical cation intermediates (Scheme 73).
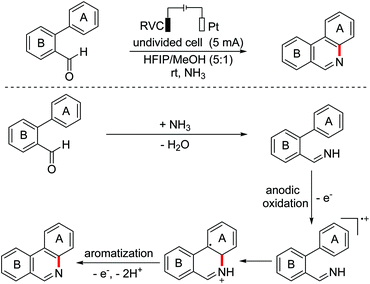 |
| Scheme 73 | |
3.2.3. C–O bond formation.
Oxygen-containing heterocycles are widely prevalent motifs in natural products and bioactive compounds, and therefore the development of efficient synthetic methods to construct them is highly desirable. Intramolecular electrochemical oxidative C–O bond formation has become a powerful tool nowadays. In 2017, the Xu group developed an intramolecular anodic dehydrogenative coupling of aromatic C–H bonds with amide groups for the synthesis of benzoxazines (Scheme 74).109
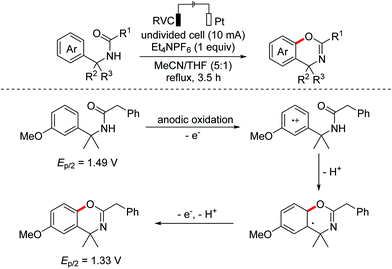 |
| Scheme 74 | |
In 2017, the Baruah group disclosed a visible-light induced intramolecular cross-dehydrogenative coupling of 1-aminoalkyl-2-naphthols for the construction of 1,3-oxazines.110 Imine intermediates were involved in the transformation (Scheme 75).
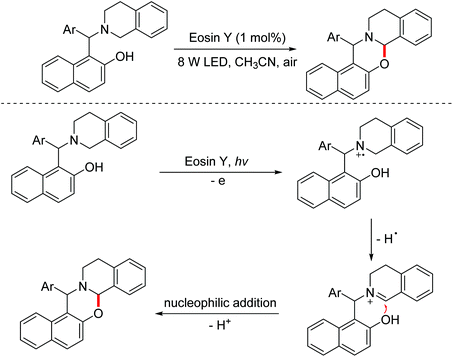 |
| Scheme 75 | |
In 2017, Gu and Wang et al. reported an I2-catalyzed intramolecular dehydrogenative C(sp3)–H and O/N–H coupling reaction for the synthesis of substituted benzoxazinones (Scheme 76).111
 |
| Scheme 76 | |
In 2018, an intramolecular anodic Kolbe oxidative/cyclization procedure of aryl phosphonic acid monoesters for the synthesis of ethoxy dibenzooxaphosphorin oxides was developed by the Mo group.112 In the key step, aryl phosphonic acids underwent Kolbe oxidation through anodic oxidation to generate phosphonic acid radicals, which were then trapped by the intramolecular aromatic ring (Scheme 77). In the same year, the same research group reported a similar C(sp2)–H functionalization/C–O bond formation reaction to afford dibenzopyranones.113
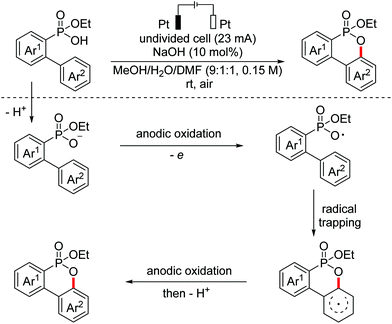 |
| Scheme 77 | |
In 2018, an electrochemical dehydrogenative lactonization reaction of 2-aryl carboxylic acids to construct benzo-3,4-coumarins was developed by the Zeng, Luo and Lei groups independently (Scheme 78).114 In 2020, the Yatham group reported a photocatalyzed version of this transformation.115
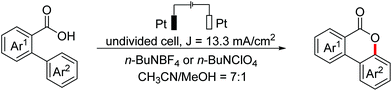 |
| Scheme 78 | |
3.3. Construction of multiple rings
Polyheterocyclic compounds widely exist in natural and synthetic compounds. In 2015, the Jia group developed a radical cation salt promoted intramolecular C(sp3)–H bond oxidation of N-aryl glycine esters with amides for the construction of lactones and lactams (Scheme 79).116 In 2019, the Jia group employed the same strategy to achieve the synthesis of polyheterocycles containing dihydroquinoline skeletons.117
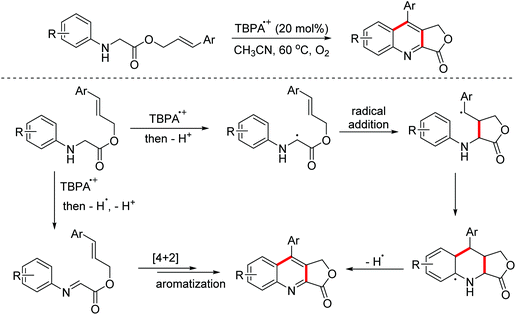 |
| Scheme 79 | |
In 2016, Lu and Xu et al. developed an electrochemical cascade cyclization to synthesize indoles and azaindoles.118 The possible mechanism is presented in Scheme 80. The in situ generated MeO−via cathodic reduction of methanol acted as a base to generate substrate anions, which then underwent the OEO process to afford nitrogen-centered radicals. Subsequently, cascade radical cyclization and the following aromatization occurred to complete the transformation. More applications of this cascade cyclization strategy were disclosed by the Xu group in 2017 and 2018.119
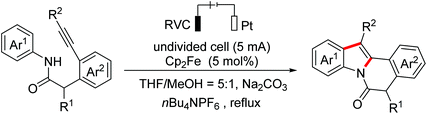 |
| Scheme 80 | |
4. Conclusion
The OEO process involved cross-dehydrogenative coupling has emerged as a concise and straightforward synthetic methodology for the construction of new C–C/C–X bonds. As one of the important puzzles, remarkable and instructive advances have been made in the construction of useful ring compounds through one-electron oxidative dehydrogenative annulation and cyclization reactions. In this review, we outlined the recent exciting progress in this area.
Despite many oxidative dehydrogenative annulation and cyclization processes being achieved by OEO approaches, this area still includes great challenges. It is still highly desirable to explore new catalytic systems and new substrates to expand the scope of the OEO cross-dehydrogenative coupling process. The synthesis of heterocycles has been intensively studied recently in this area. However, the synthetic method of carbocyclic compounds is relatively rare. Considering the requirement of green and sustainable chemistry, the development of electricity or visible-light induced OEO processes might be desirable. Asymmetric catalysis has not been well studied in oxidative dehydrogenative annulation and cyclization reactions.
