DOI:
10.1039/D0QO00987C
(Review Article)
Org. Chem. Front., 2020,
7, 3956-3966
The same oxygenation-state introduction of hypervalent sulfur under transition-metal-free conditions
Received
17th August 2020
, Accepted 16th October 2020
First published on 16th October 2020
Abstract
Sulfonyl compounds have attracted considerable interest due to their extensive applications in drug discovery. Access to the assembly of SO2-containing compounds via the oxygenation oxidative-state introduction of hypervalent sulfur has come to the fore in recent years. This review discusses the application of the same oxygenation-state introduction of hypervalent sulfur strategy under transition-metal-free conditions, and presents it in light of different atoms being linked to the sulfonyl group, including sulfones, sulfonamides, sulfonyl fluorides and thiosulfonates.
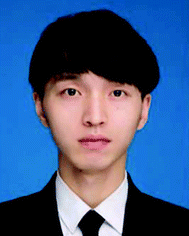 Daming Zeng | Daming Zeng received his B.S. degree in 2018 from Xingzhi College Zhejiang Normal University. He is now working with Professor Wei-Peng Deng and Professor Xuefeng Jiang toward his master's degree at East China University of Science and Technology. |
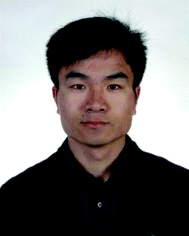 Ming Wang | Ming Wang received his Ph.D. degree in 2011 from East China University of Science and Technology under the guidance of Professor Wei-Ping Deng. From 2011 to 2014, he was a postdoctoral researcher under the guidance of Professor Yonggui Robin Chi at Nanyang Technological University, Singapore. In 2014, he joined East China Normal University as a lecturer, and is currently an associate professor at East China Normal University. |
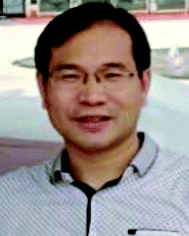 Wei-Ping Deng | Wei-Ping Deng has been a professor at East China University of Science and Technology since 2005. He received his B.S. degree in 1995 from Zhejiang Normal University. He then joined Professor Li-Xin Dai's research group at the Shanghai Institute of Organic Chemistry, Chinese Academy of Sciences, where he received his Ph.D. degree in 2000. From 2000 to 2003, Wei-Ping Deng worked as a postdoctoral researcher at the National Institute of Health (USA) and University of Rochester. From 2000 to 2003, he worked as a scientist in the Singapore company S*BIO. His current research interests are focused on asymmetric catalysis. |
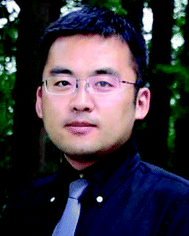 Xuefeng Jiang | Xuefeng Jiang is a professor at East China Normal University. He received his B.S. degree in 2003 from Northwest University (Xi'an, Shaanxi, China). He then joined Professor Shengming Ma's research group at the Shanghai Institute of Organic Chemistry, Chinese Academy of Sciences, where he received his Ph.D. degree in 2008. From 2008 to 2011, Xuefeng worked as a postdoctoral researcher on the total synthesis of natural products in the research group of Professor K. C. Nicolaou at The Scripps Research Institute. His independent research interests have focused on green sulfur chemistry and methodology-oriented total synthesis. |
1 Introduction
Sulfonyl compounds, having a long and rich history, most notably sulfones and sulfonamides,1 have received great attention as demonstrated by their extensive applications in medical,2 agricultural3 and materials science.4 Therefore, the construction of sulfone-containing compounds could be of considerable significance. Sulfonyl compounds are traditionally delivered via the oxidation of sulfides or the combination of sulfinates with aryl or alkyl electrophiles.5 Strategies for the synthesis of sulfonamides rely on the nucleophilic coupling of a pre-installed sulfonyl precursor, that is, sulfonyl chloride used most frequently, and appropriate amine sources.6 However, the substrates are usually derived from the odorous and unfriendly thiols, and the reactions need to be performed under harsh conditions, resulting in a low functional group tolerance and thus hampering their advancement in industrial applications. In order to distinguish the change of inorganic sulfur to organic sulfide, the concept of oxygenation-state was used (Scheme 1a). The same oxygenation-state means that the number of oxygen atoms attached to the sulfur atom remains unchanged in the reaction (Scheme 1b). Recently, the same oxygenation-state introduction of hypervalent sulfur conquered these obstacles, and allowed step- and oxidation-economical synthesis of SO2-containing compounds.7 DABSO was developed by Santos et al. in 1988, and was first applied by Willis and co-workers as a hypervalent sulfur surrogate.8 Subsequently, inorganic hypervalent sulfur sources like sodium/potassium metabisulfite could also be used as SO2 surrogates and achieve the same oxygenation-state introduction of hypervalent sulfur.9 During this process, the method of transition-metal catalysis could effectively connect sulfur dioxide with the coupling partners on both sides,5j,9c yet bringing about a series of cumbersome problems, such as expensive metal catalysts, inconvenient operation and potential β-H elimination. Accordingly, simple, efficient and transition-metal-free methods are well developed and present an upward tendency, as exemplified in the assembly of sulfones and sulfonamides, with a few examples providing sulfonyl fluorides and thiosulfonates. The process originates typically from a radical precursor, followed by trapping with a masked hypervalent sulfur source to produce the sulfonyl radical or sulfinate salt, which eventually couple with other partners to provide the desired products. The different properties of hypervalent sulfur sources lead to great differences in the same oxygenation-state introduction of hypervalent sulfur reactions. DABSO [DABCO·(SO2)2] and Na2S2O5 easily release SO2 with an π34 structure. Triplet SO2, possessing a lone pair, shows electrophilicity. Singlet SO2, which is excited to the active state with one electron transferring to the empty orbit, displays a di-radical feature. The homolytic cleavage of Na2S2O4 furnishes double sulfur dioxide radical anions, which show bilaterally nucleophilic radical and single-electron reductant features. Thiourea dioxide can generate a sulfur dioxide anion, which can easily form a sulfur dioxide radical anion under basic conditions and shows nucleophilic radical features (Scheme 2). Due to the different properties of hypervalent sulfur sources, the reactions of the same oxygenation-state introduction of hypervalent sulfur are rich and colorful.
