DOI:
10.1039/D1QO01219C
(Research Article)
Org. Chem. Front., 2021,
8, 6603-6608
Nickel-catalyzed electrochemical reductive relay cross-coupling of alkyl halides with alkyl carboxylic acids†
Received
16th August 2021
, Accepted 7th October 2021
First published on 7th October 2021
Abstract
A highly regioselective Ni-catalyzed electrochemical (undivided cell) reductive relay cross-coupling between alkyl carboxylic acids and alkyl bromides has been developed. This strategy allows the direct acylation of benzylic C(sp3)–H bonds in good yields from commercially available alkyl carboxylic acids, thus providing an alternative strategy for the synthesis of dialkyl ketones. Various functional groups are tolerated under mild reaction conditions.
Introduction
Ketones are versatile and abundant building blocks in organic synthesis and are prevalent in agrochemicals, natural products, and pharmaceuticals.1 The traditional transition metal-catalyzed cross-coupling of organometallics with carboxylic acids or their derivatives is one of the most useful methods for the preparation of ketones.2 Alternatively, reductive Ni-catalyzed cross-coupling between alkyl halides and carboxylic acids or their derivatives has emerged as an appealing strategy for constructing unsymmetrical ketones as it avoids the involvement of organometallic reagents.3 For instance, in 1981 Mukaiyama and co-workers reported an elegant example of Ni-catalyzed acylation of alkyl iodides with substituted pyridyl carboxylates using zinc dust as the reductant, although the method requires extra steps for the synthesis of the substrates, as well as an excess of alkyl iodides.3a In 2012 the groups of Weix and Gong independently developed efficient Ni-catalyzed reductive cross-couplings of alkyl iodides and alkyl carboxylic acid derivatives using Mn or Zn as the reductant (Scheme 1a).3b,c Inspired by these seminal works, numerous Ni-catalyzed reductive cross-couplings between carboxylic acid derivatives and various electrophiles have been developed,4 including asymmetric variants.5 Recently, Wang, Zhu and co-workers demonstrated a Ni-catalyzed migratory reductive acylation between alkyl bromides and alkyl carboxylic acids, although a long reaction time (24 h) was required.6 A common limitation associated with transition metal-catalyzed reductive cross-couplings between alkyl halides and carboxylic acids or their derivatives is their reliance on using stoichiometric amounts of Mn or Zn as reductants. These metals often require surface activation7 and some sensitive functional groups react readily with them. To circumvent such issues, electric current could be used directly to turn over the Ni catalyst.8,9 As early as 1989, Périchon and co-workers reported a Ni-catalyzed electrochemical cross-coupling of alkyl acyl chlorides and benzyl bromides, although Zn was used as a sacrificial anode (Scheme 1b).10 However, to the best of our knowledge, Ni-catalyzed electrochemical reductive relay cross-coupling of alkyl acids and alkyl bromides has not been disclosed.
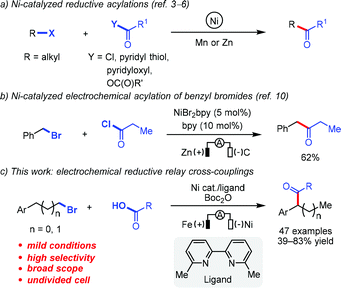 |
| Scheme 1 Reductive cross-coupling reactions. | |
As part of our ongoing interest in the merger of electrochemistry and transition metal catalysis,11 we have recently reported a Ni-catalyzed electrochemical relay cross-coupling of aryl halides and alkyl bromides;12 a very similar method was reported virtually simultaneously by Rueping and co-workers.13 We questioned whether a reductive relay cross-coupling strategy could be applied to the synthesis of ketones from commercially available carboxylic acids and alkyl bromides by taking advantage of “chain-walking” of an alkyl–nickel species.14–17 Herein, we demonstrate that an electrochemical relay cross-coupling of carboxylic acids and alkyl bromides can be executed efficiently at room temperature, affording unsymmetrical dialkyl ketones with good regioselectivity and yield (Scheme 1c).
