DOI:
10.1039/D4RA02833C
(Paper)
RSC Adv., 2024,
14, 21174-21179
An efficient alternative to DBU in the oxathiaphospholane (OTP) method for the solid phase synthesis of P-stereodefined phosphorothioate analogs†
Received
16th April 2024
, Accepted 23rd June 2024
First published on 4th July 2024
Abstract
This study presents a modified (extended) 1,3,2-oxathiaphospholane (OTP) method for the synthesis of P-stereodefined phosphorothioate analogs in the presence of previously unused organic bases. TBD (5,7-triazabicyclo[4.4.0]dec-5-ene) and Verkade's proazaphosphatrane (2,8,9-trimethyl-2,5,8,9-tetraaza-1-phosphabicyclo[3.3.3]undecane) are herein used for the first time as efficient organic bases compared to DBU, which is commonly used in the OTP approach.
Introduction
Only a few methods have been developed for stereocontrolled synthesis of phosphorothioate oligonucleotides, including methods that utilize N-acyl oxazaphospholidine1 (Beaucage et al.), oxazaphosphorinane2 (Just et al.) and bicyclic oxazaphospholidine3 (Agrawal et al.) derivatives. Wada and co-workers have developed a methodology to synthesize stereochemically regular PS-ODNs using diastereopure nucleoside 3′-O-(1,3,2-oxazaphospholidine) monomers (originally proposed by Agrawal et al.).4 The first method for stereocontrolled chemical synthesis of phosphorothioate oligonucleotides, which was developed by Stec et al., is based on a chemistry employing P-diastereomerically pure monomers possessing the 2-thio-1,3,2-oxathiaphospholane moiety.5 Their applicability and limitations have been summarized in several reports.6 Recently, Baran et al. described a method using a citrus-derived PSI reagent.7
General mechanistic principle of the OTP method
In the OTP method, the P-chiral nucleoside 3′-O-(2-thio-1,3,2-oxathiaphospholane) monomers are prepared as a mixture of both P-isomers, which can be separated by silica gel column chromatography. Separated P-diastereomers may be used for the synthesis of diastereomerically pure fragments of phosphorothioate oligonucleotides. In this synthesis, the oxathiaphospholane reacts with the 5′–OH group of a nucleoside (or a growing oligonucleotide attached to the solid support) in the presence of 1,8-diazabicyclo[5.4.0]undec-7-ene(DBU). This leads to an intermediate, which undergoes pseudorotation (permutational isomerization), followed by the elimination of the episulfide to form an internucleotide phosphorothioate linkage (Scheme 1).8 In the synthesis of PS-DNA oligomers, repetitive yields in the range of 92–94% are usually observed, making the synthesis of oligomers longer than 12–14 nucleotides problematic; although successful but very low yields of 23-mers have also been reported.9
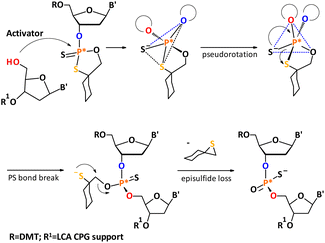 |
| Scheme 1 Synthesis of P-stereodefined phosphorothioate analogs developed by Stec et al. A mechanism of DBU-promoted condensation step. | |
The OTP approach promoted by DBU has been successfully used for the stereocontrolled synthesis of phosphorothioate oligonucleotides and their analogues. For instance, in our laboratory, the OTP method was also used for the stereocontrolled synthesis of PS-LNA analogs (Locked Nucleic Acids)10 and PS-GNA analogs (Glycol Nucleic Acids).11 Recently, there has been successful contribution to research in the field of P-diastereomerically pure PS-RNA and PS-(2′-OMe) RNA derivatives.12
The constantly increasing number of approved nucleic acid therapeutics shows their great potential.13 It is necessary to provide more detailed information on the influence of the absolute configuration of the phosphorus atoms in P-stereodefined oligonucleotides. The studies carried out so far have shown their potential. It has been demonstrated that the configuration of the phosphorus atom has an influence on biological activity when interacting with biomolecules. RP-PS linkages are preferred for duplex formation with complementary ORN strands,14 while SP-PS linkages are chosen to increase stability towards the 3′-exonuclease present in the human plasma.15 PS DNA in which both the RP and SP-PS linkages are properly arranged would therefore be better drug candidates than those synthesized in a non-stereocontrolled manner. Therefore, the stereoselective synthesis of such compounds is an important goal to achieve. In view of the growing interest of molecular biologists, biochemists, as well as pharmaceutical companies in the synthesis of modified oligonucleotides and their therapeutic application,16 more efficient organic bases must be considered.