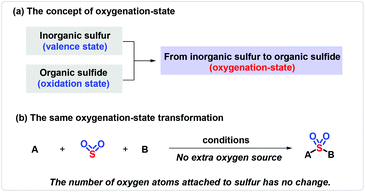 |
| Scheme 1 The concept of oxygenation-state. | |
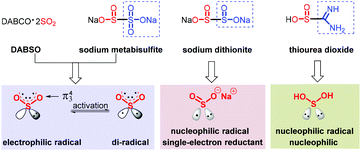 |
| Scheme 2 Various hypervalent sulfur sources. | |
2 Sulfones
Sulfones, formed by the combination of a sulfonyl and hydrocarbon group, play a prominent role in organic chemistry and drug discovery. Sulfones are commonly prepared by the oxidation of sulfides under the condition of a strong oxidant, 3-chloroperoxybenzoic acid (mCPBA) or hydrogen peroxide etc., and the starting material sulfides need to be achieved from the smelly thiols. A multicomponent cross-coupling strategy, based upon the same oxygenation-state introduction of hypervalent sulfur, has attracted much interest, owing to the flexible tuning of the substituents and step economy.9 According to the difference in the carbon moieties, which connect on both sides of the sulfonyl group, the modular assembly of sulfones can be classified into the following four categories: C(sp2)–C(sp2), C(sp2)–C(sp3), C(sp)–C(sp3) and C(sp3)–C(sp3).
2.1 C(sp2)–C(sp2)
In 2016, a metal-free strategy was disclosed by Wu and co-workers, liberating a series of 3-sulfonated coumarins via the sulfonyl radicals from aryldiazonium tetrafluoroborate and DABSO (Scheme 3a).10 Meanwhile, a plausible reaction mechanism was proposed (Scheme 3c) and indicated that diazonium 2 firstly removes N2 with the assistance of DABSO, followed by trapping of in situ formed sulfur dioxide to obtain the vital species 7, which undergoes the radical addition, spirocyclization and ester migration. Eventually, the intermediate H accesses the corresponding 3-substituted sulfones by further aromatization.
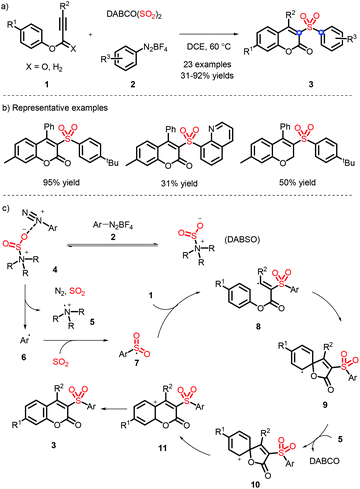 |
| Scheme 3 Synthesis of 3-sulfonated coumarins. | |
Subsequently, Wu's group achieved the ortho C(sp2)–H sulfonylation using anilines by virtue of the sulfonyl radical intermediates (Scheme 4).11 Although the process is performed under catalyst-free conditions to afford the functional products in moderate to good yields, the aniline substrates exhibit a limited scope and only methyl and methoxy groups are installed on the para-position.
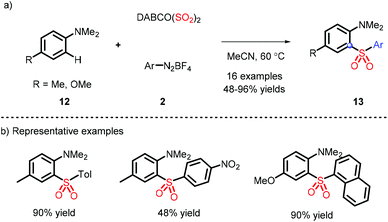 |
| Scheme 4 Sulfonylation of anilines via the sulfonyl radical intermediates. | |
The substrates that couple with the sulfonyl radical are not only restricted to electron-donating compounds; electron deficient substrates can also proceed well. In 2019, Xia et al. reported the synthesis of 2-sulfonyl-quinolines under metal- and oxidant-free conditions (Scheme 5a), which showed a wide substrate scope12 (Scheme 5b). It is different from the previous system, which was catalyzed by CuOTf.13 The intermediate 7a not only reacts with 14a, but also implements efficiently deoxygenation in combination with 16a (Scheme 5c).
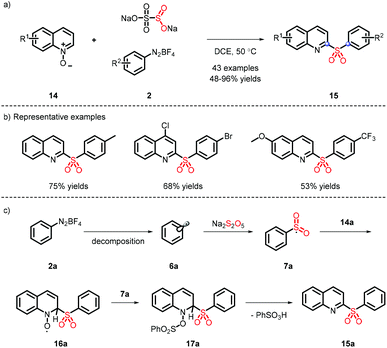 |
| Scheme 5 Synthesis of 2-sulfonated isoquinolines. | |
2.2 C(sp2)–C(sp3)
Tu and co-workers reported an unexpected discovery for the synthesis of cyclobuta[a]naphthalen-4-ols, which were efficiently decorated with sulfone and azo functionalities.14 As depicted in Scheme 6, the species 20 was formed by a cascade [2 + 2] cycloaddition, and was then captured by a sulfonyl radical and diazonium to obtain the target compounds. Accordingly, the substrate 2 plays a dual role to construct C–S and C–N bonds.
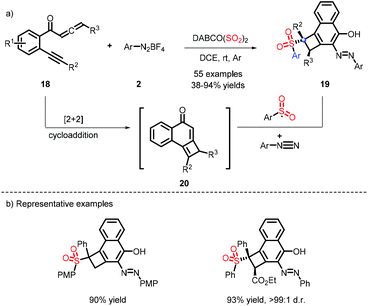 |
| Scheme 6 Synthesis of cyclobuta[a]naphthalen-4-ols from species 20. | |
When the sulfonyl radical is intercepted by an aryl olefin, followed by the attack of a nucleophilic radical, the bifunctionalization of the alkene is achieved. Based upon this protocol, Wu et al. developed a four-component reaction for oxysulfonylation by taking advantage of different sulfur dioxide sources, DABSO and K2S2O5, which are both effective in this process (Scheme 7).15
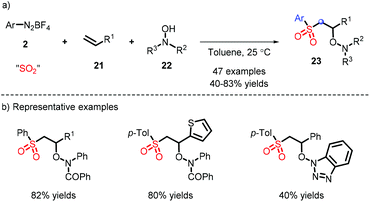 |
| Scheme 7 Difunctionalization of alkene with the insertion of SO2. | |
Diazonium is continually used as a radical precursor, yet faces unavoidable issues, such as instability and limited scope. In 2019, Wu and co-workers employed aryl or alkyl iodides, K2S2O5 and 25 under ultraviolet irradiation to obtain the corresponding radicals, and thus realized the construction of allylic sulfones (Scheme 8).16 It is worth mentioning that toluene, as a non-polar solvent, can also participate in the introduction of SO2.
