DOI:
10.1039/D0RA06488B
(Review Article)
RSC Adv., 2020,
10, 44654-44671
The bioactive components as well as the nutritional and health effects of sea buckthorn†
Received
26th July 2020
, Accepted 24th November 2020
First published on 17th December 2020
Abstract
Sea buckthorn (SB), also named sea berry, Hippophae rhamnoides L. or Elaeagnus rhamnoides L., has been used in daily life for centuries with kinds of purposes ranging from a beverage with a pleasant taste and flavor, to an agent for treatment of many disorders and diseases. SB is well known more than just a fruit. So far, a unique mixture of bioactive components was elucidated in SB including flavonoids, phenolic acids, proanthocyanidins, carotenoids, fatty acids, triterpenoids, vitamins and phytosterols, which implied the great medicinal worth of this seaberry. Both in vitro and in vivo experiments, ranged from cell lines to animals as well as a few in patients and healthy volunteers, indicated that SB possessed various biological activities including anti-inflammatory and immunomodulatory effects, antioxidant properties, anti-cancer activities, hepato-protection, cardiovascular-protection, neuroprotection, radioprotection, skin protection effect as well as the protective effect against some eye and gastrointestinal sickness. Furthermore, the toxicological results revealed neither the fruits, nor the seeds of SB were toxic. The present review summarizes the unique profile of the chemical compounds, the nutritional and health effects as well as the toxicological properties of SB, which lay the foundation for practical applications of SB in treatment of human diseases.
1. Introduction
Sea buckthorn (SB, Hippophae rhamnoides L. or Elaeagnus rhamnoides L. A. Nelson), a well-known plant consumed in different parts of the world for both nutritional and medicinal uses, was a hardy but deciduous shrub belonging to the genus Hippophae L. Presently, 6 species and 12 subspecies were generally acknowledged worldwide, as Table 1 showed, and 8 of 12 subspecies (subsp.) originated from SB, including SB subsp. carpatica, SB subsp. caucasica, SB subsp. fluviatilis, SB subsp. mongolica, SB subsp. rhamnoides, SB subsp. sinensis, SB subsp. turkestanica and SB subsp. yunnanensis, and 4 of 8, consisting of SB subsp. sinensis, SB subsp. mongolica, SB subsp. turkestanica and SB subsp. yunnanensis were flourish abundantly in northwest and southwest region of China. Besides China, SB was also widely distributed throughout Mongolia, Russia, United Kingdom, France, Denmark, Netherlands, Germany, Poland, Finland, Sweden, and Norway, which implied the strong survivability of this plant. Unexpectedly, data proved that SB could survive even under rigorous climatic conditions, with the temperatures ranged from −43 °C to +40 °C, high soil pH up to 8.0, not matter how drought, salinity and poor soil it was.1,2
Table 1 The classification of the genus Hippophae L
Species |
Subspecies |
Hippophae rhamnoides L. |
Hippophae rhamnoides subsp. carpatica Rousi |
Hippophae rhamnoides subsp. caucasica Rousi |
Hippophae rhamnoides subsp. fluviatilis van Soest |
Hippophae rhamnoides subsp. mongonica Rousi |
Hippophae rhamnoides subsp. rhamnoides |
Hippophae rhamnoides subsp. sinensis Rousi |
Hippophae rhamnoides subsp. turkestanica Rousi |
Hippophae rhamnoides subsp. yunnanensis Rousi |
Hippophae salicifolia D. Don |
— |
Hippophae goniocarpa (Lian) X. L. Chen et K. Sun |
Hippophae goniocarpa subsp. litangensis Lian et X. L. Chen |
Hippophae goniocarpa subsp. goniocarpa Lian |
Hippophae gyantsensis (Rousi) Lian |
— |
Hippophae neurocarpa S.W. Liu et T.N. He |
Hippophae neurocarpa subsp. stellatopilosa Lian et X. L. Chen |
Hippophae neurocarpa subsp. neurocarpa S. W. Liu et T. N. He |
Hippophae tibetana Schlecht. |
— |
SB was traditionally used for both food and medicinal purposes in Europe and Asia. In ancient Greece, local people fed their horses with the leaves and branches of SB for increasing weights and invigorating yang as a result of its nutritional and health effects. And according to the ancient Chinese records, SB was recorded possessing the powerful of removing phlegm, benefiting lung, nourishing stomach, strengthening spleen, promoting blood circulation, removing blood stasis, and etc.1,3 Well into the middle period of last century, to be precise in 1958, the alcohol extract of SB bark had been used for the treatment of transplantable tumors.4 And in last period of nineteenth century, SB had gradually came to the attention of researchers. Studies investigated its influence on hyperlipidemic serum cultured smooth muscle cells in vitro and suggested SB was an effective antioxidant;5 and β-sitosterol, a major constituent in SB seed oil, showed anti-gastroulcerative property in rats.6 During this period, some flavonoids and triterpenes in SB were also isolated and identified by researchers.7,8 In recent years, more and more studies have been done on SB, in which some traditional pharmacological actions were confirmed and novel pharmacological effects were discovered through modern scientific research methods. Specifically, SB oil was proved could prevent and treat experimental gastric ulcers in rats,9 which verified the traditional therapeutic effect of “Nourishing stomach”. A study paper published by Basu et al. indicated SB seed oil possessed the effect of anti-hyperlipidemia and resisting atherosclerosis;10 in addition, the total flavones of SB could inhibit platelet aggregation in vitro and thrombosis in mouse femoral artery;11 both studies corresponded to the role of SB in “promoting blood circulation and removing blood stasis”. In addition, some experiments also showed that SB possessed radiation protection, neuroprotection and anti-tumor effects.12–14 Furthermore, the underlying mechanisms involved in these biological activities also became of interest to researchers. For example, the hepatoprotective activity of the polysaccharide extract of SB was considered to be related to the activation of the Nrf-2/HO-1-SOD-2 signaling,15 whereas the anti-inflammatory activity of SB polysaccharides was associated with inhibiting TLR4/NF-kappaB pathway.16 Based on the above various pharmacological properties, several reviews about SB were also published. A review published in 2020 summarized the potential applications of SB in food and feed industry, and it focused on the development and application of SB products.17 In another review published in 2016, the biological activities and safety of SB were summarized, and the antioxidant activity of SB was evaluated emphatically, 18 antioxidant compounds in different parts of SB were listed.18 The present review aimed at summarizing the phytochemistry, biological activities and toxicological of SB comprehensively and systematically, to make clear the bioactive components as well as the nutritional and health effects of SB. On the one hand, we elaborated 10 bioactive classes of components including flavonoids, phenolic acids etc. (Fig. 1) and 12 biological activities including anti-inflammatory and antioxidant etc. (Fig. 2) in SB. On the other hand, we emphasized the relevance of anti-inflammatory and antioxidant effect to other pharmacological actions as well as the corresponding bioactive compounds of SB. Furthermore, for each of the pharmacological activities of SB, we discussed gaps of existing research and presented what should be paid attention to in further research.
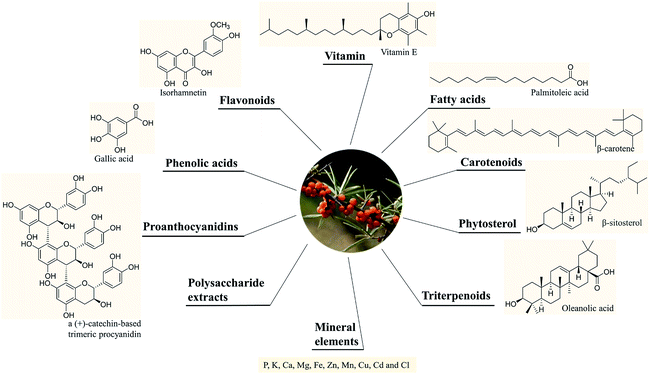 |
| Fig. 1 The main active components in SB. | |
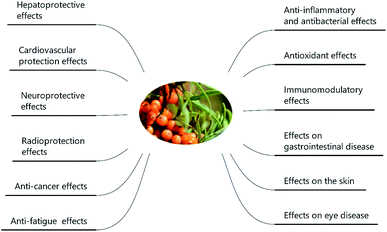 |
| Fig. 2 The main pharmacological activities of SB. | |
2. Chemical constituents and the corresponding contents
A variety of chemical ingredients including flavonoids, phenolic acids, proanthocyanidins, carotenoids, fatty acids, triterpenoids, liganas, vitamin (vitamin C and E, etc.) and phytosterols were found in fruits, leaves and seeds of SB, as ESI Tab. 1† showed, and most of their structures were descripted in Fig. 3. The phytochemistry review of SB explored that the nutrition constituents including flavonoids, vitamins, fatty acids, carotenoids and phytosterols as well as mineral elements were particularly rich in SB. Follows were the detailed information of the main constituents of SB.
