DOI:
10.1039/C7QO00866J
(Research Article)
Org. Chem. Front., 2018,
5, 366-370
A copper-catalyzed sulfonylative C–H bond functionalization from sulfur dioxide and aryldiazonium tetrafluoroborates†
Received
23rd September 2017
, Accepted 3rd October 2017
First published on 11th October 2017
Abstract
Sulfonylative C–H bond functionalization through a copper-catalyzed three-component reaction of 8-aminoquinoline amides, DABCO·(SO2)2 and aryldiazonium tetrafluoroborates is developed. Excellent selectivity in the para-position is observed for this copper-catalyzed transformation. This reaction is triggered by a copper-chelated complex via the coordination of the copper catalyst with the substrate and arylsulfonyl radical generated in situ, thus providing 5-sulfonyl-8-aminoquinoline amides in moderate to good yields.
Introduction
So far, C–H bond functionalizations catalyzed by transition metals have made significant progress.1 Diverse directing groups in transition metal-catalyzed C–H bond functionalizations have been developed.2 Recently, we have been interested in the synthesis of sulfones, due to their importance in pharmaceuticals and materials.3 Usually, sulfinic acids and their salts were used as the source of the sulfonyl group for the formation of sulfones.4 For instance, Manolikakes and co-workers described the generation of aryl sulfones through a para-selective C–H functionalization of 1-isoquinoline carboxamides with sulfinic acid salts.5 This transformation was achieved by using a catalytic amount of copper(II) acetate and a stoichiometric amount of Mn(OAc)3 as the oxidant. Other examples for the copper-catalyzed remote sulfonylation of 8-aminoquinolines by using sulfonyl chlorides or sulfinic acid salts have also been reported (Scheme 1, eqn (a)).6 A chelated complex from the metal catalyst and 8-aminoquinoline7 was proposed as the key intermediate for successful transformation through a single electron transfer. In the past few years, we have been involved in the preparation of sulfonyl compounds by using sulfur dioxide as the starting material.8,9 Approaches including transition metal catalysis and a radical process have been developed. However, there are few reports for the sulfonylation of C–H bonds with the insertion of sulfur dioxide.8e Prompted by the advancement of sulfur dioxide insertion chemistry,10 we envisioned that the para-selective C–H functionalization of 1-isoquinoline carboxamides with sulfur dioxide would be feasible under proper conditions via a copper-chelated complex of 8-aminoquinoline (Scheme 1, eqn (b)). Additionally, we postulated that the stoichiometric amount of oxidant as reported by Manolikakes5 and others6 would be avoided for the sulfonylative C–H bond functionalization with sulfur dioxide. Therefore, a broad reaction scope would be expected by using sulfur dioxide for the introduction of a sulfonyl group.
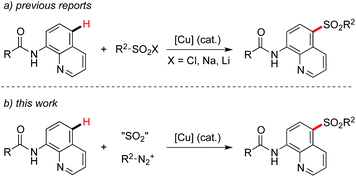 |
| Scheme 1 A proposed route for the copper-catalyzed C–H bond sulfonylation with the insertion of sulfur dioxide. | |
Since the discovery of delivering arysulfonyl radicals from aryldiazonium tetrafluoroborates and DABCO·(SO2)2
11 under mild conditions,8a diverse sulfonyl compounds have been prepared by using this strategy.9 We conceived that the para-selective C–H functionalization of 1-isoquinoline carboxamides with sulfur dioxide could be achieved as well through sulfonyl radicals. Encouraged by Manolikakes's work as described above,5 we reasoned that the combination of copper catalysis and sulfur dioxide would provide the sulfonylative products through a para-selective C–H functionalization of 1-isoquinoline carboxamides. Therefore, we started to consider the possible transformation of 1-isoquinoline carboxamides with arylsulfonyl radicals generated in situ.
From the mechanistic point of view for the copper-catalyzed C–H bond sulfonylation, we proposed a three-component reaction of 8-aminoquinoline amide 1, aryldiazonium tetrafluoroborate 2, and the sulfur dioxide surrogate of DABCO·(SO2)23, which is shown in Scheme 2. We reasoned that Cu(II) would coordinate with 8-aminoquinoline amide 1 leading to a chelated complex A.5 The arylsulfonyl radical8a generated in situ from the combination of aryldiazonium tetrafluoroborate 2 and DABCO·(SO2)23 would undergo addition to the aromatic ring of 8-aminoquinoline 1 to afford the intermediate B. Then dehydrogenation of the intermediate B would provide the intermediate C. A subsequent single electron transfer (SET) between the intermediate C and tertiary amine cation radical would give rise to the desired sulfone 4. With these considerations, we started to investigate the feasibility of the direct sulfonylation of the C–H bond with sulfur dioxide.
