DOI:
10.1039/C9QO01360A
(Research Article)
Org. Chem. Front., 2020,
7, 374-379
Cascade cyclization reactions of alkylidenecyclopropanes for the construction of polycyclic lactams and lactones by visible light photoredox catalysis†
Received
9th November 2019
, Accepted 6th December 2019
First published on 10th December 2019
Abstract
A visible light photocatalytic cascade cyclization reaction of alkylidenecyclopropanes for the rapid construction of seven- and eight-membered ring-containing polycyclic lactams and lactones has been developed. The process is proposed to proceed through a radical pathway, and the suggested radical intermediate was captured by TEMPO successfully. An intermolecular version of the reaction was also achieved, affording a variety of methyl dialinoacetate products.
The past ten years have witnessed a flourish in visible light photocatalysis,1 and the photocatalytic variants of many important reactions have been achieved, such as cross-coupling reactions, cycloadditions, fluorinations, etc.2 Using light as the driving force in the reaction, the photocatalytic reaction can often proceed under mild reaction conditions. Meanwhile, photocatalysts can serve as both electron donors and acceptors in catalytic reaction cycles1b,3 and many stoichiometrically used oxidants and reductants in traditional reactions can be replaced by air, oxygen and amines, leading to a more economical and sustainable chemical synthesis.1a,4
Alkylidenecyclopropanes are important building blocks due to their high activities and diverse reactivities, and they have been widely used in organic synthesis.5 To further explore their potential usefulness in organic chemistry, persistent efforts have been made by our group and many other groups, and significant progress and interesting results have been achieved, including some light driven reactions about a decade ago.6 Considering the immense potential of visible light photocatalysis in organic synthesis, we attempt to combine it with alkylidenecyclopropane chemistry, in order to achieve new and efficient transformations of alkylidenecyclopropanes into useful structural motifs.
Inspired by the previous work,7 we hypothesized that substrate 1a having the alkylidenecyclopropane moiety under visible light photocatalysis could produce a radical intermediate by the cleavage of the C–Br bond at the α-position of the carbonyl group, and a cascade cyclization reaction would probably take place to afford polycyclic compounds. Thus, 1a was prepared, and we subsequently examined its reactivity under visible light photocatalysis (Scheme 1). To our delight, when 1a (0.2 mmol) together with Ir(ppy)3 (3 mol%) and K2CO3 (3.0 equiv.) was exposed to blue LED light for 12 h, polycyclic product 2a was obtained successfully in a yield of 55% (Scheme 1 and Table 1, entry 6). The structure of 2a was confirmed by X-ray crystal diffraction. Its ORTEP drawing is shown in Fig. 1 and the CIF data are presented in the ESI.†
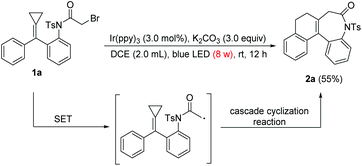 |
| Scheme 1 Our design and first attempt of the photo-catalyzed reaction. | |
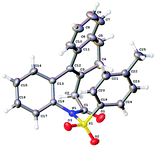 |
| Fig. 1 The X-ray crystal structure of 2a. | |
Table 1 Optimization of reaction conditions
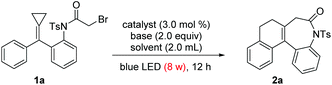
|
Entrya |
Catalyst |
Base |
Solvent |
Yieldb/% |
Reaction conditions: 1a (0.2 mmol), photocatalyst (3.0 mol%) and base (3.0 equiv.) were placed in a reaction tube and Ar was charged. Then 2.0 mL solvent was added and the mixture was stirred exposing to blue LED light (8 W) at room temperature for 12 h. NR = no reaction.
Isolated yield. All the starting materials had been consumed and instead of forming 2a, the rest of them might have decomposed into unknown complexes.
White light (40 W) and green LED light (8 W) were also employed and no desired product 2a was obtained.
1.5 mL DCE.
