DOI:
10.1039/C6RA02302A
(Paper)
RSC Adv., 2016,
6, 54377-54381
TBAI–HBr system mediated generation of various thioethers with benzenesulfonyl chlorides in PEG400†
Received
26th January 2016
, Accepted 1st June 2016
First published on 2nd June 2016
Abstract
An efficient procedure for the formation of C–S bonds via C–H functionalization was developed for the synthesis of aryl sulfides in good to excellent yields using TBAI–HBr system promoted direct sulfenylation of various compounds, such as phenols, pyrazolones, indoles and related heteroarenes. Low cost and widely available arylsulfonyl chlorides were used as the sulfur source to provide various sulfur-containing compounds. The characteristic of the present protocol is convenient, green, highly efficient with a wide-application and short reaction time.
Introduction
Aryl sulfides as important building blocks for creating molecules often appear in total synthesis, in functional materials science, and in medicinal chemistry.1 In the last few decades, many scientists have reported transition metal (e.g., Pd,2 Cu,3 Ni,4 In,5 Fe,6 Co (ref. 7) etc.) catalyzed cross-coupling reactions of aryl halides, boronic acids and triflates with S-containing nucleophiles. For the preparation of aryl thioethers, the above mentioned methods are the main techniques of traditional synthesis. Aryl thiols and disulfides are often used as the choice of sulfur sources. Nevertheless, many of these sulfur sources are often limited because of their expensiveness, instability and high-toxicity. Therefore, a new, direct, flexible and green sulfenylation of (hetero)arenes to synthesize aryl sulfides under metal-free conditions is always a hot issue for chemists.
In the past few years, many efficient methods to synthesize sulphur compounds have been reported by groups of Yi,8 Tian,9 Hiebel,10 Bolm11 and others.12 Recently, we reported the I2-mediated thiolation of phenol/phenylamine derivatives and sodium arylsulfinates in water.13 Obviously, the synthesis of sulfur-containing compounds have made great progress. From the perspective of green chemistry, scientists have found the other new sulfur sources (e.g., sodium benzenesulfinates, benzenesulfonyl hydrazides, diphenyl disulfides etc.) to replace thiophenols. Although these sulfur sources are environmentally friendly, we are hard to get their derivatives from the market directly. For example, the derivatives of sodium benzenesulfinate and benzenesulfonyl hydrazide need to be synthesized. It is noteworthy that inexpensive and easily available benzenesulfonyl chlorides are their precursor. Apparently, several issues of these strategies should be addressed: (1) use benzenesulfonyl chlorides to replace some sulfur sources that they are green but unavailable, such as sodium benzenesulfinates, benzenesulfonyl hydrazides and so on; (2) find an efficient and green reaction system to synthesize sulfur-containing compounds by directly using benzenesulfonyl chlorides as a sulfur source in the absence of a transition metal catalyst; (3) look for a reaction system is suitable for several compound to synthesize sulfocompounds using benzenesulfonyl chlorides (Scheme 1).
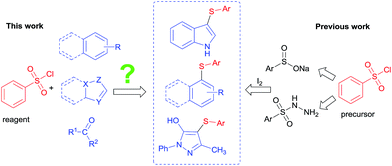 |
| Scheme 1 Methods for the construction of thioethers. | |
In order to solve these problems, PPh3-mediated sulfenylation of indolizines and related heteroarenes by directly using aryl-sulfonyl chlorides as a sulfur source has been reported by You.14 The protocol proves to be an efficient and convenient way to synthesize di(hetero)aryl sulfides using benzenesulfonyl chlorides directly. But in the view of green chemistry, it also encounters disadvantages, such as a mass of PPh3 as reducing agent was used. The reaction was finished in toluene under the conditions of high temperature and longer reaction time. Previous relevant literature indicates that benzenesulfonyl chlorides can be readily reduced to reactive aryl sulfide species,15 which reacts with electron donor compounds to produce various thioethers. To explore a convenient, green and high efficient reaction system which has a wider substrate scope by directly using arylsulfonyl chlorides as a sulfur source thus interested us.