Conflicts of interest
There are no conflicts to declare.
Acknowledgements
We thank the National Natural Science Foundation of China (21961033) for financially supporting this work.
References
-
(a) C. S. Yeung and V. M. Dong, Catalytic dehydrogenative cross-coupling: Forming carbon-carbon bonds by oxidizing two carbon-hydrogen bonds, Chem. Rev., 2011, 111, 1215–1292 CrossRef CAS PubMed;
(b) Y. Wei, P. Hu, M. Zhang and W. Su, Metal-catalyzed decarboxylative C–H functionalization, Chem. Rev., 2017, 117, 8864–8907 CrossRef CAS PubMed;
(c) Y. Qin, L. Zhu and S. Luo, Organocatalysis in inert C–H bond functionalization, Chem. Rev., 2017, 117, 9433–9520 CrossRef CAS PubMed;
(d) L. Ping, D. S. Chung, J. Bouffard and S. Lee, Transition metal-catalyzed site- and regio-divergent C–H bond functionalization, Chem. Soc. Rev., 2017, 46, 4299–4328 RSC.
- C.-J. Li, Cross-dehydrogenative coupling (CDC): exploring C–C bond formations beyond functional group transformations, Acc. Chem. Res., 2009, 42, 335–344 CrossRef CAS PubMed.
- A. S. Hay, Oxidative coupling of acetylenes. II1, J. Org. Chem., 1962, 27, 3320–3321 CrossRef CAS.
-
(a) C. Liu, J. Yuan, M. Gao, S. Tang, W. Li, R. Shi and A. Lei, Oxidative coupling between two hydrocarbons: An update of recent C–H functionalizations, Chem. Rev., 2015, 115, 12138–12204 CrossRef CAS PubMed;
(b) M. S. Segundo and A. Correa, Cross-dehydrogenative coupling reactions for the functionalization of α-amino acid derivatives and peptides, Synthesis, 2018, 2853–2866 Search PubMed;
(c) C.-Y. Huang, H. Kang, J. Li and C.-J. Li, En route to intermolecular cross-dehydrogenative coupling reactions, J. Org. Chem., 2019, 84, 12705–12721 CrossRef CAS PubMed;
(d) H. Yi, G. Zhang, H. Wang, Z. Huang, J. Wang, A. K. Singh and A. Lei, Recent advances in radical C–H activation/radical cross-coupling, Chem. Rev., 2017, 117, 9016–9085 CrossRef CAS PubMed.
-
(a) N. L. Bauld, D. J. Bellville, B. Harirchian, K. T. Lorenz, R. A. Pabon, D. W. Reynolds, D. D. Wirth, H. S. Chiou and B. K. Marsh, Cation radical pericyclic reactions, Acc. Chem. Res., 1987, 20, 371–378 CrossRef CAS;
(b) N. L. Bauld, Cation radical cycloadditions and related sigmatropic reactions, Tetrahedron, 1989, 45, 5307–5363 CrossRef CAS;
(c) R. Popielarz and D. R. Arnold, Radical ions in photochemistry. Part 24. Carbon-carbon bond cleavage of radical cations in solution: theory and application, J. Am. Chem. Soc., 1990, 112, 3068–3082 CrossRef CAS;
(d) C. Zhang, C. Tang and N. Jiao, Recent advances in copper-catalyzed dehydrogenative functionalization via a single electron transfer (set) process, Chem. Soc. Rev., 2012, 41, 3464–3484 RSC;
(e) A. Gini, T. Brandhofer and O. G. Mancheño, Recent progress in mild Csp3–H bond dehydrogenative or (mono-) oxidative functionalization, Org. Biomol. Chem., 2017, 15, 1294–1312 RSC.
- J. W. Beatty and C. R. J. Stephenson, Amine functionalization via oxidative photoredox catalysis: methodology development and complex molecule synthesis, Acc. Chem. Res., 2015, 48, 1474–1484 CrossRef CAS PubMed.
-
(a) S. A. Girard, T. Knauber and C.-J. Li, The cross-dehydrogenative coupling of Csp3–H bonds: A versatile strategy for C–C bond formations, Angew. Chem., Int. Ed., 2014, 53, 74–100 CrossRef CAS PubMed;
(b) R. Narayan, K. Matcha and A. P. Antonchick, Metal-free oxidative C–C bond formation through C–H bond functionalization, Chem. – Eur. J., 2015, 21, 14678–14693 CrossRef CAS PubMed;
(c) M. K. Lakshman and P. K. Vuram, Cross-dehydrogenative coupling and oxidative-amination reactions of ethers and alcohols with aromatics and heteroaromatics, Chem. Sci., 2017, 8, 5845–5888 RSC;
(d) S. Guo, P. S. Kumar and M. Yang, Recent advances of oxidative radical cross-coupling reactions: Direct α-C(sp3)–H bond functionalization of ethers and alcohols, Adv. Synth. Catal., 2017, 359, 2–25 CrossRef CAS;
(e) X.-Q. Hu, J.-R. Chen and W.-J. Xiao, Controllable remote C–H bond functionalization by visible-light photocatalysis, Angew. Chem., Int. Ed., 2017, 56, 1960–1962 CrossRef CAS PubMed;
(f) S. Tang, Y. Liu and A. Lei, Electrochemical oxidative cross-coupling with hydrogen evolution: A green and sustainable way for bond formation, Chem, 2018, 4, 27–45 CrossRef CAS;
(g) M. D. Kärkäs, Electrochemical strategies for C–H functionalization and C–N bond formation, Chem. Soc. Rev., 2018, 47, 5786–5865 RSC;
(h) Y. Zhao and W. Xia, Recent advances in radical-based C–N bond formation via photo-/electrochemistry, Chem. Soc. Rev., 2018, 47, 2591–2608 RSC;
(i) H. Wang, X. Gao, Z. Lv, T. Abdelilah and A. Lei, Recent advances in oxidative R1-H/R2-H cross-coupling with hydrogen evolution via photo-/electrochemistry, Chem. Rev., 2019, 119, 6769–6787 CrossRef CAS PubMed;
(j) J. C. Siu, N. Fu and S. Lin, Catalyzing electrosynthesis: A homogeneous electrocatalytic approach to reaction discovery, Acc. Chem. Res., 2020, 53, 547–560 CrossRef CAS PubMed.