 |
| Fig. 3 Structures of flavonoids, phenolic acids and triterpenoids identified in SB (the structures of compound no. 42–47 can not be supplied due to unclear connection location). | |
2.1 Flavonoids and phenolic compounds
More than 60 flavonoids and 10 phenolic acids were founded in SB (ESI Tab. 1†), and their structures were showed in Fig. 3. In SB fruits, over 98% of flavonoids were flavonols; and isorhamnetin derivatives were dominant (from 66% to 72% of total flavonols), followed by quercetin derivatives (from 25% to 32% of total flavonols).19 The variations of the contents of flavonoids were found among different subspecies, growth sites and cultivation methods,20,21 as Fig. 4(A) showed, the contents of total flavonol glycosides, isorhamnetin glycosides, quercetin glycosides and flavonol aglycones in fruits of SB subsp. mongolica and SB subsp. sinensis were totally different. Specifically, the contents of the total proanthocyanidins in fruits of SB subsp. rhamnoides, SB subsp. sinensis and SB subsp. mongolica were 340–1941 mg/100 g, 574–1587 mg/100 g, and 389–880 mg/100 g, respectively, and those differences were mainly affected by origins and growth conditions.22,23
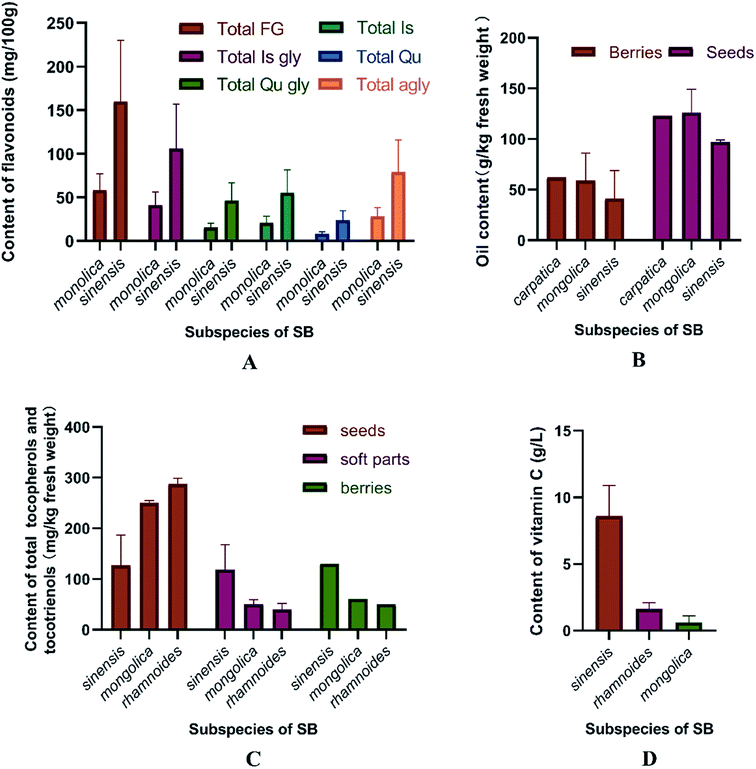 |
| Fig. 4 (A) The contents of total flavonol glycosides, oil, tocopherols, tocotrienols and vitamin C in different parts and subspecies of SB.20 (B) The oil contents in the whole fruits and seeds in three different subspecies of SB.24,25 (C) The total contents of tocopherols and tocotrienols in seeds, soft parts and the whole fruits in different subspecies of SB.33 (D) Vitamin C contents in juice of SB fruits in different subspecies.33 FG, flavonol glycosides; gly, glycoside; Is, isorhamnetin; Qu, quercetin; agly, aglycones. | |
2.2 Fatty acids, carotenoids and phytosterols
The contents of fatty acids, phytosterols and carotenoids of different parts of SB in different SB subspecies were presented in Table 2. It was a widely held view that the amount of the oil in seeds was higher than in other parts of an herbal medicinal plant; and in detail, the oil content of seeds of SB ranged from 94 to 165 mg per g of fresh weight, which was higher than of whole fruits of 20 to 105 mg per g of fresh weight. Furthermore, different subspecies of SB also presented different oil contents and fatty acids compositions as Fig. 4(B) presented.24–26 In seed oil, linoleic acid was the predominant fatty acids whereas palmitoleic acid showed an extremely low level; while in the oil of pulp/peel and whole fruits, palmitoleic acid, palmitic acid and oleic acid were all the major fatty acids;25–28 and in contrast to the whole fruits and pulp oils, seed oil showed a higher amount of polyunsaturated fatty acids. Besides fatty acids, carotenoids and phytosterols were also found rich in SB. A total of 27 carotenoids, including xanthophylls, carotenes and esterified carotenoids, were identified in SB fruits; β-carotene and zeaxanthin di-palmitate were the predominant carotenoids, the contents were 1.9–7.5 mg per 100 g and 6.4–18.3 mg per 100 g of dry weight of SB, respectively; however, when concerning the leaves of SB, only several free carotenoids including lutein, β-carotene, violaxanthin and neoxanthin were found; and among which, lutein had a highest level and its average content was 0.9 mg per 100 g of dry weight.29 Data showed that the contents of carotenoids could be affected by cultivar, harvest time, and origin of the plant.27,30 Concerning to phytosterols, β-sitosterol was the main phytosterols in SB, and a highest total sterol level was obtained by means of supercritical carbon dioxide extraction as compared with hexane and cold press extractions.31
Table 2 Composition of fatty acids, tocopherols and tocotrienols, phytosterols and carotenoids in SB
Compound |
Whole fruits |
Seeds |
Pulp/peel |
Leaves |
Subspecies |
Fatty acids (weight% of total fatty acids)26 |
Palmitic acid |
22.9 |
8.7 |
26.7 |
|
sinensis |
Palmitoleic acid |
21.5 |
<0.5 |
27.2 |
|
Stearic acid |
1.5 |
2.5 |
1.3 |
|
Oleic acid |
17.6 |
19.4 |
17.1 |
|
Vaccenic acid |
6.7 |
2.2 |
8.1 |
|
Linoleic acid |
18.6 |
40.9 |
12.7 |
|
α-Linolenic acids |
11.2 |
26.6 |
7.1 |
|
![[thin space (1/6-em)]](https://www.rsc.org/images/entities/char_2009.gif) |
Tocopherols and tocotrienols (percentage of total tocopherols and tocotrienols, %)24 |
α-Tocopherol |
75.7–89.2 |
17.2–66.1 |
|
|
sinensis & mongolica |
β-Tocopherol |
2.4–12.2 |
5.0–13.8 |
|
|
γ-Tocopherol |
4.0–10.8 |
25.3–55.8 |
|
|
δ-Tocopherol |
0.3–2.4 |
1.7–10.7 |
|
|
β-Tocotrienol |
0.4–4.8 |
1.9–7.6 |
|
|
α-Tocotrienol |
0.4–3.2 |
N. D. |
|
|
γ-Tocotrienol |
0.6–2.5 |
N. D. |
|
|
![[thin space (1/6-em)]](https://www.rsc.org/images/entities/char_2009.gif) |
Phytosterols [mean ± SD (n = 3), mg/100 g]27 |
Campesterol |
22.2 ± 0.5 |
28.1 ± 1.4 |
18.23 ± 0.5 |
|
yunnanensis |
β-Sitosterol |
579.4 ± 18.6 |
749.5 ± 18.8 |
398.3 ± 16.3 |
|
Δ5-Avenasterol |
32.5 ± 0.7 |
229.8 ± 16.5 |
N. D. |
|
Cycloartenol |
112.6 ± 3.1 |
103.2 ± 9.8 |
147.9 ± 12.8 |
|
Gramisterol |
3.9 ± 0.1 |
31.2 ± 19.6 |
N. D. |
|
Others |
111.1 ± 6.6 |
108.1 ± 6.4 |
147.8 ± 42.9 |
|
Sum |
861.6 ± 24.7 |
1249.8 ± 28.5 |
712.2 ± 27.5 |
|
![[thin space (1/6-em)]](https://www.rsc.org/images/entities/char_2009.gif) |
Carotenoids (mg/100 g of dry weight)29 |
Total carotenoids |
53.1–96.7 |
|
|
3.5–4.2 |
carpatica |
Xanthophylls |
2.0–4.4 |
|
|
1.8–2.9 |
Carotenes |
9.9–22.6 |
|
|
1.5–2.0 |
Esterified carotenoids |
40.0–74.1 |
|
|
N. D. |
2.3 Vitamin and mineral elements
The compositions of tocopherols and tocotrienols in whole fruits and seeds were showed in Table 2. α-Tocopherol was the major compound in whole fruits of SB and its content varied from 43 to 116 mg kg−1.24 Vitamin C contents were 0.98–3.65 g per kg and 22.81–46.32 g per kg of fresh weight in SB fruits and leaves, respectively.32 The contents of tocopherols and tocotrienols in different parts and subspecies of SB were showed in Fig. 4(C), and vitamin C content in juice of SB fruits of different subspecies was showed in Fig. 4(D). The large variations on the contents of above nutrition depended on harvest date, cultivar, and year of growth.33,34 In addition, vitamin B12, inositols,35 methylinositols, pantothenic acids36 and vitamin K1,37 as well as mineral elements including P, K, Ca, Mg, Fe, Zn, Mn, Cu, Cd and Cl were also identified and determined in SB fruits.38,39
3 The nutritional and health effects of SB
3.1 Anti-inflammatory and immunomodulatory effects
3.1.1 Anti-inflammatory effect. SB extract or preparation as well as the isolated component was found to possess anti-inflammatory activity, thus may be used for the prevention or treatment of periodontal inflammation,40 oropharyngeal mucositis,41 skin inflammatory disease,42,43 endotoxin induced sepsis44 and allergic symptoms,45 etc. Isorhamnetin, a flavonoid abundant in the fruit of SB, showed a variety of anti-inflammatory activities.46–49 Besides ursolic acid and oleanolic acid,45 two well-known anti-oxidant and anti-inflammatory agents widely applied in cosmetics, casuarinin was also showed potent anti-inflammatory property.50 The detailed parameters of experiments including experimental models, dosage and possible mechanisms were listed in ESI Tab. 2.† It was reported that the anti-inflammatory mechanisms were mostly related to the reduction of pro-inflammatory factors levels and inhibition of nuclear factor (NF)-κB signaling pathways as Fig. 5 described.
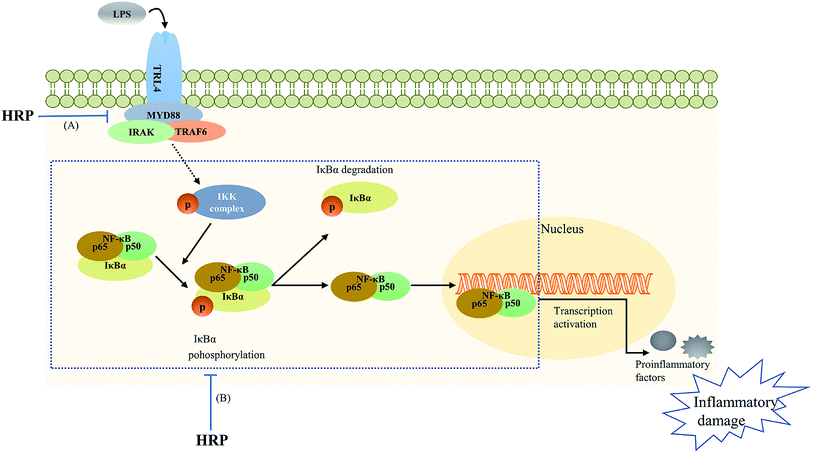 |
| Fig. 5 The polysaccharide extract of SB (HRP) protected against lipopolysaccharide (LPS)-induced inflammatory related damage via inhibiting toll-like receptor 4 (TLR4)-NF-κB signaling pathway. (A) HRP decreased TLR4 and myeloid differentiation factor 88 (MyD88) levels; (B) HRP inhibited the phosphorylation of ikappa B kinase (IKK), inhibitor of κB (IκB) as well as the activation and translocation of NF-κB.16,51 | |
3.1.2 Antibacterial property. SB possessed broad spectrum antibacterial activity (Table 3), and the inhibition activity, expressed as the diameter of inhibition zones, inhibition percentage or minimum inhibitory concentration (MIC), of SB extract was varied according to extraction solvent, organs and strains. The methanol extract of SB seeds was found to possess higher antibacterial activity than chloroform, and acetone extracts;52 and the phenolic rich fraction (ethyl acetate fraction) of the crude extract (70% ethanol extract) of SB leaves was found to be more active than that of crude extract, with the inhibition zone of 20.67 mm and 15.23 mm against Shigella dysenteriae, respectively;53 whereas the water fraction of the crude ethanol extract (leaves, stems, roots, seeds) was found to be the most efficient fraction, particularly for Bacillus cereus and Staphylococcus aureus, the water fraction of SB seeds showed inhibition percentage of 89 ± 5% against Bacillus cereus and the water fraction of SB leaves showed inhibition percentage of 85 ± 12% to Staphylococcus aureus.54 The MIC values of the ethanol and aqueous extract of SB leaves against Helicobacter pylori were 60 μg mL−1 and >100 μg mL−1, respectively.55 The aqueous extract of SB leaves (500 μg) had inhibition zones of 18 mm against Pseudomonas aeruginosa; the hydroalcoholic extract of SB leaves (500 μg) had inhibition zones of 19 mm against Bacillus cereus; whereas both only had zone of inhibition of 9 mm for Escherichia coli.56 The ethanol extract of SB leaves, stems, roots, seeds (100 μg mL−1) all showed weak inhibition against Pseudomonas aeruginosa with inhibition percentage of 16–28%.54 However, SB pulp oil had MIC value of 0.19 mg mL−1 against Bacillus subtilis; SB pulp oil and seed oil had MIC value of 0.10 mg mL−1 and 1.52 mg mL−1 against Bacillus coagulans, respectively, which all showed stronger inhibitory effects than tetracycline hydrochloride.57
Table 3 The antimicrobial activities of different part and extract of SB
Strains |
Parts/sample (zone of inhibition (mm)/MIC/inhibition percentage (%)) |
Gram+ |
Bacillus cereus |
Leaves/the aqueous and 70% ethanol extract (125–500 μg, 11–19 mm); seeds/the methanol extract (200 ppm); seeds (24.39 mg mL−1), pulp (3.05 mg mL−1), and leaves (48.78 mg mL−1)/the essential oil; leaves (32%), stems (41%), roots (45%) and seeds (64%)/the ethanol extract (100 μg mL−1)52,54,56,57 |
Bacillus coagulan |