Results and discussion
To prove the feasibility of our proposed assumption, we started the investigation by selecting the reaction of tosyl chloride 1a with 2,7-naphthalenediol 2a as the model reaction (Table 1). From the perspective of green chemistry, poly(ethylene glycol) is an ideal solvent for chemical transformation because of its non-toxic, odorless, neutral, nonvolatile, and non-irritating and is used in a variety of pharmaceuticals and medications. Tosyl chloride was treated with 2,7-naphthalenediol (2a, 1.2 equiv.), tetrabutyl-ammonium iodide (TBAI, 2.0 equiv.) and HCOOH (2.0 equiv.) in 2 mL of PEG400 at 100 °C for 5 h in the opening system (Table 1, entry 7). Not surprisingly, the reaction provided a 52% yield of the desired product 3aa. First, several iodide-containing were initially investigated, and among them TBAI showed the best efficiency to give the corresponding product 3aa in 86% (Table 1, entries 1–4). Then, we screened different solvents, PEG400 proved to be the best choice in the transformation (entries 4, 12–15). The reaction was less efficient when other acid were used (Table 1, entries 4–11). The reactions were investigated using different amounts of TBAI/HBr. The results are shown in Table 1 (entries 16–20). Under the standard conditions, different amounts of TBAI ranging from 1.5 to 2.5 equivalents were applied in the reactions. The obtained yields increased as the TBAI amounts increased up to 2 equivalents to reach the maximum (Table 1, entry 5). Decreasing or increasing the amount of HBr both slightly decreased the reaction yields (Table 1, entries 18–20). Finally, when the reaction was carried out at 50 °C with prolonged reaction time, the yield of 3aa was significantly lower (Table 1, entry 21). On the basis of the results shown in Table 1, the TBAI–HBr system at 100 °C in PEG400 for 5 h emerged as the optimized reaction conditions for follow-up studies.
Table 1 Optimization of the reaction conditiona
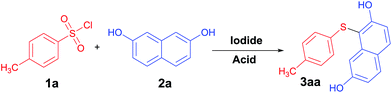
|
Entry |
Iodide (equiv.) |
Acid (equiv.) |
Solvent |
Yieldb (%) |
Conditions: 1a (0.30 mmol), 2a (0.36 mmol), solvent (2 mL), iodide (2.0 equiv.), acid (2.0 equiv.), 5 h, 100 °C, under air. Yield of isolated product. 50 °C. Under N2. |
1 |
NaI (2.0) |
HBr (2.0) |
PEG400 |
23 |
2 |
NH4I (2.0) |
HBr (2.0) |
PEG400 |
40 |
3 |
HI (2.0) |
— |
PEG400 |
27 |
4 |
TBAI (2.0) |
HBr (2.0) |
PEG400 |
86 |
5 |
TBAI (2.0) |
HF (2.0) |
PEG400 |
25 |
6 |
TBAI (2.0) |
HCl (2.0) |
PEG400 |
45 |
7 |
TBAI (2.0) |
HCOOH (2.0) |
PEG400 |
52 |
8 |
TBAI (2.0) |
AcOH (2.0) |
PEG400 |
47 |
9 |
TBAI (2.0) |
TFA (2.0) |
PEG400 |
32 |
10 |
TBAI (2.0) |
PvOH (2.0) |
PEG400 |
27 |
11 |
TBAI (2.0) |
TsOH (2.0) |
PEG400 |
57 |
12 |
TBAI (2.0) |
HBr (2.0) |
Dioxane |
82 |
13 |
TBAI (2.0) |
HBr (2.0) |
Toluene |
75 |
14 |
TBAI (2.0) |
HBr (2.0) |
EtOH |
55 |
15 |
TBAI (2.0) |
HBr (2.0) |
H2O |
42 |
16 |
TBAI (1.5) |
HBr (2.0) |
PEG400 |
69 |
17 |
TBAI (2.5) |
HBr (2.0) |
PEG400 |
85 |
18 |
TBAI (2.0) |
HBr (1.5) |
PEG400 |
71 |
19 |
TBAI (2.0) |
HBr (2.5) |
PEG400 |
84 |
20 |
TBAI (2.0) |
HBr (4.0) |
PEG400 |
76 |
21c |
TBAI (2.0) |
HBr (2.0) |
PEG400 |
51 |
22d |
TBAI (2.0) |
HBr (2.0) |
PEG400 |
80 |
With optimized conditions now in hand, a series of phenols and benzenesulfonyl chlorides were applied in the reaction to investigate the scope and generality of this protocol (Table 2). A range of benzenesulfonyl chlorides which have electron-donating and electron-withdrawing groups reacted with 2 to get the target products 3aa–3dc and 3af–3df in moderate to excellent yields. It's worth mentioning that we have not found C–halogen bond cleavage during the reaction. Besides, apart from phenols, methoxy-benzenes (3d and 3e) were able to react with benzenesulfonyl chlorides to provide the corresponding products 3ad–3de in a few hours in 85–92% yields as well. In general, benzenesulfonyl chlorides with electron-donating groups on the yield of target products were higher than those with electron-withdrawing groups.