Results and discussion
Initially, we selected 3-phenylpropionic acid (1a) and (2-bromoethyl)benzene (2a) as reaction partners. To our delight, upon using NiCl2·glyme as the precatalyst, 6,6′-dimethyl-2,2′-bipyridine (ligand 1) as the ligand, MgBr2 as the electrolyte, Boc2O as the acid activation reagent, and N,N-dimethylacetamide (DMA) as the solvent in an undivided cell with iron and Ni foam electrodes under 6 mA current for 6 hours at room temperature, the relay cross-coupling product was obtained in 72% isolated yield (Table 1, entry 1). Using NiBr2·glyme or NiCl2 as the catalyst resulted in lower yields (entries 2 and 3). The efficiency of the reaction decreased precipitously when magnesium, aluminum, or reticulated vitreous carbon (RVC) was chosen as the anode (entries 4–6). It is worth noting that Mg2+ is crucial for the reaction (entries 7 and 8). We reasoned that the magnesium salt contributes to the oxidative addition of low-valent nickel species to the in situ-generated anhydride.3c,4e Varying (higher and lower) the amount of Boc2O did not improve the efficiency (entries 9 and 10). The replacement of L1 with L2 also diminished the yield (entry 11). In addition, the C6-alkyl substituents in the ligand backbone are crucial for the relay reaction (see Table S1 in the ESI† for details). Finally, a control experiment revealed that no coupling product was produced in the absence of the catalyst or ligand (entry 12).
Table 1 Reaction optimization with substrate 1aa
With the optimized reaction conditions in hand, we next investigated the generality of this electrochemical reductive cross-coupling reaction. As shown in Table 2, the catalytic system exhibited good functional group tolerance. The carboxylic acids substituted with a variety of functional groups such as alkyl, ether, fluoro, chloro, amino, ketone, and ester groups were well tolerated. Arenes with electron-rich or electron-poor substituents also gave satisfactory results (3a–3h, 3k, and 3l). To our delight, heteroaromatic rings such as furan and thiophene were also tolerated under the standard conditions, affording acceptable yields (3i and 3j). Furthermore, this protocol could also be applied to more simple alkyl acids with moderate yields (3o–3u). It is worth noting that cyclic carboxylic acids with high ring strain (3v–3y) or simple six-membered rings (3z–3ac) were also well-tolerated and the relay cross-coupling reactions proceeded smoothly with good yields. Encouragingly, for piperidine or amylene oxide carboxylic acids, the reaction afforded the corresponding acylation products in good yields (3ad–3ag). Finally, commercially available pharmaceuticals such as chlorambucil and chrysanthemum acid smoothly underwent electrochemical reductive coupling, affording the indicated product in only a slightly diminished comparative yield (3n and 3w).
Table 2 Evaluation of scope of carboxylic acida
Standard conditions: 1 (0.3 mmol), 2a (0.3 mmol), NiCl2·glyme (10 mol%), ligand 1 (12 mol%), (Boc)2O (2.0 equiv.), MgBr2 (1.0 equiv.), and DMA (4 mL) in an undivided cell with iron (1.5 × 0.5 cm2) and Ni foam (2.5 × 1.5 cm2) as electrodes, rt, 6.0 mA, 6 h.
8.0 mA, 6 h.
7.0 mA, 6 h.
|
|
Next, we examined the breadth of the competent alkyl bromides. As shown in Table 3, alkyl bromides substituted with a variety of functional groups such as ether, chloro, fluoro, trifluoromethyl, trifluoromethoxy, and OTIPS groups were well tolerated under the standard reaction conditions, affording the relay products in good yields (4a–4i and 4k). For alkyl bromide substrates with polysubstituted aromatic rings, the reaction efficiency was not less-satisfying and the relay cross-coupled products (4j and 4m) were obtained with 39% and 40% yield, respectively. To our delight, this relay process can proceed along three carbon-long alkyl chains with only a slightly diminished yield (4n).
Table 3 Evaluation of alkyl halidesa
Standard conditions: 1a or 1k (0.3 mmol), 2 (0.3 mmol), NiCl2·glyme (10 mol%), ligand 1 (12 mol%), (Boc)2O (2.0 equiv.), MgBr2 (1.0 equiv.), and DMA (4 mL) in an undivided cell with iron (1.5 × 0.5 cm2) and Ni foam (2.5 × 1.5 cm2) as electrodes, rt, 6.0 mA, 6 h.