-
(a) M. Froimowitz, K.-M. Wu, A. Moussa, R. M. Haidar, J. Jurayj, C. George and E. L. Gardner, Slow-onset, long-duration 3-(3′,4′-dichlorophenyl)-1-indanamine monoamine reuptake blockers as potential medications to treat cocaine abuse, J. Med. Chem., 2000, 43, 4981–4992 CrossRef CAS PubMed;
(b) G. G. Bianco, H. M. C. Ferraz, A. M. Costa, L. V. Costa-Lotufo, C. Pessoa, M. O. de Moraes, M. G. Schrems, A. Pfaltz and L. F. Silva, (+)- and (–)-mutisianthol: First total synthesis, absolute configuration, and antitumor activity, J. Org. Chem., 2009, 74, 2561–2566 CrossRef CAS PubMed;
(c) C. C. Nawrat and C. J. Moody, Quinones as dienophiles in the Diels-Alder reaction: history and applications in total synthesis, Angew. Chem., Int. Ed., 2014, 53, 2056–2077 CrossRef CAS PubMed;
(d) X.-X. Guo, D.-W. Gu, Z. Wu and W. Zhang, Copper-catalyzed C–H functionalization reactions: efficient synthesis of heterocycles, Chem. Rev., 2015, 115, 1622–1651 CrossRef CAS PubMed.
-
(a)
D. Lednicer and L. A. Mitscher, The organic chemistry of drug synthesis, John Wiley & Sons, Inc., 1980, vol. 2, pp. 207–231 Search PubMed;
(b) S. Minakata, Utilization of N–X bonds in the synthesis of N-heterocycles, Acc. Chem. Res., 2009, 42, 1172–1182 CrossRef CAS PubMed;
(c) B. Godoi, R. F. Schumacher and G. Zeni, Synthesis of heterocycles via electrophilic cyclization of alkynes containing heteroatom, Chem. Rev., 2011, 111, 2937–2980 CrossRef CAS PubMed;
(d) I. Yavari and A. Khajeh-Khezri, Recent advances in the synthesis of hetero- and carbocyclic compounds- and complexes based on acenaphthylene-1,2-dione, Synthesis, 2018, 3947–3973 CrossRef CAS;
(e) N. G. Léonard, W. N. Palmer, M. R. Friedfeld, M. J. Bezdek and P. J. Chirik, Remote, diastereoselective cobalt-catalyzed alkene isomerization-hydroboration: Access to stereodefined 1,3-difunctionalized indanes, ACS Catal., 2019, 9, 9034–9044 CrossRef.
- H. Hou, S. Zhu, F. Pan and M. Rueping, Visible-light photoredox-catalyzed synthesis of nitrones: unexpected rate acceleration by water in the synthesis of isoxazolidines, Org. Lett., 2014, 16, 2872–2875 CrossRef CAS PubMed.
- N. Zhou, T. Xie, L. Liu and Z. Xie, Cu/Mn co-oxidized cyclization for the synthesis of highly substituted pyrrole derivatives from amino acid esters: A strategy for the biomimetic syntheses of lycogarubin C and chromopyrrolic acid, J. Org. Chem., 2014, 79, 6061–6068 CrossRef CAS PubMed.
- Q.-H. Deng, Y.-Q. Zou, L.-Q. Lu, Z.-L. Tang, J.-R. Chen and W.-J. Xiao, De novo synthesis of imidazoles by visible-light-induced photocatalytic aerobic oxidation/[3 + 2] cycloaddition/aromatization cascade, Chem. – Asian J., 2014, 9, 2432–2435 CrossRef CAS PubMed.
- Y.-J. Li, X. Li, S.-X. Zhang, Y.-L. Zhao and Q. Liu, Copper(II)-catalyzed oxidative [3 + 2] cycloaddition reactions of secondary amines with α-diazo compounds: a facile and efficient synthesis of 1,2,3-triazoles, Chem. Commun., 2015, 51, 11564–11567 RSC.
- C. Huo, Y. Yuan, F. Chen, J. Tang and Y. Wang, Copper-catalyzed aerobic oxidative dehydrogenative formal [2 + 3] cyclization of glycine esters with α-angelicalactone: approach to construct polysubstituted pyrrolidones, Org. Lett., 2015, 17, 4208–4211 CrossRef CAS PubMed.
- H. Zhou, X. Yang, S. Li, Y. Zhu, Y. Li and Y. Zhang, Visible light-induced aerobic oxidative cross-coupling of glycine esters with α-angelicalactone: a facile pathway to γ-lactams, Org. Biomol. Chem., 2018, 16, 6728–6734 RSC.
- J. Xie, Y. Huang, H. Song, Y. Liu and Q. Wang, Copper-catalyzed aerobic oxidative [2 + 3] cyclization/aromatization cascade reaction: atom-economical access to tetrasubstituted 4,5-biscarbonyl imidazoles, Org. Lett., 2017, 19, 6056–6059 CrossRef CAS PubMed.
- V. Bhajammanavar, S. Mallik and M. Baidya, Oxidative cross-dehydrogenative [2 + 3] annulation of α-amino ketones with α-keto esters: Concise synthesis of clausenamide analogues, Org. Biomol. Chem., 2019, 17, 1740–1743 RSC.
- M. Sengoden, A. Bhowmick and T. Punniyamurthy, Stereospecific copper-catalyzed domino ring opening and sp3 C–H functionalization of activated aziridines with N-alkylanilines, Org. Lett., 2017, 19, 158–161 CrossRef CAS PubMed.
- H. Li, S. Huang, Y. Wang and C. Huo, Oxidative dehydrogenative [2 + 3]-cyclization of glycine esters with aziridines leading to imidazolidines, Org. Lett., 2018, 20, 92–95 CrossRef CAS PubMed.
- X. Yang, Y. Zhu, Z. Xie, Y. Li and Y. Zhang, Visible-light-induced charge transfer enables Csp3–H functionalization of glycine derivatives: Access to 1,3-oxazolidines, Org. Lett., 2020, 22, 1638–1643 CrossRef CAS PubMed.
- C.-B. Miao, A.-Q. Zheng, L.-J. Zhou, X. Lyu and H.-T. Yang, Copper-catalyzed annulation of oxime acetates with α-amino acid ester derivatives: Synthesis of 3-sulfonamido/imino 4-pyrrolin-2-ones, Org. Lett., 2020, 22, 3381–3385 CrossRef CAS PubMed.
- Z. Qu, F. Zhang, G.-J. Deng and H. Huang, Regioselectivity control in the oxidative formal [3 + 2] annulations of ketoxime acetates and tetrohydroisoquinolines, Org. Lett., 2019, 21, 8239–8243 CrossRef CAS PubMed.
- K. Liu, S. Tang, P. Huang and A. Lei, External oxidant-free electrooxidative [3 + 2] annulation between phenol and indole derivatives, Nat. Commun., 2017, 8, 775 CrossRef PubMed.
