DOI:
10.1039/C5RA24629F
(Paper)
RSC Adv., 2016,
6, 2671-2677
TBAI/TBHP-catalyzed [3 + 2]cycloaddition/oxidation/aromatization cascade and online ESI-MS mechanistic studies: synthesis of pyrrolo[2,1-a]isoquinolines and indolizino[8,7-b]indoles†
Received
20th November 2015
, Accepted 16th December 2015
First published on 23rd December 2015
Abstract
A facile [3 + 2]cycloaddition/oxidation/aromatization cascade reaction for the synthesis of pyrrolo[2,1-a]isoquinolines and indolizino[8,7-b]indoles has been developed. This tandem approach was accomplished by employing tert-butyl hydroperoxide (TBHP) as the environmentally benign stoichiometric oxidant, with the catalysis of non-toxic tetrabutylammonium iodide (TBAI) and isopropanol as the green solvent. Gratifyingly, this protocol is highly versatile, as the construction of polycyclics could be tailored by readily available dipolarophiles. Only a catalytic amount of TBAI was required, as the hypervalent electrophilic iodine species (IOH) can be recovered in situ by oxidation with TBHP. Furthermore, for the first time, the mechanistic aspects and the putative intermediates associated with this cascade have been intercepted and characterized by online monitoring of the reaction by using ESI-MS/MS.
Introduction
The pyrrole ring is a common motif in numerous bioactive alkaloids and pharmaceuticals. The pyrrolo[2,1-a]isoquinoline core in the marine lamellarin alkaloids is responsible for their wide spectrum of biological activities. For instance, lamellarin D (A), is a potent inhibitor of human topoisomerase I and lamellarin α 20-sulfate (B) is an inhibitor of HIV integrase (Fig. 1).1 The indolizino[8,7-b]indole core present in β-carboline alkaloids such as harmicine (C) and fascaplysin (D, Fig. 1) has significant biological and pharmaceutical relevance.2 Despite some elegant syntheses of pyrrolo[2,1-a]isoquinolines3 and indolizino[8,7-b]indoles4 through [3 + 2]cycloadditions, the (hypo)iodite catalyzed green protocol has not yet been explored.
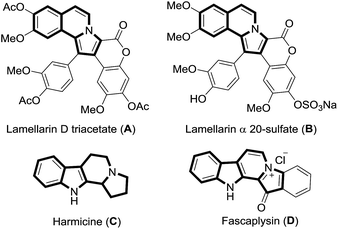 |
| Fig. 1 Representaitive natural lamellarin (A, B) and indolizino[8,7-b]indole (C, D) alkaloids. | |
In recent times, the tetrabutylammonium iodide (TBAI)/tert-butyl hydroperoxide (TBHP) catalytic system has received unique attention due to its high efficiency, simple operation, nontoxicity, inexpensiveness, and eco-friendliness.5 In continuation of our earlier efforts in the field of biologically relevant β-carbolines and their novel approaches,6 we report the first application of the green TBAI/TBHP catalysis in the [3 + 2]cycloaddition/oxidation/aromatization cascade reaction for the synthesis of pyrrolo[2,1-a]isoquinolines and indolizino[8,7-b]indoles. Furthermore, for the first time, the reaction mechanism and the intermediates associated with [3 + 2]cycloaddition/oxidation/aromatization cascade have been intercepted and characterized, by online monitoring of the reaction by employing ESI-MS/MS.
Results and discussion
The initial investigations were focused on the feasibility of the reaction between ethyl 2-(3,4-dihydroisoquinolin-2(1H)-yl)acetate (1a) and N-phenylmaleimide (2a). Optimization of the reaction conditions towards 1,3-dipoles and electron-deficient dipolarophiles were illustrated in Table 1. To our delight, the proposed reaction between 1a and 2a does indeed occur in the presence of TBAI (10 mol%) and 70% aqueous TBHP (3 equiv.) in DMF to afford the product 3a in 86% yield (entry 1, Table 1). Encouraged by this result, we then attempted to determine the ideal solvent for this transformation. The desired product 3a was obtained in all the tested solvents (entries 1–6), the highest yield of 95% was observed in both acetonitrile and isopropanol (entry 2, and 5, Table 1), and then we have chosen to proceed with isopropanol as it is a green solvent. The reaction did not proceed efficiently at room temperature (entry 7, Table 1) and a minimum temperature of 80 °C was found to be crucial.
Table 1 Optimization of the reaction conditionsa
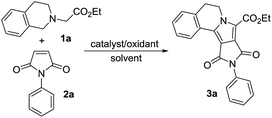
|
Entry |
Catalyst |
Oxidant |
Solvent |
Yieldb (%) |
Reaction conditions: 1a (1.5 mmol), 2a (1 mmol), catalyst (10 mol%), oxidant (3 equiv.), solvent (5.0 mL), 80 °C, 6 h.
Isolated yield.
At room temperature.
n.d. = not detected.
The amount of TBAI was increased to 20 mol%.
The amount of 70% aqueous TBHP was increased to 5 equiv.
The amount of TBAI was decreased to 5 mol%.
35% aqueous H2O2 was used.