8.0 mA, 6 h.
|
|
To gain insight into the reaction mechanism, we conducted a series of cyclic voltammetric analyses (Fig. 1 and 2, as well as S2–S5 in the ESI†). Compared to the reactants, the nickel catalyst is more easily reduced to a lower valency (Fig. S2†). When the nickel catalyst and ligand were added in an equal amount, the resulting complex exhibits two quasi-reversible reductive peaks at −1.39 V and −1.90 V versus Ag/AgNO3 in dimethylacetamide (curve d, Fig. 1), which may be attributed to the reduction potential of Ni(II)/Ni(I) and Ni(I)/Ni(0), respectively. In addition, in the absence of electricity, the stoichiometric reaction with Ni(cod)2 as the catalyst afforded the desired product in 65% yield (Scheme 2). This result indicated that the Ni(0) species generated at the cathode may act as the active catalyst.
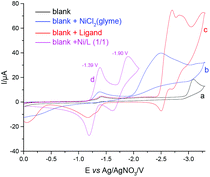 |
| Fig. 1 Cyclic voltammograms recorded on a glassy carbon electrode at 100 mV s−1: (a) blank solution, 0.1 M n-Bu4NBr in DMA; (b) solution (a) with 7.5 mM NiCl2·glyme added; (c) solution (a) with 7.5 mM 2,9-dimethyl-1,10-phenanthroline added; and (d) solution (a) with 7.5 mM NiCl2·glyme and 2,9-dimethyl-1,10-phenanthroline (Ni/L = 1/1) added. | |
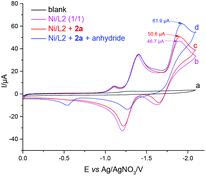 |
| Fig. 2 Cyclic voltammograms recorded on a glassy carbon electrode at 100 mV s−1: (a) blank solution, 0.1 M n-Bu4NBr in DMA; (b) solution (a) with 7.5 mM NiCl2·glyme and ligand 2 added; (c) solution (b) with 10 mM 2a added; and (d) solution (c) with 10 mM 3-phenylpropanoic anhydride added. | |
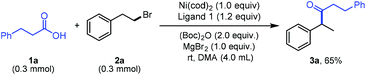 |
| Scheme 2 Stoichiometric reaction without electric current. | |
Next, we sought to understand which reactant the catalyst prefers to react with. 3-Phenylpropanoic anhydride was prepared according to a literature report.18 When the alkyl bromide (2a) was added first, the catalytic current was slightly increased whereas the catalytic current was significantly increased upon the addition of 3-phenylpropanoic anhydride (Fig. 2). Furthermore, when the anhydride was added first, the catalytic current was significantly increased whereas the catalytic current was almost unchanged following the subsequent addition of alkyl bromide (2a) (Fig. S5†). Based on the above cyclic voltammetric (CV) studies, it was found that the putative Ni(0) complex reacts more readily with the anhydride.
Based on literature reports3c,4a,e,19 and our mechanistic studies (see the ESI† for more details), a plausible mechanism is presented for the Ni-catalyzed electrochemical reductive couplings (Scheme 3). First, the Ni(II) catalyst is reduced to Ni(0) (A) via cathodic reduction. After the oxidative addition of the anhydride to Ni(0), acyl Ni(II) species (B) is formed. After the cathodic reduction of B, the resulting acyl Ni(I) species reacted with an alkyl radical to generate the Ni(II) species (C). The direct reductive elimination from C generated a linear by-product, and C is also converted into species E, a more thermodynamically stable benzylic Ni(II) intermediate, via β-hydride elimination to D and reinsertion. Upon reductive elimination, the desired cross-coupling product and the Ni(0) species (A) are formed. A reacted with an alkyl bromide, affording an alkyl radical species and the Ni(I) species (F). Upon cathodic reduction, the active Ni(0) is then regenerated. At this early stage, we cannot rule out other possible pathways, such as the one wherein a Ni(I)/Ni(III) coupling is involved.
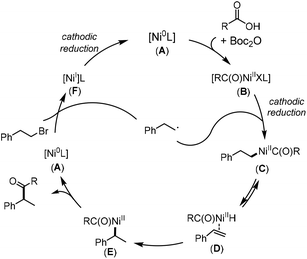 |
| Scheme 3 Plausible catalytic cycle. | |
Conclusions
In summary, we have demonstrated the first example of a Ni-catalyzed electrochemical reductive relay cross-coupling of alkyl acids to alkyl bromides in an undivided cell, affording 1,1-dialkyl ketones in good yields and regioselectivities. The protocol is operationally simple and robust. Further research on exploring the mechanism and developing more transition metal-catalyzed electrochemical reductive relay cross-couplings is currently underway in our laboratory.