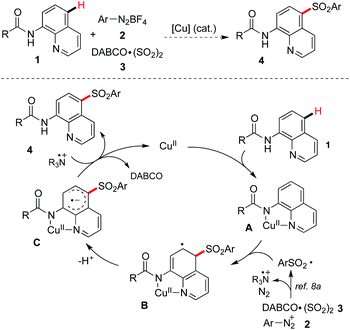 |
| Scheme 2 A plausible mechanism of the copper-catalyzed C–H bond sulfonylation with the insertion of sulfur dioxide. | |
Results and discussion
Since the direct sulfonylation of the C–H bond starting from sulfinic acids and their salts in the presence of copper salts would provide the site-selective product in the para-position of an amide,5,6 we therefore examined the copper-catalyzed reaction of N-(quinolin-8-yl)benzamide 1a, 4-methylphenyldiazonium tetrafluoroborate 2a and DABCO·(SO2)23. As expected, the corresponding product 4a was obtained in 50% yield when the reaction was catalyzed by copper(II) acetate in DCE (Table 1, entry 1). This result also indicated the excellent selectivity controlled by transition metal catalysis. The yield was inferior when copper(II) chloride or copper(II) bromide was used as the catalyst (Table 1, entries 2 and 3). Less efficiency was observed when the reaction worked in other solvents (Table 1, entries 4–6). Temperature was further screened, and the reaction afforded the desired product 4a in 49% yield at 60 °C (Table 1, entry 8). The yield could increase to 60% when the reaction concentration was changed (Table 1, entry 10). A control experiment without the addition of the copper catalyst failed to provide the corresponding product (Table 1, entry 12). We also examined the reaction by using potassium metabisulfite (K2S2O5) as the source of sulfur dioxide. However, the transformation was not effective, and only a trace amount of product was detected (data not shown in Table 1).
Table 1 Initial studies for the copper-catalyzed reaction of N-(quinolin-8-yl)benzamide 1a, 4-methylphenyldiazonium tetrafluoroborate 2a and DABCO·(SO2)23
a
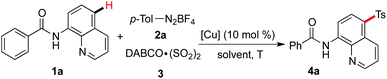
|
Entry |
Additive |
Solvent |
Temp. (°C) |
Yieldb (%) |
Reaction conditions: N-(quinolin-8-yl)benzamide 1a (0.2 mmol), 4-methylphenyldiazonium tetrafluoroborate 2a (0.4 mmol), DABCO·(SO2)2 (1.5 equiv.), copper catalyst (10 mol%), solvent (2.0 mL).
Isolated yield based on N-(quinolin-8-yl)benzamide 1a.
DCE 1.0 mL.
DCE 4.0 mL.
|
1 |
Cu(OAc)2 |
DCE |
70 |
50 |
2 |
CuBr |
DCE |
70 |
Trace |
3 |
CuCl2 |
DCE |
70 |
18 |
4 |
Cu(OAc)2 |
MeCN |
70 |
Trace |
5 |
Cu(OAc)2 |
DMF |
70 |
Trace |
6 |
Cu(OAc)2 |
1,4-Dioxane |
70 |
40 |
7 |
Cu(OAc)2 |
DCE |
50 |
44 |
8 |
Cu(OAc)2 |
DCE |
60 |
49 |
9 |
Cu(OAc)2 |
DCE |
80 |
41 |
10c |
Cu(OAc)2 |
DCE |
60 |
60 |
11d |
Cu(OAc)2 |
DCE |
60 |
47 |
12 |
— |
DCE |
60 |
NR |
We further evaluated the copper-catalyzed remote C–H bond sulfonylation under optimal reaction conditions, and the results are shown in Table 2. Firstly, an array of aryldiazonium tetrafluoroborates was investigated. We could see that the reactions worked well, leading to the desired products in moderate to good yield. For instance, the use of 2-chlorophenyldiazonium tetrafluoroborate afforded the product 4e in 72% yield. Moreover, a strong electron-donating functional group such as methoxy was also compatible, affording compounds 4h and 4i in 61% and 54% yields, respectively. However, the reaction was less effective when 4-bromophenyldiazonium tetrafluoroborate was used, and only 26% yield of the desired product 4f could be isolated.