Seeds/the methanol extract (300 ppm); seeds (6.10 mg mL−1), pulp (0.10 mg mL−1), and leaves (1.52 mg mL−1)/the essential oil52,57 |
Bacillus subtilis |
Seeds/the methanol extract (300 ppm); seeds (1.52 mg mL−1), pulp (0.19 mg mL−1), and leaves (3.05 mg mL−1)/the essential oil52,57 |
Listeria monocytogenes |
Seeds/the methanol extract (300 ppm); seeds/the aqueous extract (750 ppm)52,60 |
Staphylococcus aureus |
Leaves/the aqueous and 70% ethanol extract (250–500 μg, 10–14 mm); leaves (72%), stems (36%), roots (25%) and seeds (41%)/the ethanol extract (100 μg mL−1); seeds (12.20 mg mL−1), pulp (12.20 mg mL−1), and leaves (12.20 mg mL−1)/the essential oil; leaves/the 70% ethanol extract/phenolic rich fraction (ethyl acetate fraction) (100 μg to 1 mg, 9.35–18.84 mm)53,54,56,57 |
Methicillin-resistant Staphylococcus aureus |
Fruits/n-hexane (6 mg mL−1, 22.93 mm) and chloroform extract (6 mg mL−1, 23.37 mm); leaves/n-hexane extract (6 mg mL−1, 24.93 mm)61 |
Enterococcus faecalis |
Leaves/the aqueous and hydroalcoholic extract (250–500 μg, 9–15 mm)56 |
Enterecoccus durans |
Leaves (40%), stems (34%), roots (63%) and seeds (68%)/the ethanol extract (100 μg mL−1)54 |
Streptococcus pneumoniae |
Leaves/the 70% ethanol extract/phenolic rich fraction (ethyl acetate fraction) (100 μg to 1 mg, 10.87–19.0 mm)53 |
Staphylococcus epidermidis |
Dried fruits/the aqueous extract58 |
![[thin space (1/6-em)]](https://www.rsc.org/images/entities/char_2009.gif) |
Gram− |
Helicobacter pylori |
Leaves/the ethanol (60 μg mL−1) and aqueous (>100 μg mL−1) extract55 |
Yersinia enterocolitica |
Seeds/the methanol extract (350 ppm) |
Seeds/the aqueous extract (1000 ppm)52,60 |
Pseudomonas aeruginosa |
Leaves/the aqueous and 70% ethanol extract (125–500 μg, 12–18 mm); leaves (24%), stems (22%), roots (16%) and seeds (28%)/the ethanol extract (100 μg mL−1)54,56 |
Escherichia coli |
Leaves/the aqueous and 70% ethanol extract (500 μg, 9 mm); leaves (42%), stems (39%), roots (40%) and seeds (38%)/the ethanol extract (100 μg mL−1); seeds (6.10 mg mL−1), pulp (12.20 mg mL−1), and leaves (12.20 mg mL−1)/the essential oil; leaves/the 70% ethanol extract/phenolic rich fraction (ethyl acetate fraction) (100 μg to 1 mg, 8.0–15.38 mm)53,54,56,57 |
Salmonella typhi |
Leaves/the 70% ethanol extract/phenolic rich fraction (ethyl acetate fraction) (100 μg to 1 mg, 8.0–18.38 mm)53 |
Shigella dysenteriae |
Leaves/the 70% ethanol extract/phenolic rich fraction (ethyl acetate fraction) (100 μg to 1 mg, 8.33–20.67 mm)53 |
![[thin space (1/6-em)]](https://www.rsc.org/images/entities/char_2009.gif) |
Fungus |
Candida albicans |
Leaves (67%), stems (53%), roots (55%) and seeds (68%)/the crude ethanol extract (100 μg mL−1); twigs (250 μg mL−1) and leaves (31.5 μg mL−1)/the extract54,59 |
Candida glabrata |
Twigs (15.6 μg mL−1) and leaves (3.9 μg mL−1)/the extract59 |
Besides SB itself exhibited antibacterial activity, a synergistic effect was also found when SB combined with other agents. Synergistic effect was observed in SB aqueous extract (zone of inhibition was about 15 mm) in combination (zone of inhibition was about 30 mm) with antibiotics especially erythromycin (zone of inhibition was about 16 mm) against Staphylococcus epidermidis isolate IIDRL-SEP/W-10, which showed about 50% increase in antimicrobial activity.58 In addition, synergistic effect was also found in SB twig and leaf extracts co-action with antifungal drug fluconazole and caspofungin towards Candida albicans and Candida glabrata.59
3.1.3 Immunomodulatory activity. Dozens of references revealed that SB leaf extract, SB oil and active ingredients including flavone and polysaccharides possessed immunomodulatory activity, as ESI Tab. 2† showed. The ethanolic extract of SB leaves increased IFN-γ, CD25 and MHC II expressions thus enhancing immune activity in elderly mice.62 The supercritical carbon dioxide extract of SB leaves could increase the antibody level and cell mediated immune response in mice which immunized with tetanus and diphtheria toxoids.63 The oil prepared from SB fresh fruits, seeds and peels protected rats against chronic stress-induced inhibitory function of natural killer cells, which related to the regulation of neuroendocrine-immune-regulatory network.64 Concerning the active fractions of SB, the polysaccharides isolated from SB protected against LPS-induced IPEC-J2 cell inflammation damage via inhibiting TLR4/NF-κB signaling pathway.16 On the contrary, a natural high-methoxyl homogalacturonan (HRWP-A) isolated and identified from SB activated and enhanced viability of peritoneal macrophages from cyclophosphamide-induced immunosuppressed mice through TLR4/MyD88 pathway.65 Furthermore, the total flavonoids of SB increased cytotoxicity of NK92-MI cells against K562 cells by up-regulating the expressions of perforin and granzymes B.66
3.2 Antioxidant effect
As a famous seaberry, SB was proved to have excellent antioxidant effect in both in vitro and in vivo models, as showed in Table 4. For different part of SB, the strongest antioxidant ability was observed in SB seed extract, followed by root, leaf and stem extract according to the 2,2α-diphenyl-1-picrylhydrazil (DPPH) radical scavenging and the ferric reducing antioxidant power (FRAP) assays. Specifically, the trolox equivalent antioxidant capacity values of the crude ethanolic extract of SB ranged from 175 mg trolox equivalents (TE) per g (leaves) to 529 mg TE per g (seeds) in the DPPH assay, and varied from 137 mg TE per g (stem) to 454 mg TE per g (seeds) in the FRAP assay.54
Table 4 Antioxidant activity of SB on various oxidative stress-related injuries
Part, extract (dose) |
subject |
Pharmaceutical effect |
Ripe fruits, the hexane extract (HRe-1) (1 mL kg−1 day−1, p. o.) |
Rats |
Preventing nicotine-induced oxidative stress in erythrocytes69 |
Leaves, the 70% ethanol extract (100, and 250 mg per kg b. w. p. o.) |
Rats |
Protecting against chromium induced oxidative stress70 |
Fruits, the two aqueous extracts (room temperature and reflux condition), the ethanol extract (250 mg kg−1 p. o.) |
Mice |
Protecting against arsenic-induced oxidative injury, particularly the aqueous extract (at room temperature)71 |
Leaves, the ethanol extract; flavone from fruits (1 g kg−1; 3 doses; p. o.) |
Mice |
Protecting against sulphur mustard-induced oxidative stress72 |
Seeds, the oil (8 mL per mg b. w. p. o.) |
Rats |
Protecting against 2-amino-1-methyl-6-phenylimidazo[4,5-b] pyridine-induced oxidative stress in the rat colons73 |
Leaves and fruits, the 70% ethanol extract (500 μg mL−1) |
Murine macrophages (J-774) |
Inhibiting sodium nitroprusside-induced cytotoxicity74 |
Leaves, the subcritical water extraction (25 μg mL−1) |
Murine macrophages (raw 264.7) |
Inhibiting tertiary-butyl hydroperoxide (tert-BOOH)-induced cytotoxicity, ROS production; restoring antioxidants levels67 |
Leaves and fruits, the 70% ethanol extract (500 μg mL−1) |
Rats lymphocytes |
Inhibiting chromium-induced oxidative damage75 |
Flavones from fruits (500 μg mL−1) |
Rats lymphocytes |
Inhibiting tert-BOOH-induced cytotoxicity and free radical production; restoring the antioxidant levels76 |
Leaves, the aqueous and 70% ethanol extracts (250 μg mL−1) |
BHK-21 cell line |
Protecting against hydrogen peroxide and hypoxanthine-xanthine oxidase induced cell damage56 |
For different subspecies of SB, the SB subsp sinensis was found to possess the strongest extracellular antioxidant activity among those four subspecies (subsp. sinensis, yunnanensis, mongolica and turkestanica) and its oxygen radical absorbance capacity (ORAC) value was 369 ± 24 μmol Trolox equiv. per g dry weight and the peroxyl radical scavenging capacity (PSC) value was 211 ± 24 μmol vitamin C equiv. per g dry weight. Its excellent antioxidant property was believed to mainly correlate to the total phenolic contents in it. Meanwhile, these four subspecies of SB also exhibited cellular antioxidant activity with the intracellular antioxidant activity (CAA) values ranging from 186 ± 16 μmol quercetin equiv. per 100 g dry weight (subsp. sinensis) to 211 ± 16 μmol quercetin equiv. per 100 g dry weight (subsp. yunnanensis), which were also mainly associated to the phenolic acids and flavonoid aglycones in them.14
For different growth periods, the antioxidant activity of SB fruits was found to be higher in late and middle late ripening cultivars than other periods, which may mainly correlate to content of vitamin C, whereas the antioxidant activity of SB leaves seem not to be affected by ripening time.32
For different extraction solvent, the methanol extract of SB seeds showed the strongest antioxidant activity among chloroform, ethyl acetate and acetone extracts, which was evaluated by using DPPH radical scavenging and liposome model system as well as reducing power assay. The subcritical water extraction of SB leaves exhibited higher antioxidant activity than soxhlet and maceration extractions based on the DPPH, FRAP and reducing power assays.67 All of the 100% methanolic, 70% aqua-methanolic and 100% aqueous extracts of SB pomace without seeds showed free radical scavenging activities in vitro radicals, and among those three different extracts, the IC50 value of the 70% aqua-methanolic extract was the lowest for scavenging ABTS (2.072 mg mL−1), DPPH (143.33 μg mL−1), superoxide (240.45 μg mL−1) and nitric oxide radicals (226.84 μg mL−1); whereas the IC50 value of the 100% methanolic extract (29.77 μg mL−1) was the lowest for scavenging of hydroxyl radical.68 For active fraction and constituents, phenolic rich fraction, obtained from ethyl acetate fraction of SB leaves, exhibited better antioxidant effect with DPPH radical scavenging activity of 79.56% (0.2 mg mL−1) than that of the crude extract of SB leaves with DPPH radical scavenging activity of 47.25% (0.2 mg mL−1).53
Obviously, the antioxidant activity of SB extract was fundamentally related to antioxidants (phenolic compounds, vitamin C, etc) contained in it. SB extract and isolated active component were found to protect against various oxidative stress-related injuries, and thus possessed the activities contributed to the improvement of various diseases. Furthermore, mechanisms of antioxidant activity of SB extract and active component were mainly related to modulate levels of various antioxidant enzymes and reduced contents of MDA and ROS, as shown in Table 4 and Fig. 6.