|
1 |
TBAI |
TBHP |
DMF |
86 |
2 |
TBAI |
TBHP |
CH3CN |
95 |
3 |
TBAI |
TBHP |
Toluene |
42 |
4 |
TBAI |
TBHP |
1,4-Dioxane |
59 |
5 |
TBAI |
TBHP |
Isopropanol |
95 |
6 |
TBAI |
TBHP |
1-Butanol |
81 |
7c |
TBAI |
TBHP |
Isopropanol |
ndd |
8e |
TBAI |
TBHP |
Isopropanol |
96 |
9f |
TBAI |
TBHP |
Isopropanol |
96 |
10g |
TBAI |
TBHP |
Isopropanol |
72 |
11 |
TBAI |
Oxone |
Isopropanol |
69 |
12h |
TBAI |
H2O2 |
Isopropanol |
83 |
13 |
TBAI |
mCPBA |
Isopropanol |
65 |
14 |
TBAB |
TBHP |
Isopropanol |
77 |
The yield of 3a was not increased much when the amount of TBAI was increased up to 20 mol% (entry 8, Table 1) or when the amount of 70% aqueous TBHP was increased to 5 equiv. (entry 9, Table 1). However, when the amount of TBAI was decreased to 5 mol%, 3a was obtained in 72% yield (entry 10, Table 1). This supports the catalytic efficiency of the TBAI/TBHP oxidation system even at low concentration. Further, when other oxidants such as 35% aqueous H2O2, oxone and mCPBA were used instead of 70% aqueous TBHP, we obtained 3a in lower yields (entries 11–13, Table 1). Under the same reaction conditions, TBAB can also catalyze this transformation to give 3a in only 77% yield (entry 14, Table 1). Finally, the best yield of 3a (95%) was obtained from the reaction of 1a (1.5 mmol), 2a (1 mmol), TBAI (10 mol%), and 70% aqueous TBHP (3 equiv.) in isopropanol (5 mL) at 80 °C for 6 h (entry 5, Table 1).
With the optimal reaction conditions established, we then explored the substrate scope of this cascade reaction to construct pyrrolo[2,1-a]isoquinolines. As highlighted in Table 2, 1,4-naphthoquinone and a variety of N-substituted maleimides (2a–d) can react efficiently with 1a–c to give the corresponding products in good to excellent yields upon isolation. Encouraged by these results, we further examined the scope of structural variation in tetrahydroisoquinoline esters (methyl and tert-butyl) and allowed them to react with the dipolarophiles, without loss in the reaction efficiency. As shown, the reaction proceeded smoothly with all N-substituted maleimides regardless of bearing electron-neutral (phenyl), donating (p-methoxy phenyl) or with-drawing groups (p-fluoro phenyl). Among the tetrahydroisoquinoline esters, the reactivity was found to be in the order ethyl > methyl > tert-butyl. On the other hand, among the dipolarophiles, 1,4-naphthoquinone gave the best yields of pyrrolo[2,1-a]isoquinolines (3c, 3g and 3j; 96%, 94%, and 77%). The reactivity of the dipolarophile, N-(p-fluoro) phenyl maleimide (3b, 3e and 3i; 95%, 93%, and 78%) can be attributed to the electron withdrawing effect of the p-fluoro group. However, β-nitrostyrenes and acrylates were also used as dipolarophiles but did not react under the optimized reaction conditions.
Table 2 [3 + 2]Cycloaddition/oxidation/aromatization cascade reaction from 1 and 2a
Reaction conditions: 1 (1.5 mmol), 2 (1 mmol), TBHP 70 wt% in water (3 equiv.), TBAI (10 mol%), isopropanol (5 mL), 80 °C, 6 h.
35% aqueous H2O2 (3 equiv.) was used as oxidant.
|
|
With the successful reactivity of the tandem reaction on isoquinoline scaffolds, we then explored this method on tetrahydro-β-carbolines to give indolizino[8,7-b]indoles and the result was listed in Table 3. Interestingly, the target indolizino[8,7-b]indoles (5a–f) were obtained in moderate to good yields and the order of reactivities are similar to those observed in the case of pyrrolo[2,1-a]isoquinolines. Encouraged by these results, we applied our synthetic protocol to nitrogen heterocycles such as piperidine, indoline, pyrrolidine, and quinoline acetates (6a–d, Table 4). The reaction with 1,4-naphthoquinone was not successful in the formation of 7a and 7b except for 7c, albeit in only 5% yield. Interestingly, in the reaction with ethyl 2-(3,4-dihydroquinolin-1(2H)-yl)acetate (6d), the product obtained was pyrrolo[1,2-a]quinoline (7d) instead of dihydropyrrolo[1,2-a]quinoline, in 88% yield. Under the TBAI/TBHP oxidative conditions, the latter seems to have aromatized to the former compound. H2O2 is also employed as oxidant for the preparation of some of the representative examples such as pyrrolo[2,1-a]isoquinolines 3a, 3b, 3c (Table 2) and indolizino[8,7-b]indoles 5a and 5b (Table 3) as it is greener oxidant compared with TBHP.
Table 3 [3 + 2]Cycloaddition/oxidation/aromatization cascade reaction from 4 and 2a
Reaction conditions: 4 (1.5 mmol), 2 (1 mmol), TBHP 70 wt% in water (3 equiv.), TBAI (10 mol%), isopropanol (5 mL), 80 °C, 6 h.
35% aqueous H2O2 (3 equiv.) was used as oxidant.
|
|
Table 4 [3 + 2]Cycloaddition/oxidation/aromatization cascade reaction from 6 and 2a
Reaction conditions: 4 (1.5 mmol), 2 (1 mmol), TBHP 70 wt% in water (3 equiv.), TBAI (10 mol%), isopropanol (5 mL), 80 °C, 6 h.
No reaction.
Detected by GC-MS.
Isolated yield.
|
|
Several mechanistic considerations and kinetic studies led to the general acceptance of the [3 + 2]cycloaddition/oxidation/aromatization sequence proposed in Scheme 1.7 Several neutral zwitterionic and iminium intermediates are involved in the reaction of tertiary amines (A) with dipolarophiles (E) catalyzed by TBAI-TBHP. However, these neutral zwitterionic species (C) are expected to be in equilibrium with their protonated forms in protic solution. ESI-MS/MS seems to be the ideal technique to investigate the [3 + 2]cycloaddition reaction mechanism and catalytic cycle, since ESI could capture such ionic intermediates directly from solution to the gas phase for their unprecedented MS interception, mass identification, and MS/MS structural characterization.8 According to our interest in organic reaction mechanistic analysis by mass spectrometry (MS),9 which has greatly benefited from the development of electrospray ionization (ESI), herein, we describe the experiments aimed to intercept the cationic species resulting from the online monitoring of the reaction by using reactors coupled to ESI-MS in the positive-ion mode.
