DOI:
10.1039/D4VA00373J
(Paper)
Environ. Sci.: Adv., 2025, Advance Article
Ecotoxicity and chemical characterization of tropical soil under different periods of exposure to cattle slaughterhouse effluent
Received
31st October 2024
, Accepted 24th February 2025
First published on 17th March 2025
Abstract
This study aimed to evaluate the toxicity of effluent obtained from a bovine slaughterhouse on the reproductive parameters of the springtail Folsomia candida and on the chemical properties of natural tropical soil with different application ages. The soils used for the chemical and ecotoxicological characterization tests were collected from the Mombaça grass pasture area of Chácara Malu, Gurupi, Tocantins, which belongs to the city's cattle slaughterhouse. Four pastures were subjected to four different treatments: effluent application for 5, 10, and 15 years and no effluent application (control). Soil samples were collected from three layers of depths: 0–10, 10–20, and 20–30 cm. The experiments were performed in accordance with the ABNT NBR ISO 11267/2019 standard. Our results demonstrated that bovine slaughterhouse effluent can be used as an alternative water source and can supply macro- and micronutrients for pasture production. Additionally, the application of cattle slaughterhouse effluent for 5, 10, and 15 years remedied soil acidity, thereby increasing the pH and macro- and micronutrient contents in the soil. Furthermore, effluent treatment altered the soil's chemical properties, which in turn affected the reproduction of the springtail F. candida. Taken together, these results may facilitate the development of strategies that promote sustainable agricultural production by converting animal residues into properly managed fertilizers.
Environmental significance
This study highlights the potential of utilizing effluent from bovine slaughterhouses as a sustainable resource for pasture management. By demonstrating that such effluent can enhance soil chemical properties—specifically enriching macro- and micronutrients—it presents a viable alternative to conventional fertilizers, thereby reducing environmental impact. The positive effects on soil health and fertility, alongside the observed alterations in the reproduction of the springtail Folsomia candida, underscore the importance of responsible waste management practices. Ultimately, this research supports the transition towards more sustainable agricultural practices that effectively recycle animal by-products, contributing to ecosystem health and productivity while mitigating the environmental risks associated with livestock operations.
|
Introduction
Global meat consumption has increased by approximately 250 million metric tons in recent years.1 Disposal of slaughterhouse waste is an economic and environmental challenge worldwide, particularly in developing countries.2 Despite the nutritional value of bovine blood and rumen digesta, there are concerns regarding the possible presence of pathogens in these products, leading to their underutilization as food components.3,4 As these products can promote plant growth owing to nutrient concentrations in their composition, they can also be used as organic fertilizers.5
Sankar et al. (2022)6 developed organic briquettes using bovine blood and rumen digestive content as raw materials and employed them in seasonal okra production. They observed a considerable increase in nutrients in the soil between 3 and 4 months after planting, leading to better plant growth and fruit production. Bhunia et al. (2021)7 used bovine blood and rumen digesta in a 3
:
1 ratio for the successive cultivation of pepper and amaranth in India and found that this additive enhanced the growth as well as yield of pepper and amaranth compared with conventional chemical supplementation. Matheyarasu et al. (2016)8 evaluated how irrigation using slaughterhouse wastewater affects plant growth and development and observed that the nutrients present in the wastewater significantly increased the biomass yield and plant height of all four crops tested.
The balanced application of organic fertilizers rich in organic matter, humus, and beneficial microorganisms can have a positive influence on agroecosystem health—it promotes the stability of soil aggregates, renewal of soil organic matter (SOM), increase in soil fertility, and replacement of chemical fertilizers.9–11 Organic fertilizers provide essential micronutrients such as manganese, boron, zinc, copper, and iron, along with nitrogen, phosphorus, and potassium,9,12 whereas chemical fertilizers mainly provide ammonia, nitrate, phosphate, and potassium in the form of salts. In addition to incurring high costs for farmers, most inorganic fertilizers are persistent in nature and can cause diffuse water pollution through nutrient runoff.13
Although the use of animal-derived slaughterhouse waste as organic fertilizers has its advantages, its application remains challenging owing to the presence of pathogens, heavy metals, and organic contaminants in improperly processed waste.14 To determine the environmental effects of applying these residues to soil, an ecotoxicological testing strategy combined with other chemical and biological analyses is necessary. Tourinho et al. (2012)15 reported that it is essential to study soils that serve as sinks for most environmental contaminants after the application of sewage sludge or other types of effluent. Furthermore, there is little or no information regarding the possible toxicity of cattle slaughterhouse waste to non-target organisms present in soil and the perception of soil fertility in a tropical area.