Table 2 Scope exploration of the copper-catalyzed C–H bond sulfonylation with the insertion of sulfur dioxidea
Isolated yield based on aryldiazonium tetrafluoroborate 1.
|
|
In the meantime, various 8-aminoquinoline amides 1 featuring electron-withdrawing or electron-donating groups were examined. It was found that methyl, methoxy, trifluoromethyl, fluoro, chloro, bromo and tert-butyl were all tolerated, and the corresponding products were obtained in moderate to good yields (4l–4t). Additionally, heteroaryl-substituted 8-aminoquinoline amides worked efficiently as well, giving rise to compounds 4u and 4v in good yields, while the reaction of cyclohexyl-substituted 8-aminoquinoline showed a less successful result (4w, 47% yield).
To gain a deeper insight into the mechanism of the copper-catalyzed remote C–H bond sulfonylation, 2-(allyloxy)phenyldiazonium tetrafluoroborate 5 was used to react with N-(quinolin-8-yl)benzamide 1a and DABCO·(SO2)23 under the standard conditions (Scheme 3). Compound 6 with a dihydrobenzofuran skeleton was isolated in 40% yield (Scheme 3, eqn (a)). Additionally, the transformation was completely terminated with the addition of 2.0 equiv. of 2,2,6,6-tetramethyl-1-piperidinyloxy (TEMPO) into the reaction system (Scheme 3, eqn (b)). These two experimental results suggested that the reaction experienced a radical process.
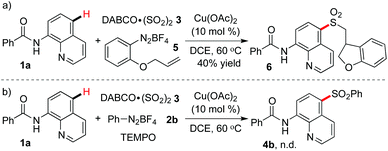 |
| Scheme 3 Mechanism studies for the copper-catalyzed C–H bond sulfonylation with the insertion of sulfur dioxide. | |
Conclusions
In conclusion, we have reported a direct sulfonylation of C–H bonds with the insertion of sulfur dioxide through a copper-catalyzed three-component reaction of 8-aminoquinoline amides 1, aryldiazonium tetrafluoroborates 2, and the sulfur dioxide surrogate of DABCO·(SO2)23. Excellent selectivity in the para-position is observed for this copper-catalyzed transformation. This reaction is triggered by a copper-chelated complex via the coordination of the copper catalyst with the substrate and an arylsulfonyl radical generated in situ, thus providing 5-sulfonyl-8-aminoquinoline amides in moderate to good yields. Further studies for C–H functionalization with the insertion of sulfur dioxide are under exploration in our laboratory.
Conflicts of interest
There are no conflicts to declare.
Acknowledgements
Financial support from the National Natural Science Foundation of China (No. 21672037 and 21532001) is gratefully acknowledged.
Notes and references
- For selected examples, see:
(a) R. Giri, B.-F. Shi, K. M. Engle, N. Maugel and J.-Q. Yu, Chem. Soc. Rev., 2009, 38, 3242 RSC;
(b) L. McMurray, F. O_Hara and M. J. Gaunt, Chem. Soc. Rev., 2011, 40, 1885 RSC;
(c) J. Wencel-Delord and F. Glorius, Nat. Chem., 2013, 5, 369 CrossRef CAS PubMed;
(d) T. Gensch, M. N. Hopkinson, F. Glorius and J. Wencel-Delord, Chem. Soc. Rev., 2016, 45, 2900 RSC;
(e) J. Yamaguchi, A. D. Yamaguchi and K. Itami, Angew. Chem., Int. Ed., 2012, 51, 8960 CrossRef CAS PubMed;
(f) C. Liu, J. Yuan, M. Gao, S. Tang, W. Li, R. Shi and A. Lei, Chem. Rev., 2015, 115, 12138 CrossRef CAS PubMed;
(g) M. Zhang, Y. Zhang, X. Jie, H. Zhao, G. Li and W. Su, Org. Chem. Front., 2014, 1, 843 RSC;
(h) Z. Huang, H. N. Lim, F. Mo, M. C. Young and G. Dong, Chem. Soc. Rev., 2015, 44, 7764 RSC;
(i) F. Wang, S. Yu and X. Li, Chem. Soc. Rev., 2016, 45, 6462 RSC;
(j) J. R. Hummel, J. A. Boerth and J. A. Ellman, Chem. Rev., 2017, 117, 9163 CrossRef CAS PubMed;
(k) C.-L. Sun, B.-J. Li and Z.-J. Shi, Chem. Rev., 2011, 111, 1293 CrossRef CAS PubMed.