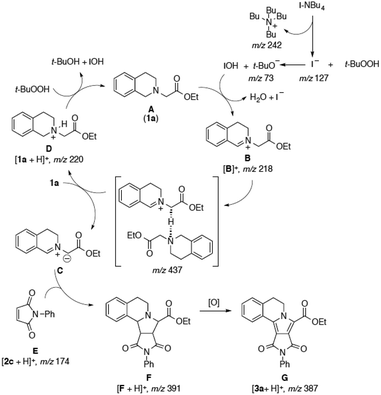 |
| Scheme 1 Proposed mechanism. | |
Initially, following our reaction protocol, the dipolarophile 2a (1.0 mmol) was added to a mixture of a tetrahydroisoquinoline acetate 1a (1.5 mmol), TBAI (10 mol%), and 70% aqueous TBHP (3.0 equiv.) in isopropanol (25.0 mL) under an air atmosphere. Then, the solution was online monitored through pressurized sample infusion (PSI) methodology,10 which allows us to screen the reaction solutions in real-time for 6 h at 80 °C under stirring. Initially, after 20 s to 2 h of reaction, three cationic species directly related to the proposed catalytic cycle can be observed (Scheme 1), which are detected as major ions (Fig. 2a): [1a + H]+ of m/z 220, the main iminium intermediate [B]+ of m/z 218, and [Bu4N]+ of m/z 242. Furthermore, the proton-bond dimer of 1a is also observed as [1a + H + 1a]+ (m/z 439). Following the reaction by ESI-MS in the negative ion-mode gives a clean spectrum of iodide ion of m/z 127. No other anionic species are observed in the ESI(−)-MS spectra. After 2 h of reaction, a proton-bond dimer of m/z 437 is observed (Fig. 2b), which was a key transient intermediate for the formation of zwitterionic species C in solution. The characterization of this species was performed by ESI-MS/MS experiments, which afforded as expected, two main fragment pathways producing intermediate D of m/z 220, and iminium ion [B]+ of m/z 218. Moreover, the intermediate [F + H]+ of m/z 391 (Fig. 2b) can be also observed in Fig. 2a. The fragmentation of the ion [F + H]+ of m/z 391 by CID experiments afforded the ion [F + H − CO]+ of m/z 363, and [F + H − 2 × CO − H2O]+ of m/z 317. Furthermore, the aromatization process can also be observed online as depicted in Fig. 2b, as the ion of m/z 389 starts to be produced and giving the final product of m/z 387 [3a + H]+ that is the protonated form of 3a.
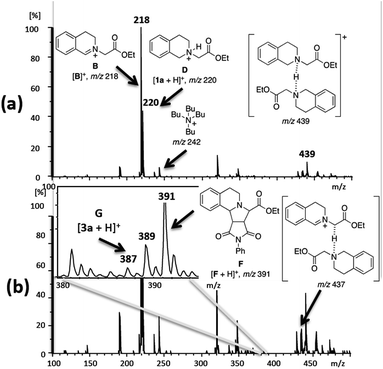 |
| Fig. 2 (a) Online ESI-MS screening of the reaction of the dipolarophile 2a (1.0 mmol), tetrahydroisoquinoline acetate 1a (1.5 mmol), TBAI (10 mol%), 70% aqueous TBHP (3.0 equiv.), and TBHP in isopropanol (25.0 mL) under an air atmosphere after 1:50 min. (b) Online ESI-MS spectra of the reaction after 2 h of experiments. | |
Experimental section
General information
All solvents were purified and dried using standard methods prior to use. Commercially available reagents were used without further purification. 1H NMR spectra were recorded on an NMR instrument operated at 500 MHz. Chemical shifts are reported in ppm with the solvent resonance as the internal standard (CDCl3: δ 7.26 ppm). 13C NMR spectra were recorded on an NMR instrument operated at 125 MHz with complete proton decoupling. Chemical shifts are reported in ppm with the solvent resonance as the internal standard (CDCl3: δ 77.16 ppm). The following abbreviations were used for 1H NMR spectra to indicate the signal multiplicity: s (singlet), d (doublet), t (triplet), q (quartet) and m (multiplet). MS and HRMS were measured in EI or ESI† mode and the mass analyzer of the HRMS was TOF. Thin layer chromatography was performed on pre-coated glass back plates and visualized with UV light at 254 nm.
Online monitoring experiments were carried out by overpressure in a Schlenk flask held at 2.0 psi (air) throughout the reaction and the temperature was controlled by an oil bath through and magnetic stirrer placed in the flask. The reaction mixture was transferred to ESI ion-source directly through PEEK tubing (200 mm in length, i.d. 0.055 mm). Mass spectra were recorded by Electrospray Ionization Mass Spectrometry (ESI-MS) and tandem mass (ESI-MS/MS), and the online mechanistic studies were carried out by using ESI(+)-MS(/MS) in a Bruker Daltonics AmaZon SLion trap equipment.
Typical reaction procedure.
The dipolarophiles 2a–d (1.0 mmol) were added to a mixture of a tetrahydroisoquinoline acetates 1a–c or tetrahydro-β-carboline acetates 4a–c (1.5 mmol), TBAI (10 mol%), 70% aqueous TBHP (3 equiv.), and TBHP in isopropanol (5.0 mL) under an air atmosphere. The solution was stirred for 6 h at 80 °C. The crude mixture was filtered and the precipitate washed with ethyl acetate followed by recrystallization from chloroform to obtain the desired products 3a–j and 5a–f.
Ethyl 9,11-dioxo-10-phenyl-6,9,10,11-tetrahydro-5H-pyrrolo[3′,4′:3,4]pyrrolo[2,1-a]isoquinoline-8-carboxylate (3a).