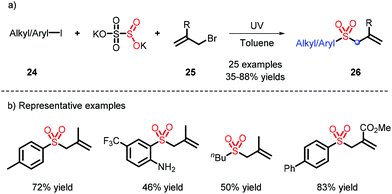 |
| Scheme 8 Proto-induced synthesis of allylic sulfones. | |
A diverse range of heteroaryl sulfones were furnished from different sulfur dioxide surrogates (thiourea17 and sodium dithionite18) under metal-free conditions, which was reported by Li's and Wu's groups (Scheme 9). The difference in their systems lies in the reaction mechanism (Scheme 9c): thiourea dioxide is subjected to hydrolysis and oxidation to provide the sulfur dioxide anion 31, which is coupled with the heteroaryl radical 32 and electrophile 28 to prepare heteroaryl alkyl sulfones; the species 31 is formed via the homolysis of sodium dithionite, without needing to be oxidized by virtue of a photocatalyst. Meanwhile, the sulfur dioxide radical anion B serves as a reductant and promotes the generation of sulfinate intermediate 33.
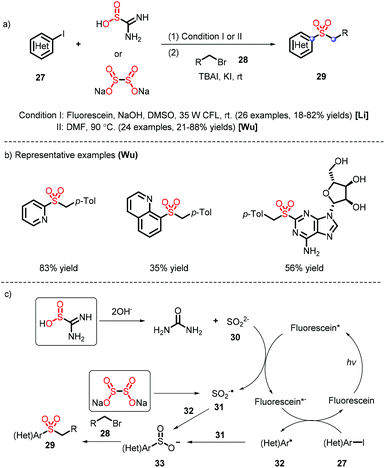 |
| Scheme 9 Synthesis of heteroaryl alkyl sulfones. | |
2.3 C(sp)–C(sp3)
In 2020, a metal-free strategy for alkynyl sulfone construction was developed by Wu et al. 4-Alkyl Hantzsch esters were used as alkyl radical sources via the activation of Eosin B (Scheme 10).19 The reaction afforded a chain of C(sp)–C(sp3) sulfones in good and excellent yields, and a few examples of the alkyl scope were also furnished.
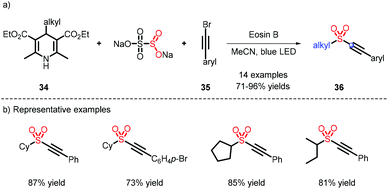 |
| Scheme 10 Synthesis of alkynyl sulfones from 4-alkyl Hantzsch esters. | |
2.4 C(sp3)–C(sp3)
Due to only a small amount of alkyl substrates being obtained in the previous work (Scheme 8), potassium alkyltrifluoroborates, serving as abundant alkyl partners, were applied by Wu's group for the construction of alkyl sulfones, and showed good alkyl compatibility (Scheme 11a).20 Cyclopropyl, which is prone to undergoing an open-ring reaction, could be tolerated in this transformation. When alkyl iodine was involved in the process, it needed a reductant to form an alkyl radical, followed by interception of DABSO, and addition to an electron-withdrawing alkene to afford alkyl-alkyl sulfones (Scheme 11b).21
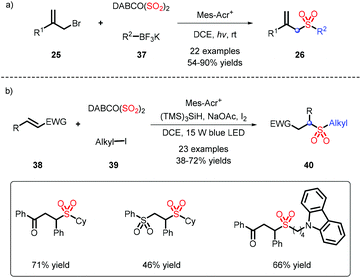 |
| Scheme 11 Photoredox-catalyzed reaction to construct alkyl sulfones. | |
In light of the transformation from inorganic sulfur to organic sulfurs by our group, the assembly of alkyl-alkyl sulfones was efficiently accomplished, which employed an available phosphate ester as an alkyl source leading to favorable results (Scheme 12).22 This strategy was also deployed in late-stage modification, as exemplified by coupling estrone or cholesterol with a SO2Me fragment.
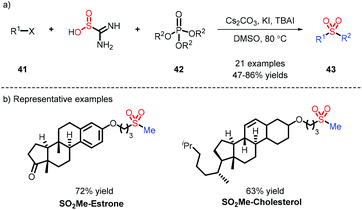 |
| Scheme 12 Synthesis of alkyl-alkyl sulfones via phosphate esters. | |
3 Sulfonamides
Sulfonamide, as an indispensable motif, showcases excellent properties and exists extensively in the pharmaceutical and agrochemical industries. Traditionally, sulfonamides are prepared through oxidation of the corresponding thiols with chlorine gas with the assistance of aqueous hydrochloric acid, and the subsequent amination of sulfonyl chloride. Analogous to the synthesis of sulfones, the introduction of sulfur dioxide is also extensively deployed in the assembly of sulfonamide. However, the study of these compounds converges mainly on carbon substitution, including aryl or alkyl diazonium, aryl halides and aryl boronic acids.
3.1 Aryl or alkyl diazonium
The metal-free synthesis of N-aminosulfonamides was reported by Wu and co-workers, who employed aryldiazonium and hydrazines in combination with DABSO under mild conditions to afford favorable yields (Scheme 13a).23 Moreover, a probable process is demonstrated in Scheme 13b, wherein the substituted nitrogen of the hydrazine attacked SO2, rather than the “free” nitrogen, and subsequently the species E was formed, where the radical tended to be installed in an unsubstituted nitrogen. Regrettably, the system was only suitable for hydrazine compounds. On the basis of this mechanism, the same group elaborated the substrate placement of an alkene moiety and implemented the construction of multiple bonds (comprising C–C, C–S and S–N). It is interesting that a one-pot synthesis could be effectively performed from anilines (Scheme 13c).24
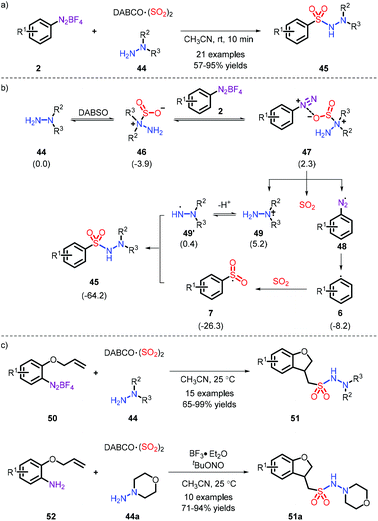 |
| Scheme 13 Synthesis of N-aminosulfonamides via aryl diazonium. | |
In addition, aryl hydrazine also acts as a precursor of diazonium. The reaction provides a range of N-aminosulfonamides with moderate yields in the presence of air, and was developed by Han and co-workers (Scheme 14).25
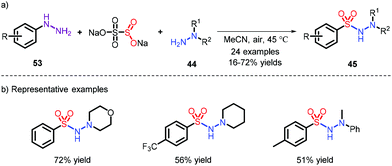 |
| Scheme 14 Synthesis of N-aminosulfonamides via aryl hydrazines. | |
Compared to aryl fragment, the synthesis of alkyl sulfonamides is less covered, owing to the fact that diazoniums bearing an alkyl moiety, possessing the risk of explosion, are not easily accessible. Accordingly, Tsai and co-workers developed a method via in situ generation of diazo compounds to realize the preparation of alkyl sulfonamides (Scheme 15).26
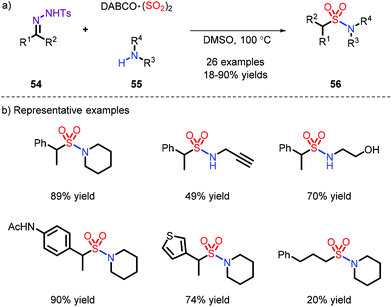 |
| Scheme 15 Synthesis of alkyl sulfonamides via the hydrazones. | |
In 2018, the first metal-free example was established toward the synthesis of free sulfonamides via Na2S2O5 as the sulfur dioxide source and sodium azide as the nitrogen source by our group (Scheme 16).27 The strategy was also extended to prepare the sulfonamide-containing drugs Celecoxib and Sulpiride at a later stage. In this process, the role of PPh3 was important (Scheme 16c): the aryl radical 6b is firstly formed through the action of PPh3via a SET pathway. Subsequently, the species 62 reacts with sodium azide, followed by combination of an aryl sulfonyl radical. Eventually, the obtained intermediate 64 (as proved by 31P NMR) is exposed to water and removes a N2 and O
PPh3, thereby releasing the corresponding products. However, the employment of explosive NaN3 makes the current strategy risky.