- Q. Zhao, J.-K. Jin, J. Wang, F.-L. Zhang and Y.-F. Wang, Radical α-addition involved electrooxidative [3 + 2] annulation of phenols and electron-deficient alkenes, Chem. Sci., 2020, 11, 3909–3913 RSC.
- T. Liang, L. Gong, H. Zhao, H. Jiang and M. Zhang, Straightforward access to novel indolo[2,3-b]indoles via aerobic copper-catalyzed [3 + 2] annulation of diarylamines and indoles, Chem. Commun., 2020, 56, 2807–2810 RSC.
- Y. Wang, R. Wu, S. Zhao, Z. Quan, Y. Su and C. Huo, Air promoted annulation of thiophenols with alkynes leading to benzothiophenes, Org. Biomol. Chem., 2018, 16, 1667–1671 RSC.
- Z. Xu, C. Zhang and N. Jiao, Synthesis of oxazoles through copper-mediated aerobic oxidative dehydrogenative annulation and oxygenation of aldehydes and amines, Angew. Chem., Int. Ed., 2012, 51, 11367–11370 CrossRef CAS PubMed.
- J. Li, Y. Wang, W. Li, H. Wang, D. Mo and Y. Pan, A facile synthesis of 2,5-disubstituted oxazoles via a copper-catalyzed cascade reaction of alkenes with azides, Chem. Commun., 2015, 51, 17772–17774 RSC.
-
(a) F. Zhang, J. Wang, X. Zhang, X. Meng and B. Chen, A regioselective synthesis of 2,5-diaryl oxazoles via TsOH/I2-mediated cascade cyclization, ChemistrySelect, 2017, 2, 8717–8720 CrossRef CAS;
(b) J. Pan, X. Li, X. Qiu, X. Luo and N. Jiao, Copper-catalyzed oxygenation approach to oxazoles from amines, alkynes, and molecular oxygen, Org. Lett., 2018, 20, 2762–2765 CrossRef CAS PubMed.
- X. Wang, C.-Y. Zhang, H.-Y. Tu and A.-D. Zhang, Facile access to multiaryl-1H-pyrrol-2(3H)-ones by copper/TEMPO-mediated cascade annulation of diarylethanones with primary amines and mechanistic insight, Eur. J. Org. Chem., 2016, 5243–5247 CrossRef CAS.
- J.-N. Zhu, L.-L. Chen, R.-X. Zhou, B. Li, Z.-Y. Shao and S.-Y. Zhao, Copper-catalyzed oxidative cyclization of maleimides with amines and alkyne esters: Direct access to fully substituted dihydropyrroles and pyrrole derivatives, Org. Lett., 2017, 19, 6044–6047 CrossRef CAS PubMed.
- J.-C. Zeng, H. Xu, R.-L. Huang, F. Yu and Z. Zhang, Facile synthesis of polysubstituted 2,3-dihydropyrroles and pyrroles from Mn(OAc)3-promoted oxidative cyclization of alkenes with amines/alkyne esters or enaminone esters, Tetrahedron Lett., 2018, 59, 1576–1580 CrossRef CAS.
- Z.-J. Wu, S.-R. Li and H.-C. Xu, Synthesis of N-heterocycles by dehydrogenative annulation of N-allyl amides with 1,3-dicarbonyl compounds, Angew. Chem., Int. Ed., 2018, 57, 14070–14074 CrossRef CAS PubMed.
- L. Kumari, Salahuddin, A. Mazumder, D. Pandey, M. S. Yar, R. Kumar, R. Mazumder, M. Sarafroz, M. J. Ahsan, V. Kumar and S. Gupta, Synthesis and biological potentials of quinoline analogues: A review of literature, Mini-Rev. Org. Chem., 2019, 16, 653–688 CrossRef CAS.
- H. Richter and O. G. Mancheño, TEMPO oxoammonium salt-mediated dehydrogenative povarov/oxidation tandem reaction of N-alkyl anilines, Org. Lett., 2011, 13, 6066–6069 CrossRef CAS PubMed.
- P. Liu, Z. Wang, J. Lin and X. Hu, An efficient route to quinolines and other compounds by iron-catalysed cross-dehydrogenative coupling reactions of glycine derivatives, Eur. J. Org. Chem., 2012, 1583–1589 CrossRef CAS.
-
(a) X. Jia, F. Peng, C. Qing, C. Huo and X. Wang, Catalytic radical cation salt induced Csp3–H functionalization of glycine derivatives: synthesis of substituted quinolines, Org. Lett., 2012, 14, 4030–4033 CrossRef CAS PubMed;
(b) X. Jia, Y. Wang, F. Peng, C. Huo, L. Yu, J. Liu and X. Wang, Catalytic sp3 C–H oxidation of peptides and their analogues by radical cation salts: From glycine amides to quinolines, J. Org. Chem., 2013, 78, 9450–9456 CrossRef CAS PubMed;
(c) J. Liu, Y. Wang, L. Yu, C. Huo, X. Wang and X. Jia, Cerium(IV)-catalyzed sp3 C–H bond oxidation of glycine derivatives: Radical cation prompted dioxygen activation in the presence of triarylamine, Adv. Synth. Catal., 2014, 356, 3214–3218 CrossRef CAS;
(d) X. Liu, Y. Shao, P. Li, H. Ji, Y. Yuan and X. Jia, TBN as a metal-free reagent initiated sp3 C–H functionalization of glycine esters: synthesis of quinoline-2-carboxylate esters, Tetrahedron Lett., 2018, 59, 637–640 CrossRef CAS.
- C. Huo, Y. Yuan, M. Wu, X. Jia, X. Wang, F. Chen and J. Tang, Auto-oxidative coupling of glycine derivatives, Angew. Chem., Int. Ed., 2014, 53, 13544–13547 CrossRef CAS PubMed.
- C. Huo, H. Xie, M. Wu, X. Jia, X. Wang, F. Chen and J. Tang, CBr4-mediated cross-dehydrogenative coupling reaction of amines, Chem. – Eur. J., 2015, 21, 5723–5726 CrossRef CAS PubMed.
- G. Liu, J. Qian, J. Hua, F. Cai, X. Li and L. Liu, An economical synthesis of substituted quinoline-2-carboxylates through the potassium persulfate-mediated cross-dehydrogenative coupling of N-aryl glycine derivatives with olefins, Org. Biomol. Chem., 2016, 14, 1147–1152 RSC.