White solid (366 mg, 95%); m.p. 190–191 °C; 1H NMR (500 MHz, CDCl3): δ = 8.59 (d, J = 7.3 Hz, 1H), 7.48 (t, J = 7.7 Hz, 2H), 7.41–7.37 (m, 5H), 7.29 (d, J = 7.1 Hz, 1H), 4.78 (t, J = 6.8 Hz, 2H), 4.45–4.42 (m, 2H), 3.18 (t, J = 6.7 Hz, 2H), 1.46 (t, J = 7.0 Hz, 3H); 13C NMR (125 MHz, CDCl3): δ = 163.0, 161.5, 159.6, 133.4, 132.5, 132.3, 130.3, 128.9, 127.9, 127.9, 127.7, 127.6, 127.1, 125.5, 125.2, 118.6, 116.2, 61.6, 43.3, 28.3, 14.7; HRMS (ESI-TOF) m/z calculated for C23H18N2O4 [M + H]+ 387.1344, found 387.1350.
Ethyl 9,11-dioxo-10-(4-fluorophenyl)-6,9,10,11-tetrahydro-5H-pyrrolo[3′,4′:3,4] pyrrolo[2,1-a] isoquinoline-8-carboxylate (3b).
White solid (383 mg, 95%); m.p. 247–248 °C; 1H NMR (500 MHz, CDCl3): δ = 8.56 (d, J = 7.0 Hz, 1H), 7.43–7.36 (m, 4H), 7.30 (d, J = 7.0 Hz, 1H), 7.17 (t, J = 8.3 Hz, 2H), 4.77 (t, J = 6.8 Hz, 2H), 4.45–4.41 (m, 2H), 3.18 (t, J = 6.8 Hz, 2H), 1.46 (t, J = 7.17 Hz, 3H); 13C NMR (125 MHz, CDCl3): δ = 163.0, 161.5, 159.5, 133.5, 132.4, 130.4, 128.9, 128.8, 128.0, 127.9, 127.6, 125.4, 125.0, 118.7, 115.9, 61.6, 43.4, 28.3, 14.1; HRMS (ESI-TOF) m/z calculated for C23H17FN2O4 [M + H]+ 405.1250, found 405.1252.
Ethyl 9,14-dioxo-5,6,9,14-tetrahydrobenzo[5,6]isoindolo[1,2-a]isoquinoline-8-carboxylate (3c).
Yellow solid (356 mg, 96%); m.p. 144–145 °C; 1H NMR (500 MHz, CDCl3): δ = 9.01 (d, J = 7.9 Hz, 1H), 8.31 (d, J = 7.3 Hz, 1H), 8.22 (d, J = 7.3 Hz, 1H), 7.74–7.68 (m, 2H), 7.46 (t, J = 7.6 Hz, 1H), 7.38 (t, J = 7.3 Hz, 1H), 7.28 (d, J = 7.4 Hz, 1H), 4.57–4.54 (m, 2H), 4.30 (t, J = 6.5 Hz, 2H), 3.11 (t, J = 6.5 Hz, 2H), 1.50 (t, J = 7.1 Hz, 3H); 13C NMR (125 MHz, CDCl3): δ = 179.7, 179.4, 161.4, 135.6, 135.6, 134.7, 133.6, 133.2, 132.9, 130.0, 128.8, 127.4, 127.3, 127.2, 126.6, 126.3, 126.0, 123.2, 117.4, 62.4, 43.1, 29.1, 14.0; HRMS (ESI-TOF) m/z calculated for C23H17NO4 [M + H]+ 372.1235, found 372.1238.
Methyl 9,11-dioxo-10-phenyl-6,9,10,11-tetrahydro-5H-pyrrolo[3′,4′:3,4]pyrrolo[2,1-a]isoquinoline-8-carboxylate (3d).
Orange solid (342 mg, 92%); m.p. 217–218 °C; 1H NMR (500 MHz, CDCl3): δ = 8.59 (d, J = 8.2 Hz, 1H), 7.49 (t, J = 7.7 Hz, 2H), 7.42–7.36 (m, 5H), 7.30 (d, J = 6.8 Hz, 1H), 4.79 (t, J = 6.7 Hz, 2H), 3.98 (s, 3H), 3.20 (t, J = 6.8 Hz, 2H); 13C NMR (125 MHz, CDCl3): δ = 162.9, 161.6, 160.0, 133.6, 132.3, 130.3, 128.8, 128.0, 127.9, 127.7, 127.6, 127.0, 125.4, 125.3, 118.1, 116.2, 52.3, 43.4, 28.3; HRMS (ESI-TOF) m/z calculated for C22H16N2O4 [M + H]+ 373.1188, found 373.1187.
Methyl 9,11-dioxo-10-(4-fluorophenyl)-6,9,10,11-tetrahydro-5H-pyrrolo [3′,4′:3,4] pyrrolo[2,1-a] isoquinoline-8-carboxylate (3e).
Orange solid (362 mg, 93%); m.p. 226–227 °C; 1H NMR (500 MHz, CDCl3): δ = 8.56 (d, J = 7.4 Hz, 1H), 7.43–7.38 (m, 4H), 7.30 (d, J = 6.8 Hz, 1H), 7.16 (t, J = 9.1 Hz, 2H), 4.78 (t, J = 6.7 Hz, 2H), 3.99 (s, 3H), 3.19 (t, J = 6.8 Hz, 2H); 13C NMR (125 MHz, CDCl3): δ = 162.9, 161.5, 159.9, 133.7, 132.4, 130.4, 128.8, 128.7, 128.0, 127.9, 127.6, 125.4, 125.1, 118.2, 115.8, 115.7, 52.3, 43.4, 28.3; HRMS (ESI-TOF) m/z calculated for C22H15FN2O4 [M + H]+ 391.1094, found 391.1089.
Methyl 9,11-dioxo-10-(4-methoxyphenyl)-6,9,10,11-tetrahydro-5H-pyrrolo [3′,4′:3,4] pyrrolo[2,1-a] isoquinoline-8-carboxylate (3f).