2.5 mL DCE.
|
1c |
Eosin Y |
K2CO3 |
DCE |
NR |
2c |
Fluorescein |
K2CO3 |
DCE |
NR |
3c |
Ru(bpy)3·Cl26H2O |
K2CO3 |
DCE |
NR |
4 |
Ir(ppy)2(dtbpy)PF6 |
K2CO3 |
DCE |
10 |
5 |
Ir[dF(CF3)ppy]2(dtbpy)PF6 |
K2CO3 |
DCE |
5 |
6
|
Ir(ppy)
3
|
K
2
CO
3
|
DCE
|
55
|
7 |
Ir(ppy)3 |
Cs2CO3 |
DCE |
52 |
8 |
Ir(ppy)3 |
Pyridine |
DCE |
10 |
9 |
Ir(ppy)3 |
Et3N |
DCE |
15 |
10 |
Ir(ppy)3 |
K2CO3 |
Toluene |
47 |
11 |
Ir(ppy)3 |
K2CO3 |
CH3CN |
33 |
12 |
Ir(ppy)3 |
K2CO3 |
DCM |
50 |
13d |
Ir(ppy)3 |
K2CO3 |
DCE |
54 |
14e |
Ir(ppy)3 |
K2CO3 |
DCE |
53 |
Encouraged by the success of the first attempt of this reaction, we tried to optimize the reaction conditions (Table 1). Firstly, we screened several commonly used photocatalysts. Photocatalysts such as eosin Y, fluorescein and Ru(bpy)3Cl2·6H2O could not catalyze this reaction, and the reaction still did not work when we employed white and green light sources instead of blue LED light (Table 1, entries 1–3). However, Ir-relevant photocatalysts smoothly catalyzed this reaction to afford the desired product 2a (Table 1, entries 4 and 5) and Ir(ppy)3 used in our first attempt was still the best (Table 1, entry 6). This maybe implied that the redox potentials of Ir-relevant photocatalysts were the most suitable for this reaction. Then, different bases were screened. The use of the inorganic base Cs2CO3 afforded 2a in a yield of 52% (Table 1, entry 7), which is similar to that obtained using K2CO3. However, employing organic bases including pyridine and NEt3 decreased the yield of 2a to 10% and 15%, respectively (Table 1, entries 8 and 9), which implied that organic bases might hamper the redox cycle of the photocatalyst in the reaction. Different solvents were also screened, and the use of toluene, CH3CN and DCM as solvents afforded product 2a in yields ranging from 30% to 50% (Table 1, entries 10–12). In comparison, DCE was the most appropriate choice. The adjustment of the volume of solvent did not improve the yield of 2a (Table 1, entries 13 and 14). The reaction conditions used in the first attempt were still the best reaction conditions.
With the optimal conditions in hand, we then examined the substrate scope (Scheme 2). Generally speaking, the products were obtained in moderate yields; although all the starting materials were consumed, some of them were decomposed to unknown complexes. Substrates 1b–1d bearing the F/Cl substituent afforded the desired products 2b–2d in a similar yield of about 50%; substrates 1e and 1f bearing the –Br substituent afforded the corresponding products 2e and 2f in relatively lower yields of 45% and 49%, respectively. Substrates 1g and 1h bearing a methoxyl group also afforded the corresponding products 2g and 2h in yields of 53% and 51%, respectively. It seemed that the electronic effect of the substituents on the substrates did not have remarkable influence on the yields of the products. Alkyl group substituted substrates 1i–1l underwent the reaction smoothly, affording products 2i–2l in yields ranging from 35% to 54%. The phenyl substituted substrate 1m afforded product 2m in a yield of 46%. Substrate 1n having the CF3 substituent underwent the reaction smoothly, affording product 2n in a yield of 42%. A polycyclic lactone product 2o was also obtained in a yield of 48%.