Springtails comprise an order of small arthropods and are one of the most abundant groups on the planet.16 They are important bioindicators of soil quality, as they respond to various environmental changes, including chemical changes such as pH,17 structural and microclimate changes,18 and modification of the frequency and diversity of species according to the quantity and quality of litter available.17,19 Thus, members of this order are commonly studied to assess the quality of contaminated soils.20,21
Folsomia candida is the best representative springtail species for evaluating soil quality22,23 and is recommended for soil testing by ISO protocols.24 Thus, evaluating Collembola species' response to soils subjected to different organic compounds, especially for natural soils, can be an effective approach to generate a more realistic understanding of effluent toxicity in soils. In summary, the increase in soil organic matter and nutrients can lead to changes in the structures of the microbial community and be reflected in the abundance, composition and body size of springtails.25
The study of the behavior of F. candida as a bioindicator of soil quality in soils fertigated with wastewater from cattle slaughterhouses is important to investigate the possible impacts of its application in the long term, with a focus on biosafety. This evaluation allows adding value to an industrial effluent that is generated in large quantities worldwide, impacting the reduction of the sector's water demand and contributing to sustainable agricultural production.
Therefore, this study aimed to evaluate the possible toxic effects of cattle slaughterhouse effluent on the reproductive parameters of the collembolan species F. candida, analyse the chemical properties of natural tropical soil with different application ages (5, 10, and 15 years) and provide recommendations for sustainable agricultural practices.
Experimental
Study location
This study was conducted at the Federal University of Tocantins, Gurupi University Campus (11°44′44.16′′S, 49°03′04.17′′N; altitude, 280 m) located in the south of the state of Tocantins, Brazil (Fig. 1). According to the Köeppen classification,26 the regional climate is B1wA‘a’ humid type with moderate water deficiency, an average annual temperature of 29.5 °C, and an average annual precipitation of 1804 mm.
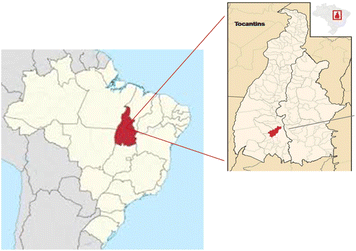 |
| Fig. 1 Study location – area in the south of the state of Tocantins, Brazil. | |
Characterization of slaughterhouse wastewater
The effluent used in this study was obtained from a small cattle slaughterhouse with an average slaughter capacity of 1200 heads of cattle per month. The generated effluent was first subjected to a primary treatment system consisting of a grease separation box and a primary decanter. Subsequently, a secondary treatment comprising primary (depth, 2.5 m; area, 1068 m2) and secondary facultative ponds (depth, 2.0 m; area, 711 m2) was applied. The collected samples were analysed in duplicate and in accordance with the Standard Methods for the Examination of Water and Wastewater.27 A high sensitivity atomic emission spectrometer with microwave plasma (Agilent 4210 MP-AES) was used for elemental quantification of the sample. Table 1 presents the physicochemical characteristics of the cattle slaughterhouse effluent used in this study to irrigate pastures over 15 years.
Table 1 Physicochemical and microbiological characterization of cattle slaughterhouse effluent used for pasture irrigationa
|
Cattle slaughterhouse wastewater |
COD = chemical oxygen demand; OD = dissolved oxygen; CFU = colony forming unit. |
pH |
7.21 |
Temperature (°C) |
26.8 |
COD (mg L−1) |
480 |
DO (mg L−1) |
3.55 |
Conductivity (μS cm−1) |
914.5 |
Salinity (mg L−1) |
435 |
Turbidity (mg L−1) |
60.6 |
Real color (μC) |
386 |
Sodium (mg L−1) |
141.11 |
Potassium (mg L−1) |
31.3 |
Magnesium (mg L−1) |
9.86 |
Calcium (mg L−1) |
62.11 |
Molybdenum (mg L−1) |
0.01 |
Phosphorus (mg L−1) |
15.54 |
Zinc (mg L−1) |
0.16 |
Iron (mg L−1) |
0.32 |
Copper (mg L−1) |
0.01 |
Nickel (mg L−1) |
0.01 |
Manganese (mg L−1) |
0.23 |
E. coli (CFU 100 mL−1) |
1350 |
Total coliforms (CFU 100 mL−1) |
8000 |
Contaminated site assessment and soil sample collection
Wastewater (effluent) obtained from the cattle slaughterhouse was applied in areas located in Gurupi, Tocantins (11°42′04′′S, 49°03′03′′W; altitude, 285 m) for different time periods. The soil in the area was classified as dystrophic argiluvic Plintosol, cultivated extensively with Mombaça grass. The experiment was conducted using a completely randomized design (CRD), with treatments assigned based on a 4 × 3 factorial scheme. The first factor involved four different durations of cattle slaughterhouse effluent application, while the second factor involved three soil depths. The area of the pasture used as control was 1.08 ha (T0) and those of the pastures with 5, 10, and 15 years of effluent application were 5.77 (T5), 1.26 (T10), and 2.62 ha (T15), respectively. The study area is represented in Fig. 2.