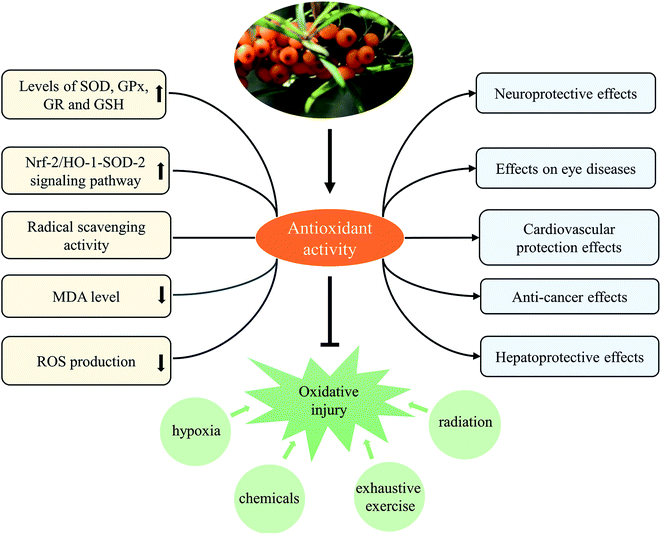 |
| Fig. 6 SB showed antioxidant activity against various oxidative damage caused by various inducers. | |
In fact, we found anti-inflammatory and antioxidant activities were two principal pharmacological effects of SB as Fig. 7 showed; most of biological activities of SB involved the regulation of inflammatory cytokines and antioxidant enzymes. Therefore, further studies should focus on the contribution of each active ingredient of SB to antioxidant activity and their mutual influence. Although some studies in animal models or cells showed changes of SB on biochemical parameters such as antioxidant enzymes, there is a lack of research on relevant antioxidant pathways and the specific targets, so it should be studied further. In addition, the antioxidant activity of SB pomace, root and stem extracts were absent and need further investigation.
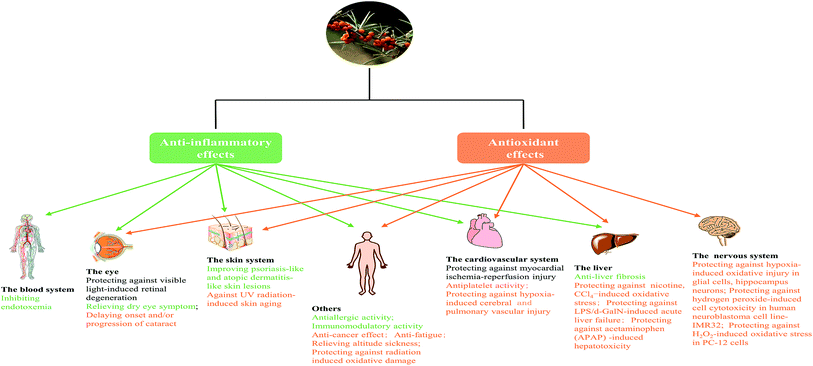 |
| Fig. 7 SB showed protection effect against various damage of body system and diseases, which based on antioxidant and anti-inflammatory activities. The green font indicated the biological activity of SB was due to its anti-inflammatory activity; and the yellow font was due to its antioxidant activity; whereas the black font was due to both the antioxidant and anti-inflammatory activities. | |
3.3 Hepatoprotective effect
The crude extract, polysaccharide and bioactive compounds of SB exhibited protective effect against liver damage induced by drugs or chemicals (ESI Tab. 2†). SB seed oil and phenolic-rich fraction of SB leaves were found to protect animals against CCl4-induced hepatic damage, and SB extract was found to alleviate nicotine-induced oxidative stress in rat liver.77–79 The polysaccharide extract isolated from SB fruits protected mice against acetaminophen induced hepatotoxicity and LPS/D-GalN-induced acute liver failure, and the former function was mainly via activating the Nrf-2/HO-1-SOD-2 signaling pathway, whereas the latter was through suppressing TLR4-NF-κB signaling.15,51 In vitro study, compounds narcissin, isorhamnetin 3,5,7,4-tetrahydroxy-3-methoxyflavon and protocatechuic acid obtained from SB fruits exhibited strong inhibitions on the activation of HSCs, with IC50 values of 46.03, 57.18 and 58.28 μM, respectively; and these active components were also found to attenuate bile duct ligation-induced-liver fibrosis in rat, which was related to modulate DNA damage signaling pathways.80
All of the data implied that SB possessed the protective effect against liver oxidative damage and liver fibrosis. Further studies should pay attention to the active compounds and molecular mechanisms of SB in the treatment of liver oxidative damage. In addition, the extract and active components of SB leaves and seeds should be also considered in anti-hepatic fibrosis studies.
3.4 Cardiovascular protective effect
3.4.1 Anti-hypertensive activity and serum lipids regulation activity. As shown in ESI Tab. 2,† total flavones from SB seed (150 mg kg−1 day−1) could inhibit high-sucrose diet induced hypertension, hyperinsulinemia and dyslipidemia in rats by regulating insulin sensitivity and angiotensin II signaling pathways,81 and the total flavones were also found to possess hypolipidaemic and hypoglycaemic properties in mice fed a high-fat diet, but the precise mechanisms were not elucidated.82 In addition, SB extraction delayed postprandial lipemia in healthy normal-weight male volunteers, which was mainly due to the fiber components of SB.83
3.4.2 Effect on myocardial disease. SB pulp oil was found to protect rats against myocardial ischemia-reperfusion injury with the dose of 20 mL kg−1, which was related to activation of Akt/eNOS signaling pathway.84 In addition, pretreatment with SB oil (20 mL kg−1 day−1) showed the protective effect against isoproterenol-induced myocardial damage in rats.85 Fundamentally, in both studies, the cardioprotective effect of SB were associated with its antioxidant and anti-inflammatory activities.
3.4.3 Effect on platelets. Total flavones of SB (3.0 μg mL−1) showed significant inhibition effect on thrombogenesis in vivo and platelet aggregation induced by collagen in vitro, but not on platelet aggregation induced by arachidonic acid and adenosine diphosphate.11 The phenolic fraction from SB fruits showed inhibitory effect on platelet adhesion but not on platelet aggregation stimulated by adenosine diphosphate (10 μM).86 In addition, the phenolic fraction was also found to reduce the oxidative stress in platelets, and it could modulate blood platelet activation by interfering with the metabolism of arachidonic acid.87 SB extract/component was proved to affect platelet adhesion, aggregation and oxidative stress in platelets, but for why did the total flavones of SB respond differently to platelet aggregation induced by different agonists, further confirmation and clarification of the cause were required. Therefore, more molecular mechanisms and signaling pathways should be evaluated in terms of effect of SB extract/component on platelets.
3.4.4 Effect on coronary heart disease. On the one hand, both in vivo and in vitro experiments implied that the flavone of Hippophae could inhibit macrophage foaming, inflammation and vascular plaque formation by up-regulating the expression of CTRP6, thus may be used to reduce atherosclerosis risk.88 On the other hand, SB seed oil decreased low density lipoprotein (LDL)-cholesterol levels and increased high density lipoprotein (HDL)-cholesterol levels in normal as well as cholesterol-fed rabbits, and showed anti-atherogenic activity, which may be related to the exist of poly-unsaturated fatty acids, phytosterols and β-carotene in SB seed oil.10
3.5 Effect on diabetes and glycometabolism
SB was found to possess α-glucosidase inhibitory effect (ESI Tab. 2†). The methanol extract and n-butanol fraction (5 μg mL−1) of SB leaves rendered the α-glucosidase inhibitory rate of 76% and 86%, respectively,89 whereas the 70% ethanol elution fraction of SB leaves showed α-glucosidase suppression with IC50 value of 0.62 μg mL−1.90 The aqueous extract of SB seed possessed hypoglycemic, hypotriglyceridemic and antioxidant properties in streptozotocin-induced diabetic rats, and the total flavonoids isolated from SB were also found to decrease serum lipids and glucose in mice fed a high-fat diet, thus both of them were considered to be effective treatments for diabetic complications associated with hyperlipidemia and oxidative stresses.91 SB fruits were considered to regulate postprandial insulin response and stabilize postprandial blood glucose in normal-weight male volunteers consumed high-glucose meal, and the 70% ethanol soluble fraction was proved as the major active fraction.92
Further investigation should be focused on hypoglycemic and hypolipidemic mechanisms of the aqueous extract and total flavonoids of SB seed.
3.6 Anti-cancer effect
Both in vivo and in vitro experiments were performed to evaluate anti-cancer effect of SB, which was descripted in ESI Tab. 2.† Concerning the in vivo studies, the hydroalcoholic extract of SB fruit pulp was found to inhibit benzo(a)pyrene-induced forestomach and DMBA-induced skin papillomagenesis in mice.93 Topical administration of 70% ethanol extract of SB branches could suppress skin papilloma which was promoted by TPA following initiated by DMBA in mice.94 A water-soluble homogenous polysaccharide from SB fruits showed inhibitory effect on the Lewis lung carcinoma in mice.95
Concerning the in vitro researches, the ethanol extract of SB leaves showed anti-proliferative effect on different substrains of human acute myeloid leukemia cell lines, with IC50 values of 62.7 μg mL−1 for HL60G, 65 μg mL−1 for HL60MF, 82.0 μg mL−1 for KG-1a, and 100 μg mL−1 for U937, respectively.96 It was found that the colon digestion samples of SB fruits exhibited potent anti-proliferation effect on human cancer cell lines HepG2, MCF-7, MDA-MB-231 and Caco-2 with the EC50 values were 1.66, 2.05, 4.12 and 9.22 mg mL−1, respectively.97 Meanwhile, three flavonoids, including quercetin, kaempferol and isorhamnetin isolated from this colon digestion samples, also showed anti-proliferation effect on HepG2 cell lines with the EC50 values of 80.0, 57.3 and 29.0 μM, respectively; and besides HepG2 cells, quercetin, kaempferol and isorhamnetin also inhibited growth and induced apoptosis in human promyelotic leukemia HL-60 cells. It was implied that the anti-proliferative activity was fairly correlated to phenolic acids and flavonoid aglycones.14 Interestingly, pentamethyl quercetin, syringetin and isorhamnetin with methoxy groups showed stronger inhibitory effect on the HL-60 cells than the above 3 flavonols but without induction of apoptosis.98
So far, mechanisms of anti-cancer effect of SB extract and active component were found to relate to the induction of apoptosis, antioxidant and immune-stimulating activities. In further studies, more molecular mechanisms about anti-cancer effect of SB should be investigated. In addition, the synergistic anti-proliferative effect of individual flavonoid aglycones should be investigated and confirmed.