Results and discussion
Novel and efficient organic bases in OTP method
In the search for new organic bases, we focused on strong, yet non-nucleophilic bases. We found bases consisting of nitrogenated bases and proazaphosphatranes (Fig. 1).
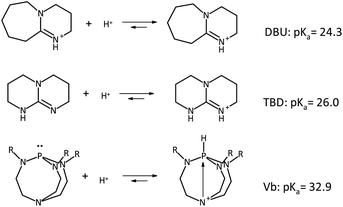 |
| Fig. 1 Non-nucleophilic bases used in this study. DBU (1,8-diazabicyclo[5.4.0]undec-7-ene), TBD (1,5,7-triazabicyclo[4.4.0]dec-5-ene), Verkade base (Vb, in this study: R = methyl group, 2,8,9-trimethyl-2,5,8,9-tetraaza-1-phosphabicyclo[3.3.3]undecane), their protonated molecules and pKas in acetonitrile.17 | |
Here we discovered that TBD (1,5,7-triazabicyclo[4.4.0]dec-5-ene) and one of the Verkade bases, 2,8,9-trimethyl-2,5,8,9-tetraaza-1-phosphabicyclo[3.3.3]undecane, can be used as alternatives and efficient bases for the 1,3,2-oxathiaphospholane ring-opening condensation (Scheme 2).
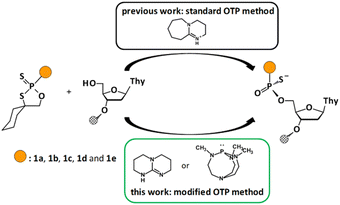 |
| Scheme 2 Synthesis of dinucleoside phosphorothioate 1PST by the oxathiaphospholane approach. | |
TBD is a strong bicyclic guanidine base (pKa in acetonitrile: 26.0)18 with a steric hindrance and the ability, when protonated, to delocalize the positive charge between amino groups and the double bond. Because of their strong basicity and relatively weak nucleophilicity, proazaphosphatranes have also attracted attention. Proazaphosphatrane bases have been used in multiple types of catalytic processes for organic synthesis.19 Pioneered by Verkade et al.,20 the ‘molecular football’ shaped compound is commonly known as Verkade's superbase (in this paper labeled as Vb).
The term ‘superbase’ is given to organic compounds whose basicity is greater than that of 1,8-bis(dimethylamino)naphthalene (proton sponge),21 with pKa of 18.6 (conjugate acid form) in acetonitrile. The pKa of the protonated form of proazaphosphatranes ranges from 31.6 to 33.6 in acetonitrile, depending on the substituents attached to the equatorial nitrogen atoms R (in this study R = Me). The conjugate acid [HP(MeNCH2CH2)3N]+ has a pKa of 32.9 in acetonitrile;22 thus, this base is by eight orders of magnitude stronger than DBU.23
General protocol of the OTP method
Synthesis of P-stereodefined oligonucleotides by oxathiaphospholane method was carried out manually at a 1 μmol scale, according to the previously published protocols. This method uses a CPG-support (controlled pore glass, LCAA CPG 500 Å, ChemGenes, USA), to which a 5′-O-DMT-nucleoside unit (in this study: loading 35 μmol g−1) is attached with a succinyl-sarcosinyl type linker (Scheme 3). To prevent the DBU-promoted intramolecular cleavage of the standard LCAA (long chain alkylamino) linker, a modified sarcosine linker [–COCH2CH2CON(CH3)CH2CO–LCAA–CPG] is used.24 Coupling steps are carried out using 20 mg of 3′-O-OTP-derivatives of the standard 5′-O-DMT-protected nucleosides in the presence of 1 M DBU in acetonitrile. Manual preparation (without the synthesizer) is considered demanding in terms of the preparation of reagents and technical skills during the oligonucleotide synthesis. Our previous observations indicate that some parameters, such as amount of monomer, coupling time, and base concentration play a major role in determining the coupling yield during synthesis.