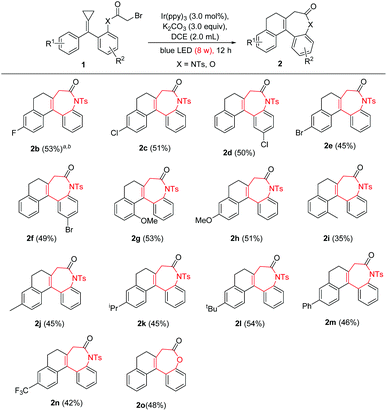 |
| Scheme 2 Substrate scope of 1. a Reaction conditions: 1 (0.2 mmol), Ir(ppy)3 (3.0 mol%) and K2CO3 (3.0 equiv.) were placed in a reaction tube and Ar was charged. Then 2.0 mL solvent was added and the mixture was stirred upon exposure to blue LED light (8 W) at room temperature for 12 h. b Isolated yield. | |
After successfully obtaining a series of polycyclic lactams and lactones containing seven-membered rings, we further proceeded to synthesize polycyclic compounds containing eight-membered rings. With a slight adjustment of the conditions, we found that when DCE was reduced to 1.0 mL, the temperature was increased to 70 °C and reaction time was prolonged to 24 h (see the ESI†), and product 4a was also obtained smoothly, though in a relatively lower yield (Scheme 3).
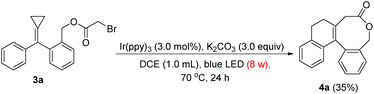 |
| Scheme 3 Attempt to construct polycyclic compound 4a using substrate 3a. | |
The substrate scope was also examined by employing a variety of alkylidenecyclopropanes 3 bearing –Cl/Me/iPr/Ph groups. The desired polycyclic lactams and lactones 4b–4j containing eight-membered rings were obtained in yields ranging from 10% to 30% (Scheme 4). In comparison, the yield of 4 was much lower than that of 2 presumably due to the large size of the eight-membered ring. The reactions of substrates 3d and 3i took place, affording products 4d and 4i as mixtures of regioisomers in 2.3
:
1 and 2
:
1 ratios, respectively.
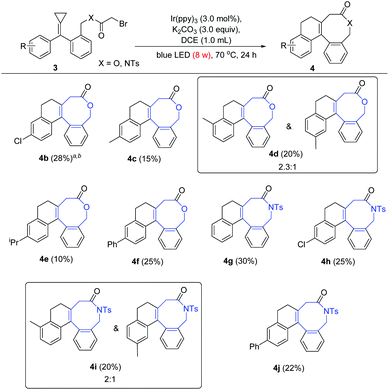 |
| Scheme 4 Substrate scope of 3. a Reaction conditions: 3 (0.2 mmol), Ir(ppy)3 (3.0 mol%) and K2CO3 (3.0 equiv.) were placed in a reaction tube and Ar was charged. Then 2.0 mL solvent was added and the mixture was stirred upon exposure to blue LED light (8 W) at 70 °C for 12 h. b Isolated yield. | |
To gain some insights into the mechanism, TEMPO was used to capture radical intermediates involved in the reaction. Fortunately, compound 1a-TMP was successfully obtained in a yield of 60% (Scheme 5). This provided evidence for the proposed radical pathway.
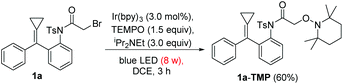 |
| Scheme 5 Capture of the radical intermediate with TEMPO. | |
Based on the previous reports7b,c,8 and the above experimental results, we proposed a radical reaction mechanism for this photo-catalyzed reaction (Scheme 6). Firstly, substrate 1a produces an active radical intermediate I through a SET process, and then intermediate I undergoes cascade cyclization to afford intermediates II and III. Afterwards, through another SET process and deprotonation, the desired polycyclic product 2a can be generated. It should be noted here that K2CO3 as the auxiliary base is also critical for this reaction; without the base, the substrate will be quickly decomposed in the presence of HBr generated during the reaction process and the yield will be dramatically decreased.