- Z. Chen, B. Wang, J. Zhang, W. Yu, Z. Liu and Y. Zhang, Org. Chem. Front., 2015, 2, 1107 RSC.
- For selected examples, see:
(a) A. El-Awa, M. N. Noshi, X. Mollat du Jourdin and P. L. Fuchs, Chem. Rev., 2009, 109, 2315 CrossRef CAS PubMed;
(b) B. M. Trost, Bull. Chem. Soc. Jpn., 1988, 61, 107 CrossRef CAS;
(c)
N. S. Simpkins, Sulfones in Organic Synthesis, Pergamon Press, Oxford, UK, 1993 Search PubMed;
(d)
The Chemistry of Sulfones and Sulfoxides, ed. S. Patai, Z. Rappoport and C. Stirling, Wiley, Chichester, UK, 1988 Search PubMed.
- For selected examples, see:
(a) G. Zhang, L. Zhang, H. Yi, Y. Luo, X. Qi, C.-H. Tong, L.-Z. Wu and A. Lei, Chem. Commun., 2016, 52, 10407 RSC;
(b) Q. Lu, J. Zhang, F. Wei, Y. Qi, H. Wang, Z. Liu and A. Lei, Angew. Chem., Int. Ed., 2013, 52, 7156 CrossRef CAS PubMed;
(c) A. Kar, I. A. Sayyed, W. F. Lo, H. M. Kaiser, M. Beller and M. K. Tse, Org. Lett., 2007, 9, 3405 CrossRef CAS PubMed;
(d) W. Zhu and D. Ma, J. Org. Chem., 2005, 70, 2696 CrossRef CAS PubMed;
(e) J. Aziz, S. Messaoudi, M. Alami and A. Hamze, Org. Biomol. Chem., 2014, 12, 9743 RSC;
(f) S. Cacchi, G. Fabrizi, A. Goggiamani, L. M. Parisi and R. Bernini, J. Org. Chem., 2004, 69, 5608 CrossRef CAS PubMed;
(g) N.-W. Liu, S. Liang and G. Manolikakes, Adv. Synth. Catal., 2017, 359, 1308 CrossRef CAS;
(h) Y. Xiang, Y. Li, Y. Kuang and J. Wu, Chem. – Eur. J., 2017, 23, 1032 CrossRef CAS PubMed;
(i) C. Shen, P.-F. Zhang, Q. Sun, S.-Q. Bai, T. S. A. Hor and X.-G. Liu, Chem. Soc. Rev., 2015, 44, 291 RSC.
- S. Liang, M. Bolte and G. Manolikakes, Chem. – Eur. J., 2017, 23, 96 CrossRef CAS PubMed.
-
(a) S. Liang and G. Manolikakes, Adv. Synth. Catal., 2016, 358, 2371 CrossRef CAS;
(b) C. Xia, K. Wang, J. Xu, Z. Wei, C. Shen, G. Duan, Q. Zhu and P. Zhang, RSC Adv., 2016, 6, 37173 RSC;
(c) J. Wei, J. Jiang, X. Xiao, D. Lin, Y. Deng, Z. Ke, H. Jiang and W. Zeng, J. Org. Chem., 2016, 81, 946 CrossRef CAS PubMed;
(d) H.-W. Liang, K. Jiang, W. Ding, Y. Yuan, L. Shuai, Y.-C. Chen and Y. Wei, Chem. Commun., 2015, 51, 16928 RSC;
(e) H. Qiao, S. Sun, F. Yang, Y. Zhu, W. Zhu, Y. Dong, Y. Wu, X. Kong, L. Jiang and Y. Wu, Org. Lett., 2015, 17, 6086 CrossRef CAS PubMed;
(f) J. Xu, C. Shen, X. Zhu, P. Zhang, M. J. Ajitha, K.-W. Huang, Z. An and X. Liu, Chem. – Asian J., 2016, 11, 882 CrossRef CAS PubMed;
(g) J.-M. Li, J. Weng, G. Lu and A. S. C. Chan, Tetrahedron Lett., 2016, 57, 2121 CrossRef CAS;
(h) G. Chen, X. Zhang, Z. Zeng, W. Peng, Q. Liang and J. Liu, ChemistrySelect, 2017, 2, 1979 CrossRef CAS.