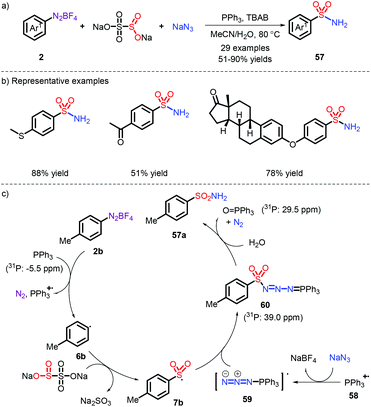 |
| Scheme 16 Synthesis of free sulfonamides via sodium azide. | |
3.2 Aryl halide and its derivative
On account of the unstable nature of aryldiazonium salts as radical precursors, a series of different substrates were employed,16,28 most frequently aryl bromides, iodides and iodoniums. Accordingly, in order to capture the momentum, Wu et al. used low-cost and available aryl bromides to achieve this reaction under metal-free conditions, which provided N-aminosulfonamides with 34% to 94% yields under the condition of UV (Scheme 17).28b Pleasingly, when the bromides were replaced with chlorines, the reaction could also effectively proceed.
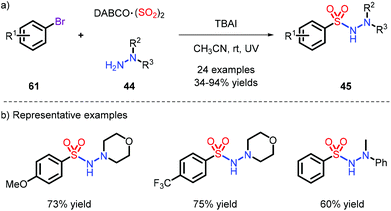 |
| Scheme 17 Synthesis of N-aminosulfonamides via aryl bromide substrates. | |
Since the use of high-energy ultraviolet irradiation leads to a very limited substrate scope, Manolikakes's group outlined a novel synthetic pathway by employing aryl-iodoniums as radical precursors with visible light to furnish the products (Scheme 18).28d It was a pity that aniline was not tolerated in their system.
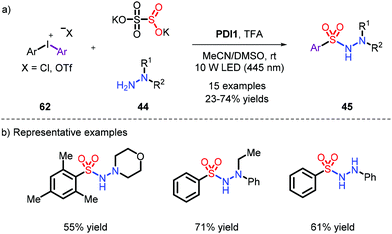 |
| Scheme 18 Synthesis of N-aminosulfonamides via diaryliodonium salts with hydrazines. | |
An additive-free reaction toward trifluoro-methylation and aminosulfonylation of alkynes was disclosed by Wu et al. Their reaction employs Togni's reagent with DABSO and hydrazines (Scheme 19).29 The trifluoromethyl radical is generated by induction of hydrazine, which removes a hydrogen radical, and provides access to the N-aminosulfonamides via coupling with a sulfonyl radical. However, the use of stoichiometric amounts of Togni's reagent makes the current reaction costly.
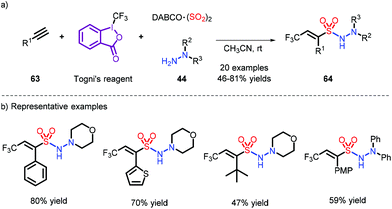 |
| Scheme 19 Vicinal difunctionalization of alkynes through trifluoromethylation and aminosulfonylation. | |
3.3 Aryl boronic acid
The introduction of sulfur dioxide is generally restricted to the assembly of N-aminosulfonamides. There are a dearth of examples of the preparation of sulfonamides, which need transition-metal catalysts such as Pd30 and Au.31 Nitroarenes, as readily available and low-cost nitrogen sources, have been successfully employed for the synthesis of sulfonamides in combination with aryl boronic acid, which was reported by Chen32 and us33 respectively (Scheme 20a). The reaction displays a wide substrate scope and was extended to the linking of different biomolecules and pharmaceuticals via the SO2NH fragment by us (Scheme 20b). A plausible mechanism was proposed: K2S2O5 was used to release SO2, which not only integrated with aryl boronic acid to form the sulfinate 33, but also facilitated the deoxygenation of 72 and 74 species (Scheme 20c).
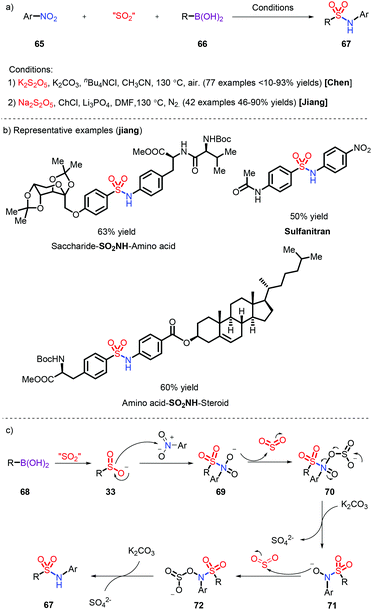 |
| Scheme 20 Synthesis of sulfonamides from nitroarenes. | |
4 Sulfonyl fluorides
SuFEx (sulfur fluoride exchange), the new embodiment of click chemistry, was first disclosed by Sharpless et al.,34 presenting powerful flexibility for modular linkage of different nucleophilic compounds. Intriguingly, a strategy where sulfonyl fluoride serves as a chemical biology probe has been applied to identify amino acid residues.35 The synthesis of sulfonyl fluorides is typically implemented via fluoride-chloride exchange, which is deemed to be the most practical method. However, the substrates, sulfonyl chlorides, face unavoidable issues as they are liable to undergo hydrolysis and redox side reactions. A strategy for steering clear of the highly active molecules can be accomplished via the in situ formed sulfinate by a Pd catalyst and electrophilic fluoride sources (Selectfluor etc.).36 Although great progress has been demonstrated, the use of a toxic metal catalyst limits this strategy to small-scale applications.