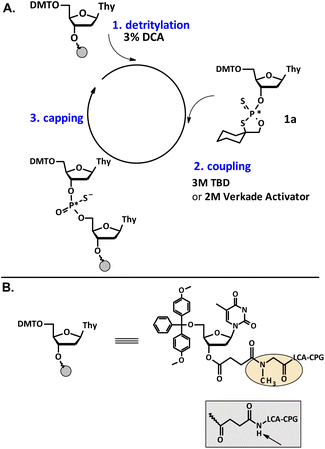 |
| Scheme 3 (A) Synthetic cycle for the preparation of oligonucleotide fragment from 1a; (B) structures of sarcosine linker and linker with no sarcosine are highlighted in light orange and grey, respectively. | |
UV monitoring of the solid-phase synthesis efficacy
The efficiency of solid phase synthesis was studied by condensation of 1a–e with thymidine (attached to the solid support). We analyzed the coupling efficiency of the set of oxathiaphospholane monomers 1 (as a mixture of P-diastereomers) in the presence of novel organic non-nucleophilic bases. The experimental protocol involves use of previously tested 5′-O-DMT-protected 3′-O-(2-thio-“spiro”-4,4-pentamethylene-1,3,2-oxathiaphospholane) derivatives, i.e. 2′-deoxynucleoside (1a), locked nucleic acid (1b), 2′-O-methyl ribonucleoside (1c), and 2′-O-TBDMS-ribonucleoside (1d) monomers. It should be noted that previously unknown oxathiaphospholane derivatives of morpholino nucleosides (1e)25 were also synthesized and used in this modified OTP protocol (Fig. 2).
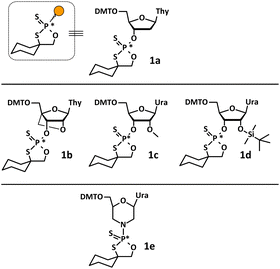 |
| Fig. 2 All OTP monomers: DNA (1a), LNA (1b), 2′–OMe (1c), 2′–OTBDMS (1d), morpholino (1e) as a mixture of P-diastereomers used in this study. | |
Detailed procedure for synthesis of mU-OTP is presented in the ESI.† For all synthesized products the same support (which possessed the thymidine 3′-O-attached to LCA CPG linker with loading 35 μmol g−1) was used to eliminate any possible effect of support variability. The coupling efficacy is controlled by measurement of absorption at 504 nm coming from the released DMT+ cation.
The efficiency of monomer DNA condensation in the standard OTP procedure using 1 M DBU (50-fold molar excess) is up to 95%. Attempts to use the same concentrations of novel bases did not lead to satisfactory results. When using 3 M TBD (150-fold molar excess) and 2 M Verkade base (25-fold molar excess), the efficacy was 93% (Table 1 and Fig. S4–18, ESI†). Even with double coupling, we did not observe a significantly increased yield of condensation. This result suggests that TBD and Verkade base can be successfully used alternatively.
Table 1 Coupling yields with OTP monomers in solid phase synthesis of the dimers calculated from the DMT+ decay assay
OTP monomer |
Standard OTP method |
Modified OTP method |
DBUa |
TBDb |
Verkadec |
1 M DBU solution in anhydrous acetonitrile. 2 M TBD solution in anhydrous acetonitrile. 3 M Verkade solution in anhydrous acetonitrile. Double coupling. |
DNA (1a) |
95 |
93 |
93 |
LNA (1b) |
88d |
86d |
86d |
2’-OMe (1c) |
84d |
86d |
83d |
2’-OTBDMS (1d) |
91d |
92d |
92 |
mU (1e) |
61d |
93d |
95 |
Working earlier with LNA-derived OTP monomers using the standard DBU-assisted OTP method, we observed that in the cycles where LNA-OTP monomers were incorporated, double coupling was necessary (20 mg + 20 mg, ∼25-fold molar excess) and in both steps the coupling time was extended to 20 min (Table 1 and Fig. S1, ESI†). Although the repetitive average yield of the coupling (88%, based on the DMT+ cation assay) was relatively low as compared with DNA OTP (1a), it was considered to be satisfactory enough to attempt synthesis of chimeric P-stereodefined PS-(DNA/LNA). In the case of 3 M TBD solution in acetonitrile, the yield of a single coupling step of LNA monomer was approximately 65%. Thus, it was necessary to use double coupling (20 mg + 20 mg of OTP monomer 1b) and the coupling time was extended to 20 minutes. Repetitive yield, calculated from the DMT+ cation assay, was 86%. It is noted that the repetitive yield of condensation reduced when using 3 M TBD and 2 M Vb (86%, assessed by the DMT+ cation assay) despite the use of double coupling. In the case