- For selected examples, see:
(a) Y. Dou, Z. Xie, Z. Sun, H. Fang, C. Shen, P. Zhang and Q. Zhu, ChemCatChem, 2016, 8, 3570 CrossRef CAS;
(b) Y. Yin, J. Xie, F.-Q. Huang, L.-W. Qi and B. Zhang, Adv. Synth. Catal., 2016, 359, 1037 CrossRef;
(c) H. Yi, H. Chen, C. Bian, Z. Tang, A. K. Singh, X. Qi, X. Yue, Y. Lan, J.-F. Leec and A. Lei, Chem. Commun., 2017, 53, 6736 RSC;
(d) A. M. Suess, M. Z. Ertem, C. J. Cramer and S. S. Stahl, J. Am. Chem. Soc., 2013, 135, 9797 CrossRef CAS PubMed;
(e) X. He, Y.-Z. Xu, L.-X. Kong, H.-H. Wu, D.-Z. Ji, Z.-B. Wang, Y.-G. Xu and Q.-H. Zhu, Org. Chem. Front., 2017, 4, 1046 RSC;
(f) J. Xu, X. Zhu, G. Zhou, B. Ying, P. Ye, L. Su, C. Shen and P. Zhang, Org. Biomol. Chem., 2016, 14, 3016 RSC;
(g) C. Shen, J. Xu, B. Ying and P. Zhang, ChemCatChem, 2016, 8, 3560 CrossRef CAS;
(h) L.-K. Jin, G.-P. Lu and C. Cai, Org. Chem. Front., 2016, 3, 1309 RSC;
(i) D. Ji, X. He, Y. Xu, Z. Xu, Y. Bian, W. Liu, Q. Zhu and Y. Xu, Org. Lett., 2016, 18, 4478 CrossRef CAS PubMed;
(j) Y. Wang, Y. Wang, K. Jiang, Q. Zhang and D. Li, Org. Biomol. Chem., 2016, 14, 10180 RSC;
(k) Z. Wu, Y. He, C. Ma, X. Zhou, X. Liu, Y. Li, T. Hu, P. Wen and G. Huang, Asian J. Org. Chem., 2016, 5, 724 CrossRef CAS;
(l) Y. Wang, Y. Wang, Q. Zhang and D. Li, Org. Chem. Front., 2017, 4, 514 RSC.
- For selected examples, see:
(a) D. Zheng, J. Yu and J. Wu, Angew. Chem., Int. Ed., 2016, 55, 11925 CrossRef CAS PubMed;
(b) D. Zheng, Y. An, Z. Li and J. Wu, Angew. Chem., Int. Ed., 2014, 53, 2451 CrossRef CAS PubMed;
(c) S. Ye and J. Wu, Chem. Commun., 2012, 48, 10037 RSC;
(d) S. Ye and J. Wu, Chem. Commun., 2012, 48, 7753 RSC;
(e) S. Ye, H. Wang, Q. Xiao, Q. Ding and J. Wu, Adv. Synth. Catal., 2014, 356, 3225 CrossRef CAS;
(f) Y. An, D. Zheng and J. Wu, Chem. Commun., 2014, 50, 11746 RSC;
(g) Y. Luo, X. Pan, C. Chen, L. Yao and J. Wu, Chem. Commun., 2015, 51, 180 RSC;
(h) D. Zheng, Y. Li, Y. An and J. Wu, Chem. Commun., 2014, 50, 8886 RSC;
(i) D. Zheng, Y. Kuang and J. Wu, Org. Biomol. Chem., 2015, 13, 10370 RSC;
(j) D. Zheng, R. Mao, Z. Li and J. Wu, Org. Chem. Front., 2016, 3, 359 RSC;
(k) Y. Li, D. Zheng, H. Xia and J. Wu, Org. Chem. Front., 2016, 3, 574 RSC;
(l) R. Mao, R. D. Zheng, H. Xia and J. Wu, Org. Chem. Front., 2016, 3, 693 RSC;
(m) K. Zhou, H. Xia and J. Wu, Org. Chem. Front., 2016, 3, 865 RSC;
(n) D. Zheng, M. Chen, L. Yao and J. Wu, Org. Chem. Front., 2016, 3, 985 RSC.