Weng and co-workers developed a metal-free method to access the sulfonyl fluorides via aryl diazoniums (Scheme 21).37 The reaction needs MeOH as a solvent and heating to obtain the corresponding products, indicating the favorable stability of sulfonyl fluoride.
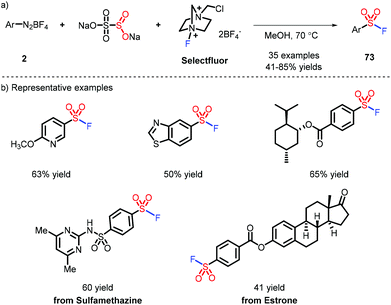 |
| Scheme 21 Synthesis of sulfonyl fluorides from Selectfluor. | |
5 Thiosulfonates
In contrast to sulfones and sulfonamides, sulfur-linked sulfonyl compounds thiosulfonates, which display biological activities such as anti-viral and anti-bacterial, are less studied.38 Moreover, thiosulfonate derivatives, as prominent building blocks, have been diffusely used in organic synthesis owing to their desirable stability and reactivity.39 Conventionally, the thiosulfonates are prepared via the oxidation of the corresponding disulfides or thiols and the reduction of sulfonyl chlorides. When an aryl or alkyl fragment is located in the thiosulfonyl skeleton, the compounds displayed symmetry and asymmetry. In general, the construction of unsymmetrical thiosulfonates was relatively difficult due to the chemoselectivity issue.
5.1 Symmetrical thiosulfonates
In 2019, Wu and co-workers reported an unexpected reaction which afforded a library of symmetrical thiosulfonates using thiourea as the sulfur source via a photoinduced strategy (Scheme 22),40 yet with the original purpose to obtain N-carbamothioylargonsulfonamide. Nevertheless, the process was not suitable for the connection of different aryl groups.
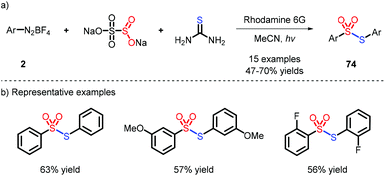 |
| Scheme 22 Synthesis of symmetrical thiosulfonates from thiourea. | |
5.2 Unsymmetrical thiosulfonates
Very recently, the preparation of unsymmetrical thiosulfonates was achieved by combination of different SO2 surrogates and thiols as sulfur sources, as described by Yang41 and Ji42 (Scheme 23). The role of TFA, noteworthily, was to react with 2 and promote the generation of the aryl radical.
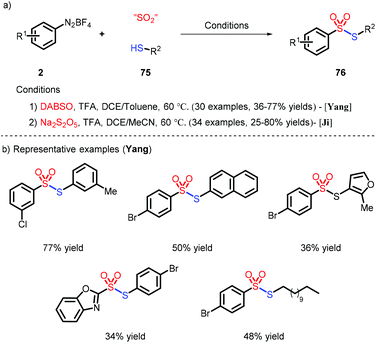 |
| Scheme 23 Synthesis of unsymmetrical thiosulfonates from thiols. | |
6 Conclusions
The sulfonyl-containing compounds are not only emerging widely in the pharmaceutical, agricultural and material fields, but are also utilized frequently in organic synthesis, implementing the modular assembly of natural products and bioactive molecules. Accordingly, the same oxygenation-state introduction of hypervalent sulfur has been an active research area for accessing SO2-containing molecules, due to the untrammeled varying of the substituents, avoiding some pre-formed precursors. Compared with transition-metal-catalyzed methods, transition-metal-free strategies are characterized by inexpensive materials, low toxicity and simple operation. The synthesis of SO2-containing compounds has witnessed much development in the past several years, as exemplified by sulfones, sulfonamides, sulfonyl fluorides and thiosulfonates. Although a chain of efficient protocols have been outlined, cumbersome issues still exist: (1) the aryl radical source relies mainly on the diazonium salts, which are not conducive to storage and exhibit a risk of explosion; (2) harsh reaction conditions, such as high temperature or UV radiation, might be needed to enable the generation of intermediates, resulting in narrow substrate scopes; (3) in most cases, the nitrogen-linked partner was restricted to the hydrazine, thereby obtaining merely the N-aminosulfonamide; (4) lower selectivity and efficiency was presented etc. Hence, optimized and practical methods via the same oxygenation-state introduction of hypervalent sulfur for the assembly of SO2-containing compounds are still indispensable. More favorable outcomes, we believe, will be exposed in the future, where new reaction patterns (different from the traditional method via the sulfonyl radical or sulfinate) are widely deployed and structurally complicated compounds are expediently furnished.
Conflicts of interest
There are no conflicts to declare.
Acknowledgements
We thank the National Key Research and Development Program of China (2017YFD0200500), NSFC (21971065, 21722202, 21672069 and 21871089 for M.W.), STCSM (20XD1421500, 20JC1416800, 18JC1415600), Professor of Special Appointment (Eastern Scholar) at Shanghai Institutions of Higher Learning, and Innovative Research Team of High-Level Local Universities in Shanghai (SSMU-ZLCX20180501).
Notes and references
-
(a) R. Bentley, Different roads to discovery; Prontosil (hence sulfa drugs) and penicillin (hence β-lactams), J. Ind. Microbiol. Biotechnol., 2009, 36, 775–786 CrossRef CAS;
(b) K. A. Scott and J. T. Njardarson, Analysis of US FDA-approved drugs containing sulfur atoms, Top. Curr. Chem., 2018, 376, 5 CrossRef.
-
(a) M. Teall, P. Oakley, T. Harrison, D. Shaw, E. Kay, J. Elliott, U. Gerhard, J. L. Castro, M. R. Shearman, G. Ball and N. N. Tsou, Aryl sulfones: a new class of γ-secretase inhibitors, Bioorg. Med. Chem. Lett., 2005, 15, 2685–2688 CrossRef CAS;
(b) Y. Harrak, G. Casula, J. Basset, G. Rosell, S. Plescia, D. Raffa, M. G. Cusimano, R. Pouplana and M. D. Pujol, Synthesis, anti-inflammatory activity, and in vitro antitumor effect of a novel class of cyclooxygenase inhibitors: 4-(Aryloyl)phenyl methyl sulfones, J. Med. Chem., 2010, 53, 6560–6571 CrossRef CAS;
(c) M. Feng, B. Tang, S. Liang and X. Jiang, Sulfur containing scaffolds in drugs: Synthesis and application in Medicinal Chemistry, Curr. Top. Med. Chem., 2016, 16, 1200–1216 CrossRef CAS.