of synthesizing LNA oligonucleotides, 1 M DBU is still the best option. In the next step, the 2′-OMe monomer (1c) was investigated. In standard protocol with 1 M DBU, only ca. 84% repetitive yield was achieved (based on a DMT+ cation assay), even with double coupling. Additional experiments using 3 M TBD revealed slightly higher repetitive yield. The dimer was obtained at 86% yield as assessed from the DMT+ cation assay. The coupling yield for 1c using the Verkade base was unsatisfactory. Even with double coupling, only ca. 83% repetitive yield was achieved (based on a DMT+ cation assay). Attempts to increase the yield by extension of the coupling time or by increasing Verkade base concentration gave similar results and were unsuccessful. Therefore, only the 3 M TBD can be used interchangeably with DBU in the 2′-OMe monomer condensation. Next, a derivative of 2′-OTBDMS (1d) was used and resulted in a 91% repetitive yield (based on a DMT+ cation assay) using the standard OTP method. A significant increase of repetitive yield of condensation for double coupling using TBD (92%, assessed by the DMT+ cation assay) was observed. The coupling of 1d monomer using Verkade base was also achieved in a higher yield (92% based on a DMT+ cation assay) than that of 1 M DBU.
The most interesting results obtained were those for the morpholino monomer (1e). At first, the standard protocol with 1 M DBU was used, and double coupling at the condensation steps led to a significant drop in coupling efficiency and a 61% repetitive yield was noted (based on a DMT+ cation assay). There was no improvement with a longer reaction time. Attempts to increase the yields by extending the coupling time or increasing the DBU concentration were also unsuccessful. In the case of 3 M TBD solution in acetonitrile, the yield of a single coupling step was approximately 85%; therefore, a double coupling (20 mg + 20 mg of the OTP monomer 1e) was required and the coupling time was extended to 20 min. Repetitive yield, calculated from the DMT+ cation assay, was 93%.
We noted a significantly increased repetitive yield of condensation for 2 M Vb (95%, assessed by the DMT+ cation assay). It should be emphasized that a single coupling is sufficient in this case. The data in Table 1 show that the initial condensations with a 2′-deoxy nucleoside bound to a solid support proceeded with the required 90+% efficacy only for monomers 1a, 1d and 1e, while 1b and 1c offered a lower but acceptable coupling yield.
Next, longer analogs were synthesized. The yield of the first coupling step of 5T_T and 5T_V (as a mixture of P-diastereomers) with the 5'–OH group of a deoxyribonucleoside attached to a solid support was acceptable (93%) and the repetitive yields associated with the subsequent coupling steps were equally high (Table 2, Fig. S19 and 20, ESI†).
Table 2 Average yield of the four consecutive coupling steps (%) in synthesis of pentamers TPSTPSTPSTPST 5T_T and 5T_V using 3 M TBD and 2 M Verkade, respectively (calculated from the DMT+ decay assay)
The coupling of OTP monomer DNA (1a) |
Modified otp method |
5T_T 3 M TBD |
5T_V 2 M Verkade |
1st coupling |
93 |
93 |
2nd coupling |
93 |
92 |
3rd coupling |
92 |
91 |
4th coupling |
93 |
90 |
The experiments with the application of new bases to the synthesis of P-stereodefined phosphorothioate dinucleosides were performed. The pure diastereomer, fast-eluted of 1a, reacted with thymidine (attached to the solid support) to yield the diastereomerically pure 5′OH–TPST–OH, and the diastereomeric purity was confirmed by HPLC analysis (Fig. S21–25, ESI†). The above experiments confirm that the tested bases can be used successfully for the highly stereoselective synthesis of P-chiral phosphorothioate analogs.