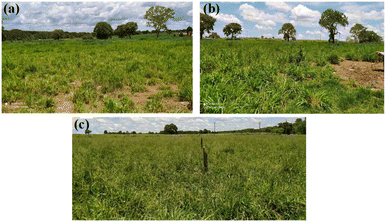 |
| Fig. 2 Mombaça grass pasture area, located in Gurupi, Tocantins, Brazil, fertigated for (a) 5 years (T5), (b) 10 years (T10) and (c) 15 years (T15). | |
Approximately 50 m3 ha−1 of effluent was applied weekly through sprinkler irrigation. For chemical and ecotoxicological characterization, soil samples were collected from these four pastures from depths of 0–10, 10–20 and 20–30 cm. Subsequently, the chemical characteristics of the soil were determined in the laboratory, according to the methodology described by Embrapa (2017).28 The soil samples were collected in 2022, in the month of November (spring).
Collembolan reproduction test (F. candida)
For this experiment, soil samples were collected from the four areas that received effluent treatment for different time periods: T0, no effluent application; T5, 5 years of effluent application; T10, 10 years of effluent application; and T15, 15 years of effluent application. In addition to these treatments, a standard soil sample (tropical artificial soil [TAS]) was prepared from a mixture of 75% washed fine sand, 20% kaolin, and 5% coconut fiber powder in order to compare the experimental results with the known results of a control group. To remove other organisms that could have been present in the collected soil, the soil samples were subjected to three cycles of freezing and thawing, sieved to 2 mm, and subsequently dried in an oven for 24 h at 50 °C.
Test organisms. Individuals of Collembola F. candida were cultivated on a substrate of gypsum and activated charcoal (9
:
1) and fed with biological yeast (Saccharomyces cerevisiae) three times a week. The culture containers were moistened whenever necessary and were kept in an incubator chamber at a controlled temperature of 20 ± 2 °C and a photoperiod of 16
:
8 light
:
dark.
Experimental design. The tests were performed in accordance with the ABNT NBR ISO 11267/2019 standard.29 Six replicates were used for each treatment, which contained 30 g of the treated and moistened soil. The soil was moistened with distilled water, considering the limit of 40–60% of its water retention capacity (WRC) according to the standard.In each replicate, 10 individuals of F. candida aged between 10 and 12 days were added. The organisms were fed S. cerevisiae weekly, and the humidity in the flasks was changed whenever necessary. The flasks were then stored in an incubator chamber (BOD) under the same cultivation conditions (controlled temperature of 20 ± 2 °C and photoperiod of 16
:
8 light
:
dark) for 28 days. Soil pH was measured at the beginning and end of the test using 1 mol L−1 KCl in a 1
:
5 ratio (soil
:
1 mol L−1 KCl).
To complete the experiment, after 28 days, tap water, dye, and water base were added to the soil samples, and the mixtures were transferred to larger containers to photograph the individuals. The observed individuals were counted using ImageJ, free software for counting organisms.
Statistical analysis
The results were analysed by analysis of variance (ANOVA) and the means were compared by Tukey's test (p ≤ 0.05), with comparisons in the breakdown of factors when there was a significant interaction. Principal component analysis (PCA) was used to reduce the dimensionality of the analysed characteristics according to treatment. Statistical analyses were conducted using R software with the packages Expdes.pt30 and FactoMineR,31 and the graphs were plotted using SigmaPlot software version 14.5.32
Results and discussion
Soil chemical characterization
The application of cattle slaughterhouse effluent over the years resulted in a significant increase in soil nutrients (Table 2). Compared with no treatment, effluent use in the pastures over the years caused a significant increase in soil pH, leading to a reduction in soil acidity. This was attributed to the pH of the effluent, which was approximately 7.2 (Table 1). The increase in soil pH is associated with the addition of ions from wastewater and the supply of organic matter, which undergoes decarboxylation and deamination during decomposition, releasing HO−.33 Based on our results, the application of cattle slaughterhouse wastewater for 5–15 years as a soil fertilizer remedied soil acidity, promoting an increase in pH and macro- and micronutrient contents in the soil.