Conditions: 1 (0.30 mmol), 2 (0.36 mmol), TBAI (0.60 mmol), HBr (0.60 mmol, 40% aqueous), PEG400 (2 mL), 100 °C, 5 h. |
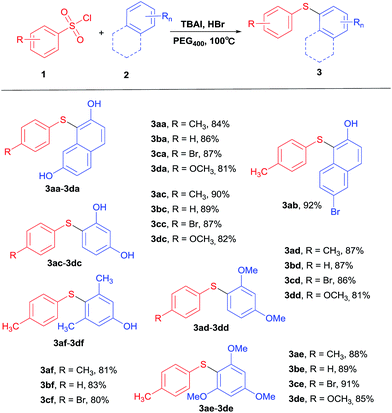 |
On the basis of satisfied results obtained under TBAI–HBr system, we further apply this system to show the functional group tolerance. For this purpose, we carried out the reactions of various benzenesulfonyl chlorides with different benzoheterocycles under the aforementioned conditions (Table 3). It was exhilarating to find that different benzenesulfonyl chlorides having electron-withdrawing and electron-donating halo groups at the 4-position were able to react with indoles (4a) to afford the desired products (5aa–5fa) in good yields under the optimized conditions. Fortunately, indolizines and related heteroarenes were also suitable for this kind of transformation in the presence of HBr. It's worth mentioning that we found benzenesulfonyl chlorides could react with benzoheterocycles under mild conditions. The reaction temperature could go down to 50 °C. Under the optimized conditions, a series of para-substituted benzenesulfonyl chlorides smoothly reacted with 4b and 4c to give desired products in moderate yields (Table 3). According to the results analysis, much lower yields were obtained when indolizines (4c) and related heteroarenes (4b) instead of indoles (4a) were employed in this kind of transformation.
Table 3 Reaction of benzenesulfonyl chlorides with indoles, indolizines and related heteroarenesa
Conditions: 1 (0.30 mmol), 4 (0.36 mmol), TBAI (0.60 mmol), HBr (0.60 mmol, 40% aqueous), PEG400 (2 mL), 50 °C, 2–4 h. |
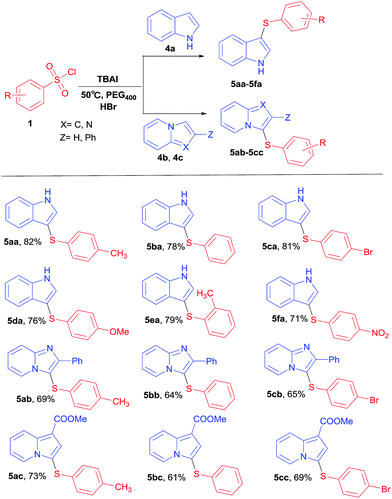 |
To further examine whether our reaction conditions are also suitable for ketone compounds, we sought to expand the scope of sulphenylation of pyrazolones with benzenesulfonyl chlorides under TBAI–HBr system. It was found that the reactions proceeded well, and the results are showed in Table 4. The result showed that pyrazolones reacted well with benzenesulfonyl chlorides bearing electron-donating substituents and electron-withdrawing groups at the para-position giving good and regioselective thioethers 7aa–7fa in good to excellent yields under an air atmosphere. At the same time, we are pleased to find that the reaction was completed in minutes. Moreover, benzenesulfonyl chlorides do not react with phenyl from pyrazolones under the same reaction conditions.