- Z. Xie, J. Jia, X. Liu and L. Liu, Copper(II) triflate-catalyzed aerobic oxidative C–H functionalization of glycine derivatives with olefins and organoboranes, Adv. Synth. Catal., 2016, 358, 919–925 CrossRef CAS.
- M. Ni, Y. Zhang, T. Gong and B. Feng, Gold-oxazoline complex-catalyzed cross-dehydrogenative coupling of glycine derivatives and alkenes, Adv. Synth. Catal., 2017, 359, 824–831 CrossRef CAS.
- X. Yang, L. Li, Y. Li and Y. Zhang, Visible-light-induced photocatalytic aerobic oxidative Csp3–H functionalization of glycine derivatives: Synthesis of substituted quinolines, J. Org. Chem., 2016, 81, 12433–12442 CrossRef CAS PubMed.
- Q.-Y. Meng, X.-W. Gao, T. Lei, Z. Liu, F. Zhan, Z.-J. Li, J.-J. Zhong, H. Xiao, K. Feng, B. Chen, Y. Tao, C.-H. Tung and L.-Z. Wu, Identifying key intermediates generated in situ from Cu(II) salt-catalyzed C–H functionalization of aromatic amines under illumination, Sci. Adv., 2017, 3, e1700666 CrossRef PubMed.
- X. Jia, W. Hou, Y. Shao, Y. Yuan, Q. Chen, P. Li, X. Liu and H. Ji, A consecutive C–H functionalization triggered by oxidation of active sp3 C–H bonds: construction of 3,4-dihydroquinoline-3-one derivatives, Chem. – Eur. J., 2017, 23, 12980–12984 CrossRef CAS PubMed.
- S. Huang, X. Bao, Y. Fu, Y. Zhang, Z. Quan and C. Huo, Auto-oxidative Povarov/aromatization tandem reaction of glycine derivatives with enamides: acylamino as both activating and leaving group, Asian J. Org. Chem., 2020, 9, 925–928 CrossRef CAS.
- E. Schendera, L.-N. Unkel, P. P. Huyen Quyen, G. Salkewitz, F. Hoffmann, A. Villinger and M. Brasholz, Visible-light-mediated aerobic tandem dehydrogenative povarov/aromatization reaction: Synthesis of isocryptolepines, Chem. – Eur. J., 2020, 26, 269–274 CrossRef CAS PubMed.
- P. Liu, Y. Li, H. Wang, Z. Wang and X. Hu, Synthesis of substituted quinolines by iron-catalyzed oxidative coupling reactions, Tetrahedron Lett., 2012, 53, 6654–6656 CrossRef CAS.
-
(a) J. Liu, F. Liu, Y. Zhu, X. Ma and X. Jia, Oxidative povarov reaction via sp3 C–H oxidation of N-benzylanilines induced by catalytic radical cation salt: synthesis of 2,4-diarylquinoline derivatives, Org. Lett., 2015, 17, 1409–1412 CrossRef CAS PubMed;
(b) J. Yang, X. Meng, K. Lu, Z. Lu, M. Huang, C. Wang and F. Sun, Acid-promoted iron-catalysed dehydrogenative [4 + 2] cycloaddition for the synthesis of quinolines under air, RSC Adv., 2018, 8, 31603–31607 RSC.
- C. Min, A. Sanchawala and D. Seidel, Dual C–H functionalization of N-aryl amines: Synthesis of polycyclic amines via an oxidative povarov approach, Org. Lett., 2014, 16, 2756–2759 CrossRef CAS PubMed.
- C. Huo, F. Chen, Z. Quan, J. Dong and Y. Wang, Cobalt-catalyzed aerobic oxidative povarov reaction of tertiary anilines with dihydrofuran for the synthesis of hexahydrofuroquinolines, Tetrahedron Lett., 2016, 57, 5127–5131 CrossRef CAS.
- M.-N. Zhao, L. Yu, R.-R. Hui, Z.-H. Ren, Y.-Y. Wang and Z.-H. Guan, Iron-catalyzed dehydrogenative [4 + 2] cycloaddition of tertiary anilines and enamides for the synthesis of tetrahydroquinolines with amido-substituted quaternary carbon centers, ACS Catal., 2016, 6, 3473–3477 CrossRef CAS.
- P. Huang, P. Wang, S. Wang, S. Tang and A. Lei, Electrochemical oxidative [4 + 2] annulation of tertiary anilines and alkenes for the synthesis of tetrahydroquinolines, Green Chem., 2018, 20, 4870–4874 RSC.
- M. Nishino, K. Hirano, T. Satoh and M. Miura, Copper-catalyzed oxidative direct cyclization of N-methylanilines with electron-deficient alkenes using molecular oxygen, J. Org. Chem., 2011, 76, 6447–6451 CrossRef CAS PubMed.
- T. P. Nicholls, G. E. Constable, J. C. Robertson, M. G. Gardiner and A. C. Bissember, Brønsted acid cocatalysis in copper(I)-photocatalyzed α-amino C–H bond functionalization, ACS Catal., 2016, 6, 451–457 CrossRef CAS.
-
(a) A. K. Yadav and L. D. S. Yadav, Intermolecular cyclization of N-methylanilines and maleimides to tetrahydroquinolines via K2S2O8 promoted C(sp3)–H activation, Tetrahedron Lett., 2016, 57, 1489–1491 CrossRef CAS;
(b) J.-R. Xin, J.-T. Guo, D. Vigliaturo, Y.-H. He and Z. Guan, Metal-free visible light driven synthesis of tetrahydroquinoline derivatives utilizing Rose Bengal, Tetrahedron, 2017, 73, 4627–4633 CrossRef CAS;
(c) J.-T. Guo, D.-C. Yang, Z. Guan and Y.-H. He, Chlorophyll-catalyzed visible-light-mediated synthesis of tetrahydroquinolines from N,N-dimethylanilines and maleimides, J. Org. Chem., 2017, 82, 1888–1894 CrossRef CAS PubMed.
- X.-L. Yang, J.-D. Guo, T. Lei, B. Chen, C.-H. Tung and L.-Z. Wu, Oxidative cyclization synthesis of tetrahydroquinolines and reductive hydrogenation of maleimides under redox-neutral conditions, Org. Lett., 2018, 20, 2916–2920 CrossRef CAS PubMed.
- C.-W. Hsu and H. Sundén,
α-aminoalkyl radical addition to maleimides via electron donor-acceptor complexes, Org. Lett., 2018, 20, 2051–2054 CrossRef CAS PubMed.