3.7 The neuroprotective effect
The neuroprotective effect of SB as well as the possible mechanisms were listed in ESI Tab. 2.† The neuroprotective effect of various extract of SB were estimated. SB juice protected mice against lead-induced memory impairment and neuronal damage. And the 75% ethanol extract of SB leaves exhibited protective effect against scopolamine induced cognitive impairment, and the mechanisms may associate with regulating AChE activity and MDA level in the brain.99,100 The aqueous extract of SB fruits protected rats against haloperidol-induced orofacial dyskinesia and dopamine; and homovanillic acid level in striatum of the rats was significantly altered when given the extract before injection of haloperidol.101 The alcoholic extract of SB leaves on the concentration of 200 μg mL−1 could protect glial cells against hypoxia induced oxidative injury;102 the aqueous extract (25, 50 and 100 μg mL−1) inhibited hypoxia induced oxidative stress in hippocampus neurons, and the hydroalcoholic extract (100 μg mL−1) suppressed hydrogen peroxide (H2O2) induced cell cytotoxicity in human neural cell line IMR32, while the ethyl acetate fraction (5, 10, and 20 μg mL−1) reduced H2O2-induced oxidative stress in neuronal PC-12 cells. The protective effect in the last three were reported in a dose dependent manner.13,103,104
3.8 The radioprotection effect
The 50% alcoholic extract of SB whole fruits (RH-3) possessed radio-protective effect, with a maximum tolerated dose of 40 mg kg−1 in mice. Pre-irradiation administration of single dose of RH-3 (30 mg kg−1) rendered 82% survival of mice as compared to no survival in irradiated control group.105 Further studies showed this RH-3 administration before irradiation protected mice against radiation induced various damages, as Table 5 showed. In addition, the aqueous extract of SB leaves (SBL-1) also showed the radio-protective action, with a maximum tolerated dose of 120 mg kg−1 and a median lethal dose (LD50) of 140 mg kg−1 in mice. SBL-1 (30 mg kg−1 single dose) countered 100% mortality in whole-body irradiated controls and rendered >90% survivors when administered 30 min before irradiation in mice.106 Further investigations showed pre-irradiation treatment with SBL-1 countered radiation induced various damages as Table 5 showed. Ethyl 3,4,5-trihydroxybenzoate (GAE), a major bioactive constituent of SBL-1, was also evaluated about the radiomodifying action. GAE possessed anti-inflammatory action and inhibited NF-κB expression; and according to biodistribution studies, GAE could penetrate the blood–brain barrier, reached plasma peak at about 15 minutes, and the concentrations found in liver and kidney were higher than those found in other organs.12
Table 5 The radioprotective effect of SB fruits and leaves extract
Preparation |
Administration |
Subject |
Against radiation induced damage |
Whole fruits, the 50% ethanol extract (RH-3) |
30 mg per kg b. w. i. p. |
Mice |
Against 10 Gy whole body lethal irradiation105 |
30 to 40 mg per kg b. w. i. p. |
Mice |
Against micronuclei in mouse bone marrow107 |
100 μg mL−1 |
Thymocyte |
Against strand breaks in the thymocyte DNA in mice108 |
Mice |
Against loss of cellularity of crypts in the jejunum and villi109 |
30 mg per kg b. w. i. p. |
Mice |
Against oxidative damage in mitochondrial system110 |
30 mg per kg b. w. i. p. |
Mice |
Against response of peritoneal macrophages and splenocytes111 |
30 mg per kg b. w. i. p. |
Mice |
Against spermatogenesis damage in mice112 |
Leaves, the aqueous extract (SBL-1) |
30 mg per kg b. w. i. p. |
Mice |
Against 10 Gy whole body lethal irradiation106 |
30 mg per kg b. w. i. p. |
Mice |
Against jejunal microbiota dysbiosis113 |
30 mg per kg b. w. i. p |
Mice |
Against kidney injuries in mice114 |
30 mg per kg b. w. i. p |
Mice |
Against changes in mouse spleen cell populations115 |
30 mg per kg b. w. i. p |
Mice |
Against decreases in jejunum crypts and villi number; against bone marrow apoptosis and micronuclei frequency116 |
12 mg per kg b. w. i. p. |
Rats |
Against conditioned taste aversion, brain injuries and disturbances in neurotransmitters117 |
GAE |
200 mg per kg b. w. p. o. |
Mice |
Radiomodifying and inflammatory action; inhibiting NF-κB expression12 |
According to these mentioned studies, the radioprotective effect of SB preparation may be associated with free radical scavenging, acceleration of stem cell proliferation and immunostimulatory activity. Both the fruits and leaves of SB showed the protective effects against gamma radiation induced damage in animal models; perhaps a compared study is needed for the radioprotective effect of RH-3 and SBL-1.
3.9 Effect on the skin
The beneficial effect of SB on skin lesions was listed in ESI Tab. 2.† The hydroalcoholic extract of SB seed was found to possess anti-melanogenesis properties on B16F10 mouse melanoma cells, thus its skin whitening potential was considered.118 The emulsion formulations containing plant extracts (SB and Cassia fistula) significantly decreased skin sebum content of patients with mild-to-moderate acne vulgaris by topical use,119 but the role of SB was not studied separately. SB fruit blend, administered orally, was found to prevent UV-induced skin aging in hairless mice and SB seed oil was probed to suppress UV-induced disturbances changes in lipid metabolism of human skin cells,120,121 which implied the radioprotection effect of SB, thus could be used as a sunscreen additive. SB seed oil, topically applied, showed wound healing activity on ovine burn wound model;122 and similarly, a polyvinyl alcohol-blended pectin hydrogel containing the extract of SB leaves also exhibited potential wound healing effect on rat acute wound model.123 However, the underlying mechanism was not mentioned. In addition, SB oil improved DNCB-induced AD-like lesions in mice by topical application,42 but the oral effect was not investigated. Furthermore, the antipsoriasis-like effect of SB oil was estimated with both in vitro (human monocytic cells) and in vivo oral/topical treatment (TPA-induced CD-1 mice psoriasis-like model).43 So far, the skin benefits of SB were mainly reflected in skin care as well as the improvements of some skin lesions.
3.10 Effect on eye disease
A clinical trial (ESI Tab. 2†) revealed oral SB oil (including seed and pulp oil) alleviated tear film osmolarity and dry eye symptoms in individuals with dry eye disease.124 Further studies explored that SB pulp oil (not seed oil) and its major fatty acids palmitoleate, orally administrated, restored tear secretion in a murine dry eye model and suppressed inflammatory cytokines in the lacrimal gland.125 Both proanthocyanidins from SB seeds126 and total flavones from SB127 exhibited protective effects against visible light-induced retinal degeneration in pigmented rabbits via antioxidant, anti-inflammatory and anti-apoptotic mechanisms. And SB seed oil could protect rat against retina damage induced by hypertensive retinopathy.128 In addition, the aqueous extract of SB leaves showed certain resistance activity of cataract during in hydrogen peroxide-induced cataract in goat lenses.129 Taken together, SB was found to possess the positive effect on dry eye symptoms, retina damage and cataract. But few studied focused on topical effect, and the beneficial effect of active components of SB on eye sickness was also not measured; and meanwhile, the mechanisms of action of SB on eye disease is also needed further study.
3.11 Effect on gastrointestinal disease
SB hexane extract was found to have a positive effect against gastric injury in ulcer models produced by stress and indomethacin in rats (ESI Tab. 2†).130 Oils from SB seeds and fruits showed the protective effect against gastric ulcer induced by water-immersion stress, reserpine, pylorus-ligation and the acetic acid in rats, but the inhibition rate and mode (dose-dependent or independently from the dose) of SB oil on ulcer was different in different models of ulcer formation.9 Besides rats, SB fruits and pulp formulation also showed positive effect against glandular ulcer in horses housed in stalls and undergoing intermittent feeding.131 In addition, the presence and digestion of polyphenolics in SB juice promoted the proliferation of beneficial gut microbiota.132
In summary, the effect of SB on the gastrointestinal tract was represented primarily in antiulcer and certain probiotic properties, but few studies involved anti-ulcer mechanism and bioactive compound of SB on gastrointestinal disease.
3.12 Other pharmacological effect
Both the water-soluble polysaccharide from SB fruits and the aqueous extract of SB leaves exhibited anti-fatigue activities.133,134 SB extract exhibited the protective effect against hypoxia induced cerebral and pulmonary vascular injury, simulated high-altitude polycythemia and cold-hypoxia-restraint-induced hypothermia in rats, thus was considered to possess therapeutic potential for high altitude disease.135–138 Details of these studies were also listed in ESI Tab. 2.†
4. Toxicological study
As a well-known seaberry consumed worldwide for both of its nutritional and medicinal uses, the safety of SB received much attention. The detailed information of the toxicity studies of SB were listed in Table 6. By employing the acute toxicity test, the oral LD50 of the aqueous extract of SB leaves and fruits in rats were proved more than 10 g per kg body weight, and non-toxic in rats were observed at their respective maximal effective dose administration for 30 days. For SB fruit and seed oil, no adverse effect was found in body and organ weights, blood biochemistry and hematology in oral toxicity study; and seed oil also showed no skin irritation in acute dermal irritation assay. Similarly, no significant treatment related changes were observed in sub-chronic intramuscular injection toxicity studies in rabbits.139–141 In addition, SB fruit oil was proved had no genotoxicity and teratogenicity in both animals and cells.142 The no-observed-adverse-effect level (NOAEL) of the aqueous extract of SB fruits was 100 mg per kg body weight per day in rats. However, changes in liver and kidney weight/body weight ratio and hematocrit value were observed in rats administered 1 g per kg and 2 g per kg body weight the aqueous extract of SB leaves for 14 days. And the change in plasma glucose level was found in female rats administered 250 and 500 mg kg−1 of the aqueous extract of SB fruits for 90 days,143–145 which might be related to the high sugar content of SB. More toxicological studies should be focused on the leaves of SB in future.