Conflicts of interest
The authors declare no competing financial interest.
Acknowledgements
This work was financially supported by the Strategic Priority Research Program of the Chinese Academy of Sciences (Grant XDB20000000), the NSF of China (Grants 21821002, 21772222, and 91956112), and the S&TCSM of Shanghai (Grants 18JC1415600 and 20JC1417100).
References
-
(a) R. K. Dieter, Reaction of Acyl Chlorides with Organometallic Reagents: A Banquet Table of Metals for Ketone Synthesis, Tetrahedron, 1999, 55, 4177–4236 CrossRef CAS;
(b) N. J. Lawrence, Aldehydes and ketones, J. Chem. Soc., Perkin Trans. 1, 1998, 1739–1749 RSC.
-
(a) D. Mistein and J. K. Stille, A General, Selective, and Facile Method for Ketone Synthesis from Acid Chlorides and Organotin Compounds Catalyzed by Palladium, J. Am. Chem. Soc., 1978, 100, 3636–3638 CrossRef;
(b) M. Asaoka, A. Kosaka, M. Tanaka, T. Ueda, T. Houkawa and H. Takei, Chelation controlled cis-selective acylation of 2-(alkoxycarbonyl)-cyclopentylzinc iodides, J. Chem. Soc., Perkin Trans. 1, 1997, 2949–2950 RSC;
(c) D. Wang and Z. Zhang, Palladium-Catalyzed Cross-Coupling Reactions of Carboxylic Anhydrides with Organozinc Reagents, Org. Lett., 2003, 5, 4645–4648 CrossRef CAS PubMed;
(d) Y. Zhang and T. Rovis, A Unique Catalyst Effects the Rapid Room-Temperature Cross-Coupling of Organozinc Reagents with Carboxylic Acid Fluorides, Chlorides, Anhydrides, and Thioesters, J. Am. Chem. Soc., 2004, 126, 15964–15965 CrossRef CAS PubMed;
(e) H. Tatamidani, F. Kakiuchi and N. Chatani, A New Ketone Synthesis by Palladium-Catalyzed Cross-Coupling Reactions of Esters with Organoboron Compounds, Org. Lett., 2004, 6, 3597–3599 CrossRef CAS PubMed;
(f) Y. Yu and L. S. Liebeskind, Copper-Mediated, Palladium-Catalyzed Coupling of Thiol Esters with Aliphatic Organoboron Reagents, J. Org. Chem., 2004, 69, 3554–3557 CrossRef CAS PubMed;
(g) N. A. Weires, E. L. Baker and N. K. Garg, Nickel-catalysed Suzuki–Miyaura coupling of amides, Nat. Chem., 2016, 8, 75–79 CrossRef CAS PubMed;
(h) B. J. Simmons, N. A. Weires, J. E. Dander and N. K. Garg, Nickel-Catalyzed Alkylation of Amide Derivatives, ACS Catal., 2016, 6, 3176–3179 CrossRef CAS PubMed.
-
(a) M. Onaka, Y. Matsuoka and T. Mukaiyama, A Convenient Method for the Direct Preparation of Ketones from 2-(6-(2-methoxyethyl)pyridyl) Carboxylates and Alkyl Iodides by Use of Zinc Dust and A Catalytic Amount of Nickel Dichloride, Chem. Lett., 1981, 10, 531–534 CrossRef;
(b) A. C. Wotal and D. J. Weix, Synthesis of Functionalized Dialkyl Ketones from Carboxylic Acid Derivatives and Alkyl Halides, Org. Lett., 2012, 14, 1476–1479 CrossRef CAS PubMed;
(c) H. Yin, C. Zhao, H. You, K. Lin and H. Gong, Mild ketone formation via Ni-catalyzed reductive coupling of unactivated alkyl halides with acid anhydrides, Chem. Commun., 2012, 48, 7034–7036 RSC.