- X. Hu, G. Zhang, F. Bu and A. Lei, Selective oxidative [4 + 2] imine/alkene annulation with H2 liberation induced by photo-oxidation, Angew. Chem., Int. Ed., 2018, 57, 1286–1290 CrossRef CAS PubMed.
- C. Song, K. Liu, X. Jiang, X. Dong, Y. Weng, C.-W. Chiang and A. Lei, Electrooxidation enables selective dehydrogenative [4 + 2] annulation between indole derivatives, Angew. Chem., Int. Ed., 2020, 59, 7193–7197 CrossRef CAS PubMed.
- M.-X. He, Z.-Y. Mo, Z.-Q. Wang, S.-Y. Cheng, R.-R. Xie, H.-T. Tang and Y.-M. Pan, Electrochemical synthesis of 1-naphthols by intermolecular annulation of alkynes with 1,3-dicarbonyl compounds, Org. Lett., 2020, 22, 724–728 CrossRef CAS PubMed.
- C. Huo, Y. Yuan, F. Chen and Y. Wang, A catalytic approach to quinoline fused lactones and lactams from glycine derivatives, Adv. Synth. Catal., 2015, 357, 3648–3654 CrossRef CAS.
-
(a) X. Ma, Y. Zhu, S. Lü, L. Zhang, L. Luo and X. Jia, Construction of quinoline-fused lactones and 2,3-disubstituted quinolines via catalytic aerobic sp3 C–H oxidation: application of fragment-reassembly strategy, Tetrahedron Lett., 2016, 57, 1528–1531 CrossRef CAS;
(b) Y. He, B. Yan, H. Tao, Y. Zhang and Y. Li, Metal-free photocatalyzed aerobic oxidative Csp3–H functionalization of glycine derivatives: One-step generation of quinoline-fused lactones, Org. Biomol. Chem., 2018, 16, 3816–3823 RSC.
- R. Rohlmann, T. Stopka, H. Richter and O. G. Mancheño, Iron-catalyzed oxidative tandem reactions with TEMPO oxoammonium salts: synthesis of dihydroquinazolines and quinolines, J. Org. Chem., 2013, 78, 6050–6064 CrossRef CAS PubMed.
- L. Luo, X. Zhao, L. Zhang, Y. Yuan, S. Lü and X. Jia, An aerobic oxidative aza-[4 + 2] cycloaddition induced by radical cation salt: synthesis of dihydroquinazoline derivatives, Tetrahedron Lett., 2016, 57, 5830–5833 CrossRef CAS.
- X. Jia, S. Lü, Y. Yuan, X. Zhang, L. Zhang and L. Luo, A dual removable activating group enabled the povarov reaction of N-arylalanine esters: Synthesis of quinoline-4-carboxylate esters, Org. Biomol. Chem., 2017, 15, 2931–2937 RSC.
- H. Guo, C. Zhu, J. Li, G. Xu and J. Sun, Photo-assisted multi-component reactions (MCR): A new entry to 2-pyrimidinethiones, Adv. Synth. Catal., 2014, 356, 2801–2806 CrossRef CAS.
- J. Ye, Y. Lin, Q. Liu, D. Xu, F. Wu, B. Liu, Y. Gao and H. Chen, Biomimetic oxidative coupling cyclization enabling rapid construction of isochromanoindolenines, Org. Lett., 2018, 20, 5457–5460 CrossRef CAS PubMed.
- R. K. Kawade, D. B. Huple, R.-J. Lin and R.-S. Liu, Cu-catalyzed oxidative povarov reactions between N-alkyl N-methylanilines and saturated oxa- and thiacycles, Chem. Commun., 2015, 51, 6625–6628 RSC.
- C. Huo, F. Chen, Y. Yuan, H. Xie and Y. Wang, Iron catalyzed dual-oxidative dehydrogenative (DOD) tandem annulation of glycine derivatives with tetrahydrofurans, Org. Lett., 2015, 17, 5028–5031 CrossRef CAS PubMed.
- C. Huo, H. Xie, F. Chen, J. Tang and Y. Wang, Double-oxidative dehydrogenative (DOD) cyclization of glycine derivatives with dioxane under metal-free aerobic conditions, Adv. Synth. Catal., 2016, 358, 724–730 CrossRef CAS.
- W. Jiang, Y. Wang, P. Niu, Z. Quan, Y. Su and C. Huo, Double-oxidative dehydrogenative (DOD) [4 + 2]-cyclization/oxidative aromatization tandem reaction of glycine derivatives with ethylbenzenes, Org. Lett., 2018, 20, 4649–4653 CrossRef CAS PubMed.
- W. Jiang, S. Wan, Y. Su and C. Huo, Double-oxidative dehydrogenative [4 + 2]-cyclization/dehydrogenation/oxygenation tandem reaction of N-arylglycine derivatives with cumenes, J. Org. Chem., 2019, 84, 8232–8241 CrossRef CAS PubMed.
- J.-L. Zhan, M.-W. Wu, F. Chen and B. Han, Cu-catalyzed [3 + 3] annulation for the synthesis of pyrimidines via β-C(sp3)–H functionalization of saturated ketones, J. Org. Chem., 2016, 81, 11994–12000 CrossRef CAS PubMed.
- G. Chen, Z. Wang, X. Zhang and X. Fan, Synthesis of functionalized pyridines via Cu(II)-catalyzed one-pot cascade reactions of inactivated saturated ketones with electron-deficient enamines, J. Org. Chem., 2017, 82, 11230–11237 CrossRef CAS PubMed.
- D. Cheng, L. Wu, H. Lv, X. Xu and J. Yan, CDC reaction and subsequent cyclization for the synthesis of 2-hydroxy-3-alkyl-1,4-naphthoquinones and pyranonaphthoquinones, J. Org. Chem., 2017, 82, 1610–1617 CrossRef CAS PubMed.
- H. Li, J. Liang and C. Huo, Oxidative dehydrogenative [3 + 3] annulation of benzylhydrazines with aziridines leading to tetrahydrotriazines, Chin. J. Chem., 2019, 37, 878–882 CrossRef CAS.
- X. Jia, Y. Wang, F. Peng, C. Huo, L. Yu, J. Liu and X. Wang, Catalytic oxidation of C(sp3)-H bonds induced by a radical cation salt: construction of 1,4-dihydropyridines using a fragment-reassembly strategy, Adv. Synth. Catal., 2014, 356, 1210–1216 CrossRef CAS.
- Z.-Q. Zhu, Z.-B. Xie and Z.-G. Le, Copper-catalyzed aerobic cascade oxidative coupling/cyclization for the construction of 1,4-dihydropyridine derivatives, J. Org. Chem., 2016, 81, 9449–9454 CrossRef CAS PubMed.