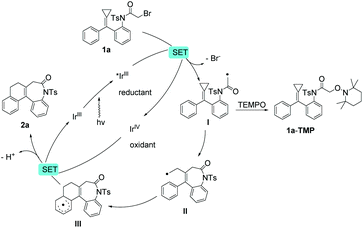 |
| Scheme 6 A plausible pathway for the reaction. | |
Furthermore, we developed an intermolecular version of the visible light photocatalyzed reaction on the basis of the above results. The reaction of 5a and methyl bromoacetate (MBA) occurred under the same conditions, and the desired product 6a was obtained in a yield of 33%, together with a radical chain product 7a in a yield of 24% (Scheme 7a). Based on the proposed mechanism (Scheme 6), we proposed that the key radical intermediate M probably underwent either cyclization (via path a) to give product 6 or a chain reaction with MBA (via path b) to generate product 7. We hypothesized that if the concentration of MBA was kept low enough, intermediate M would be favorably transformed into 6a through path a while path b would be greatly suppressed (Scheme 7b). Thus, MBA was injected dropwise into the reaction system through a syringe pump within 4 h and the yield of 6a successfully increased to 54% while the yield of 7a decreased to 10% (Scheme 7c; for details, see the ESI†).
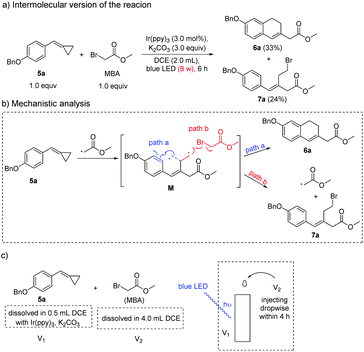 |
| Scheme 7 Intermolecular reaction of 5a with MBA. | |
We also investigated the substrate scope of 5 for this reaction (Scheme 8). Substrates 5b–5h bearing –F/Cl/Me/MeO/Ph/OBn groups underwent this reaction to afford products 6b–6h in yields ranging from 35% to 47% while the radical chain products 7b–7h were obtained in dramatically suppressed yields. As for substrate 5e, it transformed regiospecifically into product 6e. For substrate 5i, the corresponding product 6i was only obtained in a yield of 15% while the yield of 7i increased to 30%, presumably due to the electronic effect (see the ESI†).
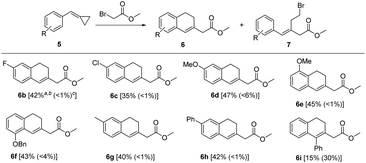 |
| Scheme 8 Substrate scope for the intermolecular reaction. a Reaction conditions: 5 (0.2 mmol), Ir(ppy)3 (3.0 mol%) and K2CO3 (3.0 equiv.) were placed in a reaction tube. Ar was charged and 0.5 mL DCE was added. Then upon exposure to blue LED light (8 W), MBA (0.2 mmol) dissolved in 4.0 mL DCE was injected dropwise through a syringe pump within 4 h. b Isolated yields of product 6. c Yields of 7 determined by 1H NMR spectroscopy with 1,3,5-trimethoxybenzene as an internal standard. | |
Products 6 can be used as precursors of many valuable compounds. For example, 6d can be dehydrogenated to form 6′d (available at a high price on the market) and then naproxen (non-steroidal anti-inflammatory drug) after further methylation and hydrolysis (Scheme 9).9
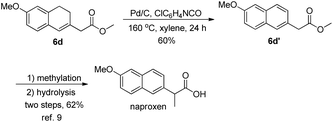 |
| Scheme 9 Synthetic transformation of 6d. | |
In summary, a facile method for the synthesis of polycyclic lactams and lactones containing seven-membered or eight-membered rings by visible light photocatalysis has been developed and a plausible radical pathway is proposed. An intermolecular version of the reaction was also achieved which could afford various substituted methyl dialinoacetate products used as valuable precursors for further transformations.
Conflicts of interest
There are no conflicts of interest to declare.