Conclusions
In conclusion, our studies highlight a new route for the development of the OTP method by applying novel bases. We have discovered TBD (1,5,7-triazabicyclo[4.4.0]dec-5-ene) and the simplest Verkade base, i.e. 2,8,9-trimethyl-2,5,8,9-tetraaza-1-phosphabicyclo[3.3.3]undecane, which have been shown to be efficient bases in the 1,3,2-oxathiaphospholane ring-opening condensation. Optimization of solid-phase synthesis of phosphorothioate dinucleotides was performed by condensation of monomers 1a–e (as a mixture of P-diastereomers) with 5′–OH–thymidine in the presence of various non-nucleophilic bases: DBU, TBD and Vb. Based on experiments, the optimal concentrations of TBD and Verkade base required to achieve maximum yields in solid phase synthesis were determined to be 3 and 2 M, respectively. Oligonucleotide synthesis with the application of TBD as a base is an economical alternative. This choice is mainly due to the inexpensiveness of TBD. However, it should be noted that a double coupling (increased consumption of handmade monomers) must be included in the synthetic protocol in this case. In the second procedure for the synthesis of P-stereodefined phosphorothioate analogs using Verkade solution, we observed a 93% yield with a single coupling using OTP. However, the Verkade base-activated coupling is more expensive. From the above-mentioned results, it may be concluded that the formation of internucleotide phosphorothioate linkage involving derivative 1 via the oxathiaphospholane route with novel bases is efficient, stereocontrolled, and with stereoselectivity.
Further studies are underway to adapt the modified oxathiaphospholane (OTP) approach to the synthesis of P-stereodefined phosphorothioate oligonucleotides.
Author contributions
K. J. supervised the project, prepared and edited the manuscript, as well as performed all the chemical synthesis and data analysis.
Data availability
The data supporting this article have been included as part of the ESI.†
Conflicts of interest
There are no conflicts of interest to declare.
Acknowledgements
This work was financially supported by National Centre of Science, Poland, grant UMO-2021/43/D/ST4/02433 (to K. J.). An Avance Neo 400 NMR spectrometer was purchased using funds provided by the EU Regional Operational Program of the Lodz Region RPLD.01.01.00-10-0008/18.
Notes and references
- A. Wilk, A. Grajkowski, L. R. Phillips and S. L. Beaucage, Deoxyribonucleoside Cyclic N-Acylphosphoramidites as a New Class of Monomers for the Stereocontrolled Synthesis of Oligothymidylyl- and Oligodeoxycytidylyl-Phosphorothioates, J. Am. Chem. Soc., 2000, 122, 2149 CrossRef CAS
. -
(a) Y. Jin, G. Biancotto and G. Just, A stereoselective synthesis of dinucleotide phosphorothioates, using chiral phosphoramidites as intermediates, Tetrahedron Lett., 1996, 37, 973–976 CrossRef CAS
;
(b) J.-C. Wang and G. Just, A stereoselective synthesis of dinucleotide phosphorothioates, using chiral indol-oxazaphosphorine intermediates, Tetrahedron Lett., 1997, 38, 3797–3800 CrossRef CAS
. - M. Guo, D. Yu, R. P. Iyer and S. Agrawal, Solid-phase stereoselective synthesis of 2′-O-methyloligoribonucleoside phosphorothioates using nucleoside bicyclic oxazaphospholidines, Bioorg. Med. Chem. Lett., 1998, 2539–2544 CrossRef CAS PubMed
. -
(a) N. Oka and T. Wada, Stereocontrolled synthesis of oligonucleotide analogs containing chiral internucleotidic phosphorus atoms, Chem. Soc. Rev., 2011, 40, 5829–5843 RSC