Table 4 Cross-coupling of pyrazolones and benzenesulfonyl chloridesa
Conditions: 1 (0.30 mmol), 6a (0.36 mmol), TBAI (0.60 mmol), HBr (0.60 mmol, 40% aqueous), PEG400 (2 mL), 100 °C, 45 min. |
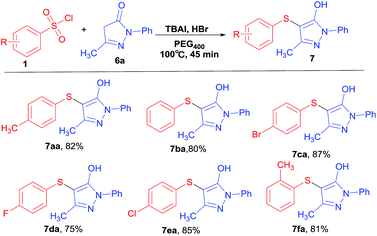 |
In order to gain further insight into the plausible reaction pathway of TBAI–HBr system mediated generation of various thioethers, some control experiments were conducted under various reaction conditions, as showed in Scheme 2. In the presence of 1.0 equivalents of iodine, tosyl chloride 1a reacted with 2,7-naphthalenediol 2a led to the desired product 3aa in 23% yield (Scheme 2a). In the absence of TBAI, treatment of tosyl chloride (1a) with 2,7-naphthalenediol (2a) and HBr (2.0 equiv.) under standard reaction conditions in a closed tube to yield the target product (3aa, 46%), and bromine was detected after the end of reaction. On the other hand, the reaction was carried out in the presence of HI led to 3aa in 27% (Scheme 2c).
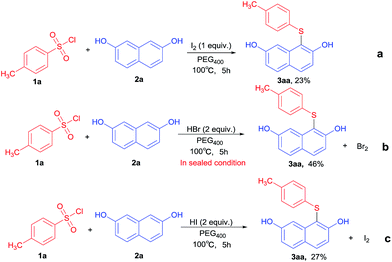 |
| Scheme 2 Control experiments. | |
On the basis of the experimental results described above and together with previous relevant mechanistic studies,14,16 a plausible reaction mechanism for aryl sulfides formation from phenols/benzoheterocycles and benzenesulfonyl chlorides is proposed below (Scheme 3). HI is formed by the reaction of TBAI with acid, then HI and HBr started to reduce benzenesulfonyl chlorides, electrophilic ArSCl (8) was generated, which continued to react with phenols, indole, indolizine and related heteroarene to give intermediates A, B, C and D and then the loss of protons from intermediates A, B, C and D to Cl− regioselectively afforded target products. In another minor pathway (Scheme 3), ArSCl (8) also be formed via reduction of benzenesulfonyl chlorides (1) with iodine.
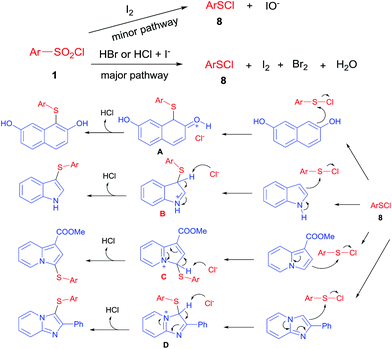 |
| Scheme 3 Proposed mechanisms for aryl sulfides formation from phenols/benzoheterocycles and benzenesulfonyl chlorides. | |
Conclusions
In summary, a classical TBAI–HBr system induced thioether-generating method is reported. By using this system, phenols, ketones, indoles and related heteroarenes thioether derivatives were easily made via using extensive source arylsulfonyl chlorides as sulfur sources, generating highly regioselective ArS-substituted products in moderate to good yields in shorter time. The method has enriched current thioether-producing methods, providing an alternative method to generate thioethers and instead of iodine-induced methods. An investigation on other types of reaction using TBAI–acid system is currently underway.
Experimental section
General methods
4b and 4c were prepared according to the literature procedure.17 The rest of chemicals were purchased from the Sinopharm Chemical Reagent Co., Adamas, Aladdin and TCI used as received.