- S. Manna and A. P. Antonchick, Copper-catalyzed (2 + 1) annulation of acetophenones with maleimides: Direct synthesis of cyclopropanes, Angew. Chem., Int. Ed., 2015, 54, 14845–14848 CrossRef CAS PubMed.
- J. Li, W. Huang, J. Chen, L. He, X. Cheng and G. Li, Electrochemical aziridination by alkene activation using a sulfamate as the nitrogen source, Angew. Chem., Int. Ed., 2018, 57, 5695–5698 CrossRef CAS PubMed.
- G.-Q. Xu, J.-T. Xu, Z.-T. Feng, H. Liang, Z.-Y. Wang, Y. Qin and P.-F. Xu, Dual C(sp3)–H bond functionalization of N-heterocycles through sequential visible-light photocatalyzed dehydrogenation/[2 + 2] cycloaddition reactions, Angew. Chem., Int. Ed., 2018, 57, 5110–5114 CrossRef CAS PubMed.
- G. Zhang, Y. Zhao and H. Ge, Copper-catalyzed aerobic intramolecular dehydrogenative cyclization of N,N-disubstituted hydrazones through C–H functionalization, Angew. Chem., Int. Ed., 2013, 52, 2559–2563 CrossRef CAS PubMed.
- Y. Li, L. Cao, X. Luo and W.-P. Deng, The synthesis of 1,2-diarylindenes via DDQ-mediated dehydrogenative intramolecular cyclization, Tetrahedron, 2014, 70, 5974–5979 CrossRef CAS.
- F. Rusch, L.-N. Unkel, D. Alpers, F. Hoffmann and M. Brasholz, A visible light photocatalytic cross-dehydrogenative coupling/dehydrogenation/6π-cyclization/oxidation cascade: Synthesis of 12-nitroindoloisoquinolines from 2-aryltetrahydroisoquinolines, Chem. – Eur. J., 2015, 21, 8336–8340 CrossRef CAS PubMed.
- C.-J. Wu, Q.-Y. Meng, T. Lei, J.-J. Zhong, W.-Q. Liu, L.-M. Zhao, Z.-J. Li, B. Chen, C.-H. Tung and L.-Z. Wu, An oxidant-free strategy for indole synthesis via intramolecular C–C bond construction under visible light irradiation: Cross-coupling hydrogen evolution reaction, ACS Catal., 2016, 6, 4635–4639 CrossRef CAS.
- S. Tang, X. Gao and A. Lei, Electrocatalytic intramolecular oxidative annulation of N-aryl enamines into substituted indoles mediated by iodides, Chem. Commun., 2017, 53, 3354–3356 RSC.
- Z.-J. Wu and H.-C. Xu, Synthesis of C3-fluorinated oxindoles through reagent-free cross-dehydrogenative coupling, Angew. Chem., Int. Ed., 2017, 56, 4734–4738 CrossRef CAS PubMed.
- Z.-J. Wu, S.-R. Li, H. Long and H.-C. Xu, Electrochemical dehydrogenative cyclization of 1,3-dicarbonyl compounds, Chem. Commun., 2018, 54, 4601–4604 RSC.
- J. Xuan, Y. Cheng, J. An, L.-Q. Lu, X.-X. Zhang and W.-J. Xiao, Visible light-induced intramolecular cyclization reactions of diamines: A new strategy to construct tetrahydroimidazoles, Chem. Commun., 2011, 47, 8337–8339 RSC.
- X.-Q. Hu, J.-R. Chen, Q. Wei, F.-L. Liu, Q.-H. Deng, A. M. Beauchemin and W.-J. Xiao, Photocatalytic generation of N-centered hydrazonyl radicals: A strategy for hydroamination of β,γ-unsaturated hydrazones, Angew. Chem., Int. Ed., 2014, 53, 12163–12167 CrossRef CAS PubMed.
- H.-B. Zhao, Z.-W. Hou, Z.-J. Liu, Z.-F. Zhou, J. Song and H.-C. Xu, Amidinyl radical formation through anodic N–H bond cleavage and its application in aromatic C–H bond functionalization, Angew. Chem., Int. Ed., 2017, 56, 587–590 CrossRef CAS.
- P. Xiong, H.-H. Xu and H.-C. Xu, Metal- and reagent-free intramolecular oxidative amination of tri- and tetrasubstituted alkenes, J. Am. Chem. Soc., 2017, 139, 2956–2959 CrossRef CAS PubMed.
- X. Wang, N. Li, Z. Li and H. Rao, Copper-catalyzed dehydrogenative C(sp2)–N bond formation via direct oxidative activation of an anilidic N–H bond: Synthesis of benzoimidazo[1,2-a]indoles, J. Org. Chem., 2017, 82, 10158–10166 CrossRef CAS PubMed.
- X. Hu, G. Zhang, F. Bu, L. Nie and A. Lei, Electrochemical-oxidation-induced site-selective intramolecular C(sp3)–H amination, ACS Catal., 2018, 8, 9370–9375 CrossRef CAS.
- M. Okimoto, T. Yoshida, M. Hoshi, K. Hattori, M. Komata, K. Numata and K. Tomozawa, Electrooxidative cyclization of hydroquinolyl alcohols, Heterocycles, 2006, 68, 2563–2570 CrossRef CAS.
- V. Satheesh, M. Sengoden and T. Punniyamurthy, “On water” C(sp3)–H functionalization/C–O/C–N bonds formations: Synthesis of functionalized oxazolidines and imidazolidines, J. Org. Chem., 2016, 81, 9792–9801 CrossRef CAS PubMed.
- T. Gieshoff, A. Kehl, D. Schollmeyer, K. D. Moeller and S. R. Waldvogel, Electrochemical synthesis of benzoxazoles from anilides – a new approach to employ amidyl radical intermediates, Chem. Commun., 2017, 53, 2974–2977 RSC.
- G. Zhang, C. Liu, H. Yi, Q. Meng, C. Bian, H. Chen, J.-X. Jian, L.-Z. Wu and A. Lei, External oxidant-free oxidative cross-coupling: A photoredox cobalt-catalyzed aromatic C–H thiolation for constructing C–S bonds, J. Am. Chem. Soc., 2015, 137, 9273–9280 CrossRef CAS PubMed.
- X.-Y. Qian, S.-Q. Li, J. Song and H.-C. Xu, TEMPO-catalyzed electrochemical C–H thiolation: Synthesis of benzothiazoles and thiazolopyridines from thioamides, ACS Catal., 2017, 7, 2730–2734 CrossRef CAS.