Table 2 Chemical characterization of tropical soils subjected to the application of cattle slaughterhouse effluent for 15 years, in Gurupi-TO, Brazil, 2023a
Means followed by the same lowercase letters in the lines do not differ from each other at the 5% level using the Tukey test. OM = organic matter, SB = sum of exchangeable bases, CEC = cation exchange capacity and V = % of saturation per base. |
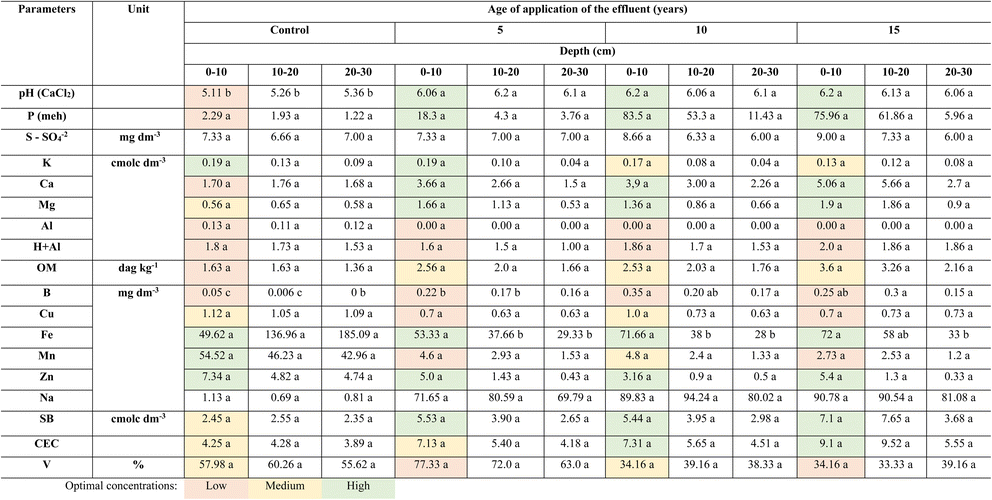 |
Determining the pH of soils is crucial because this parameter enables the analysis of the behaviour of nutrients present in the soil (both original and added nutrients) and their assimilation by plants. Table 2 shows that the concentration of some nutrients exhibited an increasing trend with irrigation time, whereas others showed a decreasing trend. According to De Souza et al. (2023),34 this change can be explained in terms of pH, as nutrients such as K, Ca, Mg, N, S, B, and P become less available at low pH values. In contrast, the concentrations of Fe, Cu, Mn, and Zn can be reduced by 100 times for each unit of increase in pH.35 The high concentration of organic matter and high moisture in the soil promote the reduction of MnO2 (insoluble) to Mn2+ (soluble) by anaerobic bacteria;36 a similar behaviour is exhibited by iron.
The content of the micronutrient boron was 77–85% higher in the different effluent-treated soils than in the soil without effluent application. Compared with the control samples, the treated soil samples showed an increase in the Fe content, but only in the most superficial layers, possibly due to its accumulation in this region. No significant interactions were observed between the other parameters (P, S, K, Ca, Mg, Al, organic matter, Cu, Mn, Zn, Na, sum of exchangeable bases (SB), cation exchange capacity (CEC), and % of saturation per base [V]), despite exhibiting an increasing trend over the years of application. This effect may be related to the concentrations of these elements in the effluent (Table 1). Organic fertilization may be an appropriate approach for improving soil fertility and increasing SOM content.
Alnaimy et al. (2021)37 recently reported that the use of wastewater in irrigation for up to 30 years improves most of the soil properties (organic matter, CEC, and available N, P, and K). The correlation between a variable and a principal component (PC) was used as the coordinate of the variable in the PC. The representation of variables differs from the graph of observations; observations are represented by their projections and correlations in colours.38 Fig. 3 shows the possible correlations between the soil chemical properties at different fertilization ages.