General procedure: (3)
HBr (40% aqueous, 88 μL, 0.6 mmol) was added to a solution of 2 (0.36 mmol), aryl sulfonyl chlorides 1 (0.30 mmol) and TBAI (221 mg, 0.60 mmol) in PEG400 (2 mL), and the reaction mixture was stirred at 100 °C for 5 h. After completion of the reaction, the reaction mixture was diluted with ethyl acetate, and quenched with saturated sodium thiosulfate solution (10 mL) and extracted twice with ethyl acetate (2 × 15 mL). The organic layer was washed with water and dried over anhyd. sodium sulfate. The solvent was evaporated in vacuo, and the residue was subjected to column chromatography using ethyl acetate in petroleum ether as the eluent to afford the pure target compound 3.
General procedure: (5)
HBr (40% aqueous, 88 μL, 0.6 mmol) was added to a solution of 4 (0.36 mmol), aryl sulfonyl chlorides 1 (0.30 mmol) and TBAI (221 mg, 0.60 mmol) in PEG400 (2 mL), and the reaction mixture was stirred at 50 °C for 2 h. After completion of the reaction, the reaction mixture was diluted with ethyl acetate, and quenched with saturated sodium thiosulfate solution (10 mL) and extracted twice with ethyl acetate (2 × 15 mL). The organic layer was washed with water and dried over anhyd. sodium sulfate. The solvent was evaporated in vacuo, and the residue was subjected to column chromatography using ethyl acetate in petroleum ether as the eluent to afford the pure target product.
General procedure: (7)
HBr (40% aqueous, 88 μL, 0.6 mmol) was added to a solution of 6a (0.36 mmol), aryl sulfonyl chlorides 1 (0.30 mmol) and TBAI (221 mg, 0.60 mmol) in PEG400 (2 mL), and the reaction mixture was stirred at 100 °C for 45 min. After completion of the reaction, the reaction mixture was diluted with ethyl acetate, and quenched with saturated sodium thiosulfate solution (10 mL) and extracted twice with ethyl acetate (2 × 15 mL). The organic layer was washed with water and dried over anhyd. sodium sulfate. The solvent was evaporated in vacuo, and the residue was subjected to column chromatography using ethyl acetate in petroleum ether as the eluent to afford the pure target product.
Acknowledgements
We are grateful for the financial support from the National Natural Science Foundation of China (21302084).
Notes and references
-
(a) T. Nakazawa, J. Xu, T. Nishikawa, T. Oda, A. Fujita, K. Ukai, R. E. P. Mangindaan, H. Rotinsulu, H. Kobayashi and M. Namikoshi, J. Nat. Prod., 2007, 70, 439 CrossRef CAS PubMed;
(b) M. Gingras, Y. M. Chabre, M. Roy and R. Roy, Chem. Soc. Rev., 2013, 42, 4823 RSC;
(c) K. Takimiya, S. Shinamura, I. Osaka and E. Miyazaki, Adv. Mater., 2011, 23, 4347–4370 CrossRef CAS PubMed;
(d) A. Y. Sizov, A. N. Kovergin and A. F. Ermolov, Russ. Chem. Rev., 2003, 72, 357 CrossRef CAS;
(e) T. Mori, T. Nishimura, T. Yamamoto, I. Doi, E. Miyazaki, I. Osaka and K. Takimiya, J. Am. Chem. Soc., 2013, 135, 13900–13913 CrossRef CAS PubMed;
(f) T. Oda, T. Fujiwara, H. Liu, K. Ukai, R. E. P. Mangindaan, M. Mochizuki and M. Namikoshi, Mar. Drugs, 2006, 4, 15 CrossRef.
- M. A. Fernández-Rodríguez, Q. Shen and J. F. Hartwig, J. Am. Chem. Soc., 2006, 128, 2180–2181 CrossRef PubMed.
- E. Sperotto, G. P. van Klink, J. G. de Vries and G. van Koten, J. Org. Chem., 2008, 73, 5625–5628 CrossRef CAS PubMed.
- Y. Zhang, K. C. Ngeow and J. Y. Ying, Org. Lett., 2007, 9, 3495–3498 CrossRef CAS PubMed.
- V. P. Reddy, K. Swapna, A. V. Kumar and K. R. Rao, J. Org. Chem., 2009, 74, 3189–3191 CrossRef CAS PubMed.