- For selected examples, see:
(a) X. Gong, Y. Ding, X. Fan and J. Wu, Adv. Synth. Catal., 2017, 359, 2999 CrossRef CAS;
(b) Y. Li, R. Mao and J. Wu, Org. Lett., 2017, 19, 4472 CrossRef CAS PubMed;
(c) K. Zhou, H. Xia and J. Wu, Org. Chem. Front., 2017, 4, 1121 RSC;
(d) J. Yu, R. Mao, Q. Wang and J. Wu, Org. Chem. Front., 2017, 4, 617 RSC;
(e) T. Liu, D. Zheng and J. Wu, Org. Chem. Front., 2017, 4, 1079 RSC;
(f) T. Liu, D. Zheng, Y. Ding, X. Fan and J. Wu, Chem. – Asian J., 2017, 12, 465 CrossRef CAS PubMed;
(g) T. Liu, D. Zheng, Z. Li and J. Wu, Adv. Synth. Catal., 2017, 359, 2653 CrossRef CAS;
(h) Y. Xiang, Y. Li, Y. Kuang and J. Wu, Adv. Synth. Catal., 2017, 359, 2605 CrossRef CAS;
(i) J. Zhang, Y. An and J. Wu, Chem. – Eur. J., 2017, 23, 9477 CrossRef CAS PubMed;
(j) Y. Xiang, Y. Kuang and J. Wu, Chem. – Eur. J., 2017, 23, 6996 CrossRef CAS PubMed;
(k) R. Mao, Z. Yuan, Y. Li and J. Wu, Chem. – Eur. J., 2017, 23, 8176 CrossRef CAS PubMed.
- For reviews:
(a) G. Liu, C. Fan and J. Wu, Org. Biomol. Chem., 2015, 13, 1592 RSC;
(b) P. Bisseret and N. Blanchard, Org. Biomol. Chem., 2013, 11, 5393 RSC;
(c) A. S. Deeming, E. J. Emmett, C. S. Richards-Taylor and M. C. Willis, Synthesis, 2014, 2701 Search PubMed;
(d)
D. Zheng and J. Wu, Sulfur Dioxide Insertion Reactions for Organic Synthesis, Nature Springer, 2017 Search PubMed.
- For selected examples, see:
(a) B. Nguyen, E. J. Emmet and M. C. Willis, J. Am. Chem. Soc., 2010, 132, 16372 CrossRef CAS PubMed;
(b) E. J. Emmet, C. S. Richards-Taylor, B. Nguyen, A. Garcia-Rubia, R. Hayter and M. C. Willis, Org. Biomol. Chem., 2012, 10, 4007 RSC;
(c) W. Li, M. Beller and X.-F. Wu, Chem. Commun., 2014, 50, 9513 RSC;
(d) X. Wang, L. Xue and Z. Wang, Org. Lett., 2014, 16, 4056 CrossRef CAS PubMed;
(e) A. S. Deeming, C. J. Russell and M. C. Willis, Angew. Chem., Int. Ed., 2015, 54, 1168 CrossRef CAS PubMed;
(f) E. J. Emmett, B. R. Hayter and M. C. Willis, Angew. Chem., Int. Ed., 2014, 38, 10204 CrossRef PubMed;
(g) M. W. Johnson, S. W. Bagley, N. P. Mankad, R. G. Bergman, V. Mascitti and F. D. Toste, Angew. Chem., Int. Ed., 2014, 53, 4404 CrossRef CAS PubMed;
(h) A. Shavnya, K. D. Hesp, V. Mascitti and A. C. Smith, Angew. Chem., Int. Ed., 2015, 54, 13571 CrossRef CAS PubMed;
(i) A. S. Deeming, C. J. Russell and M. C. Willis, Angew. Chem., Int. Ed., 2016, 55, 747 CrossRef CAS PubMed;
(j) A. S. Tsai, J. M. Curto, B. N. Rocke, A. R. Dechert-Schmitt, G. K. Ingle and V. Mascitti, Org. Lett., 2016, 18, 508 CrossRef CAS PubMed;
(k) W. Zhang and M. Luo, Chem. Commun., 2016, 52, 2980 RSC;
(l) J. Sheng, Y. Li and G. Qiu, Org. Chem. Front., 2017, 4, 95 RSC;
(m) B. Du, Y. Wang, W. Sha, P. Qian, H. Mei, J. Han and Y. Pan, Asian J. Org. Chem., 2017, 6, 153 CrossRef CAS;
(n) A. L. Tribby, I. Rodríguez, S. Shariffudin and N. D. Ball, J. Org. Chem., 2017, 82, 2294 CrossRef CAS PubMed;
(o) Y. Wang, B. Du, W. Sha, H. Mei, J. Han and Y. Pan, Org. Chem. Front., 2017, 4, 1313 RSC.
Footnote |
† Electronic supplementary information (ESI) available: Experimental details and spectral data, copies of 1H and 13C NMR spectra. See DOI: 10.1039/c7qo00866j |
|
This journal is © the Partner Organisations 2018 |