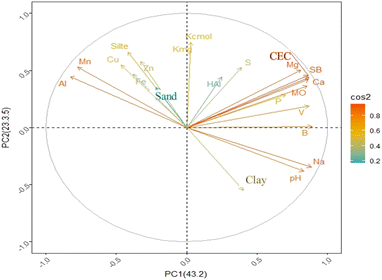 |
| Fig. 3 Temperature circle and correlation of variable contributions to PCs. | |
The cos2 values were used to estimate the quality of the representation. The closer the variable was to the correlation circle, the more the variable in the temperature graph appeared in reddish tones. This improved the representation in the factor map (and more importantly, the variable in the components). Closed variables at the center of the plot, represented by bluish tones, were less important for the first component. Thus, pH, Na, Ca, Mg, Mn, Al, Mo, and B were the variables that contributed the most to the formation of PC1 and PC2, whereas H + Al contributed the least. A multivariate PCA biplot (Fig. 4) was constructed to better explore the PCA results. The treatments that showed the highest dissimilarity and Euclidean distance were the natural soil without effluent (0 years) at the three depths in relation to the other soils (5, 10, and 15 years) at the same depths.
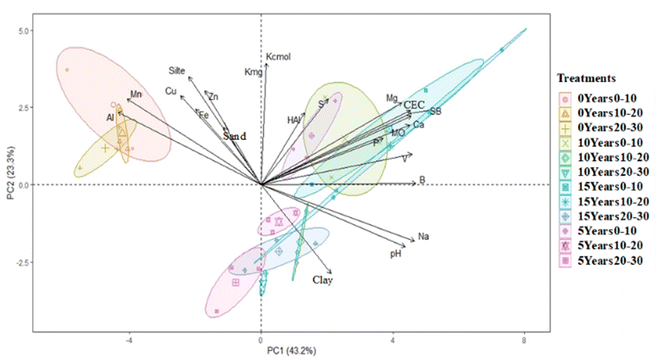 |
| Fig. 4 Biplot of the multivariate analysis of principal components (PCA) performed on the chemical characteristics of the soil and their possible correlations across different years and depths of fertilization with cattle slaughterhouse effluent. The arrows represent the correlation coefficient. | |
According to the multivariate PCA, the contents of Al and Mn were positively correlated and changed in the natural soil without effluent, whereas these elements were influenced less in soils of all ages that received treatment (5, 10, and 15 years) (Fig. 4). This demonstrates that the application of the effluent over the years reduced the influence on Al and Mn, as shown in Table 2. Parameters such as organic matter, CEC, SB, Ca, Mg, B, and P were positively influenced by the application of effluent to the soil at 10 and 15 years of age and at all depths (0–10, 10–20, and 20–30 cm). Both pH and Na were found to be influenced. An increase in Na in the soil was observed at all three depths, but mainly in the 0–10 cm layer. Na accumulation can cause the dispersion of soil particles through interactions with clay particles, thus altering the physical properties of the soil.39,40 Dispersal, when combined with swelling and dieback, can harm plants by reducing water and air permeability, causing waterlogging and inhibiting root penetration.41 If high concentrations of monovalent cations such as Na and K are present in soils, divalent cations such as Ca and Mg, which are stabilizing factors in the soil structure, can be displaced from the surface of clay particles.39,42
Collembolan reproduction test
The pH of all treatments varied between 5.5 and 6.5, which has been established as an optimal pH range for the test organisms. According to ANOVA, all treatment groups were significantly positively different from the TAS group, with the T0 condition (no effluent application) presenting the highest number of juveniles produced by exposed adults (1.5 times higher than TAS). The other treatments (T5, T10, and T15) showed no statistical differences between them, but higher fecundity was observed compared with TAS (Fig. 5). Compared with T0, the other treatments were also statistically different, presenting 16% fewer juveniles on average (Fig. 5). Compared with TAS, the natural soil provided more favourable conditions for springtail development. Domene et al. (2011)43 evaluated the effects of soil properties on the development of F. candida and observed that species reproduction can be negatively influenced in soils with higher CEC and higher silt and fine sand contents. Thus, we inferred that the lower reproduction rate of F. candida in TAS observed in this study was due to the finer texture of TAS compared with natural soils. Soils with a finer texture constitute a less favorable environment for the colonization of collembolans, mainly due to their physical structure. Because they are more compact, there is reduced mobility and greater difficulty for these organisms to penetrate, making them less suitable for reproduction.43
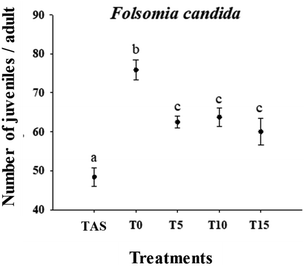 |
| Fig. 5 Individual effects of cattle slaughterhouse effluent on the reproduction of the springtail F. candida exposed to four ages of application in tropical soil (0, 5, 10 and 15 years). | |