;
(b) N. Oka, T. Wada and K. Saigo, An Oxazaphospholidine Approach for the Stereocontrolled Synthesis of Oligonucleoside Phosphorothioates, J. Am. Chem. Soc., 2003, 125, 8307–8317 CrossRef CAS PubMed
;
(c) N. Oka, M. Yamamoto, T. Sato and T. Wada, Solid-Phase Synthesis of Stereoregular Oligodeoxyribonucleoside Phosphorothioates Using Bicyclic Oxazaphospholidine Derivatives as Monomer Units, J. Am. Chem. Soc., 2008, 130, 16031–16037 CrossRef CAS PubMed
. -
(a) W. J. Stec, A. Grajkowski, M. Koziołkiewicz and B. Uznański, Novel route to oligo(deoxyribonucleoside phosphorothioates). Stereocontrolled synthesis of P-chiral oligo(deoxyribonucleoside phosphorothioates), Nucleic Acids Res., 1991, 19, 5883–5888 CrossRef CAS PubMed
;
(b) W. J. Stec, B. Karwowski, M. Boczkowska, P. Guga, M. Koziołkiewicz, M. Sochacki, M. W. Wieczorek and J. Błaszczyk, Deoxyribonucleoside 3‘-O-(2-Thio- and 2-Oxo-“spiro”-4,4-pentamethylene-1,3,2-oxathiaphospholane)s: Monomers for Stereocontrolled Synthesis of Oligo(deoxyribonucleoside phosphorothioate)s and Chimeric PS/PO Oligonucleotide, J. Am. Chem. Soc., 1998, 120, 7156–7167 CrossRef CAS
. -
(a) P. Guga, A. Okruszek and W. J. Stec, Recent Advances in Stereocontrolled Synthesis of P-Chiral Analogues of Biophosphates, Top. Curr. Chem., 2002, 220, 169–200 CrossRef CAS
;
(b) B. Nawrot, B. Rębowska, B. Michalak, M. Bulkowski, D. Błaziak, P. Guga and W. J. Stec, 1,3,2-Oxathiaphospholane approach to the synthesis of P-chiral stereodefined analogs of oligonucleotides and biologically relevant nucleoside polyphosphates, Pure Appl. Chem., 2008, 80, 1859–1871 CrossRef CAS
. -
(a) K. W. Knouse, J. N. deGruyter, M. A. Schmidt, B. Zheng, J. C. Vantourout, C. Kingston, S. E. Mercer, I. M. Mcdonald, R. E. Olson, Y. Zhu, Ch. Hang, J. Zhu, Ch. Yuan, Q. Wang, P. Park, M. D. Eastgate and P. S. Baran, Unlocking P.(V.): Reagents for chiral phosphorothioate synthesis, Science, 2018, 361, 1234–1238 CrossRef CAS PubMed
;
(b) Y. Huang, K. W. Knouse, S. Qiu, W. Hao, N. M. Padial, J. C. Vantourout, B. Zheng, S. E. Mercer, J. Lopez-Ogalla, R. Narayan, R. E. Olson, D. G. Blackmond, M. D. Eastgate, M. A. Schmidt, I. M. McDonald and P. S. Baran, A P(V) platform for oligonucleotide synthesis, Science, 2021, 373, 1265–1270 CrossRef CAS PubMed
. -
(a) T. Uchimaru, W. J. Stec and K. Taira, Mechanism of the Chemoselective and Stereoselective Ring Opening of Oxathiaphospholanes: An Ab Initio Study, J. Org. Chem., 1997, 62, 5793–5800 CrossRef CAS
;
(b) K. Misiura, D. Szymanowicz, M. Olesiak and W.
J. Stec, DBU-assisted 1,3,2-oxathiaphospholane ring-opening condensation with selected O-, S-, N- and C-nucleophiles, Tetrahedron Lett., 2004, 45, 4301–4305 CrossRef CAS
. - T. Inagawa, H. Naksima, B. Karwowski, P. Guga, W. J. Stec, H. Takeuchi and H. Takaku, Inhibition of human immunodeficiency virus type 1 replication by P-stereodefined oligo(nucleoside phosphorothioate)s in a long-term infection model, FEBS Lett., 2002, 528, 48–52 CrossRef CAS PubMed
. -
(a) K. Jastrzębska, A. Maciaszek, R. Dolot, G. Bujacz and P. Guga, Thermal Stability and Conformation of Antiparallel Duplexes Formed by P-Stereodefined Phosphorothioate DNA/LNA Chimeric Oligomers with DNA and RNA Matrices, Org. Biomol. Chem., 2015, 13, 10032–10040 RSC
;
(b) K. Jastrzębska, B. Mikołajczyk and P. Guga, LNA units present in [RP-PS]-(DNA#LNA) chimeras enhance the thermal stability of parallel duplexes and triplexes formed with (2′-OMe)-RNA strands, RSC Adv., 2020, 10, 22370–22376 RSC