-
(a) A. C. Wotal, R. D. Ribson and D. J. Weix, Stoichiometric Reactions of Acylnickel(II) Complexes with Electrophiles and the Catalytic Synthesis of Ketones, Organometallics, 2014, 33, 5874–5881 CrossRef CAS PubMed;
(b) J. Wang, B. P. Cary, P. D. Beyer, S. H. Gellman and D. J. Weix, Ketones from Nickel-Catalyzed Decarboxylative, Non-Symmetric Cross-Electrophile Coupling of Carboxylic Acid Esters, Angew. Chem., Int. Ed., 2019, 58, 12081–12085 CrossRef CAS PubMed;
(c) J. Wang, M. E. Hoerrner, M. P. Watson and D. J. Weix, Nickel-Catalyzed Synthesis of Dialkyl Ketones from the Coupling of N-Alkyl Pyridinium Salts with Activated Carboxylic Acids, Angew. Chem., Int. Ed., 2020, 59, 13484–13489 CrossRef CAS PubMed;
(d) F. Wu, W. Lu, Q. Qian, Q. Ren and H. Gong, Ketone Formation via Mild Nickel-Catalyzed Reductive Coupling of Alkyl Halides with Aryl Acid Chlorides, Org. Lett., 2012, 14, 3044–3047 CrossRef CAS PubMed;
(e) C. Zhao, X. Jia, X. Wang and H. Gong, Ni-Catalyzed Reductive Coupling of Alkyl Acids with Unactivated Tertiary Alkyl and Glycosyl Halides, J. Am. Chem. Soc., 2014, 136, 17645–17651 CrossRef CAS PubMed;
(f) X. Jia, X. Zhang, Q. Qian and H. Gong, Alkyl–aryl ketone synthesis via nickel-catalyzed reductive coupling of alkyl halides with aryl acids and anhydrides, Chem. Commun., 2015, 51, 10302–10305 RSC;
(g) M. Zheng, W. Xue, T. Xue and H. Gong, Ester Formation via Nickel-Catalyzed Reductive Coupling of Alkyl Halides with Chloroformates, Org. Lett., 2016, 18, 6152–6155 CrossRef CAS PubMed;
(h) Y. Ai, N. Ye, Q. Wang, K. Yahata and Y. Kishi, Zirconium/Nickel-Mediated One-Pot Ketone Synthesis, Angew. Chem., Int. Ed., 2017, 56, 10791–10795 CrossRef CAS PubMed.
- A. H. Cherney, N. T. Kadunce and S. E. Reisman, Catalytic Asymmetric Reductive Acyl Cross-Coupling: Synthesis of Enantioenriched Acyclic α,α-Disubstituted Ketones, J. Am. Chem. Soc., 2013, 135, 7442–7445 CrossRef CAS PubMed.
- J. He, P. Song, X. Xu, S. Zhu and Y. Wang, Migratory Reductive Acylation between Alkyl Halides or Alkenes and Alkyl Carboxylic Acids by Nickel Catalysis, ACS Catal., 2019, 9, 3253–3259 CrossRef CAS.
-
(a) D. A. Everson, B. A. Jones and D. J. Weix, Replacing Conventional Carbon Nucleophiles with Electrophiles: Nickel-Catalyzed Reductive Alkylation of Aryl Bromides and Chlorides, J. Am. Chem. Soc., 2012, 134, 6146–6159 CrossRef CAS PubMed;
(b) K. A. Johnson, S. Biswas and D. J. Weix, Cross-Electrophile Coupling of Vinyl Halides with Alkyl Halides, Chem. – Eur. J., 2016, 22, 7399–7402 CrossRef CAS PubMed;
(c) K. E. Poremba, N. T. Kadunce, N. Suzuki, A. H. Cherney and S. E. Reisman, Nickel-Catalyzed Asymmetric Reductive Cross-Coupling To Access 1,1-Diarylalkanes, J. Am. Chem. Soc., 2017, 139, 5684–5687 CrossRef CAS PubMed.