- A. Correa, M. Carril and C. Bolm, Angew. Chem., 2008, 47, 2880–2883 CrossRef CAS PubMed.
- Y.-C. Wong, T. T. Jayanth and C.-H. Cheng, Org. Lett., 2006, 8, 5613–5616 CrossRef CAS PubMed.
- Y.-m. Lin, G.-p. Lu, C. Cai and W.-b. Yi, Org. Lett., 2015, 17, 3310–3313 CrossRef CAS PubMed.
- F. L. Yang and S. K. Tian, Angew. Chem., Int. Ed., 2013, 125, 5029–5032 CrossRef.
- M.-A. Hiebel and S. Berteina-Raboin, Green Chem., 2015, 17, 937–944 RSC.
- L.-H. Zou, J. Reball, J. Mottweiler and C. Bolm, Chem. Commun., 2012, 48, 11307–11309 RSC.
-
(a) X. Zhao, L. Zhang, X. Lu, T. Li and K. Lu, J. Org. Chem., 2015, 80, 2918–2924 CrossRef CAS PubMed;
(b) S. K. R. Parumala and R. K. Peddinti, Green Chem., 2015, 17, 4068–4072 RSC;
(c) P. Katrun, S. Hongthong, S. Hlekhlai, M. Pohmakotr, V. Reutrakul, D. Soorukram, T. Jaipetch and C. Kuhakarn, RSC Adv., 2014, 4, 18933–18938 RSC;
(d) X. Kang, R. Yan, G. Yu, X. Pang, X. Liu, X. Li, L. Xiang and G. Huang, J. Org. Chem., 2014, 79, 10605–10610 CrossRef CAS PubMed;
(e) K. Yan, D. Yang, P. Sun, W. Wei, Y. Liu, G. Li, S. Lu and H. Wang, Tetrahedron Lett., 2015, 56, 4792–4795 CrossRef CAS;
(f) J. Sun, J.-K. Qiu, Y.-L. Zhu, C. Guo, W.-J. Hao, B. Jiang and S.-J. Tu, J. Org. Chem., 2015, 80, 8217–8224 CrossRef CAS PubMed;
(g) D. Yang, K. Yan, W. Wei, J. Zhao, M. Zhang, X. Sheng, G. Li, S. Lu and H. Wang, J. Org. Chem., 2015, 80, 6083–6092 CrossRef CAS PubMed.
- D. Wang, R. Zhang, S. Lin, Z. Yan and S. Guo, RSC Adv., 2015, 5, 108030–108033 RSC.
- Q. Wu, D. Zhao, X. Qin, J. Lan and J. You, Chem. Commun., 2011, 47, 9188–9190 RSC.
-
(a) S. R. Dubbaka and P. Vogel, J. Am. Chem. Soc., 2003, 125, 15292–15293 CrossRef CAS PubMed;
(b) G. A. Olah, S. C. Narang, L. D. Field and R. Karpeles, J. Org. Chem., 1981, 46, 2408 CrossRef CAS;
(c) G. A. Olah, S. C. Narang, L. D. Field and G. F. Salem, J. Org. Chem., 1980, 45, 4792 CrossRef CAS;
(d) G. W. Kabalka, M. S. Reddy and M.-L. Yao, Tetrahedron Lett., 2009, 50, 7340 CrossRef CAS.
-
(a) M.-A. Hiebel and S. Berteina-Raboin, Green Chem., 2015, 17, 937–944 RSC;
(b) C.-R. Liu and L.-H. Ding, Org. Biomol. Chem., 2015, 13, 2251–2254 RSC.
-
(a) Y. Yang, K. Cheng and Y. Zhang, Org. Lett., 2009, 11, 5606–5609 CrossRef CAS PubMed;
(b) S.-Y. Takizawa, J.-I. Nishida, T. Tsuzuki, S. Tokito and Y. Yamashita, Inorg. Chem., 2007, 46, 4308–4319 CrossRef CAS PubMed.
Footnote |
† Electronic supplementary information (ESI) available. See DOI: 10.1039/c6ra02302a |
|
This journal is © The Royal Society of Chemistry 2016 |