In the natural soils T0, T5, T10, and T15, the effects observed on springtail reproduction may be related to the increase in pH and CEC over the years of treatment. High CEC values can induce metal accumulation in springtails and affect their reproduction.44 Although the pH values of the soils were in the optimum range for F. candida reproduction (5.4–6.6), we observed that the increase in pH of the other soils in relation to the control (T0) (Table 2) reduced the number of juveniles. Greenslade & Vaughan (2003)45 compared the survival and reproduction of Collembola species in artificial soils and found that the reproduction rates were higher for most of the studied species at pH 5.4 than at 6.62; the number of juveniles declined at pH 6.62. Changes in pH can affect tissues such as the gut and the cuticle, as well as gene expression in this invertebrate.46
One consequence of the reduction in the number of F. candida is the change in the decomposition rates of organic matter, which impacts the nutrient cycling carried out by these organisms.47
From our results, it is evident that F. candida, a bioindicator of soil quality, is sensitive to environmental changes in areas subjected to effluent application. The application of cattle slaughterhouse effluents promoted changes in the chemical properties of the soil. Variability in soil characteristics demonstrates that ecotoxicity may be related to factors such as texture, soil pH, and CEC, as these factors affect the bioavailability of chemical products.48
Conclusions
The application of cattle slaughterhouse effluent for 5, 10, and 15 years functioned as a remedy for soil acidity and consequently increased the pH and macro-and micronutrient levels in the soil. This demonstrates the potential of using cattle slaughterhouse effluent as an alternative water source and for the total supply of macro- and micronutrients for pasture production. The application of effluents over the years reduced the influence of Al and Mn in the soil. An increase in soil Na was observed over the years and at all three depths, mainly in the 0–10 cm layer. Compared with unfertilized natural soil (0 years), effluent treatment in the soil over time (5, 10, and 15 years) affected the reproduction of F. candida with a reduction in the number of juveniles; however, the treated soils had a higher number of juveniles than the artificial soil. To summarize, effluent use in the soil altered its chemical properties, which, in turn, affected the reproduction of the springtail F. candida. Further studies are needed to investigate whether additional treatment can improve this aspect, as well as to conduct long-term monitoring studies to assess the long-term effects of fertigation on the soil ecosystem.
Data availability
Experimental data supporting the findings of this work are available upon reasonable request.
Author contributions
Miréia Aparecida Bezerra Pereira: investigation, writing-original draft preparation; Anna Karla dos Santos Pereira: visualization, writing—review and editing; Thayrine Dias Carlos: writing-original draft preparation, formal analysis; Gilson Araújo de Freitas: software, writing-original draft preparation; Thaynara Coutinho Menezes: methodology; Vanessa Bezerra de Menezes Oliveira: methodology; Renato Almeida Sarmento: data curation; writing—review and editing; Grasiele Soares Cavallini: conceptualization, validation, methodology; Amadeu Mortágua Velho da Maia Soares: funding acquisition, project administration, resources, supervision.
Conflicts of interest
There are no conflicts to declare.
Acknowledgements
The authors would like to acknowledge financial support from Coordenação de Aperfeiçoamento de Pessoal de Nível Superior – Coordination for the Improvement of Higher Education Personnel – Brazil (CAPES), Financing Code 001 CAPES; PROPESQ and the Federal University of Tocantins; CNPq (Conselho Nacional de Desenvolvimento Científico e Tecnológico, Brazil); Tocantins Research Support Foundation – FAPT. AKSP thanks CNPq for the Grant #350015/2025-2 and project 443317/2024-0. RAS thanks CNPq Project Number 306011/2022-0. Thanks are also due for the financial support to CESAM by FCT/MCTES (UIDP/50017/2020+UIDB/50017/2020+LA/P/0094/2020), through national funds.
References
- S. Ragasri and P. C. Sabumon, J. Environ. Manage., 2023, 327, 116823, DOI:10.1016/j.jenvman.2022.116823.
- R. K. Bhunia, G. N. Menard and P. J. Eastmond, FEBS Lett., 2022, 596, 1865–1870, DOI:10.1002/1873-3468.14365.
- A. Cherdthong, Trop. Anim. Health Prod., 2020, 52, 1–6, DOI:10.1007/s11250-019-02018-6.
- J. A. Ofori and Y.-H. P. Hsieh, Crit. Rev. Food Sci. Nutr., 2014, 54, 687–697, DOI:10.1080/10408398.2011.605229.
- P. Ragályi and I. Kádár, Arch. Agron. Soil Sci., 2012, 58, S122–S126, DOI:10.1080/03650340.2012.695863.