Acknowledgements
We are grateful for the financial support from the National Basic Research Program of China (973)-2015CB856603, the Strategic Priority Research Program of the Chinese Academy of Sciences, Grant No. XDB20000000 and sioczz201808, the National Natural Science Foundation of China (21372241, 21572052, 21421091, 21372250, 21121062, 21302203, 21772037, 21772226 and 21861132014), and the Shenzhen Nobel Prize Scientists Laboratory Project.
Notes and references
-
(a) X. Sala, I. Romero, M. Rodríguez, L. Escriche and A. Llobet, Molecular catalysts that oxidize water to dioxygen, Angew. Chem., Int. Ed., 2009, 48, 2842 CrossRef CAS PubMed;
(b) K. Zeitler, Photoredox catalysis with visible light, Angew. Chem., Int. Ed., 2009, 48, 9785 CrossRef CAS PubMed;
(c) T. P. Yoon, M. A. Ischay and J. Du, Visible light photocatalysis as a greener approach to photochemical synthesis, Nat. Chem., 2010, 2, 527 CrossRef CAS PubMed;
(d) J. M. R. Narayanam and C. R. J. Stephenson, Visible light photoredox catalysis: applications in organic synthesis, Chem. Soc. Rev., 2011, 40, 102 RSC;
(e) J. Xuan and W.-J. Xiao, Visible-light photoredox catalysis, Angew. Chem., Int. Ed., 2012, 51, 6828 CrossRef CAS;
(f) X. Dai, X. Xu and X. Li, Applications of visible light photoredox catalysis in organic synthesis, Chin. J. Org. Chem., 2013, 33, 2046 CrossRef CAS;
(g) C. K. Prier, D. A. Rankic and D. W. C. MacMillan, Visible light photoredox catalysis with transition metal complexes: Applications in organic synthesis, Chem. Rev., 2013, 113, 5322 CrossRef CAS PubMed;
(h) M.-Y. Cao, X. Ren and Z. Lu, Visible-light-induced direct alpha-C(sp(3))-H thiocyanation of tertiary amines, Tetrahedron Lett., 2015, 56, 3732 CrossRef CAS;
(i) N. Corrigan, S. Shanmugam, J. T. Xu and C. Boyer, Photocatalysis in organic and polymer synthesis, Chem. Soc. Rev., 2016, 45, 6165 RSC;
(j) X. Li, J. G. Yu and M. Jaroniec, Cooperative photoredox catalysis, Chem. Soc. Rev., 2016, 45, 2603 RSC;
(k) N. A. Romero and D. A. Nicewicz, Organic photoredox catalysis, Chem. Rev., 2016, 116, 10075 CrossRef CAS;
(l) K. L. Skubi, T. R. Blum and T. P. Yoon, Dual catalysis strategies in photochemical synthesis, Chem. Rev., 2016, 116, 10035 CrossRef CAS PubMed.