-
(a) K. Grossmann and T. Ehrhardt, On the mechanism of action and selectivity of the corn herbicide topramezone: a new inhibitor of 4−hydroxyphenylpyruvate dioxygenase, Pest Manage. Sci., 2007, 63, 429–439 CrossRef CAS;
(b) W. M. Xu, F. F. Han, M. He, D. Y. Hu, J. He, S. Yang and B. A. Song, Inhibition of tobacco bacterial wilt with sulfone derivatives containing an 1,3,4-oxadiazole moiety, J. Agric. Food Chem., 2012, 60, 1036–1041 CrossRef CAS;
(c) Y. Noutoshi, M. Ikeda, T. Saito, H. Osada and K. Shirasu, Sulfonamides identified as plant immune-priming compounds in high-throughput chemical screening increase disease resistance in Arabidopsis thaliana, Front. Plant Sci., 2012, 3, 245 Search PubMed;
(d) P. Devendar and G. F. Yang, Sulfur-Containing Agrochemicals, Top. Curr. Chem., 2017, 375, 82 CrossRef.
-
(a) M. Kakimoto, S. J. Grunzinger and T. Hayakawa, Hyperbranched poly(ether sulfone)s: preparation and application to ion-exchange membranes, Polym. J., 2010, 42, 697–705 CrossRef CAS;
(b) H. Sasabe, Y. Seino, M. Kimura and J. Kido, A m-terphenyl-modifed sulfone derivative as a host material for high-efficiency blue and green phosphorescent OLEDs, Chem. Mater., 2012, 24, 1404–1406 CrossRef CAS;
(c) G. Turkoglu, M. E. Cinar and T. Ozturk, Thiophene-Based Organic Semiconductors, Top. Curr. Chem., 2017, 375, 84 CrossRef.
-
(a) T. Markovic, P. R. D. Murray, B. N. Rocke, A. Shavnya, D. C. Blakemore and M. C. Willis, Heterocyclic allylsulfones as latent heteroaryl nucleophiles in palladium-catalyzed cross-coupling reactions, J. Am. Chem. Soc., 2018, 140, 15916–15923 CrossRef CAS;
(b) K. Kamata, T. Hirano and N. Mizuno, Highly efficient oxidation of sulfides with hydrogen peroxide catalyzed by [SeO4{WO(O2)2}2]2-, Chem. Commun., 2009, 3958–3960 RSC;
(c) T. Noguchi, Y. Hirai and M. Kirihara, Highly selective 30% hydrogen peroxideoxidation of sulfides to sulfoxides using micromixing, Chem. Commun., 2008, 3040–3042 RSC;
(d) G. Maayan, R. Popovitz-Biro and R. Neumann, Micelle directed synthesis of polyoxometalate nanoparticles and their improved catalytic activity for the aerobic oxidation of sulfides, J. Am. Chem. Soc., 2006, 128, 4968–4969 CrossRef CAS;
(e) G. A. Olah, T. Mathew and P. G. K. Surya, Nafion-H catalysed sulfonylation of aromatics with arene/alkenesulfonic acids for the preparation of sulfones, Chem. Commun., 2001, 1696–1697 RSC;
(f) W. Su, An efficient method for the oxidation of sulfides to sulfones, Tetrahedron Lett., 1994, 35, 4955–4958 CrossRef CAS;
(g) H. H. Szmant and G. Suld, Concerning the Variable Character of the Sulfone Group, J. Am. Chem. Soc., 1956, 78, 3400–3403 CrossRef CAS;
(h) J. Aziz, S. Messaoudi, M. Alami and A. Hamze, Sulfinate derivatives: dual and versatile partners in organic synthesis, Org. Biomol. Chem., 2014, 12, 9743–9759 RSC;
(i) E. J. Emmett and M. C. Willis, The development and application of sulfur dioxide surrogates in synthetic organic chemistry, Asian J. Org. Chem., 2015, 4, 602–611 CrossRef CAS;
(j) M. C. Willis, New catalytic reactions using sulfur dioxide, Phosphorus, Sulfur Silicon Relat. Elem., 2019, 194, 654–657 CrossRef CAS.
-
(a) M. Nishikawa, Y. Inaba and M. Furukawa, Behavior of sulfinic acid toward N-chloramines and related compounds, Chem. Pharm. Bull., 1983, 31, 1374–1377 CrossRef CAS;
(b) S. J. Caddick, D. Wilden and D. B. Judd, Direct synthesis of sulfonamides and activated sulfonate esters from sulfonic acids, J. Am. Chem. Soc., 2004, 126, 1024–1025 CrossRef CAS;
(c) X. Tang, L. Huang, C. Qi, X. Wu, W. Wu and H. Jiang, Copper-catalyzed sulfonamides formation from sodium sulfinates and amines, Chem. Commun., 2013, 49, 6102–6104 RSC;
(d) K. Yang, M. Ke, Y. Lin and Q. Song, Sulfonamide formation from sodium sulfinates and amines or ammonia under metal-free conditions at ambient temperature, Green Chem., 2015, 17, 1395–1399 RSC;
(e) W. Wei, C. Liu, D. Yang, J. Wen, J. You and H. Wang, Metal–Free Direct Construction of Sulfonamides via Iodine– Mediated Coupling Reaction of Sodium Sulfinates and Amines at Room Temperature, Adv. Synth. Catal., 2015, 357, 987–992 CrossRef CAS.
-
(a)
A. S. Deeming and M. C. Willis, 1,4-Disulfino-1,4-diazabicyclo [2.2.2]octane, bis(inner salt), in eEROS, Encyclo-pedia of Reagents for Organic Synthesis, Wiley, 2016 Search PubMed;
(b)
D. Zheng and J. Wu, Sulfur Dioxide Insertion Reactions for Organic Synthesis, Springer, Singapore, 2017, pp. 11–77, ISBN: 978-981-10-4202-7 CrossRef.
-
(a) P. S. Santos and M. T. S. Mello, The Raman spectra of some molecular complexes of 1-azabicyclo[2.2.2]octane and 1,4-diazabicyclo[2.2.2]octane, J. Mol. Struct., 1988, 178, 121–133 CrossRef CAS;
(b) B. Nguyen, E. J. Emmet and M. C. Willis, Palladium-catalyzed aminosulfonylation of aryl halides, J. Am. Chem. Soc., 2010, 132, 16372–16373 CrossRef CAS.