Table 6 The toxicity of SB
Preparation |
Subjects |
Toxicological data |
Leaves, the aqueous extract |
Rats |
The maximal effective adaptogenic dose: 100 mg per kg b. w. p. o.; the oral LD50: >10 g per kg b. w.; 1 g per kg and 2 g per kg b. w. p. o. per day for 14 days → a significant increase in red blood cell numbers resulting into increased hematocrit value and a decrease in kidney weight/body weight ratio (2 g kg−1), a significant increase in liver weight/body weight ratio (1 g kg−1)143 |
Fruits, the aqueous extract |
Rats |
The maximal effective dose: 75 mg per kg b. w. p. o.; the oral LD50: >10.0 g per kg b. w.; sub-acute toxicity and sub-chronic studies: no significant changes144 |
Fruits, the aqueous extract |
Rats |
The NOAEL: 100 mg per kg b. w. per day p. o.; 250 and 500 mg kg−1 p. o. for 90 days → a significant increase in plasma glucose levels in female rats and restored to normal within 2 weeks of treatment withdrawal145 |
Fruits, the oil |
Mice and rats |
The maximum tolerated dose for mice: >20 mL per kg (i.e. 18.72 g per kg) b. w. p.o.; the NOAEL for rats: 10 mL per kg (i.e. 9.36 g per kg) b. w. p. o.; 10 mL per kg b. w. p. o. for 90 days for rats → slight histopathological changes in livers and kidneys139 |
Fruits, the oil |
Mice and rats |
No mutagenic activity on S. typhimurium with tested concentrations: 8, 40, 200, 1000, and 5000 μg per plate; no significant effect on sperm morphology and micronucleus rate of polychromatic erythrocytes in mice at doses of 9.36, 4.68, and 2.34 g per kg b. w. p. o.; no treatment-related maternal toxicity or embryo toxicity in pregnant rats treated with 4.68, 2.34, and 1.17 g per kg b. w. p. o. SB oil from gestation day 7 to 16142 |
Seeds, the oil |
Rats, albino rabbit |
The maximal effective dose: 2.5 mL per kg b. w. p. o.; the acute (2.5, 5.0, 7.5 and 10.0 mL per kg b. w. p. o.) and sub-acute oral toxicity studies ((I) 2.5 mL per kg and 5.0 mL per kg b. w. p. o., once a day, for 14 days; (II) 2.5 mL per kg b. w. p. o. for 28 days): no adverse effect; acute dermal irritation assay in rabbit: no irritation140 |
Seeds, the oil |
New Zealand white rabbits |
Sub-chronic toxicity studies (0.5, 1 and 1.5 mL per kg b. w. (i. m.) once a week for 7 consecutive weeks): no deaths and no treatment related adverse effect, but tissue hardening and inflammatory reactions at administration sites in all the treated animals141 |
5. Conclusion and suggestion
The present review set out to summarize and disclose the bioactive components as well as the nutritional and health effects of SB. As mentioned in the literature review, the activities of the extracts, fractions and active components in different subspecies, parts of SB were evaluated. The aqueous, methanol/ethanol extracts and oil of SB showed a variety of biological activities, which related to bioactive components exist in these extracts. Flavonoids was one class of the major bioactive components in SB fruits, leaves and seeds, which were found to possess anti-inflammatory, immunomodulatory, antioxidant, cardiovascular protection, anti-cancer and anti-retinal injury activities.14,44,66,88,127 The polysaccharide from SB fruits was another bioactive fraction which was found to possess various biological activities including anti-inflammatory, immunomodulatory, anti-cancer, antioxidant, hepatoprotection, anti-fatigue activities.15,16,95,133 In particular, the oil extracted from SB fruits, pulp and seeds was widely investigated, and a unique mixture of bioactive constituents including fatty acids, tocopherols and tocotrienols, carotenoid and polyphenol were identified in SB;24,27 and correspondingly, the oil exhibited excellent anti-inflammatory, antioxidant, immunomodulatory, anti-gastric ulcers, hepatoprotective, cardiovascular, eye (dry eye and retina damage) and skin protection activities (anti-psoriasis like, skin photo-protection and wound healing activity).9,43,77,84,121,122,124,128,135 Thus, the role of SB oil could not be ignored, but the studies on the role of individual active components and their synergistic effects are lacking, so further researches are needed. Meanwhile, only a few studies have been conducted on the extracts of SB roots and branches, more studies are needed to fully utilize this plant. Furthermore, several possible mechanisms governing the above actions of SB were mentioned including regulation of neurotransmitters (AchE, MAO-A etc.), cell cycle and some signaling pathways (TLR4/NF-κB, Akt/eNOS etc.). However, in view of the complexity and multiplicity of the mechanisms of traditional Chinese medicine, more molecular mechanisms needed to be elucidated to make full use of the active role of SB.
Abbreviation
Akt/eNOS | Protein kinase β-endothelial nitric oxide synthase |
AchE | Acetyl-cholinesterase |
b. w. | Body weight |
CCl4 | Carbon tetrachloride |
CD25 | IL-2 receptor alpha chain |
CTRP6 | Tumor necrosis factor-related proteins 6 |
D-GalN | D-Galactosamine hydrochloride |
DNCB | 2,4-Dinitrochloro-benzene |
DMBA | 7,12-Dimethyl benzanthracene |
DPPH | 2,2α-Diphenyl-1-picrylhydrazil |
FRAP | Ferric reducing antioxidant power |
GR | Glutathione reductase |
GSH | Glutathione |
GPx | Glutathione peroxidase |
GAE | Ethyl 3,4,5-trihydroxybenzoate |
HRP | Polysaccharide extract of H. rhamnoides |
HO-1 | Hemeoxygenase-1 |
HRWP-A | A natural high-methoxyl homogalacturonan from H. rhamnoides fruits |
HRe-1 | Hexane extract of H. rhamnoides fruits |
HSCs | Hepatic stellate cells |
HDL | High density lipoprotein |
i. p. | Intraperitoneally |
i. g. | Intragastrically |
IL | Interleukin |
IFN | Interferon |
IKK | Inhibitor of κB kinase |
IκB | Inhibitor of κB |
IPEC-J2 | Intestinal porcine epithelial cells |
IC50 | Half-maximal inhibitory concentration |
LPS | Lipopolysaccharide |
LD50 | Lethal dose for 50% |
LDL | Low density lipoprotein |
MyD88 | Myeloid differentiation factor 88 |
MAO | Monoamine oxidase |
MIC | Minimum inhibitory concentration |
MDA | Malondialdehyde |
N. D. | Not detected |
NF-κB | Nuclear factor-κB |
NK cells | Natural killer cells |
Nrf-2 | Nuclear factor erythroid-2-related factor 2 |
NOAEL | No-observed-adverse-effect level |
p. o. | Per oral |
PBMCs | Peripheral blood mononuclear cells |
ROS | Reactive oxygen species |
subsp. | Subspecies |
SB | Sea buckthorn |
SOD | Superoxide dismutase |
SCE200 ET | Supercritical carbon dioxide extract of H. rhamnoides leaves by 200 bar pressure |
SCE300ET and 350 ET | Supercritical carbon dioxide extract of H. rhamnoides leaves by 300 bar and 350 bar pressures |
TLR4 | Toll-like receptor 4 |
T. A. | Topical application |
TNF | Tumor necrosis factor |
TPA | 12-O-Tetradecanoylphorbol 13-acetate |
tert-BOOH | Tertiary-butyl hydroperoxide |
TE | Trolox equivalents |
UV | Ultraviolet |
Conflicts of interest
Authors have no conflicts of interest.
Acknowledgements
This work was supported by the National Natural Science Foundation of China (No. 81860753, 81560684), the Key Research and Development Program of Ningxia (2018BHF2001), Ningxia key research and invention program of science and technology cooperation of the East and the West (No. 2018ZDKJ0219, 2017BY084, 2017BY079), the West Light Foundation of the Chinese Academy of Sciences-Young Scientists of West 2017.
References
- T. S. C. Li and T. H. J. Beveridge, Sea Buckthorn (Hippophae rhamnoides L.): Production and Utilization, NRC Research Press, Ottawa, Ontario, 2003 Search PubMed
. - C. Ruan, K. Rumpunen and H. Nybom, Crit. Rev. Biotechnol., 2013, 33, 126–144 Search PubMed
. - S. Lu, J. Lu and X. Wen, Soil and Water Conservation In China, 2019, pp. 45–49 Search PubMed
. - E. C. Pukhalskaia, Bull. Exp. Biol. Med., 1958, 45, 363–366 Search PubMed
. - Y. Wang, Y. Lu, X. Liu, Z. Gou and J. Hu, Zhongguo Zhongyao Zazhi, 1992, 17, 624–626 Search PubMed
. - M. Xiao, Z. Yang, M. Jiu, J. You and R. Xiao, Huaxi Yike Daxue Xuebao, 1992, 23, 98–101 Search PubMed
. - É. N. Novruzov, S. M. Aslanov, A. A. Imanova and Z. I. Gasanova, Chem. Nat. Compd., 1980, 15, 543 Search PubMed
. - D. B. Rasputina, N. F. Komissarenko and D. T. Tsybikova, Chem. Nat. Compd., 1977, 12, 87 Search PubMed
. - J. Xing, B. Yang, Y. Dong, B. Wang, J. Wang and H. P. Kallio, Fitoterapia, 2002, 73, 644–650 Search PubMed
. - M. Basu, R. Prasad, P. Jayamurthy, K. Pal, C. Arumughan and R. C. Sawhney, Phytomedicine, 2007, 14, 770–777 Search PubMed
. - J. Cheng, K. Kondo, Y. Suzuki, Y. Ikeda, X. Meng and K. Umemura, Life Sci., 2003, 72, 2263–2271 Search PubMed