-
(a) M. Fagnoni, D. Dondi, D. Ravelli and A. Albini, Photocatalysis for the formation of the C–C bond, Chem. Rev., 2007, 107, 2725 CrossRef CAS PubMed;
(b) M. A. Ischay, M. E. Anzovino, J. Du and T. P. Yoon, Efficient visible light photocatalysis of [2+2] enone cycloadditions, J. Am. Chem. Soc., 2008, 130, 12886 CrossRef CAS PubMed;
(c) D. A. Nicewicz and D. W. C. MacMillan, Merging photoredox catalysis with organocatalysis: the direct asymmetric alkylation of aldehydes, Science, 2008, 322, 77 CrossRef CAS PubMed;
(d) J. R. Chen, X. Q. Hu, L. Q. Lu and W. J. Xiao, Exploration of visible-light photocatalysis in heterocycle synthesis and functionalization: reaction design and beyond, Acc. Chem. Res., 2016, 49, 1911 CrossRef CAS;
(e) Y. Liu, R. Song and J. Li, The cycloaddition reaction using visible light photoredox catalysis, Sci. China: Chem., 2016, 59, 161 CrossRef CAS;
(f) S. Poplata, A. Troster, Y. Q. Zou and T. Bach, Recent advances in the synthesis of cyclobutanes by olefin 2+2 photocycloaddition reactions, Chem. Rev., 2016, 116, 9748 CrossRef CAS PubMed;
(g) J. Xie, T. Zhang, F. Chen, N. Mehrkens, F. Rominger, M. Rudolph and A. S. K. Hashmi, Gold-catalyzed highly selective photoredox C(sp2)-H difluoroalkylation and perfluoro-alkylation of hydrazones, Angew. Chem., Int. Ed., 2016, 55, 2934 CrossRef CAS PubMed;
(h) C.-Y. He, J.-W. Gu and X. Zhang, Visible-light-mediated direct perfluoroalkylation and trifluoro-methylation of free anilines, Tetrahedron Lett., 2017, 58, 3939 CrossRef CAS;
(i) J. H. Kou, C. H. Lu, J. Wang, Y. K. Chen, Z. Z. Xu and R. S. Varma, Selectivity enhancement in heterogeneous photocatalytic transformations, Chem. Rev., 2017, 117, 1445 CrossRef CAS PubMed;
(j) Q. Qin, H. Jiang, Z. Hu, D. Ren and S. Yu, Functionalization of C-H bonds by photoredox catalysis, Chem. Rec., 2017, 17, 754 CrossRef CAS PubMed;
(k) J. Twilton, C. Le, P. Zhang, M. H. Shaw, R. W. Evans and D. W. C. MacMillan, The merger of transition metal and photocatalysis, Nat. Rev. Chem., 2017, 1, 1 CrossRef;
(l) X.-K. He, B.-G. Cai, Q.-Q. Yang, L. Wang and J. Xuan, Visible-light-Promoted cascade radical cyclization: synthesis of 1,4-diketones containing chroman-4-one skeletons, Chem. – Asian J., 2019, 14, 3269 CrossRef CAS;
(m) X. Yi, F. Huang, J. B. Baell, H. Huang and Y. Yu, The formation of C(sp3)-C(sp3) by visible-light photocatalysis, Prog. Chem., 2019, 31, 505 Search PubMed.
-
(a) M. D. Kaerkaes, B. S. Matsuura and C. R. J. Stephenson, Enchained by visible light-mediated photoredox catalysis, Science, 2015, 349, 1285 CrossRef PubMed;
(b) W. Yu, X.-H. Xu and F.-L. Qing, Photoredox catalysis mediated application of methyl fluorosulfonyldifluoroacetate as the CF2CO2R radical source, Org. Lett., 2016, 18, 5130 CrossRef CAS PubMed.
-
(a) X. Lang, J. Zhao and X. Chen, Cooperative photoredox catalysis, Chem. Soc. Rev., 2016, 45, 3026 RSC;
(b) X. Cheng, X. Hu and Z. Lu, Visible-light-promoted aerobic homogenous oxygenation reactions, Chin. J. Org. Chem., 2017, 37, 251 CrossRef CAS.