- Reviews:
(a) M. Wang and X. Jiang, The recycling of sulfur dioxide, Chin. Sci. Bull., 2018, 63, 2707–2716 CrossRef;
(b) G. Qiu, K. Zhou, L. Gao and J. Wu, Insertion of sulfur dioxide via a radical process: an efficient route to sulfonyl compounds, Org. Chem. Front., 2018, 5, 691–705 RSC;
(c) G. Qiu and J. Wu, Inorganic sulfites as the sulfur dioxide surrogates in sulfonylation reactions, Chem. Commun., 2019, 55, 1013–1019 RSC;
(d) S. Ye, M. Yang and J. Wu, Recent advances in sulfonylation reactions using potassium/sodium metabisulfite, Chem. Commun., 2020, 56, 4145–4155 RSC.
- D. Zheng, J. Yu and J. Wu, Generation of sulfonyl radicals from aryldiazonium tetrafluoroborates and sulfur dioxide: The synthesis of 3-sulfonated coumarins, Angew. Chem., Int. Ed., 2016, 55, 11925–11929 CrossRef CAS.
- K. Zhou, J. Zhang, L. Lai, J. Cheng, J. Sun and J. Wu, C-H bond sulfonylation of anilines with the insertion of sulfur dioxide under metal-free conditions, Chem. Commun., 2018, 54, 7459–7462 RSC.
- G. You, D. Xi, J. Sun, L. Hao and C. Xia, Transition-metal- and oxidant-free three-component reaction of quinoline N-oxides, sodium metabisulfite and aryldiazonium tetrafluoroborates via a dual radical coupling process, Org. Biomol. Chem., 2019, 17, 9479–9488 RSC.
- G. H. Li, D. Q. Dong, Q. Deng, S. Q. Yan and Z. L. Wang, Copper-catalyzed deoxygenative C2-sulfonylation of quinoline N-oxides with DABSO and phenyldiazonium tetrafluoroborates for the synthesis of 2-sulfonylquinolines via a radical reaction, Synthesis, 2019, 3313–3319 CAS.
- F. Liu, J. Y. Wang, P. Zhou, G. Li, W. J. Hao, S. J. Tu and B. Jiang, Merging [2 + 2] cycloaddition with radical 1,4-addition: Metal-free access to functionalized cyclobuta[a]naphthalen-4-ols, Angew. Chem., Int. Ed., 2017, 56, 15570–15574 CrossRef CAS.
- J. Zhang, Y. An and J. Wu, Vicinal difunctionalization of alkenes through a multicomponent reaction with the insertion of sulfur dioxide, Chem. – Eur. J., 2017, 23, 9477–9480 CrossRef CAS.
- J. Zhang, K. Zhou, G. Qiu and J. Wu, Photoinduced synthesis of allylic sulfones using potassium metabisulfite as the source of sulfur dioxide, Org. Chem. Front., 2019, 6, 36–40 RSC.
- S. Ye, Y. Li, J. Wu and Z. Li, Thiourea dioxide as a source of sulfonyl groups: photoredox generation of sulfones and sulfonamides from heteroaryl/aryl halides, Chem. Commun., 2019, 55, 2489–2492 RSC.
- Y. Li, T. Liu, G. Qiu and J. Wu, Catalyst-free sulfonylation of (hetero)aryl iodides with sodium dithionite, Adv. Synth. Catal., 2019, 361, 1154–1159 CrossRef CAS.
- X. Gong, M. Yang, J. B. Liu, F. S. He, X. Fan and J. Wu, A metal-free route to alkynyl sulfones under photoinduced conditions with the insertion of sulfur dioxide, Green Chem., 2020, 22, 1906–1910 RSC.
- S. Ye, X. Li, W. Xie and J. Wu, Three-component reaction of potassium alkyltrifluoroborates, sulfur dioxide and allylic bromides under visible-light irradiation, Asian J. Org. Chem., 2019, 8, 893–898 CrossRef CAS.
- S. Ye, D. Zheng, J. Wu and G. Qiu, Photoredox-catalyzed sulfonylation of alkyl iodides, sulfur dioxide, and electron-deficient alkenes, Chem. Commun., 2019, 55, 2214–2217 RSC.
- S. Chen, Y. Li, M. Wang and X. Jiang, General sulfone construction via sulfur dioxide surrogate control, Green Chem., 2020, 22, 322–326 RSC.
- D. Zheng, Y. An, Z. Li and J. Wu, Metal-free aminosulfonylation of aryldiazonium tetrafluoroborates with DABCO·(SO2)2 and hydrazines, Angew. Chem., Int. Ed., 2014, 53, 2451–2454 CrossRef CAS.
- Y. An, D. Zheng and J. Wu, Synthesis of 1-(2,3-dihydrobenzofuran-3-yl)-methanesulfonohydrazides through insertion of sulfur dioxide, Chem. Commun., 2014, 50, 11746–11748 RSC.
- Y. Wang, B. Du, W. Sha, H. Mei, J. Han and Y. Pan, Transition-metal-free oxidative reaction of hydrazines and potassium metabisulfite for preparation of sulfonohydrazides, Org. Chem. Front., 2017, 4, 1313–1317 RSC.
- A. S. Tsai, J. M. Curto, B. N. Rocke, A. M. R. Dechert-Schmitt, G. K. Ingle and V. Mascitti, One-step synthesis of sulfonamides from N–tosylhydrazones, Org. Lett., 2016, 18, 508–511 CrossRef CAS.
- M. Wang, Q. Fan and X. Jiang, Metal-free construction of primary sulfonamide through three diverse salts, Green Chem., 2018, 20, 5469–5473 RSC.
-
(a) C. K. Prier, D. A. Rankic and D. W. C. MacMillan, Visible Light Photoredox Catalysis with Transition Metal Complexes: Applications in Organic Synthesis, Chem. Rev., 2013, 113, 5322–5363 CrossRef CAS;
(b) Y. Li, D. Zheng, Z. Li and J. Wu, Generation of N-aminosulfonamides via a photoinduced fixation of sulfur dioxide into aryl/alkyl halides, Org. Chem. Front., 2016, 3, 574–578 RSC;
(c) X. Gong, Y. Ding, X. Fan and J. Wu, Synthesis of β–keto sulfones via coupling of aryl/alkyl halides, sulfur dioxide and silyl enolates through metal–free photoinduced C–X bond dissociation, Adv. Synth. Catal., 2017, 359, 2999–3004 CrossRef CAS;
(d) N. W. Liu, S. Liang and G. Manolikakes, Visible–light photoredox–catalyzed aminosulfonylation of diaryliodonium salts with sulfur dioxide and hydrazines, Adv. Synth. Catal., 2017, 359, 1308–1319 CrossRef CAS.