. - P. K. Pandey, B. Ahmed, J. Prasad, M. Bala and H. A. Khan, Sci. Rep., 2019, 9, 18873 Search PubMed
. - C. H. Cho, H. Jang, M. Lee, H. Kang, H. J. Heo and D. O. Kim, J. Microbiol. Biotechnol., 2017, 27, 1257–1265 Search PubMed
. - R. X. Guo, X. Guo, T. Li, X. Fu and R. H. Liu, Food Chem., 2017, 221, 997–1003 Search PubMed
. - X. Wang, J. Liu, X. Zhang, S. Zhao, K. Zou, J. Xie, X. Wang, C. Liu, J. Wang and Y. Wang, Phytomedicine, 2018, 38, 90–97 Search PubMed
. - L. Zhao, M. Li, K. Sun, S. Su, T. Geng and H. Sun, Int. J. Biol. Macromol., 2020, 155, 1202–1215 Search PubMed
. - A. Vilas-Franquesa, J. Saldo and B. Juan, Food Production, Processing and Nutrition, 2020, 2, 1–17 Search PubMed
. - T. A. Wani, S. M. Wani, M. Ahmad, M. Ahmad, A. Gani and F. A. Masoodi, Cogent Food Agric., 2016, 2, 1–9 Search PubMed
. - K. Tkacz, A. Wojdylo, I. P. Turkiewicz, F. Ferreres, D. A. Moreno and P. Nowicka, Food Chem., 2020, 309, 125766 Search PubMed
. - X. Ma, O. Laaksonen, J. Zheng, W. Yang, M. Trepanier, H. Kallio and B. Yang, Food Chem., 2016, 200, 189–198 Search PubMed
. - M. Heinaaho, A. E. Hagerman and R. Julkunen-Tiitto, J. Agric. Food Chem., 2009, 57, 1940–1947 Search PubMed
. - W. Yang, O. Laaksonen, H. Kallio and B. Yang, J. Agric. Food Chem., 2016, 64, 1274–1282 Search PubMed
. - W. Yang, O. Laaksonen, H. Kallio and B. Yang, Food Chem., 2017, 216, 87–96 Search PubMed
. - H. Kallio, B. Yang, P. Peippo, R. Tahvonen and R. Pan, J. Agric. Food Chem., 2002, 50, 3004–3009 Search PubMed
. - F. V. Dulf, Chem. Cent. J., 2012, 6, 106 Search PubMed
. - B. Yang and H. P. Kallio, J. Agric. Food Chem., 2001, 49, 1939–1947 Search PubMed
. - L. Shi, L. Zheng, C. Zhao, Q. Jin and X. Wang, Nat. Prod. Res., 2019, 33, 3596–3600 Search PubMed
. - C. Tudor, T. Bohn, M. Iddir, F. V. Dulf, M. Focsan, D. O. Rugina and A. Pintea, Nutrients, 2019, 12, 76 Search PubMed
. - R. M. Pop, Y. Weesepoel, C. Socaciu, A. Pintea, J. P. Vincken and H. Gruppen, Food Chem., 2014, 147, 1–9 Search PubMed
. - S. C. Andersson, M. E. Olsson, E. Johansson and K. Rumpunen, J. Agric. Food Chem., 2009, 57, 250–258 Search PubMed
. - T. S. C. Li, T. H. J. Beveridge and J. C. G. Drover, Food Chem., 2007, 101, 1633–1639 Search PubMed
. - I. Sytarova, J. Orsavova, L. Snopek, J. Mlcek, L. Byczynski and L. Misurcova, Food Chem., 2019, 310, 125784 Search PubMed
. - H. Kallio, B. Yang and P. Peippo, J. Agric. Food Chem., 2002, 50, 6136–6142 Search PubMed
. - S. C. Andersson, K. Rumpunen, E. Johansson and M. E. Olsson, J. Agric. Food Chem., 2008, 56, 6701–6706 Search PubMed
. - H. Kallio, M. Lassila, E. Jarvenpaa, G. G. Haraldsson, S. Jonsdottir and B. Yang, J. Chromatogr. B: Anal. Technol. Biomed. Life Sci., 2009, 877, 1426–1432 Search PubMed
. - D. Gutzeit, B. Klaubert, M. Rychlik, P. Winterhalter and G. Jerz, J. Agric. Food Chem., 2007, 55, 3978–3984 Search PubMed
. - D. Gutzeit, G. Baleanu, P. Winterhalter and G. Jerz, J. Food Sci., 2007, 72, C491–C497 Search PubMed
. - S. M. Sabir, H. Maqsood, I. Hayat, M. Q. Khan and A. Khaliq, J. Med. Food, 2005, 8, 518–522 Search PubMed
. - K. Saeidi, A. Alirezalu and Z. Akbari, Nat. Prod. Res., 2016, 30, 366–368 Search PubMed
. - I. Smida, C. Pentelescu, O. Pentelescu, A. Sweidan, N. Oliviero, V. Meuric, B. Martin, L. Colceriu, M. Bonnaure-Mallet and Z. Tamanai-Shacoori, J. Appl. Microbiol., 2019, 126, 1594–1605 Search PubMed
. - E. Erhan, S. Terzi, M. Celiker, O. Yarali, M. Cankaya, F. K. Cimen, I. Malkoc and B. Suleyman, Clin. Exp. Otorhinolaryngol., 2017, 10, 181–187 Search PubMed
. - D. D. Hou, Z. H. Di, R. Q. Qi, H. X. Wang, S. Zheng, Y. X. Hong, H. Guo, H. D. Chen and X. H. Gao, Skin Pharmacol. Physiol., 2017, 30, 268–276 Search PubMed
. - A. Balkrishna, S. S. Sakat, K. Joshi, K. Joshi, V. Sharma, R. Ranjan, K. Bhattacharya and A. Varshney, Front. Pharmacol., 2019, 10, 1186 Search PubMed
. - B. Jayashankar, K. P. Mishra, L. Ganju and S. B. Singh, Int. Immunopharmacol., 2014, 20, 89–94 Search PubMed
. - D. Redei, N. Kusz, N. Jedlinszki, G. Blazso, I. Zupko and J. Hohmann, Planta Med., 2018, 84, 26–33 Search PubMed
. - F. Qi, J. H. Sun, J. Q. Yan, C. M. Li and X. C. Lv, Microb. Pathog., 2018, 120, 37–41 Search PubMed
. - S. Y. Kim, C. Y. Jin, C. H. Kim, Y. H. Yoo, S. H. Choi, G. Y. Kim, H. M. Yoon, H. T. Park and Y. H. Choi, Int. J. Mol. Med., 2019, 43, 682–692 Search PubMed
. - A. K. Chauhan, J. Kim, Y. Lee, P. K. Balasubramanian and Y. Kim, Molecules, 2019, 24, 3984 Search PubMed
. - J. Li, R. Wu, X. Qin, D. Liu, F. Lin and Q. Feng, Mol. Med. Rep., 2017, 16, 4253–4258 Search PubMed
. - D. J. Kwon, Y. S. Bae, S. M. Ju, A. R. Goh, G. S. Youn, S. Y. Choi and J. Park, Biochem. Biophys. Res. Commun., 2012, 417, 1254–1259 Search PubMed
. - H. Liu, W. Zhang, S. Dong, L. Song, S. Zhao, C. Wu, X. Wang, F. Liu, J. Xie, J. Wang and Y. Wang, J. Ethnopharmacol., 2015, 176, 69–78 Search PubMed
. - P. S. Negi, A. S. Chauhan, G. A. Sadia, Y. S. Rohinishree and R. S. Ramteke, Food Chem., 2005, 92, 119–124 Search PubMed
. - K. M. Yogendra, R. J. Tirpude, D. T. Maheshwari, A. Bansal and K. Misra, Food Chem., 2013, 141, 3443–3450 Search PubMed
. - T. Michel, E. Destandau, G. Le Floch, M. E. Lucchesi and C. Elfakir, Food Chem., 2012, 131, 754–760 Search PubMed
. - Y. Li, C. Xu, Q. Zhang, J. Y. Liu and R. X. Tan, J. Ethnopharmacol., 2005, 98, 329–333 Search PubMed
. - N. K. Upadhyay, M. S. Yogendra Kumar and A. Gupta, Food Chem. Toxicol., 2010, 48, 3443–3448 Search PubMed
. - X. F. Yue, X. Shang, Z. J. Zhang and Y. N. Zhang, J. Food Drug Anal., 2017, 25, 327–332 Search PubMed
. - S. H. Abidi, K. Ahmed, S. K. Sherwani and S. U. Kazmi, J. Infect. Dev. Countries, 2015, 9, 925–929 Search PubMed
. - B. Sadowska, A. Budzynska, A. Stochmal, J. Zuchowski and B. Rozalska, Microb. Pathog., 2017, 107, 372–379 Search PubMed
. - A. S. Chauhan, P. S. Negi and R. S. Ramteke, Fitoterapia, 2007, 78, 590–592 Search PubMed
. - M. I. Qadir, K. Abbas, A. Younus and R. S. Shaikh, Pak. J. Pharm. Sci., 2016, 29, 1711–1713 Search PubMed
. - K. P. Mishra, R. Mishra, A. P. Yadav, B. Jayashankar, S. Chanda and L. Ganju, Biomed. Aging Pathol., 2011, 1, 61–64 Search PubMed
. - B. Jayashankar, D. Singh, H. Tanwar, K. P. Mishra, S. Murthy, S. Chanda, J. Mishra, R. Tulswani, K. Misra, S. B. Singh and L. Ganju, Int. Immunopharmacol., 2017, 44, 123–136 Search PubMed
. - H. Diandong, G. Feng, L. Zaifu, T. Helland, F. Weixin and C. Liping, Int. J. Immunopathol. Pharmacol., 2016, 29, 76–83 Search PubMed
. - H. Wang, H. Bi, T. Gao, B. Zhao, W. Ni and J. Liu, Int. J. Biol. Macromol., 2018, 107, 1039–1045 Search PubMed
. - D. Hou, D. Wang, X. Ma, W. Chen, S. Guo and H. Guan, Int. J. Immunopathol. Pharmacol., 2017, 30, 353–361 Search PubMed
. - M. S. Kumar, R. Dutta, D. Prasad and K. Misra, Food Chem., 2011, 127, 1309–1316 Search PubMed
. - C. Varshneya, V. Kant and M. Mehta, Int. J. Food Sci. Nutr., 2012, 63, 153–159 Search PubMed
. - H. Suleyman, K. Gumustekin, S. Taysi, S. Keles, N. Oztasan, O. Aktas, K. Altinkaynak, H. Timur, F. Akcay, S. Akar, S. Dane and M. Gul, Biol. Pharm. Bull., 2002, 25, 1133–1136 Search PubMed
. - S. Geetha, R. M. Sai, S. S. Mongia, V. Singh, G. Ilavazhagan and R. C. Sawhney, J. Ethnopharmacol., 2003, 87, 247–251 Search PubMed
. - R. Gupta and S. J. Flora, Hum. Exp. Toxicol., 2006, 25, 285–295 Search PubMed
. - R. Vijayaraghavan, A. Gautam, O. Kumar, S. C. Pant, M. Sharma, S. Singh, H. T. Kumar, A. K. Singh, M. Nivsarkar, M. P. Kaushik, R. C. Sawhney, O. P. Chaurasia and G. B. Prasad, Indian J. Exp. Biol., 2006, 44, 821–831 Search PubMed
. - R. J. Li, J. J. Tian, W. Q. Li, F. Q. Cheng and G. S. Gao, J. Environ. Sci. Health, Part B, 2014, 49, 279–289 Search PubMed
. - S. Geetha, M. S. Ram, V. Singh, G. Ilavazhagan and R. C. Sawhney, Biomed. Pharmacother., 2002, 56, 463–467 Search PubMed
. - S. Geetha, M. S. Ram, V. Singh, G. Ilavazhagan and R. C. Sawhney, J. Ethnopharmacol., 2002, 79, 373–378 Search PubMed
. - S. Geetha, M. S. Ram, S. K. Sharma, G. Ilavazhagan, P. K. Banerjee and R. C. Sawhney, J. Med. Food, 2009, 12, 151–158 Search PubMed
. - Y. Hsu, C. Tsai, W. Chen and F. Lu, Food Chem. Toxicol., 2009, 47, 2281–2288 Search PubMed
. - D. T. Maheshwari, K. M. Yogendra, S. K. Verma, V. K. Singh and S. N. Singh, Food Chem. Toxicol., 2011, 49, 2422–2428 Search PubMed
. - S. Taysi, K. Gumustekin, B. Demircan, O. Aktas, N. Oztasan, F. Akcay, H. Suleyman, S. Akar, S. Dane and M. Gul, Pharm. Biol., 2010, 48, 488–493 Search PubMed