- K. J. A. Sankar, V. N. Vasudevan, B. Sunil, A. Latha, A. Irshad, D. K. D. Mathew and S. M. Saifuddeen, Waste Biomass Valorization, 2022, 13, 599–608, DOI:10.1007/s12649-021-01507-w.
- S. Bhunia, A. Bhowmik, R. Mallick, A. Debsarcar and J. Mukherjee, Sci. Hortic., 2021, 280, 109927, DOI:10.1016/j.scienta.2021.109927.
- R. Matheyarasu, N. S. Bolan and R. Naidu, Water, Air, Soil Pollut., 2016, 227, 253, DOI:10.1007/s11270-016-2947-3.
- S. Bhunia, A. Bhowmik, R. Mallick and J. Mukherjee, Agronomy, 2021, 11, 823, DOI:10.3390/agronomy11050823.
- U. De Corato, Sci. Total Environ., 2020, 738, 139840, DOI:10.1016/j.scitotenv.2020.139840.
- S. Liu, J. Xing, D. M. Westervelt, S. Liu, D. Ding, A. M. Fiore, P. L. Kinney, Y. Zhang, M. Z. He, H. Zhang, S. K. Sahu, F. Zhang, B. Zhao and S. Wang, Sci. Total Environ., 2021, 765, 144338, DOI:10.1016/j.scitotenv.2020.144338.
- X.-W. Zhang, F.-J. Liu, J. Zhai, F.-D. Li, H.-G. Bi and X.-Z. Ai, Planta, 2020, 251, 69, DOI:10.1007/s00425-020-03362-w.
- A. Tal, Sustainability, 2018, 10, 1078, DOI:10.3390/su10041078.
- J. Urra, I. Alkorta, I. Mijangos and C. Garbisu, J. Environ. Manage., 2020, 264, 110422, DOI:10.1016/j.jenvman.2020.110422.
- P. S. Tourinho, C. A. M. van Gestel, S. Lofts, C. Svendsen, A. M. V. M. Soares and S. Loureiro, Environ. Toxicol. Chem., 2012, 31, 1679–1692, DOI:10.1002/etc.1880.
- P. F. Bellinger, K. A. Christiansen and F. Janssens, Checklist of the Collembola of the World, University of Antwerp, Belgium, 2024, available: https://www.collembola.org/ Search PubMed.
- D. Baretta, C. S. Ferreira, J. P. Sousa and E. J. B. N. Cardoso, Rev. Bras. Cienc. Solo, 2008, 32, 2693–2699, DOI:10.1590/S0100-06832008000700012.
- A. Pflug and V. Wolters, Eur. J. Soil Biol., 2001, 37, 305–308, DOI:10.1016/S1164-5563(01)01101-3.
- Y. C. Chagnon, J. H. Wilmore, I. B. Borecki, J. Gagnon, L. Pérusse, M. Chagnon, G. R. Collier, A. S. Leon, J. S. Skinner, D. C. Rao and C. Bouchard, J. Clin. Endocrinol. Metab., 2000, 85, 29–34, DOI:10.1210/jcem.85.1.6263.
- R. D. Sandifer and S. P. Hopkin, Ecotoxicol. Environ. Saf., 1997, 37, 125–130, DOI:10.1006/eesa.1997.1536.
- B. R. Winck, E. L. Saccol de Sá, V. M. Rigotti and M. Chauvat, Applied Soil Ecology, 2017, 109, 49–59, DOI:10.1016/j.apsoil.2016.09.021.
- M. T. Fountain and S. P. Hopkin, Annu. Rev. Entomol., 2005, 50, 201–222, DOI:10.1146/annurev.ento.50.071803.130331.
- E. Buch, M. Share, R. Tung, P. Benharash, P. Sharma, J. Koneru, R. Mandapati, K. A. Ellenbogen and K. Shivkumar, Heart Rhythm, 2016, 13, 636–641, DOI:10.1016/j.hrthm.2015.10.031.
- ISO, International Organization for Standardization, Soil Quality – Inhibition of Reproduction of Collembola (Folsomia candida) by Soil Pollutants, ISO 11267, ISO, Geneva, 1999 Search PubMed.
- J. Ding, D. Zhu, Q.-L. Chen, F. Zheng, H.-T. Wang and Y.-G. Zhu, Soil Biol. Biochem., 2019, 130, 141–149, DOI:10.1016/j.soilbio.2018.12.015.
- W. Koeppen, Climatología. Con un estudio de los climas de a tierra, México, 1948 Search PubMed.