White solid (333 mg, 83%); m.p. 192–193 °C; 1H NMR (500 MHz, CDCl3): δ = 8.58 (d, J = 7.3 Hz, 1H), 7.42–7.37 (m, 2H), 7.29 (t, J = 8.8 Hz, 3H), 7.0 (d, J = 8.8 Hz, 2H), 4.77 (t, J = 7.0 Hz, 2H), 3.98 (s, 3H), 3.84 (s, 3H), 3.18 (t, J = 6.8 Hz, 2H); 13C NMR (125 MHz, CDCl3): δ = 163.2, 161.9, 160.1, 158.9, 133.5, 132.3, 130.3, 128.3, 128.0, 127.9, 127.6, 125.5, 125.4, 125.2, 118.0, 116.3, 114.2, 55.4, 52.2, 43.4, 28.3; HRMS (ESI-TOF) m/z calculated for C23H18N2O5 [M + H]+ 403.1294, found 403.1301.
Methyl 9,14-dioxo-5,6,9,14-tetrahydrobenzo[5,6]isoindolo[1,2-a]isoquinoline-8-carboxylate (3g).
Orange solid (335 mg, 94%); m.p. 205–206 °C; 1H NMR (500 MHz, CDCl3): δ = 9.0 (d, J = 7.7 Hz, 1H), 8.31 (d, J = 8.6 Hz, 1H), 8.22 (d, J = 8.6 Hz, 1H), 7.73–7.70 (m, 2H), 7.46 (t, J = 7.6 Hz, 1H), 7.39 (t, J = 7.3 Hz, 1H), 7.29 (d, J = 7.4 Hz, 1H), 4.30 (t, J = 6.5 Hz, 2H), 4.07 (s, 3H), 3.11 (t, J = 6.5 Hz, 2H); 13C NMR (125 MHz, CDCl3): δ = 179.6, 179.6, 161.8, 135.8, 135.6, 134.6, 133.6, 133.3, 133.0, 130.1, 128.8, 127.4, 127.4, 127.2, 126.6, 126.3, 125.4, 123.4, 117.5, 53.1, 43.2, 29.1; HRMS (ESI-TOF) m/z calculated for C22H15NO4 [M + H]+ 358.1079, found 358.1076.
tert-Butyl 9,11-dioxo-10-phenyl-6,9,10,11-tetrahydro-5H-pyrrolo[3′,4′:3,4]pyrrolo[2,1-a]isoquinoline-8-carboxylate (3h).
Orange solid (302 mg, 73%); m.p. 234–235 °C; 1H NMR (500 MHz, CDCl3): δ = 8.59 (d, J = 7.3 Hz, 1H), 7.48 (t, J = 7.9 Hz, 2H), 7.40–7.35 (m, 5H), 7.29 (d, J = 7.1 Hz, 1H), 4.75 (t, J = 6.8 Hz, 2H), 3.17 (t, J = 6.7 Hz, 2H), 1.66 (s, 9H); 13C NMR (125 MHz, CDCl3): δ = 163.2, 161.5, 158.9, 133.0, 132.6, 132.3, 130.1, 128.9, 127.9, 127.9, 127.7, 127.5, 127.2, 125.6, 124.6, 120.1, 116.0, 83.4, 43.2, 28.4, 28.2; HRMS (ESI-TOF) m/z calculated for C25H22N2O4 [M + H]+ 415.1657, found 415.1660.
tert-Butyl 9,11-dioxo-10-(4-fluorophenyl)-6,9,10,11-tetrahydro-5H-pyrrolo [3′,4′:3,4] pyrrolo[2,1-a] isoquinoline-8-carboxylate (3i).
Yellow solid (336 mg, 78%); m.p. 209–210 °C; 1H NMR (500 MHz, CDCl3): δ = 8.56 (d, J = 7.1 Hz, 1H), 7.42–7.37 (m, 4H), 7.29 (d, J = 7.1 Hz, 1H), 7.16 (t, J = 8.5 Hz, 2H), 4.75 (t, J = 6.8 Hz, 2H), 3.17 (t, J = 6.7 Hz, 2H), 1.65 (s, 9H); 13C NMR (125 MHz, CDCl3): δ = 163.1, 161.5, 158.8, 133.1, 132.4, 130.2, 129.0, 128.9, 128.5, 128.5, 127.9, 127.8, 125.5, 124.4, 120.3, 115.9, 115.7, 83.4, 43.2, 28.3, 28.2; HRMS (ESI-TOF) m/z calculated for C25H21FN2O4 [M + H]+ 433.1558, found 433.1563.
tert-Butyl 9,14-dioxo-5,6,9,14-tetrahydrobenzo[5,6]isoindolo [1,2-a]isoquinoline-8-carboxylate (3j).
Orange solid (234 mg, 76%); m.p. 170–171 °C; 1H NMR (500 MHz, CDCl3): δ = 9.02 (d, J = 7.7 Hz, 1H), 8.31 (d, J = 6.8 Hz, 1H), 8.23 (d, J = 6.1 Hz, 1H), 7.73–7.70 (m, 2H), 7.45 (t, J = 7.4 Hz, 1H), 7.38 (t, J = 7.1 Hz, 1H), 7.28 (d, J = 7.4 Hz, 1H), 4.27 (t, J = 6.4 Hz, 2H), 3.11 (t, J = 6.4 Hz, 2H), 1.68 (s, 9H); 13C NMR (125 MHz, CDCl3): δ = 179.8, 179.3, 160.5, 135.7, 135.0, 134.8, 133.5, 133.1, 132.8, 129.9, 128.7, 127.7, 127.4, 127.2, 126.5, 122.4, 117.1, 84.1, 43.0, 29.1, 28.0; HRMS (ESI-TOF) m/z calculated for C25H21NO4 [M + H]+ 400.1548, found 400.1546.
Methyl 9,14-dioxo-5,9,14,15-tetrahydro-6H-naphtho[2′,3′:1,2]indolizino[8,7-b]indole-8-carboxylate (5a).