-
(a)
A. Goti, F. M. Cordero and A. Brandi, Cycloadditions onto methylene- and alkylidenecyclopropane derivatives, in Small Ring Compounds in Organic Synthesis V, ed. A. deMeijere, 1996, vol. 178, p. 1 Search PubMed;
(b) M. Lautens, W. Klute and W. Tam, Transition metal-mediated cycloaddition reactions, Chem. Rev., 1996, 96, 49 CrossRef CAS PubMed;
(c) I. Nakamura and Y. Yamamoto, Transition metal-catalyzed reactions of methylene-cyclopropanes, Adv. Synth. Catal., 2002, 344, 111 CrossRef CAS;
(d) A. Brandi, S. Cicchi, F. M. Cordero and A. Goti, Heterocycles from alkylidenecyclopropanes, Chem. Rev., 2003, 103, 1213 CrossRef CAS PubMed;
(e) L.-X. Shao and M. Shi, Lewis and Brønsted acid mediated ring-opening reactions of methylenecyclopropanes and further transformation of the ring-opened products, Curr. Org. Chem., 2007, 11, 1135 CrossRef CAS;
(f) A. Masarwa and I. Marek, Selectivity in metal-catalyzed carbon–carbon bond cleavage of alkylidenecyclopropanes, Chem. – Eur. J., 2010, 16, 9712 CrossRef CAS PubMed;
(g) L.-Z. Yu, K. Chen, Z.-Z. Zhu and M. Shi, Recent advances in the chemical transformations of functionalized alkylidene-cyclopropanes (FACPs), Chem. Commun., 2017, 53, 5935 RSC;
(h) M. Shi, J.-M. Lu, Y. Wei and L.-X. Shao, Rapid generation of molecular complexity in the Lewis or Brønsted acid-mediated reactions of methylenecyclopropanes, Acc. Chem. Res., 2012, 45, 641 CrossRef CAS PubMed;
(i) A. Brandi, S. Cicchi, F. M. Cordero and A. Goti, Progress in the synthesis and transformations of alkylidenecyclopropanes and alkylidenecyclobutanes, Chem. Rev., 2014, 114, 7317 CrossRef CAS PubMed;
(j) H. Pellissier, Recent developments in the synthesis and reactivity of methylene- and alkylidene-cyclopropane derivatives, Tetrahedron, 2014, 70, 4991 CrossRef CAS;
(k) J. Liu, R. Liu, Y. Wei and M. Shi, Recent developments in cyclopropane cycloaddition reactions, Trends Chem., 2019, 1, 779 CrossRef;
(l) I. Marek, A. Masarwa, P.-O. Delaye and M. Leibeling, Selective carbon–carbon bond cleavage for the stereo-selective synthesis of acyclic systems, Angew. Chem., Int. Ed., 2015, 54, 414 CrossRef CAS PubMed;
(m) H. G. Cao, F. L. Chen, C. L. Su and L. Yu, Construction of carbocycles from methylenecyclopropanes, Adv. Synth. Catal., 2019 DOI:10.1002/adsc.201900615.
-
(a) L. Yu, M. Liu, F. Chen and Q. Xu, Heterocycles from methylenecyclopropanes, Org. Biomol. Chem., 2015, 13, 8379 RSC;
(b) D. Nishikawa, R. Sakae, Y. Miki, K. Hirano and M. Miura, Copper-catalyzed regioselective ring-opening hydro-amination of methylenecyclopropanes, J. Org. Chem., 2016, 81, 12128 CrossRef CAS PubMed;
(c) L.-Z. Yu and M. Shi, The construction of molecular complexity from functionalized alkylidene-cyclopropanes (FACPs), Chem. – Eur. J., 2019, 25, 7591 CAS;
(d) L. Yu, F. Chen and Y. Ding, Organoselenium-catalyzed oxidative ring expansion of methylenecyclopropanes with hydrogen peroxide, ChemCatChem, 2016, 8, 1033 CrossRef CAS;
(e) Y.-C. Yuan, H.-B. Yang, X.-Y. Tang, Y. Wei and M. Shi, Unprecedented oxycyanation of methylenecyclopropanes for the facile synthesis of benzoxazine compounds containing a cyano group, Chem. – Eur. J., 2016, 22, 5146 CrossRef CAS PubMed;
(f) A. Dey, N. Thrimurtulu and C. M. R. Volla, Cobalt-catalyzed annulation reactions of alkylidenecyclopropanes: access to spiro-cyclopropanes at room temperature, Org. Lett., 2019, 21, 3871 CrossRef CAS;