. - A. Tomaszewska-Antczak, K. Jastrzębska, A. Maciaszek, B. Mikołajczyk and P. Guga, P-Stereodefined phosphorothioate analogs of glycol nucleic acids – synthesis and structural properties, RSC Adv., 2018, 8, 24942–24952 RSC
. - K. Jastrzębska, A. Maciaszek, R. Dolot, A. Tomaszewska-Antczak, B. Mikołajczyk and P. Guga, Synthesis and hybridizing properties of P-stereodefined chimeric [PS]-{DNA:RNA} and [PS]-{DNA:(2′-OMe)-RNA} oligomers, RSC Adv., 2022, 12, 26815–26824 RSC
. - C. A. Stein and D. Castanotto, FDA-Approved Oligonucleotide Therapies in 2017, Mol. Ther., 2017, 25, 1069–1075 CrossRef CAS PubMed
. - M. Koziolkiewicz, A. Krakowiak, M. Kwinkowski, M. Boczkowska and W. J. Stec, Stereodifferentiation – the effect of P chirality of oligo(nucleoside phosphorothioates) on the activity of bacterial RNase H, Nucleic Acids Res., 1995, 23, 5000–5005 CrossRef CAS PubMed
. - M. Koziołkiewicz, M. Wójcik, A. Kobylańska, B. Karwowski, B. Rębowska, P. Guga and W. J. Stec, Stability of stereoregular oligo(nucleoside phosphorothioate)s in human plasma: diastereoselectivity of plasma 3'-exonuclease, Antisense Nucleic Acid Drug Dev., 1997, 7, 43–48 CrossRef PubMed
. -
(a) D. Collotta, I. Bertocchi, E. Chiapello and M. Collino, Antisense oligonucleotides: a novel Frontier in pharmacological strategy, Front. Pharmacol, 2023, 14, 1304342 CrossRef CAS PubMed
;
(b) J. A. Kulkarni, D. Witzigmann, S. B. Thomson, S. Chen, B. R. Leavitt, P. R. Cullis and R. van der Meel, The current landscape of nucleic acid therapeutics, Nat. Nanotechnol., 2021, 16, 630–643 CrossRef CAS PubMed
;
(c) B. T. Le, S. Paul, K. Jastrzebska, H. Langer, M. H. Caruthers and R. N. Veedu, Thiomorpholino oligonucleotides as a robust class of next generation platforms for alternate mRNA splicing, Proc. Natl. Acad. Sci. U. S. A., 2022, 119, e2207956119 CrossRef CAS PubMed
. - J. E. Taylor, S. D. Bull and J. M. Williams, Amidines, isothioureas, and guanidines as nucleophilic catalysts, Chem Soc Rev, 2012, 41, 2109 RSC
. - E. Fritz-Langhals, Unique Superbase TBD (1,5,7-Triazabicyclo[4.4.0]dec-5-ene): From Catalytic Activity and One-Pot Synthesis to Broader Application in Industrial Chemistry, Org. Process Res. Dev., 2022, 26(11), 3015–3023 CrossRef CAS
. - J. G. Verkade and P. B. Kisanga, Proazaphosphatranes: a synthesis methodology trip from their discovery to vitamin A, Tetrahedron, 2003, 59, 7819–7858 CrossRef CAS
. - C. Lensink, S. K. Xi, L. M. Daniels and J. G. Verkade, The unusually robust phosphorus-hydrogen bond in the novel cation [cyclic] HP(NMeCH2CH2)3N+, J. Am. Chem. Soc., 1989, 111, 3478–3479 CrossRef CAS
. - T. R. Puleo, S. J. Sujansky, S. E. Wright and J. S. Bandar, Organic Superbases in Recent Synthetic Methodology Research, Chem.–Eur. J., 2021, 13, 4216–4229 CrossRef PubMed
. - P. B. Kisanga, J. G. Verkade and R. Schwesinger, pKa Measurements of P(RNCH2CH3)3N, J. Org. Chem., 2000, 65, 5431–5432 CrossRef CAS PubMed
. - J. Gopalakrishnan, Aminophosphines: their chemistry and role as ligands and synthons, Appl. Organomet. Chem., 2009, 23, 291–318 CrossRef CAS
. - T. Brown, C. E. Pritchard, G. Turner and S. A. Salisbury, A new base-stable linker for solid-phase oligonucleotide synthesis, J. Chem. Soc. Chem. Commun., 1989, 14, 891–893 RSC
. - paper in preparation.
|
This journal is © The Royal Society of Chemistry 2024 |
Click here to see how this site uses Cookies. View our privacy policy here.