Red solid, yield (348 mg, 88%); m.p. 180–181 °C; 1H NMR (500 MHz, CDCl3): δ = 11.25 (s, 1H), 8.27 (d, J = 7.1 Hz, 1H), 8.20 (d, J = 7.0 Hz, 1H), 7.73–7.68 (m, 2H), 7.57 (d, J = 7.9 Hz, 1H), 7.52 (d, J = 8.0 Hz, 1H), 7.30 (t, J = 7.4 Hz, 1H), 7.16 (t, J = 7.3 Hz, 1H), 4.53 (t, J = 7.4 Hz, 2H), 4.08 (s, 3H), 3.29 (t, J = 7.3 Hz, 2H); 13C NMR (125 MHz, CDCl3): δ = 180.4, 178.6, 161.4, 136.5, 135.5, 134.5, 133.4, 133.1, 131.9, 127.2, 126.6, 126.4, 125.5, 124.8, 124.3, 122.4, 120.4, 119.0, 115.5, 112.4, 110.9, 53.0, 44.6, 20.4; HRMS (ESI-TOF) m/z calculated for C24H16N2O4 [M + H]+ 397.1188, found: 397.1185.
Ethyl 9,14-dioxo-5,9,14,15-tetrahydro-6H-naphtho[2′,3′:1,2]indolizino [8,7-b]indole-8-carboxylate (5b).
Red solid (377 mg, 92%); m.p. 208–209 °C; 1H NMR (500 MHz, CDCl3): δ = 11.28 (s, 1H), 8.29–8.28 (m, 1H), 8.24–8.22 (m, 1H), 7.74–7.69 (m, 2H), 7.58 (d, J = 7.9 Hz, 1H), 7.52 (d, J = 8.2 Hz, 1H), 7.30 (t, J = 8.0 Hz, 1H), 7.16 (t, J = 7.9 Hz, 1H), 4.58–4.52 (m, 4H), 3.30 (t, J = 7.6 Hz, 2H), 1.52 (t, J = 7.1 Hz, 3H); 13C NMR (125 MHz, CDCl3): δ = 180.4, 178.4, 161.0, 136.5, 135.5, 134.5, 133.3, 133.0, 131.7, 127.1, 127.0, 126.6, 125.5, 124.8, 124.3, 122.1, 120.3, 118.9, 115.4, 112.3, 110.7, 62.4, 44.5, 20.3, 14.0; HRMS (ESI-TOF) m/z calculated for C25H18N2O4 [M + H]+ 411.1344, found: 411.1334.
Ethyl 2-phenyl-1,3-dioxo-1,2,3,6,7,12-hexahydropyrrolo [3′,4′:1,2] indolizino[8,7-b]indole-4-carboxylate (5c).
Orange solid (293 mg, 69%); m.p. 269–270 °C; 1H NMR (500 MHz, CDCl3 + DMSO-d6): δ = 9.37 (s, 1H), 7.51 (d, J = 7.9 Hz, 1H), 7.40 (t, J = 8.0 Hz, 3H), 7.32–7.29 (m, 3H), 7.19 (t, J = 7.3 Hz, 1H), 7.08 (t, J = 7.6 Hz, 1H), 4.79 (t, J = 7.4 Hz, 2H), 4.36–4.32 (m, 2H), 3.24 (t, J = 7.3 Hz, 2H), 1.37 (t, J = 7.0 Hz, 3H); 13C NMR (125 MHz, CDCl3 + DMSO-d6): δ = 162.5, 160.7, 158.6, 137.1, 131.9, 128.1, 127.0, 126.5, 126.2, 124.8, 123.7, 123.5, 123.3, 119.9, 119.3, 118.6, 113.1, 111.6, 110.6, 60.8, 44.1, 20.1, 13.5; HRMS (ESI-TOF) m/z calculated for C25H19N3O4 [M + H]+ 426.1453, found: 426.1450.
Ethyl 2-(4-methoxy)phenyl-1,3-dioxo-1,2,3,6,7,12-hexahydropyrrolo[3′,4′:1,2]indolizino[8,7-b]indole-4-carboxylate (5d).
Yellow solid (304 mg, 67%); m.p. 202–203 °C; 1H NMR (500 MHz, CDCl3): δ = 9.06 (s, 1H), 7.60 (d, J = 7.7 Hz, 1H), 7.44 (d, J = 8.0 Hz, 1H), 7.32–7.28 (m, 3H), 7.18 (t, J = 7.4 Hz, 1H), 7.01 (d, J = 8.6 Hz, 2H), 4.88 (t, J = 7.3 Hz, 2H), 4.45–4.41 (m, 2H), 3.85 (s, 3H), 3.32 (t, J = 7.9 Hz, 2H), 1.46 (t, J = 7.1 Hz, 3H); 13C NMR (125 MHz, CDCl3): δ = 163.8, 161.9, 159.5, 159.0, 137.5, 128.2, 127.0, 125.5, 125.1, 124.5, 124.2, 124.0, 121.3, 120.7, 120.0, 119.3, 114.3, 113.9, 112.0, 111.1, 61.6, 55.4, 44.8, 20.9, 14.1; HRMS (ESI-TOF) m/z calculated for C26H21N3O5 [M + H]+ 456.1559, found 456.1564.
Ethyl 2-(4-fluoro)phenyl-1,3-dioxo-1,2,3,6,7,12-hexahydropyrrolo[3′,4′:1,2]indolizino[8,7-b]indole-4-carboxylate (5e).
Yellow solid (318 mg, 72%); m.p. 259–260 °C; 1H NMR (500 MHz, CDCl3): δ = 9.04 (s, 1H), 7.60 (d, J = 7.9 Hz, 1H), 7.44–7.38 (m, 3H), 7.30 (t, J = 7.3 Hz, 1H), 7.18 (t, J = 8.3 Hz, 3H), 4.88 (t, J = 7.1 Hz, 2H), 4.45–4.43 (m, 2H), 3.31 (t, J = 7.3 Hz, 2H), 1.47 (t, J = 7.0 Hz, 3H); 13C NMR (125 MHz, CDCl3): δ = 163.5, 162.7, 161.4, 159.4, 137.6, 128.7, 128.6, 125.5, 124.6, 124.1, 123.7, 120.8, 120.2, 119.4, 116.0, 115.8, 113.7, 112.0, 111.3, 61.7, 44.8, 20.8, 14.1; HRMS (ESI-TOF) m/z calculated for C25H18FN3O4 [M + H]+ 444.1359, found 444.1359.
tert-Butyl 9,14-dioxo-5,9,14,15-tetrahydro-6H-naphtho[2′,3′:1,2]indolizino[8,7-b]indole-8-carboxylate (5f).