- APHA, Standard Methods for the Examination of Water and Wastewater, American Public Health Association (APHA), American Water Works Association (AWWA) and Water Environment Federation (WEF), Washington, DC, USA, 22nd edn, 2012 Search PubMed.
- A. E. da Silva, A. Fontana, A. d. S. Melo, A. L. d. S. Martins, A. V. Inda, A. Santi and B. E. Madari, Manual de métodos de análise de solo, Embrapa, Brasília, DF, 3rd edn, 2017 Search PubMed.
- ABNT (Associação Brasileira de normas técnicas NRB ISO 11267), Qualidade do solo - Inibição da reprodução de Collembola (Folsomia candida) por poluentes do solo. - Determinação de efeitos sobre reprodução e sobrevivência, Rio de Janeiro, 2019 Search PubMed.
- E. B. Ferreira, P. P. Cavalcanti and D. A. Nogueira, ExpDes.pt: Pacote Experimental Designs (Portugues), 2021, 1.2.2 Search PubMed.
- F. Husson, J. Josse, S. Le and J. Mazet, 2023, 2.9, https://cran.r-project.org/web/packages/FactoMineR/index.html.
- SYSTAT, 2021, 14.5, https://systatsoftware.com/products/sigmaplot/.
- M. S. Abd-Elwahed, Ann. Agric. Sci., 2019, 64, 151–160, DOI:10.1016/j.aoas.2019.10.003.
- J. A. R. De Souza, D. A. Moreira, E. L. Silva, S. C. N. Thomazini, E. d. S. Pereira, E. Rodio, D. C. V. Rezende and N. D. Ferreira, Revista de Gestão Social e Ambiental, 2023, 17, e03208, DOI:10.24857/rgsa.v17n2-003.
- A. F. da Fonseca, E. F. Caires and G. Barth, Sci. Agric., 2010, 67, 60–70, DOI:10.1590/S0103-90162010000100009.
- H. Li, F. Santos, K. Butler and E. Herndon, Environ. Sci. Technol., 2021, 55, 12136–12152, DOI:10.1021/acs.est.1c00299.
- M. A. Alnaimy, S. A. Shahin, Z. Vranayova, M. Zelenakova and E. M. W. Abdel-Hamed, Water, 2021, 13, 2245, DOI:10.3390/w13162245.
- H. Abdi and L. J. Williams, Encyclopedia of Research Design, 2010, 3, 1–5 Search PubMed.
- S. Laurenson, N. S. Bolan, E. Smith and M. McCarthy, Aust. J. Grape Wine Res., 2012, 18, 1–10, DOI:10.1111/j.1755-0238.2011.00170.x.
- R. C. Reeve, C. A. Bower, R. H. Brooks and F. B. Gschwend, Soil Sci. Soc. Am. J., 1954, 18, 130–132, DOI:10.2136/sssaj1954.03615995001800020004x.
- A. E. Warrington, A. J. Bieber, B. Ciric, L. R. Pease, V. Van Keulen and M. Rodriguez, J. Neurosci. Res., 2007, 85, 967–976, DOI:10.1002/jnr.21217.
- J. R. V. Pils and D. A. Laird, Environ. Sci. Technol., 2007, 41, 1928–1933, DOI:10.1021/es062316y.
- X. Domene, S. Chelinho, P. Campana, T. Natal-da-Luz, J. M. Alcañiz, P. Andrés, J. Römbke and P. Sousa, Environ. Toxicol. Chem., 2011, 30, 1497–1505, DOI:10.1002/etc.533.
- Y. Zhang, Z. Li, X. Ke, L. Wu and P. Christie, Environ. Pollut., 2022, 292, 118242, DOI:10.1016/j.envpol.2021.118242.
- P. Greenslade and G. T. Vaughan, Pedobiologia, 2003, 47, 171–179, DOI:10.1078/0031-4056-00180.
- T. E. de Boer, M. Holmstrup, N. M. van Straalen and D. Roelofs, J. Insect Physiol., 2010, 56, 350–355, DOI:10.1016/j.jinsphys.2009.11.004.
- I. A. Giordani, E. Busatta, E. Bonfim, L. C. I. Oliveira, D. Baretta and C. R. D. M. Baretta, Revista Brasileira de Ciências Ambientais, 2020, 56, 1–11, DOI:10.5327/Z2176-947820200692.
- J. Filser, S. Wiegmann and B. Schröder, Applied Soil Ecology, 2014, 83, 193–199, DOI:10.1016/j.apsoil.2013.07.007.
|
This journal is © The Royal Society of Chemistry 2025 |
Click here to see how this site uses Cookies. View our privacy policy here.