- A. A. Folgueiras-Amador, X.-Y. Qian, H.-C. Xu and T. Wirth, Catalyst- and supporting-electrolyte-free electrosynthesis of benzothiazoles and thiazolopyridines in continuous flow, Chem. – Eur. J., 2018, 24, 487–491 CrossRef CAS PubMed.
- K. Oisaki, J. Abe and M. Kanai, Manganese-catalyzed aerobic dehydrogenative cyclization toward ring-fused indole skeletons, Org. Biomol. Chem., 2013, 11, 4569–4572 RSC.
- W. Hu, J.-P. Lin, L.-R. Song and Y.-Q. Long, Direct synthesis of 2-aryl-4-quinolones via transition-metal-free intramolecular oxidative C(sp3)–H/C(sp3)–H coupling, Org. Lett., 2015, 17, 1268–1271 CrossRef CAS.
- J. Tang, S. Zhao, Y. Wei, Z. Quan and C. Huo, CBr4 promoted intramolecular aerobic oxidative dehydrogenative arylation of aldehydes: Application in the synthesis of xanthones and fluorenones, Org. Biomol. Chem., 2017, 15, 1589–1592 RSC.
- A. Lee, R. C. Betori, E. A. Crane and K. A. Scheidt, An enantioselective cross-dehydrogenative coupling catalysis approach to substituted tetrahydropyrans, J. Am. Chem. Soc., 2018, 140, 6212–6216 CrossRef CAS PubMed.
- A. J. Neel, J. P. Hehn, P. F. Tripet and F. D. Toste, Asymmetric cross-dehydrogenative coupling enabled by the design and application of chiral triazole-containing phosphoric acids, J. Am. Chem. Soc., 2013, 135, 14044–14047 CrossRef CAS PubMed.
- C. Huo, M. Wu, X. Jia, H. Xie, Y. Yuan and J. Tang, Aerobic oxidative mannich reaction promoted by catalytic amounts of stable radical cation salt, J. Org. Chem., 2014, 79, 9860–9864 CrossRef CAS PubMed.
- H.-B. Zhao, Z.-J. Liu, J. Song and H.-C. Xu, Reagent-free C–H/N–H cross-coupling: Regioselective synthesis of N-heteroaromatics from biaryl aldehydes and NH3, Angew. Chem., Int. Ed., 2017, 56, 12732–12735 CrossRef CAS PubMed.
- F. Xu, X.-Y. Qian, Y.-J. Li and H.-C. Xu, Synthesis of 4H-1,3-benzoxazines via metal- and oxidizing reagent-free aromatic C–H oxygenation, Org. Lett., 2017, 19, 6332–6335 CrossRef CAS PubMed.
- P. J. Borpatra, M. L. Deb and P. K. Baruah, Visible light-promoted metal-free intramolecular cross dehydrogenative coupling approach to 13-oxazines, Tetrahedron Lett., 2017, 58, 4006–4010 CrossRef CAS.
- K. Chen, B. Gao, Y. Shang, J. Du, Q. Gu and J. Wang, I2-catalyzed cross dehydrogenative coupling: Rapid access to benzoxazinones and quinazolinones, Org. Biomol. Chem., 2017, 15, 8770–8779 RSC.
- L. Zhang, Z. Zhang, J. Zhang, K. Li and F. Mo, Oxidant-free oxidation of C–H bonds by cathodic hydrogen evolution: A phosphonic kolbe oxidation/cyclization process, Green Chem., 2018, 20, 3916–3920 RSC.
- L. Zhang, Z. Zhang, J. Hong, J. Yu, J. Zhang and F. Mo, Oxidant-free C(sp2)–H functionalization/C–O bond formation: A Kolbe oxidative cyclization process, J. Org. Chem., 2018, 83, 3200–3207 CrossRef CAS PubMed.
-
(a) S. Zhang, L. Li, H. Wang, Q. Li, W. Liu, K. Xu and C. Zeng, Scalable electrochemical dehydrogenative lactonization of C(sp2/sp3)–H bonds, Org. Lett., 2018, 20, 252–255 CrossRef CAS PubMed;
(b) L. Li, Q. Yang, Z. Jia and S. Luo, Organocatalytic electrochemical C–H lactonization of aromatic carboxylic acids, Synthesis, 2018, 2924–2929 CAS;
(c) A. Shao, N. Li, Y. Gao, J. Zhan, C.-W. Chiang and A. Lei, Electrochemical intramolecular C–H/O–H cross-coupling of 2-arylbenzoic acids, Chin.
J. Chem., 2018, 36, 619–624 CrossRef CAS.
- K. Wadekar, S. Aswale and V. R. Yatham, Cerium photocatalyzed dehydrogenative lactonization of 2-arylbenzoic acids, Org. Biomol. Chem., 2020, 18, 983–987 RSC.
- Y. Wang, F. Peng, J. Liu, C. Huo, X. Wang and X. Jia, Radical cation salt-promoted catalytic aerobic sp3 C–H oxidation: Construction of quinoline-fused lactones and lactams, J. Org. Chem., 2015, 80, 609–614 CrossRef CAS PubMed.
- Q. Chen, S. Zhang, T. Zhang, K. He, Y. Yuan and X. Jia, Radical C–H bond oxidation initiated intramolecular cyclization of glycine esters: Construction of dihydroquinoline skeletons, Asian J. Org. Chem., 2019, 8, 115–118 CrossRef CAS.
- Z.-W. Hou, Z.-Y. Mao, H.-B. Zhao, Y. Y. Melcamu, X. Lu, J. Song and H.-C. Xu, Electrochemical C–H/N–H functionalization for the synthesis of highly functionalized (aza)indoles, Angew. Chem., Int. Ed., 2016, 55, 9168–9172 CrossRef CAS PubMed.
-
(a) Z.-W. Hou, H. Yan, J.-S. Song and H.-C. Xu, Electrochemical synthesis of (aza)indolines via dehydrogenative [3 + 2] annulation: Application to total synthesis of (±)-Hinckdentine A, Chin. J. Chem., 2018, 36, 909–915 CrossRef CAS;
(b) Z.-W. Hou, Z.-Y. Mao, J. Song and H.-C. Xu, Electrochemical synthesis of polycyclic N-heteroaromatics through cascade radical cyclization of diynes, ACS Catal., 2017, 7, 5810–5813 CrossRef CAS.
Footnote |
† These two authors contributed equally to this paper. |
|
This journal is © the Partner Organisations 2020 |
Click here to see how this site uses Cookies. View our privacy policy here.