(g) H. Clavier and G. Buono, 2+1 cycloaddition affording methylene- and vinylidenecyclopropane derivatives: a Journey around the reactivity of metal-phosphinito-phosphinous acid complexes, Chem. Rec., 2017, 17, 399 CrossRef CAS PubMed;
(h) L. Tao and M. Shi, Pd(II)-catalyzed cyclization oxidation of urea-tethered alkylidenecyclopropanes, Org. Lett., 2018, 20, 3017 CrossRef CAS PubMed;
(i) W.-D. Liu, G.-Q. Xu, X.-Q. Hu and P.-F. Xu, Visible-light-induced aza-pinacol rearrangement: ring expansion of alkylidenecyclopropanes, Org. Lett., 2017, 19, 6288 CrossRef CAS PubMed;
(j) F. Verdugo, L. Villarino, J. Duran, M. Gulias, J. L. Mascarenas and F. Lopez, Enantioselective palladium-catalyzed 3C+2C and 4C+3C intramolecular cycloadditions of alkylidenecyclopropanes, ACS Catal., 2018, 8, 6100 CrossRef CAS;
(k) L.-Z. Yu, Y. Wei and M. Shi, Synthesis of polysubstituted polycyclic aromatic hydrocarbons by gold-catalyzed cyclization-oxidation of alkylidenecyclopropane-containing 1,5-enynes, ACS Catal., 2017, 7, 4242 CrossRef CAS;
(l) D. Bai, T. Xu, C. Ma, X. Zheng, B. Liu, F. Xie and X. Li, Rh(III)-catalyzed mild coupling of nitrones and azomethine imines with alkylidenecyclopropanes via C-H activation: facile access to bridged cycles, ACS Catal., 2018, 8, 4194 CrossRef CAS;
(m) Y. Liu, Z. Chen, Q.-L. Wang and K.-W. Tang, Synthesis of 2-acyl-3,4-dihydronaphthalenes by silver-promoted oxidative C-C sigma-bond acylation/arylation of alkylidenecyclopropanes with alpha-ketoacids, J. Org. Chem., 2019, 84, 9984 CrossRef CAS PubMed;
(n) L. Yu and X. Huang, Reaction of methylenecyclopropanes and diphenyl diselenide under visible-light, Synlett, 2006, 2136 CAS;
(o) L. Yu and X. Huang, Copper(II) acetate mediated reactions of methylenecyclopropane and diphenyl diselenide, Synlett, 2007, 1371 CAS.
-
(a) J. M. R. Narayanam, J. W. Tucker and C. R. J. Stephenson, Electron-transfer photoredox catalysis: development of a tin-free reductive dehalogenation reaction, J. Am. Chem. Soc., 2009, 131, 8756 CrossRef CAS PubMed;
(b) J. W. Tucker, J. M. R. Narayanam, S. W. Krabbe and C. R. J. Stephenson, Electron transfer photo-redox catalysis: intramolecular radical addition to indoles and pyrroles, Org. Lett., 2010, 12, 368 CrossRef CAS PubMed;
(c) J. Li, J. Z. Chen, W. Jiao, G. Q. Wang, Y. Li, X. Cheng and G. G. Li, Difluoro-alkylation/C-H annulation cascade reaction induced by visible-light photoredox catalysis, J. Org. Chem., 2016, 81, 9992 CrossRef CAS PubMed.
- J. Li, J. Z. Chen and G. G. Li, Difluoroalkylation/C–H Annulation Cascade Reaction Induced by Visible-Light Photoredox Catalysis, J. Org. Chem., 2016, 81, 9992 CrossRef CAS PubMed.
- Y. Tsukamoto, S. Itoh, M. Kobayashi and Y. Obora, Iridium-catalyzed α-methylation of α-aryl esters using methanol as the C1 source, Org. Lett., 2019, 21, 3299 CrossRef CAS PubMed.
Footnote |
† Electronic supplementary information (ESI) available: Experimental procedures and characterization data of new compounds. CCDC 1842217. For ESI and crystallographic data in CIF or other electronic format see DOI: 10.1039/c9qo01360a |
|
This journal is © the Partner Organisations 2020 |
Click here to see how this site uses Cookies. View our privacy policy here.