-
(a) T. J. DeLano and S. E. Reisman, Enantioselective Electroreductive Coupling of Alkenyl and Benzyl Halides via Nickel Catalysis, ACS Catal., 2019, 9, 6751–6754 CrossRef CAS PubMed;
(b) R. J. Perkins, A. J. Hughes, D. J. Weix and E. C. Hansen, Metal-Reductant-Free Electrochemical Nickel-Catalyzed Couplings of Aryl and Alkyl Bromides in Acetonitrile, Org. Process Res. Dev., 2019, 23, 1746–1751 CrossRef CAS;
(c) H. Li, C. P. Breen, H. Seo, T. F. Jamison, Y.-Q. Fang and M. M. Bio, Ni-Catalyzed Electro-chemical Decarboxylative C–C Couplings in Batch and Continuous Flow, Org. Lett., 2018, 20, 1338–1341 CrossRef CAS PubMed;
(d) F. Lian, K. Xu, W. Meng, H. Zhang, Z. Tan and C. Zeng, Nickel-catalyzed electrochemical reductive decarboxylative coupling of N-hydroxyphthalimide esters with quinoxalinones, Chem. Commun., 2019, 55, 14685–14688 RSC;
(e) T. Koyanagi, A. Herath, A. Chong, M. Ratnikov, A. Valiere, J. Chang, V. Molteni and J. Loren, One-Pot Electrochemical Nickel-Catalyzed Decarboxylative Sp2–Sp3 Cross-Coupling, Org. Lett., 2019, 21, 816–820 CrossRef CAS PubMed;
(f) C. Li, Y. Kawamata, H. Nakamura, J. C. Vantourout, Z. Liu, Q. Hou, D. Bao, J. T. Starr, J. Chen, M. Yan and P. S. Baran, Electrochemically Enabled, Nickel-Catalyzed Amination, Angew. Chem., Int. Ed., 2017, 56, 13088–13093 CrossRef CAS PubMed;
(g) Y. Kawamata, J. C. Vantourout, D. P. Hickey, P. Bai, L. Chen, Q. Hou, W. Qiao, K. Barman, M. A. Edwards, A. F. Garrido-Castro, J. N. deGruyter, H. Nakamura, K. Knouse, C. Qin, K. J. Clay, D. Bao, C. Li, J. T. Starr, C. Garcia-Irizarry, N. Sach, H. S. White, M. Neurock, S. D. Minteer and P. S. Baran, Electrochemically Driven, Ni-Catalyzed Aryl Amination: Scope, Mechanism, and Applications, J. Am. Chem. Soc., 2019, 141, 6392–6402 CrossRef CAS PubMed;
(h) Y. Wang, L. Deng, X. Wang, Z. Wu, Y. Wang and Y. Pan, Electrochemically Promoted Nickel-Catalyzed Carbon–Sulfur Bond Formation, ACS Catal., 2019, 9, 1630–1634 CrossRef CAS;
(i) B. L. Truesdell, T. B. Hamby and C. S. Sevov, General C(sp2)–C(sp3) Cross-Electrophile Coupling Reactions Enabled by Overcharge Protection of Homogeneous Electrocatalysts, J. Am. Chem. Soc., 2020, 142, 5884–5893 CrossRef CAS PubMed.
-
(a) M. Yan, Y. Kawamata and P. S. Baran, Synthetic Organic Electrochemical Methods Since 2000: On the Verge of a Renaissance, Chem. Rev., 2017, 117, 13230–13319 CrossRef CAS PubMed;
(b) J.-Y. Nédélec, J. Périchon and M. Troupel, Organic Electroreductive Coupling Reactions Using Transition Metal Complexes as Catalysts, Top. Curr. Chem., 1997, 185, 141–173 CrossRef;
(c) J. Chaussard, J.-C. Folest, J.-Y. Nédélec, J. Périchon, S. Sibille and M. Troupel, Use of Sacrificial Anodes in Electrochemical Functionalization of Organic Halides, Synthesis, 1990, 369–381 CrossRef CAS.
- H. Marzouk, Y. Rollin, J. C. Folest, J.-Y. Nédélec and J. Périchon, Electrochemical synthesis of ketones from acid chlorides and alkyl and aryl halides catalysed by nickel complexes, J. Organomet. Chem., 1989, 369, C47–C50 CrossRef CAS.
-
(a) K.-J. Jiao, Z.-M. Li, X.-T. Xu, L.-P. Zhang, Y.-Q. Li, K. Zhang and T.-S. Mei, Palladium-catalyzed reductive electrocarboxylation of allyl esters with carbon dioxide, Org. Chem. Front., 2018, 5, 2244–2248 RSC;
(b) D. Liu, H.-X. Ma, P. Fang and T.-S. Mei, Nickel-Catalyzed Thiolation of Aryl Halides and Heteroaryl Halides through Electrochemistry, Angew. Chem., Int. Ed., 2019, 58, 5033–5037 CrossRef CAS PubMed;
(c) H. Qiu, B. Shuai, Y.-Z. Wang, D. Liu, Y.-G. Chen, P.-S. Gao, H.-X. Ma, S. Chen and T.-S. Mei, Enantioselective Ni-Catalyzed Electrochemical Synthesis of Biaryl Atropisomers, J. Am. Chem. Soc., 2020, 142, 9872–9878 CrossRef CAS PubMed;
(d) P.-S. Gao, X.-J. Weng, Z.-H. Wang, C. Zheng, B. Sun, Z.-H. Chen, S.-L. You and T.-S. Mei, Cu(II)/TEMPO-Catalyzed Enanti-oselective C(sp3)–H Alkynylation of Tertiary Cyclic Amines via Shono-type Oxidation, Angew. Chem., Int. Ed., 2020, 59, 15245–15259 Search PubMed.