Red solid (275 mg, 63%); m.p. 199–200 °C; 1H NMR (500 MHz, CDCl3): δ = 11.26 (s, 1H), 8.28 (d, J = 8.8 Hz, 1H), 8.23 (d, J = 8.8 Hz, 1H), 7.73–7.69 (m, 2H), 7.56 (d, J = 7.9 Hz, 1H), 7.52 (d, J = 8.2 Hz, 1H), 7.28 (t, J = 7.7 Hz, 1H), 7.15 (t, J = 7.6 Hz, 1H), 4.48 (t, J = 7.4 Hz, 2H), 3.29 (t, J = 7.3 Hz, 2H), 1.72 (s, 9H); 13C NMR (125 MHz, CDCl3): δ = 180.5, 178.3, 160.2, 136.4, 135.7, 134.7, 133.3, 132.9, 131.1, 128.7, 127.1, 126.6, 125.6, 125.0, 124.2, 121.3, 120.3, 118.9, 115.1, 112.3, 110.5, 84.1, 44.3, 28.0, 20.4; HRMS (ESI-TOF) m/z calculated for C27H22N2O4 [M + H]+ 439.1657, found 439.1653.
Ethyl 7,12-dioxo-7,12-dihydrobenzo[5,6]isoindolo[2,1-a]quinoline-13-carboxylate (7d).
Yellow solid (324 mg, 88%); m.p. 179–180 °C; 1H NMR (500 MHz, CDCl3): δ = 8.42 (d, J = 9.3 Hz, 1H), 8.30 (d, J = 7.6 Hz, 1H), 8.26–8.19 (m, 1H), 7.94 (d, J = 8.5 Hz, 1H), 7.83 (d, J = 7.7 Hz, 1H), 7.78–7.69 (m, 2H), 7.62 (t, J = 7.6 Hz, 2H), 7.54 (t, J = 7.7 Hz, 1H), 4.75–4.70 (m, 2H), 1.55 (t, J = 7.1 Hz, 3H); 13C NMR (125 MHz, CDCl3): δ = 180.5, 179.5, 163.8, 135.9, 134.8, 134.0, 133.7, 132.9, 132.6, 129.6, 129.6, 128.4, 126.9, 126.7, 126.4, 125.8, 123.6, 121.3, 118.6, 116.6, 111.9, 63.3, 13.9; HRMS (ESI-TOF) m/z calculated for C23H25NO4 [M + H]+ 370.1079, found 370.1083.
Conclusions
In summary, we have developed an environmental friendly tandem [3 + 2]cycloaddition/oxidation/aromatization sequence of tetrahydroisoquinoline and tetrahydro-β-carboline acetates in a green solvent. This novel protocol provides a rapid and efficient access to biologically important pyrrolo[2,1-a]isoquinolines and indolizino[8,7-b]indoles in a highly concise fashion. Furthermore, for the first time, key intermediates related to proposed mechanism of this tandem reaction were identified and characterized by online monitoring of reactions through ESI-MS(/MS) experiments. With TBHP as the “green” oxidant, cheap, low toxic TBAI as the catalyst, and isopropanol as the green solvent, this protocol would be more attractive in the field of green chemistry.
Acknowledgements
SN, NPK, and PS are thankful to DoP, Ministry of Chemicals and Fertilizers Govt. of India, New Delhi for the award of Research Fellowship. FMN and LSS acknowledge InnovaChile CORFO Code FCR-CSB09CEII-6991 and Anillo Cientifico FONDECYT ACT-1107 for supporting this research activity. FMN thanks FONDECYT-Iniciacion (11130086). LSS thanks additional support from PIEI (Quimica y Bio-organica en Recursos Naturales) Universidad de Talca.
Notes and references
-
(a) H. Fan, J. Peng, M. T. Hamann and J. F. Hu, Chem. Rev., 2007, 108, 264–287 CrossRef;
(b) D. Pla, F. Albericio and M. Alvarez, MedChemComm, 2011, 2, 689–697 RSC;
(c) B. T. Fukuda, F. Ishibashi and M. Iwaob, Heterocycles, 2011, 83, 491–529 CrossRef;
(d) S. T. Handy and Y. Zhang, Org. Prep. Proced. Int., 2005, 37, 411–445 CrossRef CAS;
(e) F. A. M. A. D. Pla, Anti-Cancer Agents Med. Chem., 2008, 2008, 746–760 CrossRef.
-
(a) D. M. Roll, C. M. Ireland, H. S. M. Lu and J. Clardy, J. Org. Chem., 1988, 53, 3276–3278 CrossRef;
(b) N. L. Segraves, S. J. Robinson, D. Garcia, S. A. Said, X. Fu, F. J. Schmitz, H. Pietraszkiewicz, F. A. Valeriote and P. J. Crews, J. Nat. Prod., 2004, 67, 783–792 CrossRef CAS;
(c) T. S. Kam and K. M. Sim, Phytochemistry, 1998, 47, 145–147 CrossRef CAS;
(d) A. M. Popov and V. A. Stonik, Antibiot. Khimioter., 1991, 36, 12–14 CAS;
(e) A. Hormann, B. Chaudhuri and H. Fretz, Bioorg. Med. Chem., 2001, 9, 917–921 CrossRef CAS;
(f)