- Y. Li, Y. Xiang, Z. Li and J. Wu, Direct vicinal difunctionalization of alkynes through trifluoromethylation and aminosulfonylation via insertion of sulfur dioxide under catalyst-free conditions, Org. Chem. Front., 2016, 3, 1493–1497 RSC.
- A. S. Deeming, C. J. Russell and M. C. Willis, Palladium(II)-catalyzed synthesis of sulfinates from boronic acids and DABSO: A redox-neutral, phosphine-free transformation, Angew. Chem., Int. Ed., 2016, 55, 747–750 CrossRef CAS.
- M. W. Johnson, S. W. Bagley, N. P. Mankad, R. G. Bergman, V. Mascitti and F. D. Toste, Application of fundamental organometallic chemistry to the development of a gold-catalyzed synthesis of sulfinate derivatives, Angew. Chem., Int. Ed., 2014, 53, 4404–4407 CrossRef CAS.
- K. Chen, W. Chen, B. Han, W. Chen, M. Liu and H. Wu, Sequential C-S and S-N coupling approach to sulfonamides, Org. Lett., 2020, 22, 1841–1845 CrossRef CAS.
- Y. Li, M. Wang and X. Jiang, Straightforward sulfonamidation via metabisulfite-mediated cross coupling of nitroarenes and boronic acids under transition-metal-free conditions, Chin. J. Chem., 2020, 38, 1521–1525 Search PubMed.
- J. Dong, L. Krasnova, M. G. Finn and K. B. Sharpless, Sulfur(VI) fluoride exchange (SuFEx): Another good reaction for click chemistry, Angew. Chem., Int. Ed., 2014, 53, 9430–9448 CrossRef CAS.
-
(a) A. Narayanan and L. H. Jones, Sulfonyl fluorides as privileged warheads in chemical biology, Chem. Sci., 2015, 6, 2650–2659 RSC;
(b) O. Fadeyi, M. D. Parikh, M. Z. Chen, R. E. J. Kyne, A. P. Taylor, I. O'Doherty, S. E. Kaiser, S. Barbas, S. Niessen, M. Shi, S. L. Weinrich, J. C. Kath, L. H. Jones and R. P. Robinson, Chemoselective Preparation of Clickable Aryl Sulfonyl Fluoride Monomers: A Toolbox of Highly Functionalized Intermediates for Chemical Biology Probe Synthesis, ChemBioChem, 2016, 17, 1925–1930 CrossRef CAS.
-
(a) A. T. Davies, J. M. Curto, S. W. Bagley and M. C. Willis, One-pot palladium-catalyzed synthesis of sulfonyl fluorides from aryl bromides, Chem. Sci., 2017, 8, 1233–1237 RSC;
(b) A. L. Tribby, I. Rodriguez, S. Shariffudin and N. D. Ball, Pd-catalyzed conversion of aryl iodides to sulfonyl fluorides using SO2 surrogate DABSO and selectfluor, J. Org. Chem., 2017, 82, 2294–2299 CrossRef CAS;
(c) T. S. B. Lou, S. W. Bagley and M. C. Willis, Cyclic alkenylsulfonyl fluorides: Palladium–catalyzed synthesis and functionalization of compact multifunctional reagents, Angew. Chem., Int. Ed., 2019, 58, 18859–18863 CrossRef CAS.
- T. Zhong, M. K. Pang, Z. D. Chen, B. Zhang, J. Weng and G. Lu, Copper-free sandmeyer-type reaction for the synthesis of sulfonyl fluorides, Org. Lett., 2020, 22, 3072–3078 CrossRef CAS.
-
(a) J. P. Weidner and S. S. Block, Alkyl and aryl thiolsulfonates, J. Med. Chem., 1964, 7, 671–673 CrossRef CAS;
(b) P. Natarajan, Facile synthesis of symmetric thiosulfonates by oxidation of disulfide with oxone/MX (MX = KBr, KCl, NaBr and NaCl), Tetrahedron Lett., 2015, 56, 4131–4134 CrossRef CAS.
-
(a) Z. H. Peng, X. Zheng, Y. J. Zhang, D. L. An and W. R. Dong, H2O2-mediated metal-free protocol towards unsymmetrical thiosulfonates from sulfonyl hydrazides and disulfides in PEG-400, Green Chem., 2018, 20, 1760–1764 RSC;
(b) Q. Zhao, L. Lu and Q. Shen, Direct monofluoromethylthiolation with S-(fluoromethyl) benzenesulfonothioate, Angew. Chem., Int. Ed., 2017, 56, 11575–11578 CrossRef CAS;
(c) W. Wang, X. Peng, F. Wei, C. H. Tung and Z. Xu, Copper(I)–catalyzed interrupted click reaction: Synthesis of diverse 5−hetero–functionalized triazoles, Angew. Chem., Int. Ed., 2016, 55, 649–653 CrossRef CAS;
(d) P. Mampuys, Y. Zhu, S. Sergeyev, E. Ruijter, R. V. A. Orru, S. V. Doorslaer and B. U. W. Maes, Iodide-catalyzed synthesis of secondary thiocarbamates from isocyanides and thiosulfonates, Org. Lett., 2016, 18, 2808–2811 CrossRef CAS;
(e) P. Mampuys, Y. Zhu, T. Vlaar, E. Ruijter, R. V. A. Orru and B. U. W. Maes, Sustainable three-component synthesis of isothioureas from isocyanides, thiosulfonates, and amines, Angew. Chem., Int. Ed., 2014, 53, 12849–12854 CrossRef CAS;
(f) S. Kim, S. Kim, N. Otsuka and I. Ryu, Tin-free radical carbonylation: Thiol ester synthesis using alkyl allyl sulfone precursors, phenyl benzenethiosulfonate, and CO, Angew. Chem., Int. Ed., 2005, 44, 6183–6186 CrossRef CAS.
- X. Gong, X. Li, W. Xie, J. Wu and S. Ye, An unexpected reaction of aryldiazonium tetrafluoroborates, sodium metabisulfite, and thiourea under photoinduced conditions, Org. Chem. Front., 2019, 6, 1863–1867 RSC.
- G. Li, Z. Gan, K. Kong, X. Dou and D. Yang, Metal-free synthesis of thiosulfonates via insertion of sulfur dioxide, Adv. Synth. Catal., 2019, 361, 1808–1814 CrossRef CAS.
- C.-M. Huang, J. Li, S.-Y. Wang and S.-J. Ji, TFA promoted multi-component reaction of aryldiazonium with sodium metabisulphite and thiols: Construction of thiosulfonate under transition-metal free conditions, Chin. Chem. Lett., 2020, 31, 1923–1926 CrossRef CAS.
|
This journal is © the Partner Organisations 2020 |