- K.-J. Jiao, D. Liu, H.-X. Ma, H. Qiu, P. Fang and T.-S. Mei, Nickel-Catalyzed Electrochemical Reductive Relay Cross-Coupling of Alkyl Halides to Aryl Halides, Angew. Chem., Int. Ed., 2020, 59, 6520–6524 CrossRef CAS PubMed.
- G. S. Kumar, A. Peshkov, A. Brzozowska, P. Nikolaienko, C. Zhu and M. Rueping, Nickel-Catalyzed Chain-Walking Cross-Electrophile Coupling of Alkyl and Aryl Halides and Olefin Hydroarylation Enabled by Electrochemical Reduction, Angew. Chem., Int. Ed., 2020, 59, 6513–6519 CrossRef CAS PubMed.
-
(a) B. J. Stokes, S. M. Opra and M. S. Sigman, Palladium-Catalyzed Allylic Cross-Coupling Reactions of Primary and Secondary Homoallylic Electrophiles, J. Am. Chem. Soc., 2012, 134, 11408–11411 CrossRef CAS PubMed;
(b) B. J. Stokes, A. J. Bischoff and M. S. Sigman, Pd(quinox)-catalyzed allylic relay Suzuki reactions of secondary homostyrenyl tosylates via alkene-assisted oxidative addition, Chem. Sci., 2014, 5, 2336–2339 RSC.
-
(a) H. Sommer, F. Juliá-Hernández, R. Martin and I. Marek, Walking Metals for Remote Functionalization, ACS Cent. Sci., 2018, 4, 153–165 CrossRef CAS PubMed;
(b) A. Vassuer, J. Bruffaerts and I. Marek, Remote functionalization through alkene isomerization, Nat. Chem., 2016, 8, 209–219 CrossRef PubMed.
-
(a) F. Zhou, Y. Zhang, X. Xu and S. Zhu, NiH-Catalyzed Remote Asymmetric Hydroalkylation of Alkenes with Racemic α-Bromo Amides, Angew. Chem., Int. Ed., 2019, 58, 1754–1758 CrossRef CAS PubMed;
(b) W. Wang, C. Ding, Y. Li, Z. Li, Y. Li, L. Peng and G. Yin, Migratory Arylboration of Unactivated Alkenes Enabled by Nickel Catalysis, Angew. Chem., Int. Ed., 2019, 58, 4612–4616 CrossRef CAS PubMed;
(c) S.-Z. Sun, M. Börjesson, R. Martin-Montero and R. Martin, Site-Selective Ni-Catalyzed Reductive Coupling of α-Haloboranes with Unactivated Olefins, J. Am. Chem. Soc., 2018, 140, 12765–12769 CrossRef CAS PubMed;
(d) F. Juliá-Hernández, T. Moragas, J. Cornella and R. Martin, Remote carboxylation of halogenated aliphatic hydrocarbons with carbon dioxide, Nature, 2017, 545, 84–88 CrossRef PubMed;
(e) Y. He, Y. Cai and S. Zhu, Mild and Regioselective Benzylic C–H Functionalization: Ni-Catalyzed Reductive Arylation of Remote and Proximal Olefin, J. Am. Chem. Soc., 2017, 139, 1061–1064 CrossRef CAS PubMed;
(f) I. Buslov, F. Song and X. Hu, An Easily Accessed Nickel Nanoparticle Catalyst for Alkene Hydrosilylation with Tertiary Silanes, Angew. Chem., Int. Ed., 2016, 55, 12295–12299 CrossRef CAS PubMed;
(g) J. S. Bair, Y. Schramm, A. G. Sergeev, E. Clot, O. Eisenstein and J. F. Hartwig, Linear-Selective Hydroarylation of Unactivated Terminal and Internal Olefins with Trifluoromethyl-Substituted Arenes, J. Am. Chem. Soc., 2014, 136, 13098–13101 CrossRef CAS PubMed;
(h) W.-C. Lee, C.-H. Wang, Y.-H. Lin, W.-C. Shih and T.-G. Ong, Tandem Isomerization and C–H Activation: Regioselective Hydroheteroarylation of Allylarenes, Org. Lett., 2013, 15, 5358–5361 CrossRef CAS PubMed;
(i) L. K. Johnson, C. M. Killian and M. Brookhart, New Pd(II)- and Ni(II)-Based Catalysts for Polymerization of Ethylene and α-Olefins, J. Am. Chem. Soc., 1995, 117, 6414–6415 CrossRef CAS.