D. R. Herbert, German Patent 2,033,631, 1971Chemical abstracts, 1972, 76, 113061h.
-
(a) C. Yu, Y. Zhang, S. Zhang, H. Li and W. Wang, Chem. Commun., 2011, 47, 1036–1038 RSC;
(b) Y. Q. Zou, L. Q. Lu, L. Fu, N. J. Chang, J. Rong, J. R. Chen and W.-J. Xiao, Angew. Chem., Int. Ed., 2011, 50, 7171–7175 CrossRef CAS;
(c) M. Rueping, D. Leonori and T. Poisson, Chem. Commun., 2011, 47, 9615–9617 RSC;
(d) L. Huang and J. Zhao, Chem. Commun., 2013, 49, 3751–3753 RSC;
(e) S. Guo, H. Zhang, L. Huang, Z. Guo, G. Xiong and J. Zhao, Chem. Commun., 2013, 49, 8689–8691 RSC;
(f) H. M. Huang, Y. J. Li, Q. Ye, W. B. Yu, L. Han, J. H. Jia and J. R. Gao, J. Org. Chem., 2014, 79, 1084–1092 CrossRef CAS;
(g) H. M. Huang, F. Huang, Y. J. Li, J. H. Jia, Q. Ye, L. Han and J. R. Gao, RSC Adv., 2014, 4, 27250–27258 RSC.
-
(a) M. Jida, O. M. Soueidan, B. Deprez, G. Lacondea and R. Deprez-Poulain, Green Chem., 2012, 14, 909–911 RSC;
(b) Z. S. Nurmaganbetov, E. E. Shultz, S. V. Chernov, A. Z. Turmukhambetov, R. B. Seydakhmetova, M. M. Shakirov, G. A. Tolstikov and S. M. Adekenov, Chem. Heterocycl. Compd., 2011, 46, 1494–1499 CrossRef CAS;
(c) H. Waldmann, L. Eberhardt, K. Wittstein and K. Kumar, Chem. Commun., 2010, 46, 4622–4624 RSC;
(d) S. U. Dighe, S. Hutait and S. Batra, ACS Comb. Sci., 2012, 14, 665–672 CrossRef CAS.
- X. F. Wu, J. L. Gong and X. Qi, Org. Biomol. Chem., 2014, 12, 5807–5817 RSC.
-
(a) W. A. Silva, M. T. Rodrigues, N. Shankaraiah, R. B. Ferreira, C. K. Z. Andrade, R. A. Pilli and L. S. Santos, Org. Lett., 2009, 11, 3238–3241 CrossRef;
(b) A. Kamal, M. Sathish, V. L. Nayak, V. Srinivasulu, B. Kavitha, Y. Tangella, D. Thummuri, C. Bagul, N. Shankaraiah and N. Nagesh, Bioorg. Med. Chem., 2015, 23, 5511–5526 CrossRef CAS;
(c) N. Shankaraiah, K. P. Siraj, S. Nekkanti, V. Srinivasulu, M. Satish, P. Sharma, K. R. Senwar, M. V. P. S. Vishnuvardhan, S. Ramakrishna and A. Kamal, Bioorg. Chem., 2015, 59, 130–139 CrossRef CAS;
(d) N. Shankaraiah, S. Nekkanti, K. J. Chudasama, K. R. Senwar, P. Sharma, M. K. Jeengar, V. G. M. Naidu, V. Srinivasulu and A. Kamal, Bioorg. Med. Chem. Lett., 2014, 24, 5413–5417 CrossRef CAS PubMed;
(e) A. Kamal, V. Srinivasulu, V. L. Nayak, M. Sathish, N. Shankaraiah, C. Bagul, N. V. S. Reddy, N. Rangaraj and N. Nagesh, ChemMedChem, 2014, 9, 2084–2098 CrossRef CAS;
(f) A. Kamal, M. K. Reddy, T. S. Reddy, L. S. Santos and N. Shankaraiah, Synlett, 2011, 61–64 CrossRef CAS.
-
(a) J. Barluenga, M. Marco-Arias, F. Gonzalez-Bobes, A. Ballesteros and J. M. Gonzalez, Chem. Commun., 2004, 2616–2617 RSC;
(b) T. Nobuta, N. Tada, A. Fujiya, A. Kariya, T. Miura and A. Itoh, Org. Lett., 2013, 15, 574–577 CrossRef CAS;
(c) H.-M. Huang, F. Huang, Y.-J. Li, J.-H. Jia, Q. Ye, L. Hana and J.-R. Gao, RSC Adv., 2014, 4, 27250–27258 RSC.
-
(a) L. S. Santos and J. O. Metzger, Angew. Chem., Int. Ed., 2006, 45, 977–981 CrossRef;
(b) L. S. Santos, L. Knaack and J. O. Metzger, Int. J. Mass Spectrom., 2005, 246, 84–104 CrossRef CAS;
(c) B. A. DaSilveira, L. S. Santos, F. M. Nachtigall, M. N. Eberlin and J. Dupont, Angew. Chem., Int. Ed., 2006, 45, 7251–7254 CrossRef.
-
(a) N. Shankaraiah, N. Markandeya, V. Srinivasulu, K. Sreekanth, C. S. Reddy, L. S. Santos and A. Kamal, J. Org. Chem., 2011, 76, 7017–7026 CrossRef CAS;
(b) A. Kamal, N. Markandeya, N. Shankaraiah, C. R. Reddy, S. Prabhakar, C. S. Reddy, M. N. Eberlin and L. S. Santos, Chem.–Eur. J., 2009, 15, 7215–7224 CrossRef CAS;
(c) L. S. Santos, Eur. J. Org. Chem., 2008, 235–253 CrossRef CAS;
(d)
L. S. Santos, in Reactive intermediates: MS investigations in solution, Wiley-VCH, Weinheim, 2012 Search PubMed.
- K. L. Vikse, M. P. Woods and J. S. McIndoe, Organometallics, 2010, 29, 6615–6618 CrossRef CAS.
Footnote |
† Electronic supplementary information (ESI) available: 1H and 13C NMR. See DOI: 10.1039/c5ra24629f |
|
This journal is © The Royal Society of Chemistry 2016 |
Click here to see how this site uses Cookies. View our privacy policy here.