. - G. Zhang, Y. Liu and P. Liu, J. Agric. Food Chem., 2018, 66, 12257–12264 Search PubMed
. - X. Pang, J. Zhao, W. Zhang, X. Zhuang, J. Wang, R. Xu, Z. Xu and W. Qu, J. Ethnopharmacol., 2008, 117, 325–331 Search PubMed
. - J. Wang, W. Zhang, D. Zhu, X. Zhu, X. Pang and W. Qu, J. Sci. Food Agr., 2011, 91, 1446–1451 Search PubMed
. - K. M. Linderborg, H. M. Lehtonen, R. Jarvinen, M. Viitanen and H. Kallio, Int. J. Food Sci. Nutr., 2012, 63, 483–490 Search PubMed
. - K. Suchal, J. Bhatia, S. Malik, R. K. Malhotra, N. Gamad, S. Goyal, T. C. Nag, D. S. Arya and S. Ojha, Front. Pharmacol., 2016, 7, 155 Search PubMed
. - S. Malik, S. Goyal, S. K. Ojha, S. Bharti, S. Nepali, S. Kumari, V. Singh and D. S. Arya, Int. J. Toxicol., 2011, 30, 671–680 Search PubMed
. - B. Olas, B. Kontek, M. Szczesna, L. Grabarczyk, A. Stochmal and J. Zuchowski, J. Physiol. Pharmacol., 2017, 68, 223–229 Search PubMed
. - B. Olas, B. Kontek, P. Malinowska, J. Zuchowski and A. Stochmal, Oxid. Med. Cell. Longev., 2016, 2016, 4692486 Search PubMed
. - X. Zhuo, Y. Tian, Y. Wei, Y. Deng, Y. Wu and T. Chen, Biosci., Biotechnol., Biochem., 2019, 83, 2000–2007 Search PubMed
. - J. S. Kim, Y. S. Kwon, Y. J. Sa and M. J. Kim, J. Agric. Food Chem., 2011, 59, 138–144 Search PubMed
. - R. Li, Q. Wang, M. Zhao, P. Yang, X. Hu and D. Ouyang, Fitoterapia, 2019, 137, 104248 Search PubMed
. - W. Zhang, J. Zhao, J. Wang, X. Pang, X. Zhuang, X. Zhu and W. Qu, Phytother. Res., 2010, 24, 228–232 Search PubMed
. - H. M. Lehtonen, R. Jarvinen, K. Linderborg, M. Viitanen, M. Venojarvi, H. Alanko and H. Kallio, Eur. J. Clin. Nutr., 2010, 64, 1465–1471 Search PubMed
. - B. Padmavathi, M. Upreti, V. Singh, A. R. Rao, R. P. Singh and P. C. Rath, Nutr. Cancer, 2005, 51, 59–67 Search PubMed
. - K. Yasukawa, S. Kitanaka, K. Kawata and K. Goto, Fitoterapia, 2009, 80, 164–167 Search PubMed
. - H. Wang, T. Gao, Y. Du, H. Yang, L. Wei, H. Bi and W. Ni, Carbohydr. Polym., 2015, 131, 288–296 Search PubMed
. - G. T. Zhamanbaeva, M. K. Murzakhmetova, S. T. Tuleukhanov and M. P. Danilenko, Bull. Exp. Biol. Med., 2014, 158, 252–255 Search PubMed
. - R. Guo, X. Chang, X. Guo, C. S. Brennan, T. Li, X. Fu and R. H. Liu, Food Funct., 2017, 8, 4229–4240 Search PubMed
. - H. Hibasami, A. Mitani, H. Katsuzaki, K. Imai, K. Yoshioka and T. Komiya, Int. J. Mol. Med., 2005, 15, 805–809 Search PubMed
. - Y. Xu, G. Li, C. Han, L. Sun, R. Zhao and S. Cui, Biol. Pharm. Bull., 2005, 28, 490–494 Search PubMed
. - D. P. Attrey, A. K. Singh, T. Naved and B. Roy, Indian J. Exp. Biol., 2012, 50, 690–695 Search PubMed
. - F. Batool, A. H. Shah, S. D. Ahmed, Z. S. Saify and D. J. Haleem, Med. Sci. Monit., 2010, 16, R285–R292 Search PubMed
. - S. Narayanan, D. Ruma, B. Gitika, S. K. Sharma, T. Pauline, M. S. Ram, G. Ilavazhagan, R. C. Sawhney, D. Kumar and P. K. Banerjee, Mol. Cell. Biochem., 2005, 278, 9–14 Search PubMed
. - M. Manickam and R. Tulsawani, PLoS One, 2014, 9, e87694 Search PubMed
. - S. Shivapriya, K. Ilango and G. P. Dubey, Saudi J. Biol. Sci., 2015, 22, 645–650 Search PubMed
. - H. C. Goel, J. Prasad, S. Singh, R. K. Sagar, I. P. Kumar and A. K. Sinha, Phytomedicine, 2002, 9, 15–25 Search PubMed
. - M. Bala, J. Prasad, S. Singh, S. Tiwari and R. C. Sawhney, J. Herbs, Spices, Med. Plants, 2009, 15, 203–215 Search PubMed
. - P. K. Agrawala and H. C. Goel, Indian J. Exp. Biol., 2002, 40, 525–530 Search PubMed
. - I. P. Kumar, S. Namita and H. C. Goel, Mol. Cell. Biochem., 2002, 238, 1–9 Search PubMed
. - H. C. Goel, C. A. Salin and H. Prakash, Phytother. Res., 2003, 17, 222–226 Search PubMed
. - H. C. Goel, D. Gupta, S. Gupta, A. P. Garg and M. Bala, J. Pharm. Pharmacol., 2005, 57, 135–143 Search PubMed
. - H. Prakash, M. Bala, A. Ali and H. C. Goel, J. Pharm. Pharmacol., 2005, 57, 1065–1072 Search PubMed
. - H. C. Goel, N. Samanta, K. Kannan, I. P. Kumar and M. Bala, Andrologia, 2006, 38, 199–207 Search PubMed
. - M. Bala, C. S. Beniwal, R. P. Tripathi and J. Prasad, Int. J. Pharm. Sci. Rev. Res., 2014, 27, 238–248 Search PubMed
. - M. Saini, M. Bala, H. Farooqi, M. Abdin and J. Prasad, Int. J. Pharm. Pharm. Sci., 2014, 6, 161–166 Search PubMed
. - M. Bala and P. Kaur, Int. J. Pharm. Sci. Rev. Res., 2015, 34, 109–113 Search PubMed
. - M. Bala, M. Gupta, M. Saini, M. Z. Abdin and J. Prasad, Evid. base Compl. Alternative Med., 2015, 2015, 1–10 Search PubMed
. - M. Bala, V. Gupta and J. Prasad, Pharm. Biol., 2017, 55, 1833–1842 Search PubMed
. - J. Zhang, C. Wang, C. Wang, B. Sun and C. Qi, Food Funct., 2018, 9, 5402–5416 Search PubMed
. - B. A. Khan and N. Akhtar, Adv. Dermatol. Allergol., 2014, 31, 229–234 Search PubMed
. - I. S. Hwang, J. E. Kim, S. I. Choi, H. R. Lee, Y. J. Lee, M. J. Jang, H. J. Son, H. S. Lee, C. H. Oh, B. H. Kim, S. H. Lee and D. Y. Hwang, Int. J. Mol. Med., 2012, 30, 392–400 Search PubMed
. - A. Gegotek, A. Jastrzab, I. Jarocka-Karpowicz, M. Muszynska and E. Skrzydlewska, Antioxidants, 2018, 7, 110 Search PubMed
. - H. Ito, S. Asmussen, D. L. Traber, R. A. Cox, H. K. Hawkins, R. Connelly, L. D. Traber, T. W. Walker, E. Malgerud, H. Sakurai and P. Enkhbaatar, Burns, 2014, 40, 511–519 Search PubMed
. - J. Kim and C. M. Lee, Int. J. Biol. Macromol., 2017, 99, 586–593 Search PubMed
. - P. S. Larmo, R. L. Järvinen, N. L. Setälä, B. Yang, M. H. Viitanen, J. R. K. Engblom, R. L. Tahvonen and H. P. Kallio, J. Nutr., 2010, 140, 1462–1468 Search PubMed
. - Y. Kimura, D. Mori, T. Imada, Y. Izuta, M. Shibuya, H. Sakaguchi, E. Oonishi, N. Okada, K. Matsumoto and K. Tsubota, Nutrients, 2017, 9, 364 Search PubMed
. - Y. Wang, L. Zhao, Y. Huo, F. Zhou, W. Wu, F. Lu, X. Yang, X. Guo, P. Chen, Q. Deng and B. Ji, Nutrients, 2016, 8, 245 Search PubMed
. - Y. Wang, F. Huang, L. Zhao, D. Zhang, O. Wang, X. Guo, F. Lu, X. Yang, B. Ji and Q. Deng, J. Agric. Food Chem., 2016, 64, 161–170 Search PubMed
. - K. Bouras, K. Kopsidas, M. Bariotakis, P. Kitsiou, K. Kapodistria, G. Agrogiannis, I. Vergados, P. Theodossiadis and D. Perrea, Biomed. Hub., 2017, 2, 1–12 Search PubMed
. - S. Dubey, P. Deep and A. K. Singh, Vet. Ophthalmol., 2016, 19, 144–148 Search PubMed
. - H. Suleyman, L. O. Demirezer, M. E. Buyukokuroglu, M. F. Akcay, A. Gepdiremen, Z. N. Banoglu and F. Gocer, Phytother Res., 2001, 15, 625–627 Search PubMed
. - N. K. Huff, A. D. Auer, F. J. Garza, M. L. Keowen, M. T. Kearney, R. B. McMullin and F. M. Andrews, J. Vet. Intern. Med., 2012, 26, 1186–1191 Search PubMed
. - S. Attri, K. Sharma, P. Raigond and G. Goel, Food Res. Int., 2018, 105, 324–332 Search PubMed
. - W. Ni, T. Gao, H. Wang, Y. Du, J. Li, C. Li, L. Wei and H. Bi, J. Ethnopharmacol., 2013, 150, 529–535 Search PubMed
. - X. Zheng, W. Long, G. Liu, X. Zhang and X. Yang, J. Sci. Food Agric., 2012, 92, 736–742 Search PubMed
. - J. Purushothaman, G. Suryakumar, D. Shukla, A. S. Malhotra, H. Kasiganesan, R. Kumar, R. C. Sawhney and A. Chami, Brain Res. Bull., 2008, 77, 246–252 Search PubMed
. - J. Purushothaman, G. Suryakumar, D. Shukla, H. Jayamurthy, H. Kasiganesan, R. Kumar and R. C. Sawhney, Evid. base Compl. Alternative Med., 2011, 2011, 574524 Search PubMed
. - J. Y. Zhou, S. W. Zhou, X. H. Du and S. Y. Zeng, Molecules, 2012, 17, 11585–11597 Search PubMed
. - P. Sharma, G. Suryakumar, V. Singh, K. Misra and S. B. Singh, Int. J. Biometeorol., 2015, 59, 1115–1126 Search PubMed
. - P. Zhao, S. Wang, C. Liang, Y. Wang, P. Wen, F. Wang and G. Qin, Regul. Toxicol. Pharmacol., 2017, 91, 50–57 Search PubMed
. - N. K. Upadhyay, R. Kumar, S. K. Mandotra, R. N. Meena, M. S. Siddiqui, R. C. Sawhney and A. Gupta, Food Chem. Toxicol., 2009, 47, 1146–1153 Search PubMed
. - A. Rashid, A. Raisuddin, N. D. Kumar, J. Abhinav, T. Amit, M. Gaurav, S. Sarwat and B. Aseem, Int. J. Drug Dev. Res., 2011, 3, 356–365 Search PubMed
. - P. Wen, P. Zhao, G. Qin, S. Tang, B. Li, J. Zhang and L. Peng, Drug Chem. Toxicol., 2020, 43, 391–397 Search PubMed
. - S. Saggu, H. M. Divekar, V. Gupta, R. C. Sawhney, P. K. Banerjee and R. Kumar, Food Chem. Toxicol., 2007, 45, 609–617 Search PubMed
. - R. Tulsawani, P. Sharma, H. M. Divekar, R. N. Meena, M. Singh and R. Kumar, J. Compl. Integr. Med., 2010, 7, 1–21 Search PubMed
. - R. Tulsawani, Food Chem. Toxicol., 2010, 48, 2483–2489 Search PubMed
.
Footnotes |
† Electronic supplementary information (ESI) available. See DOI: 10.1039/d0ra06488b |
‡ The authors contributed equally to this work. |
|
This journal is © The Royal Society of Chemistry 2020 |
Click here to see how this site uses Cookies. View our privacy policy here.