-
(a) Y. He, C. Liu, L. Yu and S. Zhu, Ligand-Enabled Nickel-Catalyzed Redox-Relay Migratory Hydroarylation of Alkenes with Arylborons, Angew. Chem., Int. Ed., 2020, 59, 9186–9191 CrossRef CAS PubMed;
(b) L. Peng, Y. Li, Y. Li, W. Wang, H. Pang and G. Yin, Ligand-Controlled Nickel-Catalyzed Reductive Relay Cross-Coupling of Alkyl Bromides and Aryl Bromides, ACS Catal., 2018, 8, 310–313 CrossRef CAS;
(c) L. Peng, Z. Li and G. Yin, Photochemical Nickel-Catalyzed Reductive Migratory Cross-Coupling of Alkyl Bromides with Aryl Bromides, Org. Lett., 2018, 20, 1880–1883 CrossRef CAS PubMed;
(d) F. Chen, K. Chen, Y. Zhang, Y. He, Y.-M. Wang and S. Zhu, Remote Migratory Cross-Electrophile Coupling and Olefin Hydroarylation Reactions Enabled by in Situ Generation of NiH, J. Am. Chem. Soc., 2017, 139, 13929–13935 CrossRef CAS PubMed;
(e) F. Chen, X. Xu, Y. He, G. Huang and S. Zhu, NiH-Catalyzed Migratory Defluorinative Olefin Cross-Coupling: Trifluoromethyl-Substituted Alkenes as Acceptor Olefins to Form gem-Difluoroalkenes, Angew. Chem., Int. Ed., 2020, 59, 5398–5402 CrossRef CAS PubMed;
(f) Y. Zhang, X. Xu and S. Zhu, Nickel-catalysed selective migratory hydrothiolation of alkenes and alkynes with thiols, Nat. Commun., 2019, 10, 1752–1760 CrossRef PubMed;
(g) Y. Zhang, B. Han and S. Zhu, Rapid Access to Highly Functionalized Alkyl Boronates by NiH-Catalyzed Remote Hydroarylation of Boron-Containing Alkenes, Angew. Chem., Int. Ed., 2019, 58, 13860–13864 CrossRef CAS PubMed;
(h) Y. Li, D. Wu, H.-G. Cheng and G. Yin, Difunctionalization of Alkenes Involving Metal Migration, Angew. Chem., Int. Ed., 2020, 59, 7990–8003 CrossRef CAS PubMed;
(i) Y. Li, Y. Li, L. Peng, D. Wu, L. Zhu and G. Yin, Nickel-catalyzed migratory alkyl–alkyl cross-coupling reaction, Chem. Sci., 2020, 11, 10461–10464 RSC;
(j) W. Wang, C. Ding and G. Yin, Catalyst-controlled enantioselective 1,1-arylboration of unactivated olefins, Nat. Catal., 2020, 3, 951–958 CrossRef CAS.
- Md. C. Sheikh, S. Takagi, T. Yoshimura and H. Morita, Mechanistic studies of DCC/HOBt-mediated reaction of 3-phenylpropionic acid with benzyl alcohol and studies on the reactivities of ‘active ester’ and the related derivatives with nucleophiles, Tetrahedron, 2010, 66, 7272–7278 CrossRef CAS.
- C. Amatore, A. Jutand, J. Périchon and Y. Rollin, Mechanism of the Nickel-Catalyzed Electrosynthesis of Ketones by Heterocoupling of Acyl and Benzyl Halides, Monatsh. Chem., 2000, 131, 1293–1304 CrossRef CAS.
Footnote |
† Electronic supplementary information (ESI) available: Experimental procedures, product characterization, copies of NMR spectra. See DOI: 10.1039/d1qo01219c |
|
This journal is © the Partner Organisations 2021 |
Click here to see how this site uses Cookies. View our privacy policy here.